- 1Department of Civil Engineering and Architecture (DICEA), Università Politecnica delle Marche, Ancona, Italy
- 2Dipartimento Infrastrutture, Territorio e Protezione Civile, Regione Marche, Ancona, Italy
Climate change is raising sea level rise and storminess effects on coastal systems, affecting the morphology of coastlines and impacting coastal communities and ecosystems. It is essential to gain information at an adequate scale to identify effective adaptation measures. This is of major importance in areas combining high vulnerability to climate change with high socio-economic development, like the Northern Adriatic coastal area. To this aim, in this work two different approaches have been applied to investigate inland penetration of sea water along the Marche Region: (a) a simple “bathtub” method applied to the entire Marche coastline, to highlight areas likely prone to intense inundation; (b) a more accurate numerical model applied to two test sites, to gain detailed knowledge of inundation perimeters. Both approaches have been applied with forcing conditions provided by the Intergovernmental Panel on Climate Change and the Copernicus Climate Change Service through the RCP8.5 emission scenario projected to 2070. Results showed that a 100-year return period sea storm would cause the inundation of beaches and infrastructures located along the coast, as well as affecting harbor facilities and urban areas. Information obtained with the model has been integrated in the Regional Plan for Adaptation to climate change to define specific adaptation measures.
1 Introduction
One of the most important effects of climate change is sea level rise. Several studies (e.g., Church and White, 2006; Nerem et al., 2018; Tomasicchio et al., 2018) evidence the connection between climate change and mean sea level rise acceleration in the last decades. Climate change is also affecting sea level extremes: storm surge extremes are increasing in different parts of the world (Woth et al., 2006; Hallegatte et al., 2011; Androulidakis et al., 2015). Although the effect of climate change on storm wave height is still debated and it is strongly affected by local scale characteristics, recent studies Amarouche and Akpinar (2021) suggest that some increase is to be expected. A review on this topic is found in De Leo et al. (2023). Moreover, increase in temperatures expected for the end of twenty first century and the consequent changes in atmospheric storminess have the potential to cause the height of storm surge to change (Lowe and Gregory, 2005). Sea level affects coastal systems in terms of both inundation and erosion. Erosion of sandy beaches involves a redistribution of sand from the beach face to offshore. Inundation is a different physical process and concerns the migration landward of the high-water line in proportion to the slope of the coastal area (Zhang et al., 2004).
In addition to climate change, there are several other factors, usually related to the so-called “anthropic pressure,” causing a threat to coastal systems. Examples of processes affecting coastal systems in this regard are urbanization, coastal engineering, as well as the general land and river management causing the alteration of sediment supply regime.
The Mediterranean region is identified as a hot spot for future climate change (IPCC, 2021), especially in terms of extreme events (Paxian et al., 2015). Inside the Mediterranean area, the semi-enclosed Adriatic basin is affected by climate change consequences: several studies have assessed the vulnerability of Adriatic coasts to erosion and sea level rise (see, i.e., Rizzi et al., 2017; Bonaldo et al., 2019).
The Italian coast of the Northern Adriatic Sea has gently sloping sandy beaches, the most vulnerable to the sea level rise effects (e.g., Perini et al., 2017; Gallina et al., 2019; Noon et al., 2021). In addition, it is characterized by a strong socio-economic development. This requires increasing the effort in understanding the potential effect of climate change in order to identify adaptation measures to put in place.
In the last years, planning processes have gained importance in identifying risks and defining measures. The Integrated Coastal Zone Management (ICZM) and Maritime Spatial Planning (MSP) use an integrated approach that verifies the political choices in line with physical phenomena concerning coastal and marine space. Nevertheless, it is only with climate adaptation planning and strategies that climate change processes are really taken into account in policy choices.
To identify and put in place adaptation measures it is necessary to have an appropriate knowledge of the phenomena and their evolution. One of the most striking difficulties when dealing with coastal morphological vulnerability, especially in a climate change perspective, is to downscale the information to scale adequate to the planning.
The Marche Region (Italy, see Figure 1) already adopted its ICZM Plan1 in 2019. According to the Floods Directive 2007/60/EC, it defined inundation perimeters referred to different return times (20, 100 and 300 years). The effect of climate change was considered in the plan by simply increasing by 10% the extreme water level used to compute such perimeters. In 2022, the Marche Region started developing its Regional Plan for Adaptation to climate change, in which the climatic scenario and the impacts and risks linked to climate change in several sectors have been studied by researchers to provide the basis for the definition of specific adaptation measures. Among the analyzed sectors, the coastal system has a strategic importance for the Marche Region, being it a considerable source of raw materials and wealth, as well as a host for a large amount of population, transport networks, industries and tourism. One of the most severe consequences of climate change on the coastal system is the loss of beaches due to both sea level rise and extreme sea levels.
In this work, we have investigated inland penetration of sea water along the Marche Region, under the SSP5–8.5 (also called RCP8.5) emission scenario projected to 2070, using two different approaches. In the first approach, a simple “bathtub” method, where all the contributions to the extreme sea surface elevation were sum together, in a highly conservative fashion, was used for the entire Marche Region to highlight areas likely prone to intense inundation. In the second approach, two numerical models that more realistically reproduced some physical phenomena dealing with wave energy dissipation, were applied to two test sites to obtain more reliable inundation perimeters.
The aim of the study is 2-fold: (1) to identify the maximum extent of the flooded areas under future RCP8.5 inundation scenarios and (2) to provide guidance on the adaptation measures to be taken to limit the impact of such inundation.
A comprehensive description of the two approaches is given in Section 2, whereas results are presented in Section 3. Section 4 contains a discussion on adaptation strategies and Section 5 provides the final conclusions.
2 Materials and methods
The inland penetration of sea water along the Marche Region was evaluated for the period 2040–2070 under the SSP5–8.5 scenario, characterized by very high greenhouse gases emissions and CO2 emissions that roughly double from current levels by 2050 (IPCC, 2021). Although other scenarios are available, SSP5–8.5 allows one to consider the most pessimistic case in which no additional climate policy is undertaken and, thus, the climatic variations and their impacts are the most apparent. Moreover, for the near-to-medium term future, the differences between various scenarios and associated temperature/sea level evolution are still small but increase in the second half of the century when the effects of mitigation policies become decisive.
The analysis was carried out using two different values for the variables of interest, i.e., the storm surge and wave characteristics (significant wave height and peak period): the 90th percentile and the 100-year return period value (RP100). The former represents a condition that has a 10% probability of being exceeded in the future scenario; the latter can be indicative of how the engineering design will necessarily have to change due to climate change. In fact, coastal defense structures, widely diffused along the Adriatic Italian coast and probably becoming one of the primary adaptation measures, are typically designed using, as forcing, a 100-year return period projected wave.
The inundation extent depends on the sea surface elevation, which, in turn, is determined by the combination of meteorological and wave forcing, and on the slope of beach and land. Therefore, we computed the extreme sea surface elevation considering several contributions, namely Sea Level Rise (SLR), astronomical tide, storm surge and wave run-up. Then, we first combined such extreme sea level with the DTM of the Marche Region (see Section 2.1) and, subsequently, run more precise numerical models to better take into account physical processes (see Section 2.2). Simulations were performed for two sites, Senigallia and Pesaro (Figure 1). These cities develop along the final stretch of the Misa River and the Foglia River, respectively, and are characterized by the presence of harbor facilities adjacent to the river mouths. Such configuration makes them highly vulnerable not only to the direct effect of marine superelevation and extreme storms, but also to the combined action of sea and river. Senigallia was chosen because the Misa River mouth has been monitored for years by the MORSE system2; this has allowed to integrate observations and numerical modeling to study the hydro-morphodynamics of the site with greater accuracy (Baldoni et al., 2021, 2022). Pesaro was hit by coastal inundation in the past, with water levels reaching +3.2 m above mean sea level in 2015 and the flooding interesting also buildings and streets. Furthermore, the ICZM Plan gives a very severe inundation perimeter for Pesaro. Finally, both Senigallia and Pesaro represent popular tourist destinations for the Marche Region.
2.1 “Bathtub” approach
The first method used to rapidly highlight areas likely prone to intense inundation along the Marche Region consisted in a simple “bathtub” model, where the extreme sea surface elevation (η) was computed as a sum of multiple contributions (see, for example, Almar et al., 2021): namely, the SLR (ηSLR), astronomical tide (ηT), storm surge (ηSS) and wave run-up (ηRU).
SLR projections for the Adriatic area, relative to the period 1995–2014, were obtained from the Nasa Sea Level Projection Tool,3 based on the IPCC Sixth Assessment Report (IPCC, 2021). The SLR in the Middle-Northern Adriatic in 2070 is expected to be 0.36 m (median value), due to the superposition of the sterodynamic sea level (0.19 m), melting glaciers (0.08 m), Greenland (0.03 m) and Antarctic ice sheets (0.05 m), land water storage (0.01 m) and subsidence (-0.01 m).
Data of astronomical tide and storm surge, projected to 2041–2070, were obtained from the “Water level change indicators for the European coast from 1977 to 2100 derived from climate projections” dataset, provided by the Copernicus Climate Change Service (Yan et al., 2020). The indicators are computed by the Deltares Global Tide and Surge Model (GTSM) version 3.0 used together with regional climate forcing and sea level rise initial conditions. The regional climate forcing employed is the HIRHAM5 model (Christensen, 1998) from the Danish Meteorological Institute (DMI), a member of the EURO-CORDEX climate model ensemble, which is downscaled from the global climate model EC-EARTH. By using a regional climate model and a high-resolution forcing field, GTSM is able to produce a more consistent and high-quality dataset. The horizontal resolution of the dataset in coastal areas is 0.1°. Data were downloaded from the grid points located along the Marche coastline, as shown in Figure 2A. We considered the highest astronomic tide levels of the future period, that are, on average for the Marche Region, around 0.37 m. Both the 90th percentile and the 100-year return period value (shown in Figure 2A) were selected for the storm surge, resulting in a mean value for the Marche Region of about 0.16 m and 1.21 m, respectively. The wave run-up was computed using the simplified Stockdon formulation (Stockdon et al., 2006):
where H0 and are the wave height and the wavelength in deep waters and T is the wave period. Wave data (both 90th percentile and 100-year return period value) were obtained from the “Ocean surface wave indicators for the European coast from 1976 to 2100 derived from climate projections” dataset of the Copernicus Climate Change Service (Caires and Yan, 2020). The ocean surface wave field is computed using the ECMWF's Wave Model (Stand Alone WAM, SAW), again forced by surface wind from HIRHAM5 regional climate model downscaled from the global climate model EC-EARTH. The wave climate is defined by means of wave spectral parameters such as the significant wave height and the peak wave period, given along the 20 m bathymetric contour with a resolution of 30 km. Wave data were downloaded from the grid points located along the 20 m bathymetric contour of the Marche Region, as shown in Figure 2B. These waves were transferred at the maximum depth in front of the Marche coast, which is indicatively 75 m, using a de-shoaling procedure, assuming as approaching wave directions in deep waters 20°N and 110°N, respectively for Bora and Scirocco events. These two wave regimes were chosen since they are the prevailing ones for the Marche Region (Benetazzo et al., 2012; Bonaldo et al., 2017). Depending on the coast orientation, we chose the wave regime that maximizes the run-up contribution. Since the stretch of coast to the north of the Conero headland is oriented around 127°N (Figure 1), the Scirocco wind triggers the highest run-up levels. On the other hand, the stretch to the south of the Conero headland faces about 159°N, thus the run-up levels are maximized by the Bora storms. The Stockdon formulation tends to overestimate the run-up, giving averaged values for the Marche Region of around 0.87 m and 2.03 m, respectively for the 90th percentile and the 100-year return period. This is because in such formulation the run-up only depends on wave characteristics in deep waters, while beach configuration, defense structures and wave energy dissipation processes are not taken into account.
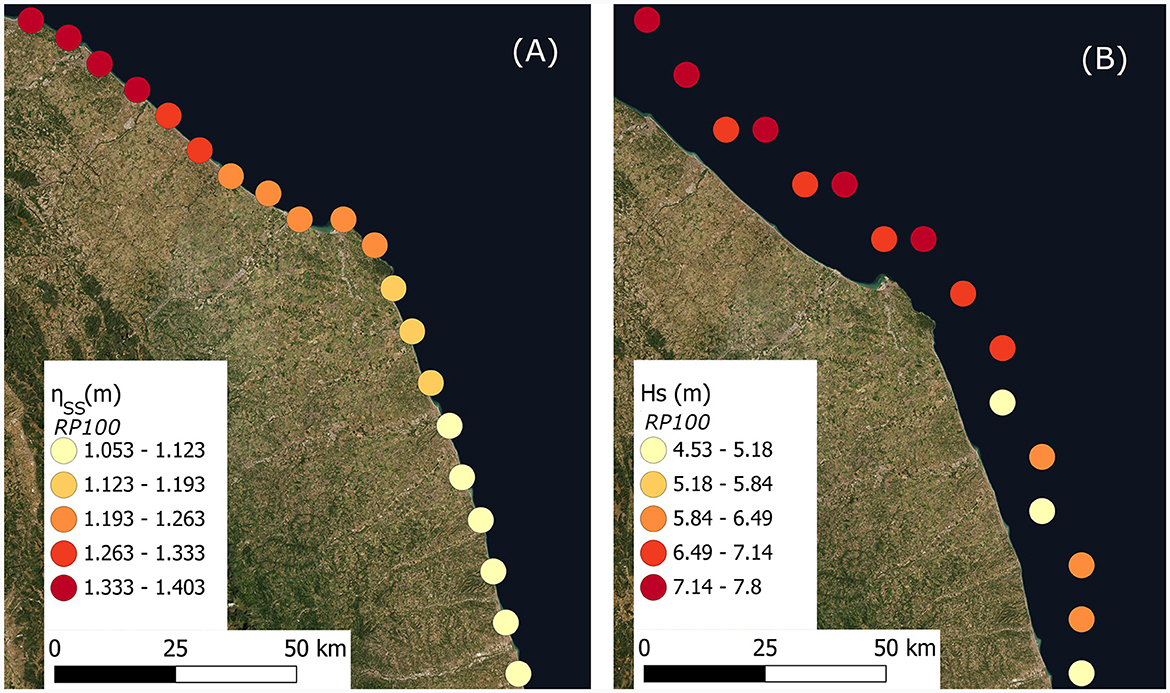
Figure 2. 100-year return period values of storm surge (A) and significant wave height (B) for the RCP8.5 scenario downloaded from the Copernicus grid points located along the Marche coastline.
Finally, the extreme sea surface elevation was computed as the sum of all the above-described contributions (Table 1). The mean value for the Marche Region was 1.76 m for the 90th percentile and 3.97 m for the 100-year return period. Two horizontal plans corresponding to such elevations were combined with the digital terrain model (DTM) of the Marche Region and the flooded areas were simply identified as those in which the terrain elevation is smaller than the sea surface elevation. The DTM was built using two different products, namely a high resolution (0.5 m) Lidar reaching around 500 m from the shoreline and a DTM covering the entire region with a lower resolution (20 m).
2.2 Numerical modeling
The main contribution to the extreme sea surface elevation, as computed in Section 2.1, were the storm surge and the run-up, with the run-up almost representing 50% of the total sea elevation. However, the wave run-up was computed using an empirical formulation (Stockdon et al., 2006) and therefore was characterized by a large uncertainty. To improve the definition of inundation perimeters, a numerical analysis was performed for two test sites: Senigallia and Pesaro. The following sections describe the numerical solvers used for such detailed procedure, which are capable of modeling the wave propagation toward the shoreline, including dissipation processes such as wave breaking and, consequently, provided more realistic inundation maps.
2.2.1 Numerical solvers
The first numerical suite used for the simulations is Delft3D (Version 4.04.01), developed by Deltares (2022a,b) and widely used and validated to model hydrodynamics, sediment transport, morphology, wave generation and propagation, water quality and bio-ecological processes in rivers, estuaries and coastal settings. Delft3D consists of several independent modules that can interact with one another. In this work, we used the FLOW and the WAVE modules. The FLOW module is the heart of Delft3D that calculates non-steady flow and transport phenomena resulting from tidal and meteorological forcing on a rectilinear or a curvilinear, boundary fitted grid. It solves the Navier Stokes equations for an incompressible fluid under the shallow water and the Boussinesq assumptions (for further details the reader is referred to the manual). The system of equations, consisting of the horizontal equations of motion, the continuity equation, and the transport equations for conservative constituents, combined with a turbulence closure model and with a set of initial and boundary conditions, is solved on a finite difference grid. The WAVE module of Delft3D computes wave propagation, wave generation by wind, non-linear wave-wave interactions and dissipation, for a given bottom topography, wind field, water level and current field in waters of deep, intermediate and finite depth. It uses the third-generation SWAN model (Booij et al., 1999) as wave driver, which is based on the discrete spectral action balance equation (for further detail, the reader is referred to the manual). The FLOW and WAVE modules were online coupled to have a dynamic interaction between them, so that the effect of currents on waves was taken into account and vice versa.
To corroborate the results of Delft3D, two additional simulations were performed with the hydrodynamic circulation model FUNWAVE-TVD (Shi et al., 2012). FUNWAVE-TVD solves the non-linear Boussinesq equations for modeling surface wave transformation from deep water to the swash zone, as well as wave-induced circulation inside the surf zone. Model applicability in shallow waters has been implemented and extended in recent years thanks to the possibility of representing physical processes, such as wave breaking and swash zone dynamics, through the automatic transition from a Boussinesq-type model to a shallow water model whenever the Froude number exceeds a certain threshold. While Delft3D is a wave-averaged model that gives a wave description averaged over the wave period, FUNWAVE-TVD is a wave-resolving model that computes velocities and water levels for all wave phases in the wave period. Both models have advantages and drawbacks, therefore the decision to use one or the other depends on the application. Although Delft3D allows one to simulate wave propagation over large domains and long timescales in relatively short time, it has limitations when dealing with large seabed gradients, as for example near harbors or barriers for coastal defense. On the other hand, FUNWAVE-TVD better computes the flow around obstacles at the expense of higher computational time. In this application, we used both models and compared their results.
2.2.2 Numerical set up
For the first case study of Senigallia, the FLOW domain of Delft3D covered an area of around 1.92 km2, extending about 2.4 km and 800 m, respectively in the alongshore and cros-shore directions, with a resolution of 2 m. The offshore boundary was forced with a water level-type boundary condition, while a Neumann-type boundary condition was imposed at the cross-shore boundaries. The innermost grid of the WAVE module had the same extension of the FLOW grid, with a resolution of 4 m, while the outermost grid extended ~3 km and 2.3 km, respectively in the alongshore and cross-shore directions, with a resolution of 32 m (Figure 3A; northing and easting coordinates are given in the Gauss-Boaga system of reference).
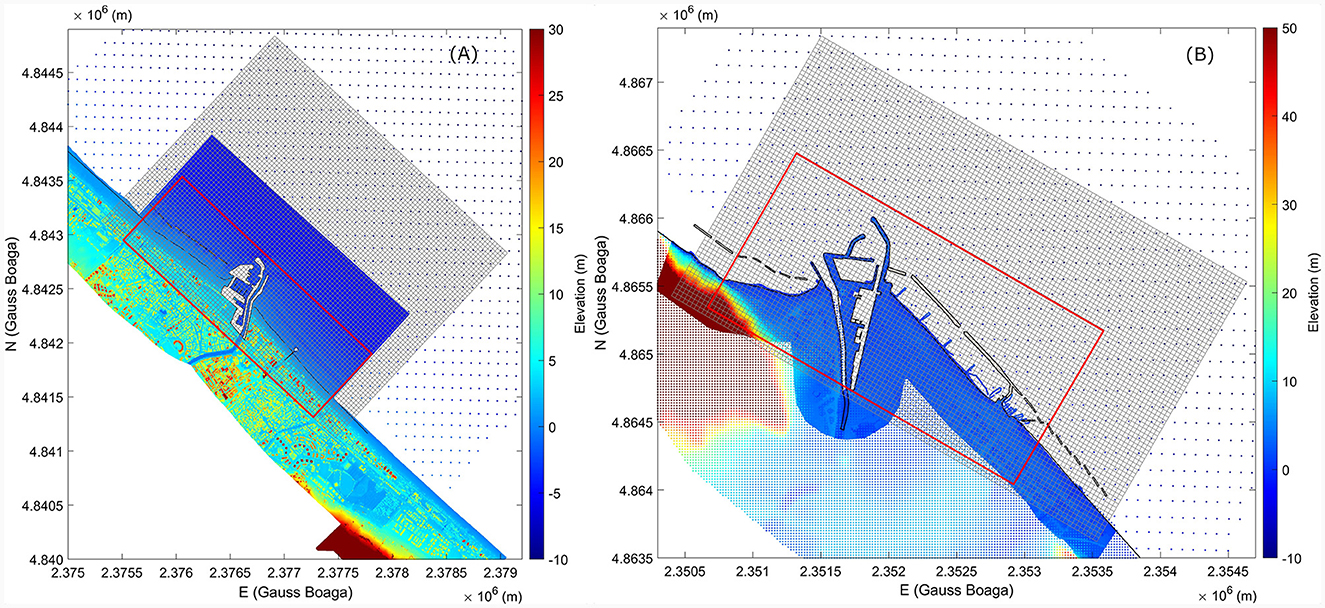
Figure 3. FLOW domain (red rectangle) and WAVE outer grid (gray grid) for Senigallia (A) and Pesaro (B). The colored dots represent the different database combined to build the bathymetry and elevation maps for the study locations.
The domain of the corresponding FUNWAVE-TVD simulation was larger, consisting in a 3 m resolution rectangular grid, that covered an area of ~5.75 km2, extending around 2.5 km in the alongshore direction and 1.7 km in the cross-shore direction. A periodic-type boundary condition was imposed at the lateral boundaries to avoid wave reflection.
The bathymetry was built using both a high-resolution survey (1 m × 1 m) of the harbor area performed by the Senigallia Municipality in 2020 and the online available EMODnet bathymetry4 (80 m × 80 m). The topography was reconstructed from the Digital Surface Model of the Misa Basin (2 m × 2 m), provided by the Italian Ministry of Ecological Transition (Figure 4A; northing and easting coordinates are given in the Gauss-Boaga system of reference).
For the second case study of Pesaro, the FLOW domain of Delft3D covered an area of around 3.39 km2, extending about 2.6 km and 1.3 km, respectively in the alongshore and cross-shore directions, with a resolution of 2 m. A water level-type boundary condition was imposed at the offshore boundary, while a Neumann-type boundary condition was applied at the cross-shore boundaries. The domain stretched more toward the south because the area to the south of the harbor is the most vulnerable to inundation, as shown in the regional ICZM Plan. The innermost WAVE grid had the same extension of the FLOW grid, with a resolution 4 m, while the outermost grid extended ~3.6 km and 2.5 km, respectively in the alongshore and cross-shore directions, with a resolution of 32 m (Figure 3B; northing and easting coordinates are given in the Gauss-Boaga system of reference).
The 3 m resolution grid of the corresponding FUNWAVE-TVD simulation covered an area or ~4.78 km2 and extended around 2.4 km in the alongshore direction and 1.3 km in the cross-shore direction. A periodic-type boundary condition was applied at the lateral boundaries to avoid wave reflection.
The bathymetry was built using both some transects surveyed by the Marche Region south of the Pesaro harbor in 2022 and the online available EMODnet bathymetry (Figure 4B; northing and easting coordinates are given in the Gauss-Boaga system of reference). To reconstruct the topography, we used the above-mentioned transects, the coastal Lidar (0.5 m × 0.5 m) and the DTM of the Marche Region (20 m × 20 m).
The main grid characteristics of the models are resumed in Table 2.
We simulated the future 100-year return period storm. Therefore, a triangular wave storm, with peak equal to the projected 100-year return period wave, was used to force the WAVE module after transferring the wave to the depth of the offshore boundary. Furthermore, the FLOW module was forced with a sinusoidal tidal timeseries having the maximum future tidal level as peak. The sum of SLR and storm surge was imposed as initial water level condition. The simulations lasted 2 days, that is the typical duration of a sea storm (Trembanis et al., 2017), and did not consider the morphological evolution of the seabed. In a conservative fashion, the peak of the storm was made to coincide with the maximum tide. The forcings were the same as for Delft3D, but the simulations lasted 1 day of model time and ended after the peak of the storm, when the maximum flooding occurred. The forcing conditions used for the simulations were resumed in Table 3.
3 Results
The extreme sea surface elevation for the Marche Region, estimated with a simple “bathtub” method for 2070, under the SSP5–8.5 emissive scenario, was 1.76 m and 3.97 m, respectively for the 90th percentile and the 100-year return period. The inundation extent, resulting from the intersection of the regional DTM with horizontal plans corresponding with such extreme elevations, showed that most sandy beaches were flooded for the 90th percentile case, while inland water penetration of the order of several hundred meters occurred for the 100-year return period case. The areas more prone to inundation were those characterized by small slopes, while locations around Monte San Bartolo (Pesaro) and Conero Headland (Ancona) were less flooded. These results provided information about the maximum flooding extent and were extremely conservative due to the approach used for their computation. When the physical processes that cause wave energy dissipation were taken into account, the inundation perimeters were significantly less severe, as described in the next two sections.
3.1 Senigallia
Figure 5 shows the inundation perimeters computed by Delft3D (cyan line) and FUNWAVE-TVD (yellow line) at the peak of the simulated 100-year return period storm. In both cases, the flooding extended up to 130 m inland north of the Senigallia harbor. The sea surface elevation at the peak of the storm was around 2.47 m, more than 1 m smaller than the extreme sea surface elevation obtained through the “bathtub” approach. The wave run-up computed by the model was about 0.50 m, while the Stockdon formulation (Equation 1) gave a run-up larger than 2 m.
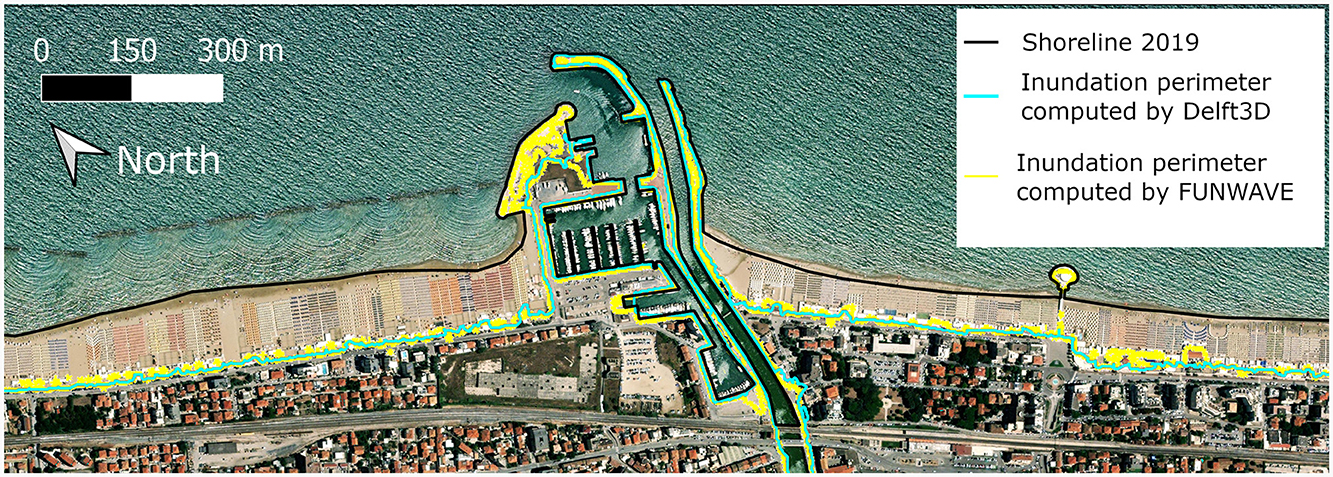
Figure 5. Inundation perimeters for Senigallia, under a 100-year return period wave storm in 2070 (SSP5–8.5). The black, cyan and yellow lines represent, respectively, the shoreline, the inundation perimeter computed by Delft3D, and the inundation perimeter computed by FUNWAVE-TVD. The year of the satellite image on the background is 2021.
The beaches to the north and south of the Senigallia harbor, and the beach resorts located along them, were flooded. The inundation perimeter reached the seafront roads (Lungomare Goffredo Mameli, Lungomare Guglielmo Marconi, Lungomare Leonardo da Vinci) and included part of the harbor facilities. The slight difference between Delft3D and FUNWAVE-TVD perimeters strengthen the results, confirming their reliability.
3.2 Pesaro
Results of the simulations for Pesaro are shown in Figure 6. The cyan and yellow lines represent the inundation perimeter computed by Delft3D and FUNWAVE-TVD, respectively. In general, the lines were in good agreement, supporting the accuracy of the results. However, the inundation extent computed by FUNWAVE was smaller than the one calculated by Delft3D in the area south of the harbor, while it was higher in the area shown to the right in Figure 6.
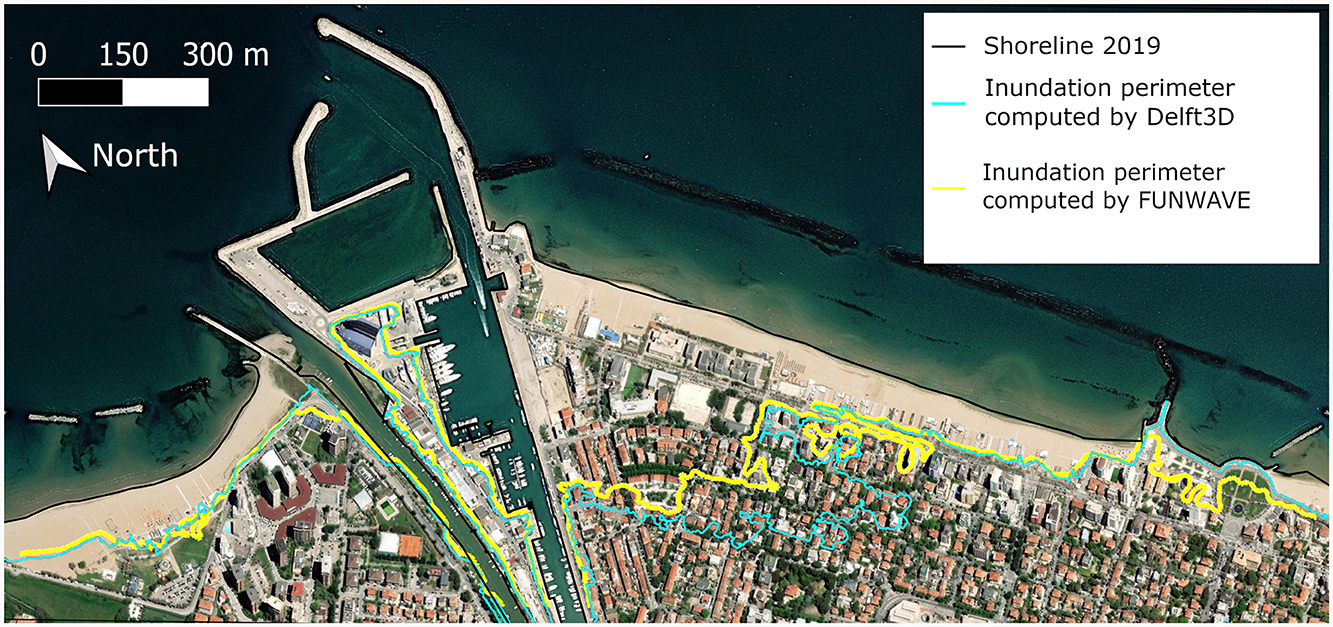
Figure 6. Inundation perimeters for Pesaro, under a 100-year return period wave storm in 2070 (SSP5–8.5). The black, cyan and yellow lines represent, respectively, the shoreline, the inundation perimeter computed by Delft3D, and the inundation perimeter computed by FUNWAVE-TVD. The year of the satellite image on the background is 2021.
The sea surface elevation at the peak of the simulated storm was around 2.64 m, more than 1 m smaller than the extreme sea surface elevation obtained with the “bathtub” method. As for Senigallia, the wave run-up was about 0.50 m, much lower than the one computed through the Stockdon formula (Equation 1).
Beaches and resorts were flooded, as well as the Levante Pier, the Commercial Port and the north Pier. The most critical situation, however, was the one that developed south of the harbor, where the flood affected the town for almost 700 m from the Levante Pier toward the southeast and about 270 m from Viale Trieste to Viale della Vittoria.
4 Discussion
4.1 Climate change impact on coastal system
Coastal areas represent the physical interface between land and water, continuously evolving and characterized by a dynamic balance. This natural balance has been altered over the years because of constant anthropic, social and economic development, which has increasingly moved from the land toward the sea. Coastal areas are of strategic importance for the development of a country as they accommodate a high percentage of citizens, constitute a significant source of raw materials and a key link for transportation and commercial activities. They also host some of the most interesting natural habitats and represent the ideal place for leisure. The entire coastal system is, however, facing increasingly serious problems. In addition to the risk of erosion due to the chronic lack of natural nourishment (solid river transport), there are also problems related to ongoing climate change, as the sea level rise and the increase of extreme meteorological events.
The impacts of climate change affect food supply, health, industry, and the integrity of transport and ecosystems and, consequently, can lead to very significant socio-economic effects. In light of these observations, assessing the impacts of climate change is now an unavoidable step to be taken in coastal zone planning. As stated by the Italian national “Guidelines for the Defense of the Coast from Erosion and the Effects of Climate Change,” “any assessment and analysis on coastal land use, including erosion phenomenon and adaptation options, should take into account the projected sea level change and climate change impacts” in order to properly decide whether and how to intervene. The “ICZM Protocol of the Mediterranean” also explicitly includes the act of “preventing and/or reducing the effects of natural hazards and in particular climate change, which may be caused by natural or human activities” among the objectives of integrated coastal zone management. The main goal to be pursued is to build coastal resilience, understood as the “inherent ability of the coast to accommodate changes induced by sea level rise, extreme events and occasional human impacts, whilst maintaining the functions fulfilled by the coastal system in the longer term” (Salman et al., 2004). This aim is to be achieved in the context of sustainable development, which can meet the needs of present generations without compromising the ability of future generations to meet their own needs, and which aims to maximize mutual benefits and minimize the risk of adverse consequences for the coast, the environment, and related human activities.
Peak increases in coastal flood hazard exposure associated with sea level rise are usually preceded by an increase in the relative amounts of beach erosion and cliff retreat (Barnard et al., 2021). Consequences of these processes on coastal zones affect natural systems as well as built or human systems. In a perspective of long-term coastal management, all the risks must be assessed. When vulnerability is caused by extreme events, then natural climate variability and anthropogenic climate change need to be considered jointly, because risks arise from the combination of the two (Füssel, 2007). This is the approach used in the ICZM (see text footnote 1) and Adaptation to Climate Change Regional Plans5 of Marche Region.
In particular, the Regional Plan for Adaptation to Climate Change, based on the inundation boundaries computed as described in the present work, has assessed the main vulnerabilities of coastal systems, following an impact chain approach (Zebisch et al., 2022). The vulnerability of coastal zones is intensified by high population density and seasonal tourism. These factors determine significant land consumption, an unsustainable exploitation of resources (e.g., water) and ecosystems, and the progressive stress on infrastructures (e.g., sewage system) which already at times appear inadequate to the changed environmental conditions. The immediate consequence of mean sea level rise coupled with the increasing frequency and intensity of storm surges is a progressive loss of emerged beach. This, together with an increase in the frequency of occurrence of extreme events, will have severe repercussions on structures and infrastructure located along the coasts, in turn negatively affecting the socio-economic sector (e.g., tourism). Moreover, the reduction of emerged beaches is also expected to cause a loss of the natural ecosystem. The interaction between rising sea levels and inland water introduces other kinds of risk, such as those on sewage systems. In case of storm surge, extreme sea level height is associated with intense rainfall: the latter overloads the run-off rainwater collection system whereas the effectiveness of sewage drainage is affected by the increased sea level, with consequent sewer backflow and flash flood in urban areas. Mean sea level rise alters the hydrodynamic equilibrium between groundwater and salt waters, enhancing problems of salt ingression in coastal aquifers, often already overexploited, with consequences on the availability of water for human consumption.
The detrimental effect of climate change, already considered within the ICZM Plan, is here assessed in more detail, based on updated sea level rise projections and storminess forecasts. This study proposes two different methods for the evaluation of coastal inundation based, respectively, on (1) a simple “bathtub” approach and (2) numerical modeling. The former is easy to use and allows to rapidly identify the areas that are more prone to flooding along the entire Marche coastline. However, it has the major flaw of excessively overestimating the inundation extent, mainly because it does not take into account the dissipative processes that the wave undergoes during its propagation from the offshore to the shoreline. The second method relies on numerical modeling and provides more realistic inundation maps. The use of two different solvers allowed us to corroborate the results, demonstrating the reliability of the inundation perimeters calculated by the models. The numerical approach, being able to reproduce the wave propagation and interaction with structures, is, overall, more precise and complete. However, it requires a good knowledge of the bathymetry and topography of the study site, and it takes more time to provide results. Therefore, it can be used specifically for locations recognized as critical, to evaluate the effectiveness of adaptation measures such as coastal defense structures.
The results of the analysis presented here show the vulnerability of mildly sloped sandy beaches to coastal flooding. Moreover, harbor facilities and urban areas are also highly exposed to present and future inundation hazard, as demonstrated by the case of Pesaro. The exposure of the coastal area to increasingly extreme weather and sea conditions, and, in the long run, to critical issues related to the disappearance of the coast, has social and economic implications that must be taken into account. In fact, the high level of urbanization concentrated along the coast and the presence of strategic facilities and infrastructure make it and the population itself highly vulnerable. Another key aspect to assess is the damage to which accommodations located along the coastline could be subject, with direct negative consequences for tourism activities. Finally, climate change has impacts on the chemical and ecological status of the coastal environment, threatening the balance of many marine ecosystems.
4.2 Adaptation measures
Adaptation is defined as the act of dealing with consequences of climate change, by means of actions targeted at a specific vulnerable system, with the objective of either limiting negative impacts or exploiting positive impacts (Kwadijk et al., 2010). Which adaptation strategies to put in place depends on many factors that include accommodation space, adaptability, migration potential, and habitat availability, many of which are controlled by anthropogenic factors, such as urbanization and land use change, as well as political will (Barnard et al., 2021). Adaptation planning focuses on the use of information about current and future climate and reviewing the suitability of current and planned management. Predictability of climate and uncertainties on climate scenarios as current and future trend of non-climatic systems must be accounted for in identifying actions. All these factors must be considered in the planning of adaptation at an adequate scale.
Planning adaptation to climate change implies the use of information about present and future climate change to review the suitability of current and planned practices, policies, and infrastructure (Füssel, 2007). The Plan for Adaptation to Climate Change of Marche Region combines climate scenarios and trends in the economic and environmental factors to find the best options of adaptation.
For coastal systems, this results in two lines of action: the increase in resilience, which has a long-term scope, and coastal defense, which has an emergency nature. The increase in average sea level and the intensification of extreme events, combined with the intense urbanization of the coastal area, makes it necessary to implement measures to combat both coastal erosion and urban flooding phenomena involving buildings and infrastructures. In this sense, adaptation does not only mean defending the present settlement and infrastructure system, but also increasing resilience by leaving more space for the action of the sea, adapting the existing urban fabric to new scenarios and providing, for example, the relocation of infrastructures at risk in the most vulnerable areas. The latter point implies measures such as limiting land consumption or delocalizing structures and infrastructures: giving the high level of land use characterizing the Marche coastline, as well as most of the Northern Adriatic coast, this latter representing the first step for any possible adaptation strategy. Delocalizing means to reduce the risk and to make possible the implementation of alternative measures of defense such as managed realignment, that imply availability of areas for flooding.
Considering the strong economic and social interest involved, it is essential to have reliable information about the effective risks. The European Directive 2007/60/EC on the assessment and management of flood risks imposes to treat different areas according to the risk level. This requires an accurate estimate of the flood perimeters, at the level of the entire coastline or for specific sections considered critical, to better calibrate the response to this type of impact. The determination of the boundaries of inundation through robust models was therefore a preliminary measure of the Regional Adaptation Plan.
Concerning the measures of protection, it is necessary that both the existing and the planned coastal defense works are adequate to sea level scenarios to maintain their efficiency. The simulations done for this work show a sea surface elevation at the peak of the storm smaller (of about 1 m in both the pilots) than the extreme sea surface elevation obtained with the “bathtub” method. This suggests the need of using robust simulation to take decision on managing defenses. A possible further measure in the regional coastal context, is the introduction of artificial submerged reef, that combine energy wave dissipation effects with benefits in terms of ecological improvement and fishery.
5 Conclusions
This work aims at providing information about the inundation extent caused by extreme sea storms under the RCP8.5 scenario along the Marche Region. The results of the analysis were used in the Regional Plan for Adaptation to Climate Change to assess the main vulnerabilities of coastal systems. The work demonstrated that a simple “bathtub” method, although simply applicable to identify the most vulnerable areas, overestimated the inundation extent. Detailed numerical modeling is, therefore, needed to gain a more accurate knowledge of inundation perimeters in order to identify effective adaptation measures to put in place in those locations recognized as critical in terms of coastal vulnerability. Senigallia and Pesaro represent important touristic cities for the Marche Region and were affected by sea inundations and river floodings in the past, this raising the level of attention on these two sites. The presence of harbor and recreative facilities and the high density of buildings and population in the coastal area, increase the potential flood risk for these locations due to future sea storms aggravated by climate change. Numerical simulations run for Senigallia and Pesaro confirmed that, under the RCP8.5 scenario, a 100-year return period sea storm projected to 2070 would cause the inundation of beaches and streets running along the coast, as well as interesting harbor facilities and urban areas. Pesaro showed the worst situation, with almost 0.2 km2 of the urban area south of the harbor affected by the flooding. Such findings are of utmost importance for the regional planning of adaptation to climate change and have been incorporated into the related plan as a basis for defining adaptation strategies.
Data availability statement
Publicly available datasets were analyzed in this study. This data can be found at: https://cds.climate.copernicus.eu/cdsapp#!/dataset/sis-water-level-change-indicators?tab=overview and https://cds.climate.copernicus.eu/cdsapp#!/dataset/sis-ocean-wave-indicators?tab=overview.
Author contributions
AB: Conceptualization, Formal analysis, Methodology, Visualization, Writing – original draft. LM: Conceptualization, Formal analysis, Methodology, Writing – review & editing. FM: Conceptualization, Methodology, Writing – review & editing. GG: Conceptualization, Funding acquisition, Methodology, Project administration, Writing – review & editing. PG: Conceptualization, Funding acquisition, Methodology, Project administration, Writing – review & editing. GF: Conceptualization, Methodology, Writing – review & editing. NB: Conceptualization, Funding acquisition, Project administration, Writing – review & editing. CL: Conceptualization, Methodology, Writing – review & editing. MB: Conceptualization, Methodology, Writing – review & editing.
Funding
The author(s) declare financial support was received for the research, authorship, and/or publication of this article. This study was funded by the AdriaClim Project - Climate change information, monitoring and management tools for adaptation strategies in Adriatic coastal areas, financed by Programma Interreg V A Italia-Croazia 2014–2020, and collaboration agreements between the Ministry of the Environment and Marche Region relating to the implementation of the Regional Strategies for Sustainable Development, approved respectively with council resolution no. 1602/2018 and n. 4/2020.
Acknowledgments
The authors would like to thank the Italian Ministry of Ecological Transition and the Municipality of Senigallia for providing data used to build the bathymetry and topography of the Senigallia test site. Additionally, the authors would like to thank their colleagues of the Dipartimento Infrastrutture, Territorio e Protezione Civile: Stefano Parlani, who provided the high-resolution coastal Lidar data of the Marche Region, and Mauro Sinigaglia, who provided coastal transects to reconstruct the bathymetry of the Pesaro test site.
Conflict of interest
The authors declare that the research was conducted in the absence of any commercial or financial relationships that could be construed as a potential conflict of interest.
Publisher's note
All claims expressed in this article are solely those of the authors and do not necessarily represent those of their affiliated organizations, or those of the publisher, the editors and the reviewers. Any product that may be evaluated in this article, or claim that may be made by its manufacturer, is not guaranteed or endorsed by the publisher.
Footnotes
1. ^Regione Marche Difesa della costa - Piano GIZC 2019.
3. ^Sea Level Projection Tool – NASA Sea Level Change Portal.
4. ^Bathymetry | European Marine Observation and Data Network (EMODnet).
References
Almar, R., Ranasinghe, R., Bergsma, E. W. J., Diaz, H., Melet, A., Papa, F., et al. (2021). A global analysis of extreme coastal water levels with implications for potential coastal overtopping. Nat. Commun. 12, 3775. doi: 10.1038/s41467-021-24008-9
Amarouche, K., and Akpinar, A. (2021). Increasing trend on storm wave intensity in the western Mediterranean. Climate 9, 11. doi: 10.3390/cli9010011
Androulidakis, Y. S., Kombiadou, K. D., Makris, C. V., Baltikas, V. N., and Krestenitis, Y. N. (2015). Storm surges in the Mediterranean Sea: Variability and trends under future climatic conditions. Dyn. Atmosphere. Oceans 71, 56–82. doi: 10.1016/j.dynatmoce.2015.06.001
Baldoni, A., Perugini, E., Penna, P., Parlagreco, L., and Brocchini, M. (2022). A comprehensive study of the river plume in a microtidal setting. Estuar. Coastal Shelf Sci. 275, 107995. doi: 10.1016/j.ecss.2022.107995
Baldoni, A., Perugini, E., Soldini, L., Calantoni, J., and Brocchini, M. (2021). Long-term evolution of an inner bar at the mouth of a microtidal river. Estuar. Coastal Shelf Sci. 262, 107573. doi: 10.1016/j.ecss.2021.107573
Barnard, P. L., Dugan, J. E., Page, H. M., Wood, N. J., Hart, J. A. F., Cayan, D. R., et al. (2021). Multiple climate change-driven tipping points for coastal systems. Sci. Rep. 11, 15560. doi: 10.1038/s41598-021-94942-7
Benetazzo, A., Fedele, F., Carniel, S., Ricchi, A., Bucchignani, E., Sclavo, M., et al. (2012). Wave climate of the Adriatic Sea: a future scenario simulation. Nat. Hazards Earth Syst. Sci. 12, 2065–2076. doi: 10.5194/nhess-12-2065-2012
Bonaldo, D., Antonioli, F., Archetti, R., Bezzi, A., Correggiari, A., Davolio, S., et al. (2019). Integrating multidisciplinary instruments for assessing coastal vulnerability to erosion and sea level rise: Lessons and challenges from the Adriatic Sea, Italy. J. Coastal Conserv. 23, 19–37. doi: 10.1007/s11852-018-0633-x
Bonaldo, D., Bucchignani, E., Ricchi, A., and Carniel, S. (2017). Wind storminess in the Adriatic Sea in a climate change scenario. Acta Adriatica. 58, 2. doi: 10.32582/aa.58.2.1
Booij, N. R. R. C., Ris, R. C., and Holthuijsen, L. H. (1999). A third-generation wave model for coastal regions: 1. Model description and validation. J. Geophys. Res. Oceans 104, 7649–7666. doi: 10.1029/98JC02622
Caires, S., and Yan, K. (2020). Ocean surface wave indicators for the European coast from 1977 to 2100 derived from climate projections. Copernicus Climate Change Service (C3S) Climate Data Store (CDS).
Christensen, O. B. (1998). Climate Simulations With the HIRHAM Limited Area Regional Climate Model Over Scandinavia. Ann Arbor, MI: Na.
Church, J. A., and White, N. J. (2006). A 20th century acceleration in global sea-level rise. Geophys. Res. Lett. 33, 826. doi: 10.1029/2005GL024826
De Leo, D., Briganti, F. R., and Besio, G. (2023). Trends in ocean waves climate within the Mediterranean Sea: a review. Clim. Dyn. 22, 1–12. doi: 10.1007/s00382-023-06984-4
Füssel, H. M. (2007). Adaptation planning for climate change: concepts, assessment approaches, and key lessons. Sust. Sci. 2, 265–275. doi: 10.1007/s11625-007-0032-y
Gallina, V., Torresan, S., Zabeo, A., Rizzi, J., Carniel, S., Sclavo, M., et al. (2019). Assessment of climate change impacts in the North Adriatic coastal area. Part II: consequences for coastal erosion impacts at the regional scale. Water 11, 1300. doi: 10.3390/w11061300
Hallegatte, S., Ranger, N., Mestre, O., Dumas, P., Corfee-Morlot, J., Herweijer, C., et al. (2011). Assessing climate change impacts, sea level rise and storm surge risk in port cities: a case study on Copenhagen. Climatic Change 104, 113–137. doi: 10.1007/s10584-010-9978-3
IPCC (2021). Climate Change 2021: The Physical Science Basis. Contribution of Working Group I to the Sixth Assessment Report of the Intergovernmental Panel on Climate Change. New York, NY: Cambridge University Press, 2391.
Kwadijk, J. C., Haasnoot, M., Mulder, J. P., Hoogvliet, M. M., Jeuken, A. B., van der Krogt, R. A., et al. (2010). Using adaptation tipping points to prepare for climate change and sea level rise: a case study in the Netherlands. Wiley Interdis. Rev. Clim. Change 1, 729–740. doi: 10.1002/wcc.64
Lowe, J. A., and Gregory, J. M. (2005). The effects of climate change on storm surges around the United Kingdom. Philos. Trans. Royal Soc. Mathematic. Phys. Eng. Sci. 363, 1313–1328. doi: 10.1098/rsta.2005.1570
Nerem, R. S., Beckley, B. D., Fasullo, J. T., Hamlington, B. D., Masters, D., Mitchum, G. T., et al. (2018). Climate-change–driven accelerated sea-level rise detected in the altimeter era. Proc. Nat. Acad. Sci. 115, 2022–2025. doi: 10.1073/pnas.1717312115
Noon, V., Rivière, C., Strosser, P., Manea, E., Gissi, E., Barbanti, A., et al. (2021). Study on Integrating an Ecosystem-based Approach into Maritime Spatial Planning. European Commission Report. p. 88. Available online at: https://www.milieu.be/wp-content/uploads/2021/08/Study-on-EBA-in-MSP-NAdriatic-case-study-final-report-25.08.2021.pdf (accessed August 25, 2021).
Paxian, A., Hertig, E., Seubert, S., Vogt, G., Jacobeit, J., Paeth, H., et al. (2015). Present-day and future Mediterranean precipitation extremes assessed by different statistical approaches. Clim. Dyn. 44, 845–860. doi: 10.1007/s00382-014-2428-6
Perini, L., Calabrese, L., Luciani, P., Olivieri, M., Galassi, G., Spada, G., et al. (2017). Sea-level rise along the Emilia-Romagna coast (Northern Italy) in 2100: scenarios and impacts. Nat. Hazards Earth Syst. Sci. 17, 2271–2287. doi: 10.5194/nhess-17-2271-2017
Rizzi, J., Torresan, S., Zabeo, A., Critto, A., Tosoni, A., Tomasin, A., et al. (2017). Assessing storm surge risk under future sea-level rise scenarios: a case study in the north Adriatic coast. J. Coastal Conserv. 21, 453–471. doi: 10.1007/s11852-017-0517-5
Salman, A., Lombardo, S., and Doody, P. (2004). Living with coastal erosion in Europe: sediment and space for sustainability. Eurosion Project Reports. Available online at: http://resolver.tudelft.nl/uuid:483327a3-dcf7-4bd0-a986-21d9c8ec274e
Shi, F., Kirby, J. T., Harris, J. C., Geiman, J. D., and Grilli, S. T. (2012). A high-order adaptive time-stepping TVD solver for Boussinesq modeling of breaking waves and coastal inundation. Ocean Modelling 43, 36–51. doi: 10.1016/j.ocemod.2011.12.004
Stockdon, H. F., Holman, R. A., Howd, P. A., and Sallenger Jr, A. H. (2006). Empirical parameterization of setup, swash, and runup. Coastal Eng. 53, 573–588. doi: 10.1016/j.coastaleng.2005.12.005
Tomasicchio, G. R., Lusito, L., D'Alessandro, F., Frega, F., Francone, A., De Bartolo, S., et al. (2018). A direct scaling analysis for the sea level rise. Stochastic Environ. Res. Risk Assessment 32, 3397–3408. doi: 10.1007/s00477-018-1568-3
Trembanis, A. C., Duo, E., Dohner, S., Grottoli, E., and Ciavola, P. (2017). Quick response assessment of the impact of an extreme storm combining aerial drone and RTK GPS. Nat. Hazards Earth Syst. Sci. Discuss. 12, 337. doi: 10.5194/nhess-2017-337
Woth, K., Weisse, R., and Von Storch, H. (2006). Climate change and North Sea storm surge extremes: an ensemble study of storm surge extremes expected in a changed climate projected by four different regional climate models. Ocean Dyn. 56, 3–15. doi: 10.1007/s10236-005-0024-3
Yan, K., Muis, S., Irazoqui, M., and Verlaan, M. (2020). Water Level Change Indicators for the European Coast from 1977 to 2100 Derived From Climate Projections. Bonn: Copernicus Climate Change Service (C3S) Climate Data Store (CDS).
Zebisch, M., Terzi, S., Pittore, M., Renner, K., and Schneiderbauer, S. (2022). “Climate impact chains —a conceptual modelling approach for climate risk assessment in the context of adaptation planning,” in Climate Adaptation Modelling, eds C. Kondrup, P. Mercogliano, and F. Bosello, F (Cham: Springer International Publishing), 217–224.
Keywords: sea level rise (SLR), climate change adaptation, coastal flooding, inundation perimeters, climate planning
Citation: Baldoni A, Melito L, Marini F, Galassi G, Giacomin P, Filomena G, Barbizzi N, Lorenzoni C and Brocchini M (2024) Modeling coastal inundation for adaptation to climate change at local scale: the case of Marche Region (central Italy). Front. Clim. 6:1334625. doi: 10.3389/fclim.2024.1334625
Received: 07 November 2023; Accepted: 26 January 2024;
Published: 08 February 2024.
Edited by:
Andrea Valentini, Regional Agency for Prevention, Environment and Energy of Emilia-Romagna (ARPAE), ItalyReviewed by:
Riccardo Briganti, University of Nottingham, United KingdomAntonio Francone, University of Salento, Italy
Copyright © 2024 Baldoni, Melito, Marini, Galassi, Giacomin, Filomena, Barbizzi, Lorenzoni and Brocchini. This is an open-access article distributed under the terms of the Creative Commons Attribution License (CC BY). The use, distribution or reproduction in other forums is permitted, provided the original author(s) and the copyright owner(s) are credited and that the original publication in this journal is cited, in accordance with accepted academic practice. No use, distribution or reproduction is permitted which does not comply with these terms.
*Correspondence: Agnese Baldoni, a.baldoni@pm.univpm.it