- 1School of Medicine, Shandong First Medical University, Jinan, China
- 2Shandong Provincial Hospital Affliated to Shandong First Medical University, Jinan, China
Objectives: Perivascular adipose tissue plays a key role in atherosclerosis, but its effects on the composition of carotid atherosclerotic plaques are unknown. This study aimed to investigate the association between inflammatory carotid artery and intraplaque hemorrhage (IPH) in the carotid artery.
Methods: This is a single-center retrospective study. Carotid inflammation was assessed by perivascular fat density (PFD) in 72 participants (mean age, 65.1 years; 56 men) who underwent both computed tomography angiography (CTA) and magnetic resonance imaging (MRI) within 2 weeks. The presence of IPH was assessed with MRI. Carotid stenosis, maximum plaque thickness, calcification, and ulceration were evaluated through CTA. The association between PFD and the occurrence of IPH was studied using generalized estimating equations analysis.
Results: Of 156 plaques, 72 plaques (46.2%) had IPH. Plaques with IPH showed higher PFD than those without [−41.4 ± 3.9 vs. −55.8 ± 6.5 Hounsfield unit (HU); p < 0.001]. After age, calcification, degree of stenosis, maximum plaque thickness, and ulceration were adjusted for, PFD (OR, 1.96; 95% CI, 1.41–2.73; p < 0.001) was found to be strongly associated with the presence of IPH.
Conclusions: A higher PFD is associated with the presence of IPH in the carotid artery. These findings may provide a novel marker to identify carotid IPH and risk stratification.
Introduction
Carotid atherosclerotic plaque is a major cause of cerebrovascular disease worldwide (1, 2). In people aged 30–79 years in 2020, the global prevalence of carotid plaque was estimated to be 21.1% (3). Recent studies have found that the composition of atherosclerotic plaque could define the severity of carotid atherosclerosis beyond vascular stenosis, particularly intraplaque hemorrhage (IPH) (4, 5). It has been proved that IPH can promote plaque progression and instability, thereby increasing the risk of occurrence and recurrence of cerebrovascular events (6). Therefore, understanding the pathology of IPH in clinical practice is important, especially after magnetic resonance imaging (MRI) emerged as a non-invasive image modality for IPH detection (7).
To date, many studies think that IPH is caused by the rupture of immature neoangiogenesis (8, 9). The pathologically impaired neovessels can result from vascular inflammation and oxidative stress (9). Perivascular adipose tissue (PVAT) can carry inflammatory components that increase vascular oxidative and vascular inflammation, suggesting PVAT may play a role in the occurrence of IPH (10). However, the specific participation of PVAT in the mechanism of IPH remains unclear. Most currently, the function of PVAT in vascular disease pathogeny has been well-accepted and supported by clinical evidence (11). In addition, an increase in perivascular fat density (PFD) is closely related to PVAT inflammatory changes (12). Saba et al. (13) have recently reported that the carotid PFD that is evaluated by computed tomography angiography (CTA) is linked to contrast plaque enhancement, which is associated with plaque inflammation. An additional study found that the carotid PFD as a marker of vulnerable plaques is closely linked to cerebrovascular ischemic events (14). To the best of our knowledge, few studies have investigated the relationship between carotid PFD and the presence of IPH.
In clinical practice, antithrombotic treatment is used to prevent the risk from IPH, including subsequent cardiovascular events caused (15). However, the recent findings have shown that the use of antithrombotic treatment increases the frequency of IPH in carotid plaques (16). To avoid this paradoxical situation, studying the relationship between pericarotid fat inflammation and IPH and thus proposing a theoretical basis for novel treatment are warranted.
This study aimed to investigate the association between carotid inflammatory, as assessed by PFD, and the presence of IPH in the carotid artery.
Materials and Methods
Study Population
Institutional review board approval was obtained for all study procedures, and informed consent was waived because of the retrospective nature of the study. This is a single-center retrospective study. We screened consecutive patients who underwent both CTA and high-resolution vessel wall MRI examinations for suspected atherosclerotic disease of the carotid arteries from January 2018 to December 2020 at Shandong Provincial Hospital Affiliated to Shandong First Medical University. Most patients are examined because of symptoms, such as stroke. The rest of the patients were asymptomatic, and the abnormalities were accidentally found by ultrasonography. The inclusion criterion was that the interval between the two imaging examinations was within 2 weeks. Exclusions criteria were as follows: (1) diseases other than atherosclerotic disease (i.e., dissection); (2) history of carotid endarterectomy and stenting; and (3) CTA and magnetic resonance (MR) images with poor quality. Demographic and clinical data including age, sex, body mass index (BMI), hypertension, hyperlipidemia, diabetes, smoking, coronary artery disease (CAD), antihypertension use, statin utilization, and antiplatelet use were collected from medical record. If patients had a transient ischemic attack or stroke occurring in the vascular territory supplied by the index carotid artery, plaques in that carotid vascular were considered symptomatic. If plaques are not in that vascular or in the vascular of asymptomatic patients, they were considered asymptomatic. Patients were considered asymptomatic if they had no cerebrovascular symptoms in the past 6 months.
MRI
The high-resolution MRI was performed on a whole-body 3.0-T MR scanner (Ingenia, Philips Healthcare, Best, the Netherlands) with a standard 64-channel head–neck coil. The high-resolution vessel wall MRI protocol was employed with the following parameters: for three-dimensional time-of-flight (3D TOF), fast field echo (FFE), repeat time (TR)/echo time (TE) = 20 ms/3.27 ms, field of view (FOV) = 230 mm × 230 mm, matrix = 256 × 256, and slice thickness = 1 mm; for two-dimensional (2D) T2-weighed imaging, turbo spin echo (TSE), TR/TE = 1,000 ms/26 ms, FOV = 140 mm × 140 mm, matrix = 256 × 256, and slice thickness = 2 mm; for 2D T1-weighted imaging, TSE, TR/TE = 2,500 ms/60 ms, FOV = 140 mm × 140 mm, matrix = 256 × 256, and slice thickness = 2 mm; and for magnetization-prepared rapid acquisition gradient echo (MPRAGE), FFE, TR/TE = 8.8 ms/5.3 ms, flip angle = 15°, FOV = 140 mm × 140 mm, matrix = 256 × 256, and slice thickness = 1 mm.
CTA Protocol
CTA examination was performed on a third-generation dual-source CT scanner (SOMATOM Force; Siemens Healthineers, Erlangen, Germany). CTA studies were obtained in a helical scanning mode with coverage from the aortic arch to skull vertex. A 60- to 80-ml volume of contrast media (Omnipaque-350, GE Healthcare) was injected at the speed of 4 ml/s, followed by 40 ml of saline flush, using a power injector. Bolus tracking was used to trigger the acquisition 5 s after an attenuation threshold of 100 Hounsfield units (HU) was reached into the aortic arch. The carotid CTA scanning parameters were as follows: tube voltage of 100 kV, pitch of 1.0, reconstructed slice thickness of 0.5 mm, reconstructed slice interval of 0.5 mm, and rotation time of 350 ms.
Image Analysis
All images were independently evaluated by two radiologists with more than 10 years' experience in vascular imaging, both of whom were blinded to patient information, with any disagreement in assessment being resolved by consensus.
The carotid plaque is defined as a vessel wall thickness of more than 1.5 mm or more encroaching into the lumen or at least 0.5 mm or 50% compared with the surrounding carotid thickness values (1, 3). Carotid IPH was defined by MPRAGE-positive plaque with at least one voxel, demonstrating 1.5 times higher signal intensity relative to adjacent sternocleidomastoid muscle in MRI (17). The kappa values of the IPH parameters within inter-observer and intra-observer were 0.91 (95 CI%, 0.263–1.557) and 0.97 (95 CI%, 0.935–1.005), respectively.
The measurements of CTA markers including the presence of calcification, degree of luminal stenosis, maximum plaque thickness, and occurrence of ulceration were obtained by using post-processing workstation (syngo.via, Siemens Force, Germany). The degree of stenosis was determined in accordance with the North American Symptomatic Carotid Endarterectomy Trial (NASCET) criteria on CTA (18). Plaque ulceration was defined as the presence of at least 2 mm of contrast media outpouching into the plaque on any single plane (19).
The density of the perivascular fat surrounding the carotid plaque was analyzed in a dedicated software (Perivascular Fat Analysis Tool, Shukun Technology, Beijing, China). Perivascular fat was defined as the adipose tissue surrounding the carotid artery, whose radial distance from the outer vessel wall is equal to the diameter of the vessel (Figure 1) (11). We adapted an established approach used in the coronary arteries and measured the density of the perivascular fat in a semi-automated manner by tracking the contour of vascular stenosis (11). PFD was determined by quantifying the weighed perivascular fat attenuation after adjusting for technical parameters on the basis of the attenuation histogram of perivascular fat within the range −190 to −30 HU. All fat density measurements are reported in HU. The analysis of the PVAT of plaques with and without IPH is shown in Figure 2.
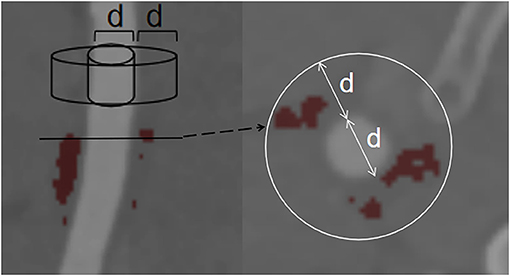
Figure 1. Example of PVAT phenotyping around the carotid artery. Perivascular fat was defined as fat within a radial distance equal to the diameter (d) of the vessel. PVAT, perivascular adipose tissue.
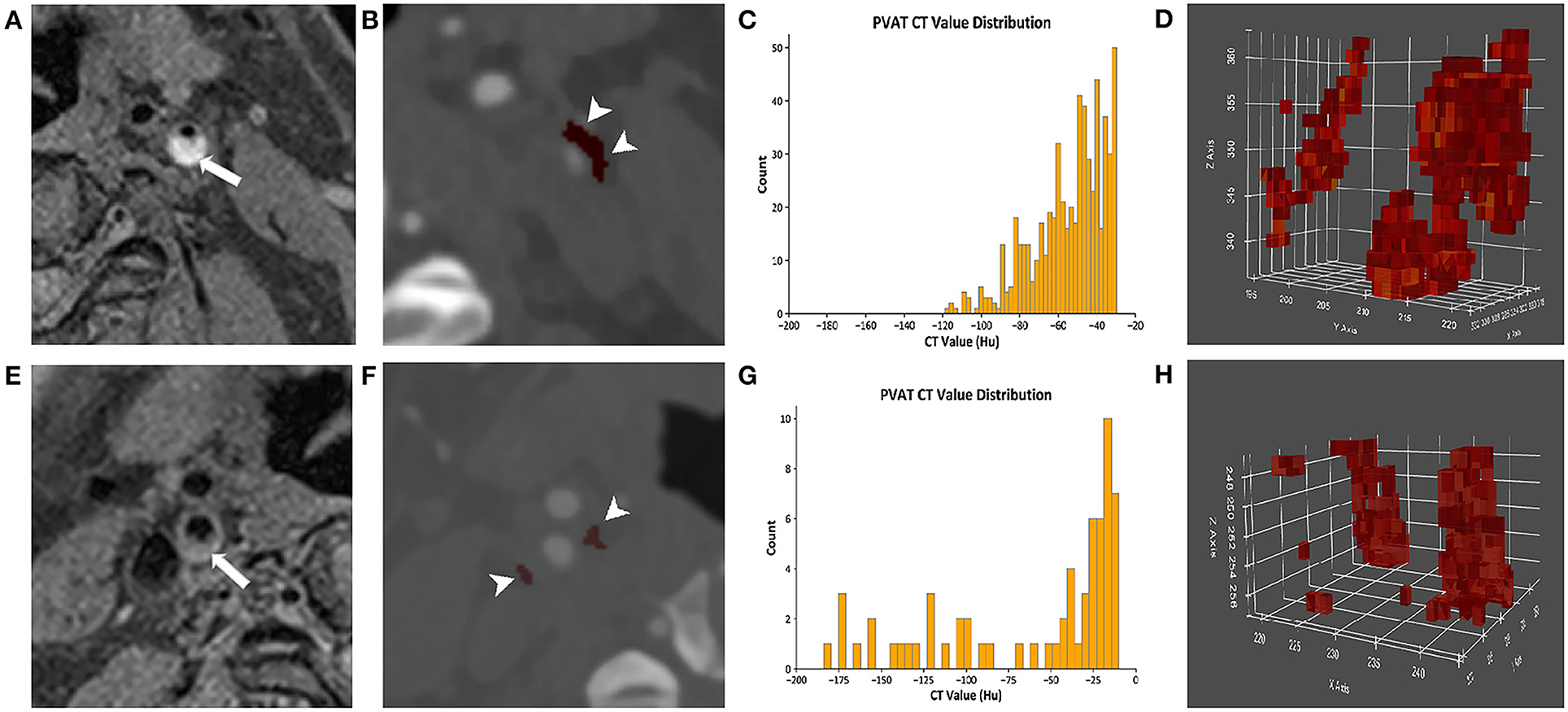
Figure 2. The analysis of the PVAT of plaques with and without IPH. (A–D) Come from the same patient. (A) A presence of IPH (arrow) was identified on MRI. (B) The PVAT (arrowhead) surrounding carotid plaque with IPH was visualized on CTA by red pixels. (C) The histogram of PVAT density of plaque with IPH was shown. (D) The pixel diagram of PVAT density of plaque with IPH was shown. (E–H) Come from the same patient. (E) The plaques without IPH (arrow) were identified on MRI. (F) The PVAT (arrowhead) surrounding carotid plaque without IPH was visualized on CTA by red pixels. (G) The histogram of PVAT density of plaque without IPH was shown. (H) The pixel diagram of PVAT density of plaque without IPH was shown. PVAT, perivascular adipose tissue; IPH, intraplaque hemorrhage.
Statistical Analysis
Continuous variables are described as mean ± standard deviation (SD), and categorical variables are presented as a percentage. Clinical information and carotid plaque characteristics were compared between plaques with and without IPH by using t-test and χ2 test. Carotid plaque characteristics were compared between plaques with and without symptoms by using Student's t-test and χ2 test. Correlation coefficients were calculated between the maximum plaque thickness and PFD by Pearson analysis. Correlation analysis was assessed between the ulceration, calcification, and PFD by t-test. Logistic regression analysis with generalized estimating equation correction was used to calculate the odds ratio (OR) and corresponding 95% confidence interval (CI) of carotid PFD in discriminating the presence of IPH in the carotid artery. The association between PFD and IPH was analyzed into three models: model 1, adjusted for age; model 2, adjusted for age, calcification, degree of stenosis, maximum plaque thickness, and ulceration; model 3, adjusted for age, BMI, hypertension, hyperlipidemia, diabetes, calcification, degree of stenosis, maximum plaque thickness, and ulceration. Statistical significance was considered at p < 0.05. All statistical analyses were performed by using SPSS 22.0 (IBM, Chicago, IL).
Results
Of 101 subjects who underwent both CTA and high-resolution MRI, 29 were excluded due to normal carotid imaging (n = 8), carotid dissection (n = 3), poor image quality (n = 12), and history of stenting (n = 6). Of 72 eligible patients (mean age, 65.1 ± 9.1 years), 56 (77.8%) were male, 48 (66.7%) had hypertension, 34 (47.2%) had hyperlipidemia, 20 (27.8%) had diabetes, 36 (50%) had smoke, and 26 (36.1%) had CAD. The majority was on medical therapy (47.2% on antihypertension, 47.2% on statin, and 56.9% on antiplatelet). The demographic and clinical characteristics are summarized in Table 1.
In this population, 156 plaques were found in the carotid arteries. Of all 156 plaques, 72 (46.2%) had IPH, 118 (75.6%) had calcification, and 10 (6.4%) had ulceration. As shown in Table 2, compared with the carotid plaques without IPH, those with IPH tended to be older (70.8 ± 3.9 vs. 62.4 ± 7.4 years; p < 0.001). No significant difference was founded in other clinical information between plaques with and without IPH (p > 0.05). Compared with the carotid plaques without IPH, those with IPH showed a higher prevalence of ulceration (12.5% vs. 1.2%; p = 0.004), greater stenosis (43.9 ± 21.2% vs. 34.5 ± 16.8%; p = 0.002), and greater maximum plaque thickness (3.9 ± 1.5 vs. 2.9 ± 1.0 mm; p < 0.001). Calcification was frequently present in the plaques without IPH compared with those with IPH (66.7 vs. 83.3%; p = 0.016). The carotid plaques with IPH had higher PFD (−41.4 ± 3.9 vs. −55.8 ± 6.5 HU; p < 0.001) than those without IPH. In this population, 47 patients had symptoms, including 22 patients with stroke and 25 patients with transient ischemic attack. Of all 156 plaques, there were 50 plaques without symptoms and 106 plaques with symptoms. Compared with carotid plaques without symptoms, those with symptoms had greater maximum plaque thickness (3.6 ± 1.4 vs. 2.9 ± 1.1 mm; p < 0.001), higher PFD (−33.5 ± 11.6 vs. −54.1 ± 8.9 HU; p < 0.001), and higher prevalence of IPH (65.1 vs. 4%; p < 0.001).
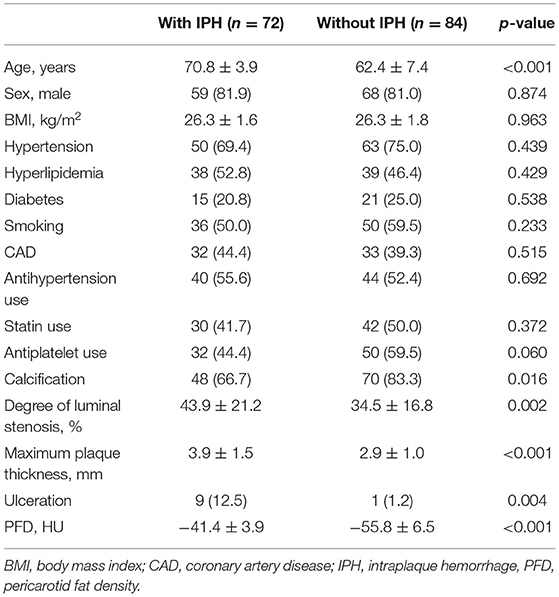
Table 2. Comparison of clinical risk factors and CTA characteristics between plaques with and without IPH (n = 156).
In addition, there was a statistically significant positive correlation between maximum plaque thickness and PFD (r = 0.292, p < 0.001). We found increased PFD around the plaques without calcification compared with the plaques with calcification (p < 0.05). And there was no obvious differences in PFD around plaques with or without ulceration (p > 0.05).
In univariate logistic regression analysis, the PFD (OR, 1.70; 95% CI, 1.43–2.04; p < 0.001) was found to be strongly associated with the presence of IPH. Multivariate logistic regression revealed that the association between PDF (OR, 1.64; 95% CI, 1.36–1.97; p < 0.001) and the occurrence of IPH remained statistically significant, after adjusting for age (model 1). After calcification, degree of stenosis, maximum plaque thickness, and ulceration (model 2) were further adjusted for, the association of PFD (OR, 1.96; 95% CI, 1.41–2.73; p < 0.001) with IPH remained significant. After age, BMI, hypertension, hyperlipidemia, diabetes, calcification, degree of stenosis, maximum plaque thickness, and ulceration (model 3) were adjusted for, the association of PFD (OR, 2.33; 95% CI, 1.46–3.72; p < 0.001) with IPH remained significant (Table 3).
We also performed the same patient analysis comparing pericarotid fat density differences between the carotid arteries on the contralateral and same sides. We found that the PFD around the plaques with IPH was higher than that around the contralateral plaques without IPH (−35.5 ± 2.4 vs. −55.6 ± 0.6 HU; p < 0.05). In addition, there was no obvious differences in PFD between the left carotid plaques with IPH and contralateral plaques with IPH (−29.8 ± 7.4 vs. −27.6 ± 8.2 HU; p > 0.05) and between the left carotid plaques without IPH and contralateral plaques without IPH (−47.6 ± 7.3 vs. −49.4 ± 5.6 HU; p > 0.05). In one vessel, the carotid plaques with IPH had higher PFD than those without IPH (−34.9 ± 2.0 vs. −48.7 ± 5.5 HU; p < 0.05).
Discussion
This study investigated the relationship between the PFD and IPH in carotid plaques. We found that the PFD was significantly associated with the presence of IPH before and after adjusting for confounding factors. Our findings indicate that the PFD might be independent indicators for IPH in carotid plaques.
In this study, we found that the plaques with IPH had greater stenosis and maximum plaque thickness than those without IPH. This finding can be ascribed to the fact that IPH can induce inflammation to accelerate plaque growth and thus result in lumen narrowing and plaque thickening (20). These findings are consistent with previous studies (21, 22). Eisenmenger et al. (21) reported that carotid stenosis is worse in plaques with IPH than in those without (53.5 vs. 24.9%; p < 0.001) and that the maximum plaque thickness is higher in groups positive for IPH than in groups negative for IPH (5.93 vs. 3.42; p < 0.001). The strong association between ulceration and IPH in the present study agrees with previous findings (19, 23). A recent study has shown a significantly higher prevalence of ulceration (87.3 vs. 31.8%; p < 0.001) in plaques with IPH than in those without (23). In the present study, the carotid plaques without IPH showed a higher prevalence of calcification than those with IPH. This finding is consistent with those of previous studies (24, 25). Some researchers found that asymptomatic patients have more calcium deposits in carotid plaques than symptomatic ones, supporting that calcification is a protective factor (24). However, the association between calcification and IPH is not uniform in several studies (22, 26). Lin et al. (22) reported that calcification is usually accompanied by IPH compared to those without (87.5 vs. 55.9%; p < 0.05). Microcalcifications are difficult to detect with MRI in their studies. The CTA that we used has higher accuracy in detecting calcification. The different examination modality and the number of patients may account for the difference. Moreover, in recent studies about carotid PFD, several researchers reported the association between contrast plaque enhancement and cerebrovascular ischemic events with PFD (13, 14). However, they did not use software specifically designed to measure carotid PFD. To our knowledge, this study is the first to investigate the association between carotid PFD and the presence of IPH.
The relationship between the PVAT and IPH is complex and likely bidirectional. To date, the pathophysiology of IPH is not completely clear, but the prevailing viewpoints are that IPH develops by rupture of the immature neovessels (8, 9). A wide variety of bioactive molecules from PVAT can diffuse directly into the vascular wall in a paracrine way, thus directly affecting its biology, including inflammation and oxidative stress (10). In advanced atherosclerotic diseases, inflammation and oxidative stress can lead to the secretion of the vascular endothelial growth factor (VEGF) and thus increase immature neoangiogenesis (9). These neovessels, which lack smooth muscle cells and endothelial gap junctions, are pathological and impaired and thus are prone to rupture (27). The rupture of these neovessels results in the formation of IPH. Conversely, some studies have recently shown that the inflamed human vascular wall caused by IPH also releases inflammatory cytokines, which also disseminate into the perivascular space, triggering PVAT inflammatory changes (28, 29). These PVAT changes can be assessed by differences in the fat attenuation as measured by HU on CT. In addition, increased PFD is closely associated with histopathological markers of inflammation (30). Therefore, the PFD is closely associated with the occurrence of IPH.
The role of PVAT in the pathogenesis of vascular diseases is presently well-accepted and supported by translational and clinical evidence (11, 31, 32). Oikonomou et al. (11) reported that the perivascular fat attenuation index, which can capture coronary inflammation, can predict and stratify cardiovascular risk. Thus, this index can serve as a guide for early targeted prevention. Systemic markers of inflammation, including C-reaction protein or proinflammatory cytokines, have been associated with cardiovascular risk prediction, but they are often driven by other inflammatory conditions and unable to pinpoint local inflammation-induced changes (29, 33). Conversely, measuring PFD in CTA is helpful in identifying localized perivascular inflammation (34, 35). Positron emission tomography (PET) imaging is the gold standard in evaluating PVAT inflammation assessed by FDG uptake, but its use is limited by its high exposure, high cost, and low clinical availability (36). Recent studies have reported that local coronary inflammation associated with vulnerable plaques can be detected by using standard non-invasive CTA modality, providing information similar to 18F-NaF PET-CT (37). In addition, CTA is more cost-effective than PET.
IPH, a compositional characteristics of vulnerable plaques, contributes to plaque progression and rupture, thus increasing the risk of cerebrovascular ischemic events (5, 6, 38). Recently, several studies have shown that the use of antithrombotic treatment is associated with a higher frequency of IPH in the carotid atherosclerotic plaques (16, 39). These findings seem to contradict current knowledge because antithrombotic treatment is used to reduce the risk from IPH, including subsequent cardiovascular events caused (15, 40). In the present study, we found the association between IPH and carotid inflammation, providing a theoretical basis for new targeted anti-inflammatory therapy. At present, considerable studies have focused on preventing atherosclerosis by intervening in PVAT (41, 42). Therefore, PVAT may be emerging as the therapeutic target for IPH.
Several limitations of our study should be noted. First, this is a retrospective study, and the cause–effect of perivascular inflammation and IPH cannot be determined. Future prospective studies could test this causality relationship. Second, histological validation of IPH remains the goal standard, but this was not available in this study. However, MRI is currently the best in vivo modality for IPH, and the technique used in this study has previously been validated in comparison with histology (7). Third, the range of PFD measurements was uncertain, and our study adapted an established method used in coronary arteries (11). Finally, we cannot obtain clinical data on specific measurements, such as blood pressure and laboratory data from clinical records in our study. The patients were indeed hypertensive or hyperlipidemic, but specific measurements were not recorded in the medical records for some reasons. For example, the laboratory data of several patients were done in other hospital, and the clinical doctors only recorded whether they had hypertension or hyperlipidemia and did not record specific measurements in the medical records.
In conclusion, our study shows the PFD is independently associated with IPH in the carotid artery, suggesting that PVAT may play a key role in the occurrence of IPH. Our findings may provide a novel marker to identify carotid IPH and risk stratification.
Data Availability Statement
The original contributions presented in the study are included in the article/supplementary material, further inquiries can be directed to the corresponding author/s.
Ethics Statement
The studies involving human participants were reviewed and approved by Medical Ethics Committee of Shandong Provincial Hospital. Written informed consent for participation was not required for this study in accordance with the national legislation and the institutional requirements. Written informed consent was not obtained from the individual(s) for the publication of any potentially identifiable images or data included in this article.
Author Contributions
SZ and BK have substantial contributions to the conception or design of the work and to the acquisition, analysis, and interpretation of data for the work. HG, XYu, and XYua have substantial contributions to drafting the work or revising it critically for important intellectual content. XW has substantial contributions to the final approval of the version to be published and agreed to be accountable for all aspects of the work in ensuring that questions related to the accuracy or integrity of any part of the work are appropriately investigated and resolved. All authors contributed to the article and approved the submitted version.
Funding
The present study was supported by the National Natural Science Foundation of China (grant nos. 8187354, 81571672, and 81371548) and Academic Promotion Programme of Shandong First Medical University (grant no. 2019QL023).
Conflict of Interest
The authors declare that the research was conducted in the absence of any commercial or financial relationships that could be construed as a potential conflict of interest.
Publisher's Note
All claims expressed in this article are solely those of the authors and do not necessarily represent those of their affiliated organizations, or those of the publisher, the editors and the reviewers. Any product that may be evaluated in this article, or claim that may be made by its manufacturer, is not guaranteed or endorsed by the publisher.
References
1. Mozaffarian D. Global scourge of cardiovascular disease: time for health care systems reform and precision population health. J Am Coll Cardiol. (2017) 70:26–8. doi: 10.1016/j.jacc.2017.05.007
2. Piepoli MF, Hoes AW, Agewall S, Albus C, Brotons C, Catapano AL, et al. 2016 European Guidelines on cardiovascular disease prevention in clinical practice: the sixth joint task force of the european society of cardiology and other societies on cardiovascular disease prevention in clinical practice (constituted by representatives of 10 societies and by invited experts) developed with the special contribution of the european association for cardiovascular prevention & rehabilitation (EACPR). Eur Heart J. (2016) 37:2315–81. doi: 10.1093/eurheartj/ehw106
3. Song P, Fang Z, Wang H, Cai Y, Rahimi K, Zhu Y, et al. Global and regional prevalence, burden, and risk factors for carotid atherosclerosis: a systematic review, meta-analysis, and modelling study. Lancet Glob Health. (2020) 8:721–9. doi: 10.1016/S2214-109X(20)30117-0
4. Saba L, Saam T, Jäger HR, Yuan C, Hatsukami TS, Saloner D, et al. Imaging biomarkers of vulnerable carotid plaques for stroke risk prediction and their potential clinical implications. Lancet Neurol. (2019) 18:559–72. doi: 10.1016/S1474-4422(19)30035-3
5. Brunner G, Virani SS, Sun W, Liu L, Dodge RC, Nambi V, et al. Associations between carotid artery plaque burden, plaque characteristics, and cardiovascular events: the ARIC carotid magnetic resonance imaging study. JAMA Cardiol. (2021) 6:79–86. doi: 10.1001/jamacardio.2020.5573
6. Schindler A, Schinner R, Altaf N, Hosseini AA, Simpson RJ, Esposito-Bauer L, et al. Prediction of stroke risk by detection of hemorrhage in carotid plaques: meta-analysis of individual patient data. JACC Cardiov Imag. (2020) 13:395–406. doi: 10.1016/j.jcmg.2019.03.028
7. van den Bouwhuijsen QJ, Vernooij MW, Hofman A, Krestin GP, van der Lugt A, Witteman JC. Determinants of magnetic resonance imaging detected carotid plaque components: the rotterdam study. Eur Heart J. (2012) 33:221–9. doi: 10.1093/eurheartj/ehr227
8. Michel JB, Virmani R, Arbustini E, Pasterkamp G. Intraplaque haemorrhages as the trigger of plaque vulnerability. Eur Heart J. (2011) 32:1977–85. doi: 10.1093/eurheartj/ehr054
9. Chistiakov DA, Orekhov AN, Bobryshev YV. Contribution of neovascularization and intraplaque haemorrhage to atherosclerotic plaque progression and instability. Acta Physiol. (2015) 213:539–53. doi: 10.1111/apha.12438
10. Margaritis M, Sanna F, Lazaros G, Akoumianakis I, Patel S, Antonopoulos AS, et al. Predictive value of telomere length on outcome following acute myocardial infarction: evidence for contrasting effects of vascular vs. blood oxidative stress. Eur Heart J. (2017) 38:3094–104. doi: 10.1093/eurheartj/ehx177
11. Oikonomou EK, Marwan M, Desai MY, Mancio J, Alashi A, Hutt Centeno E, et al. Non-invasive detection of coronary inflammation using computed tomography and prediction of residual cardiovascular risk (the CRISP CT study): a post-hoc analysis of prospective outcome data. Lancet. (2018) 392:929–39. doi: 10.1016/S0140-6736(18)31114-0
12. Antoniades C, Antonopoulos AS, Deanfield J. Imaging residual inflammatory cardiovascular risk. Eur Heart J. (2020) 41:748–58. doi: 10.1093/eurheartj/ehz474
13. Saba L, Zucca S, Gupta A, Micheletti G, Suri JS, Balestrieri A, et al. Perivascular fat density and contrast plaque enhancement: does a correlation exist? AJNR Am J Neuroradiol. (2020) 41:1460–5. doi: 10.3174/ajnr.A6710
14. Baradaran H, Myneni PK, Patel P, Askin G, Gialdini G, Al-Dasuqi K, et al. Association between carotid artery perivascular fat density and cerebrovascular ischemic events. J Am Heart Assoc. (2018) 7:e010383. doi: 10.1161/JAHA.118.010383
15. Aboyans V, Ricco JB, Bartelink MEL, Björck M, Brodmann M, Cohnert T, et al. 2017 ESC guidelines on the diagnosis and treatment of peripheral arterial diseases, in collaboration with the European society for vascular surgery (ESVS): document covering atherosclerotic disease of extracranial carotid and vertebral, mesenteric, renal, upper and lower extremity arteriesEndorsed by: the European stroke organization (ESO)the task force for the diagnosis and treatment of peripheral arterial diseases of the European society of cardiology (ESC) and of the European society for vascular surgery (ESVS). Eur Heart J. (2018) 39:763–816. doi: 10.1093/eurheartj/ehx095
16. Mujaj B, Bos D, Muka T, Lugt AV, Ikram MA, Vernooij MW, et al. Antithrombotic treatment is associated with intraplaque haemorrhage in the atherosclerotic carotid artery: a cross-sectional analysis of the rotterdam study. Eur Heart J. (2018) 39:3369–76. doi: 10.1093/eurheartj/ehy433
17. Liu Y, Wang M, Zhang B, Wang W, Xu Y, Han Y, et al. Size of carotid artery intraplaque hemorrhage and acute ischemic stroke: a cardiovascular magnetic resonance chinese atherosclerosis risk evaluation study. J Cardiovasc Magn Reson. (2019) 21:36. doi: 10.1186/s12968-019-0548-1
18. North American Symptomatic Carotid Endarterectomy Trial Collaborators, Barnett HJM, Taylor DW, Haynes RB, Sackett DL, Peerless SJ, et al. Beneficial effect of carotid endarterectomy in symptomatic patients with high-grade carotid stenosis. N Engl J Med. (1991) 325:445–53. doi: 10.1056/NEJM199108153250701
19. U-King-Im JM, Fox AJ, Aviv RI, Howard P, Yeung R, Moody AR, et al. Characterization of carotid plaque hemorrhage: a CT angiography and MR intraplaque hemorrhage study. Stroke. (2010) 41:1623–9. doi: 10.1161/STROKEAHA.110.579474
20. Lu M, Peng P, Cui Y, Qiao H, Li D, Cai J, et al. Association of progression of carotid artery wall volume and recurrent transient ischemic attack or stroke: a magnetic resonance imaging study. Stroke. (2018) 49:614–20. doi: 10.1161/STROKEAHA.117.019422
21. Eisenmenger LB, Aldred BW, Kim SE, Stoddard GJ, de Havenon A, Treiman GS, et al. Prediction of carotid intraplaque hemorrhage using adventitial calcification and plaque thickness on CTA. AJNR Am J Neuroradiol. (2016) 37:1496–503. doi: 10.3174/ajnr.A4765
22. Lin R, Chen S, Liu G, Xue Y, Zhao X. Association between carotid atherosclerotic plaque calcification and intraplaque hemorrhage: a magnetic resonance imaging study. Arterioscler Thromb Vasc Biol. (2017) 37:1228–33. doi: 10.1161/ATVBAHA.116.308360
23. Yang J, Pan X, Zhang B, Yan Y, Huang Y, Woolf AK, et al. Superficial and multiple calcifications and ulceration associate with intraplaque hemorrhage in the carotid atherosclerotic plaque. Eur Radiol. (2018) 28:4968–77. doi: 10.1007/s00330-018-5535-7
24. Kwee RM. Systematic review on the association between calcification in carotid plaques and clinical ischemic symptoms. J Vasc Surg. (2010) 51:1015–25. doi: 10.1016/j.jvs.2009.08.072
25. Huang H, Virmani R, Younis H, Burke AP, Kamm RD, Lee RT. The impact of calcification on the biomechanical stability of atherosclerotic plaques. Circulation. (2001) 103:1051–6. doi: 10.1161/01.CIR.103.8.1051
26. Teng Z, He J, Sadat U, Mercer JR, Wang X, Bahaei NS, et al. How does juxtaluminal calcium affect critical mechanical conditions in carotid atherosclerotic plaque? An exploratory study. IEEE Trans Biomed Eng. (2014) 61:35–40. doi: 10.1109/TBME.2013.2275078
27. Sluimer JC, Kolodgie FD, Bijnens AP, Maxfield K, Pacheco E, Kutys B, et al. Thin-walled microvessels in human coronary atherosclerotic plaques show incomplete endothelial junctions relevance of compromised structural integrity for intraplaque microvascular leakage. J Am Coll Cardiol. (2009) 53:1517–27. doi: 10.1016/j.jacc.2008.12.056
28. Takaoka M, Suzuki H, Shioda S, Sekikawa K, Saito Y, Nagai R, et al. Endovascular injury induces rapid phenotypic changes in perivascular adipose tissue. Arterioscler Thromb Vasc Biol. (2010) 30:1576–82. doi: 10.1161/ATVBAHA.110.207175
29. Margaritis M, Antonopoulos AS, Digby J, Lee R, Reilly S, Coutinho P, et al. Interactions between vascular wall and perivascular adipose tissue reveal novel roles for adiponectin in the regulation of endothelial nitric oxide synthase function in human vessels. Circulation. (2013) 127:2209–21. doi: 10.1161/CIRCULATIONAHA.112.001133
30. Antonopoulos AS, Sanna F, Sabharwal N, Thomas S, Oikonomou EK, Herdman L, et al. Detecting human coronary inflammation by imaging perivascular fat. Sci Transl Med. (2017) 9:eaal2658. doi: 10.1126/scitranslmed.aal2658
31. Hedgire S, Baliyan V, Zucker EJ, Bittner DO, Staziaki PV, Takx RAP, et al. Perivascular epicardial fat stranding at coronary CT angiography: a marker of acute plaque rupture and spontaneous coronary artery dissection. Radiology. (2018) 287:808–15. doi: 10.1148/radiol.2017171568
32. Goeller M, Achenbach S, Cadet S, Kwan AC, Commandeur F, Slomka PJ, et al. Pericoronary adipose tissue computed tomography attenuation and high-risk plaque characteristics in acute coronary syndrome compared with stable coronary artery disease. JAMA Cardiol. (2018) 3:858–63. doi: 10.1001/jamacardio.2018.1997
33. Ridker PM. C-reactive protein and the prediction of cardiovascular events among those at intermediate risk: moving an inflammatory hypothesis toward consensus. J Am Coll Cardiol. (2007) 49:2129–38. doi: 10.1016/j.jacc.2007.02.052
34. Konishi M, Sugiyama S, Sato Y, Oshima S, Sugamura K, Nozaki T, et al. Pericardial fat inflammation correlates with coronary artery disease. Atherosclerosis. (2010) 213:649–55. doi: 10.1016/j.atherosclerosis.2010.10.007
35. Lu MT, Park J, Ghemigian K, Mayrhofer T, Puchner SB, Liu T, et al. Epicardial and paracardial adipose tissue volume and attenuation - association with high-risk coronary plaque on computed tomographic angiography in the ROMICAT II trial. Atherosclerosis. (2016) 251:47–54. doi: 10.1016/j.atherosclerosis.2016.05.033
36. Mazurek T, Kobylecka M, Zielenkiewicz M, Kurek A, Kochman J, Filipiak KJ, et al. PET/CT evaluation of 18F-FDG uptake in pericoronary adipose tissue in patients with stable coronary artery disease: independent predictor of atherosclerotic lesions' formation? J Nucl Cardiol. (2017) 24:1075–84. doi: 10.1007/s12350-015-0370-6
37. Antoniades C, Kotanidis CP, Berman DS. State-of-the-art review article. Atherosclerosis affecting fat: What can we learn by imaging perivascular adipose tissue? J Cardiovasc Comput Tomogr. (2019) 13:288–96. doi: 10.1016/j.jcct.2019.03.006
38. McNally JS, McLaughlin MS, Hinckley PJ, Treiman SM, Stoddard GJ, Parker DL, et al. Intraluminal thrombus, intraplaque hemorrhage, plaque thickness, and current smoking optimally predict carotid stroke. Stroke. (2015) 46:84–90. doi: 10.1161/STROKEAHA.114.006286
39. Liem MI, Schreuder FH, van Dijk AC, de Rotte AA, Truijman MT, Daemen MJ, et al. Use of antiplatelet agents is associated with intraplaque hemorrhage on carotid magnetic resonance imaging: the plaque at risk study. Stroke. (2015) 46:3411–5. doi: 10.1161/STROKEAHA.115.008906
40. Kirchhof P, Benussi S, Kotecha D, Ahlsson A, Atar D, Casadei B, et al. 2016 ESC Guidelines for the management of atrial fibrillation developed in collaboration with EACTS. Eur Heart J. (2016) 37:2893–962. doi: 10.1093/eurheartj/ehw210
41. Xiong W, Zhao X, Villacorta L, Rom O, Garcia-Barrio MT, Guo Y, et al. Brown adipocyte-specific PPARγ (Peroxisome Proliferator-Activated Receptor γ) deletion impairs perivascular adipose tissue development and enhances atherosclerosis in mice. Arterioscler Thromb Vasc Biol. (2018) 38:1738–47. doi: 10.1161/ATVBAHA.118.311367
42. Skiba DS, Nosalski R, Mikolajczyk TP, Siedlinski M, Rios FJ, Montezano AC, et al. Anti-atherosclerotic effect of the angiotensin 1-7 mimetic AVE0991 is mediated by inhibition of perivascular and plaque inflammation in early atherosclerosis. Br J Pharmacol. (2017) 174:4055–69. doi: 10.1111/bph.13685
Keywords: atherosclerosis, carotid artery, intraplaque hemorrhage, perivascular fat, inflammation
Citation: Zhang S, Gu H, Yu X, Kang B, Yuan X and Wang X (2021) Association Between Carotid Artery Perivascular Fat Density and Intraplaque Hemorrhage. Front. Cardiovasc. Med. 8:735794. doi: 10.3389/fcvm.2021.735794
Received: 14 July 2021; Accepted: 23 August 2021;
Published: 20 September 2021.
Edited by:
Daiju Fukuda, Tokushima University, JapanReviewed by:
Guillaume Goudot, Institut National de la Santé et de la Recherche Médicale (INSERM), FranceYasutomi Higashikuni, University of Tokyo, Japan
Copyright © 2021 Zhang, Gu, Yu, Kang, Yuan and Wang. This is an open-access article distributed under the terms of the Creative Commons Attribution License (CC BY). The use, distribution or reproduction in other forums is permitted, provided the original author(s) and the copyright owner(s) are credited and that the original publication in this journal is cited, in accordance with accepted academic practice. No use, distribution or reproduction is permitted which does not comply with these terms.
*Correspondence: Ximing Wang, d3htaW5nMzY5QDE2My5jb20=