- 1Department of Cardiology, Hospital do Espírito Santo de Évora, Évora, Portugal
- 2Department of Cardiology, Barts Heart Centre, Barts Health NHS Trust, London, United Kingdom
- 3Department of Cardiology, Newham University Hospital, Barts Health NHS Trust, London, United Kingdom
- 4Institute of Health Informatics Research, University College London, London, United Kingdom
- 5Cardiovascular Division, University of Pennsylvania, Philadelphia, PA, United States
- 6Department of Cardiovascular Medicine, Mayo Clinic, Rochester, MN, United States
- 7Centre for Inherited Cardiovascular Diseases, WellSpan Cardiology, Lancaster, PA, United States
- 8Division of Cardiology, Department of Medical Biotechnologies, University of Siena, Siena, Italy
- 9Department of Cardio-Thoraco-Vascular Sciences and Public Health, University of Padua, Padua, Italy
- 10Department of Neuroscience, Imaging and Clinical Sciences, “G.d'Annunzio” University of Chieti-Pescara, Chieti, Italy
- 11Department of Cardiology, Casa di Cura Villa Serena, Città Sant'Angelo, Italy
- 12Department of Clinical Sciences, Lund University, Malmö, Sweden
- 13NIHR Biomedical Research Unit, William Harvey Research Institute, Queen Mary University, London, United Kingdom
Sudden cardiac arrest (SCA) in young athletes is rare, with an estimated incidence ranging from 0.1 to 2 per 100,000 per athlete year. The creation of SCA registries can help provide accurate data regarding incidence, treatment, and outcomes and help implement primary or secondary prevention strategies that could change the course of these events. Early cardiopulmonary resuscitation (CPR) and defibrillation are the most important determinants of survival and neurological prognosis in individuals who suffer from SCA. Compared with the general population, individuals with clinically silent cardiac disease who practice regular physical exercise are at increased risk of SCA events. While the implementation of national preparticipation screening has been largely debated, with no current consensus, the number of athletes who will be diagnosed with cardiac disease and have an indication for implantable defibrillator cardioverter defibrillator (ICD) is unknown. Many victims of SCA do not have a previous cardiac diagnosis. Therefore, the appropriate use and availability of automated external defibrillators (AEDs) in public spaces is the crucial part of the integrated response to prevent these fatalities both for participating athletes and for spectators. Governments and sports institutions should invest and educate members of the public, security, and healthcare professionals in immediate initiation of CPR and early AED use. Smartphone apps could play an integral part to allow bystanders to alert the emergency services and CPR trained responders and locate and utilize the nearest AED to positively influence the outcomes by strengthening the chain of survival. This review aims to summarize the available evidence on sudden cardiac death prevention among young athletes and to provide some guidance on strategies that can be implemented by governments and on the novel tools that can help save these lives.
Introduction
The annual incidence of out-of-hospital cardiac arrest (OHCA) in the general population is estimated between 67 and 170 per 100,000 inhabitants in Europe (1) and 57 per 100,000 inhabitants in the United States (US) (2), widely varying between and within countries. In addition, the causes of sudden cardiac death (SCD) might also differ among different countries, possibly because of differences in population genetics and myocardial substrate and the systematic preparticipation evaluation of athletes (3). Cardiopulmonary resuscitation (CPR) initiated by bystanders is reported to be performed in about half of cases, with significant differences between countries (4).
Sudden cardiac arrest (SCA) or SCD in young athletes is even rarer, although it is often an event of great public attention. In 2014, Harmon and colleagues reviewed the incidence of SCD in athletes and concluded that studies with higher methodological quality consistently yielded incidence rates in the range of 1:40,000–1:80,000, and assumed an overall incidence of 1:50,000 in young athletes is a reasonable estimate (5). In Table 1 and Figure 1, we summarize data from studies published from 2006 to 2021, with incidences of SCA ranging from 0.1 to 2 per 100,000 athlete-year (6–24). Most of these studies have shown that the majority of SCA events occur during exercise, despite possible selection bias because of the study sources including databases of more commonly sports organization and media report reviews.
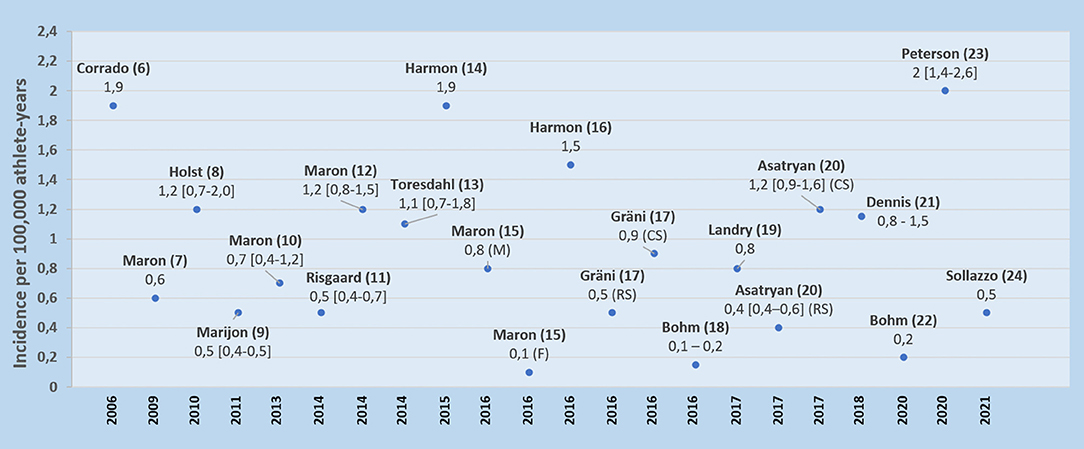
Figure 1. Representation of incidences of sports-related sudden cardiac arrests by ascending date of publication. Mean incidence [95% CI included where available]. CS, competitive sports; F, female; M, male; RS, recreational sports.
The wide variation in incidences reported might reflect the underreporting of SCA events and the lack of appropriate national sports registries (25) that might allow a more precise epidemiological description of the problem.
This document aims at summarizing the available evidence on SCD prevention among young athletes, and to provide some guidance on strategies that can be implemented by governments and on the novel tools that can help save these lives.
Increasing Training and Delivery of Bystander CPR
Governments and sports institutions could invest in educating members of the public, security personnel, and healthcare professionals in the identification of SCA, calling for appropriate help, early initiation of effective CPR, and automated external defibrillator (AED) use, which can be highly lifesaving (26, 27). People are generally unaware of how to deal with SCA events, although teaching CPR maneuvers is valuable and easy, as evidenced that even training as short as 2 h can lead to a major increase in the willingness to start CPR and AED use (28). In addition, after the Australian government provided basic life support (BLS) and AED use training, a 6-month follow-up survey demonstrated highly accurate answers to clinical scenarios involving AED use, although only half of the respondents reported having access to an AED (29).
Training and raising awareness among the population to CPR and eventually AED use should be provided as part of the school civic education, as it is an important issue of public health. This has already been recognized by governments of many countries across Europe and in the US that have legal requirements for CPR education in schools (30, 31). However, it is not known whether legislation has translated into implementation, as demonstrated by a Danish group that performed a nationwide study and demonstrated that school CPR training has not been successfully implemented following 8 years of mandating legislation (32). Over 10 years, temporal trends in volunteer CPR delivery and long-term survival were studied in Denmark, after several national initiatives were implemented to strengthen bystander resuscitation attempts (33). These initiatives included mandatory CPR training in elementary schools, as well as when acquiring a driving license, combined with an increase in voluntary first-aid training (33). An increase in bystander CPR was verified, and it was significantly associated with a concomitant increase in survival following OHCA (33). In 2015, the WHO endorsed the European Resuscitation Council initiative “Kids save lives.” This initiative is meant to deal with the gap in the education of CPR, starting with training children from the age of 12 years, for 2-h every year, as a part of educational project of the schools (30). However, in 2018, Semeraro et al. found that although education of children in resuscitation is mandatory by law in schools in six countries in Europe and it is a recommendation in another 24 countries, full implementation has not yet been achieved in the majority of them (34). In the US, the effectiveness of school-based AED programs was also studied and it was high, with an AED application in 85% of SCA victims and 85% survival to hospital discharge among students after an event (35).
Effectiveness of Bystander Defibrillation
Efficacy of CPR and AED Use
Sudden cardiac arrest may be caused by asystole, complete heart block with ventricular standstill, electromechanical dissociation/pulseless electrical activity (PEA), pulseless ventricular tachycardia (VT), or ventricular fibrillation (VF). While patients who present with asystole or pulseless electrical activity have a poor prognosis despite CPR delivery, those in whom the first documented rhythm is VT or VF can be effectively treated by defibrillation. In a prospective national survey of the national French ambulance service, involving subjects with 10 to 75 years who suffered sports-related SCD, the first reported rhythm was VF or pulseless VT in 47%, asystole in 42%, and PEA in 11% (9). Therefore, a relevant proportion of underlying arrhythmias for sports-related SCA can potentially be reverted by an AED shock (36).
Although primary prevention by screening professional athletes for cardiovascular diseases at risk for SCA is undertaken, combining this with increased CPR training and the availability of AED, will increase the likelihood of survival of individuals with unpredictable SCA (37). The important role of bystander-provided defibrillation in individuals who suffer OHCA is corroborated by several studies. After the implementation of a nationwide CPR and AED use training in school students in Japan, a retrospective study in elementary and middle school students demonstrated that children were more likely to be defibrillated by bystanders and had better neurological outcomes and 1-month survival when the cardiac arrest occurred in schools and other public places (38). Similarly, a systematic review of SCA in schools has shown that outcomes are better than when occurring at other locations, probably because of more frequent witnessed collapses and bystander CPR delivery (39). Aside from schools, other public places such as airports, that serve millions of passengers each year, where the risk of occurrence of SCA events is higher, and where short response times that save lives have been evaluated. As an example, in a prospective study in the three Chicago airports, equipped with seventeen AEDs, eighteen ventricular fibrillations occurred throughout years 2 years (40). In four of these cases, defibrillators were neither nearby nor used within 5 min, and the patients died (40). The overall one-year survival rate with a good neurological outcome was 56% (40). AEDs were also proven to be useful and efficacious when used on commercial airplanes, where early response by the prehospital emergency services is usually not possible (41). In a study performed in this setting, the rate of survival to hospital discharge shock after the shock was 40% and there were no inappropriate shocks (41). Finally, in a prospective observational cohort which included 2,500 shockable OHCA observed by the public, the authors found that survival to hospital discharge [adjusted odds ratio (OR) 2.62, 95% CI 2.07–3.31] and favorable functional outcome (adjusted OR 2.73, 95% CI 2.17–3.44) were significantly higher when a bystander rather than emergency medical services (EMSs) professionals provided the initial shock, and the benefit of bystander shock increased progressively as EMS response time became longer (42). Despite the high efficacy of AED use in public places, there is limited AED availability in public spaces in the majority of countries (1) and the scenario is as abysmal in nonprofessional athletic clubs (28, 43, 44). Furthermore, bystander AED use occurs in <2% of OHCAs, and the median arrival time of EMS can extend to 30 min in remote areas, where drone-delivery AED systems might increase the chances of survival (45).
Cost-Effectiveness of AED Use
The cost-effectiveness of training lay volunteers in CPR and AED use and public access to AED has not been well-studied (46), but may be considered a limitation of the strategy. In the Public Access Defibrillation (PAD) trial, 993 community facilities were randomly assigned to a structured emergency response strategy involving lay volunteers trained either in CPR alone or in CPR and the use of AEDs (47). Community facilities included shopping centers, office buildings, recreational/entertainment complexes, hotels, and apartment complexes that were eligible if an equivalent of at least 250 adults of more than 50 years were present for 16 h a day, or if there was a history of one SCA every 2 years (47). The mean number of volunteers trained per facility was 20 (range 1–149). These were laypersons who received training at enrollment and were retrained after 3–6 months and at least once after that (47). The addition of AED use before EMS arrival improved SCA survival to hospital discharge by 2-fold (95% CI 1.07–3.77) (47) and defibrillation by volunteers was associated with an incremental cost of mean $46,700 (95% CI $23,100–$68,600) per quality-adjusted life year, compared with CPR alone, which was stated to be an acceptable difference (48). In the CPR plus AED group, equipment plus training costs $4,453 per cardiac arrest (48). Therefore, in the athlete population, assuming an incidence of 1 per 50,000 athletes-year (5), one should expect to spend 28 times more than in the general population (estimated incidence of SCA in the US: 57 per 100,000 inhabitants—see Introduction part), thus $124,684 per 1 athlete-life saved. Although this may not be cost-effective if only considering the athlete, we should not forget about the risk of SCA in spectators, particularly those older and with cardiovascular risk factors. Furthermore, in a meta-analysis of 1,583 cases (including data from the PAD trial), the number of SCA needed to be treated (NNT) by nonhealthcare professionals trained in CPR plus AED to gain one survival to hospital admission was 17 (49).
Availability of AEDs
Competitive athletes who collapse on exertion can theoretically be rapidly assisted by trained healthcare professionals or club personnel and be defibrillated when an AED is available. SCA was associated with an 8-times higher survival rate compared with nonsports-related SCA, mainly because of better initial management, including bystander CPR and AED use (50). Nevertheless, many studies have demonstrated suboptimal CPR and AED application (28, 43, 44). While professional athletes frequently play in competitions under the supervision of a medical team that is ready to act whenever there is a collapse on the field, amateur athletes are more vulnerable to death after SCA gave the poorer ability of bystanders to assist in this setting. In the Gaelic Athletic Association (GAA), one of the great amateur sporting associations in the world, a survey demonstrated that 60% of the respondents reported that their club owned an AED and only 53% noted to have received formal training to use it (43). Several other studies have demonstrated that the knowledge and willingness to use AED is relatively low among participants in amateur clubs (28). As an example, among 218 amateur sports clubs in Ireland, 81.3% owned an AED and 12.9% admitted to not maintaining it on a regular basis (44).
Recent Developments in AED Technology
Recently, AED suppliers have worked on devices that are smaller, weight lightweight, and designed to be used by anyone, even children. As an example, HeartHero AED is a miniaturized, portable, and user-friendly AED that guides the user through the CPR process with auditory and visual guides (https://hearthero.com). Although it is not yet FDA approved, it is already available in 33 countries (cost ~€595, £495) with the potential to become a useful tool for people at increased risk of SCA that can store the potentially life-saving device at home or carry it with them, ensuring instant access to an AED. In addition, some new AEDs technology allows recording data from the moment it is attached to the patient and makes it transmittable to emergency services and hospitals, thus providing more accurate patient care. Potential limitations for wider use could include the cost for individuals and potential cost-effectiveness given the need for maintenance for appropriate functioning and the fact that those at increased risk of SCA may already have or be eligible for an implantable defibrillator.
Smartphone APP To Locate Nearby AEDS and CPR Trained Laypersons
In the 2021 European Resuscitation Council guidelines, it is highly encouraged that potential first responders (layperson, police officers, firefighters, and off-duty healthcare professionals) who are near the SCA victim should be notified through an alerting system using a smartphone app or text messaging (Table 2) (30). An example of such a system has been reported by Dutch investigators, consisting of a text-message alert system activated by the EMS to dispatch lay rescuers who are close to the victim and locate nearby AEDs (51). This system implementation was associated with a connection of the patient to AED in <6 min in 12.3% and early (≤ 6 min) defibrillation in 7.3% of the cases. In addition, a Swedish group published a blinded randomized controlled trial, where a mobile phone positioning system was used to locate trained responders within 500 meters of patients suffering SCA, at the moment EMS ambulances were dispatched. This system was associated with significantly increased rates of bystander-initiated CPR (62 vs. 48%, p < 0.001) (52). In another study from Sweden, when testing a similar system, lay responders arrived at the scene before the EMSs in 26% of the cases, and in 9% it they was able to attach an AED (53). Heartrunner™ (Heartrunner Sweden AB, Sweden) is the Swedish app that connects the EMS with 188,500 citizen responders and 5,000,000 AEDs around Sweden and Denmark (https://heartrunner.com/about-the-system/). When comparing citizen responders arriving before EMS, the early arrival of Heartrunner™-dispatched citizen responders was associated with almost 2-fold increased odds for bystander CPR and more than 3-fold increase in odds for bystander defibrillation (54). Another big player in lay response recruiting and AED localization in the United Kingdom is the GoodSAM™ app (GoodSAM LTD, United Kingdom), which accounts for a database of more than 50,000 AEDs and over 40,000 volunteers registered worldwide (https://www.goodsamapp.org). This app also has a GoodSAM Alerter™ version (GoodSAM LTD, United Kingdom), where laypeople can not only register AEDs, but also press the “Call for Help button” when they witness an emergency or need to get help quickly, and therefore activate both the EMS and the nearest lay volunteer. Finally, many useful apps are yielding multiple exercises, knowledge quizzes, and other information about CPR and cardiac arrest and can be easily downloaded for free by lay people [e.g., Hartstichting™, Netherlands, and the Resuscitation Council UK Lifesavers game app (https://www.resus.org.uk/public-resource/how-we-save-lives/lifesaver-learning/lifesaver)].
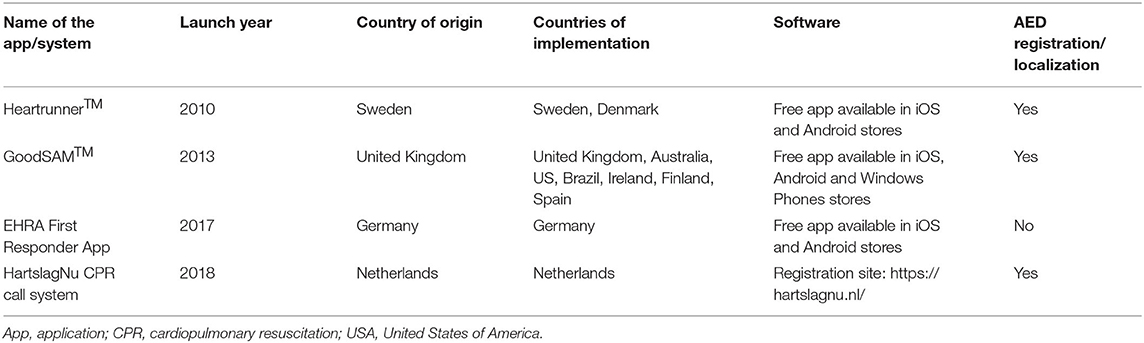
Table 2. First responder notification and contactless cardiac arrest detection systems using smart devices.
Athletes With Potentially Arrhythmogenic Diseases
Causes of SCA in Athletes
The causes of SCA/D in young athletes vary among different series and countries due in part to the varying methods of referral and ascertainment. While some authors recognized hypertrophic cardiomyopathy (HCM) as the most frequent cause of SCD in athletes from the United States (15, 23) others identified autopsy negative sudden unexplained death (AN-SUD) as the single most common etiology (14, 55). Arrhythmogenic right ventricular cardiomyopathy has been reported to account for approximately one-fifth of fatal cases in the Veneto Region of Italy (56). Different age groups, ethnicity, and genetics distribution, and also the inclusion of cases of SCA in SCD cohorts may all account for the varying etiologies found in these studies. Peterson and collaborators investigated the etiology of SCA/D in US competitive athletes (mean age 16.7 (11–29) years), by reviewing autopsy reports, death certificates, and medical records (23). In this prospective study, the most common cause of SCA/D across all age levels was HCM (21%), followed by idiopathic left ventricular hypertrophy (LVH) (13%), coronary artery anomalies (12%), AN-SUD (10%), arrhythmogenic cardiomyopathy (6%), long QT syndrome (5%) and commotion cordis (5%) (23). Similarly, Maron and collaborators found that HCM (36%) was the single most common cause of SCD in young athletes (mean age 19 ± 6 years), followed by coronary artery anomalies (19%) and idiopathic LVH (9%) (15). This contrasts with finding from another US study in which AN-SUD was the most frequent etiology of SCD, found in 25% among athletes 17–24 years of age (14). It was followed by coronary artery anomalies (11%), myocarditis (10%), and coronary artery disease (10%), and the incidence of cases of HCM and idiopathic LVH were even lower (8% each) (14). In a United Kingdom registry including athletes aged 29 ± 11 years (range: 7–67 years), AN-SUD (42%) was the most common cause across all age levels, followed by idiopathic LVH or fibrosis (16%) and arrhythmogenic right ventricular cardiomyopathy (13%), HCM and coronary artery anomalies accounting for only 6 and 5%, respectively (55).
Sports Practice and Borderline Indications for an ICD Implantation in Athletes
Implantable cardioverter defibrillator (ICD) indications in athletes should not be different from those in the general population (57, 58). Also, the desire of the athlete to continue sports competition should not represent the primary indication for ICD implantations (58–60), an option that may seem particularly appealing in some patients with cardiomyopathies and channelopathies in whom exertion may increase the risk of arrhythmias. As an example, in patients with asymptomatic long QT syndrome (LQTS) without a prolonged QTc interval (genotype-positive/phenotype-negative), an ICD should only be considered if clinically indicated, namely, if the patient develops symptoms such as palpitations or syncope despite treatment with beta-blockers (57).
In these patients with LQTS, sports participation can be considered, depending on the type and setting of sports, type of genetic mutation, and symptoms (60). Although recent 2020 European guidelines (60) still restrict all the phenotype-positive athletes with LQTS from competitive sports, there is data to support return-to-play approval when patients are optimally treated and have preventive measures and annual follow-up appropriately implemented (61). This study included 494 athletes with LQTS who were given return-to-play approval by a single genetic cardiologist, 16% of whom were symptomatic before diagnosis, and 12% of whom had an ICD. Over a combined follow-up of 2 years, there was no LQTS-sports associated mortality and only 6% had one or more nonlethal LQTS-associated cardiac events.
Recommendations regarding sports participation in patients with channelopathies and arrhythmogenic cardiomyopathies may differ depending on geography. For instance, the 2015 American Heart Association/American College of Cardiology (AHA/ACC) guidelines allowed competitive sports participation in patients with symptomatic or electrocardiographic evident channelopathies (except for swimming in previously symptomatic LQTS1 (KCNQ1) mutation carriers), as long as appropriate precautionary measures (e.g., available AED) and disease-specific treatments are in place, and the athlete has been asymptomatic on treatment for at least 3 months (Class IIb, Level of evidence C) (62). On the other hand, European groups have been stricter regarding allowance for high-intensity sports practice in patients with the potentially arrhythmogenic disease. This is reflected in a recent EHRA position paper, in which sports participation is only recommended provided lower risk factors are guaranteed (Table 3) (63). As an example, patients with arrhythmogenic cardiomyopathy and gene-mutation carriers should avoid competitive sports and high-intensity leisure physical activity as it may worsen ventricular function, trigger life-threatening ventricular arrhythmias and promote disease progression (64, 65). However, in young patients with mild disease, low-to-moderate exercise does not seem to be entirely detrimental and should not be deprived of the health benefits of such activity (65). In addition, in situations where there is an agreement to participate in all competitive sports, these more lenient recommendations are frequently accompanied by an exception including those in whom occurrence of syncope may be associated with serious harm or death (e.g., driving, climbing, and diving) (63).
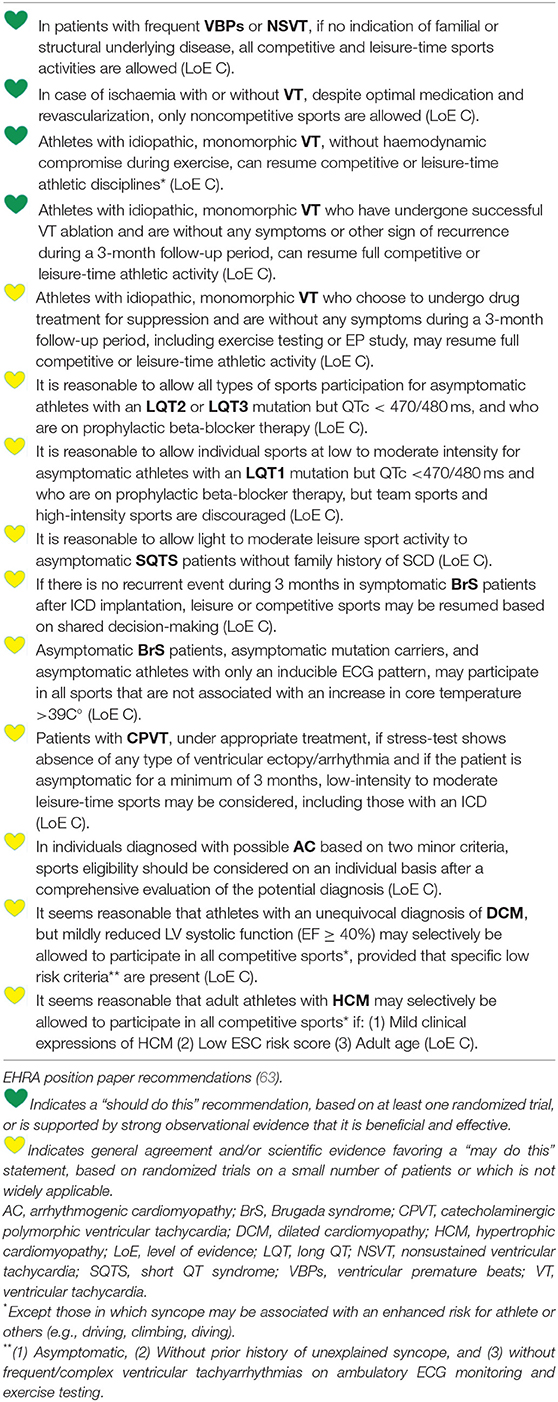
Table 3. Disease-specific recommendation for sports practice in patients with potentially arrhythmogenic conditions.
Efficacy and Safety of ICD in Athletes
Despite safety and efficacy concerns, many patients with ICDs continue regular sports practice, and some participate in competitions. The ICD Sports Safety Registry eased some of these concerns in competitive athletes (66). The investigators enrolled 440 athletes (10–60 years old) who were already engaged in organized competitive sports despite having an ICD. The most frequent diagnoses in this registry were LQTS (20%), HCM (17%), and arrhythmogenic right ventricular cardiomyopathy (13%). A 4-year follow-up study has shown that there were no cases of physical injury or failure to terminate arrhythmia despite participation in vigorous competitive sports (36). Heidbuchel et al. published a comparative analysis of these patients to 80 other patients with ICD who were participating in recreational moderate-to-high intensity sports, included in a parallel registry (67). They found similar safety and efficacy outcomes, as well as comparable freedom from 5- and 10-year probable or definite lead malfunction of 97 and 93%, respectively. On the contrary, in addition to the psychological benefits for an athlete, there may also be the potential cardiovascular benefit of continuing sports. A meta-analysis has also shown that in patients with heart failure and an ICD (mean age 54–66 years old, the majority with a history of myocardial infarction), exercise training was associated with significant improvement in cardiorespiratory fitness (68).
Although the agreement for sports participation should first be tailored to underlying disease of each patient (Table 3) (63), there are also general recommendations for patients who have an ICD. The AHA/ACC stated in 2015 that it is reasonable that patients with an ICD participate in sports with low dynamic and static components (e.g., golf, yoga, bowling) (69), as long as they are free from arrhythmic events requiring device therapy for 3 months (Class IIa, Level of evidence C) (58). In addition, participation in sports with higher intensity may be considered, taking into account the likelihood of appropriate or inappropriate shocks and device-related trauma (Class IIb, Level of evidence C) (58). In the European guidelines, shared decision-making relating to the continuation of intensive or competitive sports participation is recommended, taking into account the underlying disease, the psychological impact of shocks, the potential risk for third parties, and the fact that intensive sport will trigger more appropriate and inappropriate shocks (Class IIa, Level of evidence C) (63, 70). Empowerment of athletes with disorders with potential arrhythmic risk might have advantages such as allowing a more transparent and doctor–patient relationship, avoiding “doctor shopping” and acquiring more knowledge in “gray zone areas” (i.e., exercise in recipients of ICD) (71).
Participation in sports that involve collision (e.g., boxing or rugby) is not recommended because of the risk of damaging the device components, risk of hematoma formation, and subsequent pocket infection (70). For other team sports with some degree of physical contact (e.g., football, basketball, and baseball), a protective shield is recommended, although its effectiveness has never been proven (70). In addition, there are country-specific rules, such as in Italy where competitive sports eligibility for recipients of ICD can be granted in the following situations: (1) asymptomatic subjects; (2) no heart disease in which sport is contraindicated; (3) sports at low-moderate intensity; (4) sports without traumatic risk or with intrinsic risk; (5) sports in which the arm ipsilateral to the device is not repeatedly used; (6) at least 3 months after the last device intervention (72).
Type and Implantation Technique of ICDs in Athletes
The choice of the type of ICD should primarily be based on the underlying disease and potential for arrhythmia. In general, a subcutaneous ICD should be considered in patients who pass screening test (i.e., large enough QRS and small T-waves) and have an indication for ICD when pacing therapy for bradycardia and cardiac resynchronization is not needed, or in whom sustained monomorphic ventricular tachycardia requiring antitachycardia pacing is not anticipated (Class IIa, Level of Evidence C) (57). There is no specific evidence supporting either transvenous or subcutaneous ICDs in athletes. In a propensity-matched case-control study of patients aged 35–40 years, the majority (60%) having a diagnosis of HCM and a mean ejection fraction of 58%, subcutaneous ICD was associated with a 70% relative risk reduction of device-related complications and inappropriate shocks, mainly because of higher rates of lead failures in the transvenous group (73). Despite a lower risk of complications at a mean of 31 months follow-up, subcutaneous ICDs were more expensive, even when accounting for the lower complication-related costs (73). Further comparisons of the safety and efficiency of both systems must be derived from other studies of populations (74, 75), such as the one studied by Knops et al. in the only randomized conrolled trial (RCT) published on this subject so far (the PRAETORIAN trial) (76). These were patients with a median age of 63 years, 69% with ischemic cardiomyopathy, and a median left ventricle ejection fraction of 30%, who indicated ICD but no indication for pacing (76). At a median follow-up of 49 months, subcutaneous ICD was noninferior to the transvenous ICD in terms of inappropriate shocks and device-related complications, as fewer lead-related complications were counterbalanced by more frequent pocket hematomas with the subcutaneous ICD (76). A meta-analysis of case–control studies derived similar results, and the reasons for inappropriate shocks differed between both groups: in the subcutaneous ICD, they were primarily due to oversensing (T-wave or noise), whereas in the transvenous ICD they were mostly due to supraventricular tachycardias (77). One can therefore argue that in athletes who do not need an antibradycardic device, a subcutaneous ICD should be preferred. Finally, the ATLAS S-ICD trial is an ongoing RCT (NCT02881255, estimated completion date—February 2022) that aims to study the benefit and risks of Avoiding Transvenous Leads in Appropriate Subjects who have either inherited arrhythmia syndrome, prior device removal for infection, need for hemodialysis, prior heart valve surgery or chronic obstructive pulmonary disease (78).
Some technical aspects should be considered during the implantation of an ICD in an athlete: namely (1) right-side approach in the case of left arm dominance; (2) submuscular placement of generator; and (3) axillary or cephalic venous access to prevent a subclavian crush (63, 70). In addition, device programming should contemplate adequate rate response pacing, higher detection zones, longer arrhythmia detection intervals, and proactive exclusion of myopotentials interference to prevent inappropriate shocks (63, 70). In a subanalysis of the ICD Sports Registry, detection rates > 200 bpm and detection intervals longer than nominal were associated with decreased risk of total and inappropriate shocks during competition or practice, and higher shock-free survival, respectively (79). To avoid inappropriate shocks during sports activity, a Holter ECG monitoring and exercise testing can be performed to evaluate maximal heart rate during effort and set a threshold for shock delivery at least 20 bpm above the maximal sinus rate observed (72). Finally, treating physicians should have a lower threshold for referring patients/athletes with ICD for ablation of atrial and ventricular arrhythmias that may be the cause of appropriate and inappropriate therapies (63).
Conclusion
Sudden cardiac arrest in young athletes is a rare event, although accurate registries are needed to allow more accurate recording of SCA events to facilitate appropriate public health interventions. While some athletes with arrhythmic conditions may be allowed to continue sports practice, particularly in absence of structural heart disease or a channelopathy, some others should be disqualified from sports competition. In those who receive an ICD, special device and implantation choices may apply, and shared decision-making is recommended, taking into account the underlying disease, the psychological impact of shocks, and the type of sports. Nevertheless, athletes with ICDs may be excluded from competitions, depending upon country-specific and competition rules.
Cardiopulmonary resuscitation maneuvers are effective in preventing SCD and are responsible for an 8-times higher survival rates in sports-related SCA, compared with SCA that are not sports related. Initiatives to increase bystander delivery of CPR should be promoted by sports institutions and public health institutions, such as coordinated CPR training starting from school years, as part of the “Kids Save Lives” campaign. Although the distribution of AED in all sports clubs/venues might not be cost effective, further research and modeling into more cost-effective strategies are required but could include a quick and effective app-based mapping and location too to identify the nearest public access AED might help to save lives if an athlete or spectator collapses and requires resuscitation.
Author Contributions
MK, FR, RP, CC, and MC conceived the idea for the work. MC and MK drafted the manuscript. RP, FR, CC, FD'A, and AC reviewed the manuscript and provided critical edits. All the authors approved the final manuscript and agreed to be accountable for the content of this study.
Conflict of Interest
The authors declare that the research was conducted in the absence of any commercial or financial relationships that could be construed as a potential conflict of interest.
Publisher's Note
All claims expressed in this article are solely those of the authors and do not necessarily represent those of their affiliated organizations, or those of the publisher, the editors and the reviewers. Any product that may be evaluated in this article, or claim that may be made by its manufacturer, is not guaranteed or endorsed by the publisher.
References
1. Gräsner JT, Herlitz J, Tjelmeland IBM, Wnent J, Masterson S, Lilja G et al. European Resuscitation Council Guidelines 2021: epidemiology of cardiac arrest in Europe. Resuscitation. (2021) 161:61–79. doi: 10.1016/j.resuscitation.2021.02.007
2. Vellano K, Crouch A, Rajdev M, Mcnally B. Cardiac Arrest Registry to Enhance Survival (CARES) Report on the Public Health Burden of Out-of-Hospital Cardiac Arrest. Online Inst Med. (2015).
3. D'Ascenzi F, Valentini F, Pistoresi S, Frascaro F, Piu P, Cavigli L et al. Causes of sudden cardiac death in young athletes and non-athletes: systematic review and meta-analysis: Sudden cardiac death in the young. Trends Cardiovasc Med. (2021) 19:460–6. doi: 10.1016/j.tcm.2021.06.001
4. Gräsner JT, Wnent J, Herlitz J, Perkins GD, Lefering R, Tjelmeland I et al. Survival after out-of-hospital cardiac arrest in Europe - Results of the EuReCa TWO study. Resuscitation. (2020) 148:218–26. doi: 10.1016/j.resuscitation.2019.12.042
5. Harmon KG, Drezner JA, Wilson MG, Sharma S. Incidence of sudden cardiac death in athletes: a state-of-the-art review. Br J Sports Med. (2014) 48:1185–92. doi: 10.1136/bjsports-2014-093872
6. Corrado D, Basso C, Pavei A, Michieli P, Schiavon M, Thiene G. Trends in sudden cardiovascular death in young competitive athletes. JAMA. (2006) 296:1593–601. doi: 10.1001/jama.296.13.1593
7. Maron BJ, Doerer JJ, Haas TS, Tierney DM, Mueller FO. Sudden deaths in young competitive athletes analysis of 1866 deaths in the united states, 1980-2006. Circulation. (2009) 119:1085–92. doi: 10.1161/CIRCULATIONAHA.108.804617
8. Holst AG, Winkel BG, Theilade J, Kristensen IB, Thomsen JL, Ottesen GL et al. Incidence and etiology of sports-related sudden cardiac death in Denmark - Implications for preparticipation screening. Hear Rhythm. (2010) 7:1365–71. doi: 10.1016/j.hrthm.2010.05.021
9. Marijon E, Tafflet M, Celermajer DS, Dumas F, Perier MC, Mustafic H et al. Sports-related sudden death in the general population. Circulation. (2011) 124:672–81. doi: 10.1161/CIRCULATIONAHA.110.008979
10. Maron BJ, Haas TS, Ahluwalia A, Rutten-Ramos SC. Incidence of cardiovascular sudden deaths in Minnesota high school athletes. Hear Rhythm. (2013) 10:374–7. doi: 10.1016/j.hrthm.2012.11.024
11. Risgaard B, Winkel B, Jabbari R, Glinge C, Ingemann-hansen O, Thomsen J, et al. Sports related sudden cardiac death in a competitive and non-competitive athlete population aged 12-49 years: data from an unselected nationwide study in Denmark. Hear Rhythm. (2014) 11:1673–81. doi: 10.1016/j.hrthm.2014.05.026
12. Maron BJ, Haas TS, Murphy CJ, Ahluwalia A, Rutten-Ramos S. Incidence and causes of sudden death in U. S college athletes. J Am Coll Cardiol. (2014) 63:1636–43. doi: 10.1016/j.jacc.2014.01.041
13. Toresdahl BG, Rao AL, Harmon KG, Drezner JA. Incidence of sudden cardiac arrest in high school student athletes on school campus. Hear Rhythm. (2014) 11:1190–4. doi: 10.1016/j.hrthm.2014.04.017
14. Harmon KG, Asif IM, Maleszewski JJ, Owens DS, Prutkin JM, Salerno JC et al. Incidence, cause, and comparative frequency of sudden cardiac death in national collegiate athletic association athletes a decade in review. Circulation. (2015) 132:10–9. doi: 10.1161/CIRCULATIONAHA.115.015431
15. Maron BJ, Haas TS, Ahluwalia A, Murphy CJ, Garberich RF. Demographics and epidemiology of sudden deaths in young competitive athletes : from the United States National Registry. Am J Med. (2016) 129:1170–7. doi: 10.1016/j.amjmed.2016.02.031
16. Harmon KG, Asif IM, Maleszewski JJ, Owens DS, Prutkin JM, Salerno JC, et al. Incidence and etiology of sudden cardiac arrest and death in high school athletes in the United States. Mayo Clin Proc. (2016) 91:1493–502. doi: 10.1016/j.mayocp.2016.07.021
17. Gräni C, Chappex N, Fracasso T, Vital C, Kellerhals C, Schmied C et al. Sports-related sudden cardiac death in Switzerland classified by static and dynamic components of exercise. Eur J Prev Cardiol. (2016) 23:1228–36. doi: 10.1177/2047487316632967
18. Bohm P, Scharhag J, Meyer T. Data from a nationwide registry on sports-related sudden cardiac deaths in Germany. Eur J Prev Cardiol. (2016) 23:649–56. doi: 10.1177/2047487315594087
19. Landry CH, Allan KS, Connelly KA, Cunningham K, Morrison LJ, Dorian P. Sudden cardiac arrest during participation in competitive sports. N Engl J Med. (2017) 377:1943–53. doi: 10.1056/NEJMoa1615710
20. Asatryan B, Vital C, Kellerhals C, Medeiros-Domingo A, Grani C, Trachsel LD et al. Sports-related sudden cardiac deaths in the young population of Switzerland. PLoS ONE. (2017) 12:1–13. doi: 10.1371/journal.pone.0174434
21. Dennis M, Elder A, Semsarian C, Orchard J, Brouwer I, Puranik R, et al. 10-year review of sudden death during sporting activities. Hear Rhythm. (2018) 15:1477–83. doi: 10.1016/j.hrthm.2018.04.019
22. Bohm P, Scharhag J, Egger F, Tischer KH, Niederseer D, Schmied C et al. Sports-related sudden cardiac arrest in Germany. Can J Cardiol. (2020) 37:105–12. doi: 10.1016/j.cjca.2020.03.021
23. Peterson DF, Kucera K, Thomas LC, Maleszewski J, Siebert D, Lopez-Anderson M, et al. Aetiology and incidence of sudden cardiac arrest and death in young competitive athletes in the USA: A 4-year prospective study. Br J Sports Med. (2020) 55:1196–203. doi: 10.1136/bjsports-2020-102666
24. Sollazzo F, Palmieri V, Gervasi SF, Cuccaro F, Modica G, Narducci ML, et al. Sudden cardiac death in athletes in italy during 2019: Internet-based epidemiological research. Med. (2021) 57:1–9. doi: 10.3390/medicina57010061
25. Schmied C, Drezner J, Kramer E, Dvorak J. Cardiac events in football and strategies for first-responder treatment on the field. Br J Sports Med. (2013) 47:1175–8. doi: 10.1136/bjsports-2012-091918
26. Kiyohara K, Nishiyama C, Kiguchi T, Nishiuchi T, Hayashi Y, Iwami T et al. Exercise-related out-of-hospital cardiac arrest among the general population in the era of public-access defibrillation: a populationbased observation in Japan. J Am Heart Assoc. (2017) 6:1–10. doi: 10.1161/JAHA.117.005786
27. Khanji MY, Chahal CAA, Ricci F, Akhter MW, Patel RS. Cardiopulmonary resuscitation training to improve out-of-hospital cardiac arrest survival : addressing potential health inequalities. Eur J Prev Cardiol. (2021) 44:0–2. doi: 10.1093/eurjpc/zwab214
28. Ryan P, Twomey G, Falvey É. Assessment of Layperson Knowledge of AED use in Sports Clubs. Ir Med J. (2021) 114:405.
29. Fortington LV, West L, Morgan D, Finch CF. Implementing automated external defibrillators into community sports clubs/facilities: a cross-sectional survey of community club member preparedness for medical emergencies. BMJ Open Sport Exerc Med. (2019) 5:1–8. doi: 10.1136/bmjsem-2019-000536
30. Semeraro F, Greif R, Böttiger BW, Burkart R, Cimpoesu D, Georgiou M et al. European Resuscitation Council Guidelines 2021: Systems saving lives. Resuscitation. (2021) 161:80–97. doi: 10.1016/j.resuscitation.2021.02.008
31. Cave DM, Aufderheide TP, Beeson J, Ellison A, Gregory A, Hazinski MF et al. Importance and implementation of training in cardiopulmonary resuscitation and automated external defibrillation in schools: a Science Advisory from the American Heart Association. Circulation. (2011) 123:691–706. doi: 10.1161/CIR.0b013e31820b5328
32. Hansen CM, Zinckernagel L, Ersbøll AK, Tjørnhøj-Thomsen T, Wissenberg M, et al. Cardiopulmonary resuscitation training in schools following 8 years of mandating legislation in denmark: a nationwide survey. J Am Heart Assoc. (2017) 6:e004128. doi: 10.1161/JAHA.116.004128
33. Wissenberg M, Lippert FK, Folke F, Weeke P, Hansen CM, Christensen EF et al. Association of national initiatives to improve cardiac arrest management with rates of bystander intervention and patient survival after out-of-hospital cardiac arrest. JAMA - J Am Med Assoc. (2013) 310:1377–84. doi: 10.1001/jama.2013.278483
34. Semeraro F, Wingen S, Schroeder DC, Ecker H, Scapigliati A, Ristagno G, et al. KIDS SAVE LIVES — Three years of implementation in Europe. Resuscitation. (2018) 131:e9–11. doi: 10.1016/j.resuscitation.2018.08.008
35. Drezner JA, Toresdahl BG, Rao AL, Huszti E, Harmon KG. Outcomes from sudden cardiac arrest in US high schools: a 2-year prospective study from the national registry for AED use in sports. Br J Sports Med. (2013) 47:1179–83. doi: 10.1136/bjsports-2013-092786
36. Lampert R, Olshansky B, Heidbuchel H, Lawless C, Saarel E, Ackerman M, et al. Safety of sports for athletes with implantable cardioverter-defibrillators -long-term results of a prospective multinational registry. Circulation. (2017) 135:2310–2. doi: 10.1161/CIRCULATIONAHA.117.027828
37. Corrado D, Pelliccia A, Basso C, Zorzi A. Screening professional athletes for cardiovascular diseases at risk of cardiac arrest. Eur Heart J. (2021). doi: 10.1093/eurheartj/ehab440. [Epub ahead of print].
38. Mitani Y, Ohta K, Ichida F, Nii M, Arakaki Y, Ushinohama H, et al. Circumstances and outcomes of out-of-hospital cardiac arrest in elementary and middle school students in the era of public-access defibrillation: Implications for emergency preparedness in schools. Circ J. (2014) 78:701–7. doi: 10.1253/circj.CJ-13-1162
39. Smith CM, Colquhoun MC. Out-of-hospital cardiac arrest in schools: a systematic review. Resuscitation. (2015) 96:296–302. doi: 10.1016/j.resuscitation.2015.08.021
40. Caffrey S, Willoughby PJ, Pepe PE, Becker L. Public use of automated external defibrillators. N Engl J Med. (2002) 347:1242–7. doi: 10.1056/NEJMoa020932
41. Page RL, Joglar JA, Kowal RC, Zagrodzky JD, Nelson LL, Ramaswamy K, et al. Use of automated external defibrillators by a U. S Airline. N Engl J Med. (2000) 343:1210–6. doi: 10.1056/NEJM200010263431702
42. Pollack R, Siobhan PB, Rae T, Aufderheide T, Barbic D, Buick JE, et al. Impact of bystander automated external defibrillator use on survival and functional outcomes in shockable observed public cardiac arrests. Circulation. (2018) 137:2104–13. doi: 10.1161/CIRCULATIONAHA.117.030700
43. O'Connor S, Whyte E, Fortington L. Are irish gaelic athletic association clubs prepared to use an AED following a sudden cardiac arrest? a cross-sectional survey. Phys Sportsmed. (2020) 48:320–6. doi: 10.1080/00913847.2019.1704666
44. Cronin O, Jordan J, Quigley F, Molloy MG. Prepared for sudden cardiac arrest? A cross-sectional study of automated external defibrillators in amateur sport. Br J Sports Med. (2013) 47:1171–4. doi: 10.1136/bjsports-2013-092919
45. Schierbeck S, Hollenberg J, Nord A, Svensson L, Nordberg P, Ringh M, et al. Automated external defibrillators delivered by drones to patients with suspected out-of-hospital cardiac arrest. Eur Heart J. (2021). doi: 10.1093/eurheartj/ehab724.0656. [Epub ahead of print].
46. Pell JP, Walker A, Cobbe SM. Cost-effectiveness of automated external defibrillators in public places: con. Curr Opin Cardiol. (2007) 22:5–10. doi: 10.1097/HCO.0b013e3280118fec
47. Hallstrom AP, Ornato JP, Weisfeldt M, Travers A, Christenson J, McBurnie MA et al. Public-access defibrillation and survival after out-of-hospital cardiac arrest. N Engl J Med. (2004) 351:637–46. doi: 10.1056/NEJMoa040566
48. Nichol G, Huszti E, Birnbaum A, Mahoney B, Weisfeldt M, Travers A, et al. Cost-Effectiveness of Lay Responder Defibrillation for Out-of-Hospital Cardiac Arrest. Ann Emerg Med. (2009) 54:226–235.e2. doi: 10.1016/j.annemergmed.2009.01.021
49. Sanna T, La Torre G, de Waure C, Scapigliati A, Ricciardi W, Dello Russo A, et al. Cardiopulmonary resuscitation alone vs. cardiopulmonary resuscitation plus automated external defibrillator use by non-healthcare professionals: a meta-analysis on 1583 cases of out-of-hospital cardiac arrest. Resuscitation. (2008) 76:226–32. doi: 10.1016/j.resuscitation.2007.08.001
50. Pechmajou L, Sharifzadehgan A, Bougouin W, Dumas F, Beganton F, Jost D et al. Does occurrence during sports affect sudden cardiac arrest survival? Resuscitation. (2019) 141:121–7. doi: 10.1016/j.resuscitation.2019.06.277
51. Zijlstra JA, Stieglis R, Riedijk F, Smeekes M, van der Worp WE, Koster RW. Local lay rescuers with AEDs, alerted by text messages, contribute to early defibrillation in a Dutch out-of-hospital cardiac arrest dispatch system. Resuscitation. (2014) 85:1444–9. doi: 10.1016/j.resuscitation.2014.07.020
52. Ringh M, Rosenqvist M, Hollenberg J, Jonsson M, Fredman D, Nordberg P, et al. Mobile-phone dispatch of laypersons for CPR in out-of-hospital cardiac arrest. N Engl J Med. (2015) 372:2316–25. doi: 10.1056/NEJMoa1406038
53. Berglund E, Claesson A, Nordberg P, Djärv T, Lundgren P, Folke F, et al. A smartphone application for dispatch of lay responders to out-of-hospital cardiac arrests. Resuscitation. (2018) 126:160–5. doi: 10.1016/j.resuscitation.2018.01.039
54. Andelius L, Malta Hansen C, Lippert FK, Karlsson L, Torp-Pedersen C, Kjær Ersbøll A et al. Smartphone activation of citizen responders to facilitate defibrillation in out-of-hospital cardiac arrest. J Am Coll Cardiol. (2020) 76:43–53. doi: 10.1016/j.jacc.2020.04.073
55. Finocchiaro G, Papadakis M, Robertus J, Dhutia H, Steriotis A, Tome M, et al. Etiology of sudden death in sports. J Am Coll Cardiol. (2016) 67:2108–2015. doi: 10.1016/j.jacc.2016.02.062
56. Corrado D, Basso C, Rizzoli G, Schiavon M, Thiene G. Does sports activity enhance the risk of sudden death in adolescents and young adults? J Am Coll Cardiol. (2003) 42:1959–63. doi: 10.1016/j.jacc.2003.03.002
57. Priori SG, Blomström-Lundqvist C, Mazzanti A, Blom N, Borggrefe M, Camm J, et al. 2015 ESC Guidelines for the management of patients with ventricular arrhythmias and the prevention of sudden cardiac death. Eur Heart J. (2015) 36:2793–867. doi: 10.1093/eurheartj/ehv316
58. Zipes DP, Link MS, Ackerman MJ, Kovacs RJ, Myerburg RJ, Estes NAM. Eligibility and disqualification recommendations for competitive athletes with cardiovascular abnormalities: task force 9: arrhythmias and conduction defects: a scientific statement from the American Heart Association and American College of Cardiology. Circulation. (2015) 132:e315–25. doi: 10.1161/CIR.0000000000000245
59. Maron BJ, Udelson JE, Bonow RO, Nishimura RA, Ackerman MJ, Estes NAM et al. AHA/ACC scientific statement eligibility and disqualification recommendations for competitive athletes with cardiovascular abnormalities: Task force 3: hypertrophic cardiomyopathy, arrhythmogenic right ventricular cardiomyopathy and other cardiomyopath. Circulation. (2015) 132:273–80. doi: 10.1161/CIR.0000000000000239
60. Pelliccia A, Sharma S, Gati S, Bäck M, Börjesson M, Caselli S, et al. 2020 ESC Guidelines on sports cardiology and exercise in patients with cardiovascular disease. Eur Heart J. (2021) 42:17–96. doi: 10.1093/eurheartj/ehaa605
61. Tobert KE, Bos JM, Garmany R, Ackerman MJ. Return-to-Play for Athletes With Long QT Syndrome or Genetic Heart Diseases Predisposing to Sudden Death. J Am Coll Cardiol. (2021) 78:594–604. doi: 10.1016/j.jacc.2021.04.026
62. Ackerman MJ, Zipes DP, Kovacs RJ, Maron BJ. Eligibility and disqualification recommendations for competitive athletes with cardiovascular abnormalities: task force 10: the cardiac channelopathies: a scientific statement from the American Heart Association and American College of Cardiology. Circulation. (2015) 132:e326–9. doi: 10.1161/CIR.0000000000000246
63. Heidbuchel H, Arbelo E, D'ascenzi F, Borjesson M, Boveda S, Castelletti S, et al. Recommendations for participation in leisure-time physical activity and competitive sports of patients with arrhythmias and potentially arrhythmogenic conditions Part 2: Ventricular arrhythmias, channelopathies, and implantable defibrillators Europace. (2021) 23:147–8. doi: 10.1093/europace/euaa106
64. De Innocentiis C, Ricci F, Khanji MY, Aung N, Tana C, Verrengia E, et al. Athlete's heart: diagnostic challenges and future perspectives. Sport Med. (2018) 48:2463–77. doi: 10.1007/s40279-018-0985-2
65. Zorzi A, Cipriani A, Bariani R, Pilichou K, Corrado D, Bauce B. Role of exercise as a modulating factor in arrhythmogenic cardiomyopathy. Curr Cardiol Rep. (2021) 23:17–23. doi: 10.1007/s11886-021-01489-0
66. Lampert R, Olshansky B, Heidbuchel H, Lawless C, Saarel E, Ackerman M, et al. Safety of sports for athletes with implantable cardioverter-defibrillators: results of a prospective, multinational registry. Circulation. (2013) 127:2021–30. doi: 10.1161/CIRCULATIONAHA.112.000447
67. Heidbuchel H, Willems R, Jordaens L, Olshansky B, Carre F, Lozano IF et al. Intensive recreational athletes in the prospective multinational ICD Sports Safety Registry: results from the European cohort. Eur J Prev Cardiol. (2019) 26:764–75. doi: 10.1177/2047487319834852
68. Pandey A, Parashar A, Moore C, Ngo C, Salahuddin U, Bhargava M, et al. Safety and efficacy of exercise training in patients with an implantable cardioverter-defibrillator: a meta-analysis. JACC Clin Electrophysiol. (2017) 3:117–26. doi: 10.1016/j.jacep.2016.06.008
69. Levine B, Baggish A, Kovacs R, Link M, Maron M. Eligibility and disqualification recommendations for competitive athletes with cardiovascular of sports : dynamic, static, and impact and American College of Cardiology. Circulation. (2015) 132:262–6. doi: 10.1161/CIR.0000000000000237
70. Glikson M, Nielsen JC, Kronborg MB, Michowitz Y, Auricchio A, Barbash IM, et al. 2021 ESC Guidelines on cardiac pacing and cardiac resynchronization therapy. Eur Heart J. (2021) 42:3427–520. doi: 10.1093/eurheartj/ehab364
71. Providencia R, Teixeira C, Segal OR, Ullstein A, Mueser K, Lambiase PD. Empowerment of athletes with cardiac disorders: a new paradigm. Europace. (2018) 20:1243–51. doi: 10.1093/europace/eux268
72. Delise P, Mos L, Sciarra L, Basso C, Biffi A, Cecchi F, et al. Italian Cardiological Guidelines (COCIS) for competitive sport eligibility in athletes with heart disease. J Cardiovasc Med. (2021) 22:874–91. doi: 10.2459/JCM.0000000000001186
73. Honarbakhsh S, Providencia R, Srinivasan N, Ahsan S, Lowe M, Rowland E, et al. A propensity matched case–control study comparing efficacy, safety and costs of the subcutaneous vs. transvenous implantable cardioverter defibrillator. Int J Cardiol. (2017) 228:280–5. doi: 10.1016/j.ijcard.2016.11.017
74. Pettit SJ, McLean A, Colquhoun I, Connelly D, McLeod K. Clinical experience of subcutaneous and transvenous implantable cardioverter defibrillators in children and teenagers. PACE - Pacing Clin Electrophysiol. (2013) 36:1532–8. doi: 10.1111/pace.12233
75. Brouwer TF, Knops RE, Kutyifa V, Barr C, Mondésert B, Boersma LVA, et al. Propensity score matched comparison of subcutaneous and transvenous implantable cardioverter-defibrillator therapy in the SIMPLE and EFFORTLESS studies. Europace. (2018) 20(FI2):f240–8. doi: 10.1093/europace/euy083
76. Knops RE, Olde Nordkamp LRA, Delnoy P-PHM, Boersma LVA, Kuschyk J, El-Chami MF et al. Subcutaneous or transvenous defibrillator therapy. N Engl J Med. (2020) 383:526–36. doi: 10.1056/NEJMoa1915932
77. Basu-Ray I, Liu J, Jia X, Gold M, Ellenbogen K, DiNicolantonio J, et al. Subcutaneous versus transvenous implantable defibrillator therapy: a meta-analysis of case-control studies. JACC Clin Electrophysiol. (2017) 3:1475–83. doi: 10.1016/j.jacep.2017.07.017
78. Mondésert B, Bashir J, Philippon F, Dubuc M, Amit G, Exner D, et al. Rationale and design of the randomized prospective ATLAS study: avoid transvenous leads in appropriate subjects. Am Heart J. (2019) 207:1–9. doi: 10.1016/j.ahj.2018.09.008
Keywords: cardiopulmonary resuscitation, automated electrical defibrillator, implantable cardioverter defibrillator, sudden cardiac death, cardiac arrest, out of hospital cardiac arrest, athlete, sports cardiology
Citation: Carrington M, Providência R, Chahal CAA, D'Ascenzi F, Cipriani A, Ricci F and Khanji MY (2022) Cardiopulmonary Resuscitation and Defibrillator Use in Sports. Front. Cardiovasc. Med. 9:819609. doi: 10.3389/fcvm.2022.819609
Received: 21 November 2021; Accepted: 04 January 2022;
Published: 15 February 2022.
Edited by:
Marina Cerrone, NYU Grossman School of Medicine, United StatesReviewed by:
Can Hasdemir, Ege University, TurkeySilvia Magnani, New York University, United States
Copyright © 2022 Carrington, Providência, Chahal, D'Ascenzi, Cipriani, Ricci and Khanji. This is an open-access article distributed under the terms of the Creative Commons Attribution License (CC BY). The use, distribution or reproduction in other forums is permitted, provided the original author(s) and the copyright owner(s) are credited and that the original publication in this journal is cited, in accordance with accepted academic practice. No use, distribution or reproduction is permitted which does not comply with these terms.
*Correspondence: Mohammed Y. Khanji, bS5raGFuamlAcW11bC5hYy51aw==