Treatment of Hyperlactatemia in Acute Circulatory Failure Based on CO2-O2-Derived Indices: Study Protocol for a Prospective, Multicentric, Single, Blind, Randomized, Superiority Study (The LACTEL Study)
- 1Anaesthesiology and Critical Care Department, Dijon Bourgogne University Hospital, Dijon, France
- 2University of Burgundy Franche-Comté, LNC UMR1231, Dijon, France
- 3Department of Anesthesiology and Intensive Care Medicine, University Hospital of Besançon, Besançon, France
- 4EA3920, University of Franche-Comté, Besançon, France
Background: Hyperlactatemia is a biological marker of tissue hypoperfusion with well-known diagnostic, prognostic, and therapeutic implications in shock states. In daily clinical practice, it is difficult to find out the exact mechanism underlying hyperlactatemia. Central venous to arterial CO2 difference (pCO2 gap) is a better parameter of tissue hypoperfusion than the usual ones (clinical examination and mixed venous saturation). Furthermore, the ratio between the pCO2 gap and p(v–a)CO2/C(a–v)O2 may be a promising indicator of anaerobic metabolism, allowing for the identification of different causes of tissue hypoxia and hyperlactatemia. The main aim of the study is to demonstrate that initial hemodynamic resuscitation based on an algorithm integrating the pCO2 gap and p(v–a)CO2/C(a–v)O2 ratio vs. usual clinical practice in acute circulatory failure improves lactate clearance.
Methods: LACTEL is a randomized, prospective, multicentric, controlled study. It compares the treatment of hyperlactatemia using an algorithm based on the pCO2 gap and P(v–a)CO2/C(a–v)O2 ratio vs. usual clinical practice in acute circulatory failure. A total of 90 patients were enrolled in each treatment group. The primary endpoint is the number of patients with a lactate clearance of more than 10% 2 h after inclusion. Lactate levels were monitored during the first 48 h of treatment as hemodynamic parameters, biological markers of organ failure, and 28-day mortality.
Discussion: pCO2 derivate indices may be of better interest than routine clinical indices to differentiate causes of hyperlactatemia and diagnose anaerobiosis. LACTEL results will provide clinical insights into the role of these indices in the early hemodynamic management of acute circulatory failure in the ICU.
Clinical Trial Registration: www.clinicaltrials.gov; identifier: NCT05032521.
Introduction
Acute circulatory failure (i.e., shock) is a very common life-threatening condition among patients admitted to intensive care units (ICUs) (1). Historically, treatment of shock has been based on physical examination (skin mottling, capillary refill time, and urine output) and biological macrohemodynamic parameters (blood pressure, arterial lactate, and mixed venous saturation).
During acute circulatory failure, hyperlactatemia remains a parameter widely used to assess tissue hypoperfusion despite its limitations (2). Hyperlactatemia and the clearance of arterial lactate have been demonstrated to be associated with morbidity and mortality in patients with shock and even during/following surgery (3–6). From a physiological perspective, hyperlactatemia can be the result of increased production (type A), reduced elimination (type B), or both (6, 7). Although identification of the exact cause underlying hyperlactatemia is very important for targeted therapy (fluid resuscitation, inotropic or vasopressor support), in clinical practice, it is very difficult to accurately identify the cause of hyperlactatemia (6).
From work carried out over the last 20 years, the pCO2 gap has been increasingly recognized as a reliable tool to evaluate tissue perfusion, because it was suggested as a marker of the relationship between cardiac output (CO) and global metabolic demand (8–11). Later, several studies have evaluated the P(v–a)CO2/C(a–v)O2 ratio as a parameter that was able to provide additional information about anaerobic metabolism in order to identify the causes of hyperlactatemia and to define shock severity (12–14). Based on these studies, authors have proposed different algorithms based on CO2-O2-derived indices to treat shock and arterial hyperlactatemia (7, 9).
Our hypothesis is that treatment of hyperlactatemia based on CO2-O2-derived indices would allow for a faster and higher lactate clearance, thus reducing inappropriate therapy and improving outcomes.
Materials and Methods
Study Design
LACTEL (Traitement de l'hyperLACtaTEmie du patient en insuffisance circuLatoire aigue basée sur l'analyse des indices dérivés du CO2: étude randomisée prospective de supériorité) is a randomized, prospective, multicentric, single-blind, parallel-group, superiority study. It compares the treatment of hyperlactatemia using an algorithm based on the pCO2 gap and the pCO2 gap/P(a-v)O2 ratio vs. usual clinical practice in patients with shock. The study was approved by an independent ethics committee (Comité de Protection des Personnes Sud Ouest et Outre-Mer on 8 July 2021, 2021-A101451-40) and was registered (ClinicalTrials.gov: NCT05032521). The study is conducted in ICUs of the University-affiliated tertiary Hospital of Dijon and the University-affiliated tertiary Hospital of Besancon, France. All the patients or their next of kin received written information about the study and gave consent to participate. The design of the study respects the requirements of the Medical Research Involving Human Subjects Act and the Declaration of Helsinki.
Standard protocol items: the study follows the SPIRIT Recommendations for Interventional Trials, and the SPIRIT protocol chronology is presented in Table 1.
The first patient was included in December 2021, and the last follow-up was planned until October 2024.
Study Population
Adult patients are considered for enrollment if they have acute circulatory failure and arterial blood lactate levels ≥3 mmol L−1. Acute circulatory failure was defined as systolic blood pressure < 90 mmHg, and/or mean blood pressure <65 mmHg, need for infusion of vasopressor catecholamines, and/or skin mottling, and/or diuresis <0.5 ml kg−1 h−1 (1, 15). All types of shock will be included: septic, cardiogenic, hemorrhagic, and post-operative.
The inclusion, non-inclusion, and exclusion criteria are provided in Table 2.
Study Intervention
Care of the patients is standardized and follows international guidelines and in-hospital protocols (1, 16–19). Preload dependence is assessed using the passive leg raising maneuver and/or the fluid challenge maneuver. Preload dependence is treated with a fluid bolus (250–500 ml) of Ringer Lactate for over 15 min. Cardiac output is optimized first by optimizing preload, and in the absence of preload dependency, by intravenous administration of dobutamine to obtain a cardiac index (CI) >2.5 L min−1 m−2. Cardiogenic failure is treated by intravenous administration of dobutamine (2.5–10 μg kg−1 min−1). Cardiac output can be measured by using echocardiography (Affinity or Epiq Philips™), pulmonary artery catheter, trans-pulmonary thermodilution (PiCCO device, Maquet™), or by Pressure Recording Analytical Method (Mostcare, Vygon™, France) (15). Vasoplegic syndrome is treated by intravenous infusion of norepinephrine (0.01 to 2 μg kg−1 min−1). Additional use of vasopressin if the norepinephrine dose is over 0.2 μg kg−1 min−1 is permitted. In accordance with the guidelines, we use a threshold of Hb <70 g/L for transfusion, in the absence of coronary artery disease. In patients with coronary artery disease, the threshold for transfusion is Hb <100 g/L. The mechanical ventilation parameters are adjusted on the basis of arterial blood analysis results. Baseline ventilator settings are as follows: respiratory rate of 10–18 breaths/min, tidal volume of 4–8 ml/kg, positive end-expiratory pressure of 5–10 cmH2O, a fraction of inspired oxygen of 0.25–1, targeting proper PaO2 of 100–120 mmHg, and PaCO2 of 35–45 mmHg. None of the non-hemodynamic therapies will be discontinued, and the drug dose regimen will not be modified. These therapeutic strategies do not differ in the two study groups. In the algorithm group, pCO2 is measured by central blood gas analysis.
The study intervention consists of treatment of hyperlactatemia following either an algorithm based on the pCO2 gap/P(a–v)O2 ratio and pCO2 gap or usual clinical practice based on macrohemodynamics, respecting randomization. If hemodynamic deterioration occurs despite hemodynamic optimization, a patient is excluded from the study and is treated following usual clinical protocols.
The algorithm is shown in Figure 1. Usual clinical practice is shown in Figure 2.
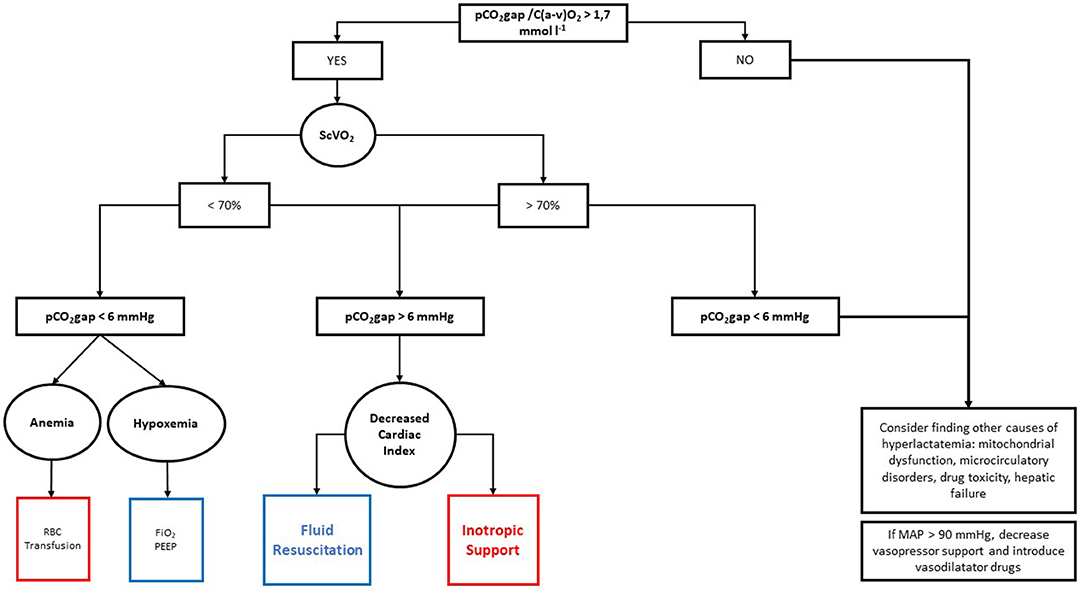
Figure 1. Hemodynamic algorithm based on pCO2 gap/P(a–v)O2 ratio. RBC, red blood cell; SvO2, mixed venous oxygen saturation; PEEP, positive end-expiratory pressure; FiO2, fraction of inspired oxygen.
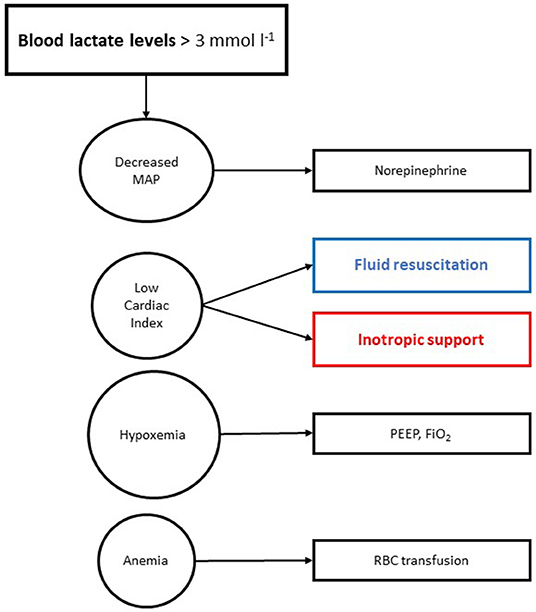
Figure 2. Usual hemodynamic algorithm. MAP, mean arterial pressure; PEEP, positive end-expiratory pressure; FiO2, fraction of inspired oxygen; RBC, red blood cell.
Randomization
The 1:1 ratio randomization (stratified on center and type of shock) is performed by the investigator after the patient's enrollment, using a dedicated e-platform made with CleanWebTM. The security of this process is assured by individual usernames and newly created passwords for each enrolment. A second check for the inclusion, non-inclusion, and exclusion criteria is required before continuing with the randomization. Technical support is provided by the Research Methodology Support Unit from our university-affiliated hospital, which also keeps a record of the full randomization process. Statistical analysis is performed by a statistician of the Research Methodology Support Unit who is not involved in the study process.
Objectives
The primary objective is to compare hyperlactatemia treatment effectiveness between an algorithm based on CO2-O2-derived indices and usual clinical practice after 2 h (3, 20).
The secondary objectives are as follows: (1) to compare lactate clearance at H12 and H24 between the two strategies; (2) to compare the number of patients with blood lactate levels <2 mmol l−1 at H12, H24, and H48; (3) to evaluate the ability of P(v–a)CO2/C(a–v)O2 to predict blood lactate levels > 2 mmol l−1; (4) to evaluate the ability of P(v–a)CO2/C(a–v)O2 to predict lactate clearance after hemodynamic optimization; (5) to evaluate the ability of P(v–a)CO2/C(a–v)O2 to predict VO2 changes after hemodynamic optimization; (6) to compare the number of patients with organ failure; and (7) to compare these effects on ICU length of stay, hospital length of stay, and 28-day mortality.
Endpoints
The primary endpoint is the number of patients with a lactate clearance over 10% between H0 and H2.
The secondary endpoints are as follows: (1) blood lactate levels at H12, H24, and H48; (2) lactate clearance at H12, H24, and H48; (3) relationship between pCO2 gap and blood lactate levels at H2, H12, and H24; (4) relationship between p(v–a)CO2/C(a–v)O2 and lactate clearance at H2, H12, and H24; (5) relationship between P(v–a)CO2 /C(a–v)O2 and VO2 at H2, H12, and H24; (6) SOFA score on days 0, 1, 2, 7, and 28; and (7) ICU length of stay, hospital length of stay, and 28-day mortality. Patients' follow-up is 28 days after enrolment.
Data Collection
Data are collected and recorded on eCRF by a physician who is not involved in the care of the patient, and who is blind to the study groups. All patient-related data are anonymously and numerically coded. Data collection encompasses the following: (1) the demographic characteristics of the population (age, sex, weight, SOFA score, SAPS II score, hospital and ICU admission reason, past medical history, and comorbidities), (2) the pharmacological treatment (type and infusion rate of sedation drugs, maximal dose and duration of norepinephrine and dobutamine, amount of fluid, fluid balance, and amount of blood transfusion), (3) the respiratory parameters (tidal volume, respiratory rate, positive end-expiratory pressure, inspiratory O2 fraction, end-tidal CO2, and plateau and peak pressure), (4) the hemodynamic state (heart rate, arterial blood pressure, pulse pressure, hourly urinary output, cardiac output, respiratory variation of pulse pressure, and/or stroke volume), and (5) the biological marker (hemoglobin, arterial and mixed venous oxygen saturation, partial pressure of CO2 in arterial and venous blood, blood lactate level, bicarbonate, base excess, creatinine, sodium, potassium, and chloride).
The study may be discontinued for an individual patient if the patient (or the person designated by the patient) so desires or if decided by the investigator. Every effort will be made to comply with the study protocol. However, the clinician in charge of the patient may deviate from these instructions at any time if he/she considers it necessary. He/she must note this decision and the reason for it in the case report form. If the study is discontinued, no provision is made for patient replacement. Likewise, patients who discontinued the study for whatever reason will receive standard care for the disease in question.
Statistics
Sample Size Calculation
Based on actual literature, lactate clearance over 10% over a 2-h period is between 50 and 60%. Assuming our hypothesis that an algorithm based on CO2-O2-derived indices can reach a 2-h lactate clearance over 80%, 82 patients should be randomized in each treatment group with a bilateral alpha risk of 5% and a power of 80%. After taking into account the risk of missing data and patients lost to follow-up (10% of incomplete data), we fixed the sample size to 180 patients, with 90 in each treatment arm.
Data Analysis Plan
Normality will be checked graphically with histograms and QQ plots and by the Shapiro-Wilk normality test. Continuous variables will be described as mean ± SD or as median (25–75%, IQR) as appropriate, and categorical variables will be described using a frequency table with a 95% confidence interval for the proportion. The data will be analyzed by intention to treat and per protocol. The two groups will be presented comparatively after randomization. Evaluation of the primary endpoint will be performed by Fisher's exact test. Categorical variables will be compared by Fisher's exact test. Continuous variables will be compared by Student's t-test or the Wilcoxon-Mann-Whitney test, as appropriate. Statistical analysis will be carried out using the R studio software. A p-value <0.05 will be considered statistically significant.
Discussion
Early hemodynamic management of patients with shock is a daily challenge for intensivists. The primary endpoint of each treatment is to correct tissue perfusion by optimizing hemodynamics in order to improve oxygen delivery to tissues (DO2), thus meeting tissue oxygen consumption (VO2) demands. Macrohemodynamic parameters (i.e., blood pressure and blood lactate levels) have limited adequacy to detect tissue hypoxia because they are often influenced by other factors not strictly linked to circulatory failure. Above all, although the relationship between hyperlactatemia and severity of shock, morbidity, and mortality is well-established (20, 21), high blood lactate levels may be the result of many pathological mechanisms, thus bringing false-positive results and overtreatment.
Over the past 20 years, researchers have constantly been searching for simple indicators that reflect tissue hypoperfusion with accuracy in various settings. The ideal marker should (1) be easy to measure and monitor; (2) should not require technically demanding tests; (3) if needed, the test must be rapid and rapidly available to deliver rapid results, reflecting the current (and not the past) condition of the patient; (4) should maintain its relationship with lactate clearance during ICU stays; and (5) should identify and distinguish anaerobic metabolism from other underlying conditions. In this context, CO2-O2-derived indices seem to be promising indicators to guide early resuscitation (7, 9).
In particular, the pCO2 gap, the difference between venous and arterial pCO2, was demonstrated to be associated with mortality in several diseases (sepsis and cardiogenic or perioperative shock) (8, 22, 23). Based on physiological assumptions and simplifications, the pCO2 gap was demonstrated to be associated with cardiac output and tissue perfusion (16, 23). In the same way, after studying the pCO2 gap, authors have studied the P(v–a)CO2/C(a–v)O2 ratio (12, 22, 24, 25). This ratio was studied as an indirect blood indicator of respiratory quotient that can detect anaerobic metabolism with better precision than other usual markers. P(v–a)CO2/C(a–v)O2 ratio may be able to predict VO2 responsiveness and hyperlactatemia (7, 14).
Considering the promising evidence, several authors have proposed clinical practice algorithms based on CO2-O2-derived indices, but the literature on the subject is based on observational studies, and some results appear contradictory (9, 20, 24). The originality of our research is that it consists of a randomized study, allowing us to evaluate the real ability of CO2-O2-derived indices in the treatment of hyperlactatemia based on the determination of its cause. We focused on arterial lactate clearance during the first 2 h of hemodynamic optimization, because this endpoint was widely studied and demonstrated as associated with mortality (3, 20, 21).
We included the possibility of exclusion of patients in case of hemodynamic degradation. This point can represent a limitation of the study that can decrease the application of the algorithm in a restricted population. Nevertheless, patient care and safety must be taken as a priority. Furthermore, by focusing on the first 2 h of treatment, the results of our study will clarify whether an algorithm based on CO2-O2-derived indices are able to identify the primary cause of hyperlactatemia, thus providing a better targeted hemodynamic treatment and reducing inappropriate management. If the results confirm our hypothesis, they will represent the first step for further clinical studies. A limitation could be the fact that we did not standardize CO measurement because all CO devices are not interchangeable. However, the same CO technique measure will be used for each patient.
In conclusion, the results of this study will be of major importance in the early treatment of hyperlactatemia during acute circulatory failure and will add further information on the potential clinical use of CO2-O2-derived parameters in acute care.
Ethics Statement
This study involving human participants was reviewed and approved by the Comité de Protection des Personnes Sud Ouest et Outre-Mer on 8 July 2021. The patients/participants provided their written informed consent to participate in this study.
Lactel Study Group
Audrey Martin, Mohamed Radhouani, Tiberiu Constandache, Sandrine Grosjean, Pierre Voizeux, Valerian Duclos, Vivien Berthoud, Alexandra Spitz, Marc Ginet, Yannick Brunin, Dejan Ilic, Corentin Evezard, and Laurent Carteron.
Author Contributions
P-GG, MN, and BB contributed to the conception and design. P-GG, MN, VC, and GB searched for the associated data. VC and P-GG drafted the manuscript. BB provided supervision support. MN performed data analysis. All authors contributed to the critical revision and final approval of the manuscript.
Funding
The authors perform this study in the course of their normal duties as full-time employees of public healthcare institutions.
Conflict of Interest
The authors declare that the research was conducted in the absence of any commercial or financial relationships that could be construed as a potential conflict of interest.
Publisher's Note
All claims expressed in this article are solely those of the authors and do not necessarily represent those of their affiliated organizations, or those of the publisher, the editors and the reviewers. Any product that may be evaluated in this article, or claim that may be made by its manufacturer, is not guaranteed or endorsed by the publisher.
References
1. Evans L, Rhodes A, Alhazzani W, Antonelli M, Coopersmith CM, French C, et al. Surviving sepsis campaign: international guidelines for management of sepsis and septic shock 2021. Crit Care Med. (2021) 49:e1063–143. doi: 10.1097/CCM.0000000000005337
2. Vincent J-L, Silva AQE, Couto L Jr, Taccone FS. The value of blood lactate kinetics in critically ill patients: a systematic review. Crit Care Lond Engl. (2016) 20:257. doi: 10.1186/s13054-016-1403-5
3. Gu W-J, Zhang Z, Bakker J. Early lactate clearance-guided therapy in patients with sepsis: a meta-analysis with trial sequential analysis of randomized controlled trials. Intensive Care Med. (2015) 41:1862–3. doi: 10.1007/s00134-015-3955-2
4. Bar S, Nguyen M, de Broca B, Bernard E, Dupont H, Guinot P-G. Risk factors and determinants of intraoperative hyperlactatemia in major non-cardiac surgery. J Clin Anesth. (2021) 74:110359. doi: 10.1016/j.jclinane.2021.110359
5. Abe T, Uchino S, Sasabuchi Y, Takinami M. The incidence and outcome of hyperlactatemia in patients admitted to the intensive care unit after elective surgery. Am J Surg. (2018) 216:886–92. doi: 10.1016/j.amjsurg.2018.04.007
6. Hernandez G, Bellomo R, Bakker J. The ten pitfalls of lactate clearance in sepsis. Intensive Care Med. (2019) 45:82–5. doi: 10.1007/s00134-018-5213-x
7. Waldauf P, Jiroutkova K, Duska F. Using pCO2 gap in the differential diagnosis of hyperlactatemia outside the context of sepsis: a physiological review and case series. Crit Care Res Pract. (2019) 2019:5364503. doi: 10.1155/2019/5364503
8. Huette P, Ellouze O, Abou-Arab O, Guinot P-G. Venous-to-arterial pCO2 difference in high-risk surgical patients. J Thorac Dis. (2019) 11:S1551–7. doi: 10.21037/jtd.2019.01.109
9. Mallat J, Lemyze M, Tronchon L, Vallet B, Thevenin D. Use of venous-to-arterial carbon dioxide tension difference to guide resuscitation therapy in septic shock. World J Crit Care Med. (2016) 5:47–56. doi: 10.5492/wjccm.v5.i1.47
10. Mallat J, Pepy F, Lemyze M, Gasan G, Vangrunderbeeck N, Tronchon L, et al. Central venous-to-arterial carbon dioxide partial pressure difference in early resuscitation from septic shock: a prospective observational study. Eur J Anaesthesiol. (2014) 31:371–80. doi: 10.1097/EJA.0000000000000064
11. Gavelli F, Teboul J-L, Monnet X. How can CO 2 -derived indices guide resuscitation in critically ill patients? J Thorac Dis. (2019) 11(Suppl. 11):S1528–37. doi: 10.21037/jtd.2019.07.10
12. Mekontso-Dessap A, Castelain V, Anguel N, Bahloul M, Schauvliege F, Richard C, et al. Combination of venoarterial PCO2 difference with arteriovenous O2 content difference to detect anaerobic metabolism in patients. Intensive Care Med. (2002) 28:272–7. doi: 10.1007/s00134-002-1215-8
13. Mesquida J, Saludes P, Gruartmoner G, Espinal C, Torrents E, Baigorri F, et al. Central venous-to-arterial carbon dioxide difference combined with arterial-to-venous oxygen content difference is associated with lactate evolution in the hemodynamic resuscitation process in early septic shock. Crit Care Lond Engl. (2015) 19:126. doi: 10.1186/s13054-015-0858-0
14. Mallat J, Lemyze M, Meddour M, Pepy F, Gasan G, Barrailler S, et al. Ratios of central venous-to-arterial carbon dioxide content or tension to arteriovenous oxygen content are better markers of global anaerobic metabolism than lactate in septic shock patients. Ann Intensive Care. (2016) 6:10. doi: 10.1186/s13613-016-0110-3
15. Cecconi M, De Backer D, Antonelli M, Beale R, Bakker J, Hofer C, et al. Consensus on circulatory shock and hemodynamic monitoring. Task force of the European Society of Intensive Care Medicine. Intensive Care Med. (2014) 40:1795–815. doi: 10.1007/s00134-014-3525-z
16. 2021 ESC Guidelines for the diagnosis and treatment of acute and chronic heart failure - PubMed. Available online at: https://pubmed.ncbi.nlm.nih.gov/34447992/ (accessed March 10, 2022).
17. Consensus on circulatory shock hemodynamic monitoring. Task force of the European Society of Intensive Care Medicine - PubMed. Available online at: https://pubmed.ncbi.nlm.nih.gov/25392034/ (accessed March 10, 2022).
18. Guinot P-G, Martin A, Berthoud V, Voizeux P, Bartamian L, Santangelo E, et al. Vasopressor-sparing strategies in patients with shock: a scoping-review and an evidence-based strategy proposition. J Clin Med. (2021) 10:3164. doi: 10.3390/jcm10143164
19. An Official American Thoracic Society/European Society of Intensive Care Medicine/Society of Critical Care Medicine Clinical Practice Guideline: Mechanical Ventilation in Adult Patients with Acute Respiratory Distress Syndrome - PubMed. Available online at: https://pubmed.ncbi.nlm.nih.gov/28459336/ (accessed March 10, 2022).
20. Zhang Z, Xu X. Lactate clearance is a useful biomarker for the prediction of all-cause mortality in critically ill patients: a systematic review and meta-analysis*. Crit Care Med. (2014) 42:2118–25. doi: 10.1097/CCM.0000000000000405
21. Jansen TC, van Bommel J, Woodward R, Mulder PGH, Bakker J. Association between blood lactate levels, Sequential Organ Failure Assessment subscores, and 28-day mortality during early and late intensive care unit stay: a retrospective observational study. Crit Care Med. (2009) 37:2369–74. doi: 10.1097/CCM.0b013e3181a0f919
22. Ospina-Tascón GA, Madriñán HJ. Combination of O2 and CO2-derived variables to detect tissue hypoxia in the critically ill patient. J Thorac Dis. (2019) 11:S1544–50. doi: 10.21037/jtd.2019.03.52
23. Ellouze O, Nguyen M, Missaoui A, Berthoud V, Aho S, Bouchot O, et al. Prognosis value of early veno arterial PCO2 difference in patients under peripheral veno arterial extracorporeal membrane oxygenation. Shock Augusta Ga. (2020) 54:744–50. doi: 10.1097/SHK.0000000000001554
24. Pavez N, Kattan E, Vera M, Ferri G, Valenzuela ED, Alegría L, et al. Hypoxia-related parameters during septic shock resuscitation: pathophysiological determinants and potential clinical implications. Ann Transl Med. (2020) 8:784. doi: 10.21037/atm-20-2048
Keywords: acute circulatory failure, hyperlactatemia, pCO2 gap, resuscitation, anaerobic metabolism, sepsis, cardiac surgery shock, lactic acidosis
Citation: Caruso V, Besch G, Nguyen M, Pili-Floury S, Bouhemad B, Guinot P-G and the Lactel Study Group (2022) Treatment of Hyperlactatemia in Acute Circulatory Failure Based on CO2-O2-Derived Indices: Study Protocol for a Prospective, Multicentric, Single, Blind, Randomized, Superiority Study (The LACTEL Study). Front. Cardiovasc. Med. 9:898406. doi: 10.3389/fcvm.2022.898406
Received: 17 March 2022; Accepted: 09 May 2022;
Published: 23 June 2022.
Edited by:
Sebastiano A. G. Lava, Centre Hospitalier Universitaire Vaudois (CHUV), SwitzerlandReviewed by:
Cho Ng, Great Ormond Street Hospital for Children NHS Foundation Trust, United KingdomPaul Philipp Heinisch, German Heart, Germany
Copyright © 2022 Caruso, Besch, Nguyen, Pili-Floury, Bouhemad, Guinot and the Lactel Study Group. This is an open-access article distributed under the terms of the Creative Commons Attribution License (CC BY). The use, distribution or reproduction in other forums is permitted, provided the original author(s) and the copyright owner(s) are credited and that the original publication in this journal is cited, in accordance with accepted academic practice. No use, distribution or reproduction is permitted which does not comply with these terms.
*Correspondence: Vincenza Caruso, vincenzacaruso22@gmail.com