- 1Center for Interdisciplinary Sustainability Research and Institute for Political Sciences, University of Münster, Münster, Germany
- 2iES Landau, RPTU Kaiserslautern-Landau, Landau, Germany
- 3Peace Academy Rhineland-Palatinate, RPTU Kaiserslautern-Landau, Landau, Germany
- 4Institute of Soil Science, Leibniz University Hannover, Hannover, Germany
- 5Center for Development Research, University of Bonn, Bonn, Germany
- 6Center for Environmental Systems Research, Kassel Institute for Sustainability, University of Kassel, Kassel, Germany
- 7Center for Earth System Research and Sustainability, University of Hamburg, Hamburg, Germany
- 8Institute for Microbiology, Leibniz University Hannover, Hannover, Germany
- 9Woodwell Climate Research Center, Falmouth, MA, United States
- 10Institute for Latin American Studies, Free University of Berlin, Berlin, Germany
- 11Plant Production Systems Group, Wageningen University and Research, Wageningen, Netherlands
- 12Department of Soil and Environment, Swedish University of Agricultural Sciences, Uppsala, Sweden
- 13Universidade Federal do Acre, Rio Branco, Brazil
- 14EcosConsult, Santa Cruz de la Sierra, Bolivia
- 15Centro de Innovación Científica Amazónica, Madre de Dios, Peru
- 16ACEAA Conservación Amazónica, La Paz, Bolivia
- 17Wyss Academy for Nature at the University of Bern, Bern, Switzerland
Humans play an interconnecting role in social-ecological systems (SES), they are part of these systems and act as agents of their destruction and regulation. This study aims to provide an analytical framework, which combines the concept of SES with the concept of tipping dynamics. As a result, we propose an analytical framework describing relevant dynamics and feedbacks within SES based on two matrixes: the “tipping matrix” and the “cross-impact matrix.” We take the Southwestern Amazon as an example for tropical regions at large and apply the proposed analytical framework to identify key underlying sub-systems within the study region: the soil ecosystem, the household livelihood system, the regional social system, and the regional climate system, which are interconnected through a network of feedbacks. We consider these sub-systems as tipping elements (TE), which when put under stress, can cross a tipping point (TP), resulting in a qualitative and potentially irreversible change of the respective TE. By systematically assessing linkages and feedbacks within and between TEs, our proposed analytical framework can provide an entry point for empirically assessing tipping point dynamics such as “tipping cascades,” which means that the crossing of a TP in one TE may force the tipping of another TE. Policy implications: The proposed joint description of the structure and dynamics within and across SES in respect to characteristics of tipping point dynamics promotes a better understanding of human-nature interactions and critical linkages within regional SES that may be used for effectively informing and directing empirical tipping point assessments, monitoring or intervention purposes. Thereby, the framework can inform policy-making for enhancing the resilience of regional SES.
1. Introduction
While the Earth System, as a major social-ecological system (SES), has sustained global human well-being throughout the Holocene, its sustaining functions are being put under stress by human activities and their consequences, such as climate change, land use, and land cover change (LUCC) and the loss of biodiversity and associated ecosystem services (IPCC, 2014; IPBES, 2019). These processes reduce “the safe and just operating space for humanity” (Rockström et al., 2009; Dearing et al., 2014) and have driven a transition into unstable environmental conditions in the Anthropocene (Steffen et al., 2018; IPCC, 2022). The scientific discourse about the “Anthropocene” asserts that investigation of the Earth System nowadays is only feasible considering human impacts (Donges et al., 2017). The integration of human interaction with the environment is conceptualized in the literature on SES, which exist at different scales and forms sub-systems of larger SES (Reyers et al., 2018). SES assume that environmental, economic, and social (including cultural and political) sub-systems are interconnected (Folke, 2006), e.g., a disturbance in a sub-system may affect others, via self-amplifying or stabilizing feedback loops that operate within or across sub-systems (Senge, 1991; Sterman, 2002; Hamilton et al., 2022). These dynamics may not be linear and lead to rapid, hardly predictable changes. One specific case of an extreme dynamic system behavior may occur after crossing a tipping point (TP), which drives a potentially irreversible system transformation from one state into another (Lenton, 2013). Approaching and eventually crossing a TP happens particularly when disturbances lead to internal self-amplifying feedback loops (Lenton et al., 2008). TPs and their impact on SES have been documented in recent studies (Lauerburg et al., 2020), but common definitions are lacking, e.g., regarding (i) the speed and pathway of a system shift and (ii) the potential (time) of system shift recovery (Milkoreit et al., 2018). Moreover, the empirical evidence for certain magnitudes of TPs is still limited (Hillebrand et al., 2020). However, whether there is a certain magnitude (threshold) or a range of magnitude (corridor) where dynamic system responses accelerate, a common understanding of the tipping behavior in SES is important for a more sustainable ecosystem-based management (Lauerburg et al., 2020).
In this regard, a joint analysis of the SES structure, dynamics, and interactions is useful and needed not only to better understand human-nature interactions and relationships but also to formulate policy recommendations at a regional scale where impacts become visible and actions are being implemented (Dearing et al., 2014).
In the present paper, we propose an analytical framework, which applies a global TP concept to a regional SES, and systematically assesses interactions and feedbacks within. The framework examines impacts across system elements that might turn out to expose the behavior of tipping dynamics.
We demonstrate the functioning of our analytical framework based on qualitative expert and literature-driven assumptions rather than novel empirical data. Our main contribution is the proposition of the framework. The illustrating examples are strongly dependent on the assumptions made but can be adapted to the situation of interest. Thus, the reader might disagree with some of the assumptions while retaining an appreciation for the value of the general framework.
The case study region is located at the tri-national border in the Southwestern Amazon, across the three states Madre de Dios (Peru), Acre (Brazil), and Pando (Bolivia), also known as the MAP region. The region comprises three different social and economic country situations but similar ecological conditions: the tropical humid rainforest, which is particularly important for the Earth System in terms of moist convection, biodiversity, and its function as a carbon sink and habitat to diverse social groups (Perz et al., 2013; Selaya et al., 2017; Callo-Concha et al., 2021).
To systematically describe the dynamics within the Southwestern Amazon, we apply and adjust the TP terminology proposed by Lenton et al. (2008) and decompose the SES into our framework of sub-systems and respective interconnected TEs: (1) the soil ecosystem, (2) the household livelihood system, (3) the regional social system, and (4) the regional climate system. We first characterize the key components and functioning of each TE before we identify linkages across them. Based on this description, we introduce the so-called tipping cascade (Lenton, 2020; Sharpe and Lenton, 2021), which describes the interdependencies between TEs, i.e., the crossing of the TP(s) of one TE may cause the tipping of another TE (and so forth). Finally, we demonstrate the cascading effect by describing one potential tipping pathway initialized by human disturbances originating from LUCC.
Our proposed analytical framework can be applied to any kind of regional SES, for systematically describing complex dynamic interconnections within and between individual TEs/sub-systems, independent whether a TP exists or not.
The paper is structured as follows: in section two, we provide an overview of our example, the Southwestern Amazon. In section three, we first describe the tipping point terminology for global Earth System analysis (3.1.1), apply this terminology to the regional SES and develop the “tipping matrix” (3.1.2). In a second step, we focus on key linkages and the cascading effects among the TEs of the regional SES and develop the “cross-impact matrix” (3.2), which provides an entry point for assessing “tipping cascades.” We summarize the section with some limitations and implications for future research (3.3) and summarize our findings in section four.
2. Example: the Southwestern Amazon
The MAP (Madre de Dios, Acre, Pando) region (Figure 1) is located in the Amazon Basin and is part of the Southwestern Amazon Moist Forests ecoregion (Olson et al., 2001) comprising an extension of about 31 million ha (Selaya et al., 2017). It is known for its vast areas of standing natural forests and its high biodiversity, its regulation function for regional and global climate processes (Arraut et al., 2012), and its substantial social diversity (Perz et al., 2013).
Since the 2000s, the MAP SES is undergoing rapid changes through heavy impacts of ongoing LUCC. The pavement of the Interoceanic Highway during the 2000s has triggered social, environmental, and economic processes with significant effects on increasing deforestation rates associated with road connectivity and migration (Southworth et al., 2011). Furthermore, gold mining has also significantly increased, posing major threats to biodiversity, landscape alterations, water quality, forest carbon stocks, and human health (Swenson et al., 2011; Asner and Tupayachi, 2016; Velásquez Zapata, 2020). Additionally, forest clearings through fires, agricultural activities, as well as regional climate change, such as droughts and floods, have further increased pressure on regional SES (Southworth et al., 2011; Perz et al., 2013; Da Silva et al., 2018; Schilling et al., 2021).
The climate of the Southwestern Amazon (about 10°S) features the typical characteristics of the moist tropics which are low thermal seasonality with an average annual temperature of 26–27°C and a mean annual precipitation of 1,500–3,500 mm, declining from west to east and with dryer conditions during austral winter (June–September) and more humid conditions during austral summer (November–February). However, distinct intrinsic natural, temporal variations, such as El-Niño-Southern-Oscillation (ENSO), reinforce seasonal patterns resulting in both frequent droughts and extreme precipitation events (Aragão et al., 2007; Marengo et al., 2011; Sulca et al., 2018; Souza et al., 2022).
The soils of the MAP region are derived from a wide variety of parent materials, landforms, geomorphic elements, and soil age (Quesada et al., 2011; Gómez et al., 2019). The three provinces within the MAP region share the presence of strongly weathered soils being poor in weatherable minerals and nutrients and rich in iron and aluminum oxyhydroxides, such as Acrisols, Ferralsols, and Lixisols (Wieder et al., 2014). In the region of Madre de Dios, additionally, soils of floodplains (Fluvisols) and less weathered Cambisols are present and characterized by higher nutrient availability (Batjes, 2016). According to Selaya et al. (2017), most of the region shows an elevation of 100–600 m above sea level and is considered submontane, while the predominant physiographic types are alluvial, i.e., ancient mostly not flooded or seasonally flooded floodplains (43% Madre de Dios, 6% Acre, and 19% Pando), and terra firme forests (48% Madre de Dios, 80% Acre, and 78% Pando). Furthermore, the same authors report the aboveground biomass stocks have been estimated to range between 100 and 300 Mg ha−1, harboring great diversity of tree and palm species with high ecological importance. However, with future climate scenarios indicating more frequent and extensive droughts, an increase in the use of fire for new deforestation may lead to a potential increase in bamboo density and a decrease in species diversity (Da Silva et al., 2021).
The regional social system is characterized by a high social diversity comprising indigenous peoples and traditional forest extractivists as well as recent migrants including settlers, ranchers, and miners, along with a growing urban population (Perz et al., 2015). In addition, the region faces political and economic marginalization from the political centers of the three states due to their remote location and low political will, resulting in a lack of law enforcement and thriving organized crime (Froese et al., 2022a). At the same time, the transition between rural and urban involves complex mobility pathways and multi-sited households as well as diverse economic and social relations. For example, it is common for a family to live in the city while some members work in the city and others frequently travel to their agricultural plots where they produce crops and fruits for subsistence or to sell on the market. These result in much more permeable categories of the “urban” and “rural” compared to other regions in Latin America.
Consequently, the household livelihood systems of the MAP region comprise a complex combination of activities, with the most prominent being the harvest of Brazil nuts. Açai is collected in Acre and Pando, while formerly important rubber tapping is close to extinction due to a loss of marketability of forest-collected latex. Hunting and collecting of non-timber forest products (NTFP) such as sap resins, oils, and palm fruits are practiced for subsistence (Da Lima et al., 2020). Furthermore, crop cultivation, cattle ranching, and mining are important income sources, with distinct differences between the three regions. While Acre has a longer tradition of large-scale cattle ranch farming, partly due to federal cattle subsidies up to the year 1991 (Valentim et al., 2002; Duchelle, 2009), Madre de Dios and Pando are largely characterized by small-scale farming practices (mainly cassava, rice, maize, and beans). Illegal gold mining and deforestation for coca plantations as well as poaching are increasing in the whole MAP region with the main emphasis in Madre de Dios, where also legal gold mining occurs on large scale (Salisbury and Fagan, 2013; Espin and Perz, 2021). This leads to negative impacts in environmental, economic, and social contexts (Vadjunec et al., 2009; Asner et al., 2013; Duchelle et al., 2014).
3. Analytical framework
The analytical framework indicates the complexity of the tropical systems, i.e., regarding (i) interactions and feedbacks and (ii) potential pathways that “disturbances” could trigger. Additionally, we design the analytical framework to reflect upon the different contexts and histories that the sub-systems are embedded in and that shape the current state and sensitivity to disturbances of each sub-system.
The analytical framework consists of two supplementing, structured approaches. First, the development of the Tipping Matrix (see Table 1 and Section 3.1), which applies the global tipping point concept (Lenton et al., 2008) to the regional SES and its four identified sub-systems [referred to in the following as “tipping elements” (TE)]: (i) the soil ecosystem, (ii) the household livelihood system, (iii) the regional social system, and (iv) the regional climate system. The tipping matrix defines the core components and functioning of each TE (in isolation) and structures information, relevant for researching tipping dynamics. Second, the development of the Cross-Impact Matrix (Table 2), by highlighting critical linkages and feedbacks between the regions' TE. Critical linkages and feedbacks are considered to affect key processes and features of TE, initially identified in the tipping matrix (see Table 1 and the crucial system feature and control parameters—shown in bold letters in Table 2). In this regard, the analytical framework supports interdisciplinary, cross-scale research (of relevant processes within/between TE) on tipping dynamics in tropical SES. It provides an entry point for assessing potential cascading effects and dynamics in the Southwestern Amazon.
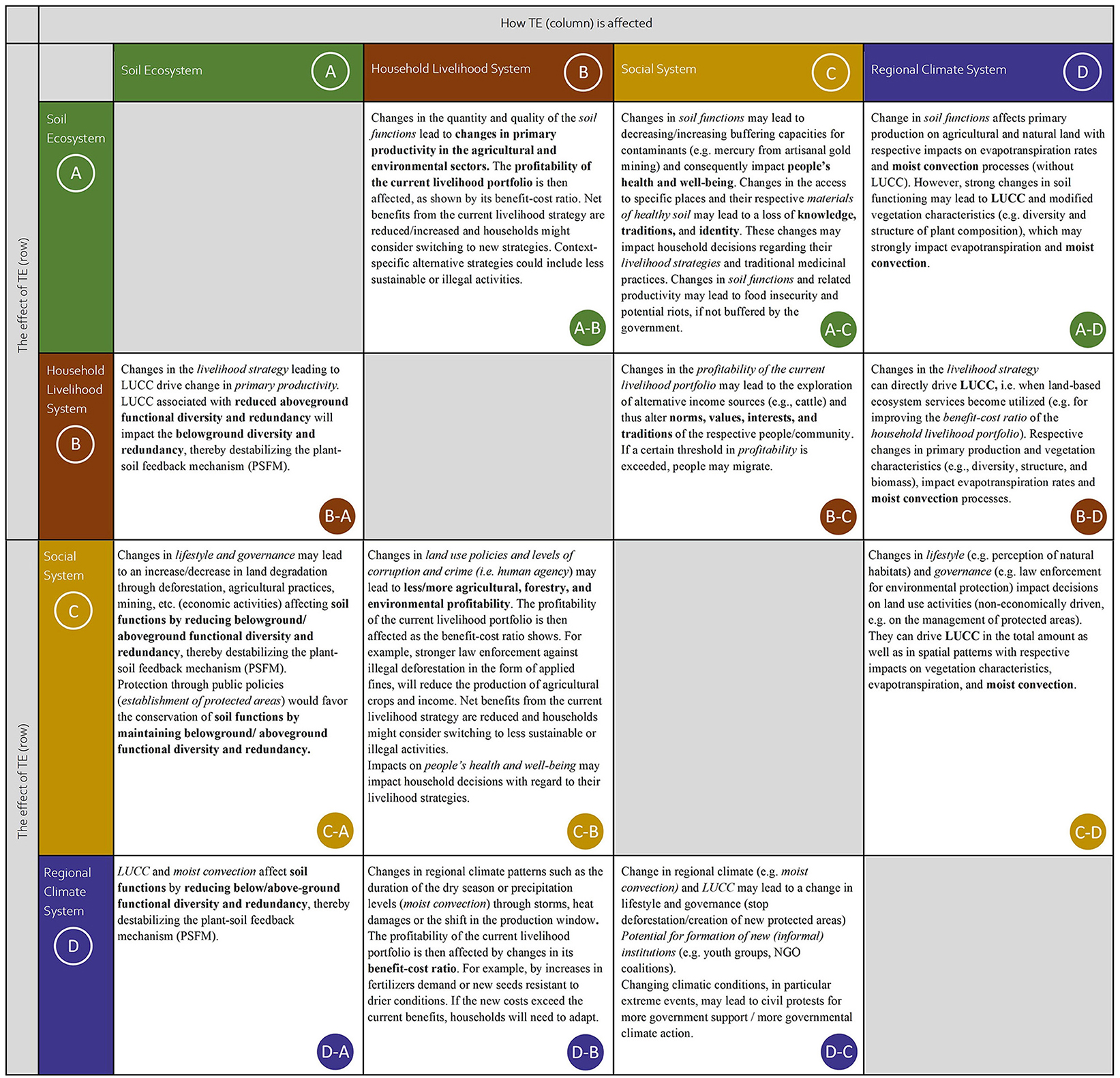
Table 2. Cross-impact matrix indicating the multiple interactions across tipping elements [bold: crucial system feature and control parameter (from Table 1), italic: linkages to other TEs].
Within our analytical framework, we use the term “link” for any interaction between TE and the term “cascade,” to refer to the dynamic of tipping that follows after an initial tipping point in one sub-system has been crossed. In the following, we introduce the tipping matrix and the cross-impact matrix that together form the analytical framework.
3.1. The tipping matrix
3.1.1. The tipping terminology
We base our tipping matrix framework on Lenton's (2011) approach to tipping dynamics which defines a TE as a large-scale sub-system of the Earth system or, simply put, a sub-system of the Earth system, in which a small perturbation can trigger a large response. The crucial system feature is the main property of the system, in other words, the core feature or combination of sub-features through which the complex system can be defined (Lenton, 2011). The parameters controlling the crucial system feature can be combined into a single control parameter, an independent variable. The control parameter includes a critical control value, defined as the value at which the control parameter reaches a point at which the crucial system feature experiences an abrupt/non-linear change after some observation time (Lenton et al., 2008). The critical control value remains difficult to predict and is currently a focus of research to identify early warning indicators to narrow down the interval in which a critical control value is anticipated (Dakos et al., 2015). Finally, driving forces are any natural or human-induced factor that causes a change in the controlling parameter and interacts across spatial, temporal, and organizational scales. Likewise any complex system, SES may respond to driving forces showing internal and external feedbacks with the surrounding environment, when the outputs of a (sub-)system are forwarded as inputs, as part of a chain of causation producing loops with stabilizing or self-enhancing effects on the (sub-)system response (Anderson, 1999; Lenton, 2013). While stabilizing feedback mechanisms buffer the response of the system to a driving force, self-enhancing feedback mechanisms act destabilizing, potentially causing the system to shift to a different state, thus facilitating the TEs to cross a TP. While we use the tipping terminology across human and natural systems, we acknowledge that these systems operate at very different scales and with different underlying mechanisms of feedback-driven non-linear abrupt changes, in particular concerning agency and networking behavior (see Steffen et al., 2018; Betsill et al., 2020; Winkelmann et al., 2022).
3.1.2. Application of the tipping terminology to the Southwestern Amazon
3.1.2.1. The regional soil ecosystem
Soils are a key component of the Critical Zone of the continental surface, which extends from the atmosphere to the bedrock, and ensures the functioning of the Earth's ecosystems and the continuation of life on Earth (Brantley et al., 2007). We assume that highly biodiverse and functional soils provide the underpinning of indispensable services that ensure the basis for sustainable economic livelihoods and societies. We choose the soils of the Southwestern Amazon region as a tipping element and the soil buffering functions (i.e., supply of water and nutrients to plants) as crucial system feature in the soil-plant-human continuum. Soil resilience to the regional driving forces is based on the interplay between belowground and aboveground functional diversity and redundancies of different structural biotic components (control parameters). Functional diversity involves three interrelated and distinct plant/microbial components: (1) variety, i.e., how many different components occur; (2) the balance of how many of each type of component there are; and (3) disparity, i.e., how different the components are from each other (Brinkman et al., 2010; Birgé et al., 2016; Barot et al., 2018). While redundancy involves the diversity of below and aboveground replication of certain functions in a system. In this regard, redundancy provides a safeguard to ensure soil's safe operation, by allowing plant/microbial components to compensate for the loss or failure of others (Yachi and Loreau, 1999; Fraccascia et al., 2018). Maintaining functional diversity and redundancy may allow soils to perform the same task in multiple ways and respond with different capabilities to various driving forces, thus enabling the persistence of its above/belowground elements and maintaining their stability under changing conditions (Wertz et al., 2006; Jurburg and Salles, 2015; Jia and Whalen, 2020).
Identifying and investigating the above and belowground feedback loops provides insights into the mechanisms by which the soil system regulates itself. While internal stabilizing feedback loops (negative feedback loops) are likely to lead to a stabilization of both above and belowground biotic communities, internal self-amplifying feedback loops (positive feedback loops) may accentuate slight inequalities that lead to the dominance or exclusion of various biotic communities, thus, partial impairment of the soil functions (Eisenhauer, 2012; Lou et al., 2014). This identification of relevant feedback mechanisms maintaining or destabilizing soil TE relies on scientific evidence accrued since the 1990s studying the plant-soil feedback mechanisms (PSFM) (Bennett and Klironomos, 2019). Studies of the interaction mechanisms between the physical, chemical, biogeochemical, and biological components of the soil system and the distinct plant communities have contributed to these findings (Ehrenfeld et al., 2005). The PSFMs have revealed their essential role in maintaining plant diversity, showing that primary production tends to increase in highly diverse plant communities and that the beneficial correlation between plant diversity-productivity seems to be reinforced over time (Thakur et al., 2021).
The soil physical factors that have the greatest influence on plant-soil interactions are water content, temperature, and particle structure resulting from the aggregation of mineral grains. These properties not only affect plant growth but are also conditioned by the nature of that plant growth (Ehrenfeld et al., 2005). While chemical and biogeochemical interactions between plants and soil are more complex to unravel and analyze when compared to the physical properties of soils. The reason is that plant-soil chemical and biogeochemical interactions often involve complex mechanistic pathways involving soil chemical components, soil moisture, temperature, and soil biota (Ehrenfeld et al., 2005; Mariotte et al., 2018; Wang et al., 2021). In this regard, van der Putten et al. (2016) identified the three major categories of soil biota shaping PSFMs: plant pathogens (microbial soil pathogens, herbivorous nematodes, insect larvae, and other invertebrates), symbionts (mycorrhizal fungi, non-mycorrhizal endophytic fungi, endophytic bacteria, nitrogen-fixing microbes, and plant growth promoting microorganisms), and decomposers (organisms involved in carbon and nutrient cycles that break down litter, root exudates, and soil organic matter). Each may have an impact on plant development both directly and indirectly, by influencing soil physicochemical qualities such as pH, organic matter content, water holding capacity, temperature, and soil structure. Furthermore, a better understanding of aboveground-belowground feedbacks and underlying mechanisms will assist in better predicting and mitigating the consequences of human-induced global changes, improve restoration and conservation efforts, and promote the sustainable provision of soil ecosystem services in a rapidly changing world (van der Putten et al., 2013).
We hypothesize that soils will show an enhanced resilience to regional driving forces when they hold a greater diversity and redundancy of functional traits provided by aboveground /belowground components. We expect enhanced PSFMs, such as plant-mediated nutrient cycling and plant–microbial interactions if there is a greater diversity and redundancy of plant and microbial functional traits. Reduced above and belowground functional diversity and redundancy will result in a reduction of the soil functions supporting water and nutrient supply to plants. We finalize the characterization of our soil TE by offering a suitable set of indicators used to quantify the qualitative change in aboveground/belowground diversity and redundancy derived from the critical control values (Table 1).
3.1.2.2. The regional household livelihood system
The second TE in our tipping matrix is the household livelihood system. To define it we draw on the conceptual framework developed by Niehof and Price (2001). This system is composed of several subsystems, processes, activities, inputs, and outputs, and interacts with other systems such as ecological systems, markets, and sociocultural contexts. The system includes the family, farm, and household subsystems, and the process of “householding” or “household production,” which entails the activities and inputs (resources and assets) used to satisfy the material needs of household members and generate the basis for meeting their immaterial needs. The system's throughput is determined by the processing, use, and management of these inputs. One of the main outputs of the system is livelihood security when the household can provide for its members' needs sustainably. Typically, there are numerous different activities targeted at securing and improving livelihood. A household that gathers Brazil nuts, for instance, might also grow corn for their consumption and to feed their chickens, as well as produce grains for the market (Duchelle et al., 2014). Such activities are planned and structured based on strategies, which together with the decision-making (e.g., land use decisions) and management of strategies' implementation are part of the system's throughput.
In our matrix, we consider this strategy, or more specifically, the livelihood strategy, as the crucial system feature. The parameters controlling the livelihood strategy comprise (1) livelihood assets (human capital, natural capital, financial capital, social capital, and physical capital), (2) structures and processes that affect livelihoods assets (e.g., market, policies, institutions, migration), and (3) livelihood outcomes themselves, that is, through a loop (e.g., more/less income, reduced/increased vulnerability, more/less sustainable use of land, improved/deteriorated income security) (DFID, 1999). In the tipping matrix, we focus on the profitability of the portfolio of activities as a key control parameter of the livelihood strategy.
In our matrix, the critical control value is the one at which a particular livelihood strategy will change qualitatively, say from a legal to an illegal activity-dominated strategy, or vice-versa. For example, a livelihood strategy that includes only legal activities will be maintained as long as this strategy is profitable. Once overall costs increase and outweigh the benefits, a household could switch its portfolio to include some illegal activities (Yonariza and Webb, 2007; Vasco et al., 2017; Chaves et al., 2021). It is important to note that the costs will also comprise the risk of being fined or prosecuted for having committed crimes. The risk will depend on the governance context (Börner et al., 2015a). In the same way, benefits do not only consider tangible but immaterial ones (Huynh et al., 2022). At the extreme, a household's livelihood strategy could completely switch to be based on illegal activities when these provide significantly larger profitability than that provided by the legal ones. In theory, the trend could be reversed, from illegal activities to legal ones, if profits associated with legal activities increase again. However, as in all complex systems, other controlling parameters not exemplified here could come into play (e.g., cultural aspects, see for example Hoelle, 2021).
Several driving forces affect the profitability of livelihood strategies. Examples are agricultural technologies (Angelsen and Kaimowitz, 2001), levels of natural resource use (Coomes et al., 2004), historical conditions (Coomes et al., 2016), soil fertility (Heger et al., 2020) and the availability of other ecosystem services (Junqueira et al., 2016), environmental variability (Börner et al., 2015b; Ajefu et al., 2020; Alfani et al., 2021; Girard et al., 2021), farm/forest output level (i.e., productivity) (Klemick, 2011), market prices (e.g., the decline in Brazil nut retail prices) (Ubiali and Alexiades, 2022), and institutional constraints (e.g., land tenure insecurity, encroaching) (Tseng et al., 2021).
The livelihood strategy as the crucial system feature plays a key role in determining potential feedback mechanisms within the household livelihood system (Girard et al., 2021). Re-investments in the current livelihood strategy can be considered as an internal stabilizing feedback mechanism if households that could afford to reinvest part of their income in their current production activities could maintain their livelihood strategy (Su et al., 2019). On the other hand, internal self-amplifying feedback mechanisms could be indicated, when falling prices of agricultural products lead to expanding agriculture at the expense of forests to maintain the same level of income (Angelsen and Kaimowitz, 2001). Similarly, falling prices of forest and environmental products could lead to overharvesting and depletion of the natural resource base. The adopted changes in the livelihood strategy increase income which is then used to continue with the potentially unsustainable livelihood strategy. The depletion of the natural resource base can become an incentive for actors to rethink and change their livelihood strategy.
3.1.2.3. The regional social system
As a third tipping element in our tipping matrix, we consider the regional social system. We define the regional social system as corresponding to the boundaries of the social-ecological sub-system under consideration to embrace all dynamics relevant to LUCC within this SES. These boundaries do not necessarily correspond to political or administrative boundaries. However, the consideration of administrative boundaries in a second step adds more complexity to the system analysis by introducing sub-systems that may differ substantially in terms of institutions and applicable laws, resulting in land use patterns and vulnerabilities to external shocks, such as varying climatic conditions. At the same time, this differentiation facilitates the analysis of interactions between the different systems, through migration, flows of products, criminal networks, etc.
Land use change and related changes in soil functions and subsequent changes in livelihood strategies may impact the social fabric within the Southwestern Amazonian regional society—and vice-versa. Hence, we consider social cohesion as the crucial system feature (see also Uzzell et al., 2002; Fonseca et al., 2019). The actors/agents between which cohesion is analyzed can be social sub-groups, such as indigenous peoples (ethnic groups) and traditional populations (extractivists, quilombolas, ribeirinhos, etc.), farmers, miners, youth, and migrants, or political sub-systems such as resource governance or community leadership structures. To approach social cohesion, we orient our research along a definition by Chan et al. (2006) as “a state of affairs concerning both the vertical and the horizontal interactions among members of society as characterized by a set of attitudes and norms that includes trust, a sense of belonging and the willingness to participate and help, as well as their behavioral manifestations.” We advance this definition through its systematic threefold constitution into (1) shared identities, (2) mutual trust, and (3) perceived equalities—which have been widely discussed in the social cohesion literature (Bernard, 1999; Chan et al., 2006; Green et al., 2009; Dickes et al., 2014; Dragolov et al., 2016). These three constituents will be relevant when identifying the qualitative state and potential approach of a TP within the regional social system.
The parameters that control social cohesion are manifold and can be subdivided into parameters of different sub-systems: (1) beliefs, norms, interests, experiences (norms and value system; e.g., Mann, 1970; Holtug, 2017); (2) class, ethnicity, gender, age (social stratification system; e.g., Lockwood, 1999); (3) monetary income, subsistence activities (production system; e.g., Coburn, 2000); (4) pensions, education, insurance, housing, healthcare (social security system; e.g., Berger-Schmitt, 2002); (5) participation, political representation (political system; e.g., Aall and Crocker, 2019); (6) knowledge diffusion, cooperation (knowledge systems; e.g., Green et al., 2009; Radzvilavicius et al., 2021). In the tipping matrix, we combine these parameters into one key control parameter: the reflexive capabilities of the social system to govern change and transformation (Sen, 1985; Dryzek, 2016)—in other words, human agency (Betsill et al., 2020). The control parameter includes a critical control value, which we define as the critical reflexivity of formal and informal institutions, meaning the dynamic transformation of institutions to overcome path dependencies and to react to the ever-increasing dynamic and unstable conditions of the Anthropocene.
We will understand the tipping of the regional social system as ruptures in these functions of the social system. We hypothesize that the regional society is more resilient to driving forces, such as incoherent laws or lifestyle changes when social cohesion is generally high, i.e., more functions of the social system are fulfilled and the society holds capabilities to govern change, transformation (and even crisis). These capabilities control what we defined as determining a cohesive society: mutual trust, shared identities, and perceived equality. Human agency is crucial for designing the adaptive capacities of the social system and depends on the critical reflexivity of formal and informal institutions to govern change and transformation to ensure the maintenance or creation of functions of the social system. Formal and informal institutions and governance structures are how people and societies organize themselves and their interactions with nature at different scales. They are the underlying steering elements of change and influence all aspects of relationships between people and the environment. Their effect can be positive or negative, either in absolute terms or context-dependent. Thus, we conceptualize that a social TP is crossed after society experiences a qualitative change in the region's social fabric, e.g., through norms and value changes that reduce reflexivity and social learning within social groups or the crossing of critical reflexivity (meaning the escape of path dependencies) within formal and informal institutions. An additional layer of complexity is added through potentially catalytic drivers such as the social-economic effects of climate change and lately the COVID-19 pandemic (Froese et al., 2022b).
Within the regional social system, stabilizing and self-amplifying feedbacks are manifold and often strongly connected to the economic and environmental system. In addition, due to the volatile and highly context-specific nature of the regional social system, we include normative assumptions to determine a potentially stable state of the system and respective stabilizing feedback mechanisms that alter or stabilize this state of the social tipping element. For the creation of the human agency and the strengthening of reflexive capabilities, institutional learning (social and political) is crucial. Hence, the major self-amplifying feedback mechanism we consider in Table 1 is the interruption of institutional learning which may lead to non-adaptive management of changing environmental conditions which again implicates potential path dependencies and a lack of knowledge-holding capacities (Dryzek, 2016; Pickering, 2019). Other feedback loops, that contribute to the amplification of this major loop are manifold and only some examples can be mentioned here: (1) normalization of non-adherence and group pressure may lead to inactivity and fear (victim) or unavailability of an independent body to report crimes which again may lead to increasing impunity and less fear (perpetrator) of being reported which may again lead to increasing non-adherence (Schönenberg, 2002); (2) marginalization due to inaccessibility of public policies may lead to repeated intergenerational marginalization (spiral of poverty) (Bradshaw, 2007); (3) unemployment or reduction of income may lead to exploration of alternative income sources and shifts in lifestyles and related norms and values (Hoelle, 2017); (4) nepotism and the co-optation of power structures may lead to one-sided representation, or even state capture that may support further nepotism and increasing unequal access to resources (Damonte, 2016); (5) frustration with current public system may lead to poor voting participation and consequent poor representation of interests which may lead to further frustration; (6) shifting control over democratic spaces may affect the agency of community organizations and further shift the control over democratic spaces (Carretero, 2008).
3.1.2.4. The regional climate system
The fourth tipping element, that we consider in our tipping matrix, is the regional climate system. It is highly dynamic with strong self-amplifying feedback mechanisms (Staal et al., 2020). The climate is a principal driver of environmental processes affecting the soil ecosystem TE, the household livelihood TE, and the regional society TE. In turn, particularly the regional climate is a process response system affected by regional environmental and societal changes such as agricultural expansion and intensification or infrastructure development (settlements, roads), which drive deforestation and fragmentation of natural ecosystems (LUCC). Regional LUCC drives regional spatial variations in thermally driven turbulent mixing, deep convection, and related cloud and precipitation formation (Rieck et al., 2014). Detailed systematic analyses of the influence of surface features and processes on the local hydrological cycle still suffer from lacking observational data (Böhner et al., 2020). However, the tight coupling between surface heterogeneities (i.e., human-made surfaces), moist convection, and related atmospheric circulations is confirmed by simulations explicitly resolving local scale surface processes and moist convection (Rieck, 2015). In this regard, we argue that diverse (i.e., species and structure-rich) evergreen rainforest land cover types and their high primary production and evapotranspiration rates contribute greater to water recycling, i.e., cloud and precipitation formation than less diverse land cover types such as cropland, pasture or urban areas with their lower evapotranspiration rates.
In our matrix, we assume moist convection to be an integral crucial system feature of the regional climate system, not only controlled by the extent of forest cover but likewise by the pattern and size of the forest and non-forested patches (control parameter). In accordance with our tipping matrix, the ratio and shape of forested and non-forested areas is considered as critical control value. We identify LUCC as driving force that impact our control parameter. Given that LUCC and especially deforestation alter the surface energy and moisture fluxes this will have significant consequences for the diurnal circle of moist convection (D'Almeida et al., 2007). Ongoing LUCC and respective forest cover loss beyond the critical control value result in reduced moist convection (i.e., reduced convective rain rates), prolonged dry spells and increased droughts. It ultimately provokes internal positive self-amplifying feedback mechanism due to an increase in the Bowen Ratio (i.e., the ratio of sensible heat to latent heat transport from the ground to the atmosphere), further reducing moist convection. This feedback may cause a decline in primary production as well as a response e.g., in the composition, structure and diversity of forest systems, which again lead to less evapotranspiration, cloud and precipitation formation (Leite-Filho et al., 2021). The indicator used to quantify qualitative change is the quantity and patchiness of forest cover and LUCC activities.
3.2. Cross-impact matrix
To analytically describe the multiple interactions between our four TE, we use a systematic approach (Table 2) that illustrates cross-linkages. A depiction of the Table 2 is shown in Figure 2.
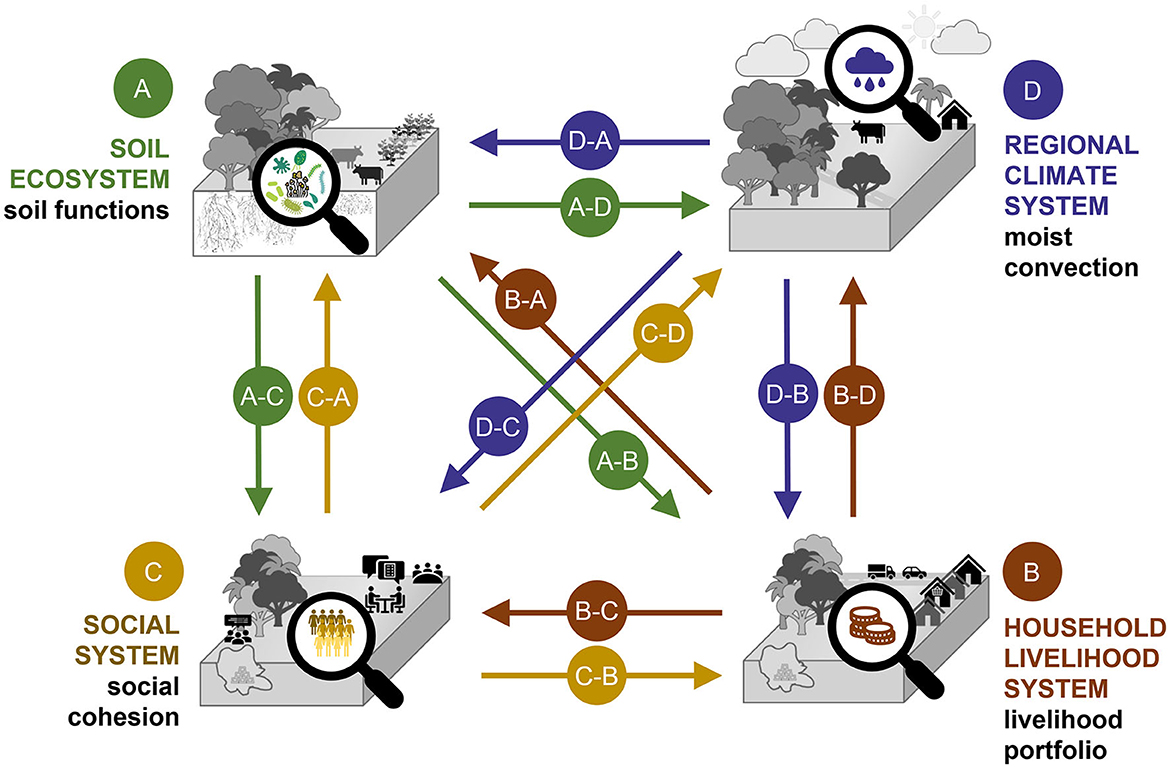
Figure 2. Depiction of the interconnections in the Southwestern Amazon, including selected tipping elements, respective crucial system features and cross-linkages (arrows).
Table 2 can be read in two directions: (1) row-wise, where each row describes how changes in the TE (row) influence each key TE functioning (column), and (2) column-wise, where each column indicates how the functioning of the TE (column) is influenced by changes in each TE (row).
To illustrate the functioning within and across sub-systems, we select one (among many) potential tipping pathways in the Southwestern Amazon. In the following, we illustrate what a potential tipping cascade might look like. For simplicity we assume a two-phase like cascade dynamic emerging from linear unidirectional couplings of pairs of TE (i.e., from A to B, from B to C, and from C to D, see Figure 2). That is, only after one TE tips, will the second TE tip (Klose et al., 2021). However, other dynamics, such as the domino cascade and the joint cascade could occur (Klose et al., 2021). Moreover, we recognize that multiple tipping cascade dynamics could simultaneously emerge from linear and non-linear bidirectional coupling of pairs of the TE.
As depicted in Table 1, we consider land use and land cover change as the starting driving force affecting the soil ecosystem TE (A) and triggering the tipping cascade. In particular, we assume such change as an outcome of the livelihood strategies of local households (e.g., forest conversion for agricultural expansion and increasing cattle herd size, logging, roads, and mining). Thus, such strategies lead to conversion of forests in order for the household to provide livelihood. Consequently, land use and land cover change may result in a reduction in functional diversity and redundancy above and belowground leading to PSFM destabilization and reduction in primary production. This can be aggravated when erosion comes into play, which may lead to the creation of bare soil elements in the landscape through certain land uses causing the TE soil to tip. A tipping soil system affects in turn the livelihood system (B) because the levels of production are constrained due to a lower primary productivity. Specifically, the reduced levels of production imply less income for the household, forcing it to adjust its livelihood strategy. Households can no longer reinvest in the same livelihood strategy and expand then their agricultural lands further in order to compensate for the reduced level of income in a feedback loop mechanism: less primary productivity leads to more forest clearing, which leads to less primary productivity and less income, which again leads to more forest clearing. At some point along this path, forest conversion will exceed the legal limits set by environmental laws. That is, households would have to make the decision to deforest more than allowed by the local laws and risk the chance of being caught and sanctioned. If the risk is taken, the livelihood strategy of the household changes to one that could no longer be considered legal and the household livelihood system (B) has also tipped. What follows is the alteration of norms, values, interests and traditions of the households and communities affected. The outcome could be seen as the stable income from cattle raising or monocultural crops confirming people's decision of lifestyle change. Also, such economic transformations lead to out- and in-migration, processes that further change the social system by changing lifestyle, culture and governance. In this sense the social system (C) has also tipped. At the aggregated level, that is, at the social TE, such changes impact household's land use decisions back, amplifying the pattern of land use change (e.g., deforestation). Total increases as well as the spatial configuration of forest cover loss affect vegetation characteristics, evapotranspiration and moist convection, causing the tipping of the regional climate system (D). Reduced moist convection transforms the evergreen tropical rainforest into another (natural) vegetation type showing less diversity and/or structure causing further reductions in evapotranspiration and moist convection respectively. It further reduces precipitation and primary production on agricultural land.
As such, Table 2 provides an entry point for analysis on cascading tipping processes in the region's SES. We apply the term tipping cascade (Klose et al., 2021) to describe the interdependencies between the TE, i.e., that the stable state in one TE favors stability in the other TE. In contrast, the tipping of one TE may destabilize the crucial system feature of another TE and trigger its tipping once a threshold of the respective critical control values has passed. We assume that the direction and linkages in the tipping cascade are neither static nor linear. Instead, the tipping cascade may follow different multidimensional pathways, depending on the history and initial state of the TEs and the source(s) and strength(s) of the initial disturbance [driving force(s)] that causes stress to the TEs. Following this argumentation, the tipping of one TE may finally lead to the tipping of the entire regional's SES.
3.3. Application, limitations, and outlook
Our analytical framework qualitatively describes relevant variables, features and interconnections within a regional SES in a common language applied across disciplinary boundaries. In this respect, it can guide monitoring programs or supplementing interdisciplinary empiric analysis on concrete causal-consequences and respective quantitative relationships. The framework itself does not provide empirical data and the potential tipping cascade is described as a hypothetical cascade. This means, that the interactions and pathways we describe are only some out of many more interconnections to be described. Hence, the framework can only inform decision-makers about the complexity of the system while specific policy recommendations cannot be derived from the analytical framework. However, a supplementing empirical analysis along the analytical framework would allow concrete decision making in the light of tipping cascades. Hence, the provided framework can provide further information to the indication of early warning indicators (Lenton, 2011). While the framework is limited in its complete depiction of reality, we believe that the simplification we take is necessary to cover the various different sub-systems in one framework. This means that we had to sacrifice detailed description of internal processes within each TE in order to focus on the interconnections between the TEs. Nevertheless, the analytical framework bears the potential to be adaptable and transferable to other regional tropical SES and is therefore particularly relevant for interdisciplinary research approaches.
4. Conclusion
This study provides an analytical framework to identify entry points to potential tipping cascades, via (1) the application of a global tipping points (TP) concept to a regional tropical SES (tipping matrix), and (2) the systematic assessment of interactions and feedbacks within it (cross-impact matrix). In this regard, the analytical framework supports interdisciplinary, cross scale research (of relevant processes within/between sub-systems) on tipping dynamics. Along the example of the Southwestern Amazon we have identified the key underlying sub-systems (TE) and their interlinkages: the soil ecosystem, the household livelihood system, the regional social system and the regional climate system. The example from the Southwestern Amazon shows the analytical potential of our proposed framework and advances the understanding of the regional SES by identifying core processes and functioning of each TE. The identified cross-linkages between the TEs are considered to be a first step to the identification of potential tipping cascades. For a tipping cascade to be prevalent, the crossing of a TP in one TE leads to critical changes in another TE. Such dynamics are increasingly covered by the literature, both in life-sustaining and detrimental directions (Lenton, 2020; Klose et al., 2021; Franzke et al., 2022). The joint analysis of interactions and potential cascading TPs as proposed through the analytical framework, promotes a better understanding of human-nature interactions and critical linkages that may serve to indicate early warning indicators for cascading tipping dynamics. Additionally, the analytical framework can be applied for monitoring or intervention purposes or information for policy makers to better understand interconnections that are relevant to promote resilient SES.
Data availability statement
The raw data supporting the conclusions of this article will be made available by the authors, without undue reservation.
Author contributions
RF, AA, RG, and BS contributed equally to the conceptualization, further elaboration of the initial idea of the manuscript and which was conceived by HJ, JBo, JBö, OF, GG, MH, RScha, JS, and RSchö. RF led the writing process and the organization and communication among all co-authors. AA, RG, BS, SK, JBö, DB, JBo, FB, DC-C, ED, DF, OF, GG, MH, SH, MJ, CJ, EL, KM, CP, RScha, SR, JS, FS, RSchö, GS, CV, VV, MV, and HJ contributed on the writing and editing and literature curation on every version of the manuscript. All authors contributed critically to the drafts and gave final approval for publication.
Funding
This research was funded by the German Ministry for Education and Research (BMBF) under Grant Number 01LC1824A to 01LC1824F, project PRODIGY. MJ was supported by the Swiss National Science Foundation under Grant Number P400PB_191055/1.
Acknowledgments
We would like to thank two external reviewers for their helpful comments in improving earlier versions of the manuscript. We thank Gabriel Frey for preparing Figure 1. We also particularly thank the people in the MAP region for their hospitality and openness during the field research.
Conflict of interest
GS was employed by EcosConsult.
The remaining authors declare that the research was conducted in the absence of any commercial or financial relationships that could be construed as a potential conflict of interest.
Publisher's note
All claims expressed in this article are solely those of the authors and do not necessarily represent those of their affiliated organizations, or those of the publisher, the editors and the reviewers. Any product that may be evaluated in this article, or claim that may be made by its manufacturer, is not guaranteed or endorsed by the publisher.
References
Aall, P., and Crocker, C. A. (2019). Building resilience and social cohesion in conflict. Glob. Policy 10, 68–75. doi: 10.1111/1758-5899.12681
Ajefu, J. B., Efobi, U., and Beecroft, I. (2020). Coping with negative shocks and the role of the farm input subsidy programme in rural Malawi. Environ. Dev. Econ. 26, 1–21. doi: 10.1017/S1355770X20000285
Alfani, F., Arslan, A., McCarthy, N., Cavatassi, R., and Sitko, N. (2021). Climate resilience in rural Zambia: evaluating farmers' response to el niño-induced drought. Environ. Dev. Econ. 26, 582–604. doi: 10.1017/S1355770X21000097
Anderson, P. (1999). Perspective: complexity theory and organization science. Organ. Sci. 10, 216–232. doi: 10.1287/orsc.10.3.216
Angelsen, A., and Kaimowitz, D. (2001). Introduction: the Role of Agricultural Technologies in Tropical Deforestation. Wallingford, Oxon: CABI Publishing. Available online at https://www.cifor.org/library/832/introduction-the-role-of-agricultural-technologies-in-tropical-deforestation/ (accessed October 26, 2017).
Aragão, L. E. O. C., Malhi, Y., Roman-Cuesta, R. M., Saatchi, S., Anderson, L. O., Shimabukuro, Y. E., et al. (2007). Spatial patterns and fire response of recent amazonian droughts. Geophys. Res. Lett. 34, L07701. doi: 10.1029/2006GL028946
Arraut, J. M., Nobre, C., Barbosa, H. M. J., Obregon, G., and Marengo, J. (2012). Aerial rivers and lakes: looking at large-scale moisture transport and its relation to amazonia and to subtropical rainfall in South America. J. Climate 25, 543–556. doi: 10.1175/2011JCLI4189.1
Asner, G. P., Llactayo, W., Tupayachi, R., and Luna, E. R. (2013). Elevated rates of gold mining in the amazon revealed through high-resolution monitoring. Proc. Natl. Acad. Sci. U.S.A. 110, 18454–18459. doi: 10.1073/pnas.1318271110
Asner, G. P., and Tupayachi, R. (2016). Accelerated losses of protected forests from gold mining in the Peruvian Amazon. Environ. Res. Lett. 12, 94004. doi: 10.1088/1748-9326/aa7dab
Barot, S., Blouin, M., and Lemanceau, P. (2018). “Actions and feedback: consequences for soil management,” in Soils as a Key Component of the Critical Zone 6, eds P. Lemanceau, and M. Blouin (Hoboken, NJ: John Wiley and Sons, Inc), 163–171. doi: 10.1002/9781119438274.ch9
Batjes, N. H. (2016). Harmonized soil property values for broad-scale modelling (WISE30sec) with estimates of global soil carbon stocks. Geoderma 269, 61–68. doi: 10.1016/j.geoderma.2016.01.034
Bennett, J. A., and Klironomos, J. (2019). Mechanisms of plant–soil feedback: interactions among biotic and abiotic drivers. New Phytol. 222, 91–96.
Berger-Schmitt, R. (2002). Considering social cohesion in quality of life assessments: concept and measurement. Soc. Indic. Res. 58, 403–428. doi: 10.1023/A:1015752320935
Bernard, P. (1999). Social Cohesion: A Dialectical Critique of a Quasi-Concept: Canadian Policy Research Networks. Ottawa: Department of Canadian Heritage.
Betsill, M. M., Benney, T. M., and Gerlak, A. K. (eds), (2020). Agency in Earth System Governance. Cambridge: Cambridge University Press. doi: 10.1017/9781108688277
Birgé, H. E., Bevans, R. A., Allen, C. R., Angeler, D. G., Baer, S. G., Wall, D. H., et al. (2016). Adaptive management for soil ecosystem services. J. Environ. Manage. 183(Pt 2), 371–378. doi: 10.1016/j.jenvman.2016.06.024
Böhner, J., Hasson, S. U., and Kilian, M. (2020). Evaluation of spatial variation characteristics of dynamically modelled precipitation and temperature fields – a comparative analysis of wrf simulations over Western Amazonia and the Central Himalayas. GeoÖko 41, 41–66.
Börner, J., Marinho, E., and Wunder, S. (2015a). Mixing carrots and sticks to conserve forests in the Brazilian Amazon: a spatial probabilistic modeling approach. PLoS ONE 10, e0116846. doi: 10.1371/journal.pone.0116846
Börner, J., Shively, G., Wunder, S., and Wyman, M. (2015b). How do rural households cope with economic shocks? Insights from global data using hierarchical analysis. J. Agric. Econ. 66, 392–414. doi: 10.1111/1477-9552.12097
Bradshaw, T. K. (2007). Theories of poverty and anti-poverty programs in community development. Commun. Dev. 38, 7–25. doi: 10.1080/15575330709490182
Brantley, S. L., Goldhaber, M. B., and Ragnarsdottir, K. V. (2007). Crossing disciplines and scales to understand the critical zone. Elements 3, 307–314. doi: 10.2113/gselements.3.5.307
Brinkman, P. E., van der Putten, W. H., Bakker, E.-J., and Verhoeven, K. J. F. (2010). Plant-soil feedback: experimental approaches, statistical analyses and ecological interpretations. J. Agrarian Change 98, 1063–1073. doi: 10.1111/j.1365-2745.2010.01695.x
Callo-Concha, D., Lagneaux, E., Zenteno, R., and Frör, O. (2021). Assessing Householder Social-Ecological Resilience in Transboundary Conditions: The Case of the MAP Region in Southwestern Amazonia. ZEF Working Paper Series. Center for Development Research, University of Bonn. Bonn, Germany.
Carretero, J. M. M. (2008). Participación de la Sociedad Civil y Cohesión Social: Fundación Internacional y para Iberoamérica de Administración y Políticas. Madrid: Fundación Internacional y para Iberoamérica de Administración y Políticas Públicas (FIIAPP).
Chan, J., To, H.-P., and Chan, E. (2006). Reconsidering social cohesion: developing a definition and analytical framework for empirical research. Soc. Indic. Res. 75, 273–302. doi: 10.1007/s11205-005-2118-1
Chaves, W. A., Valle, D., Tavares, A. S., Mühlen, E. M., and Wilcove, D. S. (2021). Investigating illegal activities that affect biodiversity: the case of wildlife consumption in the Brazilian Amazon. Ecol. Appl. 31, e02402. doi: 10.1002/eap.2402
Coburn, D. (2000). Income inequality, social cohesion and the health status of populations: the role of neo-liberalism. Soc. Sci. Med. 51, 135–146. doi: 10.1016/S0277-9536(99)00445-1
Coomes, O. T., Barham, B. L., and Takasaki, Y. (2004). Targeting conservation–development initiatives in tropical forests: insights from analyses of rain forest use and economic reliance among Amazonian peasants. Ecol. Econ. 51, 47–64. doi: 10.1016/j.ecolecon.2004.04.004
Coomes, O. T., Takasaki, Y., Abizaid, C., and Arroyo-Mora, J. P. (2016). Environmental and market determinants of economic orientation among rain forest communities: evidence from a large-scale survey in Western Amazonia. Ecol. Econ. 129, 260–271. doi: 10.1016/j.ecolecon.2016.06.001
Da Lima, N. S., Napiwoski, S. J., and Oliveira, M. A. (2020). Human-wildlife conflict in the Southwestern Amazon: poaching and its motivations. Nat. Conserv. Res. 5, 109–114. doi: 10.24189/ncr.2020.006
Da Silva, S. S., Fearnside, P. M., Graça, P. M. L. A, Brown, I. F., Alencar, A., Melo, A. W. F., et al. (2018). Dynamics of forest fires in the Southwestern Amazon. For. Ecol. Manage. 424, 312–322. doi: 10.1016/j.foreco.2018.04.041
Da Silva, S. S., Fearnside, P. M., Graça, P. M. L. A., Numata, I., Melo, A. W. F., Ferreira, E. L., et al. (2021). Increasing bamboo dominance in Southwestern Amazon forests following intensification of drought-mediated fires. For. Ecol. Manage. 490, 119139. doi: 10.1016/j.foreco.2021.119139
Dakos, V., Carpenter, S. R., van Nes, E. H., and Scheffer, M. (2015). Resilience indicators: prospects and limitations for early warnings of regime shifts. Philos. Trans. R. Soc. Lond. B Biol. Sci. 370, 1–10. doi: 10.1098/rstb.2013.0263
D'Almeida, C., Vörösmarty, C. J., Hurtt, G. C., Marengo, J. A., Dingman, S. L., Keim, B. D., et al. (2007). The effects of deforestation on the hydrological cycle in amazonia: a review on scale and resolution. Int. J. Climatol. 27, 633–647. doi: 10.1002/joc.1475
Damonte, G. H. (2016). The “blind” state: government quest for formalization and conflict with small-scale miners in the Peruvian Amazon. Antipode 48, 956–976. doi: 10.1111/anti.12230
Dearing, J. A., Wang, R., Zhang, K., Dyke, J. G., Haberl, H., Hossain, M. S., et al. (2014). Safe and just operating spaces for regional social-ecological systems. Glob. Environ. Change 28, 227–238. doi: 10.1016/j.gloenvcha.2014.06.012
DFID (1999). Sustainable Livelihoods Guidance Sheets: Department for International Development. Available online at http://www.ennonline.net/dfidsustainableliving (accessed June 2, 2020).
Dickes, P., Borsenberger, M., and Fleury, C. (2014). “Measures of social cohesion,” in Encyclopedia of Quality of Life and Well-being Research, ed A. C. Michalos (Dordrecht: Springer), 3922–3926. doi: 10.1007/978-94-007-0753-5_3725
Donges, J. F., Winkelmann, R., Lucht, W., Cornell, S. E., Dyke, J. G., Rockström, J., et al. (2017). Closing the loop: reconnecting human dynamics to earth system science. Anthr. Rev. 4, 151–157. doi: 10.1177/2053019617725537
Dragolov, G., Ignácz, Z. S., Lorenz, J., Delhey, J., Boehnke, K., Unzicker, K., et al. (2016). “Theoretical framework of the social cohesion radar,” in Social Cohesion in the Western World, eds Dragolov, Z. S. Ignácz, and Lorenz (Cham: Springer), 1–13. doi: 10.1007/978-3-319-32464-7_1
Dryzek, J. S. (2016). Institutions for the anthropocene: governance in a changing earth system. Br. J. Polit. Sci. 46, 937–956. doi: 10.1017/S0007123414000453
Duchelle, A. E. (2009). Conservation and Livelihood Development in Brazil Nut-producing Communities in a Tri-national Amazonian Frontier. Available online at https://search.proquest.com/openview/9570dceff548b8dbc5b34a9f5fa5bea7/1?pq-origsite=gscholarandcbl=18750 (accessed October 6, 2022).
Duchelle, A. E., Almeyda Zambrano, A. M., Wunder, S., Börner, J., and Kainer, K. A. (2014). Smallholder specialization strategies along the forest transition curve in Southwestern Amazonia. World Dev. 64, S149–S158. doi: 10.1016/j.worlddev.2014.03.001
Ehrenfeld, J. G., Ravit, B., and Elgersma, K. (2005). Feedback in the plant-soil system. Annu. Rev. Environ. Resour. 30, 75–115. doi: 10.1146/annurev.energy.30.050504.144212
Eisenhauer, N. (2012). Aboveground-belowground interactions as a source of complementarity effects in biodiversity experiments. Plant Soil 351, 1–22. doi: 10.1007/s11104-011-1027-0
Espin, J., and Perz, S. (2021). Environmental crimes in extractive activities: explanations for low enforcement effectiveness in the case of illegal gold mining in Madre de Dios, Peru. Extr. Ind. Soc. 8, 331–339. doi: 10.1016/j.exis.2020.12.009
Folke, C. (2006). Resilience: the emergence of a perspective for social–ecological systems analyses. Glob. Environ. Change 16, 253–267. doi: 10.1016/j.gloenvcha.2006.04.002
Fonseca, X., Lukosch, S., and Brazier, F. (2019). Social cohesion revisited: a new definition and how to characterize it. Innovation 32, 231–253. doi: 10.1080/13511610.2018.1497480
Fraccascia, L., Giannoccaro, I., and Albino, V. (2018). Resilience of complex systems: state of the art and directions for future research. Complexity 2018, 1–44. doi: 10.1155/2018/3421529
Franzke, C. L. E., Ciullo, A., Gilmore, E. A., Matias, D. M., Nagabhatla, N., Orlov, A., et al. (2022). Perspectives on tipping points in integrated models of the natural and human earth system: cascading effects and telecoupling. Environ. Res. Lett. 17, 15004. doi: 10.1088/1748-9326/ac42fd
Froese, R., Pinzón, C., Aceitón, L., Argentim, T., Arteaga, M., Navas-Guzmán, J. S., et al. (2022a). Conflicts over land as a risk for social-ecological resilience: a transnational comparative analysis in the Southwestern Amazon. Sustainability 14, 6520. doi: 10.3390/su14116520
Froese, R., Schilling, J., Pinzón, C., and Schönenberg, R. (2022b). Between cooperation and conflict: a social-ecological perspective on the impacts of COVID-19 in South-western Amazonia. Die Friedens-Warte 95, 67–88. doi: 10.35998/fw-2022-0003
Girard, J., Delacote, P., and Leblois, A. (2021). Agricultural households' adaptation to weather shocks in Sub-Saharan Africa: implications for land-use change and deforestation. Environ. Dev. Econ. 26, 538–560. doi: 10.1017/S1355770X2000056X
Gómez, J., Schobbenhaus, C., and Montes Ramírez, N. E. (2019). Geological Map of South America 2019. Scale 1:5 000 000. Commission for the Geological Map of the World (CGMW). Edited by Colombian Geological Survey, and Geological Survey of Brazil. Paris. Available online at: https://www2.sgc.gov.co/MGC/Paginas/gmsa5M2019.aspx (accessed September 9, 2022).
Green, A., Janmaat, G., and Han, C. (2009). Regimes of Social Cohesion. Edited by Centre for Learning and Life Chances in Knowledge Economies and Societies. London: University of London. Available online at http://www.llakes.org/wp-content/uploads/2010/08/Z.-Regimes-of-Social-Cohesion.pdf (accessed September 29, 2022).
Hamilton, M., Salerno, J., and Fischer, A. P. (2022). Cognition of feedback loops in a fire-prone social-ecological system. Glob. Environ. Change 74, 102519. doi: 10.1016/j.gloenvcha.2022.102519
Heger, M. P., Zens, G., and Bangalore, M. (2020). Land and poverty: the role of soil fertility and vegetation quality in poverty reduction. Environ. Dev. Econ. 25, 315–333. doi: 10.1017/S1355770X20000066
Hillebrand, H., Donohue, I., Harpole, W. S., Hodapp, D., Kucera, M., Lewandowska, A. M., et al. (2020). Thresholds for ecological responses to global change do not emerge from empirical data. Nat. Ecol. Evol. 4, 1502–1509. doi: 10.1038/s41559-020-1256-9
Hoelle, J. (2017). Jungle beef: consumption, production and destruction, and the development process in the Brazilian Amazon. J. Political Ecol. 24, 743. doi: 10.2458/v24i1.20964
Hoelle, J. (2021). Rainforest Cowboys: The Rise of Ranching and Cattle Culture in Western Amazonia. University of Texas Press. Available online at https://www.degruyter.com/document/doi/10.7560/761346/html?lang=en (accessed September 27, 2022).
Holtug, N. (2017). Identity, causality and social cohesion. J. Ethn. Migr. Stud. 43, 1084–1100. doi: 10.1080/1369183X.2016.1227697
Huynh, L. T. M., Gasparatos, A., Su, J., Dam Lam, R., Grant, E. I., Fukushi, K., et al. (2022). Linking the nonmaterial dimensions of human-nature relations and human well-being through cultural ecosystem services. Sci. Adv. 8, eabn8042. doi: 10.1126/sciadv.abn8042
IPBES (2019). “Summary for policymakers of the global assessment report on biodiversity and ecosystem services of the intergovernmental science-policy platform on biodiversity and ecosystem services,” in Intergovernmnetal Platform on Biodiversity and Ecosystem Services, eds S. Díaz, J. Settele, E. S. Brondízio, H. T. Ngo, M. Guèze, and J. Agard (Bonn, Germany: Intergovernmental Platform on Biodiversity and Ecosystem Services).
IPCC (2014). “Summary for policymakers,” in Intergovernmental Panel on Climate Change, eds C. B. Field, V. R. Barros, D. J. Dokken, K. J. Mach, M. D., Mastrandrea, and T. E. Bilir (Cambridge: Cambridge University Press) (Climate Change 2014: Impacts, Adaptation, and Vulnerability. Part A: Global and Sectoral Aspects. Contribution of Working Group II to the Fifth Assessment Report of the Intergovernmental Panel on Climate Change).
IPCC (2022). “Climate change 2022: impacts, adaptation, and vulnerability. Contribution of working group II to the sixth assessment report of the intergovernmental panel on climate change,” in Intergovernmental Panel on Climate Change, eds H.-O. Pörtner, D. C. Roberts, M. Tignor, E. S. Poloczanska, K. Mintenbeck, A. Alegría, et al. (Cambridge). Available online at https://www.ipcc.ch/report/sixth-assessment-report-working-group-ii/ (accessed May 23, 2023).
Jia, Y., and Whalen, J. K. (2020). A new perspective on functional redundancy and phylogenetic niche conservatism in soil microbial communities. Pedosphere 30, 18–24. doi: 10.1016/S1002-0160(19)60826-X
Junqueira, A. B., Stomph, T. J., Clement, C. R., and Struik, P. C. (2016). Variation in soil fertility influences cycle dynamics and crop diversity in shifting cultivation systems. Agric. Ecosyst. Environ. 215, 122–132. doi: 10.1016/j.agee.2015.09.015
Jurburg, S. D., and Salles, J. F. (2015). “Functional redundancy and ecosystem function — the soil microbiota as a case study,” in Biodiversity in Ecosystems - Linking Structure and Function, eds Y.-H. Lo, J. A. Blanco, and S. Roy (Houston TX: InTech), 29–50. doi: 10.5772/58981
Klemick, H. (2011). Shifting cultivation, forest fallow, and externalities in ecosystem services: evidence from the Eastern Amazon. J. Environ. Econ. Manage. 61, 95–106. doi: 10.1016/j.jeem.2010.07.003
Klose, A. K., Wunderling, N., Winkelmann, R., and Donges, J. F. (2021). What do we mean, ‘tipping cascade'? Environ. Res. Lett. 16, 125011. doi: 10.1088/1748-9326/ac3955
Lauerburg, R. A. M., Diekmann, R., Blanz, B., Gee, K., Held, H., Kannen, A., et al. (2020). Socio-ecological vulnerability to tipping points: a review of empirical approaches and their use for marine management. Sci. Total Environ. 705, 135838. doi: 10.1016/j.scitotenv.2019.135838
Leite-Filho, A. T., Soares-Filho, B. S., Davis, J. L., Abrahão, G. M., and Börner, J. (2021). Deforestation reduces rainfall and agricultural revenues in the Brazilian Amazon. Nat. Commun. 12, 2591. doi: 10.1038/s41467-021-22840-7
Lenton, T. M. (2011). Early warning of climate tipping points. Nat. Clim. Change 1, 201–209. doi: 10.1038/nclimate1143
Lenton, T. M. (2013). Environmental tipping points. Annu. Rev. Environ. Resour. 38, 1–29. doi: 10.1146/annurev-environ-102511-084654
Lenton, T. M. (2020). Tipping positive change. Philos. Trans. R. Soc. Lond. B Biol. Sci. 375, 20190123. doi: 10.1098/rstb.2019.0123
Lenton, T. M., Held, H., Kriegler, E., Hall, J. W., Lucht, W., Rahmstorf, S., et al. (2008). Tipping elements in the earth's climate system. Proc. Natl. Acad. Sci. U.S.A. 105, 1786–1793. doi: 10.1073/pnas.0705414105
Lockwood, D. (1999). “Civic integration and social cohesion,” in Capitalism and Social Cohesion, eds I. Gough, and G. Olofsson (Cham: Springer), 63–84. doi: 10.1057/9780230379138_4
Lou, Y., Clay, S. A., Davis, A. S., Dille, A., Felix, J., Ramirez, A. H. M., et al. (2014). An affinity-effect relationship for microbial communities in plant-soil feedback loops. Microb. Ecol. 67, 866–876. doi: 10.1007/s00248-013-0349-2
Mann, M. (1970). The social cohesion of liberal democracy. Am. Sociol. Rev. 35, 423. doi: 10.2307/2092986
Marengo, J. A., Tomasella, J., Alves, L. M., Soares, W. R., and Rodriguez, D. A. (2011). The drought of 2010 in the context of historical droughts in the Amazon region. Geophys. Res. Lett. 38, 1–5. doi: 10.1029/2011GL047436
Mariotte, P., Mehrabi, Z., Bezemer, T. M., De Deyn, G. B., Kulmatiski, A., Drigo, B., et al. (2018). Plant–soil feedback: Bridging natural and agricultural sciences. Trends Ecol. Evol. 33, 129–142. doi: 10.1016/j.tree.2017.11.005
Milkoreit, M., Hodbod, J., Baggio, J., Benessaiah, K., Calderón-Contreras, R., Donges, J. F., et al. (2018). Defining tipping points for social-ecological systems scholarship — an interdisciplinary literature review. Environ. Res. Lett. 13, 33005. doi: 10.1088/1748-9326/aaaa75
Niehof, A., and Price, L. (2001). Rural Livelihood Systems: A Conceptual Framework. Wageningen: UPWARD.
Olson, D. M., Dinerstein, E., Wikramanayake, E. D., Burgess, N. D., Powell, G. V. N., Underwood, E. C., et al. (2001). Terrestrial ecoregions of the world: a new map of life on earth. Bioscience 51, 933. doi: 10.1641/0006-3568(2001)051[0933:TEOTWA]2.0.CO;2
Perz, S., Chavez, A. B., Cossio, R., Hoelle, J., Leite, F. L., Rocha, K., et al. (2015). Trans-boundary infrastructure, access connectivity, and household land use in a tri-national frontier in the Southwestern Amazon. J. Land Use Sci. 10, 342–368. doi: 10.1080/1747423X.2014.898104
Perz, S., Shenkin, A., Rondon, X., and Qiu, Y. (2013). Infrastructure upgrades and rural–urban connectivity: distance disparities in a tri-national Frontier in the Amazon. Prof. Geogr. 65, 103–115. doi: 10.1080/00330124.2011.639636
Pickering, J. (2019). Ecological reflexivity: characterising an elusive virtue for governance in the anthropocene. Env. Polit. 28, 1145–1166. doi: 10.1080/09644016.2018.1487148
Quesada, C. A., Lloyd, J., Anderson, L. O., Fyllas, N. M., Schwarz, M., Czimczik, C. I., et al. (2011). Soils of Amazonia with particular reference to the RAINFOR sites. Biogeosciences 8, 1415–1440. doi: 10.5194/bg-8-1415-2011
Radzvilavicius, A. L., Kessinger, T. A., and Plotkin, J. B. (2021). Adherence to public institutions that foster cooperation. Nat. Commun. 12, 3567. doi: 10.1038/s41467-021-23783-9
Reyers, B., Folke, C., Moore, M.-L., Biggs, R., and Galaz, V. (2018). Social-ecological systems insights for navigating the dynamics of the anthropocene. Annu. Rev. Environ. Resour. 43, 267–289. doi: 10.1146/annurev-environ-110615-085349
Rieck, M. (2015). The Role of Heterogeneities and Land-atmosphere Interactions in the Development of Moist Convection. Hamburg: Universität Hamburg.
Rieck, M., Hohenegger, C., and van Heerwaarden, C. C. (2014). The influence of land surface heterogeneities on cloud size development. Mon. Weather Rev. 142, 3830–3846. doi: 10.1175/MWR-D-13-00354.1
Rockström, J., Steffen, W., Noone, K., Persson, A., Chapin, F. S., Lambin, E. F., et al. (2009). A safe operating space for humanity. Nature 461, 472–475. doi: 10.1038/461472a
Salisbury, D. S., and Fagan, C. (2013). Coca and conservation: cultivation, eradication, and trafficking in the Amazon Borderlands. Geo J. 78, 41–60. doi: 10.1007/s10708-011-9430-x
Schilling, J., Schilling-Vacaflor, A., Flemmer, R., and Froese, R. (2021). A political ecology perspective on resource extraction and human security in Kenya, Bolivia and Peru. Extr. Ind. Soc. 8, 100826. doi: 10.1016/j.exis.2020.10.009
Schönenberg, R. (2002). Kriminalisierung Gesellschaftlicher Transformationsprozesse. HSFK-Report 09/2002. Frankfurt, Germany: Peace Research Institute Frankfurt, JSTOR. Available online at https://www.jstor.org/stable/resrep14598.1 (accessed October 6, 2022).
Selaya, N. G., Zuidema, P. A., Baraloto, C., Vos, V. A., Brienen, R. J. W., Pitman, N., et al. (2017). Economically important species dominate aboveground carbon storage in forests of Southwestern Amazonia. Ecol. Soc. 22, 40. doi: 10.5751/ES-09297-220240
Sen, A. (1985). Commodities and Capabilities. Amsterdam: Elsevier Science Publishing Company (Professor Doctor P. Hennipman Lectures in Economics Theory, Institutions, Policy, 7).
Senge, P. M. (1991). The fifth discipline, the art and practice of the learning organization. Nonprofit Manag. Leadersh. 30, 37. doi: 10.1002/pfi.4170300510
Sharpe, S., and Lenton, T. M. (2021). Upward-scaling tipping cascades to meet climate goals – plausible grounds for hope. EconPapers 21, 421–433. doi: 10.1080/14693062.2020.1870097
Southworth, J., Marsik, M., Qiu, Y., Perz, S., Cumming, G., Stevens, F., et al. (2011). Roads as drivers of change: trajectories across the tri-national frontier in MAP, the Southwestern Amazon. Remote Sens. 3, 1047–1066. doi: 10.3390/rs3051047
Souza, R. D. A., Moura, V., Paloschi, R. A., Aguiar, R. G., Webler, A. D., Borma, L. D. S., et al. (2022). Assessing drought response in the Southwestern Amazon forest by remote sensing and in situ measurements. Remote Sens. 14, 1733. doi: 10.3390/rs14071733
Staal, A., Flores, B. M., Aguiar, A. P. D., Bosmans, J. H. C., Fetzer, I., Tuinenburg, O. A., et al. (2020). Feedback between drought and deforestation in the Amazon. Environ. Res. Lett. 15, 44024. doi: 10.1088/1748-9326/ab738e
Steffen, W., Rockström, J., Richardson, K., Lenton, T. M., Folke, C., Liverman, D., et al. (2018). Trajectories of the earth system in the anthropocene. Proc. Natl. Acad. Sci. U. S. A. 115, 8252–8259. doi: 10.1073/pnas.1810141115
Sterman, J. (2002). System Dynamics: Systems Thinking and Modeling for a Complex World: Massachusetts Institute of Technology. Engineering Systems Division (ESD Working Papers). Available online at: http://hdl.handle.net/1721.1/102741 (accessed October 6, 2022).
Su, Z., Aaron, J. R., Guan, Y., and Wang, H. (2019). Sustainable livelihood capital and strategy in rural tourism households: a seasonality perspective. Sustainability 11, 4833. doi: 10.3390/su11184833
Sulca, J., Takahashi, K., Espinoza, J.-C., Vuille, M., and Lavado-Casimiro, W. (2018). Impacts of different ENSO flavors and tropical pacific convection variability (ITCZ, SPCZ) on austral summer rainfall in South America, with a focus on Peru. Int. J. Climatol. 38, 420–435. doi: 10.1002/joc.5185
Swenson, J. J., Carter, C. E., Domec, J.-C., and Delgado, C. I. (2011). Gold mining in the Peruvian Amazon: global prices, deforestation, and mercury imports. PLoS ONE 6, e0018875. doi: 10.1371/journal.pone.0018875
Thakur, M. P., van der Putten, W. H., Wilschut, R. A., Veen, G. F. (Ciska)., Kardol, P., van Ruijven, J., et al. (2021). Plant–soil feedbacks and temporal dynamics of plant diversity–productivity relationships. Trends Ecol. Evol. 36, 651–661. doi: 10.1016/j.tree.2021.03.011
Tseng, T.-W. J., Robinson, B. E., Bellemare, M. F., BenYishay, A., Blackman, A., Boucher, T., et al. (2021). Influence of land tenure interventions on human well-being and environmental outcomes. Nat. Sustain. 4, 242–251. doi: 10.1038/s41893-020-00648-5
Ubiali, B., and Alexiades, M. (2022). Forests, fields, and pastures: unequal access to brazil nuts and livelihood strategies in an extractive reserve, Brazilian Amazon. Land 11, 967. doi: 10.3390/land11070967
Uzzell, D., Pol, E., and Badenas, D. (2002). Place identification, social cohesion, and enviornmental sustainability. Environ. Behav. 34, 26–53. doi: 10.1177/0013916502034001003
Vadjunec, J. M., Gomes, C. V. A., and Ludewigs, T. (2009). Land-use/land-cover change among rubber tappers in the chico mendes extractive reserve, Acre, Brazil. J. Land Use Sci. 4, 249–274. doi: 10.1080/17474230903222499
Valentim, J. F., Sa, C. P., Gomes, F. C. R., and Santos, J. C. (2002). Tenências da Pecuária Bovina no Acre entre 1970 e 2000. Edited by Embrapa Acre. Rio Branco, Brazil. Available online at https://www.infoteca.cnptia.embrapa.br/infoteca/handle/doc/492648 (accessed October 6, 2022).
van der Putten, W. H., Bardgett, R. D., Bever, J. D., Bezemer, T. M., Casper, B. B., Fukami, T., et al. (2013). Plant–soil feedbacks: the past, the present and future challenges. J. Ecol. 101, 265–276. doi: 10.1111/1365-2745.12054
van der Putten, W. H., Bradford, M. A., Brinkman, E. P., Voorde, T. F. J. v. d., Veen, G. F., van der Putten, W. H., et al. (2016). Where, when and how plant–soil feedback matters in a changing world. Funct. Ecol. 30, 1109–1121. doi: 10.1111/1365-2435.12657
Vasco, C., Torres, B., Pacheco, P., and Griess, V. (2017). The socioeconomic determinants of legal and illegal smallholder logging: evidence from the Ecuadorian Amazon. For. Policy Econ. 78, 133–140. doi: 10.1016/j.forpol.2017.01.015
Velásquez Zapata, G. Y. (2020). Problemas medioambientales de la minería aurífera ilegal en Madre de Dios (Perú). Obs. Medioambient. 23, 229–241. doi: 10.5209/obmd.73177
Wang, G., Bei, S., Li, J., Bao, X., Zhang, J. J., Schultz, P. A., et al. (2021). Soil microbial legacy drives crop diversity advantage: Linking ecological plant–soil feedback with agricultural intercropping. J. Appl. Ecol. 58, 496–506. doi: 10.1111/1365-2664.13802
Wertz, S., Degrange, V., Prosser, J. I., Poly, F., Commeaux, C., Freitag, T., et al. (2006). Maintenance of soil functioning following erosion of microbial diversity. Environ. Microbiol. 8, 2162–2169. doi: 10.1111/j.1462-2920.2006.01098.x
Wieder, W. R., Boehnert, J., Bonan, G. B., and Langseth, M. (2014). Regridded Harmonized World Soil Database v1, 2. Oak Ridge, TN: Oak Ridge ORNL DAAC.
Winkelmann, R., Donges, J. F., Smith, E. K., Milkoreit, M., Eder, C., Heitzig, J., et al. (2022). Social tipping processes towards climate action: a conceptual framework. Ecol. Econ. 192, 107242. doi: 10.1016/j.ecolecon.2021.107242
Yachi, S., and Loreau, M. (1999). Biodiversity and ecosystem productivity in a fluctuating environment: the insurance hypothesis. Proc. Natl. Acad. Sci. U.S.A. 96, 1463–1468. doi: 10.1073/pnas.96.4.1463
Keywords: cross-impact matrix, feedbacks, livelihood strategy, moist convection, social cohesion, soil functional diversity, Southwestern Amazon, tipping matrix
Citation: Froese R, Andrino A, Giudice R, Stuch B, Kilian Salas S, Böhner J, Boy D, Boy J, Brown F, Díaz García E, Figueroa D, Frör O, Guggenberger G, Horn MA, Hasson Su, Jung C, Lagneaux EG, Meurer KHE, Pinzón Cuellar C, Schaldach R, Ribeiro SC, Schilling J, Schmidt FA, Schönenberg R, Selaya G, Vega CM, Vetter VMS, Villavicenio M, Callo-Concha D, Jansen M and Jungkunst HF (2023) Describing complex interactions of social-ecological systems for tipping point assessments: an analytical framework. Front. Clim. 5:1145942. doi: 10.3389/fclim.2023.1145942
Received: 16 January 2023; Accepted: 15 May 2023;
Published: 06 July 2023.
Edited by:
Pia-Johanna Schweizer, Research Institute for Sustainability – Helmholtz Centre Potsdam, GermanyReviewed by:
Victor Nnamdi Dike, Chinese Academy of Sciences (CAS), ChinaRenzo Taddei, Federal University of São Paulo, Brazil
Copyright © 2023 Froese, Andrino, Giudice, Stuch, Kilian Salas, Böhner, Boy, Boy, Brown, Díaz García, Figueroa, Frör, Guggenberger, Horn, Hasson, Jung, Lagneaux, Meurer, Pinzón Cuellar, Schaldach, Ribeiro, Schilling, Schmidt, Schönenberg, Selaya, Vega, Vetter, Villavicenio, Callo-Concha, Jansen and Jungkunst. This is an open-access article distributed under the terms of the Creative Commons Attribution License (CC BY). The use, distribution or reproduction in other forums is permitted, provided the original author(s) and the copyright owner(s) are credited and that the original publication in this journal is cited, in accordance with accepted academic practice. No use, distribution or reproduction is permitted which does not comply with these terms.
*Correspondence: Alberto Andrino, YW5kcmlub0BpZmJrLnVuaS1oYW5ub3Zlci5kZQ==