- 1Faculty of Pharmacy and Pharmaceutical Sciences, University of Alberta, Edmonton, AB, Canada
- 2Cardiovascular Research Centre, University of Alberta, Edmonton, AB, Canada
- 3Department of Pharmacology, Faculty of Medicine and Dentistry, University of Alberta, Edmonton, AB, Canada
- 4Mazankowski Alberta Heart Institute, University of Alberta, Edmonton, AB, Canada
Although lacking a nucleus, platelets are increasingly recognized not only for their complexity, but also for their diversity. Some 50 years ago platelet subpopulations were characterized by size and density, and these characteristics were thought to reflect platelet aging. Since, our knowledge of platelet heterogeneity has grown to recognize that differences in platelet biochemistry and function exist. This includes the identification of vanguard and follower platelets, platelets with differing procoagulant ability including “COAT-platelets” which enhance procoagulant protein retention on their surface, and most recently, the identification of platelet subpopulations with a differential ability to generate and respond to nitric oxide. Hence, in this mini-review, we summarize the current knowledge of platelet subpopulation diversity focusing on their physical, biochemical, and functional heterogeneity. In addition, we review how platelet subpopulations may change between health and disease and how differences among platelets may influence response to anti-platelet therapy. Finally, we look forward and discuss some of the future directions and challenges for this growing field of platelet research.
Introduction
Compared to leukocytes, which exist as functionally distinct subpopulations, platelets have often been considered simple. This general apathy for platelet complexity may stem from the fact that platelets lack nuclei and because they are so widely recognized for their roles in hemostasis and thrombosis. However, increasingly platelets are recognized for their biochemical and functional complexity despite being anucleate. Platelet proteome and transcriptome studies have revealed that platelets contain nearly 4,000 different proteins (1, 2) and an abundance of mRNA (3, 4) and miRNA (5–7). It's also appreciated within the field that platelets play diverse roles beyond hemostasis including contributing to wound healing, angiogenesis, and immunity and inflammation (8–11). Pathophysiologically, they are also recognized to contribute to cancer metastasis (12, 13), as well as to various inflammatory disorders (14, 15). To fulfill these diverse roles the question arises whether there is predetermined biochemical diversity and functional heterogeneity among platelets? That is, do platelets subpopulations with predetermined specialized functions exist?
Historical Perspectives
Initial studies into platelet subpopulations occurred 50 years ago and focused on characterizing human platelet subpopulations based on size and buoyant density and largely isolated these subpopulations by density gradient centrifugation. Studies by Karpatkin (16) showed that large, dense platelets have greater glycogen, orthophosphate and adenine nucleotide content than smaller less dense platelets. The biochemical processes of glycolysis, glycogenolysis, and protein synthesis were reported to be greater in large-dense platelets but no differences were observed in lipid content or synthesis. Large-dense platelets were shown to aggregate more than small-light platelets largely due to greater ADP release and lower ADPase activity (17). Subsequently, large-dense platelets were shown to adhere to collagen at a faster rate and this was thought to reflect more surface GPIa/IIa (integrin α2β1) on this subpopulation (18). Electron microscopy examination of light platelets demonstrated lower granule content than that of dense platelets, but not fewer mitochondria (19). Although, currently it's recognized that an anuclear programmed cell death limits platelet lifespan (20, 21), the biochemical, ultrastructural, and functional differences among platelets with differing size and density were thought to reflect platelet aging with large-heavy platelets representing young platelets recently released into the circulation while light-small platelets represented older platelets that have circulated for a number of days. Using rabbit platelets, Rand et al. (22) demonstrated that the least dense platelets contained less sialic acid than the densest. They suggested loss of surface sialic acid from the least dense platelets was a mechanism by which old platelets are recognized and removed from the circulation.
Studies by Penington et al. (23, 24) argued that in fact megakaryocycte heterogeneity was responsible for platelet heterogeneity and that platelet density does not change with aging. Based on morphometric studies, they contended that the three-ploidy classes of megakaryocytes (8n, 16n, 32n) differ in their organelle content concentration and relate to the density of their platelet progeny. Large-dense platelets would be expected to arise from 8n megakaryocytes with greater granule content, while small-light platelets arise from 32n megakaryocytes. This view was more in line with that of Paulus that thrombopoiesis is likely responsible for platelet heterogeneity and not aging in circulation, but that only a single platelet population exists and the only size heterogeneity is that inherent to the log normally distributed population (25). Consistent with this thesis, other studies demonstrated that in the steady state platelet density does not change with circulatory age (26, 27), while others still showed that platelet density may increase with time due to accumulation of 5-hydroxytryptamine (5-HT) (28).
In addition to categorizing platelets into subpopulations based on size and density, platelets were also separated into subpopulations based on volume using counterflow centrifugation. Platelets with larger volume were shown to have more rapid and complete aggregation, and to be slightly denser (29). These studies argued that fundamentally platelets of different volume have similar function but the absolute abilities to aggregate and secrete granular contents correlates with their volume (30). Further, it was argued that platelet size heterogeneity likely results from their production from megakaryocytes and that this influences platelet function independently of time within circulation (30, 31).
Several groups attempted to unify the hypotheses that both megakaryocyte heterogeneity and platelet time in circulation may contribute to platelet heterogeneity and the formation of size and density-based subpopulations (32–34). However, it's also important to note that some discrepancies in characterizing size and density-based platelet subpopulations may have occurred due to species differences. It appears rabbit platelets decrease in density with circulatory age, while in humans high-density platelets may be enriched with those that may have circulated longer and accumulated more 5-HT (28). Other characterization discrepancies may have also occurred due to methodology, and theoretical aspects of density separation need to be considered when interpreting early platelet subpopulation studies. As explained by Martin and Trowbridge if centrifugation is stopped before equilibrium is reached platelets may be separated based on a mixture of volume and density variation (35). This problem appears to be greater for discontinuous vs. continuous gradients as a greater time is needed to reach equilibrium necessary for separation of density-based subpopulations. Further, it has been suggested that platelets held at equilibrium between the opposing forces of the gravitational field and medium buoyancy may cause trauma to the platelet potentially causing secretion of granular contents; thus, potentially changing the characteristics of the isolated subpopulations (35).
Characterization of Platelet Subpopulations Based on Differential Function and Biochemistry
In addition to being characterized by size and density, platelet subpopulations have also been characterized based on function. Using a biotinylation technique to label dog platelets in vivo, it was demonstrated that reactivity to thrombin declines with platelet circulatory age (36). Similarly, utilizing 35S-labeled rabbit platelets Hirsh et al. (37) demonstrated that younger platelets with lower 35S-specific activity adhered to collagen fibers more readily than older platelets. Alike, two human platelet subpopulations were shown to have different collagen adhering kinetics, with 20% of platelets adhering within 1 min and with a second larger subpopulation accounting for 80% of platelets adhering more slowly between 1 and 60 min (38). The biochemical basis of this finding was unknown; however, several explanations were proposed. The one favored was the existence of functionally discrete subpopulations due to the sharp discontinuity between the two adhesion phases and the simple kinetic behavior of the second phase. Using time-lapse videomicroscopy and a microchamber model, Patel et al. (39) defined platelets that first adhered and spread on collagen as “vanguard” platelets and those that subsequently tether to and spread on vanguard platelets or nearby collagen as “followers.” Whether vanguard and follower platelets represent functionally and biochemically distinct platelets was not investigated, but analysis of platelet deposition showed that adhesion events occurred randomly.
In addition to characterizing based on function, some studies characterized platelet subpopulations based on biochemical differences including the ability to bind adenosine and release 5-HT (40), as well as the presence or absence of acid phosphatase although no functional differences were observed (41). However, biochemical comparison of low vs. high density human platelets by Opper et al. (42) revealed that low density platelets have an enhanced intracellular Ca2+ response to thrombin, increased ADP-ribosylation of the inhibitory G-protein (Giα1−3) and rho A, and decreased ADP-ribosylation of the stimulatory G-protein (Gsα), as well as lower levels of the phosphorylated form of vasodilator-stimulated protein compared to high density platelets. These biochemical differences likely further explain the authors' findings that low density platelets demonstrate enhanced aggregation in response to thrombin, weaker inhibitory effects and a smaller rise in cGMP to the nitric oxide (NO) donor sodium nitroprusside, and a smaller increase in cAMP in response to prostaglandin E1 compared to high density platelets (43, 44). The authors proposed that the biochemical basis of the functional heterogeneity between low and high density platelets depends on differences in their G- and phospho-protein signaling within stimulatory and inhibitory signaling pathways (42). Others suggested that the increased reactivity of low density platelets may be attributed in part due to elevated α-granule content (45), although another study suggested α-granule content is greater in high density platelets (46).
Similar to the findings of Opper et al. (42), which suggested differences in NO-signaling between low and high density platelet subpopulations, recently we identified human platelet subpopulations based on the presence or absence of endothelial nitric oxide synthase (eNOS-positive and -negative platelets) and the differential ability to produce NO (47). We showed that eNOS-negative platelets fail to produce NO, have a down-regulated soluble guanylate cyclase-protein kinase G-vasodilator-stimulated protein (sGC-PKG-VASP) signaling pathway, primarily initiate adhesion to collagen, more readily activate integrin αIIbβ3, and secrete more of the platelet activating protease matrix metalloproteinase-2 (MMP-2) than their eNOS-positive counterparts. eNOS-positive platelets have an intact eNOS-sGC-PKG-VASP signaling pathway, are more abundant (~80% of total platelets) and form the bulk of an aggregate via greater cyclooxygenase-1-mediated signaling. However, eNOS-positive platelets ultimately limit aggregate size via NO generation. It appears that eNOS-based platelet subpopulations are of the same circulatory age as the levels of activated caspase-3, the downstream effector of the platelet internal apoptotic clock (21), within them are equal. Moreover, eNOS-negative and –positive platelet volume was found to be equal also (47).
Based on these data, we proposed a novel model of thrombosis and hemostasis called the seed platelet hypothesis, in which eNOS-negative platelets initiate adhesion and aggregation reactions (Figure 1) (47). This initial response arises from their enhanced adhesiveness and reactivity due to an absence of endogenous NO generation (48, 49). Decreased sGC-PKG signaling within eNOS-negative platelets also facilitates refractoriness to endothelial-derived NO and increases integrin αIIbβ3 activation (50, 51), which stabilizes initial rolling and adhesion (52). We further proposed that enhanced MMP-2 secretion by eNOS-negative platelets promotes eNOS-negative platelet activation and recruitment of eNOS-positive platelets to the forming aggregate (53–55). eNOS-positive platelets then form the bulk of an aggregate/thrombus due to their higher COX-1 content and greater thromboxane A2 generation. However, eNOS-positive platelets ultimately limit aggregate/thrombus size via NO generation as both increasing the ratio of eNOSneg to eNOSpos platelets and pharmacologically inhibiting eNOS enhances aggregation.
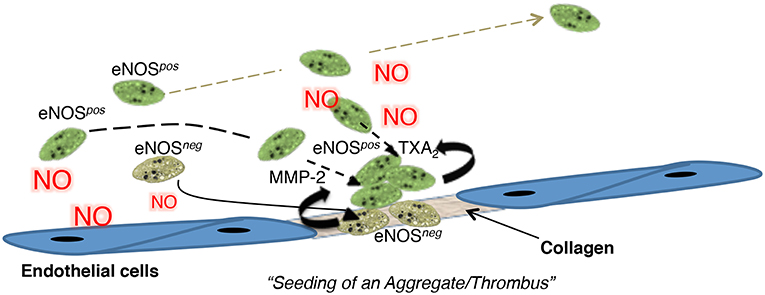
Figure 1. A cartoon summarizing the “seed platelet” hypothesis in which NO-refractory eNOSneg platelets preferentially initiate platelet adhesion and aggregation, while eNOSpos platelets form the bulk of an aggregate and limit its size. Figure S21. Radziwon-Balicka et al. (47), by permission of Oxford University Press. MMP-2, matrix metalloproteinase-2; NO, nitric oxide, TXA2, Thromboxane A2.
The differential ability of platelets to generate NO has been shown by others (56). However, how eNOS-based platelet subpopulations and the seed-platelet model of thrombosis and hemostasis relate to other models, such as a core and shell model of hemostatic plug formation by Stalker et al. (57), still needs to be determined. Similarly, what role these subpopulations play in coagulation and how they relate to subpopulations with differential procoagulant ability also remains to be seen (58).
Characterization of Platelet Subpopulations Based on Differential Procoagulant Ability
For a thorough review of procoagulant platelets we refer readers to a paper by Reddy and Rand within this series of articles on the Established and Novel Roles of Platelets in Health and Disease. However, briefly, it has been long recognized that a subpopulation of platelets becomes procoagulant exposing a phosphatidylserine-(PS)-surface upon activation (59). Alberio et al. (60) described a platelet subpopulation demonstrating α-granule-derived surface-bound factor V (FV), which they named COAT-platelets, due to their appearance upon collagen and thrombin stimulation. This subpopulation accounts for approximately 30% of platelets and was shown to utilize 5-HT to retain other α-granule-derived procoagulant proteins such fibrinogen and thrombospondin on the platelet surface (58, 61). COAT-platelets may also retain factors VIII, IX, and X (62), and they may be generated via stimulation of platelets with thrombin alone (63). These platelets are also sometimes referred to as “coat” or “coated” platelets due to their surface retention of procoagulant proteins. Additionally, heterogeneity within the procoagulant subpopulation itself may exist wherein procoagulant platelets that are highly PS-positive and have a sustained increase in Ca2+-signaling surprisingly aggregate poorly, due in part to a lack of active integrin αIIbβ3. Conversely, a COAT-platelet subpopulation with lower intracellular Ca2+-signaling exhibits greater proaggregatory potential (64). Using mathematical modeling to support their experimental observations, Yakimenko Alena et al. (65) and Abaeva et al. (66) suggested that highly PS-positive COAT-platelets are recruited into developing aggregates by non-COAT platelets, as a result of a high surface density of α-granule-derived fibrinogen/fibrin retained on the COAT-platelet surface, and largely do not bind each other. Others have demonstrated that in fact integrin αIIbβ3 may initially activate but subsequently deactivate, while PS surface exposure occurs more slowly in these COAT-platelets (67). Nonetheless, a model of platelet-based coagulation was proposed by Heemskerk et al. (68) wherein two different subpopulations of platelets with differential roles exist (procoagulant vs. aggregating platelets). Collagen-adhered platelets, and later in the growing thrombus thrombin-activated platelets, expose PS on their membranes serving as a substrate for coagulation factors, thrombin generation, and fibrin coat formation. Aggregating platelets, due to their activated integrin αIIbβ3, on the other hand are proposed to be responsible for contracting and retracting the clot by interacting with fibrin.
Whether all platelets have the capacity to become procoagulant/COAT vs. aggregating platelet subpopulations or whether these subpopulations are predetermined requires further investigation. Studying platelet adhesion to glass some have argued that all platelets can form these subpopulations and that they simply reflect snapshots in time of a dynamic platelet activation process (69). However, multi-parameter flow cytometry studies of platelet responses to increasing concentrations of thrombin and CRP-XL showed that only a fraction of platelets can take on the procoagulant phenotype supporting the theory of distinct platelet subpopulations (70).
Changes in Platelet Subpopulations Between Health and Disease
Relatively little is known about how platelet subpopulations change between physiological and pathological conditions, although recently a number of studies have investigated procoagulant/COAT“ed” platelet levels in stroke and transient ischemic attack (71–78). Coated-platelets were demonstrated to be elevated in patients with large-artery atherosclerotic stroke compared to small artery lacunar strokes with the authors suggesting this reflects distinct pathological processes of ischemic stroke subtypes (71). Similarly, elevated coated-platelet levels were reported during transient ischemic attacks (TIA) (79). High coated-platelet levels were also reported to be associated with early stroke recurrence in large-artery stroke patients (72, 74); and in patients with asymptomatic carotid artery stenosis high coat-platelet levels (≥45% of platelets) improved stroke and TIA prediction (75). Conversely, a pilot study reported that non-lacunar stroke patients with early haemorrhagic transformation exhibited lower coated-platelet levels (78). Likewise, among subarachnoid hemorrhage and spontaneous intracerebral hemorrhage patients low coated-platelet levels were associated with increased mortality at 1-month (76, 77). The association of low levels of coated-platelets with bleeding events was also noted in a study aimed at evaluating the diagnostic utility of platelet flow cytometry analysis in haemorrhagic diathesis patients with normal standard laboratory workup (80).
Interestingly, a recent study utilizing a microfabricated chip capable of measuring individual platelet contractile forces identified platelet subpopulations with varying contractility (81). This platelet-contraction cytometry revealed generally high levels of highly-contractile platelets in healthy donors. These were absent in patients with Wiskott-Aldrich syndrome, and a subpopulation of platelets with low-contractility was also noted among a subset of patients with chronic bleeding but normal clinical haemostasis tests. Whether subpopulations of high- and low-contractile platelets correspond to subpopulations of coated- or aggregating-platelet subpopulations remains to be studied.
Older studies characterizing platelets based on buoyant density and/or volume also demonstrated differences between platelets of healthy controls and patients. Using continuous gradients of Percoll to isolate platelets, it was shown that compared to healthy controls insulin-dependent diabetics with poor glycemic control have lower density platelets but with apparently normal granule levels (82). The change in density profiles was proposed to be due to abnormal platelet subpopulations, although what appeared to be log normally distributed populations were observed (83). A shift toward an increase in low-density platelet subpopulations was also observed in patients with hypercholesterolemia and it was argued that these platelets are more reactive in response to agonist stimulation (83). Conversely, a small increase in platelet size with increased surface exposure of integrin αIIbβ3 was noted following acute myocardial infarction (84).
Interestingly, recent preliminary studies suggest that FACS separated platelets into the smallest and largest 10% may differ in some of their RNA transcripts (85). A sub-analysis of the RNA profiles of these platelet subpopulations demonstrated a trend in which the large-platelet subpopulation RNA profile was associated with hemostasis and wound healing, while the small-platelet subpopulation profile was associated with vascular cell function likely reflecting a potential differential ability to uptake RNA from vascular cells. The differential uptake of RNA by platelet subpopulations may allow for these subpopulations to serve as novel biomarkers for various diseases. In this context, tumor-educated platelets (platelets containing tumor-associated mRNA) have been identified as a potential platform for blood-based liquid biopsies for cancer (86, 87). How platelet subpopulations change in cancer and how these changes impact their mRNA content is unknown but likely of important significance to liquid biopsy utility.
Differential Responses of Platelet Subpopulations to Anti-platelet Drugs
Less is still known about how various platelet subpopulations respond to anti-platelet drugs. Using counterflow centrifugation to isolate platelet subpopulations of different volume, Jakubowski et al. (88) demonstrated a correlation between increasing mean platelet volume and prostacyclin concentration necessary to inhibit aggregation suggesting greater platelet mass is associated with decreased inhibitory effect of prostacyclin. Similar, a study of FACS-sorted platelets into the 20% smallest and largest subpopulations showed that following incubation with acetylsalicylic acid (ASA) large platelets generate more thromboxane B2 (TXB2) compared to the small platelet subpopulation (89). However, the percent reduction in TXB2 generation caused by ASA tended to be greater in the small platelet subpopulation. Potential differences in response to anti-platelet therapy by platelet subpopulations may be clinically important as Hoefer et al. (90) have shown that subpopulations of drug-free/uninhibited platelets can either intermingle with ASA-inhibited platelets within an aggregate or form aggregate cores around which ASA- and P2Y12-inhibited platelets may activate potentially seeding thrombus formation.
Challenges and Future Directions
Some of the challenges with studying platelet subpopulations are their identification, labeling and separating for functional studies. In the past these challenges were met with advances in research methodology and technology, such as the application of flow cytometry to platelet studies (91, 92). Recent advances in cytometry (Table 1) that have enhanced our ability to study platelet subpopulations include that application of fluorescence activated cell sorting and confocal microscopy (47), laser scanning cytometry (93), interfacial platelet cytometry (94), platelet-contraction cytometry (81), and mass cytometry (95). Moreover, the application of flowRNA technology to platelets studies may further help with RNA profiling of platelet subpopulations (96). These technologies will not only be crucial in aiding to delineate the roles of various platelet subpopulations in hemostasis and thrombosis, but also to understanding their potential differing roles in wound healing, angiogenesis, malignancy, and immunity and inflammation. Lastly, isolation of functionally distinct platelet subpopulations may be desirable for platelet concentrate preparation and various transfusion medicine applications (97, 98).
Author Contributions
GL and PJ contributed to manuscript generation, editing, and approval of the submitted manuscript.
Funding
This work was funded by grants from the Canadian Institutes of Health Research (MOP-162128) to PJ.
Conflict of Interest Statement
The authors declare that the research was conducted in the absence of any commercial or financial relationships that could be construed as a potential conflict of interest.
Acknowledgments
We thank Dr. M. Rand for discussions and critical review of our manuscript.
References
1. Burkhart JM, Vaudel M, Gambaryan S, Radau S, Walter U, Martens L, et al. The first comprehensive and quantitative analysis of human platelet protein composition allows the comparative analysis of structural and functional pathways. Blood. (2012) 120:e73–82. doi: 10.1182/blood-2012-04-416594
2. Senis YA, Tomlinson MG, García Á, Dumon S, Heath VL, Herbert J, et al. A comprehensive proteomics and genomics analysis reveals novel transmembrane proteins in human platelets and mouse megakaryocytes including G6b-B, a novel immunoreceptor tyrosine-based inhibitory motif protein. Mol Cell Proteomics. (2007) 6:548–64. doi: 10.1074/mcp.D600007-MCP200
3. Gnatenko DV, Dunn JJ, McCorkle SR, Weissmann D, Perrotta PL, Bahou WF. Transcript profiling of human platelets using microarray and serial analysis of gene expression. Blood. (2003) 101:2285. doi: 10.1182/blood-2002-09-2797
4. Rowley JW, Oler AJ, Tolley ND, Hunter BN, Low EN, Nix DA, et al. Genome-wide RNA-seq analysis of human and mouse platelet transcriptomes. Blood. (2011) 118:e101. doi: 10.1182/blood-2011-03-339705
5. Landry P, Plante I, Ouellet DL, Perron MP, Rousseau G, Provost P. Existence of a microRNA pathway in anucleate platelets. Nat Struct Mol Biol. (2009) 16:961–6. doi: 10.1038/nsmb.1651
6. Sunderland N, Skroblin P, Barwari T, Huntley Rachael P, Lu R, Joshi A, et al. MicroRNA biomarkers and platelet reactivity. Circ Res. (2017) 120:418–35. doi: 10.1161/CIRCRESAHA.116.309303
7. McManus DD, Freedman JE. MicroRNAs in platelet function and cardiovascular disease. Nat Rev Cardiol. (2015) 12:711. doi: 10.1038/nrcardio.2015.101
8. Golebiewska EM, Poole AW. Platelet secretion: from haemostasis to wound healing and beyond. Blood Rev. (2015) 29:153–62. doi: 10.1016/j.blre.2014.10.003
9. Nurden AT. The biology of the platelet with special reference to inflammation, wound healing and immunity. Front Biosci Landmark Ed. (2018) 23:726–51. doi: 10.2741/4613
10. Radziwon-Balicka A, Moncada de la Rosa C, Jurasz P. Platelet-associated angiogenesis regulating factors: a pharmacological perspective. Can J Physiol Pharmacol. (2012) 90:679–88. doi: 10.1139/y2012-036
11. Jenne CN, Kubes P. Platelets in inflammation and infection. Platelets. (2015) 26:286–92. doi: 10.3109/09537104.2015.1010441
12. Yan M, Jurasz P. The role of platelets in the tumor microenvironment: from solid tumors to leukemia. Biochim Biophys Acta (BBA) Mol Cell Res. (2016) 1863:392–400. doi: 10.1016/j.bbamcr.2015.07.008
13. Menter DG, Kopetz S, Hawk E, Sood AK, Loree JM, Gresele P, et al. Platelet “first responders” in wound response, cancer, and metastasis. Cancer Metastasis Rev. (2017) 36:199–213. doi: 10.1007/s10555-017-9682-0
14. Thachil J. Platelets in inflammatory disorders: a pathophysiological and clinical perspective. Semin Thromb Hemost. (2015) 41:572–81. doi: 10.1055/s-0035-1556589
15. Middleton EA, Weyrich AS, Zimmerman GA. Platelets in pulmonary immune responses and inflammatory lung diseases. Physiol Rev. (2016) 96:1211–59. doi: 10.1152/physrev.00038.2015
16. Karpatkin S. Heterogeneity of human platelets. I. Metabolic and kinetic evidence suggestive of young and old platelets. J Clin Invest. (1969) 48:1073–82. doi: 10.1172/JCI106063
17. Karpatkin S. Heterogeneity of human platelets. II. Functional evidence suggestive of young and old platelets. J Clin Invest. (1969) 48:1083–7. doi: 10.1172/JCI106064
18. Polanowska-Grabowska R, Raha S, Gear ARL. Adhesion efficiency, platelet density and size. Br J Haematol. (1992) 82:715–20. doi: 10.1111/j.1365-2141.1992.tb06949.x
19. Corash L, Tan H, Gralnick HR. Heterogeneity of human whole blood platelet subpopulations. I. Relationship between buoyant density, cell volume, and ultrastructure. Blood. (1977) 49:71.
20. Dowling MR, Josefsson EC, Henley KJ, Hodgkin PD, Kile BT. Platelet senescence is regulated by an internal timer, not damage inflicted by hits. Blood. (2010) 116:1776–8. doi: 10.1182/blood-2009-12-259663
21. Mason KD, Carpinelli MR, Fletcher JI, Collinge JE, Hilton AA, Ellis S, et al. Programmed anuclear cell death delimits platelet life span. Cell. (2007) 128:1173–86. doi: 10.1016/j.cell.2007.01.037
22. Rand ML, Greenberg JP, Packham MA, Mustard JF. Density subpopulations of rabbit platelets: size, protein, and sialic acid content, and specific radioactivity changes following labeling with 35S-sulfate in vivo. Blood. (1981) 57:741–6.
23. Penington DG, Streatfield K, Roxburgh AE. Megakaryocytes and the heterogeneity of circulating platelets. Br J Haematol. (1976) 34:639–53. doi: 10.1111/j.1365-2141.1976.tb03611.x
24. Penington DG, Lee NLY, Roxburgh AE, McGready JR. Platelet density and size: the interpretation of heterogeneity. Br J Haematol. (1976) 34:365–76. doi: 10.1111/j.1365-2141.1976.tb03583.x
26. Boneu B, Boneu A, Raisson Cl, Guiraud R, Bierme R. Kinetics of platelet populations in the statinary state. Thromb Res. (1973) 3:605–11. doi: 10.1016/0049-3848(73)90009-1
27. Caranobe C, Sie P, Boneu B. Serotonin uptake and storage in human platelet density subpopulations. Br J Haematol. (1982) 52:253–8. doi: 10.1111/j.1365-2141.1982.tb03887.x
28. Mezzano D, Aranda E, Rodriguez S, Foradori A, Lira P. Increase in density and accumulation of serotonin by human aging platelets. Am J Hematol. (1984) 17:11–21. doi: 10.1002/ajh.2830170103
29. Thompson CB, Eaton KA, Princiotta SM, Rushin CA, Valeri CR. Size dependent platelet subpopulations: relationship of platelet volume to ultrastructure, enzymatic activity, and function. Br J Haematol. (1982) 50:509–19. doi: 10.1111/j.1365-2141.1982.tb01947.x
30. Thompson CB, Love DG, Quinn PG, Valeri CR. Platelet size does not correlate with platelet age. Blood. (1983) 62:487–94.
31. Thompson CB, Jakubowski JA, Quinn PG, Deykin D, Valeri CR. Platelet size and age determine platelet function independently. Blood. (1984) 63:1372–5.
32. Corash L, Shafer B, Perlow M. Heterogeneity of human whole blood platelet subpopulations. II. Use of a subhuman primate model to analyze the relationship between density and platelet age. Blood. (1978) 52:726.
33. Corash L, Costa JL, Shafer B, Donlon JA, Murphy D. Heterogeneity of human whole blood platelet subpopulations. III. Density-dependent differences in subcellular constituents. Blood. (1984) 64:185.
34. Rand ML, Packham MA, Mustard JF. Survival of density subpopulations of rabbit platelets: use of 51Cr-or 111In-labeled platelets to measure survival of least dense and most dense platelets concurrently. Blood. (1983) 61:362.
35. Martin JF, Trowbridge EA. Theoretical requirements for the density separation of platelets with comparison of continuous and discontinuous gradients. Thromb Res. (1982) 27:513–22. doi: 10.1016/0049-3848(82)90298-5
36. Peng J, Friese P, Heilmann E, George JN, Burstein SA, Dale GL. Aged platelets have an impaired response to thrombin as quantitated by P-selectin expression. Blood. (1994) 83:161.
37. Hirsh J, Glynn MF, Mustard JF. The effect of platelet age on platelet adherence to collagen. J Clin Invest. (1968) 47:466–73. doi: 10.1172/JCI105743
38. Dolowy K, Cunnigham LW. Adhesion of human platelets to collagen: evidence for two states of platelet activation. Coll Relat Res. (1984) 4:111–8. doi: 10.1016/S0174-173X(84)80019-9
39. Patel D, Vaananen H, Jirouskova M, Hoffmann T, Bodian C, Coller BS. Dynamics of GPIIb/IIIa-mediated platelet-platelet interactions in platelet adhesion/thrombus formation on collagen in vitro as revealed by videomicroscopy. Blood. (2003) 101:929–36. doi: 10.1182/blood.V101.3.929
40. Zhao B, Filler TJ, Rickert CH, Liu JF, Skholl-Engberts AD, Gurevich V. Different adhesion types and active sensitivity of platelet subpopulations. Am J Hematol. (1996) 52:322–4.
41. Behnke O, Forer A. Blood platelet heterogeneity: evidence for two classes of platelets in man and rat. Br J Haematol. (1993) 84:686–93. doi: 10.1111/j.1365-2141.1993.tb03147.x
42. Opper C, Schuessler G, Kuschel M, Clement H-W, Gear ARL, Hinsch E, et al. Analysis of GTP-binding proteins, phosphoproteins, and cytosolic calcium in functional heterogeneous human blood platelet subpopulations. Biochem Pharmacol. (1997) 54:1027–35. doi: 10.1016/S0006-2952(97)00317-1
43. Opper C, Fett C, Capito B, Raha S, Wesemann W. Plasma membrane properties in heterogeneous human blood platelet subfractions modulate the cellular response at the second messenger level. Thromb Res. (1993) 72:39–47. doi: 10.1016/0049-3848(93)90171-J
44. Opper C, Schrumpf E, Gear ARL, Wesemann W. Involvement of guanylate cyclase and phosphodiesterases in the functional heterogeneity of human blood platelet subpopulations. Thromb Res. (1995) 80:461–70. doi: 10.1016/0049-3848(95)00201-4
45. Milovanovic M, Lysen J, Ramström S, Lindahl TL, Richter A, Järemo P. Identification of low-density platelet populations with increased reactivity and elevated α-granule content. Thromb Res. (2003) 111:75–80. doi: 10.1016/j.thromres.2003.08.019
46. van Oost BA, Timmermans AP, Sixma JJ. Evidence that platelet density depends on the alpha-granule content in platelets. Blood. (1984) 63:482.
47. Radziwon-Balicka A, Lesyk G, Back V, Fong T, Loredo-Calderon EL, Dong B, et al. Differential eNOS-signalling by platelet subpopulations regulates adhesion and aggregation. Cardiovasc Res. (2017) 113:1719–31. doi: 10.1093/cvr/cvx179
48. Radomski MW, Palmer RM, Moncada S. An L-arginine/nitric oxide pathway present in human platelets regulates aggregation. Proc Natl Acad Sci USA. (1990) 87:5193–7. doi: 10.1073/pnas.87.13.5193
49. Freedman JE, Sauter R, Battinelli EM, Ault K, Knowles C, Huang PL, et al. Deficient platelet-derived nitric oxide and enhanced hemostasis in mice lacking the NOSIII gene. Circ Res. (1999) 84:1416–21. doi: 10.1161/01.RES.84.12.1416
50. Roberts W, Michno A, Aburima A, Naseem KM. Nitric oxide inhibits von Willebrand factor-mediated platelet adhesion and spreading through regulation of integrin αIIbβ3 and myosin light chain. J Thromb Haemost. (2009) 7:2106–15. doi: 10.1111/j.1538-7836.2009.03619.x
51. Jurasz P, Stewart MW, Radomski A, Khadour F, Duszyk M, Radomski MW. Role of von Willebrand factor in tumour cell-induced platelet aggregation: differential regulation by NO and prostacyclin. Br J Pharmacol. (2001) 134:1104–12. doi: 10.1038/sj.bjp.0704343
52. Savage B, Almus-Jacobs F, Ruggeri ZM. Specific synergy of multiple substrate-receptor interactions in platelet thrombus formation under flow. Cell. (1998) 94:657–66. doi: 10.1016/S0092-8674(00)81607-4
53. Sawicki G, Salas E, Murat J, Miszta-Lane H, Radomski MW. Release of gelatinase A during platelet activation mediates aggregation. Nature. (1997) 386:616–9. doi: 10.1038/386616a0
54. Jurasz P, Chung AWY, Radomski A, Radomski MW. Nonremodeling properties of matrix metalloproteinases: the platelet connection. Circ Res. (2002) 90:1041–3. doi: 10.1161/01.RES.0000021398.28936.1D
55. Falcinelli E, Guglielmini G, Torti M, Gresele P. Intraplatelet signaling mechanisms of the priming effect of matrix metalloproteinase-2 on platelet aggregation. J Thromb Haemost. (2005) 3:2526–35. doi: 10.1111/j.1538-7836.2005.01614.x
56. Cozzi MR, Guglielmini G, Battiston M, Momi S, Lombardi E, Miller EC, et al. Visualization of nitric oxide production by individual platelets during adhesion in flowing blood. Blood. (2014) 125:697–705. doi: 10.1182/blood-2014-06-579474
57. Stalker TJ, Traxler EA, Wu J, Wannemacher KM, Cermignano SL, Voronov R, et al. Hierarchical organization in the hemostatic response and its relationship to the platelet-signaling network. Blood. (2013) 121:1875. doi: 10.1182/blood-2012-09-457739
58. Dale GL, Friese P, Batar P, Hamilton SF, Reed GL, Jackson KW, et al. Stimulated platelets use serotonin to enhance their retention of procoagulant proteins on the cell surface. Nature. (2002) 415:175–9. doi: 10.1038/415175a
59. Dachary-Prigent J, Freyssinet JM, Pasquet JM, Carron JC, Nurden AT. Annexin V as a probe of aminophospholipid exposure and platelet membrane vesiculation: a flow cytometry study showing a role for free sulfhydryl groups. Blood. (1993) 81:2554.
60. Alberio L, Safa O, Clemetson KJ, Esmon CT, Dale GL. Surface expression and functional characterization of α-granule factor V in human platelets: effects of ionophore A23187, thrombin, collagen, and convulxin. Blood. (2000) 95:1694.
61. Szasz R, Dale GL. Thrombospondin and fibrinogen bind serotonin-derivatized proteins on COAT-platelets. Blood. (2002) 100:2827. doi: 10.1182/blood-2002-02-0354
62. Kempton CL, Hoffman M, Roberts HR, Monroe DM. Platelet heterogeneity. Arterioscler Thromb Vasc Biol. (2005) 25:861–6. doi: 10.1161/01.ATV.0000155987.26583.9b
63. Panteleev MA, Ananyeva NM, Greco NJ, Ataullakhanov FI, Saenko EL. Two subpopulations of thrombin-activated platelets differ in their binding of the components of the intrinsic factor X-activating complex. J Thromb Haemost. (2005) 3:2545–53. doi: 10.1111/j.1538-7836.2005.01616.x
64. Topalov Nikolay N, Yakimenko Alena O, Canault M, Artemenko Elena O, Zakharova Natalia V, Abaeva Anastasia A, et al. Two types of procoagulant platelets are formed upon physiological activation and are controlled by integrin αIIbβ3. Arterioscler Thromb Vasc Biol. (2012) 32:2475–83. doi: 10.1161/ATVBAHA.112.253765
65. Yakimenko Alena O, Verholomova Faina Y, Kotova Yana N, Ataullakhanov Fazoil I, Panteleev Mikhail A. Identification of different proaggregatory abilities of activated platelet subpopulations. Biophys J. (2012) 102:2261–9. doi: 10.1016/j.bpj.2012.04.004
66. Abaeva AA, Canault M, Kotova YN, Obydennyy SI, Yakimenko AO, Podoplelova NA, et al. Procoagulant platelets form an α-granule protein-covered “cap” on their surface that promotes their attachment to aggregates. J Biol Chem. (2013) 288:29621–32. doi: 10.1074/jbc.M113.474163
67. Mattheij NJA, Swieringa F, Mastenbroek TG, Berny-Lang MA, May F, Baaten CCFMJ, et al. Coated platelets function in platelet-dependent fibrin formation via integrin αIIbβ3 and transglutaminase factor XIII. Haematologica. (2016) 101:427. doi: 10.3324/haematol.2015.131441
68. Heemskerk JWM, Mattheij NJA, Cosemans JMEM. Platelet-based coagulation: different populations, different functions. J Thromb Haemost. (2013) 11:2–16. doi: 10.1111/jth.12045
69. Donati A, Gupta S, Reviakine I. Subpopulations in purified platelets adhering on glass. Biointerphases. (2016) 11:029811. doi: 10.1116/1.4953866
70. Södergren AL, Ramström S. Platelet subpopulations remain despite strong dual agonist stimulation and can be characterised using a novel six-colour flow cytometry protocol. Sci Rep. (2018) 8:1441. doi: 10.1038/s41598-017-19126-8
71. Prodan CI, Joseph PM, Vincent AS, Dale GL. Coated-platelets in ischemic stroke: differences between lacunar and cortical stroke. J Thromb Haemost. (2008) 6:609–14. doi: 10.1111/j.1538-7836.2008.02890.x
72. Prodan CI, Stoner JA, Cowan LD, Dale GL. Higher coated-platelet levels are associated with stroke recurrence following nonlacunar brain infarction. J Cereb Blood Flow Metab. (2012) 33:287–92. doi: 10.1038/jcbfm.2012.168
73. Prodan CI, Vincent AS, Dale GL. Higher coated-platelet levels are associated with chronic hypertension in patients with transient ischemic attack. Platelets. (2013) 24:316–9. doi: 10.3109/09537104.2012.696747
74. Kirkpatrick AC, Stoner JA, Dale GL, Prodan CI. Elevated coated-platelets in symptomatic large-artery stenosis patients are associated with early stroke recurrence. Platelets. (2014) 25:93–6. doi: 10.3109/09537104.2013.775570
75. Kirkpatrick AC, Tafur AJ, Vincent AS, Dale GL, Prodan CI. Coated-platelets improve prediction of stroke and transient ischemic attack in asymptomatic internal carotid artery stenosis. Stroke. (2014) 45:2995–3001. doi: 10.1161/STROKEAHA.114.006492
76. Prodan CI, Stoner JA, Dale GL. Lower coated-platelet levels are associated with increased mortality after spontaneous intracerebral hemorrhage. Stroke. (2015) 46:1819–25. doi: 10.1161/STROKEAHA.115.009068
77. Prodan CI, Vincent AS, Kirkpatrick AC, Hoover SL, Dale GL. Higher levels of coated-platelets are observed in patients with subarachnoid hemorrhage but lower levels are associated with increased mortality at 30days. J Neurol Sci. (2013) 334:126–9. doi: 10.1016/j.jns.2013.08.008
78. Prodan CI, Stoner JA, Cowan LD, Dale GL. Lower coated-platelet levels are associated with early hemorrhagic transformation in patients with non-lacunar brain infarction. J Thromb Haemost. (2010) 8:1185–90. doi: 10.1111/j.1538-7836.2010.03851.x
79. Prodan CI, Vincent AS, Dale GL. Coated-platelet levels are elevated in patients with transient ischemic attack. Transl Res. (2011) 158:71–5. doi: 10.1016/j.trsl.2011.02.010
80. Daskalakis M, Colucci G, Keller P, Rochat S, Silzle T, Biasiutti FD, et al. Decreased generation of procoagulant platelets detected by flow cytometric analysis in patients with bleeding diathesis. Cytometry Part B. (2014) 86B:397–409. doi: 10.1002/cyto.b.21157
81. Myers DR, Qiu Y, Fay ME, Tennenbaum M, Chester D, Cuadrado J, et al. Single-platelet nanomechanics measured by high-throughput cytometry. Nat Mater. (2016) 16:230. doi: 10.1038/nmat4772
82. Collier A, Watson HHK, Matthews DM, Strain L, Ludlam CA, Clarke BF. Platelet-density analysis and intraplatelet granule content in young insulin-dependent diabetics. Diabetes. (1986) 35:1081. doi: 10.2337/diabetes.35.10.1081
83. Opper C, Clement C, Schwarz H, Krappe J, Steinmetz A, Schneider J, et al. Increased number of high sensitive platelets in hypercholesterolemia, cardiovascular diseases, and after incubation with cholesterol. Atherosclerosis. (1995) 113:211–7. doi: 10.1016/0021-9150(94)05448-R
84. Giles H, Smith REA, Martin JF. Platelet glycoprotein IIb-IIIa and size are increased in acute myocardial infarction. Eur J Clin Invest. (1994) 24:69–72. doi: 10.1111/j.1365-2362.1994.tb02062.x
85. Clancy L, Beaulieu LM, Tanriverdi K, Freedman JE. The role of RNA uptake in platelet heterogeneity. Thromb Haemost. (2017) 117:948–61. doi: 10.1160/TH16-11-0873
86. Best MG, Sol N, Kooi I, Tannous J, Westerman Bart A, Rustenburg F, et al. RNA-seq of tumor-educated platelets enables blood-based pan-cancer, multiclass, and molecular pathway cancer diagnostics. Cancer Cell. (2015) 28:666–76. doi: 10.1016/j.ccell.2015.09.018
87. Best MG, Wesseling P, Wurdinger T. Tumor-educated platelets as a noninvasive biomarker source for cancer detection and progression monitoring. Cancer Res. (2018) 78:3407. doi: 10.1158/0008-5472.CAN-18-0887
88. Jakubowski JA, Alder B, Thompson CB, Valeri CR, Deykin D. Influence of platelet volume on the ability of prostacyclin to inhibit platelet aggregation and the release reaction. J Lab Clin Med. (1985) 105:271–6.
89. Mangalpally KKR, Siqueiros-Garcia A, Vaduganathan M, Dong J-F, Kleiman NS, Guthikonda S. Platelet activation patterns in platelet size sub-populations: differential responses to aspirin in vitro. J Thromb Thrombol. (2010) 30:251–62. doi: 10.1007/s11239-010-0489-x
90. Hoefer T, Armstrong PC, Finsterbusch M, Chan MV, Kirkby NS, Warner TD. Drug-free platelets can act as seeds for aggregate formation during antiplatelet therapy. Arterioscler Thromb Vasc Biol. (2015) 35:2122–33. doi: 10.1161/ATVBAHA.115.306219
91. Michelson AD. Flow cytometric analysis of platelet surface glycoproteins: phenotypically distinct subpopulations of platelets in children with chronic myeloid leukemia. J Lab Clin Med. (1987) 110:346–54.
92. Frojmovic M, Wong T. Dynamic measurements of the platelet membrane glycoprotein IIb-IIIa receptor for fibrinogen by flow cytometry. II. Platelet size-dependent subpopulations. Biophys J. (1991) 59:828–37. doi: 10.1016/S0006-3495(91)82295-0
93. Claytor RB, Li JM, Furman MI, Garnette CS, Rohrer MJ, Barnard MR, et al. Laser scanning cytometry: a novel method for the detection of platelet–endothelial cell adhesion. Cytometry. (2001) 43:308–13. doi: 10.1002/1097-0320(20010401)43:4<308::AID-CYTO1063>3.0.CO;2-9
94. Basabe-Desmonts L, Ramstrom S, Meade G, O'Neill S, Riaz A, Lee LP, et al. Single-step separation of platelets from whole blood coupled with digital quantification by interfacial platelet cytometry (iPC). Langmuir. (2010) 26:14700–6. doi: 10.1021/la9039682
95. Blair TA, Michelson AD, Frelinger AL. Mass cytometry reveals distinct platelet subtypes in healthy subjects and novel alterations in surface glycoproteins in glanzmann thrombasthenia. Sci Rep. (2018) 8:10300. doi: 10.1038/s41598-018-28211-5
96. Soh KT, Wallace PK. RNA flow cytometry using the branched DNA technique. Methods Mol Biol. (2018) 1678:49–77. doi: 10.1007/978-1-4939-7346-0_4
97. Handtke S, Steil L, Greinacher A, Thiele T. Toward the relevance of platelet subpopulations for transfusion medicine. Front Med. (2018) 5:17. doi: 10.3389/fmed.2018.00017
Keywords: platelet subpopulations, vanguard and follower platelets, COAT-platelets, eNOS-based platelet subpopulations, thrombosis, hemostasis
Citation: Lesyk G and Jurasz P (2019) Advances in Platelet Subpopulation Research. Front. Cardiovasc. Med. 6:138. doi: 10.3389/fcvm.2019.00138
Received: 24 July 2019; Accepted: 29 August 2019;
Published: 13 September 2019.
Edited by:
Christian Schulz, Ludwig Maximilian University of Munich, GermanyReviewed by:
George L. Dale, University of Oklahoma Health Sciences Center, United StatesBenoît Ho-Tin-Noé, Institut National de la Santé et de la Recherche Médicale (INSERM), France
Copyright © 2019 Lesyk and Jurasz. This is an open-access article distributed under the terms of the Creative Commons Attribution License (CC BY). The use, distribution or reproduction in other forums is permitted, provided the original author(s) and the copyright owner(s) are credited and that the original publication in this journal is cited, in accordance with accepted academic practice. No use, distribution or reproduction is permitted which does not comply with these terms.
*Correspondence: Paul Jurasz, anVyYXN6QHVhbGJlcnRhLmNh