- 1Shantou University Medical College, Shantou, China
- 2Division of Cardiology, Guangdong Cardiovascular Institute, Guangdong Provincial Key Laboratory of Coronary Heart Disease Prevention, Guangdong Provincial People's Hospital, Guangdong Academy of Medical Sciences, Guangzhou, China
- 3Division of Geriatric Intensive Medicine, Guangdong Provincial Geriatrics Institute, Guangdong Provincial People Hospital, Guangdong Academy of Medical Sciences, Guangzhou, China
Ischemia with non-obstructive coronary arteries (INOCA) has gained increasing attention due to its high prevalence, atypical clinical presentations, difficult diagnostic procedures, and poor prognosis. There are two endotypes of INOCA—one is coronary microvascular dysfunction and the other is vasospastic angina. Diagnosis of INOCA lies in evaluating coronary flow reserve, microcirculatory resistance, and vasoreactivity, which is usually obtained via invasive coronary interventional techniques. Non-invasive diagnostic approaches such as echocardiography, single-photon emission computed tomography, cardiac positron emission tomography, and cardiac magnetic resonance imaging are also valuable for assessing coronary blood flow. Some new techniques (e.g., continuous thermodilution and angiography-derived quantitative flow reserve) have been investigated to assist the diagnosis of INOCA. In this review, we aimed to discuss the pathophysiologic basis and contemporary and novel diagnostic approaches for INOCA, to construct a better understanding of INOCA evaluation.
Introduction
Ischemia with non-obstructive coronary arteries (INOCA) is defined as the stenotic reduction in diameter <50% detected by investigation of coronary arteries, either invasive coronary angiography (CAG) or coronary CT angiography (CCTA), in patients with symptoms of myocardial ischemia such as angina or dyspnea following physical activities (1–5). This particular population accounted for 70% of all the patients presenting with evidence of myocardial ischemia that eventually underwent CAG (6, 7). There are approximately 3–4 million American women and men who suffered from stable INOCA (8, 9). Epidemiologic analysis also demonstrated an evidently higher percentage of female patients that suffered from INOCA (10–12).
Strong pieces of evidence have shown that patients with INOCA have a higher risk of heart failure with preserved ejection fraction, major adverse cardiovascular events (MACEs), and mortality (4, 10, 13, 14). Compared to patients with no apparent coronary artery disease (no stenosis > 20%), patients with non-obstructive coronary arteries (≥1 stenosis ≥ 20% but no stenosis ≥ 70%) suffered a 2- to 4.5-fold higher risk of myocardial infarction, while the risk of myocardial infarction and mortality was similar to patients with single-vessel obstruction (stenosis ≥ 70% or left the main stenosis ≥ 50%) (13). The prevalence of coronary microvascular dysfunction (CMD), which is one of the major pathological mechanisms of INOCA, was as high as 75% in heart failure with preserved ejection fraction (15). The degree of severity in CMD was also associated with markers of heart failure severity, such as right ventricular dysfunction and N-terminal pro-B-type natriuretic peptide, and the rate of major adverse cardiovascular events (MACE) and heart failure hospitalization (15, 16). Particularly, female patients with INOCA face higher risks of unfavorable prognosis (8, 17–19). The first-year risk for MACE was 2.43 times higher in women with INOCA when compared to men (18). Apart from the above, many patients with INOCA encountered issues on quality of life, exercise capacity, and frequent hospital visits due to unsatisfying symptoms control (20).
The pathophysiological mechanisms of INOCA include CMD and/or epicardial vasospasm (21–23). Confirmatory diagnosis of INOCA requires an assessment of coronary microvasculature via invasive coronary routes (24). Fractional flow reserve (FFR), coronary flow reserve (CFR), index of microcirculatory resistance (IMR), hyperemic myocardial velocity resistance (HMR), and vasoreactivity in response to vasoactive agents are commonly used to assess the epicardial and microvascular conditions, and help to classify different endotypes of INOCA (1). However, such a technique is time-consuming and usually not widely available in some facilities, and therefore may not be able to meet the large demands of indicated candidates (25). Non-invasive methods that visualize myocardial perfusion, such as positron emission tomography (PET), can assess microvasculature more quickly (26). Also, there are some new techniques in invasive methods, such as continuous thermodilution and quantitative flow ratio (QFR), that are under research and are promising in complementing the diagnostic process of INOCA (27–29). In this review, we aimed to discuss the pathophysiologic basis and contemporary and novel diagnostic approaches for INOCA, to construct a better understanding of INOCA evaluation.
Pathophysiologic Basis for Ischemia With Non-Obstructive Coronary Arteries
Coronary microcirculation refers to vessels smaller than 500 um in diameter, namely, the prearterioles (500–200 μm), arterioles (200–40 μm), and capillaries (<10 μm). The proximal segment to coronary microcirculation is the epicardial artery (500 μm to 5 mm), which is also known as the macrocirculation (Figure 1) (21, 30, 31). The pathophysiological mechanisms of INOCA include CMD and epicardial vasospasm that can overlap in some cases (21–23).
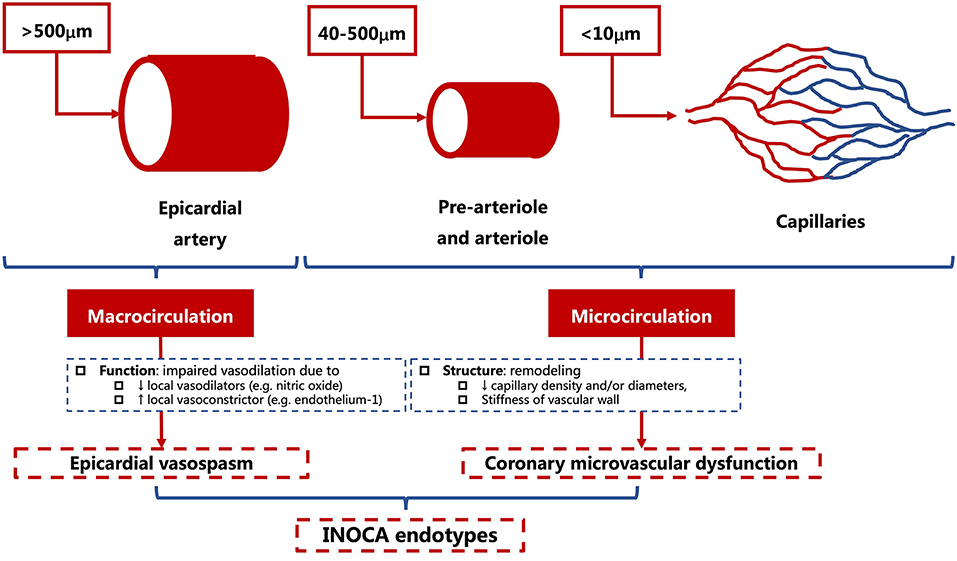
Figure 1. Anatomical structures and pathophysiological endotypes of INOCA. INOCA, ischemia with non-obstructive coronary arteries.
Coronary Microvascular Dysfunction
The endothelial function is crucial in regulating the vascular tone of coronary microcirculation and maintaining cardiovascular homeostasis (32). During physical activity, increased oxygen consumption and local metabolites serve as triggers for the release of vasoactive substances, such as nitric oxide (NO) (33–36). NO has an anti-inflammatory function and plays a crucial role in protecting the integrity of the endothelium.
The structural (e.g., vascular remodeling, etc.) and functional abnormalities (e.g., endothelial dysfunction, microvascular spams, etc.) are proposed to be associated with the development of CMD. These mechanisms interact and are attributable for reduced coronary flow reserve and increased microcirculatory resistance (Figure 2) (37–39).
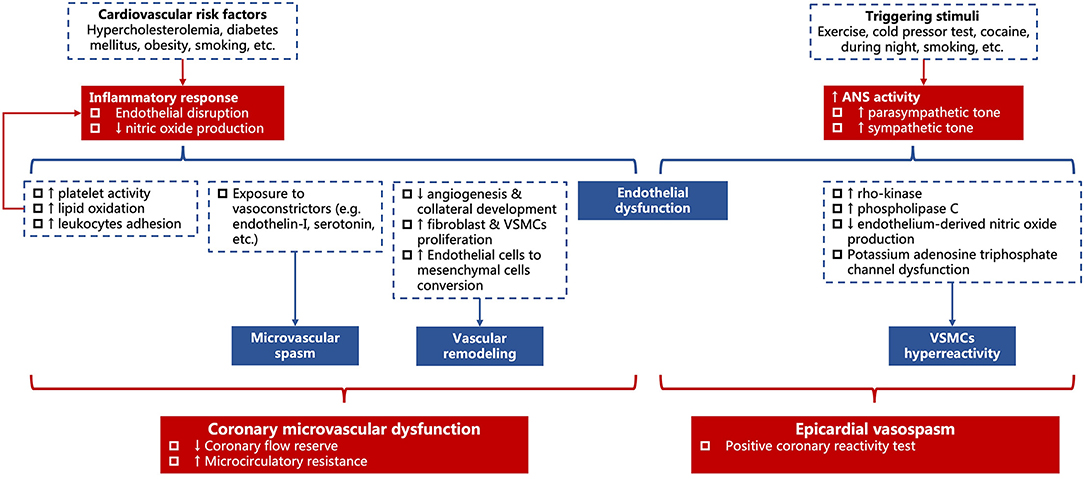
Figure 2. Diagram for the association between risk factors and hemodynamic changes in coronary microvascular dysfunction and epicardial vasospasm. VSMCs, vascular smooth muscle cells.
In response to common cardiovascular risk factors (e.g., hypercholesterolemia, diabetes mellitus, obesity, smoking, etc.), low-grade inflammation occurs and results in endothelium disruption and decreases the production of NO. The shortage of NO leads to increased platelet activity, lipid oxidation, and leukocytes adhesion, which, in turn, accelerates the inflammatory process (40, 41). It also impairs the process of angiogenesis and collateral development, meanwhile, promotes the proliferation of fibroblasts and vascular smooth muscle cells (VSMCs) and converts endothelial cells into mesenchymal cells (42, 43). The increased fibronectin and collagen deposition elongates the distance from capillary to adjacent cardiomyocytes and anticipates hypoxia on exertion (44–47). In addition, there is hypersensitivity toward the vasoconstrictor stimuli such as endothelin-I (48, 49) and serotonin (50), thus, a microvascular spasm might occur (Figure 2).
Chronic microvascular dysfunction is related to structural remodeling in patients with atherosclerosis, left ventricular hypertrophy cardiomyopathy, and arterial hypertension (21, 51, 52), where the arterial medial wall is thickened due to VSMCs proliferation and perivascular fibrosis, and the density of microvasculature is reduced (aka. microvascular rarefaction) due to diminished NO level (Figure 2) (51, 53, 54).
Epicardial Vasospasm
Vasospasm can also occur in the epicardial artery (55). Common cardiovascular risk factors show no significant association with epicardial vasospasm, except for smoking (56, 57). Though inflammation and oxidative stress have been documented in vasospasm, they hardly constitute a major cause (56). Instead, the activity of the autonomous nervous system is important in the pathogenesis of epicardial vasospasm. Increased tone in the sympathetic nervous system [e.g., exercise (58), cold pressor test (59), and cocaine (60)] and/or parasympathetic nervous system [e.g., during the night (61)] can trigger epicardial vasospasm (Figure 2).
The two major mechanisms are endothelial dysfunction and VSMC hyperreactivity (57, 62).
Endothelium, as discussed before, can release NO to induce vasodilation. Normally, vasoactive stimuli (e.g., acetylcholine, serotonin, and histamine) mediate vasodilation via endothelium-dependent release of vasodilators, especially NO. When endothelium bears significant damage, these vasoactive stimuli might directly act on VSMCs and cause paradoxical vasoconstriction (Figure 2) (56, 63).
On the other hand, VSMC itself can become overreactive to vasoconstrictors, which is known as VSMC hyperreactivity. VSMC contraction or relaxation is controlled by rho-kinase myosin light chain phosphorylation or dephosphorylation (56, 64–66). However, there are multiple pathways involved in VSMC contraction, and theoretically, any disturbance on these pathways could lead to VSMC hyperreactivity. Increased activity of rho-kinase (65) and phospholipase C (67), disrupted endothelium-dependent release of NO (68), and potassium adenosine triphosphate channels dysfunction (69, 70) have been identified in epicardial vasospasm (Figure 2).
Diagnostic Approaches for Ischemia With Non-Obstructive Coronary Arteries
Diagnostic approaches for INOCA are divided into non-invasive and invasive approaches, which have been studied extensively in recent years. The non-invasive approaches mainly include echocardiography, cardiac positron emission tomography (PET), single-photon emission CT (SPECT), CCTA, and cardiac MRI. The invasive approaches mainly include coronary function test (CFT) to measure CFR and/or microcirculatory resistance, coronary reactivity test to assess vasoreactivity, and some new-evolving techniques that facilitate these measurements (Table 1).
The 2019 European Society of Cardiology guideline for chronic coronary syndrome has clear recommendations for each of these diagnostic approaches. For patients with suspected CMD, that is, having persistent symptoms but coronary arteries that are either angiographically normal or have moderate stenoses with preserved FFR, the invasive approaches for measuring CFR and microcirculatory resistance should be considered, while the coronary reactivity test and non-invasive approaches for measuring CFR (i.e., echocardiography, cardiac PET, and cardiac MRI) may be considered. For patients with suspected vasospasm, that is, having a clinical picture of coronary spasm but normal findings or non-obstructive lesions on CAG, the coronary reactivity test should be considered (7).
Non-Invasive Diagnostic Approaches
Stress Transthoracic Doppler Echocardiography
Transthoracic color Doppler echocardiography is commonly performed at the mid-to-distal portion of the left anterior descending artery, and pulse wave Doppler is used to detecting flow velocity signal at rest and during hyperemia under stress. Stress can be induced by physical exercise (e.g., treadmill, exercise) or pharmacological agents (e.g., dobutamine, adenosine, dipyridamole) (71). Coronary flow velocity reserve [CF(V)R] is the ratio of coronary flow velocity during peak hyperemia and mean coronary flow velocity at rest (15, 72–76). A CFR <2.5 is defined as CMD (15, 77).
Though CFR is generally available and reproducible, its clinical use is limited by the lack of integration of the whole myocardium, in addition to the high requirement for operators (72, 73, 76, 78, 79).
Myocardial Contrast Echocardiography (MCE)
Myocardial contrast echocardiography requires injection of ultrasonic contrast agent and microbubbles that have a similar size as red blood cells (<7 μm diameter). These microbubbles bounce echo signal, which is positively correlated with the capillary blood volume (80, 81). As 90% of coronary circulation is at capillaries at rest, a decayed signal intensity during continuous microbubble infusion would provide a hint of microvascular dysfunction (80, 82, 83). To obtain CFR, myocardial blood flow (MBF) needs to be measured by the product of peak contrast intensity (db) and myocardial flow velocity (db/s) in each myocardial segment first. Then, CFR is the ratio of MBF at peak hyperemia and MBF at rest. The normal interval of MCE-measured CFR was 4–5 (84).
Myocardial contrast echocardiography-measured CFR has been compared with the gold standard of CMD, which showed acceptable consistency. However, considerable variation of results among operators can disturb the reproducibility, which is due to the high variability of image quality and might be improved by increasing the numbers of operators (80–82). Therefore, quantitative and qualitative measurement of myocardial perfusion from MCE requires further validation (85).
Cardiac PET
Cardiac PET is a technique based on the use of radiotracers labeled with isotope emission positrons. Common radiotracers are 15O-water, 82Rubidium, 13N-ammonia, and 18F-labeled agents (86). Their high myocardial extraction fraction allows the acquisition of myocardial perfusion images (MPI). MBF and myocardial flow reserve (MFR) are commonly obtained parameters (26). MBF is measured on dynamic PET. To measure MBF, “time-attenuation curves” that measure radioactive tracer activity transported by coronary arterial blood to myocardial tissue over time are required. The “time-attenuation curve” is fitted to a mathematic model, most commonly the one-tissue compartment model or simplified retention model. MBF is then estimated from the equation in the respective mathematic model (87–91). MFR is the ratio of MBF during pharmacological induced hyperemia to MBF at rest (86, 92) an MFR > 2.3 represents a favorable prognosis while an MFR <1.5 is suggestive of CMD and a high risk of future cardiac events (93, 94).
Cardiac PET is until now the most reliable method of non-invasive assessment of INOCA (74, 76, 91, 93, 95). The procedure is generally safe, time-saving, and accurate (96). The prognostic value has been verified for certain diseases, namely, hypertrophic cardiomyopathy (97), cardiac allograft vasculopathy (98), metabolic syndrome (99–101), heart failure with preserved ejection fraction (16), and female patients with INOCA (94), in which CMD plays an important role and impaired MFR is predictive of MACE (102, 103). However, PET is limited by its unavailability, cost-ineffectiveness, and radiation exposure even with a novel radiotracer (76, 93, 104). In addition, the cutoff value of MFR might vary among different PET tracers due to their unique kinetics (Figure 3) (105).
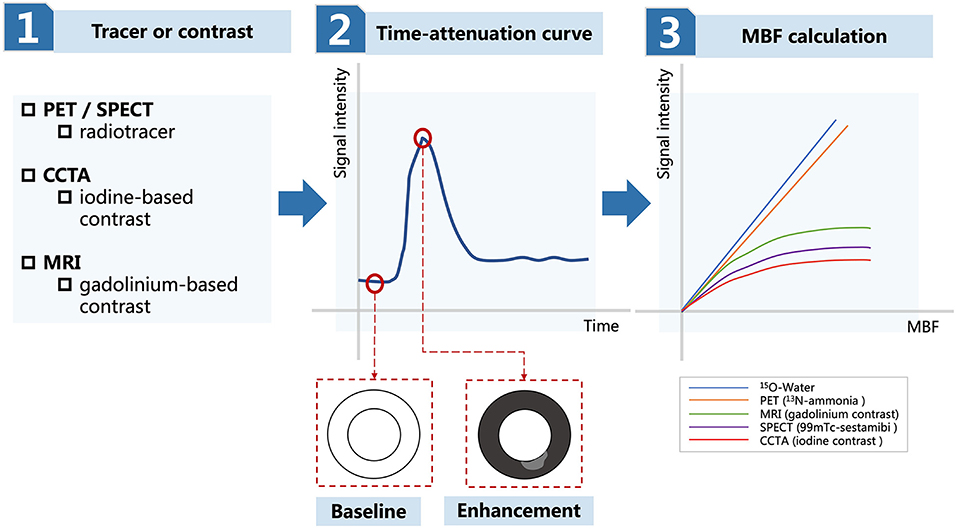
Figure 3. Diagram for the measurement of MBF in PET, SPECT, CCTA, and MRI. MBF, myocardial blood flow; PET, positron emission tomography; SPECT, single-photon emission computed tomography; CCTA, coronary computed tomography angiography; MRI, magnetic resonance imaging.
Single-Photon Emission CT
Single-photon emission CT is a technique using different radioactive tracers from PET (i.e., 99mTc-sestamibi or 99mTc-tetrofosmin). MPI obtained from SPECT is used to estimate MBF and CFR (106). According to the “microsphere method” proposed by Sugihara et al. (107) it is assumed that radioactive tracers are taken up by myocardial tissue. MBF is the ratio of myocardial retention of tracers over the integral arterial concentration of tracers. CFR is the ratio of MBF during peak hyperemia to MBF at rest (108).
Compared to PET, SPECT has wider availability and MPI is more commonly performed (106). However, the estimation of SPECT-measured CFR was believed to be inaccurate, especially at the higher flow rate, and the optimal threshold was inconsistent across studies (Figure 3) (107, 109–111). Poor image quality is also a disadvantage due to tracer attenuation (106). To solve these problems, a cadmium-zinc-telluride semiconductor detector is a recently developed cardiac dedicated gamma camera system that can be used to reduce radiation exposure and improve time efficacy (112, 113). MPI obtained from dynamic SPECT reserves high quality of images and accuracy of data (108). MBF and CFR measurement from this cardiac dedicated SPECT are still under research, but the prognostic value of dynamic SPECT has been demonstrated (104, 114).
Coronary CT Angiography (CCTA)
Coronary CT angiography is a commonly used screening test by using an iodine-based contrast agent to detect plaque and stenosis (106). However, CCTA is limited by the inability to identify ischemia, thus unable to provide diagnostic value for INOCA (7, 115). Dynamic computed tomographic perfusion image (CPI) is a recently developed technique that can be used to quantify MBF and other parameters. To calculate MBF, displacement of the myocardium is corrected and poor-quality images resulting from rhythm irregularities are excluded before data acquisition. Then, the ventricular myocardium is discretized into small volumetric elements, where each element creates their “time-attenuation curve,” respectively. The function of arterial input is generated by taking a sample volume in descending aorta. The arterial input function and “time-attenuation curve” are coupled in a hybrid deconvolution model. MBF is the ratio of the convoluted maximal slope of the “time-attenuation curve” of the myocardium and arterial input function (116). The normal range of MBF varies among studies: from 75 to 164 ml/min/100 ml (117). CFR is the ratio of MBF during hyperemia to MBF at rest (118). To calculate FFR, coronary blood flow simulation from CCTA images is required. Resting coronary blood flow is computed from myocardial territory-specific ventricular volume. Coronary resistance is computed from resting coronary flow according to the morphometry laws. Specifically, the microvascular resistance is inversely proportional to the size of vessels which carried a certain amount of blood (119, 120). Decrease in microvascular resistance in response to adenosine is modeled to simulate hyperemia. Mean aortic pressure is estimated from the mean brachial artery pressure. FFR is the ratio of computational mean coronary pressure distal to a lesion to the mean blood pressure in the aorta during simulated peak hyperemia (119–123).
The advance of CPI allows non-invasive quantification of FFR and CFR. Furthermore, the combination of CCTA and CPI can increase the diagnostic accuracy of INOCA and provide equivocal information with PET (124). Nevertheless, CPI is rarely used in clinical practice and its usefulness is yet to be tested in terms of INOCA (92, 106, 125).
Cardiac MRI
Cardiac MRI is based on a gadolinium-based contrast agent and a first-pass perfusion image is commonly performed to assess myocardial ischemia. MBF can be obtained via visual assessment or semi-quantitative methods. As gadolinium first passes the myocardium, the signal intensity in the well-perfused myocardium is brighter than the ischemic area due to the shorter T1 relaxation time in the myocardium, which is visually evident. Without obstructive coronary arteries, diminished MBF might be related to CMD. MBF can also be quantified by the myocardial perfusion reserve (MPR) index (126–131). The myocardium is sliced into several segments. The signal intensity of the left ventricular blood pool and myocardium are measured. The myocardial upslope during contrast enhancement generates a “time-attenuation curve” which allows measurement of myocardial perfusion. The myocardial upslope is also normalized to adjacent ventricular blood pool upslope (132). MPR index is the ratio of myocardial perfusion during hyperemia to myocardial perfusion at rest. MPR index ≤ 1.5 is defined as pathological (126).
Myocardial perfusion reserve index has a significant correlation with CMD and shows good consistency with measurement from PET and invasive methods, and thus could be proposed as a non-invasive assessment tool for INOCA (131, 133–135). MPR index can be used in CMD for clinical outcome prediction, where one unit increase leads to 90% decrease in the risk of long-term MACE (136). Cardiac MRI has the advantages of high-resolution images and are free of radiation exposure, but can be limited by its cost ineffectiveness, time consumption, unavailability, and influence of baseline myocardial perfusion and tissue contrast concentration on the MPR index (Figure 3) (92, 106, 132).
Summary for Myocardial Blood Flow Measurement in PET, SPECT, CCTA, and Cardiac MRI
The principle of measuring MBF in PET, SPECT, CCTA, and cardiac MRI is similar (Figure 3) (92, 106). The first step is to select a radiotracer in PET/SPECT or contrast agent in CCTA and cardiac MRI, which is then infused through vessels and taken up by the myocardium. The second step is to record myocardial signal intensity during enhancement and attenuation with time, which is illustrated as the “time-attenuation curve.” Well-perfused myocardium during enhancement is represented as the “black area” and ischemic myocardium during enhancement is represented as the “gray area.” The third step is to calculate MBF by fitting the “time-attenuation curve” to mathematic models. MBF estimation is related to signal extraction of the myocardium. There is a constant with 100% extraction for 15O-water and almost 100% extraction for 13N-ammonia. The extraction for agents commonly used in MRI, SPECT, and CCTA decreases as MBF increases, therefore underestimating MBF when MBF is high.
Invasive Diagnostic Approaches in Inoca
Coronary Function Test (CFT)
Coronary function test is a wire-based technique and is performed invasively when INOCA is considered after initial coronary angiography (7, 137). Multiple parameters are generated from this test, including but not limited to FFR, CFR, IMR, and HMR.
With a guiding catheter placed at the ostium of the coronary artery, a pressure monitoring guide wire is calibrated and advanced distally to the stenotic lesion of the coronary artery. Hyperemia is induced by intravenous injection of a vasodilator such as adenosine. The distal coronary pressure (Pd) and aortic pressure (Pa) during maximal coronary flow are recorded. FFR is the ratio of Pd to Pa (Figure 4) (138, 139). Most of the recent studies defined 0.80 as the optimal threshold, and FFR ≤ 0.80 is indicated for PCI therapy (140–142). In the assessment of INOCA, FFR serves as the physiologic parameter of the epicardial artery and is particularly useful in distinguishing intermediate stenotic severity visualized on angiogram (143).
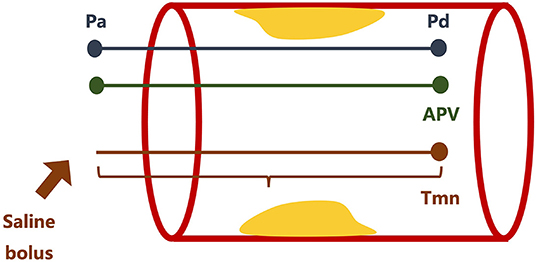
Figure 4. Illustration of coronary function test. Pd, distal coronary pressure; Pa, aortic pressure; APV, average peak velocity; Tmn, transit time.
Coronary flow reserve (CFR) is the ratio of maximal to basal coronary flow. CFR can be measured via Doppler or thermodilution methods, and their equations to obtain CFR are slightly different. For the Doppler-based method, a Doppler guiding wire is used to measure phasic flow velocity and determine the average peak velocity (APV). CFRDoppl is the ratio of hyperemic APV to resting APV (Figure 4) (144, 145). For the thermodilution method, a bolus of saline is injected manually into the guiding catheter and the mean transit time (Tmn) is defined as the time needed for the bolus to travel from the guiding catheter to the sensor located in the distal part of the artery. CFRthermo is the ratio of resting Tmn to hyperemic Tmn (Figure 4) (145–147). IMR is the product of Pd and Tmn during hyperemia via the thermodilution method (Figure 4) (148–150). HMR is the ratio of Pd to APV during hyperemia via the Doppler-based method (Figure 4) (151, 152). CFR is not as reproducible and specific as IMR owing to the influence of systemic hemodynamics (i.e., blood pressure, heart rate, etc.) and myocardial contractility. Therefore, IMR is usually necessary to assess microvascular resistance accurately (72, 153–155). CFR <2.0, IMR ≥ 25, and HMR ≥ 1.9 are defined as pathological and accounting for CMD (147, 154, 156–158).
A coronary reactivity test can be used to provide further information about vasospasm. Endothelial function was evaluated via endothelium-dependent (acetylcholine, substance P, and bradykinin) and endothelium-independent (adenosine and sodium nitroprusside) agents (1, 5, 159). Epicardial spasm is diagnosed if (1) reproducible chest pain, (2) detection of ischemic changes of electrocardiography, and (3) more than 90% reduction of epicardial arterial diameter. Microvascular spasm is diagnosed if (1) and (2) are present and excluding (3) (Figure 5) (160).
The invasive evaluation of INOCA was proposed by the Coronary Vasomotion Disorders International Study Group. First of all, an initial assessment of the epicardial coronary artery is required to exclude an obstructive lesion, where FFR is strongly recommended in addition to the angiogram. Next, CFR, IMR, and HMR can be measured routinely to provide information regarding microvascular resistance. Last but not least, a coronary reactivity test is performed to reveal vasospasm. CMD is defined as FFR > 0.80, CFR <2.0, IMR ≥ 25, HMR ≥ 1.9, and positive microvascular spasm. Vasospastic angina is defined as FFR > 0.80, CFR ≥ 2.0, IMR <25, HMR <1.9, and epicardial spasm. Mixed type of CMD and vasospastic angina is defined as FFR > 0.80, CFR <2.0, IMR ≥ 25, HMR ≥ 1.9, and microvascular and/or epicardial spasm (Figure 6) (1, 24, 161, 162).
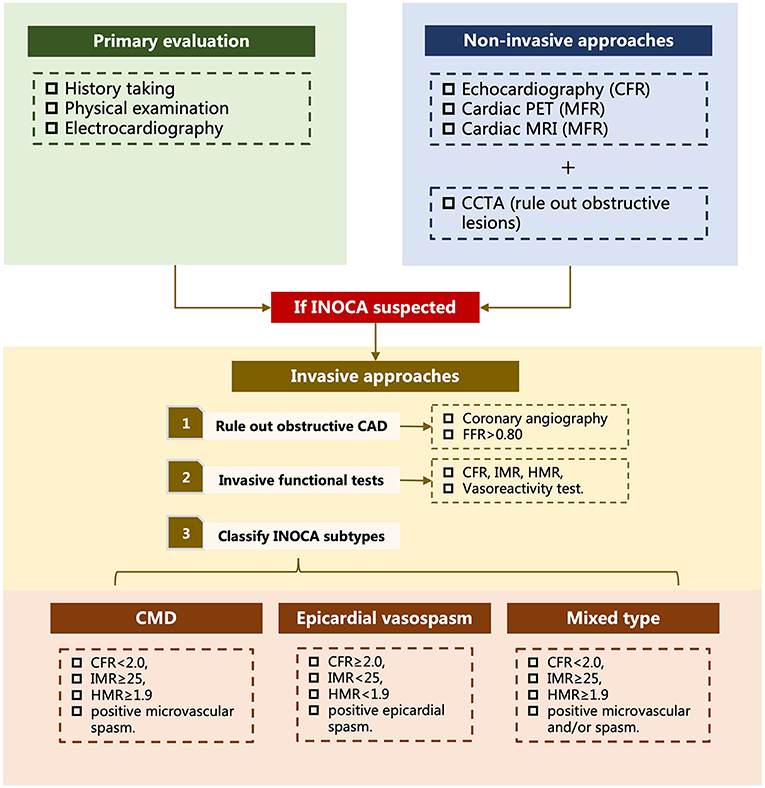
Figure 6. Diagram for the evaluation of INOCA. INOCA, ischemia with non-obstructive arteries; PET, positron emission tomography; MRI, magnetic resonance imaging; CCTA, coronary computed tomography angiography; CFR, coronary flow reserve; MFR, myocardial flow reserve; CAD, coronary artery disease; FFR, fractional flow reserve; IMR, index of microcirculatory resistance; HMR, hyperemic myocardial velocity resistance; CMD, coronary microvascular dysfunction. Mixed type refers to combination of coronary microvascular dysfunction and epicardial vasospasm.
Besides confirmatory diagnosis and classification of INOCA, the role of CFT in predicting the prognosis of INOCA is widely acknowledged. Both CMD and epicardial vasospasm are relevant to MACE (163, 164). Patients with both CMD and epicardial vasospasm, which is defined as the mixed type of INOCA, have a particularly higher risk of unstable angina, myocardial infarction, and cardiac death (165, 166). CFT was also proven to be feasible in stratifying patients with INOCA for different treatment strategies (159, 167). Categorizing patients into different pathophysiological endotypes and treating them with specific protocol has improved symptom control of angina and quality of life to a significant extent (165, 167). Coronary blood flow changes in response to endothelium-dependent reactivity, i.e., acetylcholine infusion, which also has prognostic value. Coronary blood flow change of <50% is considered abnormal microvascular reactivity and is associated with long-term mortality (163). Non-invasive diagnostic approaches, when compared to CFT, showed extreme limitation in regards to detection of vasospasm, which is frequently encountered in INOCA (137). Therefore, CFT and coronary reactivity test are encouraged as soon as possible when INOCA is suspected, to prevent unnecessary diagnostic tests and promote early stratified treatment (137). The major limitation of CFT is its invasive nature and unavailability (25). However, the safety of invasive diagnostic procedures can be guaranteed by the experienced and dedicated interventional team and the rate of life-threatening complications such as coronary artery dissection, myocardial infarction, and ventricular arrhythmia are rare (168, 169). Overall, CFT is a safe and reliable diagnostic approach of INOCA.
Continuous Thermodilution
Several disadvantages of Doppler and bolus thermodilution methods have been noticed, such as intraoperator variability and side effects resulting from vasodilators (115). Reliable results occurred only in 60–70% of the cases (170). Continuous thermodilution is a novel method that measures absolute flow (AF) and absolute resistance (AR) in a coronary artery, which is independent of operator quantification (28, 29). By infusing room temperature saline (Ti) at the rate of 8–20 ml/min (Qi), maximal hyperemia could be achieved in seconds (171, 172). Temperature of blood mixing with saline is denoted as T, which decreases as saline infused to the coronary artery. AF is the result of 1.08 × Qi × Ti/T.” AR is then calculated by dividing Pd by AF (28). Higher AR has been noticed to be associated with abnormal CFR and IMR, but neither AF nor AR is correlated with vasospasm (173). In addition, large interpersonal variability makes it difficult to establish the reference range of AF and AR. Further studies are required for assessing the validity of AF and AR (174).
Angiography-Derived Quantitative Flow Ratio
Recently, a three-dimensional quantitative coronary angiography that enables rapid computation of FFR, which is denoted as QFR, has been developed. Coronary angiography is performed and two angiographic images that are at least 25 degrees apart from the artery of interest are selected. Three-dimensional reconstruction is automatically done and manual correction is allowed in case of suboptimal image quality. QFR can be calculated in three different flow models: (1) the fixed-flow QFR pullback: a fixed empiric hyperemic flow velocity of 0.35 m/s derived from Shengxian Tu et al. is used (27); (2) the contrast-flow QFR pullback: frame count analysis is performed on two diagnostic angiographic images without pharmacological induction. Modeled hyperemic flow velocity is derived by calculating two new QFR pullbacks (27); (3) the adenosine-flow QFR pullback: frame count analysis is performed on two diagnostic angiographic images with adenosine-induced hyperemia. Measured hyperemic flow velocity is derived by calculating two new QFR pullbacks (175). QFR shows good consistency with pressure wire-derived FFR and can be used to promote rapid computation of FFR (175–178). Furthermore, the formula of angiography-based IMR has been proposed. Without induction of hyperemia and use of pressure guidewire, angiography-based IMR also has a good correlation with IMR (179). QFR allows faster and more accurate evaluation of coronary microcirculation, therefore showing great potential in future practice. However, the reference range of FFR and IMR needs to be corrected in a larger sample size. CMD and acute coronary syndrome can influence the diagnostic performance of QFR. Therefore, indication for the use of QFR needs to be addressed before it becomes a routine tool in modern catheterization labs (179, 180).
Discussion
Ischemia with non-obstructive coronary arteries is recognized as a clinical condition with strong evidence for unfavorable prognosis, including issues on quality of life, heart failure with preserved ejection fraction, MACE, and mortality. CMD and/or epicardial vasospasm are the two major pathophysiological mechanisms for INOCA. To establish a confirmatory diagnosis of INOCA, coronary blood flow, microvascular resistance, and vasoreactivity are required to be assessed, in which invasive coronary function test and coronary reactivity test are the gold standards. Several new techniques help complement the diagnosis of INOCA, such as continuous thermodilution and QFR. As for non-invasive methods, cardiac PET is a more reliable method compared to echocardiography, SPECT, CCTA, and cardiac MRI. Nevertheless, the non-invasive methods are unable to evaluate vasospasm, which is a frequently encountered endotype of INOCA. Another disadvantage is the variability of computed parameters due to poor image quality and low reproducibility.
A straightforward diagnostic diagram for INOCA evaluation has been proposed by Kunadian et al. (1). The evaluation of INOCA begins with history taking to address risk factors and events of ischemia, followed by physical examination and electrocardiography to exclude acute coronary syndrome at primary healthcare facilities. If patients are highly suspected to have INOCA, then they should be referred to cardiologists, where further diagnostic tests are performed. Non-invasive diagnostic approaches are the first-line tools. Techniques that can provide information about the coronary function (i.e., FFR, CFR, MBF, etc.) are preferred, such as echocardiography, cardiac PET, and cardiac MRI, based on local expertise and availability. CCTA may be performed to reveal any obstructive lesions on coronary arteries. According to the 2019 European Society of Cardiology guideline for the chronic coronary syndrome, invasive diagnostic approaches should be considered for patients with suspected CMD, that is, having persistent symptoms but coronary arteries that are either angiographically normal or have moderate stenoses with preserved FFR, or for patients with suspected vasospasm, that is, having a clinical picture of coronary spasm but normal findings or non-obstructive lesions on CAG (7). After ruling out obstructive CAD on CAG, invasive functional tests measuring CFR, IMR, HMR, and assessing vasoreactivity are performed to further classify INOCA into different endotypes, that is, CMD, epicardial spasm, and mixed type of CMD and epicardium (Figure 6).
In clinical practice, however, thorough diagnostic procedures on all suspected patients are unrealistic. Therefore, patients' follow-ups are essential for the assessment of the quality of life, identification of adverse events, prompt evaluation, and modification of treatment plans. In addition, though the confirmatory diagnosis has relied on invasive diagnostic approaches, non-invasive diagnostic approaches are promising as fast and accurate screening tests to meet the large demands of indicated candidates, if the limitations can be improved in future investigations. Last but not least, coronary microvascular dysfunction could be a regional presentation of systemic microvascular diseases. Diagnostic techniques that assess microvasculature in the kidney, retina, and cerebral white matter could also be an interesting research direction (181). The abbreviation and definition of this article are listed in Table 2.
Author Contributions
DY and JC conceived of the presented idea. BF wrote the manuscript. XW and YL revised the manuscript. All authors contributed to the article and approved the final version of the manuscript.
Funding
This study was supported by grants from the Science and Technology Projects of Guangzhou (Grant No. 201903010097). The funders had no role in the study design, data collection and analysis, decision to publish, or preparation of the manuscript.
Conflict of Interest
The authors declare that the research was conducted in the absence of any commercial or financial relationships that could be construed as a potential conflict of interest.
Publisher's Note
All claims expressed in this article are solely those of the authors and do not necessarily represent those of their affiliated organizations, or those of the publisher, the editors and the reviewers. Any product that may be evaluated in this article, or claim that may be made by its manufacturer, is not guaranteed or endorsed by the publisher.
Acknowledgments
We gratefully acknowledged the authors of all of our references.
References
1. Kunadian V, Chieffo A, Camici PG, Berry C, Escaned J, Maas A, et al. An EAPCI expert consensus document on ischaemia with non-obstructive coronary arteries in collaboration with European society of cardiology working group on coronary pathophysiology & microcirculation endorsed by coronary vasomotor disorders international study group. Eur Heart J. (2020) 41:3504–20. doi: 10.1093/eurheartj/ehaa503
2. Sedlak T, Herscovici R, Cook-Wiens G, Handberg E, Wei J, Shufelt C, et al. Predicted versus observed major adverse cardiac event risk in women with evidence of ischemia and no obstructive coronary artery disease: a report from WISE (Women's Ischemia Syndrome Evaluation). J Am Heart Assoc. (2020) 9:e013234. doi: 10.1161/JAHA.119.013234
3. Ford TJ, Yii E, Sidik N, Good R, Rocchiccioli P, McEntegart M, et al. Ischemia and no obstructive coronary artery disease: prevalence and correlates of coronary vasomotion disorders. Circ Cardiovasc Interv. (2019) 12:e008126. doi: 10.1161/CIRCINTERVENTIONS.119.008126
4. Herscovici R, Sedlak T, Wei J, Pepine CJ, Handberg E, Bairey Merz CN. Ischemia and No Obstructive Coronary Artery Disease (INOCA): what is the risk? J Am Heart Assoc. (2018) 7:e008868. doi: 10.1161/JAHA.118.008868
5. Bairey Merz CN, Pepine CJ, Walsh MN, Fleg JL. Ischemia and No Obstructive Coronary Artery Disease (INOCA): developing evidence-based therapies and research agenda for the next decade. Circulation. (2017) 135:1075–92. doi: 10.1161/CIRCULATIONAHA.116.024534
6. Reeh J, Therming CB, Heitmann M, Hojberg S, Sorum C, Bech J, et al. Prediction of obstructive coronary artery disease and prognosis in patients with suspected stable angina. Eur Heart J. (2019) 40:1426–35. doi: 10.1093/eurheartj/ehy806
7. Knuuti J, Wijns W, Saraste A, Capodanno D, Barbato E, Funck-Brentano C, et al. 2019 ESC Guidelines for the diagnosis and management of chronic coronary syndromes. Eur Heart J. (2020) 41:407–77. doi: 10.1093/eurheartj/ehz425
8. Johnson BD, Shaw LJ, Buchthal SD, Bairey Merz CN, Kim HW, Scott KN, et al. Prognosis in women with myocardial ischemia in the absence of obstructive coronary disease: results from the National Institutes of Health-National Heart, Lung, and Blood Institute-Sponsored Women's Ischemia Syndrome Evaluation (WISE). Circulation. (2004) 109:2993–9. doi: 10.1161/01.CIR.0000130642.79868.B2
9. Davis MB, Maddox TM, Langner P, Plomondon ME, Rumsfeld JS, Duvernoy CS. Characteristics and outcomes of women veterans undergoing cardiac catheterization in the Veterans Affairs Healthcare System: insights from the VA CART Program. Circ Cardiovasc Qual Outcomes. (2015) 8(2 Suppl 1):S39–47. doi: 10.1161/CIRCOUTCOMES.114.001613
10. Jespersen L, Hvelplund A, Abildstrom SZ, Pedersen F, Galatius S, Madsen JK, et al. Stable angina pectoris with no obstructive coronary artery disease is associated with increased risks of major adverse cardiovascular events. Eur Heart J. (2012) 33:734–44. doi: 10.1093/eurheartj/ehr331
11. Pepine CJ, Anderson RD, Sharaf BL, Reis SE, Smith KM, Handberg EM, et al. Coronary microvascular reactivity to adenosine predicts adverse outcome in women evaluated for suspected ischemia results from the National Heart, Lung and Blood Institute WISE (Women's Ischemia Syndrome Evaluation) study. J Am Coll Cardiol. (2010) 55:2825–32. doi: 10.1016/j.jacc.2010.01.054
12. Sharaf B, Wood T, Shaw L, Johnson BD, Kelsey S, Anderson RD, et al. Adverse outcomes among women presenting with signs and symptoms of ischemia and no obstructive coronary artery disease: findings from the National Heart, Lung, and Blood Institute-sponsored Women's Ischemia Syndrome Evaluation (WISE) angiographic core laboratory. Am Heart J. (2013) 166:134–41. doi: 10.1016/j.ahj.2013.04.002
13. Maddox TM, Stanislawski MA, Grunwald GK, Bradley SM, Ho PM, Tsai TT, et al. Nonobstructive coronary artery disease and risk of myocardial infarction. JAMA. (2014) 312:1754–63. doi: 10.1001/jama.2014.14681
14. Ahmad A, Corban MT, Toya T, Verbrugge FH, Sara JD, Lerman LO, et al. Coronary microvascular dysfunction is associated with exertional haemodynamic abnormalities in patients with heart failure with preserved ejection fraction. Eur J Heart Fail. (2021) 23:765–72. doi: 10.1002/ejhf.2010
15. Shah SJ, Lam CSP, Svedlund S, Saraste A, Hage C, Tan RS, et al. Prevalence and correlates of coronary microvascular dysfunction in heart failure with preserved ejection fraction: PROMIS-HFpEF. Eur Heart J. (2018) 39:3439–50. doi: 10.1093/eurheartj/ehy531
16. Taqueti VR, Solomon SD, Shah AM, Desai AS, Groarke JD, Osborne MT, et al. Coronary microvascular dysfunction and future risk of heart failure with preserved ejection fraction. Eur Heart J. (2018) 39:840–9. doi: 10.1093/eurheartj/ehx721
17. Kenkre TS, Malhotra P, Johnson BD, Handberg EM, Thompson DV, Marroquin OC, et al. Ten-Year Mortality in the WISE Study (Women's Ischemia Syndrome Evaluation). Circ Cardiovasc Qual Outcomes. (2017) 10:e003863. doi: 10.1161/CIRCOUTCOMES.116.003863
18. Sedlak TL, Lee M, Izadnegahdar M, Merz CN, Gao M, Humphries KH. Sex differences in clinical outcomes in patients with stable angina and no obstructive coronary artery disease. Am Heart J. (2013) 166:38–44. doi: 10.1016/j.ahj.2013.03.015
19. Gulati M, Cooper-DeHoff RM, McClure C, Johnson BD, Shaw LJ, Handberg EM, et al. Adverse cardiovascular outcomes in women with nonobstructive coronary artery disease: a report from the Women's Ischemia Syndrome Evaluation Study and the St James Women Take Heart Project. Arch Intern Med. (2009) 169:843–50. doi: 10.1001/archinternmed.2009.50
20. Shaw LJ, Merz CN, Pepine CJ, Reis SE, Bittner V, Kip KE, et al. The economic burden of angina in women with suspected ischemic heart disease: results from the National Institutes of Health–National Heart, Lung, and Blood Institute–sponsored Women's Ischemia Syndrome Evaluation. Circulation. (2006) 114:894–904. doi: 10.1161/CIRCULATIONAHA.105.609990
21. Camici PG, d'Amati G, Rimoldi O. Coronary microvascular dysfunction: mechanisms and functional assessment. Nat Rev Cardiol. (2015) 12:48–62. doi: 10.1038/nrcardio.2014.160
22. Crea F, Camici PG, Bairey Merz CN. Coronary microvascular dysfunction: an update. Eur Heart J. (2014) 35:1101–11. doi: 10.1093/eurheartj/eht513
23. Sara JD, Widmer RJ, Matsuzawa Y, Lennon RJ, Lerman LO, Lerman A. Prevalence of coronary microvascular dysfunction among patients with chest pain and nonobstructive coronary artery disease. JACC Cardiovasc Interv. (2015) 8:1445–53. doi: 10.1016/j.jcin.2015.06.017
24. Ong P, Camici PG, Beltrame JF, Crea F, Shimokawa H, Sechtem U, et al. International standardization of diagnostic criteria for microvascular angina. Int J Cardiol. (2018) 250:16–20. doi: 10.1016/j.ijcard.2017.08.068
25. Masi S, Rizzoni D, Taddei S, Widmer RJ, Montezano AC, Luscher TF, et al. Assessment and pathophysiology of microvascular disease: recent progress and clinical implications. Eur Heart J. (2021) 42:2590–604. doi: 10.1093/eurheartj/ehaa857
26. Klein R, Celiker-Guler E, Rotstein BH, deKemp RA. PET and SPECT tracers for myocardial perfusion imaging. Semin Nucl Med. (2020) 50:208–18. doi: 10.1053/j.semnuclmed.2020.02.016
27. Tu S, Barbato E, Koszegi Z, Yang J, Sun Z, Holm NR, et al. Fractional flow reserve calculation from 3-dimensional quantitative coronary angiography and TIMI frame count: a fast computer model to quantify the functional significance of moderately obstructed coronary arteries. JACC Cardiovasc Interv. (2014) 7:768–77. doi: 10.1016/j.jcin.2014.03.004
28. Xaplanteris P, Fournier S, Keulards DCJ, Adjedj J, Ciccarelli G, Milkas A, et al. Catheter-based measurements of absolute coronary blood flow and microvascular resistance: feasibility, safety, and reproducibility in humans. Circ Cardiovasc Interv. (2018) 11:e006194. doi: 10.1161/CIRCINTERVENTIONS.117.006194
29. van 't Veer M, Adjedj J, Wijnbergen I, Toth GG, Rutten MC, Barbato E, et al. Novel monorail infusion catheter for volumetric coronary blood flow measurement in humans: in vitro validation. EuroIntervention. (2016) 12:701–7. doi: 10.4244/EIJV12I6A114
30. Camici PG, Crea F. Coronary microvascular dysfunction. N Engl J Med. (2007) 356:830–40. doi: 10.1056/NEJMra061889
31. Chilian WM. Coronary microcirculation in health and disease. Summary of an NHLBI workshop. Circulation. (1997) 95:522–8. doi: 10.1161/01.CIR.95.2.522
32. Brutsaert DL. Cardiac endothelial-myocardial signaling: its role in cardiac growth, contractile performance, and rhythmicity. Physiol Rev. (2003) 83:59–115. doi: 10.1152/physrev.00017.2002
33. Pries AR, Badimon L, Bugiardini R, Camici PG, Dorobantu M, Duncker DJ, et al. Coronary vascular regulation, remodelling, and collateralization: mechanisms and clinical implications on behalf of the working group on coronary pathophysiology and microcirculation. Eur Heart J. (2015) 36:3134–46. doi: 10.1093/eurheartj/ehv100
34. Kuo L, Davis MJ, Chilian WM. Longitudinal gradients for endothelium-dependent and -independent vascular responses in the coronary microcirculation. Circulation. (1995) 92:518–25. doi: 10.1161/01.CIR.92.3.518
35. Gutierrez E, Flammer AJ, Lerman LO, Elizaga J, Lerman A, Fernandez-Aviles F. Endothelial dysfunction over the course of coronary artery disease. Eur Heart J. (2013) 34:3175–81. doi: 10.1093/eurheartj/eht351
36. Li H, Forstermann U. Prevention of atherosclerosis by interference with the vascular nitric oxide system. Curr Pharm Des. (2009) 15:3133–45. doi: 10.2174/138161209789058002
37. Mohammed SF, Hussain S, Mirzoyev SA, Edwards WD, Maleszewski JJ, Redfield MM. Coronary microvascular rarefaction and myocardial fibrosis in heart failure with preserved ejection fraction. Circulation. (2015) 131:550–9. doi: 10.1161/CIRCULATIONAHA.114.009625
38. Tsagalou EP, Anastasiou-Nana M, Agapitos E, Gika A, Drakos SG, Terrovitis JV, et al. Depressed coronary flow reserve is associated with decreased myocardial capillary density in patients with heart failure due to idiopathic dilated cardiomyopathy. J Am Coll Cardiol. (2008) 52:1391–8. doi: 10.1016/j.jacc.2008.05.064
39. Mejia-Renteria H, van der Hoeven N, van de Hoef TP, Heemelaar J, Ryan N, Lerman A, et al. Targeting the dominant mechanism of coronary microvascular dysfunction with intracoronary physiology tests. Int J Cardiovasc Imaging. (2017) 33:1041–59. doi: 10.1007/s10554-017-1136-9
40. Vita JA. Endothelial function. Circulation. (2011) 124:e906–12. doi: 10.1161/CIRCULATIONAHA.111.078824
41. Huang AL, Vita JA. Effects of systemic inflammation on endothelium-dependent vasodilation. Trends Cardiovasc Med. (2006) 16:15–20. doi: 10.1016/j.tcm.2005.10.002
42. O'Riordan E, Mendelev N, Patschan S, Patschan D, Eskander J, Cohen-Gould L, et al. Chronic NOS inhibition actuates endothelial-mesenchymal transformation. Am J Physiol Heart Circ Physiol. (2007) 292:H285–94. doi: 10.1152/ajpheart.00560.2006
43. Goligorsky MS. Microvascular rarefaction: the decline and fall of blood vessels. Organogenesis. (2010) 6:1–10. doi: 10.4161/org.6.1.10427
44. Zannad F, Radauceanu A. Effect of MR blockade on collagen formation and cardiovascular disease with a specific emphasis on heart failure. Heart Fail Rev. (2005) 10:71–8. doi: 10.1007/s10741-005-2351-3
45. Gonzalez A, Ravassa S, Beaumont J, Lopez B, Diez J. New targets to treat the structural remodeling of the myocardium. J Am Coll Cardiol. (2011) 58:1833–43. doi: 10.1016/j.jacc.2011.06.058
46. Westermann D, Lindner D, Kasner M, Zietsch C, Savvatis K, Escher F, et al. Cardiac inflammation contributes to changes in the extracellular matrix in patients with heart failure and normal ejection fraction. Circ Heart Fail. (2011) 4:44–52. doi: 10.1161/CIRCHEARTFAILURE.109.931451
47. Escaned J, Flores A, Garcia-Pavia P, Segovia J, Jimenez J, Aragoncillo P, et al. Assessment of microcirculatory remodeling with intracoronary flow velocity and pressure measurements: validation with endomyocardial sampling in cardiac allografts. Circulation. (2009) 120:1561–8. doi: 10.1161/CIRCULATIONAHA.108.834739
48. Ford TJ, Corcoran D, Padmanabhan S, Aman A, Rocchiccioli P, Good R, et al. Genetic dysregulation of endothelin-1 is implicated in coronary microvascular dysfunction. Eur Heart J. (2020) 41:3239–52. doi: 10.1093/eurheartj/ehz915
49. Halcox JP, Nour KR, Zalos G, Quyyumi AA. Endogenous endothelin in human coronary vascular function: differential contribution of endothelin receptor types A and B. Hypertension. (2007) 49:1134–41. doi: 10.1161/HYPERTENSIONAHA.106.083303
50. Odaka Y, Takahashi J, Tsuburaya R, Nishimiya K, Hao K, Matsumoto Y, et al. Plasma concentration of serotonin is a novel biomarker for coronary microvascular dysfunction in patients with suspected angina and unobstructive coronary arteries. Eur Heart J. (2017) 38:489–96. doi: 10.1093/eurheartj/ehw448
51. Sorop O, van den Heuvel M, van Ditzhuijzen NS, de Beer VJ, Heinonen I, van Duin RW, et al. Coronary microvascular dysfunction after long-term diabetes and hypercholesterolemia. Am J Physiol Heart Circ Physiol. (2016) 311:H1339–51. doi: 10.1152/ajpheart.00458.2015
52. Sara JD, Taher R, Kolluri N, Vella A, Lerman LO, Lerman A. Coronary microvascular dysfunction is associated with poor glycemic control amongst female diabetics with chest pain and non-obstructive coronary artery disease. Cardiovasc Diabetol. (2019) 18:22. doi: 10.1186/s12933-019-0833-1
53. Suzuki H, Takeyama Y, Koba S, Suwa Y, Katagiri T. Small vessel pathology and coronary hemodynamics in patients with microvascular angina. Int J Cardiol. (1994) 43:139–50. doi: 10.1016/0167-5273(94)90003-5
54. Lindemann H, Petrovic I, Hill S, Athanasiadis A, Mahrholdt H, Schaufele T, et al. Biopsy-confirmed endothelial cell activation in patients with coronary microvascular dysfunction. Coron Artery Dis. (2018) 29:216–22. doi: 10.1097/MCA.0000000000000599
55. Aziz A, Hansen HS, Sechtem U, Prescott E, Ong P. Sex-related differences in vasomotor function in patients with angina and unobstructed coronary arteries. J Am Coll Cardiol. (2017) 70:2349–58. doi: 10.1016/j.jacc.2017.09.016
56. Lanza GA, Careri G, Crea F. Mechanisms of coronary artery spasm. Circulation. (2011) 124:1774–82. doi: 10.1161/CIRCULATIONAHA.111.037283
57. Picard F, Sayah N, Spagnoli V, Adjedj J, Varenne O. Vasospastic angina: a literature review of current evidence. Arch Cardiovasc Dis. (2019) 112:44–55. doi: 10.1016/j.acvd.2018.08.002
58. Specchia G, de Servi S, Falcone C, Bramucci E, Angoli L, Mussini A, et al. Coronary arterial spasm as a cause of exercise-induced ST-segment elevation in patients with variant angina. Circulation. (1979) 59:948–54. doi: 10.1161/01.CIR.59.5.948
59. Raizner AE, Chahine RA, Ishimori T, Verani MS, Zacca N, Jamal N, et al. Provocation of coronary artery spasm by the cold pressor test. Hemodynamic, arteriographic and quantitative angiographic observations. Circulation. (1980) 62:925–32. doi: 10.1161/01.CIR.62.5.925
60. El Menyar AA. Drug-induced myocardial infarction secondary to coronary artery spasm in teenagers and young adults. J Postgrad Med. (2006) 52:51–6.
61. Waters DD, Miller DD, Bouchard A, Bosch X, Theroux P. Circadian variation in variant angina. Am J Cardiol. (1984) 54:61–4. doi: 10.1016/0002-9149(84)90304-7
62. Shimokawa H. 2014 Williams Harvey Lecture: importance of coronary vasomotion abnormalities-from bench to bedside. Eur Heart J. (2014) 35:3180–93. doi: 10.1093/eurheartj/ehu427
63. Matta A, Bouisset F, Lhermusier T, Campelo-Parada F, Elbaz M, Carrie D, et al. Coronary artery spasm: new insights. J Interv Cardiol. (2020) 2020:5894586. doi: 10.1155/2020/5894586
64. Hirano K. Current topics in the regulatory mechanism underlying the Ca2+ sensitization of the contractile apparatus in vascular smooth muscle. J Pharmacol Sci. (2007) 104:109–15. doi: 10.1254/jphs.CP0070027
65. Kandabashi T, Shimokawa H, Miyata K, Kunihiro I, Kawano Y, Fukata Y, et al. Inhibition of myosin phosphatase by upregulated rho-kinase plays a key role for coronary artery spasm in a porcine model with interleukin-1beta. Circulation. (2000) 101:1319–23. doi: 10.1161/01.CIR.101.11.1319
66. Somlyo AP, Somlyo AV. Ca2+ sensitivity of smooth muscle and nonmuscle myosin II: modulated by G proteins, kinases, and myosin phosphatase. Physiol Rev. (2003) 83:1325–58. doi: 10.1152/physrev.00023.2003
67. Nakano T, Osanai T, Tomita H, Sekimata M, Homma Y, Okumura K. Enhanced activity of variant phospholipase C-delta1 protein (R257H) detected in patients with coronary artery spasm. Circulation. (2002) 105:2024–9. doi: 10.1161/01.CIR.0000014613.36469.3F
68. Bussemaker E, Pistrosch F, Forster S, Herbrig K, Gross P, Passauer J, et al. Rho kinase contributes to basal vascular tone in humans: role of endothelium-derived nitric oxide. Am J Physiol Heart Circ Physiol. (2007) 293:H541–7. doi: 10.1152/ajpheart.00770.2006
69. Chutkow WA, Pu J, Wheeler MT, Wada T, Makielski JC, Burant CF, et al. Episodic coronary artery vasospasm and hypertension develop in the absence of Sur2 K(ATP) channels. J Clin Invest. (2002) 110:203–8. doi: 10.1172/JCI0215672
70. Kakkar R, Ye B, Stoller DA, Smelley M, Shi NQ, Galles K, et al. Spontaneous coronary vasospasm in KATP mutant mice arises from a smooth muscle-extrinsic process. Circ Res. (2006) 98:682–9. doi: 10.1161/01.RES.0000207498.40005.e7
71. Picano E, Pasanisi E, Venneri L, Agrusta M, Mottola G, Sicari R. Stress echocardiography. Curr Pharm Des. (2005) 11:2137–49. doi: 10.2174/1381612054367454
72. Ford TJ, Corcoran D, Berry C. Stable coronary syndromes: pathophysiology, diagnostic advances and therapeutic need. Heart. (2018) 104:284–92. doi: 10.1136/heartjnl-2017-311446
73. Michelsen MM, Mygind ND, Pena A, Olsen RH, Christensen TE, Ghotbi AA, et al. Transthoracic Doppler echocardiography compared with positron emission tomography for assessment of coronary microvascular dysfunction: the iPOWER study. Int J Cardiol. (2017) 228:435–43. doi: 10.1016/j.ijcard.2016.11.004
74. Lanza GA. Diagnostic approach to patients with stable angina and no obstructive coronary arteries. Eur Cardiol. (2019) 14:97–102. doi: 10.15420/ecr.2019.22.2
75. Tesic M, Djordjevic-Dikic A, Giga V, Stepanovic J, Dobric M, Jovanovic I, et al. Prognostic value of transthoracic doppler echocardiography coronary flow velocity reserve in patients with nonculprit stenosis of intermediate severity early after primary percutaneous coronary intervention. J Am Soc Echocardiogr. (2018) 31:880–7. doi: 10.1016/j.echo.2018.02.011
76. Vancheri F, Longo G, Vancheri S, Henein M. Coronary microvascular dysfunction. J Clin Med. (2020) 9:2880. doi: 10.3390/jcm9092880
77. Gan LM, Svedlund S, Wittfeldt A, Eklund C, Gao S, Matejka G, et al. Incremental value of transthoracic doppler echocardiography-assessed coronary flow reserve in patients with suspected myocardial ischemia undergoing myocardial perfusion scintigraphy. J Am Heart Assoc. (2017) 6:875. doi: 10.1161/JAHA.116.004875
78. Caiati C, Montaldo C, Zedda N, Montisci R, Ruscazio M, Lai G, et al. Validation of a new noninvasive method (contrast-enhanced transthoracic second harmonic echo Doppler) for the evaluation of coronary flow reserve: comparison with intracoronary Doppler flow wire. J Am Coll Cardiol. (1999) 34:1193–200. doi: 10.1016/S0735-1097(99)00342-3
79. Michelsen MM, Pena A, Mygind ND, Frestad D, Gustafsson I, Hansen HS, et al. Coronary flow velocity reserve assessed by transthoracic doppler: the iPOWER study: factors influencing feasibility and quality. J Am Soc Echocardiogr. (2016) 29:709–16. doi: 10.1016/j.echo.2016.02.011
80. Senior R, Becher H, Monaghan M, Agati L, Zamorano J, Vanoverschelde JL, et al. Clinical practice of contrast echocardiography: recommendation by the European Association of Cardiovascular Imaging (EACVI) 2017. Eur Heart J Cardiovasc Imaging. (2017) 18:1205. doi: 10.1093/ehjci/jex182
81. Karogiannis N, Senior R. Contrast echocardiography for detection of myocardial perfusion abnormalities: a clinical perspective. Herz. (2017) 42:287–94. doi: 10.1007/s00059-017-4536-7
82. Liu Y, Kong B, Ma H, Guo L, Bai B, Yu X, et al. Consistency of Positron Emission Tomography and Myocardial Contrast Echocardiography in Diagnosing Mental Stress-Induced Myocardial Ischemia: Study Protocol of a Prospective Study-Background, Design and Method. Ultrasound Med Biol. (2020) 46:3200–9. doi: 10.1016/j.ultrasmedbio.2020.07.029
83. Aggarwal S, Xie F, High R, Pavlides G, Porter TR. Prevalence and predictive value of microvascular flow abnormalities after successful contemporary percutaneous coronary intervention in acute ST-segment elevation myocardial infarction. J Am Soc Echocardiogr. (2018) 31:674–82. doi: 10.1016/j.echo.2018.01.009
84. Wei K, Ragosta M, Thorpe J, Coggins M, Moos S, Kaul S. Noninvasive quantification of coronary blood flow reserve in humans using myocardial contrast echocardiography. Circulation. (2001) 103:2560–5. doi: 10.1161/01.CIR.103.21.2560
85. Vogel R, Indermuhle A, Reinhardt J, Meier P, Siegrist PT, Namdar M, et al. The quantification of absolute myocardial perfusion in humans by contrast echocardiography: algorithm and validation. J Am Coll Cardiol. (2005) 45:754–62. doi: 10.1016/j.jacc.2004.11.044
86. Anagnostopoulos C, Georgakopoulos A, Pianou N, Nekolla SG. Assessment of myocardial perfusion and viability by positron emission tomography. Int J Cardiol. (2013) 167:1737–49. doi: 10.1016/j.ijcard.2012.12.009
87. Nesterov SV, Deshayes E, Sciagra R, Settimo L, Declerck JM, Pan XB, et al. Quantification of myocardial blood flow in absolute terms using (82)Rb PET imaging: the RUBY-10 Study. JACC Cardiovasc Imaging. (2014) 7:1119–27. doi: 10.1016/j.jcmg.2014.08.003
88. Lortie M, Beanlands RS, Yoshinaga K, Klein R, Dasilva JN, DeKemp RA. Quantification of myocardial blood flow with 82Rb dynamic PET imaging. Eur J Nucl Med Mol Imaging. (2007) 34:1765–74. doi: 10.1007/s00259-007-0478-2
89. Dekemp RA, Declerck J, Klein R, Pan XB, Nakazato R, Tonge C, et al. Multisoftware reproducibility study of stress and rest myocardial blood flow assessed with 3D dynamic PET/CT and a 1-tissue-compartment model of 82Rb kinetics. J Nucl Med. (2013) 54:571–7. doi: 10.2967/jnumed.112.112219
90. Murthy VL, Bateman TM, Beanlands RS, Berman DS, Borges-Neto S, Chareonthaitawee P, et al. Clinical quantification of myocardial blood flow using PET: joint position paper of the SNMMI cardiovascular council and the ASNC. J Nucl Cardiol. (2018) 25:269–97. doi: 10.1007/s12350-017-1110-x
91. AlBadri A, Piccinelli M, Cho SG, Lee JM, Jaber W, De Cecco CN, et al. Rationale and design of the quantification of myocardial blood flow using dynamic PET/CTA-fused imagery (DEMYSTIFY) to determine physiological significance of specific coronary lesions. J Nucl Cardiol. (2020) 27:1030–9. doi: 10.1007/s12350-020-02052-0
92. Tonet E, Pompei G, Faragasso E, Cossu A, Pavasini R, Passarini G, et al. Coronary microvascular dysfunction: PET, CMR and CT assessment. J Clin Med. (2021) 10:1848. doi: 10.3390/jcm10091848
93. Dilsizian V, Bacharach SL, Beanlands RS, Bergmann SR, Delbeke D, Dorbala S, et al. ASNC imaging guidelines/SNMMI procedure standard for positron emission tomography (PET) nuclear cardiology procedures. J Nucl Cardiol. (2016) 23:1187–226. doi: 10.1007/s12350-016-0522-3
94. Taqueti VR, Shaw LJ, Cook NR, Murthy VL, Shah NR, Foster CR, et al. Excess cardiovascular risk in women relative to men referred for coronary angiography is associated with severely impaired coronary flow reserve, not obstructive disease. Circulation. (2017) 135:566–77. doi: 10.1161/CIRCULATIONAHA.116.023266
95. Florian A. Diastolic dysfunction in women with ischemia and non-obstructive coronary arteries (INOCA) - Could non-invasive imaging reveal the missing piece of the puzzle? Int J Cardiol. (2021) 4:24. doi: 10.1016/j.ijcard.2021.04.024
96. Bateman TM, Dilsizian V, Beanlands RS, DePuey EG, Heller GV, Wolinsky DA. American Society of nuclear cardiology and society of nuclear medicine and molecular imaging joint position statement on the clinical indications for myocardial perfusion PET. J Nucl Med. (2016) 57:1654–6. doi: 10.2967/jnumed.116.180448
97. Bravo PE, Pinheiro A, Higuchi T, Rischpler C, Merrill J, Santaularia-Tomas M, et al. PET/CT assessment of symptomatic individuals with obstructive and nonobstructive hypertrophic cardiomyopathy. J Nucl Med. (2012) 53:407–14. doi: 10.2967/jnumed.111.096156
98. Chih S, Chong AY, Erthal F, deKemp RA, Davies RA, Stadnick E, et al. PET Assessment of epicardial intimal disease and microvascular dysfunction in cardiac allograft vasculopathy. J Am Coll Cardiol. (2018) 71:1444–56. doi: 10.1016/j.jacc.2018.01.062
99. Di Carli MF, Charytan D, McMahon GT, Ganz P, Dorbala S, Schelbert HR. Coronary circulatory function in patients with the metabolic syndrome. J Nucl Med. (2011) 52:1369–77. doi: 10.2967/jnumed.110.082883
100. Quinones MJ, Hernandez-Pampaloni M, Schelbert H, Bulnes-Enriquez I, Jimenez X, Hernandez G, et al. Coronary vasomotor abnormalities in insulin-resistant individuals. Ann Intern Med. (2004) 140:700–8. doi: 10.7326/0003-4819-140-9-200405040-00009
101. Yokoyama I, Momomura S, Ohtake T, Yonekura K, Nishikawa J, Sasaki Y, et al. Reduced myocardial flow reserve in non-insulin-dependent diabetes mellitus. J Am Coll Cardiol. (1997) 30:1472–7. doi: 10.1016/S0735-1097(97)00327-6
102. Herzog BA, Husmann L, Valenta I, Gaemperli O, Siegrist PT, Tay FM, et al. Long-term prognostic value of 13N-ammonia myocardial perfusion positron emission tomography added value of coronary flow reserve. J Am Coll Cardiol. (2009) 54:150–6. doi: 10.1016/j.jacc.2009.02.069
103. Farhad H, Dunet V, Bachelard K, Allenbach G, Kaufmann PA, Prior JO. Added prognostic value of myocardial blood flow quantitation in rubidium-82 positron emission tomography imaging. Eur Heart J Cardiovasc Imaging. (2013) 14:1203–10. doi: 10.1093/ehjci/jet068
104. Sharir T, Brodkin B. Can myocardial perfusion imaging predict outcome in patients with angina and ischemia but no obstructive coronary artery disease (INOCA)? J Nucl Cardiol. (2020) 28:3038–43. doi: 10.1007/s12350-020-02338-3
105. Gould KL, Johnson NP, Bateman TM, Beanlands RS, Bengel FM, Bober R, et al. Anatomic versus physiologic assessment of coronary artery disease. Role of coronary flow reserve, fractional flow reserve, and positron emission tomography imaging in revascularization decision-making. J Am Coll Cardiol. (2013) 62:1639–53. doi: 10.1016/j.jacc.2013.07.076
106. Dewey M, Siebes M, Kachelriess M, Kofoed KF, Maurovich-Horvat P, Nikolaou K, et al. Clinical quantitative cardiac imaging for the assessment of myocardial ischaemia. Nat Rev Cardiol. (2020) 17:427–50. doi: 10.1038/s41569-020-0341-8
107. Sugihara H, Yonekura Y, Kataoka K, Fukai D, Kitamura N, Taniguchi Y. Estimation of coronary flow reserve with the use of dynamic planar and SPECT images of Tc-99m tetrofosmin. J Nucl Cardiol. (2001) 8:575–9. doi: 10.1067/mnc.2001.115934
108. Petretta M, Storto G, Pellegrino T, Bonaduce D, Cuocolo A. Quantitative assessment of myocardial blood flow with SPECT. Prog Cardiovasc Dis. (2015) 57:607–14. doi: 10.1016/j.pcad.2014.12.007
109. Storto G, Cirillo P, Vicario ML, Pellegrino T, Sorrentino AR, Petretta M, et al. Estimation of coronary flow reserve by Tc-99m sestamibi imaging in patients with coronary artery disease: comparison with the results of intracoronary Doppler technique. J Nucl Cardiol. (2004) 11:682–8. doi: 10.1016/j.nuclcard.2004.08.007
110. Ito Y, Katoh C, Noriyasu K, Kuge Y, Furuyama H, Morita K, et al. Estimation of myocardial blood flow and myocardial flow reserve by 99mTc-sestamibi imaging: comparison with the results of [15O]H2O PET. Eur J Nucl Med Mol Imaging. (2003) 30:281–7. doi: 10.1007/s00259-002-1031-y
111. Taki J, Fujino S, Nakajima K, Matsunari I, Okazaki H, Saga T, et al. (99m)Tc-sestamibi retention characteristics during pharmacologic hyperemia in human myocardium: comparison with coronary flow reserve measured by Doppler flowire. J Nucl Med. (2001) 42:1457–63.
112. Nudi F, Iskandrian AE, Schillaci O, Peruzzi M, Frati G, Biondi-Zoccai G. Diagnostic accuracy of myocardial perfusion imaging with CZT technology: systemic review and meta-analysis of comparison with invasive coronary angiography. JACC Cardiovasc Imaging. (2017) 10:787–94. doi: 10.1016/j.jcmg.2016.10.023
113. Slomka PJ, Miller RJH, Hu LH, Germano G, Berman DS. Solid-state detector SPECT myocardial perfusion imaging. J Nucl Med. (2019) 60:1194–204. doi: 10.2967/jnumed.118.220657
114. Liu L, Abdu FA, Yin G, Xu B, Mohammed AQ, Xu S, et al. Prognostic value of myocardial perfusion imaging with D-SPECT camera in patients with ischemia and no obstructive coronary artery disease (INOCA). J Nucl Cardiol. (2020) 28:3025–37. doi: 10.1007/s12350-020-02252-8
115. Jansen TPJ, Konst RE, Elias-Smale SE, van den Oord SC, Ong P, de Vos AMJ, et al. Assessing microvascular dysfunction in angina with unobstructed coronary arteries: JACC review topic of the week. J Am Coll Cardiol. (2021) 78:1471–9. doi: 10.1016/j.jacc.2021.08.028
116. Rossi A, Dharampal A, Wragg A, Davies LC, van Geuns RJ, Anagnostopoulos C, et al. Diagnostic performance of hyperaemic myocardial blood flow index obtained by dynamic computed tomography: does it predict functionally significant coronary lesions? Eur Heart J Cardiovasc Imaging. (2014) 15:85–94. doi: 10.1093/ehjci/jet133
117. Nieman K, Balla S. Dynamic CT myocardial perfusion imaging. J Cardiovasc Comput Tomogr. (2020) 14:303–6. doi: 10.1016/j.jcct.2019.09.003
118. Seitun S, De Lorenzi C, Cademartiri F, Buscaglia A, Travaglio N, Balbi M, et al. CT myocardial perfusion imaging: a new Frontier in cardiac imaging. Biomed Res Int. (2018) 2018:7295460. doi: 10.1155/2018/7295460
119. Conte E, Sonck J, Mushtaq S, Collet C, Mizukami T, Barbato E, et al. FFRCT and CT perfusion: a review on the evaluation of functional impact of coronary artery stenosis by cardiac CT. Int J Cardiol. (2020) 300:289–96. doi: 10.1016/j.ijcard.2019.08.018
120. Taylor CA, Fonte TA, Min JK. Computational fluid dynamics applied to cardiac computed tomography for noninvasive quantification of fractional flow reserve: scientific basis. J Am Coll Cardiol. (2013) 61:2233–41. doi: 10.1016/j.jacc.2012.11.083
121. West GB, Brown JH, Enquist BJ. A general model for the origin of allometric scaling laws in biology. Science. (1997) 276:122–6. doi: 10.1126/science.276.5309.122
122. Steele BN, Olufsen MS, Taylor CA. Fractal network model for simulating abdominal and lower extremity blood flow during resting and exercise conditions. Comput Methods Biomech Biomed Engin. (2007) 10:39–51. doi: 10.1080/10255840601068638
123. Itu L, Rapaka S, Passerini T, Georgescu B, Schwemmer C, Schoebinger M, et al. A machine-learning approach for computation of fractional flow reserve from coronary computed tomography. J Appl Physiol. (2016) 121:42–52. doi: 10.1152/japplphysiol.00752.2015
124. Xu Y, Yu L, Shen C, Lu Z, Zhu X, Zhang J. Prevalence and disease features of myocardial ischemia with non-obstructive coronary arteries: insights from a dynamic CT myocardial perfusion imaging study. Int J Cardiol. (2021) 334:142–7. doi: 10.1016/j.ijcard.2021.04.055
125. Abdelrahman KM, Chen MY, Dey AK, Virmani R, Finn AV, Khamis RY, et al. Coronary computed tomography angiography from clinical uses to emerging technologies: JACC State-of-the-art review. J Am Coll Cardiol. (2020) 76:1226–43. doi: 10.1016/j.jacc.2020.06.076
126. Al-Saadi N, Nagel E, Gross M, Bornstedt A, Schnackenburg B, Klein C, et al. Noninvasive detection of myocardial ischemia from perfusion reserve based on cardiovascular magnetic resonance. Circulation. (2000) 101:1379–83. doi: 10.1161/01.CIR.101.12.1379
127. Nagel E, Klein C, Paetsch I, Hettwer S, Schnackenburg B, Wegscheider K, et al. Magnetic resonance perfusion measurements for the noninvasive detection of coronary artery disease. Circulation. (2003) 108:432–7. doi: 10.1161/01.CIR.0000080915.35024.A9
128. Panting JR, Gatehouse PD, Yang GZ, Grothues F, Firmin DN, Collins P, et al. Abnormal subendocardial perfusion in cardiac syndrome X detected by cardiovascular magnetic resonance imaging. N Engl J Med. (2002) 346:1948–53. doi: 10.1056/NEJMoa012369
129. Shufelt CL, Thomson LE, Goykhman P, Agarwal M, Mehta PK, Sedlak T, et al. Cardiac magnetic resonance imaging myocardial perfusion reserve index assessment in women with microvascular coronary dysfunction and reference controls. Cardiovasc Diagn Ther. (2013) 3:153–60. doi: 10.3978/j.issn.2223-3652.2013.08.02
130. Doyle M, Weinberg N, Pohost GM, Bairey Merz CN, Shaw LJ, Sopko G, et al. Prognostic value of global MR myocardial perfusion imaging in women with suspected myocardial ischemia and no obstructive coronary disease: results from the NHLBI-sponsored WISE (Women's Ischemia Syndrome Evaluation) study. JACC Cardiovasc Imaging. (2010) 3:1030–6. doi: 10.1016/j.jcmg.2010.07.008
131. Thomson LE, Wei J, Agarwal M, Haft-Baradaran A, Shufelt C, Mehta PK, et al. Cardiac magnetic resonance myocardial perfusion reserve index is reduced in women with coronary microvascular dysfunction. A national heart, lung, and blood institute-sponsored study from the Women's Ischemia Syndrome Evaluation. Circ Cardiovasc Imaging. (2015) 8:e002481. doi: 10.1161/CIRCIMAGING.114.002481
132. Utz W, Niendorf T, Wassmuth R, Messroghli D, Dietz R, Schulz-Menger J. Contrast-dose relation in first-pass myocardial MR perfusion imaging. J Magn Reson Imaging. (2007) 25:1131–5. doi: 10.1002/jmri.20910
133. Wohrle J, Nusser T, Merkle N, Kestler HA, Grebe OC, Marx N, et al. Myocardial perfusion reserve in cardiovascular magnetic resonance: correlation to coronary microvascular dysfunction. J Cardiovasc Magn Reson. (2006) 8:781–7. doi: 10.1080/10976640600737649
134. Mygind ND, Pena A, Mide Michelsen M, Ali Qayyum A, Frestad D, Emil Christensen T, et al. Myocardial first pass perfusion assessed by cardiac magnetic resonance and coronary microvascular dysfunction in women with angina and no obstructive coronary artery disease. Scand J Clin Lab Invest. (2019) 79:238–46. doi: 10.1080/00365513.2019.1587670
135. Engblom H, Xue H, Akil S, Carlsson M, Hindorf C, Oddstig J, et al. Fully quantitative cardiovascular magnetic resonance myocardial perfusion ready for clinical use: a comparison between cardiovascular magnetic resonance imaging and positron emission tomography. J Cardiovasc Magn Reson. (2017) 19:78. doi: 10.1186/s12968-017-0388-9
136. Zhou W, Lee JCY, Leung ST, Lai A, Lee TF, Chiang JB, et al. Long-term prognosis of patients with coronary microvascular disease using stress perfusion cardiac magnetic resonance. JACC Cardiovasc Imaging. (2021) 14:602–11. doi: 10.1016/j.jcmg.2020.09.034
137. Konst RE, Damman P, Pellegrini D, van Royen N, Maas A, Elias-Smale SE. Diagnostic approach in patients with angina and no obstructive coronary artery disease: emphasising the role of the coronary function test. Neth Heart J. (2021) 29:121–8. doi: 10.1007/s12471-020-01532-9
138. Pijls NH, De Bruyne B, Peels K, Van Der Voort PH, Bonnier HJ, Bartunek JKJJ, et al. Measurement of fractional flow reserve to assess the functional severity of coronary-artery stenoses. N Engl J Med. (1996) 334:1703–8. doi: 10.1056/NEJM199606273342604
139. Bishop AH, Samady H. Fractional flow reserve: critical review of an important physiologic adjunct to angiography. Am Heart J. (2004) 147:792–802. doi: 10.1016/j.ahj.2003.12.009
140. Neumann FJ, Sousa-Uva M, Ahlsson A, Alfonso F, Banning AP, Benedetto U, et al. 2018 ESC/EACTS Guidelines on myocardial revascularization. Eur Heart J. (2019) 40:87–165. doi: 10.1093/eurheartj/ehy855
141. Adjedj J, De Bruyne B, Flore V, Di Gioia G, Ferrara A, Pellicano M, et al. Significance of intermediate values of fractional flow reserve in patients with coronary artery disease. Circulation. (2016) 133:502–8. doi: 10.1161/CIRCULATIONAHA.115.018747
142. Gotberg M, Christiansen EH, Gudmundsdottir IJ, Sandhall L, Danielewicz M, Jakobsen L, et al. Instantaneous wave-free ratio versus fractional flow reserve to guide PCI. N Engl J Med. (2017) 376:1813–23. doi: 10.1056/NEJMoa1616540
143. Toth G, Hamilos M, Pyxaras S, Mangiacapra F, Nelis O, De Vroey F, et al. Evolving concepts of angiogram: fractional flow reserve discordances in 4000 coronary stenoses. Eur Heart J. (2014) 35:2831–8. doi: 10.1093/eurheartj/ehu094
144. Doucette JW, Corl PD, Payne HM, Flynn AE, Goto M, Nassi M, et al. Validation of a Doppler guide wire for intravascular measurement of coronary artery flow velocity. Circulation. (1992) 85:1899–911. doi: 10.1161/01.CIR.85.5.1899
145. Everaars H, de Waard GA, Driessen RS, Danad I, van de Ven PM, Raijmakers PG, et al. Doppler flow velocity and thermodilution to assess coronary flow reserve: a head-to-head comparison with [(15)O]H2O PET. JACC Cardiovasc Interv. (2018) 11:2044–54. doi: 10.1016/j.jcin.2018.07.011
146. Pijls NH, De Bruyne B, Smith L, Aarnoudse W, Barbato E, Bartunek J, et al. Coronary thermodilution to assess flow reserve: validation in humans. Circulation. (2002) 105:2482–6. doi: 10.1161/01.CIR.0000017199.09457.3D
147. Luo C, Long M, Hu X, Huang Z, Hu C, Gao X, et al. Thermodilution-derived coronary microvascular resistance and flow reserve in patients with cardiac syndrome X. Circ Cardiovasc Interv. (2014) 7:43–8. doi: 10.1161/CIRCINTERVENTIONS.113.000953
148. Adjedj J, Toth GG, De Bruyne B. Invasive measures of myocardial perfusion and ischemia. Prog Cardiovasc Dis. (2015) 57:555–65. doi: 10.1016/j.pcad.2015.03.002
149. Fearon WF, Balsam LB, Farouque HM, Caffarelli AD, Robbins RC, Fitzgerald PJ, et al. Novel index for invasively assessing the coronary microcirculation. Circulation. (2003) 107:3129–32. doi: 10.1161/01.CIR.0000080700.98607.D1
150. De Bruyne B, Barbato E. Quantitative assessment of the coronary microvasculature: new tools for the black box. Circulation. (2013) 127:2378–9. doi: 10.1161/CIRCULATIONAHA.113.003361
151. de Waard GA, Fahrni G, de Wit D, Kitabata H, Williams R, Patel N, et al. Hyperaemic microvascular resistance predicts clinical outcome and microvascular injury after myocardial infarction. Heart. (2018) 104:127–34. doi: 10.1136/heartjnl-2017-311431
152. van de Hoef TP, Nolte F, EchavarrIa-Pinto M, van Lavieren MA, Damman P, Chamuleau SA, et al. Impact of hyperaemic microvascular resistance on fractional flow reserve measurements in patients with stable coronary artery disease: insights from combined stenosis and microvascular resistance assessment. Heart. (2014) 100:951–9. doi: 10.1136/heartjnl-2013-305124
153. Ford TJ, Corcoran D, Berry C. Coronary artery disease: physiology and prognosis. Eur Heart J. (2017) 38:1990–2. doi: 10.1093/eurheartj/ehx226
154. Blair JE, Ricciardi MJ. Assessment of coronary blood flow in the cardiac catheterization laboratory. Curr Probl Cardiol. (2014) 39:159–84. doi: 10.1016/j.cpcardiol.2014.02.002
155. De Bruyne B, Oldroyd KG, Pijls NHJ. Microvascular (Dys)Function and Clinical Outcome in Stable Coronary Disease. J Am Coll Cardiol. (2016) 67:1170–2. doi: 10.1016/j.jacc.2015.11.066
156. Kern MJ. Coronary physiology revisited: practical insights from the cardiac catheterization laboratory. Circulation. (2000) 101:1344–51. doi: 10.1161/01.CIR.101.11.1344
157. Baumgart D, Haude M, Liu F, Ge J, Goerge G, Erbel R. Current concepts of coronary flow reserve for clinical decision making during cardiac catheterization. Am Heart J. (1998) 136:136–49. doi: 10.1016/S0002-8703(98)70194-2
158. Solberg OG, Ragnarsson A, Kvarsnes A, Endresen K, Kongsgard E, Aakhus S, et al. Reference interval for the index of coronary microvascular resistance. EuroIntervention. (2014) 9:1069–75. doi: 10.4244/EIJV9I9A181
159. AlBadri A, Mavromatis K, Bairey Merz CN. The role of coronary reactivity testing in women with no obstructive coronary artery disease. Curr Opin Cardiol. (2019) 34:656–62. doi: 10.1097/HCO.0000000000000682
160. Konst RE, Meeder JG, Wittekoek ME, Maas A, Appelman Y, Piek JJ, et al. Ischaemia with no obstructive coronary arteries. Neth Heart J. (2020) 28(Suppl 1):66–72. doi: 10.1007/s12471-020-01451-9
161. Whayne TF Jr, Sousa MJ, Abdel-Latif A. Use and value of fractional flow reserve in coronary arteriography. Angiology. (2020) 71:5–9. doi: 10.1177/0003319719848559
162. Lee JM, Jung JH, Hwang D, Park J, Fan Y, Na SH, et al. Coronary flow reserve and microcirculatory resistance in patients with intermediate coronary stenosis. J Am Coll Cardiol. (2016) 67:1158–69. doi: 10.1016/j.jacc.2015.12.053
163. AlBadri A, Bairey Merz CN, Johnson BD, Wei J, Mehta PK, Cook-Wiens G, et al. Impact of abnormal coronary reactivity on long-term clinical outcomes in women. J Am Coll Cardiol. (2019) 73:684–93. doi: 10.1016/j.jacc.2018.11.040
164. Seitz A, Gardezy J, Pirozzolo G, Probst S, Athanasiadis A, Hill S, et al. Long-term follow-up in patients with stable angina and unobstructed coronary arteries undergoing intracoronary acetylcholine testing. JACC Cardiovasc Interv. (2020) 13:1865–76. doi: 10.1016/j.jcin.2020.05.009
165. Ford TJ, Stanley B, Sidik N, Good R, Rocchiccioli P, McEntegart M, et al. 1-year outcomes of angina management guided by invasive coronary function testing (CorMicA). JACC Cardiovasc Interv. (2020) 13:33–45. doi: 10.1016/j.jcin.2019.11.001
166. Suda A, Takahashi J, Hao K, Kikuchi Y, Shindo T, Ikeda S, et al. Coronary functional abnormalities in patients with angina and nonobstructive coronary artery disease. J Am Coll Cardiol. (2019) 74:2350–60. doi: 10.1016/j.jacc.2019.08.1056
167. Ford TJ, Stanley B, Good R, Rocchiccioli P, McEntegart M, Watkins S, et al. Stratified medical therapy using invasive coronary function testing in angina: the CorMicA trial. J Am Coll Cardiol. (2018) 72(23 Pt A):2841–55. doi: 10.1016/j.jacc.2018.09.006
168. Ciliberti G, Seshasai SRK, Ambrosio G, Kaski JC. Safety of intracoronary provocative testing for the diagnosis of coronary artery spasm. Int J Cardiol. (2017) 244:77–83. doi: 10.1016/j.ijcard.2017.05.109
169. Sueda S, Kohno H. Overview of complications during pharmacological spasm provocation tests. J Cardiol. (2016) 68:1–6. doi: 10.1016/j.jjcc.2016.03.005
170. Barbato E, Aarnoudse W, Aengevaeren WR, Werner G, Klauss V, Bojara W, et al. Validation of coronary flow reserve measurements by thermodilution in clinical practice. Eur Heart J. (2004) 25:219–23. doi: 10.1016/j.ehj.2003.11.009
171. De Bruyne B, Adjedj J, Xaplanteris P, Ferrara A, Mo Y, Penicka M, et al. Saline-induced coronary hyperemia: mechanisms and effects on left ventricular function. Circ Cardiovasc Interv. (2017) 10:719. doi: 10.1161/CIRCINTERVENTIONS.116.004719
172. Pijls NHJ, de Vos AMJ, Keulards DCJ. Measurement of absolute coronary blood flow and microvascular resistance: a new window to coronary microcirculation. J Am Coll Cardiol. (2021) 77:742–4. doi: 10.1016/j.jacc.2020.12.016
173. Konst RE, Elias-Smale SE, Pellegrini D, Hartzema-Meijer M, van Uden BJC, Jansen TPJ, et al. Absolute coronary blood flow measured by continuous thermodilution in patients with ischemia and nonobstructive disease. J Am Coll Cardiol. (2021) 77:728–41. doi: 10.1016/j.jacc.2020.12.019
174. Candreva A, Gallinoro E, van 't Veer M, Sonck J, Collet C, Di Gioia G, et al. Basics of coronary thermodilution. JACC Cardiovasc Interv. (2021) 14:595–605. doi: 10.1016/j.jcin.2020.12.037
175. Tu S, Westra J, Yang J, von Birgelen C, Ferrara A, Pellicano M, et al. Diagnostic accuracy of fast computational approaches to derive fractional flow reserve from diagnostic coronary angiography: the international multicenter FAVOR pilot study. JACC Cardiovasc Interv. (2016) 9:2024–35. doi: 10.1016/j.jcin.2016.07.013
176. Westra J, Andersen BK, Campo G, Matsuo H, Koltowski L, Eftekhari A, et al. Diagnostic performance of in-procedure angiography-derived quantitative flow reserve compared to pressure-derived fractional flow reserve: the FAVOR II Europe-Japan Study. J Am Heart Assoc. (2018) 7:e009603. doi: 10.1161/JAHA.118.009603
177. Xu B, Tu S, Qiao S, Qu X, Chen Y, Yang J, et al. Diagnostic accuracy of angiography-based quantitative flow ratio measurements for online assessment of coronary stenosis. J Am Coll Cardiol. (2017) 70:3077–87. doi: 10.1016/j.jacc.2017.10.035
178. Ties D, van Dijk R, Pundziute G, Lipsic E, Vonck TE, van den Heuvel AFM, et al. Computational quantitative flow ratio to assess functional severity of coronary artery stenosis. Int J Cardiol. (2018) 271:36–41. doi: 10.1016/j.ijcard.2018.05.002
179. Tebaldi M, Biscaglia S, Di Girolamo D, Erriquez A, Penzo C, Tumscitz C, et al. Angio-based index of microcirculatory resistance for the assessment of the coronary resistance: a proof of concept study. J Interv Cardiol. (2020) 2020:8887369. doi: 10.1155/2020/8887369
180. Mejia-Renteria H, Lee JM, Lauri F, van der Hoeven NW, de Waard GA, Macaya F, et al. Influence of microcirculatory dysfunction on angiography-based functional assessment of coronary stenoses. JACC Cardiovasc Interv. (2018) 11:741–53. doi: 10.1016/j.jcin.2018.02.014
Keywords: ischemia with non-obstructive coronary arteries (INOCA), coronary microvascular dysfunction, coronary function test, diagnosis, pathophysiology, vasospasm
Citation: Fu B, Wei X, Lin Y, Chen J and Yu D (2022) Pathophysiologic Basis and Diagnostic Approaches for Ischemia With Non-obstructive Coronary Arteries: A Literature Review. Front. Cardiovasc. Med. 9:731059. doi: 10.3389/fcvm.2022.731059
Received: 26 June 2021; Accepted: 31 January 2022;
Published: 17 March 2022.
Edited by:
Ali Ahmad, Mayo Clinic, United StatesReviewed by:
Janet Wei, Smidt Heart Institute, United StatesMiodrag Janic, University Medical Centre Ljubljana, Slovenia
Copyright © 2022 Fu, Wei, Lin, Chen and Yu. This is an open-access article distributed under the terms of the Creative Commons Attribution License (CC BY). The use, distribution or reproduction in other forums is permitted, provided the original author(s) and the copyright owner(s) are credited and that the original publication in this journal is cited, in accordance with accepted academic practice. No use, distribution or reproduction is permitted which does not comply with these terms.
*Correspondence: Danqing Yu, gdydq100@126.com