- 1International Collaboration on Repair Discoveries, University of British Columbia, Vancouver, BC, Canada
- 2Experimental Medicine Program, Faculty of Medicine, University of British Columbia (UBC), Vancouver, BC, Canada
- 3School of Sport, Exercise, and Rehabilitation Sciences, University of Birmingham, Birmingham, United Kingdom
- 4Centre for Trauma Science Research, University of Birmingham, Birmingham, United Kingdom
- 5Department of Kinesiology, Michigan State University, East Lansing, MI, United States
- 6Department of Cellular and Physiological Sciences, Faculty of Medicine, UBC, Vancouver, BC, Canada
- 7Department of Cardiology, Vancouver General and UBC Hospitals, Vancouver Coastal Health, Vancouver, BC, Canada
- 8Department of Urology, University Hospital Basel, University of Basel, Basel, Switzerland
- 9Division of Physical Medicine and Rehabilitation, Faculty of Medicine, UBC, Vancouver, BC, Canada
- 10GF Strong Rehabilitation Centre, Vancouver Coastal Health, Vancouver, BC, Canada
Individuals with cervical spinal cord injury (SCI) experience deleterious changes in cardiac structure and function. However, knowledge on when cardiac alterations occur and whether this is dependent upon neurological level of injury remains to be determined. Transthoracic echocardiography was used to assess left ventricular structure, function, and mechanics in 10 male individuals (median age 34 years, lower and upper quartiles 32–50) with cervical (n = 5, c-SCI) or thoracolumbar (n = 5, tl-SCI) motor-complete SCI at 3- and 6-months post-injury. Compared to the 3-month assessment, individuals with c-SCI displayed structural, functional, and mechanical changes during the 6-month assessment, including significant reductions in end diastolic volume [121 mL (104–139) vs. 101 mL (99–133), P = 0.043], stroke volume [75 mL (61–85) vs. 60 mL (58–80), P = 0.042], myocardial contractile velocity (S') [0.11 m/s (0.10–0.13) vs. 0.09 m/s (0.08–0.10), P = 0.043], and peak diastolic longitudinal strain rate [1.29°/s (1.23–1.34) vs. 1.07°/s (0.95–1.15), P = 0.043], and increased early diastolic filling over early myocardial relaxation velocity (E/E') ratio [5.64 (4.71–7.72) vs. 7.48 (6.42–8.42), P = 0.043]. These indices did not significantly change in individuals with tl-SCI between time points. Ejection fraction was different between individuals with c-SCI and tl-SCI at 3 [61% (57–63) vs. 54% (52–55), P < 0.01] and 6 months [58% (57–62) vs. 55% (52–56), P < 0.01], though values were considered normal. These results demonstrate that individuals with c-SCI exhibit significant reductions in cardiac function from 3 to 6 months post-injury, whereas individuals with tl-SCI do not, suggesting the need for early rehabilitation to minimize cardiac consequences in this specific population.
Introduction
Left ventricular (LV) atrophy and decreased volumes are well documented in individuals with chronic spinal cord injury (SCI) (1). Decreased functional outcomes can result from impaired supraspinal sympathetic control over the cardiovascular system, such that cardiac responses to stress or exercise are diminished in individuals with high-level SCI (i.e., injury above the 6th thoracic spinal cord level) compared with non-injured individuals (2). Compounded with the higher prevalence of physical inactivity in the SCI population (3), it is perhaps unsurprising that cardiovascular disease (CVD) is the leading cause of morbidity and mortality in this population (4). These aforementioned factors have more substantial effects for individuals with cervical SCI compared to thoracolumbar SCI due to the combination of impaired sympathetic control to the heart (5), lack of skeletal muscle pump (i.e., decrease in venous return) (6), and greater functional impairments limiting physical activity (7). These factors predispose individuals with cervical SCI to daily cardiovascular disturbances, consequentially resulting in outcomes such as autonomic dysreflexia, orthostatic hypotension, and pooling of blood below the level of injury (8). Ultimately, previous literature suggests that these events can lead to detrimental cardiac consequences (9–11).
Similar to SCI, microgravity exposure during spaceflight has been associated with an overall physiological decline, providing a potential model to better understand the alterations in these specific populations over time (12). While the time course of changes in the autonomic nervous system following spaceflight and SCI have been described (12), temporal echocardiographic changes shortly after SCI have not been followed longitudinally. This is surprising as other cardiac changes, such as an increased prevalence of arrhythmias, occur in the months following SCI (13). Furthermore, previous research has demonstrated reduced echocardiographic indices of LV systolic function in individuals with cervical SCI at the chronic stage of injury (>1 year) compared to non-injured individuals (1), potentially due to the disrupted sympathetic input to the heart originating from T1 to T5 (14). Recently, a cross-sectional study revealed associations between increased time since injury and reduced LV size and function; these alterations have been linked to consequences such as congestive heart failure and increased mortality (15). Ultimately, the cardiac consequences (1) of decreased cardiac reserve has important clinical implications as it can limit the ability of an individual to perform regular activities of daily living (16). Furthermore, characterizing the time frame of structural and functional cardiac alterations may provide further insight into the increased risk of CVD in those with SCI and aid in the timing of cardiac rehabilitation interventions.
No study to date has examined the longitudinal changes in cardiac function post-SCI in the sub-acute setting (i.e., within the first 6 months), stratifying for neurological level of injury (NLI). Thus, the aim of this present longitudinal case series was to investigate changes in LV structure, function, and mechanics, in individuals with cervical SCI and thoracolumbar SCI at 3- and 6-months post-injury using transthoracic echocardiography (TTE) (17).
Materials and Methods
Participants
Clinical protocols were approved by the University of British Columbia Clinical Research Ethics Board (H13-03072) and conducted in accordance with the second Helsinki Declaration. The participants provided written informed consent prior to data collection. The NLI and the severity of SCI were classified according to the International Standards for Neurological Classification of SCI (ISNCSCI) by a trained physician [i.e., providing an American Spinal Injury Association Impairment Scale (AIS) grade] (18). Participants were asked to report the frequency and duration of moderate (some physical effort) and heavy (maximum physical effort) intensity leisure time physical activity (LTPA) over the preceding 7 days using the validated LTPA questionnaire for individuals with SCI (LTPAQ-SCI) (19). These data provide an indication of the volume of weekly LTPA performed by participants. The validity of the LTPAQ-SCI is comparable to questionnaires used in the general population (19). Exclusion criteria for all participants included any history of CVD and any language or cognitive barrier that prevented the participant from following English instructions.
Echocardiography
TTE was performed on a commercially available ultrasound (Vivid 7/i; GE Medical, Horton, Norway) and stored for offline analysis using specialized computer software (EchoPAC; GE Healthcare, Horton, Norway) according to the recommendations of the American Society for Echocardiography (ASE) (20), by a single analyzer, blinded to time point and group.
The average of three cardiac cycles was used to determine LV structure and functional indices. Measures of LV structure at end-diastole and end-systole were reported from the parasternal long-axis views, and used to derive relative wall thickness (21). Volumetric measurements and systolic functions were derived from the apical four- and two-chamber views using the modified Simpson's biplane method. Cardiac output (Q) was calculated as the product of stroke volume (SV) and heart rate (HR) and systolic myocardial velocity (S') was derived from pulsed-wave Doppler. LV diastolic indices were calculated using early septal relaxation velocity (E'), early (E) and late (A) transmitral flow, and early-to-late transmitral filling velocity (E/A) ratio derived from pulsed-wave Doppler. The E/E' ratio was calculated to estimate LV filling pressure. Deceleration time and isovolumetric relaxation time were also determined on the spectral Doppler trace. LV mass index was calculated with the Devereux method and indexed to body surface area using the DuBois method (22, 23).
Indices of LV mechanics were derived from apical four-chamber and parasternal short-axis images at the level of the mitral valve (basal), papillary muscle (mid), and apex (apical). Images were analyzed using 2D speckle-tracking software in accordance with recommended guidelines (24). Raw speckle-tracking traces were imported into customized post-processing software (2D Strain Analysis Tool, Stuttgart, Germany), and data was interpolated into 600 points in systole and 600 points in diastole using a cubic spline algorithm. Peak strain and strain rate in systole and diastole were determined for each parasternal short-axis view (radial, circumferential) and the apical four-chamber view (longitudinal). Basal and apical peak rotation and rotation rate in systole and diastole were determined. Twist was determined as the maximum value obtained when subtracting the frame-by-frame basal rotation from the frame-by-frame apical rotation. Torsion, a measure of twist normalized to LV chamber size, was calculated by dividing peak twist by the LV end-diastolic length.
Statistical Analysis
Data are presented as raw values and percentages. Statistical analyses were performed using Statistical Package for Social Science software (SPSS Version 27, IBM, Chicago, IL, USA) with statistical significance set at P ≤ 0.05. Wilcoxon signed rank test were used to analyze outcomes between time points within groups. Group differences were analyzed using the Mann Whitney U-test. Results are presented as median with upper and lower quartiles (i.e., 25–75%). Graphical representations were made in Prism (Version 9.1.1, GraphPad Software, San Diego, CA), SPSS, and Adobe Illustrator (Version 25.2.3, Adobe Inc., San Jose, CA).
Results
Participant Characteristics
A total of 10 male participants (median age 34 years, 32–50) with motor-complete (AIS A/B) cervical SCI (n = 5) or thoracolumbar SCI (n = 5) were included. TTE was conducted 79 days (65–108) and 204 (191–218) post-injury. Demographics are highlighted in Table 1, with no significant differences observed between groups.
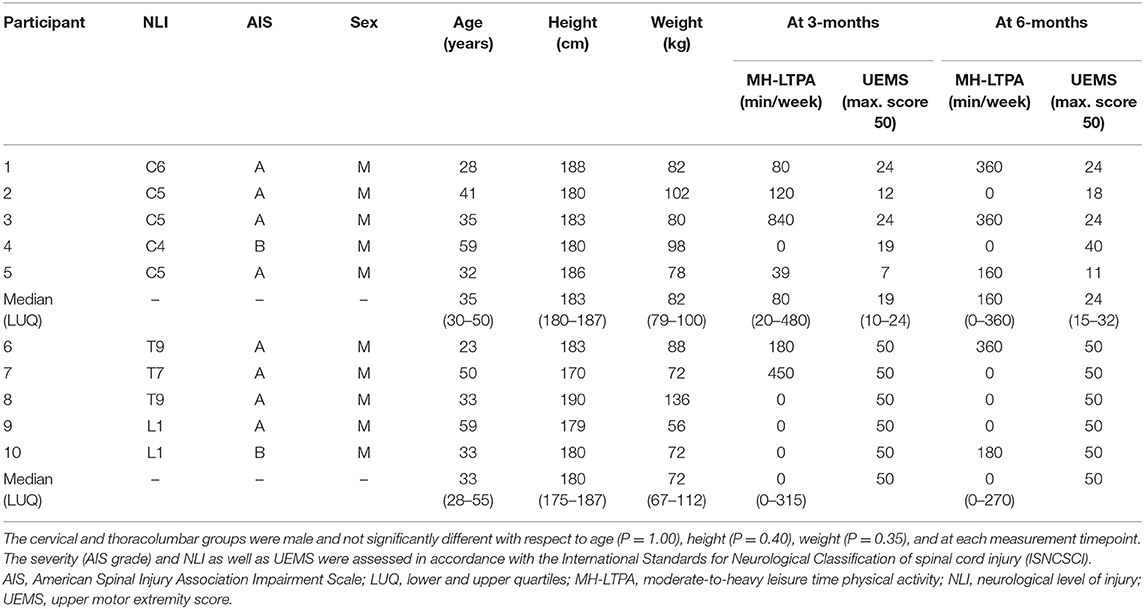
Table 1. Demographics, injury characteristics, and perceived physical activity level of participants.
Changes in Cardiac Structure, Function, and Mechanics
At 3- vs. 6-months post-injury, TTE revealed a significant decrease in LV internal diameter in diastole (LVIDd) [5.0 cm (4.6–5.3) vs. 4.9 (4.4–5.2), P = 0.043], end-diastolic volume (EDV) [120.83 mL (103.58–139.42) vs. 101.33 (99.17–133.18), P = 0.043], end-systolic volume (ESV) [45.67 mL (41.42–55.83) vs. 43.33 (40.17–52.75), P = 0.043], SV [75.17 mL (61.08–84.67) vs. 60.00 (58.00–80.33), P = 0.042], S' [0.11 m/s (0.10–0.13) vs. 0.09 (0.08–0.10), P = 0.043], and increased E/E' ratio [5.64 (4.71–7.72) vs. 7.48 (6.42–8.42), P = 0.043] for individuals with cervical SCI. For LV diastolic mechanics, longitudinal strain rate was lower in the cervical group at three compared to 6 months [1.29 degrees/s (1.23–1.34) vs. 1.07 (0.95–1.15), P = 0.043] (Figure 1). These indices did not significantly change in individuals with thoracolumbar SCI between time points. Further indices for LV dimensions, systolic function, and diastolic function are highlighted in Table 2.
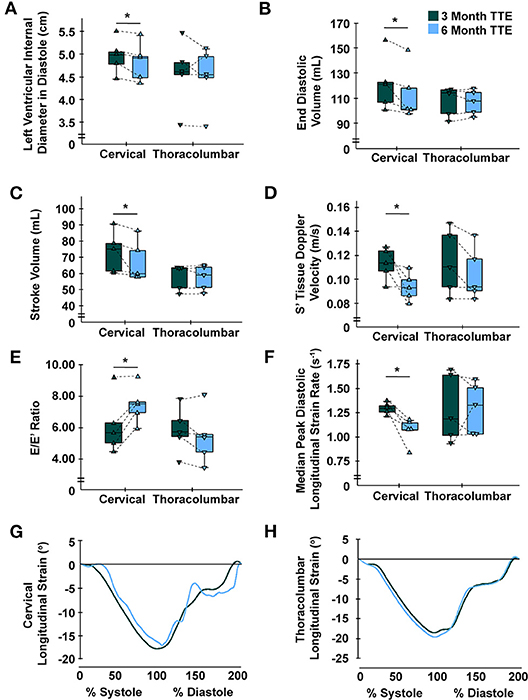
Figure 1. Time-course alterations following cervical spinal cord injury at 6-months for left ventricular indices. (A) Left ventricular internal diameter in diastole was significantly reduced in the cervical group at 6- vs. 3-months, yet not in the thoracolumbar group. (B) End diastolic volume was significantly reduced in the cervical group at 6- vs. 3-months, yet not in the thoracolumbar group. (C) Stroke volume was significantly reduced in the cervical group at 6- vs. 3-months, yet not in the thoracolumbar group. (D) Myocardial contractile velocity (S') was significantly reduced in the cervical group at 6- vs. 3-months, yet not in the thoracolumbar group (25). (E) Early diastolic filling over early myocardial relaxation velocity (E/E') ratio was significantly increased in the cervical group at 6- vs. 3-months, yet not in the thoracolumbar group. (F) Median peak diastolic longitudinal strain rate was significantly lower at 6- vs. 3-months in the cervical group, yet not in the thoracolumbar group. (G,H) Global longitudinal strain standardized to cardiac cycle length for all participants at the 3- and 6-month time points for the cervical (G) and thoracolumbar (H) SCI groups; no differences were observed within groups. C, cervical; L, lumbar; S, sacral; T, thoracic; TTE, transthoracic echocardiography. * Cervical 6-months different from cervical 3-months (P < 0.05). Data are displayed individually (i.e., each triangle represents one individual) and grouped (i.e., box-and-whisker plot).
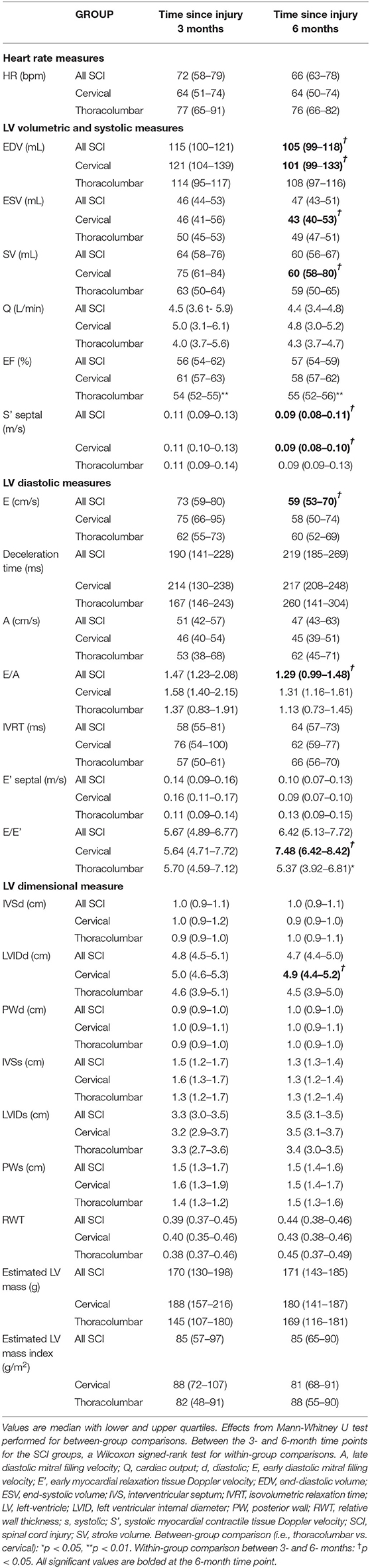
Table 2. Echocardiographic indices for LV structure and function between cervical and thoracolumbar SCI groups and within 3- and 6-month time points.
There were no differences between the cervical SCI and thoracolumbar SCI groups for echocardiographic measures at 3- or 6-month time points, except for EF (P < 0.01), which was still within the normal range of 52–72% for males as per ASE guidelines (20). LV mechanics parameters can be found in Table 3. For LV systolic mechanics, apical rotation was higher in the thoracolumbar group at 6-months compared to 3-months, whereas radial strain rate at the mid-level was lower.
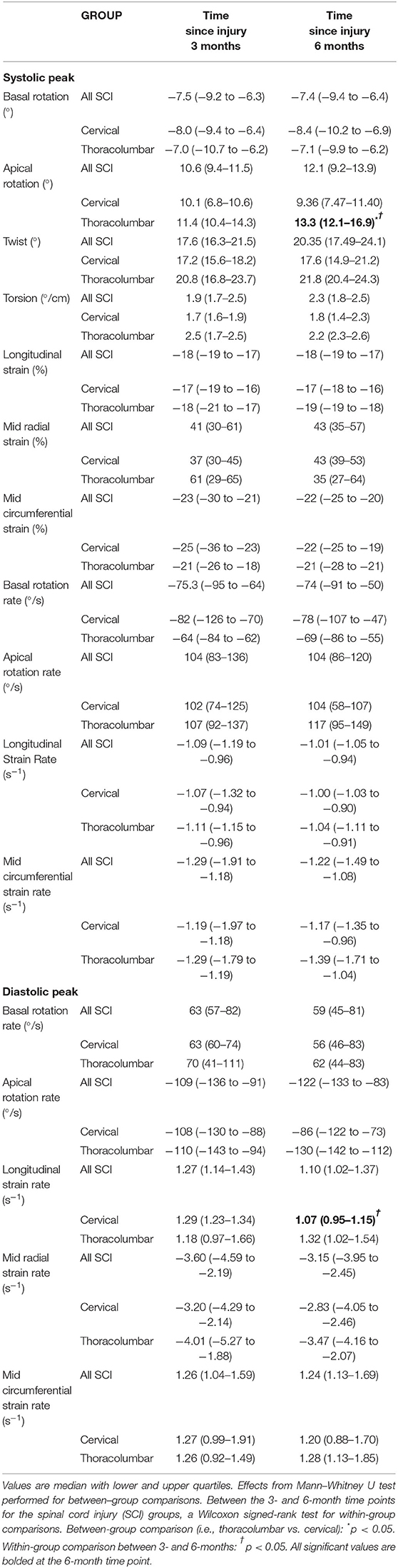
Table 3. Echocardiographic indices for LV mechanics between cervical and thoracolumbar SCI groups and within 3- and 6-month time points.
Discussion
To our knowledge, this is the first study to investigate the time-course of cardiac changes in humans with sub-acute SCI (i.e., serial echocardiography measurements performed within the first 6 months post injury). While a recent meta-analysis demonstrated that structural and functional changes are apparent for individuals with chronic SCI compared to non-injured individuals (1), these current findings suggest changes may occur during the sub-acute stage post cervical SCI. The novel aim of this study was to longitudinally track the same individuals in each group over a 3-month period in the sub-acute stage of injury. The reduced cardiac capacity for the cervical SCI group may limit the ability of these individuals to perform regular activities of daily living (16), consequently putting these individuals at a greater risk for developing chronic diseases (i.e., CVD) (26) and impacting aspects of health-related quality of life.
Reductions in LV structural and functional cardiac indices were found for individuals with cervical SCI between 3- and 6-months post-injury, whereas these changes were not apparent for individuals with thoracolumbar SCI. As the LTPA measures were highly variable but somewhat comparable amongst the cervical SCI and thoracolumbar SCI participants, the most likely explanation for the differences between groups can be attributed to the disrupted supraspinal regulation of sympathetic activity to the heart following cervical SCI (5). To support this, our group has previously published translational work suggesting the mechanism behind the reduction in cardiac function is underpinned by interrupted bulbospinal sympathetic control (27). Conversely, cardiac sympathetic control is preserved in those with thoracolumbar SCI. It has been demonstrated in individuals with chronic SCI that a higher NLI is likely to result in more devastating consequences for cardiac function (28). However, these longitudinal cardiac adaptations, assessed using echocardiography, have never previously been captured in the first 6 months following human SCI with the same individuals.
Despite reductions in LV structure and global function in the cervical SCI group, indices of LV systolic mechanics were similar over time. Perhaps this can be attributed to a mechanical compensation post-SCI to maintain systolic function in the cervical SCI group. The observation of preserved systolic mechanics and reduced SV has been previously reported (29), and is thought to be attributed to reduced afterload from the chronically hypotensive state of individuals with SCI, even with reductions in preload.
Leisure Time Physical Activity
Despite recommended exercise guidelines to improve cardiometabolic health following SCI (3), 40 and 50% of the SCI participants at 3- and 6-months post-injury, respectively, self-reported 0 min of moderate-to-heavy intensity LTPA per week. This is in agreement with previous literature revealing that 50% of community-dwelling individuals with SCI do not perform any LTPA (30). While the LTPAQ-SCI does not include activities of daily living in its assessment, literature suggests that activities of daily living are not associated with cardiovascular fitness in this population (31) and are therefore unlikely to have an impact on the cardiac indices measured in this study. During in-patient rehabilitation in Canada, participants have self-reported performing > 60 min of vigorous-intensity physical activity per day (31). However, the same study indicated that only 5 min per day was spent performing physical activity > 40% heart rate reserve. Therefore, self-reported minutes of moderate-to-vigorous-intensity physical activity do not reflect actual time spent performing activities at higher intensities as measured objectively via a heart rate monitor. Therefore, In-keeping with other results, it could be implied that individuals with SCI do not reach an effective cardiovascular training threshold to improve neurologic, cardiovascular, or musculoskeletal health during inpatient therapy (31). This suggests further work to optimize rehabilitation strategies is warranted.
Previous literature suggests the cardiovascular deconditioning following SCI can be improved with exercise training (2). However, given the reduced upper-extremity motor scores (range 11–40), individuals with cervical SCI can struggle to perform volitional upper-body exercise of a sufficient intensity or volume to offset CVD risk. These reduced upper-extremity motor scores may reflect an overestimation of reported LTPA, though this suggestion is outside the scope of our study. Thus, individuals with cervical SCI may require alternative forms of exercise to prevent cardiac decline. Pre-clinical rodent models have shown improvements in LVIDd, EDV, and SV following passive hind-limb exercise (32), suggesting passive lower-limb manipulation or stimulation (33) may improve cardiac function (34).
We contend that disrupted sympathetic control to the heart and blood vessels after cervical SCI (5) is primarily responsible for the accelerated cardiac decline in these participants. Physical activity may mitigate this to some degree (35), but the effectiveness of volitional upper-body exercise, especially in individuals with cervical SCI during the sub-acute phase requires further research. Additional work is required to promote exercise in humans with SCI in the sub-acute setting and investigate the efficacy of different exercise intensities and modalities to improve or maintain cardiac health.
Future Directions
Our novel findings, while preliminary and requiring verification with a larger cohort, reveal that significant cardiac changes occur in the sub-acute period following cervical SCI. It would be of interest to track these individuals into the chronic period of injury to observe whether these changes plateau or decline further. In addition to exercise, early pharmacological strategies may mitigate this cardiac decline, which potentially has implications for the risk of developing CVD. A recent pre-clinical study investigating the use of dobutamine in the acute setting to augment LV contractility, appears to preserve cardiac function in the chronic setting post-SCI (36). Furthermore, novel neuromodulation strategies, such as the application of epidural stimulation (37) to facilitate supraspinal control of the sympathetic nervous system could complement acute rehabilitation strategies. Although the cardiac indices measured in our participants are somewhat variable and within the normal range compared to clinical guidelines (20), the major strength of our study is the longitudinal design, which tracks the same participants during this novel sub-acute period.
Study Limitations
Although our groups are comparable for demographic characteristics, the progression of each type of injury can only be truly compared by measuring indices prior to injury. This is an implausible study design; therefore, pre-clinical models would help to complement these findings. Furthermore, while NLI and severity of SCI were reported using validated methods, autonomic completeness of the injury was not measured. However, associations between sensorimotor and sympathetic impairments have previously been observed in non-athletic individuals with SCI (38). Consequently, it is not possible to state with certainty that these findings are due to disrupted supraspinal cardiac control. MH-LTPA variations also emphasize the potential limitations of using self-report questionnaires. Participants were taken from a sample of inpatients at a single, local rehabilitation center who had access to transportation for testing appointments following discharge, therefore potentially subjecting the study to a degree of sampling bias. These individuals were not highly trained athletes or deconditioned before injury, therefore our sample represents individuals from an urban developed country that have sustained a SCI. Future research should incorporate validated, research-grade multi-sensor devices (39) to precisely quantify physical activity levels and to ascertain how the modification of certain physical activity dimensions might alter cardiac indices in the sub-acute setting. TTE recommendations to scan participants in the left-lateral supine position to avoid foreshortening (20) may present a limitation, as reductions to preload in an upright position (1) are common in individuals with SCI and should be considered for future studies. Finally, the role of vasoactive medications on these cardiac findings would be of interest in future studies as the optimal dose of these pharmaceutical strategies are still being investigated, particularly in the sub-acute period following injury (40).
Conclusion
Our data demonstrate a decline in several cardiac indices using TTE following cervical SCI, as early as 6-months post-injury. A lack of descending sympathetic control of the heart may be responsible for the rapid adaptive changes in cardiac indices. Worryingly, these changes occur over a relatively short time frame following cervical SCI. This highlights the need to investigate the effectiveness of different therapeutic strategies to mitigate the decline of cardiovascular function in this population during the sub-acute period.
Data Availability Statement
The raw data supporting the conclusions of this article will be made available by the authors, without undue reservation.
Ethics Statement
The studies involving human participants were reviewed and approved by University of British Columbia Clinical Research Ethics Board (H13-03072). The patients/participants provided their written informed consent to participate in this study.
Author Contributions
MW and AK had full access to all the data in the study and take responsibility for the integrity of the data and the accuracy of the data analysis. SB, TN, MW, KC, and AK contributed to conception and experimental design. SB acquired the data. SB, TN, and MW analyzed the data. SB, TN, KC, CW, TT, MW, and AK interpreted the data. SB and TN drafted the manuscript. SB, MW, TN, KC, CW, TT, and AK contributed to editing the final version of the manuscript. AK is the principle investigator of the project. TN and MW co-supervised the project. All authors contributed to the article and approved the submitted version.
Funding
This work was supported by a grant (Principal Investigator: AK) from the Craig H. Neilsen Foundation (Grant H13-03072), the Canadian Foundation of Innovation (CFI; 35869 - John R. Evans Leaders Fund), and the BC Knowledge Development Fund (BCKDF; 53869). SB was supported by The Robert H.N. Ho Scholarship, UBC President's Academic Excellence Award, and Peter McLardie Award (Sonography Canada). TN was supported by a Postdoctoral Research Trainee Award from the Michael Smith Foundation for Health Research (MSFHR) in partnership with the International Collaboration on Repair Discoveries, (Grant Number: 17767). KC was supported by a Craig H. Neilsen Foundation Postdoctoral Fellowship (Grant Number: 281863). MW was supported by a Postdoctoral Research Trainee Award from MSFHR in partnership with the Rick Hansen Foundation (Grant Number: 17110). AK holds the Endowed Chair in Rehabilitation Medicine.
Conflict of Interest
The authors declare that the research was conducted in the absence of any commercial or financial relationships that could be construed as a potential conflict of interest.
Publisher's Note
All claims expressed in this article are solely those of the authors and do not necessarily represent those of their affiliated organizations, or those of the publisher, the editors and the reviewers. Any product that may be evaluated in this article, or claim that may be made by its manufacturer, is not guaranteed or endorsed by the publisher.
Acknowledgments
The authors would like to thank all individuals with SCI for their commitment, time, and support, without which this study would not have been possible. We extend our gratitude to Dr. Eric Stöhr (Cardiff Metropolitan University) and Dr. Robert Shave (UBC Okanagan) for the use of the custom strain analysis software in this study. We acknowledge the support of Dr. Nathan Hitchman in assistance with data collection.
Supplementary Material
The Supplementary Material for this article can be found online at: https://www.frontiersin.org/articles/10.3389/fcvm.2022.881741/full#supplementary-material
Supplementary Material 1. STROBE statement checklist.
References
1. Williams AM, Gee CM, Voss C, West CR. Cardiac consequences of spinal cord injury: systematic review and meta-analysis. Heart. (2019) 105:217–25. doi: 10.1136/heartjnl-2018-313585
2. Grigorean VT, Sandu AM, Popescu M, Iacobini MA, Stoian R, Neascu C, et al. Cardiac dysfunctions following spinal cord injury. J Med Life. (2009) 2:133–45.
3. Martin Ginis KA, Van Der Scheer JW, Latimer-Cheung AE, Barrow A, Bourne C, Carruthers P, et al. Evidence-based scientific exercise guidelines for adults with spinal cord injury: an update and a new guideline. Spinal Cord. (2018) 56:308–21. doi: 10.1038/s41393-017-0017-3
4. Garshick E, Kelley A, Cohen SA, Garrison A, Tun CG, Gagnon D, et al. A prospective assessment of mortality in chronic spinal cord injury. Spinal Cord. (2005) 43:408–16. doi: 10.1038/sj.sc.3101729
5. Biering-Sørensen F, Biering-Sørensen T, Liu N, Malmqvist L, Wecht JM, Krassioukov A. Alterations in cardiac autonomic control in spinal cord injury. Auton Neurosci. (2018) 209:4–18. doi: 10.1016/j.autneu.2017.02.004
6. Popa C, Popa F, Grigorean VT, Onose G, Sandu AM, Popescu M, et al. Vascular dysfunctions following spinal cord injury. J Med Life. (2010) 3:275–85.
7. Sisto SA, Evans N. Activity and fitness in spinal cord injury: review and update. Curr Phys Med Rehabil Reports. (2014) 2:147–57. doi: 10.1007/s40141-014-0057-y
8. Phillips AA, Krassioukov AV. Contemporary cardiovascular concerns after spinal cord injury: mechanisms, maladaptations, and management. J Neurotrauma. (2015) 32:1927–42. doi: 10.1089/neu.2015.3903
9. West CR, Squair JW, McCracken L, Currie KD, Somvanshi R, Yuen V, et al. Cardiac consequences of autonomic dysreflexia in spinal cord injury. Hypertension. (2016) 68:1281–9. doi: 10.1161/HYPERTENSIONAHA.116.07919
10. Claydon VE, Steeves JD, Krassioukov A. Orthostatic hypotension following spinal cord injury: understanding clinical pathophysiology. Spinal Cord. (2006) 44:341–51. doi: 10.1038/sj.sc.3101855
11. de Groot PC, van Dijk A, Dijk E, Hopman MT. Preserved cardiac function after chronic spinal cord injury. Arch Phys Med Rehabil. (2006) 87:1195–200. doi: 10.1016/j.apmr.2006.05.023
12. Scott JM, Warburton DER, Williams D, Whelan S, Krassioukov A. Challenges, concerns and common problems: physiological consequences of spinal cord injury and microgravity. Spinal Cord. (2011) 49:4–16. doi: 10.1038/sc.2010.53
13. Balthazaar SJT, Sengeløv M, Bartholdy K, Malmqvist L, Ballegaard M, Hansen B, et al. Cardiac arrhythmias six months following traumatic spinal cord injury. J Spinal Cord Med. (2021). doi: 10.1080/10790268.2021.1950453. [Epub ahead of print].
14. Krassioukov A. Autonomic function following cervical spinal cord injury. Respir Physiol Neurobiol. (2009) 169:157–64. doi: 10.1016/j.resp.2009.08.003
15. Ely MR, Singh TK, Baggish AL, Taylor JA. Reductions in cardiac structure and function 24 months after spinal cord injury: a cross-sectional study. Arch Phys Med Rehabil. (2021) 102:1490–8. doi: 10.1016/j.apmr.2021.01.070
16. Strait JB, Lakatta EG. Aging-associated cardiovascular changes and their relationship to heart failure. Heart Fail Clin. (2012) 8:143–64. doi: 10.1016/j.hfc.2011.08.011
17. Balthazaar SJT. Cardiac Consequences and Effects of Exercise Interventions Following Spinal Cord Injury in Humans. Vancouver, BC: University of British Columbia (2021).
18. Kirshblum SC, Burns SP, Biering-Sorensen F, Donovan W, Graves DE, Jha A, et al. International standards for neurological classification of spinal cord injury (revised 2011). J Spinal Cord Med. (2011) 34:535–46. doi: 10.1179/204577211X13207446293695
19. Martin Ginis KA, Úbeda-Colomer J, Alrashidi AA, Nightingale TE, Au JS, Currie KD, et al. Construct validation of the leisure time physical activity questionnaire for people with SCI (LTPAQ-SCI). Spinal Cord. (2020) 59:311–8. doi: 10.1038/s41393-020-00562-9
20. Lang RM, Badano LP, Mor-Avi V, Afilalo J, Armstrong A, Ernande L, et al. Recommendations for cardiac chamber quantification by echocardiography in adults: an update from the american society of echocardiography and the European Association of Cardiovascular Imaging. J Am Soc Echocardiogr. (2015) 28:1–39.e14. doi: 10.1016/j.echo.2014.10.003
21. Ganau A, Devereux RB, Roman MJ, de Simone G, Pickering TG, Saba PS, et al. Patterns of left ventricular hypertrophy and geometric remodeling in essential hypertension. J Am Coll Cardiol. (1992) 19:1550–8. doi: 10.1016/0735-1097(92)90617-V
22. Devereux RB, Alonso DR, Lutas EM, Gottlieb GJ, Campo E, Sachs I, et al. Echocardiographic assessment of left ventricular hypertrophy: comparison to necropsy finding. Am J Cardiol. (1986) 57:450–8. doi: 10.1016/0002-9149(86)90771-X
23. Du Bois D, Du Bois E. A formula to estimate the approximate surface area if height and weight be known. 1916. Nutrition. (1989) 5:303–11.
24. Mor-Avi V, Lang R, Badano L, Belohlavek M, Cardim N, Derumeaux G, et al. Current and evolving echocardiographic techniques for the quantitative evaluation of cardiac mechanics: ASE/EAE consensus statement on methodology and indications endorsed by the japanese society of echocardiography. Eur J Echocardiogr. (2011) 24:277–313. doi: 10.1016/j.echo.2011.01.015
25. Caballero L, Kou S, Dulgheru R, Gonjilashvili N, Athanassopoulos GD, Barone D, et al. Echocardiographic reference ranges for normal cardiac Doppler data: results from the NORRE Study. Eur Hear J Cardiovasc Imaging. (2015) 16:1031–41. doi: 10.1093/ehjci/jev083
26. Sweis R, Biller J. Systemic complications of spinal cord injury. Curr Neurol Neurosci Reports. (2017) 17:8. doi: 10.1007/s11910-017-0715-4
27. Fossey MPM, Balthazaar SJT, Squair JW, Williams AM, Poormasjedi-Meibod MS, Nightingale TE, et al. Spinal cord injury impairs cardiac function due to impaired bulbospinal sympathetic control. Nat Commun. (2022) 13:1–16. doi: 10.1038/s41467-022-29066-1
28. Rodrigues D, Tran Y, Guest R, Middleton J, Craig A. Influence of neurological lesion level on heart rate variability and fatigue in adults with spinal cord injury. Spinal Cord. (2016) 54:292–7. doi: 10.1038/sc.2015.174
29. Currie KD, West CR, Krassioukov A V. Differences in left ventricular global function and mechanics in paralympic athletes with cervical and thoracic spinal cord injuries. Front Physiol. (2016) 7:110. doi: 10.3389/fphys.2016.00110
30. Ginis KAM, Latimer AE, Arbour-Nicitopoulos KP, Buchholz AC, Bray SR, Craven BC, et al. Leisure time physical activity in a population-based sample of people with spinal cord injury part I: demographic and injury-related correlates. Arch Phys Med Rehabil. (2010) 91:722–8. doi: 10.1016/j.apmr.2009.12.027
31. Zbogar D, Eng JJ, Noble JW, Miller WC, Krassioukov AV, Verrier MC. Cardiovascular stress during inpatient spinal cord injury rehabilitation. Arch Phys Med Rehabil. (2017) 98:2449–56. doi: 10.1016/j.apmr.2017.05.009
32. West CR, Crawford MA, Poormasjedi-Meibod M-S, Currie KD, Fallavollita A, Yuen V, et al. Passive hind-limb cycling improves cardiac function and reduces cardiovascular disease risk in experimental spinal cord injury. J Physiol. (2014) 592:1771–83. doi: 10.1113/jphysiol.2013.268367
33. Nash MS, Bilsker S, Marcillo AE, Isaac SM, Botelho LA, Klose KJ, et al. Reversal of adaptive left ventricular atrophy following electrically-stimulated exercise training in human tetraplegics. Paraplegia. (1991) 29:590–9. doi: 10.1038/sc.1991.87
34. Latimer AE, Ginis KAM, Craven BC, Hicks AL. The physical activity recall assessment for people with spinal cord injury: validity. Med Sci Sports Exerc. (2006) 38:208–16. doi: 10.1249/01.mss.0000183851.94261.d2
35. Davis GM, Shephard RJ, Leenen FH. Cardiac effects of short term arm crank training in paraplegics: echocardiographic evidence. Eur J Appl Physiol Occup Physiol. (1987) 56:90–6. doi: 10.1007/BF00696382
36. Williams AM, Manouchehri N, Erskine E, Tauh K, So K, Shortt K, et al. Cardio-centric hemodynamic management improves spinal cord oxygenation and mitigates hemorrhage in acute spinal cord injury. Nat Commun. (2020) 11:1–12. doi: 10.1038/s41467-020-18905-8
37. West CR, Phillips AA, Squair JW, Williams AM, Walter M, Lam T, et al. Association of epidural stimulation with cardiovascular function in an individual with spinal cord injury. JAMA Neurol. (2018) 75:630–2. doi: 10.1001/jamaneurol.2017.5055
38. Currie KD, West CR, Hubli M, Gee CM, Krassioukov AV. Peak heart rates and sympathetic function in tetraplegic nonathletes and athletes. Med Sci Sport Exerc. (2015) 47:1259–64. doi: 10.1249/MSS.0000000000000514
39. Nightingale TE, Rouse PC, Thompson D, Bilzon JLJ. Measurement of physical activity and energy expenditure in wheelchair users: methods, considerations and future directions. Sport Med Open. (2017) 3:10. doi: 10.1186/s40798-017-0077-0
Keywords: spinal cord injuries, echocardiography, ventricular function, time course, autonomic nervous system
Citation: Balthazaar SJT, Nightingale TE, Currie KD, West CR, Tsang TSM, Walter M and Krassioukov AV (2022) Temporal Changes of Cardiac Structure, Function, and Mechanics During Sub-acute Cervical and Thoracolumbar Spinal Cord Injury in Humans: A Case-Series. Front. Cardiovasc. Med. 9:881741. doi: 10.3389/fcvm.2022.881741
Received: 23 February 2022; Accepted: 24 May 2022;
Published: 15 June 2022.
Edited by:
Rajesh Katare, University of Otago, New ZealandReviewed by:
Joseph Brady Moore IV, University of Louisville, United StatesShaoping Hou, Drexel University, United States
Bharat Guthikonda, Louisiana State University Health Shreveport, United States
Copyright © 2022 Balthazaar, Nightingale, Currie, West, Tsang, Walter and Krassioukov. This is an open-access article distributed under the terms of the Creative Commons Attribution License (CC BY). The use, distribution or reproduction in other forums is permitted, provided the original author(s) and the copyright owner(s) are credited and that the original publication in this journal is cited, in accordance with accepted academic practice. No use, distribution or reproduction is permitted which does not comply with these terms.
*Correspondence: Andrei V. Krassioukov, a3Jhc3Npb3Vrb3ZAaWNvcmQub3Jn
†These authors have contributed equally to this work and share first authorship
‡These authors have contributed equally to this work and share senior authorship