- 1Department of Cardiology, St. Vincent’s Hospital, Melbourne, VIC, Australia
- 2Department of Cardiology, Peninsula Health, Melbourne, VIC, Australia
- 3Department of Medicine, Monash University, Melbourne, VIC, Australia
Background: Inflammation and microvascular dysfunction (MVD) are independently associated with adverse cardiovascular outcomes in patients with ischemic heart disease. This study aimed to assess the relationship between inflammation, MVD, and myocardial injury.
Methods: Coronary microvascular function was assessed in 74 patients undergoing percutaneous coronary intervention (PCI) using the index of microvascular resistance (IMR) by a pressure–temperature sensor-tipped wire. Serum high-sensitivity C-reactive protein (hsCRP) level was quantified by rate turbidimetry. Severe MVD was defined as IMR ≥ 30. Pearson correlation was computed to assess the relationships between hsCRP, troponin, and IMR of culprit vessel. Predictors of severe MVD were assessed by regression analysis.
Results: Acute coronary syndromes (ACSs) represented 49% of the total cohort. Study cohort was divided into low C-reactive protein (CRP) (hsCRP < 3 mg/L) and high CRP (hsCRP ≥ 3 mg/L) groups. There was higher representation of smokers (78 vs. 52%), diabetics (39 vs. 18%), and ACS (61 vs. 33%), as well as higher body mass index (29.4 ± 4.6 vs. 27.2 ± 4.1) in the high CRP group. Pre-PCI and post-PCI IMR were significantly elevated in the high CRP group compared to the low CRP group (pre-PCI IMR: 29.0 ± 13.9 vs. 17.4 ± 11.1, p < 0.0001; post-PCI IMR: 23.0 ± 16.8 vs. 15.5 ± 8.4, p = 0.02). Peak troponin levels were significantly raised in the high CRP group (9.96 ± 17.19 vs. 1.17 ± 3.00 μg/L, p = 0.002). There was a strong positive correlation between hsCRP and pre-PCI IMR (r = 0.85, p < 0.0001). Pre- and post-PCI IMR levels were correlated with peak troponin level (r = 0.45, p < 0.0001; r = 0.33, p = 0.005, respectively). Predictors of severe MVD include male gender (OR 3.0), diabetes (OR 3.7), smoking history (OR 4.0), ACS presentation (OR 8.5), and hsCRP ≥ 3 mg/L (OR 5.6).
Conclusion: hsCRP is a significant predictor of MVD while MVD is associated with myocardial injury, supporting the central role of inflammation and MVD in the pathophysiology and complications of coronary artery disease.
Clinical Trial Registration: Australian New Zealand Clinical Trials Registry (ACTRN): 12617000648325. Universal Trial Number (UTN): U1111-1196-2246.
Introduction
The paradigm is shifting in our understanding of atherosclerosis and acute coronary syndrome (ACS). The observation that inflammation plays a major role in atherogenesis and cardiovascular outcomes has a significant impact on current management of coronary artery disease (1). Multiple risk markers have been evaluated in the past to improve risk stratification and prediction of adverse cardiac events (2, 3). Among myriads of inflammatory markers, C-reactive protein (CRP), an acute-phase reactant produced predominantly in hepatocytes, emerges as a valuable biomarker in refining risk assessment (4–6). However, conflicting data regarding its clinical utility in addition to current risk prediction models have hindered its widespread use in routine clinical practice (7–9).
The Coronary Microcirculation refers to small pre-arterioles and arterioles (<500 μm in diameter) that are the major determinant of coronary vascular resistance and modulates coronary blood flow in response to neural, mechanical, and metabolic factors (10). Microvascular dysfunction (MVD) is commonly considered a consequence of ACS secondary to distal embolization of atherothrombotic debris (11), as well as functional impairment of microcirculatory flow due to release of vasoactive factors and inflammatory mediators from the disrupted atherosclerotic plaques (12, 13). Conversely, MVD is associated with atherosclerotic disease progression, and it predates the clinical manifestation of myocardial ischemia (11). The presence of MVD is a predictor of long-term adverse cardiovascular outcomes even in those without significant epicardial disease (14–16). On the other hand, the systemic nature of MVD is evidenced by a diminished microvascular density in non-ischemic myocardium and presence of severe MVD in myocardium distant from the site of infarction (17, 18).
Both inflammation and MVD lead to adverse cardiovascular outcomes. To date, there is a paucity of evidence to suggest the link between inflammation and MVD despite this strong potential association. Therefore, this study will evaluate the relationships between inflammation, MVD, and myocardial injury.
Materials and Methods
Study Population
The study population consisted of consecutive patients undergoing percutaneous coronary intervention (PCI) for ischemic heart disease (IHD). Informed consent was obtained from the participants before coronary angiography. Patients were excluded if they had active inflammatory/autoimmune disorders, receiving immunosuppressant therapy, previous history of myocardial infarction, or PCI of the culprit vessel in the previous 12 months, previous coronary artery bypass graft surgery, severe renal impairment [estimated glomerular filtration rate (eGFR) < 30 mL/min], severe left ventricular dysfunction (ejection fraction ≤ 35%), contraindication to prolonged dual antiplatelet therapy, and significant valvular heart disease. ACS was defined as ST-segment elevation myocardial infarction (STEMI) or non-ST-segment elevation myocardial infarction (NSTEMI).
Study Protocol
All patients had their blood samples taken upon initial presentation to hospital. Serum high-sensitivity C-reactive protein (hsCRP) levels were quantified by rate turbidimetry on a Beckman Coulter AU Analyzer. Study patients received an initial weight-adjusted bolus of 100 U/kg intravenous heparin with additional bolus dosing to maintain an activated clotting time of >250 s. Right femoral vein was cannulated and a 5F venous sheath was inserted to allow drug delivery.
A 6F coronary guiding catheter was used to engage the selected coronary artery. A standard dose of 200 μg of intravenous nitroglycerine was administered to each study artery to minimize changes in coronary volume. Coronary microvascular function was assessed using a dual sensor pressure–temperature wire. The guide wire was calibrated with distal sensor placed at the ostium of the coronary artery to equalize the guiding catheter pressure, and then advanced beyond the stenosis into the distal third of the vessel. Hyperemia was achieved by intravenous adenosine infusion at a rate of 140 μg/kg/min via right femoral vein. A physiological response to adenosine was observed in all patients.
Microvascular resistance of culprit vessel was assessed utilizing index of microvascular resistance (IMR) as previously described (19). Thermodilution curves and hyperemic transit time (TmnHyp) were derived by injecting 3 mL of room temperature saline into the coronary artery through the guiding catheter. IMR was calculated as the distal coronary pressure divided by the inverse of mean transit time at maximal hyperemia using the following formula (20):
where Pa was mean hyperemic aortic pressure and Pd was the mean distal coronary pressure. Pw was referred as the coronary wedge pressure, which was defined as the distal coronary pressure obtained during a 30-s balloon occlusion of the culprit vessel during the initial balloon inflation (21). Fractional flow reserve (FFR) was defined as the mean distal coronary pressure divided by the mean aortic pressure during hyperemia. Coronary flow reserve (CFR) was defined by dividing the baseline transit time by the hyperemic transit time (22). Care was taken to ensure that the distal sensor was in the same position between measurements to avoid errors in transit time acquisition. Significant MVD was defined as IMR > 30 in accordance with previously published data (23, 24).
Index of microvascular resistance, FFR, and CFR was measured in the culprit artery at baseline. Stenting of the culprit vessel was then performed and physiological measures were repeated. The decision to intervene and the use of glycoprotein IIb/IIIa inhibitors and direct thrombin inhibitors were at the discretion of operators. Troponin I and creatine kinase were sequentially measured every 6 h up to a maximum of 24 h following PCI.
The study protocol was approved by the Human Research Ethics Committee at St Vincent’s Hospital Melbourne. All subjects gave written informed consent in accordance with the Declaration of Helsinki.
Statistical Analysis
Statistical analysis was performed using SPSS statistical software system. Continuous variables were summarized as mean ± SD and were compared with the Student’s t-test. Non-parametric tests were used where appropriate. Normality of data was assessed with the Kolmogorov–Smironov statistic. Logarithmic transformation of data was performed for non-normally distributed data. A Pearson product–moment correlation coefficient was computed to assess the relationship between hsCRP, peak troponin, and IMR of culprit vessels. Binary logistic regression analyses were used to investigate the predictors of severe MVD. Data are presented as odds ratios and 95% CI. p < 0.05 was considered statistically significant.
Results
Study Population
Consecutive patients admitted to hospital for PCI were screened for eligibility and a total of 74 patients were enrolled in the study. There were 36 ACS patients (9 STEMI and 27 NSTEMI) and 38 stable angina patients in the cohort. The study population included 66% smokers, 73% hypertension, 65% hyperlipidemia, 30% diabetes, 42% family history of IHD, and 23% previous acute myocardial infarction (AMI). Coronary angiography was performed via femoral access in 17 (23%) of patients.
Baseline, clinical, and angiographic characteristics of the cohort divided into low CRP (hsCRP < 3 mg/L) and high CRP (hsCRP ≥ 3 mg/L) were summarized in Table 1. There were no clinically significant differences in age, gender and traditional cardiovascular risk factors between the two groups, except history of smoking (52% in low CRP group vs. 78% in high CRP group, p = 0.02) and diabetes (18 vs. 39%, p = 0.048). Mean body mass index was also significantly different between the two groups (27.2 ± 4.1 vs. 29.4 ± 4.6, p = 0.03). All study patients except five STEMI patients were commenced on statin therapy before coronary angiography and physiology assessment. The high CRP group comprised more ACS patients compared with low CRP group (61 vs. 33%, p = 0.02). Target vessels and lesion characteristics were similar between both groups.
Coronary physiological data are presented in Table 2. Mean IMR levels pre- and post-PCI were higher in high CRP cohort (29.0 ± 13.9 vs. 17.4 ± 11.1, p < 0.0001; 23.0 ± 16.8 vs. 15.5 ± 8.4, p = 0.02). All patients had TIMI 2 and TIMI 3 flow pre-PCI and underwent successful PCI with TIMI 3 flow at the conclusion of the procedure. No-reflow phenomenon did not occur post-PCI in any of our study patients. No major procedural and in-hospital complications were observed. There was no significant difference in relation to stent type, size, length, and number of stents deployed per patient.
Table 3 summarizes biochemical results between the two groups. Mean hsCRP level was higher in high CRP group compared to low CRP group (15.1 ± 19.3 vs. 1.4 ± 0.7 mg/L, p < 0.0001). Peak troponin levels were significantly elevated in high CRP group (9.96 ± 17.19 vs. 1.17 ± 3.00 μg/L, p < 0.0001). Serum levels of total cholesterol, low-density lipoprotein cholesterol, glycated hemoglobin (HbA1c), and eGFR were similar between the two groups.
Correlations between IMR, hsCRP, and Cardiac Enzymes
Baseline hsCRP level was positively correlated with pre-PCI culprit vessel IMR (Pearson r = 0.85, p < 0.0001) (Figure 1). This positive correlation was consistent in both ACS and non-ACS populations (r = 0.87 and r = 0.83, respectively, p < 0.0001). hsCRP level also correlated with post-PCI IMR (r = 0.38, p = 0.001) and peak troponin level (r = 0.44, p < 0.0001). Furthermore, there was positive correlation between peak troponin level with pre-PCI IMR (r = 0.45, p < 0.0001), and with post-PCI IMR (r = 0.33, p = 0.005). There were no statistically significant associations between hsCRP with age, gender, traditional cardiovascular risk factors, and angiographic characteristics.
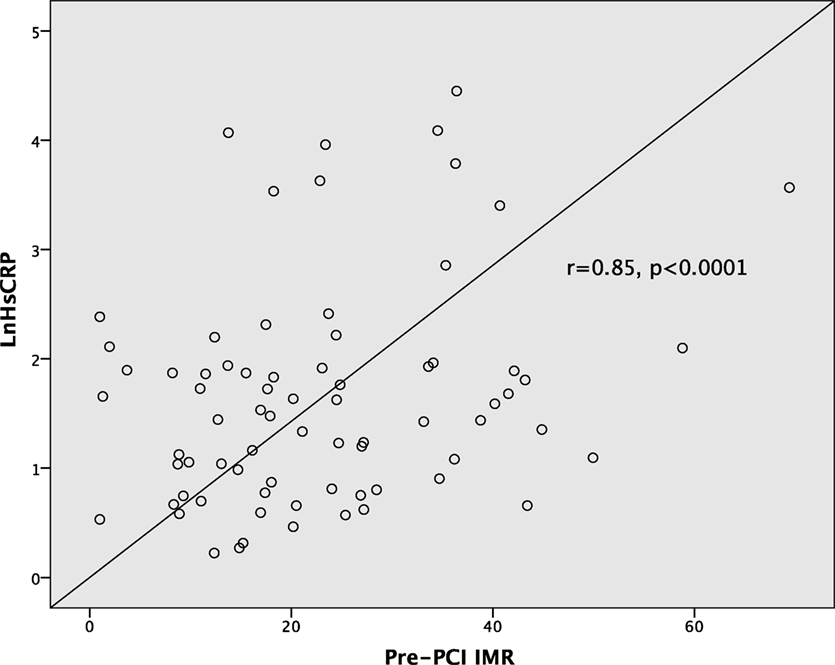
Figure 1. Correlations between high-sensitivity C-reactive protein and pre-percutaneous coronary intervention (PCI) index of microvascular resistance (IMR).
Predictors of Significant MVD
Using IMR ≥ 30 as cutoff threshold, patients with severe MVD (IMR ≥ 30) were compared against patients with normal microvasculature or mild MVD (IMR < 30). hsCRP level was significantly elevated in patients with significant MVD (16.0 ± 22.8 vs. 6.4 ± 11.6 mg/L, p = 0.02). Statistically significant difference in peak troponin levels was also observed between the two groups (12.6 ± 19.0 vs. 2.7 ± 8.2 μg/L, p = 0.003).
Table 4 demonstrates the predictors of severe MVD at baseline (using IMR > 30 as the threshold) by logistic regression analysis. ACS presentation was associated with a 8.5 times increased likelihood of impaired microvascular function while hsCRP level of ≥3 mg/L was associated with 5.6 times increased likelihood of severe pre-PCI MVD. In this study cohort, males were three times more likely to exhibit significant MVD. In addition, both smoking history and diabetes were also significant predictors of severe MVD (OR 4.0, p = 0.006; OR 3.7, p < 0.0001, respectively).
By ROC curve analysis, the optimal cutoff value for hsCRP in predicting significant MVD was 4.3 mg/L (AUC 0.89, 95% CI [0.81, 0.97], p < 0.0001) with a sensitivity of 85% and a specificity of 72% (Figure 2).
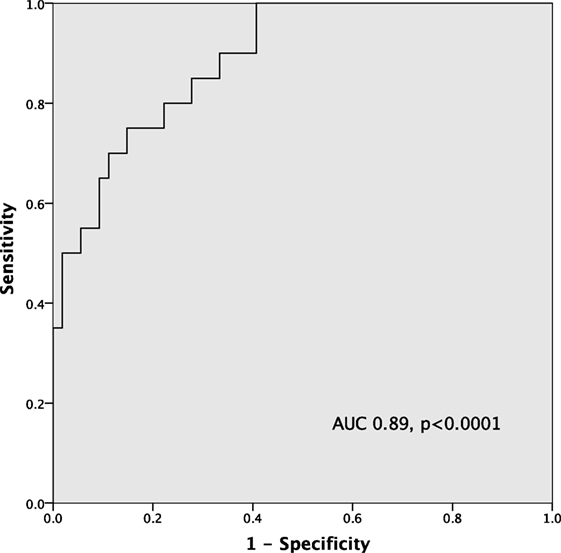
Figure 2. ROC curve of high-sensitivity C-reactive protein (hsCRP) in predicting severe microvascular dysfunction (MVD) (index of microvascular resistance > 30).
Discussion
We demonstrate that hsCRP is strongly correlated with resting coronary microvascular status. Furthermore, both hsCRP and IMR are associated with degree of myocardial injury. Diabetes, smoking history, ACS presentation, and hsCRP ≥3 mg/L are important predictors of significant MVD.
Inflammation and MVD
It is well known that inflammation plays a crucial role across all stages of atherosclerosis including plaque progression, rupture, and thrombotic complications (1), and CRP is deposited in the arterial intima at the sites of atherogenesis (25). Among patients with ACS, inflammation has been shown to be a systemic phenomenon that affects multiple vascular beds (26). In our study, we compared patients with high vs. low resting inflammatory state using hsCRP cutoff value of 3 mg/L as previous studies have consistently shown CRP’s association with adverse cardiovascular events above levels of 3 mg/L (27, 28).
In our study, patients with a heightened inflammatory state exhibited significant MVD at baseline and even after successful PCI. This could represent a “microvascular stunning” phenomenon similar to myocardial stunning, which could be a reversible and transient process driven by systemic inflammation. In fact, one previous study observed recovery of microvascular function on follow-up angiography after recanalization of a chronic total coronary occlusion (29). In addition, MVD could be a result of distal embolization of atherothrombotic debris or release of vasoactive and inflammatory mediators from the atherosclerotic plaques (11, 30, 31).
Several large-scale epidemiological studies show that elevation in markers of inflammation such as hsCRP prognosticates future cardiovascular events and poor outcomes, independent of traditional risk factors and across different populations (32, 33). On the other hand, it is unclear why patients with impaired microvascular function have adverse long-term cardiovascular outcomes (34), even in the absence of significant coronary artery stenosis (14–16). It may be that inflammation is the missing link between microvascular and macrovascular complications of cardiovascular disease.
Inflammation, MVD, and Myocardial Injury
Inflammation predates the clinical manifestation of coronary artery disease and ACS. Numerous studies support the relationship between elevated levels of circulating inflammatory markers and adverse cardiovascular events (2, 35). Moreover, inflammatory response during and after a myocardial infarction plays a crucial role in repair and remodeling of damaged myocardium (36). We demonstrate an association between hsCRP level and peak troponin level (r = 0.44, p < 0.0001), which implies that there is a relationship between inflammation and periprocedural myocardial injury or tissue necrosis. We also show that peak troponin level is correlated with pre-PCI IMR. This observation is consistent with findings from a previous study which showed baseline microvascular function is an important determinant of periprocedural myocardial injury in patients undergoing elective PCI (37).
Inflammation As a Common Link between Epicardial and Microvascular Disease
Studies have shown that MVD is an important predictor of adverse cardiovascular events (11, 14, 15). In congruence with this premise, our study indicates that inflammation could be the missing link between epicardial and microvascular disease. We have demonstrated positive correlations between baseline hsCRP, pre-PCI IMR, and peak troponin. In fact, inflammation may herald MVD, just as it contributes to plaque progression and thrombotic sequelae of ACS.
Both MVD and inflammation share a similar feature, of which their effect is systemic instead of being confined to a single vascular territory. Using positron-emission tomography, Uren et al. measured coronary vasodilator response by administering intravenous dipyridamole at 1 week after single-vessel AMI and reported the presence of MVD not only in infarcted myocardium but also in remote myocardium (18). This finding is further supported by a histopathological study which showed reduced microvasculature density in non-ischemic myocardium in patients who had a recent AMI (17). On the other hand, numerous studies confirmed the presence of widespread inflammation in ACS (38, 39), which extends beyond coronary vascular beds (26).
Clinical Implications
It is important to appreciate the relationship between inflammation and MVD, as both factors are associated with heightened risks of future cardiovascular events and adverse outcomes. High baseline IMR is associated with increased cardiac death in STEMI patients (34) and is a predictor of periprocedural myocardial injury in elective PCI (37). Despite being a non-specific inflammatory marker, hsCRP could potentially be utilized as a surrogate marker to assess microvascular health or be incorporated into current risk prediction models to enhance discriminative value in identifying high-risk patients.
Prospective identification of patients with significant inflammatory milieu or microvascular dysfunction may allow clinicians to tailor the intensity of risk modification strategies. Patients with elevated hsCRP level may be offered intensive statin therapy as statins have been shown to exert pleiotropic effects on improving endothelial and microvascular function (40, 41). Inhibition of renin–angiotensin system should also be considered in high-risk population as it was shown to improve microvascular function (42).
The advent of knowledge in understanding the association between inflammation and MVD will likely give rise to development of novel agents and therapeutic strategies targeting inflammation and MVD. In fact, one such novel therapy include phosphodiesterase 5 inhibitor which has been shown to improve microvascular function in women who presented with ischemic symptoms but without obstructive coronary artery disease (43). Moreover, another recent study involving anti-inflammatory therapy with Canakinumab, a monoclonal antibody targeting interleukin-1β, demonstrated a reduction in recurrent cardiovascular events in patients with previous myocardial infarction and raised CRP but at a cost of more fatal infection (44). Many other anti-inflammatory therapeutic trials are currently underway including colchicine and methotrexate and will hopefully add to our armamentarium to treat cardiovascular disease.
Study Limitations
While we have demonstrated the association between inflammation, MVD, and myocardial injury, this is still a relatively small sample. A strong correlation between CRP and IMR shown in our study is nevertheless not a proof of causation. In addition, CRP is a non-specific marker of inflammation and becomes elevated in a wide range of inflammatory conditions. There is also variability among individuals due to genetic polymorphisms, lifestyle factors, and medical comorbidities (45, 46). In this study, we excluded patients with history of active autoimmune or inflammatory disorders. Although widespread use of CRP to assess cardiovascular risk is not currently advocated, there is assemblage of clinical data to support CRP’s association with cardiovascular events and improving coronary risk prediction (28, 47).
Another study limitation is lack of comprehensive data on the duration and adherence of statin therapy before coronary physiology measurements. The intensity and duration of statin treatment may be confounding factors for the CRP vs. IMR relationship given the pleiotropic effects of statin in modulating inflammatory response and microvascular function (4, 41, 48). Nevertheless, there were no significant differences in the number of patients receiving statin therapy before IMR measurements in low CRP vs. high CRP groups or ACS vs. non-ACS groups.
Conclusion
Inflammation is associated with MVD and myocardial injury and is a predictor of significant MVD. Inflammation may serve as a common link between epicardial and microvascular disease. Future studies are warranted to affirm this association and to establish the potential role of hsCRP measurement in tailoring risk modification strategies to manage cardiovascular disease.
Ethics Statement
The study was carried out in accordance with the recommendations of St Vincent’s Hospital Melbourne Human Research Ethics Committee with written informed consent from all subjects. All subjects gave written informed consent in accordance with the Declaration of Helsinki. The protocol was approved by St Vincent’s Hospital Melbourne Human Research Ethics Committee.
Author Contributions
DT and JL conceived the idea for the study, recruited patients, and wrote the initial draft of the manuscript. All other authors recruited patients and were involved in rewriting later versions of the manuscript.
Conflict of Interest Statement
The authors declare that the research was conducted in the absence of any commercial or financial relationships that could be construed as a potential conflict of interest.
Acknowledgments
We would like to thank the nursing staff in the cardiac catheterization laboratory and coronary care unit for their support.
Funding
The project was supported by Research Endowment Fund of St Vincent’s Hospital Melbourne.
References
1. Libby P, Theroux P. Pathophysiology of coronary artery disease. Circulation (2005) 111:3481–8. doi:10.1161/CIRCULATIONAHA.105.537878
2. Koukkunen H, Penttilä K, Kemppainen A, Halinen M, Penttila I, Rantanen T, et al. C-reactive protein, fibrinogen, interleukin-6 and tumour necrosis factor-alpha in the prognostic classification of unstable angina pectoris. Ann Med (2001) 33:37–47. doi:10.3109/07853890109002058
3. Kavousi M, Elias-Smale S, Rutten JH, Leening MJ, Vliegenthart R, Verwoert GC, et al. Evaluation of newer risk markers for coronary heart disease risk classification: a cohort study. Ann Intern Med (2012) 156:438–44. doi:10.7326/0003-4819-156-6-201203200-00006
4. Ridker PM, Cannon CP, Morrow D, Rifai N, Rose LM, McCabe CH, et al. C-reactive protein levels and outcomes after statin therapy. N Engl J Med (2005) 352:20–8. doi:10.1056/NEJMoa042378
5. Ridker PM, Rifai N, Rose L, Buring JE, Cook NR. Comparison of C-reactive protein and low-density lipoprotein cholesterol levels in the prediction of first cardiovascular events. N Engl J Med (2002) 347:1557–65. doi:10.1056/NEJMoa021993
6. Ridker PM, Cushman M, Stampfer MJ, Tracy RP, Hennekens CH. Inflammation, aspirin, and the risk of cardiovascular disease in apparently healthy men. N Engl J Med (1997) 336:973–9. doi:10.1056/NEJM199704033361401
7. Arad Y, Goodman KJ, Roth M, Newstein D, Guerci AD. Coronary calcification, coronary disease risk factors, C-reactive protein, and atherosclerotic cardiovascular disease events: the St. Francis Heart Study. J Am Coll Cardiol (2005) 46:158–65. doi:10.1016/j.jacc.2005.02.088
8. Emerging Risk Factors CollaborationKaptoge S, Di Angelantonio E, Lowe G, Pepys MB, Thompson SG, et al. C-reactive protein concentration and risk of coronary heart disease, stroke, and mortality: an individual participant meta-analysis. Lancet (2010) 375:132–40. doi:10.1016/S0140-6736(09)61717-7
9. Lloyd-Jones DM, Liu K, Tian L, Greenland P. Narrative review: assessment of C-reactive protein in risk prediction for cardiovascular disease. Ann Intern Med (2006) 145:35–42. doi:10.7326/0003-4819-145-1-200607040-00129
10. Camici PG, Crea F. Coronary microvascular dysfunction. N Engl J Med (2007) 356:830–40. doi:10.1056/NEJMra061889
11. Lerman A, Holmes DR, Herrmann J, Gersh BJ. Microcirculatory dysfunction in ST-elevation myocardial infarction: cause, consequence, or both? Eur Heart J (2007) 28:788–97. doi:10.1093/eurheartj/ehl501
12. Maier W, Altwegg LA, Corti R, Gay S, Hersberger M, Maly FE, et al. Inflammatory markers at the site of ruptured plaque in acute myocardial infarction: locally increased interleukin-6 and serum amyloid A but decreased C-reactive protein. Circulation (2005) 111:1355–61. doi:10.1161/01.CIR.0000158479.58589.0A
13. Kinlay S, Behrendt D, Wainstein M, Beltrame J, Fang JC, Creager MA, et al. Role of endothelin-1 in the active constriction of human atherosclerotic coronary arteries. Circulation (2001) 104:1114–8. doi:10.1161/hc3501.095707
14. Marks DS, Gudapati S, Prisant LM, Weir B, diDonato-Gonzalez C, Waller JL, et al. Mortality in patients with microvascular disease. J Clin Hypertens (Greenwich) (2004) 6:304–9. doi:10.1111/j.1524-6175.2004.03254.x
15. Britten MB, Zeiher AM, Schachinger V. Microvascular dysfunction in angiographically normal or mildly diseased coronary arteries predicts adverse cardiovascular long-term outcome. Coron Artery Dis (2004) 15:259–64. doi:10.1097/01.mca.0000134590.99841.81
16. Pepine CJ, Anderson RD, Sharaf BL, Reis SE, Smith KM, Handberg EM, et al. Coronary microvascular reactivity to adenosine predicts adverse outcome in women evaluated for suspected ischemia results from the National Heart, Lung and Blood Institute WISE (Women’s Ischemia Syndrome Evaluation) study. J Am Coll Cardiol (2010) 55:2825–32. doi:10.1016/j.jacc.2010.01.054
17. Campbell DJ, Somaratne JB, Jenkins AJ, Prior DL, Yii M, Kenny JF, et al. Reduced microvascular density in non-ischemic myocardium of patients with recent non-ST-segment-elevation myocardial infarction. Int J Cardiol (2013) 167:1027–37. doi:10.1016/j.ijcard.2012.03.075
18. Uren NG, Crake T, Lefroy DC, de Silva R, Davies GJ, Maseri A. Reduced coronary vasodilator function in infarcted and normal myocardium after myocardial infarction. N Engl J Med (1994) 331:222–7. doi:10.1056/NEJM199407283310402
19. Fearon WF, Balsam LB, Farouque HM, Caffarelli AD, Robbins RC, Fitzgerald PJ, et al. Novel index for invasively assessing the coronary microcirculation. Circulation (2003) 107:3129–32. doi:10.1161/01.CIR.0000080700.98607.D1
20. Aarnoudse W, Fearon WF, Manoharan G, Geven M, van de Vosse F, Rutten M, et al. Epicardial stenosis severity does not affect minimal microcirculatory resistance. Circulation (2004) 110:2137–42. doi:10.1161/01.CIR.0000143893.18451.0E
21. Meier B, Luethy P, Finci L, Steffenino GD, Rutishauser W. Coronary wedge pressure in relation to spontaneously visible and recruitable collaterals. Circulation (1987) 75:906–13. doi:10.1161/01.CIR.75.5.906
22. Baumgart D, Haude M, Liu F, Ge J, Goerge G, Erbel R. Current concepts of coronary flow reserve for clinical decision making during cardiac catheterization. Am Heart J (1998) 136:136–49. doi:10.1016/S0002-8703(98)70194-2
23. Solberg OG, Ragnarsson A, Kvarsnes A, Endresen K, Kongsgård E, Aakhus S, et al. Reference interval for the index of coronary microvascular resistance. EuroIntervention (2014) 9:1069–75. doi:10.4244/EIJV9I9A181
24. Luo C, Long M, Hu X, Huang Z, Hu C, Gao X, et al. Thermodilution-derived coronary microvascular resistance and flow reserve in patients with cardiac syndrome X. Circ Cardiovasc Interv (2014) 7:43–8. doi:10.1161/CIRCINTERVENTIONS.113.000953
25. Torzewski M, Rist C, Mortensen RF, Zwaka TP, Bienek M, Waltenberger J, et al. C-reactive protein in the arterial intima: role of C-reactive protein receptor-dependent monocyte recruitment in atherogenesis. Arterioscler Thromb Vasc Biol (2000) 20:2094–9. doi:10.1161/01.ATV.20.9.2094
26. Lombardo A, Rizzello V, Natale L, Lombardi M, Coli S, Snider F, et al. Magnetic resonance imaging of carotid plaque inflammation in acute coronary syndromes: a sign of multisite plaque activation. Int J Cardiol (2009) 136:103–5. doi:10.1016/j.ijcard.2008.03.077
27. Möhlenkamp S, Lehmann N, Moebus S, Schmermund A, Dragano N, Stang A, et al. Quantification of coronary atherosclerosis and inflammation to predict coronary events and all-cause mortality. J Am Coll Cardiol (2011) 57:1455–64. doi:10.1016/j.jacc.2010.10.043
28. Park R, Detrano R, Xiang M, Fu P, Ibrahim Y, LaBree L, et al. Combined use of computed tomography coronary calcium scores and C-reactive protein levels in predicting cardiovascular events in nondiabetic individuals. Circulation (2002) 106:2073–7. doi:10.1161/01.CIR.0000033819.29662.09
29. Werner GS, Emig U, Bahrmann P, Ferrari M, Figulla HR. Recovery of impaired microvascular function in collateral dependent myocardium after recanalisation of a chronic total coronary occlusion. Heart (2004) 90:1303–9. doi:10.1136/hrt.2003.024620
30. Herrmann J, Lerman A. The endothelium: dysfunction and beyond. J Nucl Cardiol (2001) 8:197–206. doi:10.1067/mnc.2001.114148
31. Bonderman D, Teml A, Jakowitsch J, Adlbrecht C, Gyöngyösi M, Sperker W, et al. Coronary no-reflow is caused by shedding of active tissue factor from dissected atherosclerotic plaque. Blood (2002) 99:2794–800. doi:10.1182/blood.V99.8.2794
32. Ridker PM. High-sensitivity C-reactive protein: potential adjunct for global risk assessment in the primary prevention of cardiovascular disease. Circulation (2001) 103:1813–8. doi:10.1161/01.CIR.103.13.1813
33. Libby P, Ridker PM, Maseri A. Inflammation and atherosclerosis. Circulation (2002) 105:1135–43. doi:10.1161/hc0902.104353
34. Fearon WF, Low AF, Yong AS, McGeoch R, Berry C, Shah MG, et al. Prognostic value of the index of microcirculatory resistance measured after primary percutaneous coronary intervention. Circulation (2013) 127:2436–41. doi:10.1161/CIRCULATIONAHA.112.000298
35. Morrow DA, Rifai N, Antman EM, Weiner DL, McCabe CH, Cannon CP, et al. Serum amyloid A predicts early mortality in acute coronary syndromes: a TIMI 11A substudy. J Am Coll Cardiol (2000) 35:358–62. doi:10.1016/S0735-1097(99)00574-4
36. Frangogiannis NG. The inflammatory response in myocardial injury, repair, and remodelling. Nat Rev Cardiol (2014) 11:255–65. doi:10.1038/nrcardio.2014.28
37. Layland JJ, Whitbourn RJ, Burns AT, Somaratne J, Leitl G, Macisaac AI, et al. The index of microvascular resistance identifies patients with periprocedural myocardial infarction in elective percutaneous coronary intervention. Heart (2012) 98:1492–7. doi:10.1136/heartjnl-2012-302252
38. Mauriello A, Sangiorgi G, Fratoni S, Palmieri G, Bonanno E, Anemona L, et al. Diffuse and active inflammation occurs in both vulnerable and stable plaques of the entire coronary tree: a histopathologic study of patients dying of acute myocardial infarction. J Am Coll Cardiol (2005) 45:1585–93. doi:10.1016/j.jacc.2005.01.054
39. Buffon A, Biasucci LM, Liuzzo G, D’Onofrio G, Crea F, Maseri A. Widespread coronary inflammation in unstable angina. N Engl J Med (2002) 347:5–12. doi:10.1056/NEJMoa012295
40. Ishida K, Geshi T, Nakano A, Uzui H, Mitsuke Y, Okazawa H, et al. Beneficial effects of statin treatment on coronary microvascular dysfunction and left ventricular remodeling in patients with acute myocardial infarction. Int J Cardiol (2012) 155:442–7. doi:10.1016/j.ijcard.2011.11.015
41. Eshtehardi P, McDaniel MC, Dhawan SS, Binongo JN, Krishnan SK, Golub L, et al. Effect of intensive atorvastatin therapy on coronary atherosclerosis progression, composition, arterial remodeling, and microvascular function. J Invasive Cardiol (2012) 24:522–9.
42. Pauly DF, Johnson BD, Anderson RD, Handberg EM, Smith KM, Cooper-DeHoff RM, et al. In women with symptoms of cardiac ischemia, nonobstructive coronary arteries, and microvascular dysfunction, angiotensin-converting enzyme inhibition is associated with improved microvascular function: a double-blind randomized study from the National Heart, Lung and Blood Institute Women’s Ischemia Syndrome Evaluation (WISE). Am Heart J (2011) 162:678–84. doi:10.1016/j.ahj.2011.07.011
43. Denardo SJ, Wen X, Handberg EM, Bairey Merz CN, Sopko GS, Cooper-Dehoff RM, et al. Effect of phosphodiesterase type 5 inhibition on microvascular coronary dysfunction in women: a Women’s Ischemia Syndrome Evaluation (WISE) ancillary study. Clin Cardiol (2011) 34:483–7. doi:10.1002/clc.20935
44. Ridker PM, Everett BM, Thuren T, MacFadyen JG, Chang WH, Ballantyne C, et al. Antiinflammatory therapy with canakinumab for atherosclerotic disease. N Engl J Med (2017) 377(12):1119–31. doi:10.1056/NEJMoa1707914
45. Woloshin S, Schwartz LM. Distribution of C-reactive protein values in the United States. N Engl J Med (2005) 352:1611–3. doi:10.1056/NEJM200504143521525
46. Pankow JS, Folsom AR, Cushman M, Borecki IB, Hopkins PN, Eckfeldt JH, et al. Familial and genetic determinants of systemic markers of inflammation: the NHLBI family heart study. Atherosclerosis (2001) 154:681–9. doi:10.1016/S0021-9150(00)00586-4
47. Buckley DI, Fu R, Freeman M, Rogers K, Helfand M. C-reactive protein as a risk factor for coronary heart disease: a systematic review and meta-analyses for the U.S. Preventive Services Task Force. Ann Intern Med (2009) 151:483–95. doi:10.7326/0003-4819-151-7-200910060-00009
Keywords: inflammation, C-reactive protein, microvascular dysfunction, myocardial injury, ischemic heart disease, index of microvascular resistance
Citation: Tong DC, Whitbourn R, MacIsaac A, Wilson A, Burns A, Palmer S and Layland J (2018) High-Sensitivity C-Reactive Protein Is a Predictor of Coronary Microvascular Dysfunction in Patients with Ischemic Heart Disease. Front. Cardiovasc. Med. 4:81. doi: 10.3389/fcvm.2017.00081
Received: 04 October 2017; Accepted: 04 December 2017;
Published: 12 January 2018
Edited by:
Jun-ichi Abe, University of Texas MD Anderson Cancer Center, United StatesReviewed by:
Xinchun Pi, Baylor College of Medicine, United StatesScott James Cameron, University of Rochester, United States
Dennis W. T. Nilsen, Stavanger University Hospital, Norway
Copyright: © 2018 Tong, Whitbourn, MacIsaac, Wilson, Burns, Palmer and Layland. This is an open-access article distributed under the terms of the Creative Commons Attribution License (CC BY). The use, distribution or reproduction in other forums is permitted, provided the original author(s) or licensor are credited and that the original publication in this journal is cited, in accordance with accepted academic practice. No use, distribution or reproduction is permitted which does not comply with these terms.
*Correspondence: Jamie Layland, SkxheWxhbmRAcGhjbi52aWMuZ292LmF1