Impact of El Niño Southern Oscillation on the first inter-monsoon rainfall over Sri Lanka in the post-El Niño years
- 1Graduate School of Life and Environmental Sciences, University of Tsukuba, Tsukuba, Japan
- 2Department of Meteorology, Colombo, Sri Lanka
- 3Faculty of Life and Environmental Sciences, University of Tsukuba, Tsukuba, Japan
Sri Lanka has a tropical monsoon climate. The first intermonsoon (FIM) season is one of four rainfall seasons. Previous investigations have explored the concurrent ramifications of the El Niño Southern Oscillation (ENSO); however, its impact on seasonal rainfall patterns in the country in the post-El Niño years remains unexplored. This study aims to investigate anomalous FIM rainfall in Sri Lanka during the post-El Niño years. Weather station rainfall data from the Department of Meteorology for 114 stations for 1975–2019, Japanese 55-year reanalysis data, and COBE sea surface temperature data were used in this study. A significant negative correlation between rainfall at most stations and the preceding year’s November, December, and January Niño3.4 index (NDJ Niño3.4) was identified for the FIM season. All three super El Niño events and one of the two strong El Niño events agree with the above results, showing a clear negative rainfall anomaly pattern. The Indo-western Pacific Ocean Capacitor effect was identified as the cause of rainfall reduction during the FIM season in Sri Lanka and in contrast, the post-El Niño season in 1988 exhibited a positive rainfall anomaly. A rapid transition from El Niño to La Niña and the associated atmospheric conditions were identified as significant contributors to these features. The findings of this study will help overcome this research gap and improve the accuracy of seasonal forecasts for the country.
Introduction
The El Niño Southern Oscillation (ENSO), the world’s most energetic variation in the climate system, occurs because of ocean and atmosphere interactions in the tropical Pacific Ocean (e.g., Ropelewski and Halpert, 1987; Wallace et al., 1998; McPhaden et al., 2020). El Niño occurs every two to seven years (McPhaden et al., 2006), develops during the boreal summer, peaks during early winter, and decays in the following spring (Xie et al., 2009). During El Niño events, positive sea surface temperature (SST) anomalies have been observed in the eastern equatorial Pacific Ocean east of the international date line (Ueda, 2014). Sea-level pressure rises in the western Pacific and falls in the eastern Pacific, weakening the trade winds along the equator. Weakened trade winds and increased SST are enhanced by each other via Bjerknes feedback (McPhaden et al., 2006).
The remote effects of the ENSO on the Indian Ocean have been investigated in previous studies. Wang et al. (2000, 2003) identified a robust anticyclone signal over the subtropical western North Pacific (WNP) during the ENSO mature phase (winter) and the following spring. This anticyclone is mediated by wind–evaporation–SST feedback and modulates the atmospheric circulation pattern over the subtropical eastern Indian Ocean and the subtropical WNP. Xie et al. (2009, 2016) studied the connection between winter El Niño in the eastern equatorial Pacific Ocean and its subsequent delayed impact on the Indian Ocean during spring and summer. They introduced the inter-basin ocean–atmosphere coupled mode, referred to as the “Indo-western Pacific Ocean Capacitor (IPOC) mode.” During December–February, El Niño exerts its influence on the South Indian Ocean by propagating westward Rossby waves, which induce warming in the Southwest Indian Ocean, consequently generating an antisymmetrical wind pattern over the tropical Indian Ocean from March to May. Subsequently, a second warming event in the Indian Ocean activates a tropospheric Kelvin wave that propagates into the western Pacific. This wave-driven phenomenon modulates the anomalous anticyclone and the Pacific-Japan-East Asia pattern, ultimately influencing the East Asian climate during the subsequent summer season. The IPOC is sustained by wind–evaporation–SST feedback and inter-basin interactions in the Indo-western Pacific from spring to summer, triggered by ENSO through thermodynamic processes such as anomalous surface heat flux, radiation and dynamical processes such as oceanic Rossby waves (Kosaka et al., 2021).
Sri Lanka is a tropical island in the Indian Ocean located between 5° 55′ to 9° 51′ N latitude and 79° 42′ to 81° 53′ E longitude. The total land area of the country is 65,610 km2. Figure 1 shows the rainfall observation sites and a topography map of Sri Lanka. As can be seen in the map, the central part of the southern half of the country is a mountainous area with complex topographical features such as ridges, peaks, plateaus, basins, valleys, and escarpments with a maximum height of more than 2,500 m. Annual rainfall (ARF) in the country varies between 900 mm and 5,000 mm. The country is divided into three climate zones: dry (ARF <1,750 mm), intermediate zone (2,500 mm > ARF > 1,750 mm), and wet (ARF >2,500 mm). Sri Lanka experiences a monsoonal climate with four seasons owing to its location between the summer and winter positions of the Inter-Tropical Convergence Zone (ITCZ). In addition, rainfall patterns in the country are affected by atmospheric changes that occur due to oscillations in the Indian and Pacific Oceans (Jayawardene et al., 2005).
The first intermonsoon (FIM) is one of the country’s four rainfall seasons, and between March and April, the country receives 14% of the ARF. The ITCZ is located on or near the equator during the inter-monsoon seasons, leading to high convective activity in the vicinity of the Sri Lankan region (Punyawardena, 2008). The country mainly experiences calm or light variable winds during this season, and thundershowers occur in many areas in the afternoon or evening.
The concurrent effects of ENSO on Sri Lankan seasonal weather during the genesis period of El Niño have been identified in previous studies. Different findings have been reported on the FIM season. Abeysekera et al. (2019) found that the FIM rainfall considerably decreased in wet and intermediate zones during El Niño years, and a positive anomaly was found during La Niña years. Most other studies have not identified this pattern in the FIM season during years with ENSO events (Suppiah, 1989, 1996, 1997; Punyawardena and Cherry, 1999; Premalal, 2013; Hapuarachchi and Jayawardena, 2015). Previous studies have only been conducted to find the concurrent impact of ENSO in the genesis period. They did not account for the meteorological features (sea level pressure, large-scale circulation, and water vapor transport) that are closely related to the cause of anomalous rainfall.
According to Xie et al. (2016), El Niño impacts the South Indian Ocean through westward Rossby waves during December–February. These Rossby waves induce Southwest Indian Ocean warming during March–May, lagging the peak of SST anomalies over the eastern Pacific by about 3 months. The IPOC effect should also influence Sri Lanka because of its location in the Indian Ocean. Therefore, to determine the effect of ENSO on the FIM rainfall in Sri Lanka, it is necessary to determine the lagged impact of ENSO in the post-ENSO years. Although the IPOC is the most important mechanism related to ENSO in the Indian Ocean region, its impact on Sri Lankan seasonal rainfall has not been investigated in previous studies.
Our study aimed to determine the impact of ENSO on FIM rainfall in Sri Lanka in the post-ENSO years. Researchers have found an increasing trend of El Niño events in the Pacific Ocean (Wang et al., 2019), possibly resulting from global warming. Moreover, the warming of the Indian Ocean influences seasonal rainfall in the country (Kajakokulan et al., 2023). The findings of this study will help provide more accurate seasonal rainfall forecasts for Sri Lanka. A more accurate seasonal forecast will guide decision-makers in disaster management, water management, agriculture, and crop management, and most of the other stakeholders who depend on the weather to succeed will help achieve the sustainable development goals in Sri Lanka in many ways.
Materials and methods
Three types of data are used in this study. Observational rainfall data were obtained from the Department of Meteorology (DOM) in Sri Lanka. For SST data, COBE-SST2 (Hirahara et al., 2014), with a spatial resolution of 1.0° × 1.0° was used. In addition, we used the Japanese 55-year reanalysis (JRA-55) data (Kobayashi et al., 2015) at a 1.25° × 1.25° spatial resolution to investigate atmospheric circulation patterns.
El Niño events were identified according to the Oceanic Niño Index in the Niño 3.4 region, which was defined by the National Oceanic and Atmospheric Administration (NOAA) of the United States Climate Prediction Center. Warm El Niño events were defined as five consecutive overlapping 3-month periods at or above the +0.5 K anomaly (Kousky and Higgins, 2007). Furthermore, strong El Niño events (SST anomalies greater than or equal to 1.5 K and less than 2.0 K) and super El Niño events (SST anomalies greater than or equal to 2.0 K) were identified according to the NOAA classification. Table 1 lists the super and strong El Niño events recorded between 1975 and 2019.
Eastern Pacific SST anomalies began to develop from June to August, reached their peak in December, and then decayed rapidly from April of the following year. This study focused mainly on the decay period. Therefore, the study was conducted during the March–April season in the year following El Niño events. The SST patterns in winter and the following year’s FIM season were investigated for all super and strong El Niño events using the COBE-SST2 dataset.
According to Xie et al. (2010), El Niño-induced Indian Ocean warming persists throughout the following year’s summer, which is consistent with the IPOC only after the 1970s. Xie et al. (2016) identified a high correlation between summer North Indian Ocean SST and the preceding winter ENSO from the mid-1970s. Furthermore, the correlation between atmospheric anomalies in the Indo-tropical North Pacific and ENSO is high because of the high variance of ENSO after the 1970s (Kubota et al., 2015). Therefore, we selected the 1975–2019 period for our study.
Weather station observational monthly and daily rainfall data from the DOM, Sri Lanka, for the above period for 220 stations were used to identify rainfall patterns. Data quality and accuracy are of great concern in this study. Monthly rainfall data from weather stations were checked, and stations with missing values for more than 3 months were eliminated. After elimination, 114 stations (shown in Figure 1) were selected for the FIM season. Unnatural rainfall values were identified by investigating rainfall data time-series plots for each station. The related daily rainfall values were then checked, and unnatural values were corrected by checking the daily records of nearby rainfall stations. Information was also obtained from rainfall and extreme event record books of the DOM. In the FIM season, convective showers may occur within the limit of the base of cumulonimbus clouds. Therefore, the probability of receiving contrasting rainfall at nearby stations under convective activities and extreme rainfall in short periods in some areas is high. Mountain interactions also influence convective rainfall. These factors were also considered during data validation.
The correlation between station seasonal rainfall for the FIM and the preceding year’s November–December–January Niño3.4 SST anomaly (NDJ Niño3.4) was investigated. Rainfall anomalies for the FIM during the decay period of El Niño events were checked for each super and strong El Niño event recorded between 1975 and 2019.
JRA-55 data from 1975 were used to examine the atmospheric behavior associated with the occurrence of rainfall anomalies. Spatial patterns of temporal regression coefficients of geopotential height, horizontal wind, sea level pressure, and anomalous water vapor transport in the FIM in the post-El Niño years were investigated using the NDJ Niño3.4 for 1975–2019. The geopotential height and the u- and v-component horizontal winds were obtained from the isobaric analysis field (anl_p125). Sea-level pressure was analyzed using surface analysis fields (anl_surf125). The total water vapor transport was investigated using the zonal and meridional water vapor flux parameters of the total column analysis field (anl_column125). The behavior of the atmospheric parameters for the FIM in the post-El Niño years was checked for each super and strong El Niño event recorded between 1975 and 2019.
Results
First, we considered the SST patterns of all super and strong El Niño events identified between 1975 and 2019. Figure 2 shows the SST anomaly patterns for October–November–December (OND) in each El Niño year and the SST patterns from March to April for each event. Figures 2J,L show five-year composited fields of SST anomaly patterns. The decay of the warm SST anomaly in the eastern equatorial Pacific in March–April (Figure 2L) can be observed for each super and strong El Niño event (Figures 2C,D,G,H,K). Over the subtropical Northwestern Pacific, a cool SST anomaly (Wang et al., 2000, 2003) was consistently observed during spring. Positive SST anomalies over the Southwest Indian Ocean can be identified in the March–April season (see Figure 3B of Xie et al., 2016), which is a sign of downwelling Rossby waves.
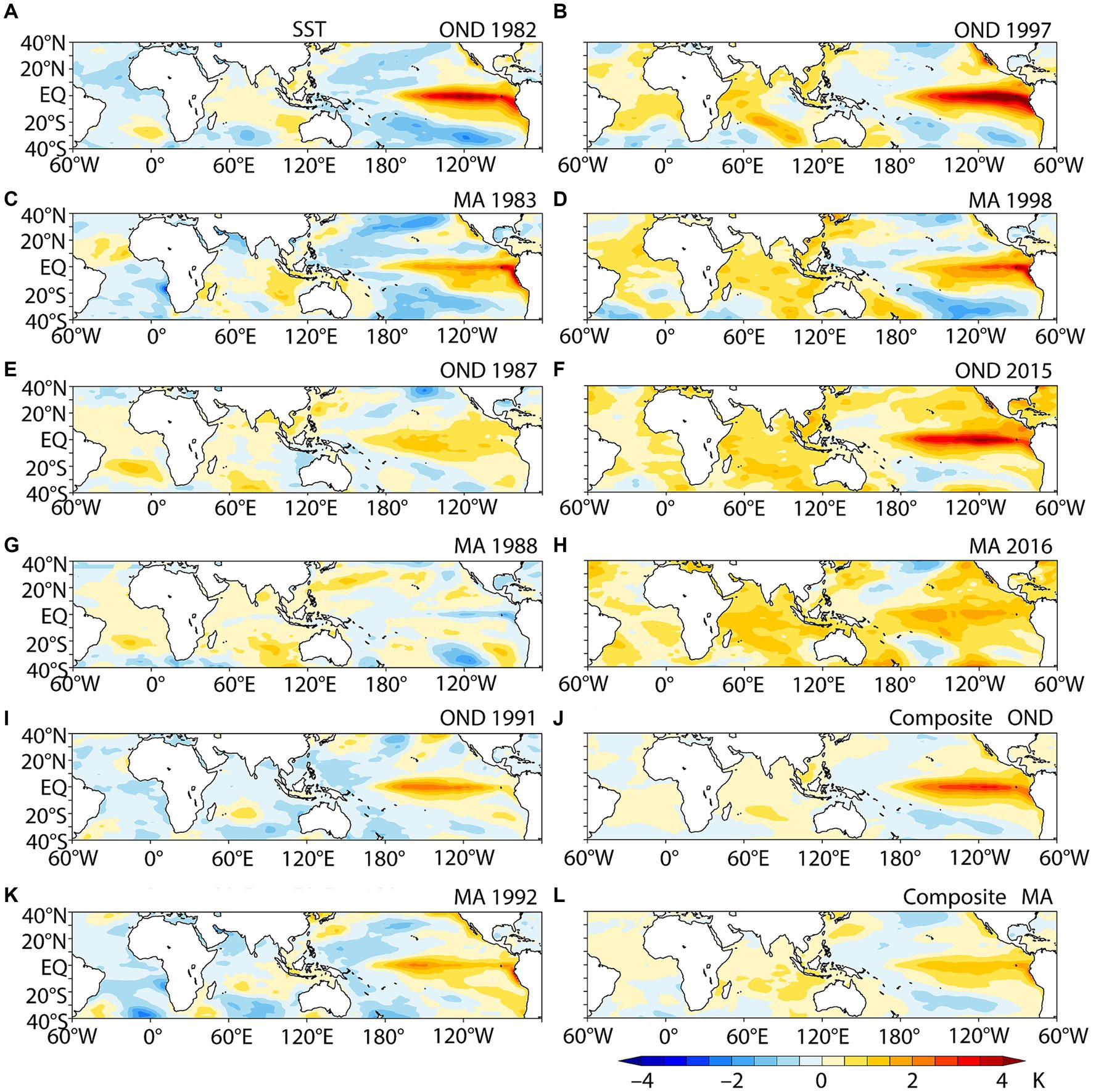
Figure 2. SST anomaly patterns in super and strong El Niño years and the following year, March–April. (A,E,I,B,F) The SST patterns for OND 1982, 1987, 1991, 1997, and 2015, respectively. (C,G,K,D,H) The SST patterns in the following year, March–April. (J,L) The five-year composited SST anomalies for OND and following year March–April, respectively.
As shown in Figure 2A for OND 1982 and in Figure 2B for OND 1997, warmer SST anomalies were observed in the eastern Pacific (160–80° W). In contrast, for OND 2015 (Figure 2F), areas with warmer SST anomalies were observed in the Central Pacific (170–90° W). Moreover, a relatively strong positive SST anomaly was observed over the entire Indian Ocean during this event. During OND 1997, the Indian Ocean exhibited an SST pattern of the Indian Ocean Dipole (IOD; Saji et al., 1999; Webster et al., 1999) mode (cool SST in the southeastern equatorial Indian Ocean and warm SST in the western equatorial Indian Ocean). In 1987 and 1991 (Figures 2E,I), OND showed a weaker positive SST anomaly in the eastern Pacific compared with the super El Niño events shown in Figures 2A,B,F. Peak SST anomalies were identified over the central equatorial Pacific, similar to those observed in 2015. The SST in the Indian Ocean around the Sri Lankan region showed a significant difference between the 1987 and 1991 events (a higher positive SST anomaly during the 1987 event than the 1991 event). Moreover, a positive IOD event was observed during OND 1987 (Figure 2E). In March–April 1988, the development of a negative SST anomaly can be observed in Figure 2G in the eastern Pacific, exhibiting the rapid termination of summertime La Niña.
To investigate the relationship between stations’ seasonal rainfall and NDJ Niño3.4, we plotted the correlation coefficient between stations’ seasonal rainfall in the FIM season and NDJ Niño3.4 for the period 1975–2019 in Figure 3. A negative correlation (−0.6 to 0.0) between almost all stations’ rainfall and the NDJ Niño3.4 index was seen for the FIM season. Although a limited number of stations exhibits a statistically significant correlation, the overall reduction tendency in rainfall is clearly found. To examine the possible atmospheric factors contributing to the nationwide negative correlation, we plotted the horizontal wind anomalies, geopotential height anomalies, sea-level pressure anomalies, and vertically integrated water vapor fluxes associated with NDJ Niño3.4. Figure 4A shows large-scale fields of regression coefficients of the March–April geopotential height and wind at the 850 hPa level onto the preceding year NDJ Niño3.4 for 1958–2021. Figure 4B shows the local regression coefficients for Sri Lanka. The positive geopotential height and anomalous wind patterns that extended from the WNP to the Bay of Bengal were accompanied by an easterly wind anomaly over 10° S–10° N (Figure 4A). As shown in Figure 4B, the easterly wind anomaly can be observed across the country. The geopotential height and wind patterns were consistent with delayed ENSO impacts, as explained by Wang et al. (2000, 2003) and Xie et al. (2009, 2016). Here, the easterly wind anomaly over the tropical Indian Ocean also suggests a weaker Walker circulation over the tropical Indian Ocean. As shown in Figure 2, El Niño tends to decay in March–April, but weak positive SST anomaly sometimes remains over the central-to-eastern tropical Pacific (4 out of 5 years). Therefore, both the IPOC effect and the weak El Niño effect contribute to the easterly wind anomaly. Figure 4C illustrates the large-scale field of the March–April sea-level pressure regression coefficient and vertically integrated water vapor flux onto the preceding year NDJ Niño3.4 for 1958–2021. A high-pressure anomaly pattern is observed over the WNP, which is consistent with Figure 4A. Another feature observed in this figure is a robust low-pressure anomaly over the southern Indian Ocean. Figure 4D shows the local regression coefficients for Sri Lanka. An easterly water vapor flux anomaly was observed, similar to the low-level wind pattern (Figure 4B). This pattern indicates the overall weakening of the climatological water vapor flow from west to east across the country. These atmospheric circulation anomalies mediated by the IPOC and ENSO effects are closely related to the reduction in rainfall over the country in the FIM during the ENSO decay period (Figure 3).
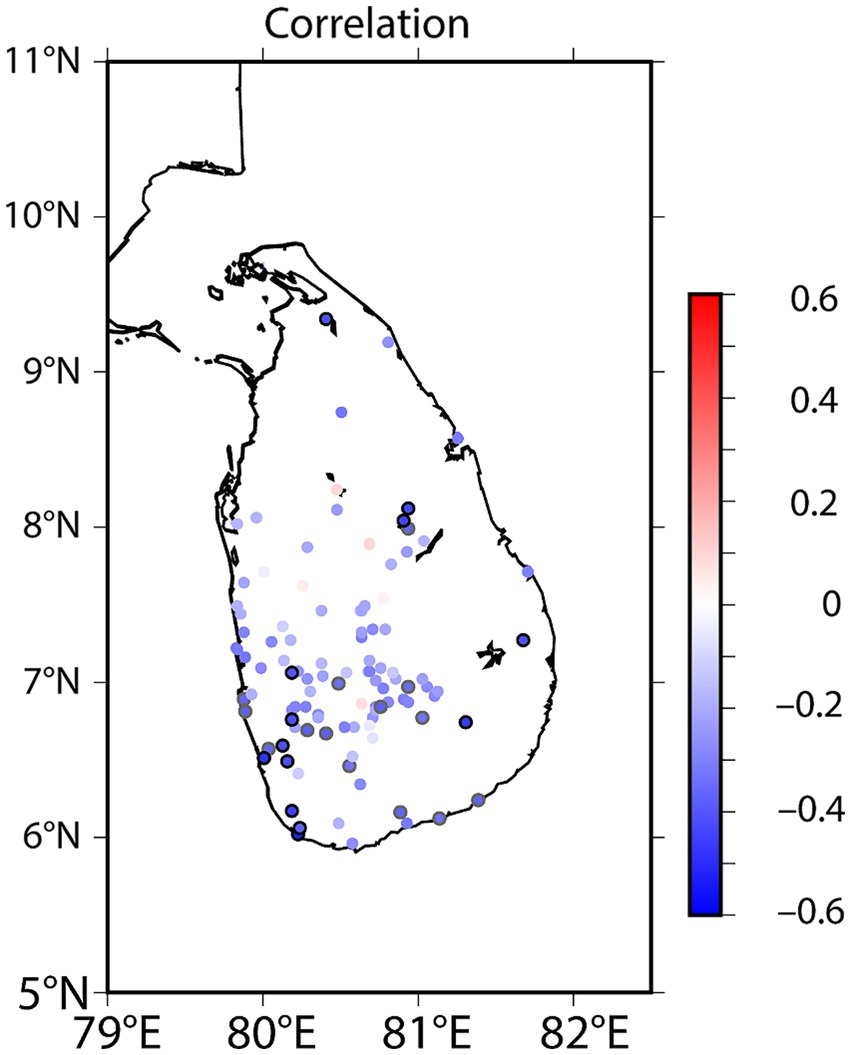
Figure 3. Correlation coefficient between stations’ seasonal rainfall in the FIM and NDJ Niño3.4 for 1975–2019. Black and gray outlined circles indicate that the correlation is statistically significant at 99 and 95% levels, respectively.
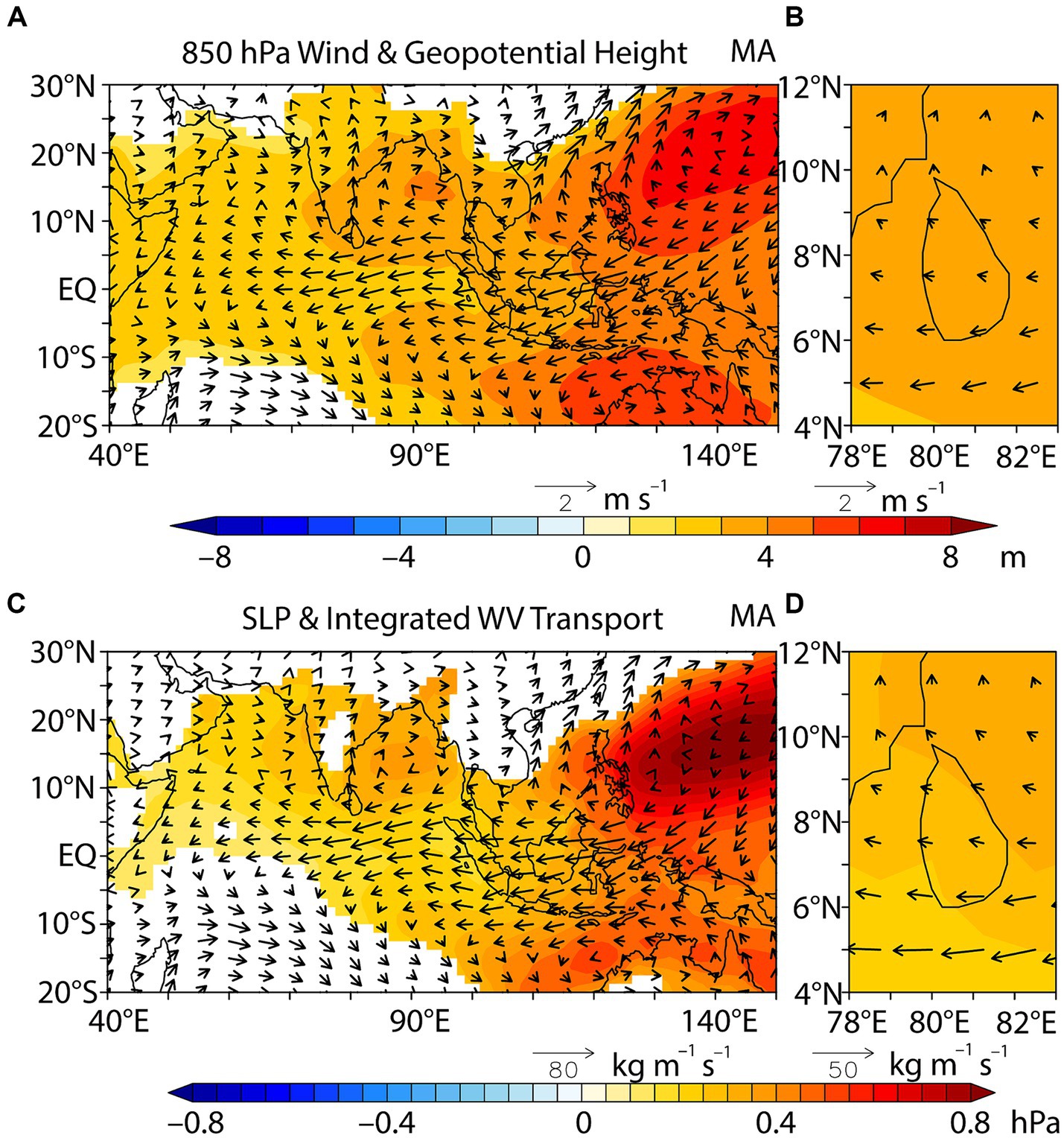
Figure 4. (A,B) Spatial patterns of temporal regression coefficients of geopotential height (m; shading) and horizontal wind (m/s; vectors) at 850 hPa level between March and April onto NDJ Niño3.4 (K) for 1958–2021 over (A) the western Pacific and Indian Ocean, and (B) Sri Lanka. (C,D) Spatial patterns of temporal regression coefficients of sea level pressure (hPa; shading) and vertically integrated water vapor flux (kg m−1 s−1) between March and April onto NDJ Niño3.4 for 1958–2021 over (C) the western Pacific and Indian Ocean, and (D) Sri Lanka. Only regression coefficients of geopotential height significant at the 95% confidence level are shown.
Thereafter, we investigated seasonal rainfall anomalies during each FIM season during the decay period of super and strong El Niño events from 1975 to 2019 to identify the reliability and consistency of the negative correlation. Figure 5 shows the seasonal rainfall anomalies at each station in the FIM during the decay periods of super and strong El Niño events. Figures 5A,C,D,E show negative rainfall anomalies for the FIM during the decay period of all super El Niño events and a strong El Niño event. The percentages of stations showing negative anomalies in 1983, 1998, 2016, and 1992 were 99, 95, 95, and 85%, respectively. In contrast, this consistent pattern was not observed in the FIM season during the decay period of the super El Niño event in 1987 (Figure 5B). This year, 87% of the stations on the island exhibited positive rainfall anomalies.
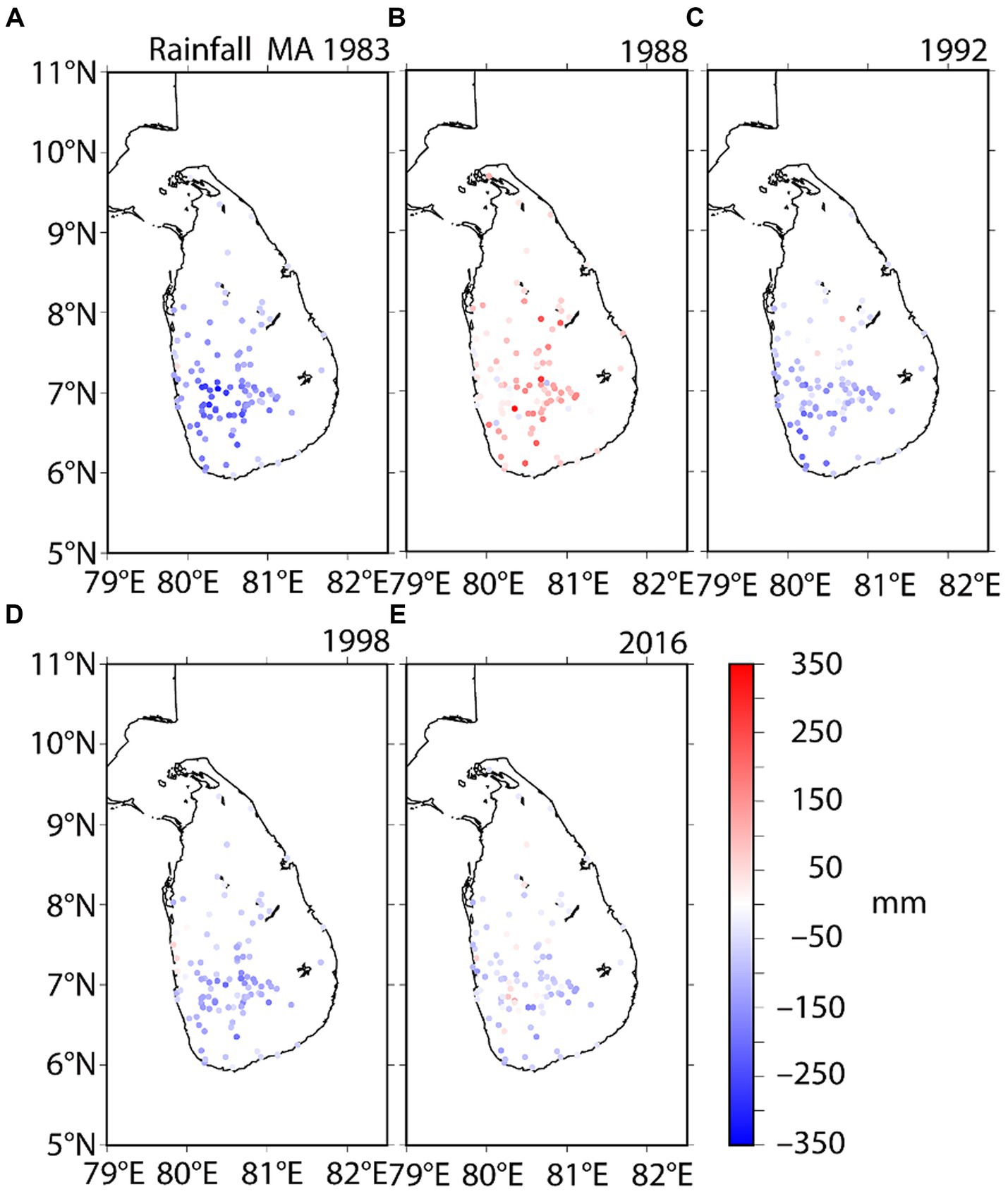
Figure 5. Seasonal rainfall anomalies (mm) in each station in the FIM during the decaying period of super and strong El Niño events between 1975–2021. (A) 1983, (B) 1988, (C) 1992, (D) 1998, and (E) 2016.
To examine possible atmospheric factors contributing to this opposite tendency in the event of 1988, we investigated the atmospheric parameters in the 1988 event. Figure 6 shows the behavior of atmospheric parameters during March–April 1988. The negative geopotential height and negative sea-level pressure observed in the entire Indian Ocean region are shown in Figures 6A,C, respectively. Furthermore, westerly water vapor flux anomalies are observed in Figure 6D. The high-pressure and positive geopotential height anomalies over the WNP resulting from delayed ENSO impacts are shown in Figures 6A,C. However, their intensities and horizontal extents are limited. Over the Indian Ocean and Bay of Bengal, a westerly wind anomaly was broadly found in March–April 1988, in contrast to that shown in Figure 4. The total water vapor transport from the west was enhanced over the Sri Lankan region (Figure 6D), which is consistent with the overall increase in rainfall (Figure 5B).
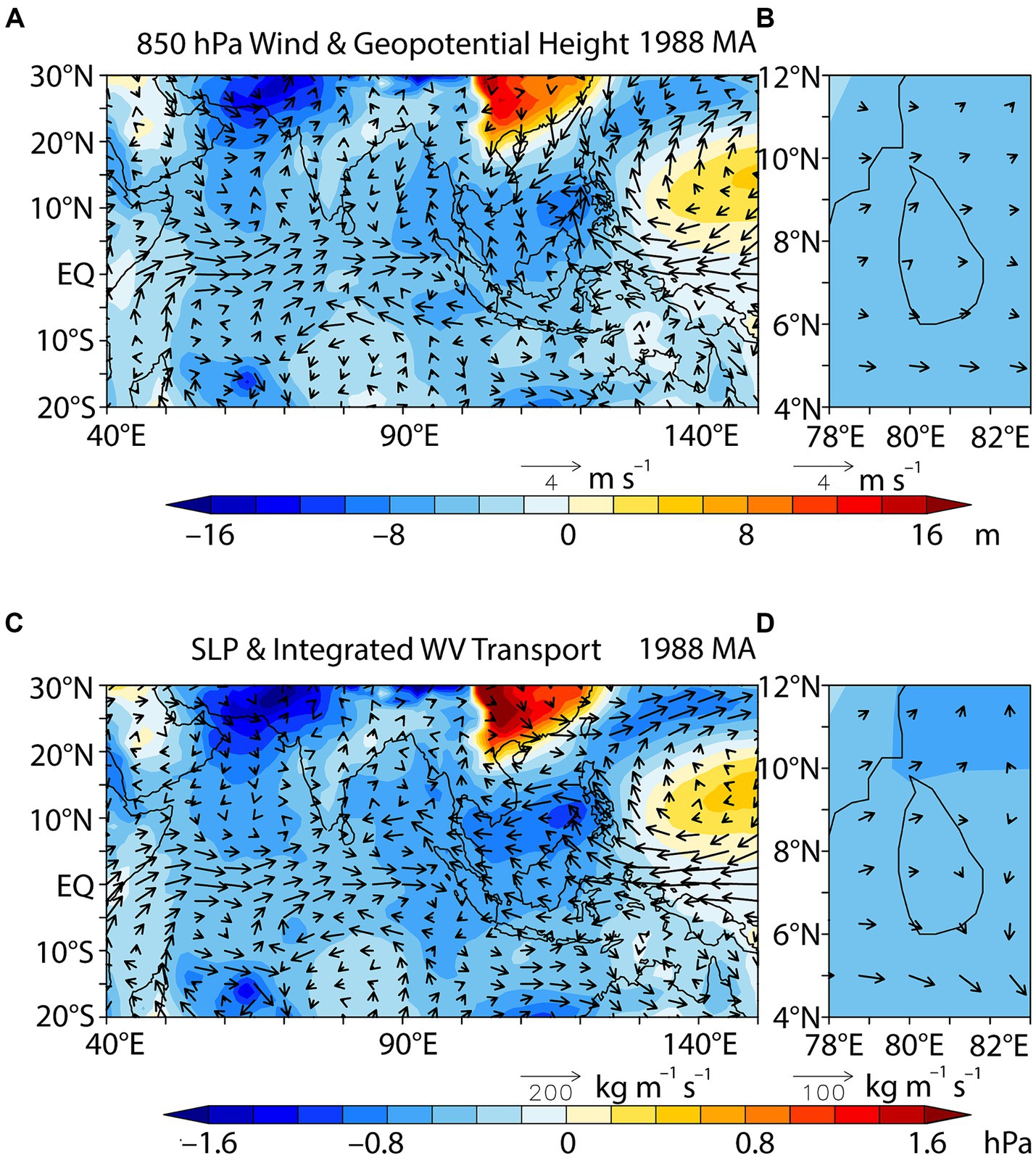
Figure 6. Behavior of atmospheric parameters during March–April 1988. (A) Geopotential height (m; shading) and horizontal wind (m/s; vectors) anomalies at 850 hPa level over the western Pacific and Indian Ocean, and (B) Sri Lanka. (C) Sea level pressure anomalies (hPa) and vertically integrated water vapor flux (kg m−1 s−1) anomalies between March and April 1988 over the western Pacific and Indian Ocean, and (D) Sri Lanka.
Furthermore, as illustrated in Figure 2G, a negative SST anomaly was observed in the eastern equatorial Pacific. A positive SST anomaly over the southwestern Indian Ocean, which is a sign of a downwelling Rossby wave, cannot be observed in this figure.
Discussion
These results show a negative correlation between station rainfall and the preceding year’s NDJ Niño3.4 index during the FIM season. The IPOC effect and the resulting atmospheric mechanisms were associated with a reduction in rainfall over the country in the FIM during the decaying period of El Niño. The negative rainfall anomaly patterns in all three super El Niño events and one of the two strong El Niño events provide further evidence for this finding. In contrast, the FIM in the decay period of a strong El Niño event in 1987–1988 shows a positive rainfall anomaly at 83% of the stations. No tropical cyclones were recorded in the Bay of Bengal from March to April 1988. Therefore, it is unlikely that these systems influenced the rainfall enhancement in Sri Lanka during the FIM season. In this study, the possible factors were investigated, and further discussion is as follows.
Negative SST anomalies can be observed in the central to eastern equatorial Pacific during the decay period of the 1987 strong El Niño event and are distinct from the warm SST anomaly in the following year, March–April, in other super and strong El Niño years (1982, 1997, 2015, and 1991). This indicates the rapid termination of the summer La Niña events in 1988. This could be the main reason for the contrasting results of the 1987 event. La Niña strongly affects Walker circulation, trade winds over the equatorial Pacific Ocean, and diabatic heating in the tropical atmosphere, resulting in a significant anomalous pattern in atmospheric circulation and rainfall over the tropical Indian Ocean (e.g., Naoi et al., 2020).
A few previous studies have identified the impact of the IOD on seasonal rainfall in Sri Lanka only during the second intermonsoon season. Furthermore, no previous research has been conducted on the relationship between the FIM seasonal rainfall and IOD. We investigated the impact of the IOD on the FIM rainfall during five selected El Niño events. Table 2 shows the average IOD index and the percentage of stations that showed a negative anomaly in March–April in the year following El Niño events. As shown in Table 2, the IOD averages for 1983, 1992, 1998, and 2016 (with an overall negative rainfall tendency in Sri Lanka) did not show consistent signs (negative for 1983 and 1992, almost neutral for 1998, and positive for 2016). In 1988, the average IOD value was negative; however, the percentage of stations showing negative anomalies was low (13%). Therefore, a reliable and consistent relationship between the IOD and seasonal rainfall anomaly patterns during the decay period of El Niño events could not be identified. Further studies are needed to determine the connection between seasonal rainfall in Sri Lanka and the impact of the IOD on the FIM.
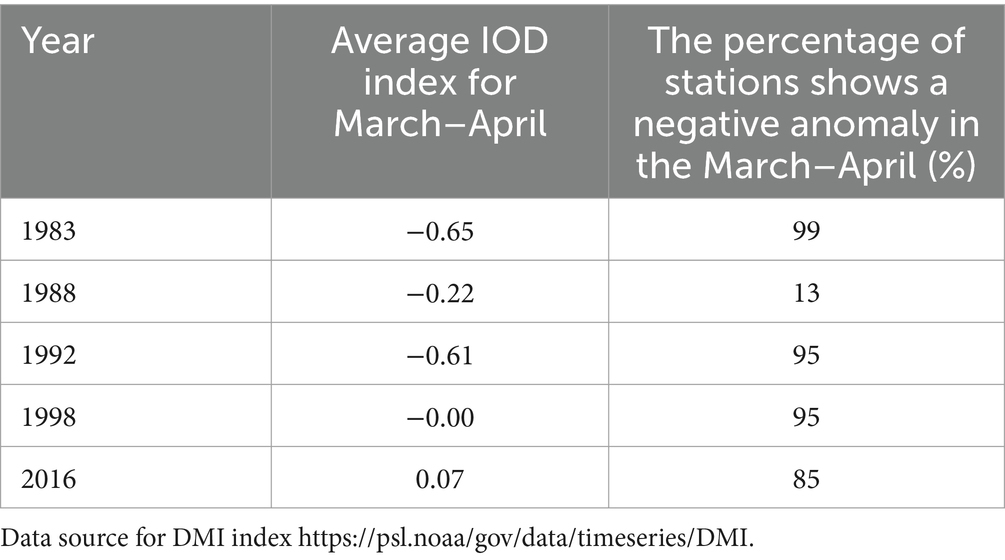
Table 2. Average IOD index and percentage of the stations that show a negative anomaly in March–April in the year following El Niño events.
Jayawardena et al. (2020) identified that the MJO in phases 1, 2, 3, and 8 tended to enhance the FIM seasonal rainfall in Sri Lanka. In March 1988, MJO was in phases 1, 2, and 3, and in phases 8, 1, and 2 in April. These conditions may have also contributed to the enhancement of rainfall during the FIM season in 1988.
Previous research findings have shown that different types of El Niño events (Central Pacific El Niño or Eastern Pacific El Niño) may have different impacts in many areas of the world. The position of the warmest SST anomaly resulted in a different Walker circulation response through latent heating (Weng et al., 2009; Blamey et al., 2018). Moreover, the slightly different peak periods of each ENSO event can also result in different impacts on seasonal climates worldwide. This could be another reason for the different impacts of seasonal rainfall in Sri Lanka.
When considering all the reported El Niño events in the period between 1975–2019, it should be noted that all the events from 1997 showed comparatively high SST anomalies in the Indian Ocean and Arabian Sea regions during the decay period of those events. Over the long term, the Indian Ocean has exhibited a faster warming trend than other ocean basins (Roxy et al., 2015). More detailed research on the possible impacts of global warming and decadal climate variability on seasonal rainfall in Sri Lanka is also necessary.
Data availability statement
The original contributions presented in the study are included in the article/supplementary material, further inquiries can be directed to the corresponding author.
Author contributions
KR: Data curation, Formal analysis, Investigation, Visualization, Writing – original draft. YK: Conceptualization, Funding acquisition, Methodology, Project administration, Resources, Software, Supervision, Validation, Writing – review & editing.
Funding
The author(s) declare that financial support was received for the research, authorship, and/or publication of this article. We acknowledge the Project for Human Resource Development Scholarship (JDS) through Japanese Grant Aid (No. B0012021LKA002). This research was supported by the JSPS KAKENHI (Grant numbers: JP19H05704 and JP24H02228).
Acknowledgments
The authors thank H. Ueda at the University of Tsukuba, S. Sugata, and T. Nagashima at the National Institute for Environmental Studies for their helpful comments. We are also grateful to three reviewers for their comments. We would like to thank Editage (www.editage.com) for English language editing.
Conflict of interest
The authors declare that the research was conducted in the absence of any commercial or financial relationships that could be construed as a potential conflict of interest.
Publisher’s note
All claims expressed in this article are solely those of the authors and do not necessarily represent those of their affiliated organizations, or those of the publisher, the editors and the reviewers. Any product that may be evaluated in this article, or claim that may be made by its manufacturer, is not guaranteed or endorsed by the publisher.
References
Abeysekera, A. B., Punyawardena, B. V. R., Marambe, B., Jayawardena, I. M., Wickremasinghe, V. N., Senarathna, S. D., et al. (2019). Effect of El Niño Southern Oscillation (ENSO) events on inter-seasonal variability of rainfall in wet and intermediate zones of Sri Lanka. Trop. Agric. 167, 14–27.
Blamey, R. C., Kolusu, S. R., Mahlalela, P., Todd, M. C., and Reason, C. J. C. (2018). The role of regional circulation features in regulating El Niño climate impacts over southern Africa: a comparison of the 2015/2016 drought with previous events. Int. J. Climatol. 38, 4276–4295. doi: 10.1002/joc.5668
Hapuarachchi, H. A. S. U., and Jayawardena, I. M. S. P. (2015). Modulation of seasonal rainfall in Sri Lanka by ENSO extremes. Sri Lanka J. Meteorol. 1, 3–11.
Hirahara, S., Ishii, M., and Fukuda, Y. (2014). Centennial-scale sea surface temperature analysis and its uncertainty. J. Clim. 27, 57–75. doi: 10.1175/JCLI-D-12-00837.1
Jayawardena, I. S. P., Wheeler, M. C., Sumathipala, W. L., and Basnayake, B. R. S. B. (2020). Impacts of the Madden–Julian oscillation (MJO) on rainfall in Sri Lanka. Mausam 71, 405–422. doi: 10.54302/mausam.v71i3.39
Jayawardene, H. K. W. I., Sonnadara, D. U. J., and Jayewardene, D. R. (2005). Trends of rainfall in Sri Lanka over the last century. Sri Lankan J. Phys. 6, 7–17. doi: 10.4038/sljp.v6i0.197
Kajakokulan, P., Pathirana, G., Dheerasinghe, M., and Edirisooriya, I. (2023). Influence of Indian Ocean warming on rainfall of Sri Lanka. Int. J. Climatol. 43, 4917–4926. doi: 10.1002/joc.8124
Kobayashi, S., Ota, Y., Harada, Y., Ebita, A., and Moriya, M. (2015). The JRA-55 reanalysis: general specifications and basic characteristics. J. Meteorol. Soc. Jpn. 93, 5–48. doi: 10.2151/jmsj.2015-001
Kosaka, Y., Takaya, Y., and Kamae, Y. (2021). “The Indo-western Pacific Ocean capacitor effect” in Tropical and extratropical air-sea interactions. ed. S. K. Behera, (Amsterdam: Elsevier) 141–169.
Kousky, V. E., and Higgins, R. W. (2007). An alert classification system for monitoring and assessing the ENSO cycle. Climate Prediction Center, Maryland. Weather Forecast. 22, 353–371. doi: 10.1175/WAF987.1
Kubota, H., Kosaka, Y., and Xie, S.-P. (2015). A 117-year long index of the Pacific-Japan pattern with application to interdecadal variability. Int. J. Climatol. 36, 1575–1589. doi: 10.1002/joc.4441
McPhaden, M. J., Santoso, A., and Cai, W. (2020). El Niño Southern Oscillation in a changing climate. New Jersey: John Wiley & Sons, 528.
McPhaden, M. J., Zebiak, S. E., and Glantz, M. H. (2006). ENSO as an integrating concept in earth science. Science 314, 1740–1745. doi: 10.1126/science.1132588
Naoi, M., Kamae, Y., Ueda, H., and Mei, W. (2020). Impacts of seasonal transitions of ENSO on atmospheric river activity over East Asia. J. Meteorol. Soc. Jpn. 98, 655–668. doi: 10.2151/jmsj.2020-027
Premalal, K. H. M. S.. (2013). Change and behavior of rainfall pattern in Sri Lanka with Southern Oscillation (SO)—El Niño and La Niña. SAARC Meteorological Research Centre, Dhaka, Bangladesh.
Punyawardena, B. V. R.. (2008). Rainfall and agro-ecological regions of Sri Lanka (Sinhala). Peradeniya, Sri Lanka, Department of Agriculture 43.
Punyawardena, B. V. R., and Cherry, N. J. (1999). Assessment of the predictability of seasonal rainfall in Ratnapura using the Southern Oscillation and its two extremes. J. Natl. Sci. Found. Sri Lanka 27, 187–196. doi: 10.4038/jnsfsr.v27i3.3059
Ropelewski, C. F., and Halpert, M. S. (1987). Global and regional scale precipitation patterns associated with the El Niño/Southern Oscillation. Mon. Weather Rev. 115, 1606–1626. doi: 10.1175/1520-0493(1987)115<1606:GARSPP>2.0.CO;2
Roxy, M. K., Ritika, K., Terray, P., Murtugudde, R., Ashok, K., and Goswami, B. N. (2015). Drying of Indian subcontinent by rapid Indian Ocean warming and a weakening land-sea thermal gradient. Nat. Commun. 6:7423. doi: 10.1038/ncomms8423
Saji, N., Goswami, B., Vinayachandran, P., and Yamagata, T. (1999). A dipole mode in the tropical Indian Ocean. Nature 401, 360–363. doi: 10.1038/43854
Suppiah, R. (1989). Relationship between the Southern Oscillation and the rainfall of Sri Lanka. Int. J. Climatol. 9, 601–618. doi: 10.1002/joc.3370090605
Suppiah, R. (1996). Spatial and temporal variations in the relationships between the Southern Oscillation phenomenon and the rainfall of Sri Lanka. Int. J. Climatol. 16, 1391–1407. doi: 10.1002/(SICI)1097-0088(199612)16:12<1391::AID-JOC94>3.0.CO;2-X
Suppiah, R. (1997). Extremes of the Southern Oscillation phenomenon and the rainfall of Sri Lanka. Int. J. Climatol. 17, 87–101. doi: 10.1002/(SICI)1097-0088(199701)17:1<87::AID-JOC95>3.0.CO;2-X
Ueda, H. (2014). Climate system study-global monsoon perspective. Tsukuba: University of Tsukuba Press, 23–87.
Wallace, J. M., Mitchell, T. P., Rasmusson, E. M., Kousky, V. E., Sarachik, E. S., and von Storch, H. (1998). On the structure and evolution of ENSO-related climate variability in the tropical Pacific: lessons from TOGA. J. Geophys. Res. 103, 14241–14259. doi: 10.1029/97JC02905
Wang, B., Luo, X., Yang, Y. M., Sun, W., Cane, M. A., Cai, W., et al. (2019). Historical change of El Niño properties sheds light on future changes of extreme El Niño. Proc. Natl. Acad. Sci. U.S.A. 116, 22512–22517. doi: 10.1073/pnas.1911130116
Wang, B., Wu, R., and Fu, X. (2000). Pacific–East Asian teleconnection: how does ENSO affect East Asian climate? J. Clim. 13, 1517–1536. doi: 10.1175/1520-0442(2000)013<1517:PEATHD>2.0.CO;2
Wang, B., Wu, R., and Li, T. (2003). Atmosphere–warm ocean interaction and its impacts on Asian–Australian monsoon variation. J. Clim. 16, 1195–1211. doi: 10.1175/1520-0442(2003)16<1195:AOIAII>2.0.CO;2
Webster, P. J., Moore, A. M., Loschnigg, J. P., and Lebel, R. R. (1999). Coupled oceanic-atmospheric dynamics in the Indian Ocean during 1997–1998. Nature 401, 356–360. doi: 10.1038/43848
Weng, H., Behera, S. K., and Yamagata, T. (2009). Anomalous winter climate conditions in the Pacific rim during recent El Niño Modoki and El Niño events. Clim. Dyn. 32, 663–674. doi: 10.1007/s00382-008-0394-6
Xie, S.-P., Du, Y., Huang, G., Zheng, X.-T., Tokinaga, H., Hu, K., et al. (2010). Decadal shift in El Nino influences on Indo-western Pacific and East Asian climate in the 1970s. J. Clim. 23, 3352–3368. doi: 10.1175/2010JCLI3429.1
Xie, S.-P., Hu, K., Hafner, J., Tokinaga, H., Du, Y., Huang, G., et al. (2009). Indian Ocean capacitor effect on Indo-western Pacific climate during the summer following El Niño. J. Clim. 22, 730–747. doi: 10.1175/2008JCLI2544.1
Keywords: first inter-monsoon, seasonal rainfall, El Niño Southern Oscillation, decaying period of El Niño, Indo-western Pacific Ocean Capacitor effect
Citation: Ranaweera KRKDN and Kamae Y (2024) Impact of El Niño Southern Oscillation on the first inter-monsoon rainfall over Sri Lanka in the post-El Niño years. Front. Clim. 6:1361322. doi: 10.3389/fclim.2024.1361322
Edited by:
Karumuri Ashok, University of Hyderabad, IndiaReviewed by:
Feba Francis, Université Catholique de Louvain, BelgiumSwadhin Kumar Behera, Japan Agency for Marine-Earth Science and Technology (JAMSTEC), Japan
Raju Pathak, King Abdullah University of Science and Technology, Saudi Arabia
Copyright © 2024 Ranaweera and Kamae. This is an open-access article distributed under the terms of the Creative Commons Attribution License (CC BY). The use, distribution or reproduction in other forums is permitted, provided the original author(s) and the copyright owner(s) are credited and that the original publication in this journal is cited, in accordance with accepted academic practice. No use, distribution or reproduction is permitted which does not comply with these terms.
*Correspondence: Youichi Kamae, kamae.yoichi.fw@u.tsukuba.ac.jp