Calcium Dobesilate (CaD) Attenuates High Glucose and High Lipid-Induced Impairment of Sarcoplasmic Reticulum Calcium Handling in Cardiomyocytes
- 1Department of Endocrinology, Shenzhen Second People's Hospital, The First Affiliated Hospital of Shenzhen University, Health Science Center of Shenzhen University, Shenzhen, China
- 2Center for Medical Experiments, University of Chinese Academy of Science-Shenzhen Hospital, Shenzhen, China
- 3School of Basic Medical Science, Health Science Center of Shenzhen University, Shenzhen, China
- 4Molecular Cardiology Program, Department of Biomedical Engineering, University of Alabama at Birmingham, Birmingham, AL, United States
Calcium dobesilate (CaD) is used effectively in patients with diabetic microvascular disorder, retinopathy, and nephropathy. Here we sought to determine whether it has an effect on cardiomyocytes calcium mishandling that is characteristic of diabetic cardiomyopathy. Cardiomyocytes were sterile isolated and cultured from 1 to 3 days neonatal rats and treated with vehicle (Control), 25 mM glucose+300 μM Palmitic acid (HG+PA), 100 μM CaD (CaD), or HG+PA+CaD to test the effects on calcium signaling (Ca2+ sparks, transients, and SR loads) and reactive oxygen species (ROS) production by confocal imaging. Compared to Control, HG+PA treatment significantly reduced field stimulation-induced calcium transient amplitudes (2.22 ± 0.19 vs. 3.56 ± 0.21, p < 0.01) and the levels of caffeine-induced calcium transients (3.19 ± 0.14 vs. 3.72 ± 0.15, p < 0.01), however significantly increased spontaneous Ca2+ sparks firing levels in single cardiomyocytes (spontaneous frequency 2.65 ± 0.23 vs. 1.72 ± 0.12, p < 0.01) and ROS production (67.12 ± 4.4 vs. 47.65 ± 2.12, p < 0.05), which suggest that HG+PA treatment increases the Spontaneity Ca2+ spark frequency, and then induced partial reduction of SR Ca2+ content and subsequently weaken systolic Ca2+ transient in cardiomyocyte. Remarkably, these impairments in calcium signaling and ROS production were largely prevented by pre-treatment of the cells with CaD. Therefore, CaD may contribute to a good protective effect on patients with calcium mishandling and contractile dysfunction in cardiomyocytes associated with diabetic cardiomyopathy.
Introduction
The worldwide prevalence of diabetes mellitus increases markedly to cause various cardiovascular complications, which are the major cause of morbidity and mortality in this population (1, 2). Among patients with the same degree of cardiovascular diseases, the mortality rate from cardiovascular diseases in diabetic patients is 2–4 times higher than that of non-diabetic patients. In addition, diabetic cardiomyopathy can occur without any vascular pathogenesis (3–5); The disease progress from initial asymptomatic left ventricular (LV) diastolic dysfunction to impaired LV systolic function, and eventually advances to heart failure (6). Therefore, a better understanding of mechanisms of diabetes-associated cardiomyocytes impairments will aid the development of novel strategies for protecting heart function in these patients (7–9).
As we know, Ca2+ plays an essential role in cardiac EC-coupling. Ca2+ major stores in sarcoplasmic reticulum (SR) in the mammalian heart. At systole, instantaneous high concentration of Ca2+ is released from SR trigged by Ca2+ influx trough L-type Ca2+ channel, consequently Ca2+ transient that triggers myofilaments contraction in heart (10). Ryanodine receptors play essential role in Ca2+ release from SR via CICR mechanism. Spontaneous Ca2+ sparks, the elementary intracellular Ca2+ release from 4 to 6 neighboring RyRs, is sporadic occurs in the cadiomyocytes under physiological condition. The frequency of Ca2+ sparks is closely related to the activity of RyR at diastole, and control SR Ca2+ content. High Spontaneous Ca2+ sparks frequency, often happened in kind of diseases, have been reported to reduce SR Ca2+ content (11). RyR dysfunction has been shown to cause various of cardiac dysfunction, such as heart failure, hypertrophy (12, 13) and ischemia-reperfusion (14). Abnormal RyR function has also been shown in type 1 diabetes (15), and STZ-induced type-2 diabetes rat model (2), despite the pathological significance is not well-characterized.
Calcium dobesilate (CaD) is a vascular protective agent, highly effective in treating patients with various diabetes-associated microvascular disorders, retinopathy, nephropathy, and cataract (16–18). CaD has been shown to decrease intracellular ROS production, improve the biosynthesis of collagen in the basement membrane, and inhibit the high permeability caused by vasoactive substances. However, whether CaD has an effect on diabetes-associated or high glucose-induced cardiomyocytes dysfunction is currently unknown. In this study, we sought to examine the potential effects of CaD on calcium regulation of primary ventricular cardiomyocytes. We found that CaD efficiently protects high glucose-induced SR calcium leaks and depletion. Thus, CaD may be used to protect impairment of cardiomyocytes calcium signaling and contractile function associated with diabetic cardiomyopathy.
Methods
Isolation, Culture, and Treatments of Neonatal Rat Ventricular Myocytes (NRVMs)
Sprague–Dawley rats of 1–3-days-old were purchased from the Animal center of Guangdong Experimental Animal Center and handled according to a protocol approved by the Institutional Care and Use Committee of Shenzhen University, which conforms to the Guide for the Care and Use of Laboratory Animals published by the US National Institutes of Health (NIH Publication No. 85-23, revised 1996). NRVMs were isolated and cultured as previously described (19). After cultured for 24 h, the cells were stimulated with 25 mM glucose+300 μM PA (sigma, USA) for 24 h. In the case of CaD (Merck Serono Co., Ltd) treatment, NRVMs were pretreated with 100 μM CaD for 24 h.
Ca2+ Spark Recording
Briefly, NRVMs loaded with Fluo-4 AM (ThermoFisher Scientific, F14201, dissolved in DMSO contains 20% pluronic F-127) for 10 min at 37°C and then perfused with Tyrode's solution (137 mM NaCl, 1.2 mM MgCl2, 1.2 mM NaH2PO4, 20 mM HEPES, 5.4 mM KCl, 1.8 mM CaCl2, 10 mM glucose, pH 7.4) in a recording chamber. Confocal line-scan imaging was carried out in resting cells at 488 nm excitation and 505 nm collection with a Zeiss 780 inverted confocal microscope (Carl Zeiss) with a 40x oil immersion lens for detecting Ca2+ sparks as described previously (20). Line-scan images were acquired at a sampling rate of 3.84 ms per line.
Measurement of Action Potential (AP)-Elicited Ca2+ Transient
After cells loaded with Fluo-4 AM, Cells were superfused with a normal external solution containing (same as calcium spark recording) remove the dye and complete de-esterification. After the cardiomyocytes reached a steady-state with field stimulation (1 Hz), line-scan imaging was acquired along the longitudinal axis of the cells.
Measurement of SR Ca2+ Content
Short puffs of caffeine (20 mM) were applied to completely empty the SR, after a train of 1-Hz field stimulation to achieve steady-state SR Ca2+ loading. SR Ca2+ content was assessed by detecting the amplitude of the Ca2+ transient. Cells were superfused with normal external solution.
Measurement of Reactive Oxygen Species (ROS)
Cells were loaded with the probe 5-(6)-chloromethyl-2′,7′-dichlorodihydrofluorescein diacetate (CM-H2DCFDA, 10 μM, Molecular Probes) for 10 min at 37°C before the experiment. The 2′,7′-dichlorodihydrofluorescein (DCF) fluorescent intensity was measured by using LSM 780 (Zeiss) at an excitation wavelength of 485 nm and an emission wavelength of 530 nm (21).
Statistical Analysis
All values are given as mean ± SE. Unpaired Student's t-test between 2 groups was performed, one-way analysis of variance (ANOVA) followed by the Dunnett's post-hoc test was used for comparisons among four groups. In all tests, values of p < 0.05 were considered be statistically significant.
Results
CaD Attenuates HG+PA–Induced Increase in Ca2+ Spark Frequency
We initiated our study by analyzing effects of HG+PA and CaD on the occurrence and properties of Ca2+ sparks in NRVMs. HG+PA increased the frequency of Ca2+ sparks by 1.54-fold (2.65 ± 0.23 vs. 1.72 ± 0.12 sparks/100 μm·s in Control, p < 0.01. Figures 1A,B) and to a lesser extent, decreased the amplitude(F/F0) of Ca2+ sparks (1.93 ± 0.04 vs. 2.12 ± 0.05 in Control, p < 0.05) (Figure 1C). In addition, HG+PA decreased full width of half maximum (FWHM) of Ca2+ sparks but did not alter the full duration of half maximum (FDHM) (Figures 1D,E). Remarkably, pre-treatment of cells with CaD restored HG+PA–induced alterations in Ca2+ spark frequency, amplitudes, and FWHM (Figures 1A–E). These results suggest that CaD normalizes HG+PA induced RyR dysfunction in the cardiomyocytes at baseline.
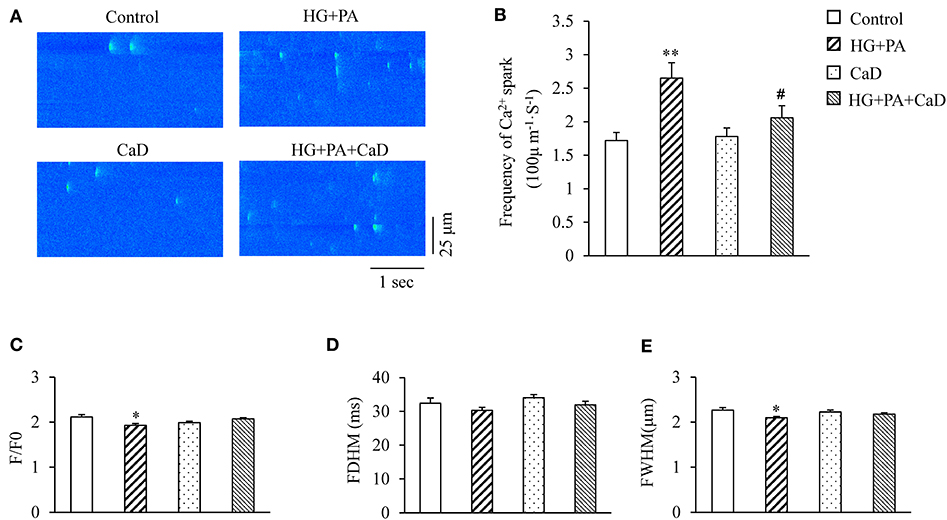
Figure 1. HG+PA increases Ca2+ sparks frequency in cardiomyocytes. (A) Representative Ca2+ spark images. (B–E) Statistic analyses of the frequency (B), amplitude (F/F0, C), full duration of half maximum (FDHM, D) and full width of half maximum (FWHM, E) of Ca2+ sparks in Control, HG+PA, CaD, and HG+PA+CaD treatments. **p < 0.01, *p < 0.05 vs. control; #p < 0.05 vs. HG+PA. n = 103–258.
CaD Attenuates HG+PA–Induced Reduction in SR Ca2+ Content
SR Ca2+ content affects the frequency and amplitude of Ca2+ sparks in cardiomyocytes; the higher of SR Ca2+ content results in the higher frequency of spontaneous Ca2+sparks (22). To determine whether HG+PA–increased increase in the spark frequency is due to an increased SR load, we analyzed the amplitude of caffeine-elicited Ca2+ transient (ΔF/F0) indicating SR Ca2+ content. High concentration of Caffeine (20 mM) was used to stimulate the change of calcium storage capacity in each group. We found that HG+PA treatment significantly decreased SR Ca2+ content (ΔF/F0, Figure 2A). In contrast, pre-treatment of cells with CaD completely prevented the HG+PA–induction of SR Ca2+ depletion (Figure 2B).
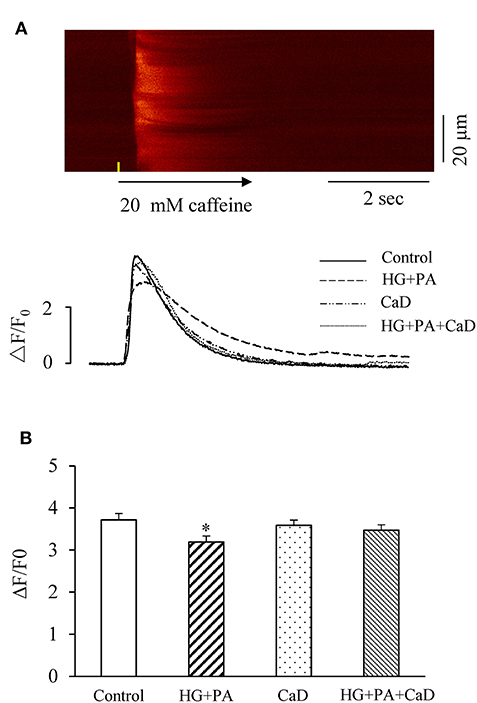
Figure 2. HG+PA reduces SR Ca2+ content in cardiomyocytes. (A) Representative confocal image in control and representative time-courses of caffeine-elicited Ca2+ transient traces in Control, HG+PA, CaD, and HG+PA+CaD. (B) Average SR Ca2+ contents indicated by the amplitude of caffeine-elicited Ca2+ transient (ΔF/F0) in Control, HG+PA, CaD, and HG+PA+CaD. *p < 0.05 vs. control. n = 10–20.
CaD Ameliorates HG+PA–Induced Reduction in Systolic Ca2+ Transient
Previous reports suggest that the reduction of systolic calcium transients in cardiomyocytes is the main cause of impaired contractility in diabetic cardiomyopathy (15). We elicited systolic Ca2+ transient by 1 Hz-field stimulation in the treatment groups. HG+PA treatment caused a significantly reduced amplitude (ΔF/F0, 2.22 ± 0.19 vs. 3.56 ± 0.21 in Control, p < 0.01, Figures 3A,B) and a significantly prolonged rise time (Figure 3C) of Ca2+ transient, suggesting an impairment of the synchrony of SR Ca2+ release. Treatment with CaD did not affect the calcium transient amplitude (3.68 ± 0.3 vs. 3.56 ± 0.21 in Control, p > 0.05), however, ameliorated HG+PA–induced impairment of SR Ca2+ release (ΔF/F0, 3.28 ± 0.25 vs. 2.22 ± 0.19 in HG+PA, p < 0.05; Rise time, 89.68 ± 2.81 vs. 78.59 ± 2.12 in HG+PA, p < 0.05. Figures 3A–C). We did not observe a statistical significance in T50 between the four treatment groups (Figure 3D).
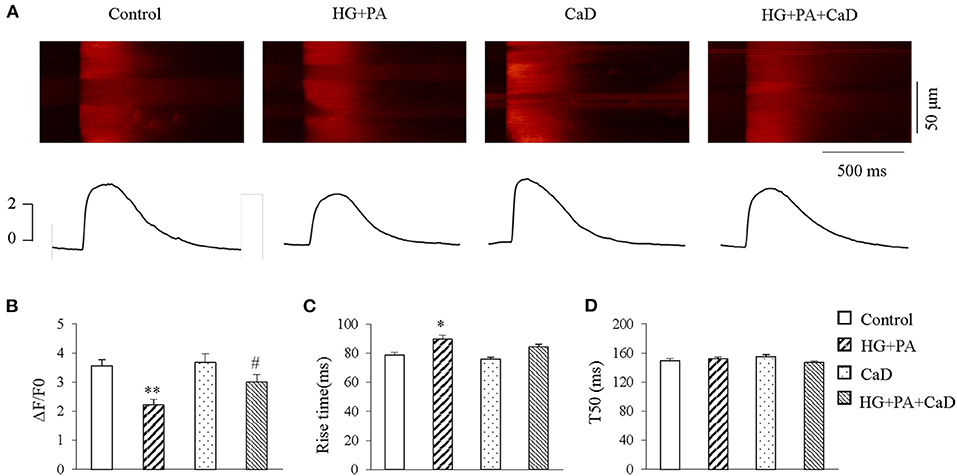
Figure 3. HG+PA reduces systolic Ca2+ transient in cardiomyocytes. (A) Representative confocal line-scan images of Ca2+ transients (upper panels) along with the spatial average (lower panels) in Control, HG+PA, CaD, and HG+PA+CaD treatments. (B–D) Average amplitudes (ΔF/F0, B), Rise time (C) and half time of decay (T50, D) of Ca2+ transient in Control, HG+PA, CaD, and HG+PA+CaD treatments. **p < 0.01, *p < 0.05 vs. control; #p < 0.05 vs. HG+PA. n = 25–45.
CaD Suppress HG+PA–Induced Oxidative Stress
Because oxidation and nitrosylation of cysteine residues on RyR are key post-translational modifications that make the channel open to Ca2+ leaks, whereas reversal of these modifications closes the channel (23, 24), we examined whether the HG+PA induced hyperactive Ca2+ sparks is accounted by the increased ROS level in cardiomyocytes. We found that HG+PA treatment dramatically increased superoxide concentration in cardiomyocytes, and this change was markedly attenuated by treatment with CaD (Figure 4). Thus, CaD suppresses HG+PA–induced ROS production.
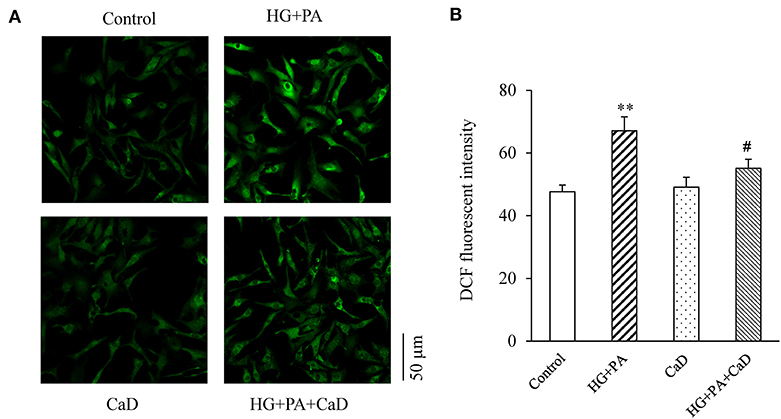
Figure 4. HG+PA increases the level of ROS in neonatal cardiomyocytes. Representative fluorescence images (A) and quantification (B) of ROS production. **p < 0.01 vs. control; #p < 0.05 vs. HG+PA.
Discussion
In this study, we found that exposure of neonatal rat ventricular cardiomyocytes to high glucose and high lipid (HG+PA) results in a marked increase in the intracellular ROS and Ca2+ sparks the frequency, a partial depletion of the SR Ca2+ content, and contractile dysfunction. Treatment of these cells with CaD attenuates the ROS production, spontaneous Ca2+ sparks, and SR Ca2+ depletion, restoring cardiomyocyte function. Although the beneficial effects of CaD in diabetic microangiopathy (e.g., diabetic retinopathy, nephropathy, and neuropathy) have been well-known, we study for first-time documents that CaD protects cardiomyocytes from HG+PA induced injury and Ca2+ mishandling.
Our findings have a high translational value for the treatment of diabetic cardiomyopathy. Since the 1970's, CaD has been front-line medication for the prevention and treatment of diabetic retinopathy with an excellent safety profile. Our data suggest that it may have a direct protective effect on cardiomyocytes in diabetes and that its use may be extended to diabetic heart disease. This is particularly important because currently there is no drug to specifically target high-glucose and high-fat induced cardiomyocyte injury. Notably, our results indicate that CaD is effective after HG+PA treatment and does not affect cardiomyocyte function at baseline. Therefore, it is probably safe and consistent with its safety profile in the clinic.
Diabetes mellitus is a metabolic disorder characterized by abnormal glucose and lipid metabolism. Hyperglycemia and high fat can cause cardiac damage, leading to diabetic cardiomyopathy (3, 4, 25). Mechanistically, long-time high blood glucose leads to excessive protein glycosylation and accumulation of advanced glycation end products (AGEs), which in sync with an elevation of the receptor for AGEs (RAGE) in the HG milieu, induces the production of cytokines and free radicals, resulting in cardiomyocyte injury. Importantly, we have previously shown that superfluous AGEs can influence Ca2+ spark firing frequency in cardiomyocytes, and that inhibition of RAGE significantly reduces the calcium spark frequency and protects heart function (26). Additionally, high blood glucose may induce apoptosis of cardiomyocytes through the deposition of oxidative stress, and now ample evidence suggests an increase of RyR in diabetes that is associated with oxidative stress. In addition to hyperglycemia, high fat is also found to induce ROS production. For example, free fatty acid (FFA) can induce cardiomyocyte apoptosis by increasing the concentrations of ceramide and ROS. Because overproduction of ROS contributes to the pathogenesis of diabetic cardiomyopathy, treatment with antioxidants can reverse the cardiac dysfunction (27, 28).
Recently, a series of studies found that SR calcium leakage increases in the cardiomyocytes of heart failure patients and contributes to the pathogenesis of heart failure (29, 30). Notably, ROS has been shown directly associated with Ca2+ sparks in the cardiomyocytes (31, 32). Enhanced diastolic SR Ca2+ leak has been suggested to be a potential cause of reduction of SR Ca2+ content (12), and the systolic intracellular Ca2+ concentration determines cardiac contractility. In rodent heart, Ca2+ influx through LCC accounts for no more than 30% while SR Ca2+ release contributes to over 70% of Ca2+ transient in systolic (33). Our data suggested that HG+PA significantly decreased SR Ca2+ content, which is consistent with the alteration of the magnitude of the systolic Ca2+ transient. Our finding indicates that HG+PA decreases cardiac contractile function, which may be an important mechanism for cardiac dysfunction in diabetic cardiomyopathy.
CaD, as an oxygen free radical scavenger, have been shown inhibits free radical production both in vitro and in vivo (34–37). In this study, we found that HG+PA significantly increases the oxidative stress level in cardiomyocytes, and this effect was effectively attenuated by CaD treatment. Thus, CaD reduces oxidative stress levels in the cells, thereby reducing the frequency of spontaneous calcium sparks, restoring the sarcoplasmic reticulum calcium pool, and stabilizing calcium transients in cardiomyocytes.
One limitation of this study is that our experimental system was primarily based on the cultured neonatal rat cardiomyocytes, which may have electrophysiological features different from the adult cells or the in vivo diabetic cardiomyopathy models. Thus, cautions should be taken when translating these findings into potential clinic use of the drug. In addition, the molecular targets of CaD that mediates its effects on ROS reduction and consequent Ca2+ signaling in cardiomyocytes are yet to be identified, which remains a subject of our future studies.
In conclusion, CaD protects cardiomyocytes from HG and PA-induced elevation in oxidative stress and spontaneous spark frequency and restores sarcoplasmic reticulum calcium levels. Thus, it may be utilized for the treatment of cardiac contractile dysfunction in diabetic cardiomyopathy.
Data Availability Statement
The raw data supporting the conclusions of this article will be made available by the authors, without undue reservation.
Ethics Statement
The animal study was reviewed and approved by Laboratory Animal Science Research Committee of Shenzhen University Health Science Center.
Author Contributions
JD and DY conceptualized the study and contributed to resources. XC, ZC, MH, YL, HF, HC, HL, and JL collected data. JD and GQ wrote the manuscript. All authors contributed to the article and approved the submitted version.
Funding
This work was supported by the National Science Foundation of China (No. 81670759 to JD), Basic Research Foundation of Shenzhen (No. JCYJ20170306092910641 to JD), Bethune charitable foundation (No. G-X-2019-056 to JD), and Sanming project of medicine in Shenzhen (No. SZSM201612007 to DY).
Conflict of Interest
The authors declare that the research was conducted in the absence of any commercial or financial relationships that could be construed as a potential conflict of interest.
References
1. Chen L, Magliano DJ, Zimmet PZ. The worldwide epidemiology of type 2 diabetes mellitus–present and future perspectives. Nat Rev Endocrinol. (2011) 8:228–36. doi: 10.1038/nrendo.2011.183
2. Tripathi BK, Srivastava AK. Diabetes mellitus: complications and therapeutics. Med Sci Monit. (2006) 12:RA130–47.
3. Rubler S, Dlugash J, Yuceoglu YZ, Kumral T, Branwood AW, Grishman A. New type of cardiomyopathy associated with diabetic glomerulosclerosis. Am J Cardiol. (1972) 30:595–602. doi: 10.1016/0002-9149(72)90595-4
4. Harper SJ, Saporito MS, Hewson L, Young L, Smith D, Rigby M, et al. CEP-1347 increases ChAT activity in culture and promotes cholinergic neurone survival following fimbria-fornix lesion. Neuroreport. (2000) 11:2271–6. doi: 10.1097/00001756-200007140-00041
5. Cai X, Zhang Y, Li M, Wu JH, Mai L, Li J, et al. Association between prediabetes and risk of all-cause mortality and cardiovascular disease: updated meta-analysis. BMJ. (2020) 370:m2297. doi: 10.1136/bmj.m2297
6. Kane GC, Karon BL, Mahoney DW, Redfield MM, Roger VL, Burnett JC Jr, et al. Progression of left ventricular diastolic dysfunction and risk of heart failure. JAMA. (2011) 306:856–63. doi: 10.1001/jama.2011.1201
7. Beisswenger PJ, Howell SK, Touchette AD, Lal S, Szwergold BS. Metformin reduces systemic methylglyoxal levels in type 2 diabetes. Diabetes. (1999) 48:198–202. doi: 10.2337/diabetes.48.1.198
8. Zhou G, Myers R, Li Y, Chen Y, Shen X, Fenyk-Melody J, et al. Role of AMP-activated protein kinase in mechanism of metformin action. J Clin Invest. (2001) 108:1167–74. doi: 10.1172/JCI13505
9. Wu J, Zheng H, Liu X, Chen P, Zhang Y, Luo J, et al. Prognostic value of secreted frizzled-related protein 5 in heart failure patients with and without type 2 diabetes mellitus. Circ Heart Fail. (2020) 13:e007054. doi: 10.1161/CIRCHEARTFAILURE.120.007054
10. Bers DM. Cardiac excitation-contraction coupling. Nature. (2002) 415:198–205. doi: 10.1038/415198a
11. Cannell MB, Cheng H, Lederer WJ. The control of calcium release in heart muscle. Science. (1995) 268:1045–9. doi: 10.1126/science.7754384
12. Jiang X, Liu W, Deng J, Lan L, Xue X, Zhang C, et al. Polydatin protects cardiac function against burn injury by inhibiting sarcoplasmic reticulum Ca2+ leak by reducing oxidative modification of ryanodine receptors. Free Radic Biol Med. (2013) 60:292–9. doi: 10.1016/j.freeradbiomed.2013.02.030
13. Lyon AR, Bannister ML, Collins T, Pearce E, Sepehripour AH, Dubb SS, et al. SERCA2a gene transfer decreases sarcoplasmic reticulum calcium leak and reduces ventricular arrhythmias in a model of chronic heart failure. Circ Arrhythm Electrophysiol. (2011) 4:362–72. doi: 10.1161/CIRCEP.110.961615
14. Fauconnier J, Meli AC, Thireau J, Roberge S, Shan J, Sassi Y, et al. Ryanodine receptor leak mediated by caspase-8 activation leads to left ventricular injury after myocardial ischemia-reperfusion. Proc Natl Acad Sci U S A. (2011) 108:13258–63. doi: 10.1073/pnas.1100286108
15. Belke DD, Swanson EA, Dillmann WH. Decreased sarcoplasmic reticulum activity and contractility in diabetic db/db mouse heart. Diabetes. (2004) 53:3201–8. doi: 10.2337/diabetes.53.12.3201
16. Sun J, Wang B, Hao Y, Yang X. Effects of calcium dobesilate on NRF2, KEAP1 and HO-1 in the lenses of D-galactose-induced cataracts in rats. Exp Ther Med. (2018) 15:719–22. doi: 10.3892/etm.2017.5435
17. Liu J, Li S, Sun D. Calcium dobesilate and micro-vascular diseases. Life Sci. (2019) 221:348–53. doi: 10.1016/j.lfs.2019.02.023
18. Haller H, Ji L, Stahl K, Bertram A, Menne J. Molecular mechanisms and treatment strategies in diabetic nephropathy: new avenues for calcium dobesilate-free radical scavenger and growth factor inhibition. Biomed Res Int. (2017) 2017:1909258. doi: 10.1155/2017/1909258
19. Liu W, Deng J, Xu J, Wang H, Yuan M, Liu N, et al. High-mobility group box 1 (HMGB1) downregulates cardiac transient outward potassium current (Ito) through downregulation of Kv4.2 and Kv4.3 channel transcripts and proteins. J Mol Cell Cardiol. (2010) 49:438–48. doi: 10.1016/j.yjmcc.2010.05.004
20. Deng J, Wang G, Huang Q, Yan Y, Li K, Tan W, et al. Oxidative stress-induced leaky sarcoplasmic reticulum underlying acute heart failure in severe burn trauma. Free Radic Biol Med. (2008) 44:375–85. doi: 10.1016/j.freeradbiomed.2007.09.023
21. Luo X, Deng J, Liu N, Zhang C, Huang Q, Liu J. Cellular mechanism underlying burn serum-generated bidirectional regulation of excitation-contraction coupling in isolated rat cardiomyocytes. Shock. (2011) 35:388–95. doi: 10.1097/SHK.0b013e3182000379
22. Hasenfuss G, Pieske B. Calcium cycling in congestive heart failure. J Mol Cell Cardiol. (2002) 34:951–69. doi: 10.1006/jmcc.2002.2037
23. Lim G, Venetucci L, Eisner DA, Casadei B. Does nitric oxide modulate cardiac ryanodine receptor function? Implications for excitation-contraction coupling. Cardiovasc Res. (2008) 77:256–64. doi: 10.1093/cvr/cvm012
24. Meissner G. Regulation of mammalian ryanodine receptors. Front Biosci. (2002) 7:d2072–80. doi: 10.2741/A899
25. Singh JP, Larson MG, O'Donnell CJ, Wilson PF, Tsuji H, Lloyd-Jones DM, et al. Association of hyperglycemia with reduced heart rate variability (The Framingham Heart Study). Am J Cardiol. (2000) 86:309–12. doi: 10.1016/S0002-9149(00)00920-6
26. Yan D, Luo X, Li Y, Liu W, Deng J, Zheng N, et al. Effects of advanced glycation end products on calcium handling in cardiomyocytes. Cardiology. (2014) 129:75–83. doi: 10.1159/000364779
27. Huynh K, Bernardo BC, McMullen JR, Ritchie RH. Diabetic cardiomyopathy: mechanisms and new treatment strategies targeting antioxidant signaling pathways. Pharmacol Ther. (2014) 142:375–415. doi: 10.1016/j.pharmthera.2014.01.003
28. Kain V, Kumar S, Sitasawad SL. Azelnidipine prevents cardiac dysfunction in streptozotocin-diabetic rats by reducing intracellular calcium accumulation, oxidative stress and apoptosis. Cardiovasc Diabetol. (2011) 10:97. doi: 10.1186/1475-2840-10-97
29. Terentyev D, Gyorke I, Belevych AE, Terentyeva R, Sridhar A, Nishijima Y, et al. Redox modification of ryanodine receptors contributes to sarcoplasmic reticulum Ca2+ leak in chronic heart failure. Circ Res. (2008) 103:1466–72. doi: 10.1161/CIRCRESAHA.108.184457
30. van Oort RJ, McCauley MD, Dixit SS, Pereira L, Yang Y, Respress JL, et al. Ryanodine receptor phosphorylation by calcium/calmodulin-dependent protein kinase II promotes life-threatening ventricular arrhythmias in mice with heart failure. Circulation. (2010) 122:2669–79. doi: 10.1161/CIRCULATIONAHA.110.982298
31. Sun QA, Hess DT, Nogueira L, Yong S, Bowles DE, Eu J, et al. Oxygen-coupled redox regulation of the skeletal muscle ryanodine receptor-Ca2+ release channel by NADPH oxidase 4. Proc Natl Acad Sci U S A. (2011) 108:16098–103. doi: 10.1073/pnas.1109546108
32. Zhang C, Mo M, Ding W, Liu W, Yan D, Deng J, et al. High-mobility group box 1 (HMGB1) impaired cardiac excitation-contraction coupling by enhancing the sarcoplasmic reticulum (SR) Ca(2+) leak through TLR4-ROS signaling in cardiomyocytes. J Mol Cell Cardiol. (2014) 74:260–73. doi: 10.1016/j.yjmcc.2014.06.003
33. Dibb KM, Eisner DA, Trafford AW. Regulation of systolic [Ca2+]i and cellular Ca2+ flux balance in rat ventricular myocytes by SR Ca2+, L-type Ca2+ current and diastolic [Ca2+]i. J Physiol. (2007) 585:579–92. doi: 10.1113/jphysiol.2007.141473
34. Bogdanov P, Sola-Adell C, Hernandez C, Garcia-Ramirez M, Sampedro J, Simo-Servat O, et al. Calcium dobesilate prevents the oxidative stress and inflammation induced by diabetes in the retina of db/db mice. J Diabetes Complications. (2017) 31:1481–90. doi: 10.1016/j.jdiacomp.2017.07.009
35. Foster RR, Hole R, Anderson K, Satchell SC, Coward RJ, Mathieson PW, et al. Functional evidence that vascular endothelial growth factor may act as an autocrine factor on human podocytes. Am J Physiol Renal Physiol. (2003) 284:F1263–73. doi: 10.1152/ajprenal.00276.2002
36. Ferrara N, Gerber HP, LeCouter J. The biology of VEGF and its receptors. Nat Med. (2003) 9:669–76. doi: 10.1038/nm0603-669
Keywords: calcium dobesilate, cardiomyocytes, calcium sparks, hyperglycemia, laser scanning confocal microscope
Citation: Deng J, Cai X, Hao M, Liu X, Chen Z, Li H, Liu J, Liao Y, Fu H, Chen H, Qin G and Yan D (2021) Calcium Dobesilate (CaD) Attenuates High Glucose and High Lipid-Induced Impairment of Sarcoplasmic Reticulum Calcium Handling in Cardiomyocytes. Front. Cardiovasc. Med. 8:637021. doi: 10.3389/fcvm.2021.637021
Received: 02 December 2020; Accepted: 11 January 2021;
Published: 02 February 2021.
Edited by:
Yuli Huang, Southern Medical University, ChinaReviewed by:
Hongwen Zhu, Lanzhou University, ChinaMingqing Dong, Chengdu Fifth People's Hospital, China
Copyright © 2021 Deng, Cai, Hao, Liu, Chen, Li, Liu, Liao, Fu, Chen, Qin and Yan. This is an open-access article distributed under the terms of the Creative Commons Attribution License (CC BY). The use, distribution or reproduction in other forums is permitted, provided the original author(s) and the copyright owner(s) are credited and that the original publication in this journal is cited, in accordance with accepted academic practice. No use, distribution or reproduction is permitted which does not comply with these terms.
*Correspondence: Jianxin Deng, cheehsin@126.com; kinsum666@email.szu.edu.cn; Dewen Yan, yandw963@126.com
†These authors have contributed equally to this work