- 1Department of Military Medical Geography, Army Medical Training Base, Army Medical University (Third Military Medical University), Chongqing, China
- 2College of Basic Medicine, Army Medical University, Chongqing, China
- 3NCO School, Army Medical University, Shijiazhuang, China
The prevalence and severity of hypertension-induced cognitive impairment increase with the prolonging of hypertension. The mechanisms of cognitive impairment induced by hypertension primarily include cerebral blood flow perfusion imbalance, white and gray matter injury with blood–brain barrier disruption, neuroinflammation and amyloid-beta deposition, genetic polymorphisms and variants, and instability of blood pressure. High homocysteine (HHcy) is an independent risk factor for hypertension that also increases the risk of developing early cognitive impairment. Homocysteine (Hcy) levels increase in patients with cognitive impairment induced by hypertension. This review summarizes a new mechanism whereby HHcy-mediated aberrant DNA methylation and exacerbate hypertension. It involves changes in Hcy-dependent DNA methylation products, such as methionine adenosyltransferase, DNA methyltransferases, S-adenosylmethionine, S-adenosylhomocysteine, and methylenetetrahydrofolate reductase (MTHFR). The mechanism also involves DNA methylation changes in the genes of hypertension patients, such as brain-derived neurotrophic factor, apolipoprotein E4, and estrogen receptor alpha, which contribute to learning, memory, and attention deficits. Studies have shown that methionine (Met) induces hypertension in mice. Moreover, DNA hypermethylation leads to cognitive behavioral changes alongside oligodendroglial and/or myelin deficits in Met-induced mice. Taken together, these studies demonstrate that DNA methylation regulates cognitive dysfunction in patients with hypertension. A better understanding of the function and mechanism underlying the effect of Hcy-dependent DNA methylation on hypertension-induced cognitive impairment will be valuable for early diagnosis, interventions, and prevention of further cognitive defects induced by hypertension.
Introduction
The prevalence of hypertension is increasing rapidly worldwide. More than 1.5 billion individuals currently experience hypertension (1). Hypertension not only affects the cerebral vasculature, parenchyma, and metabolism but is also related to an increased risk of cognitive decline and vascular cognitive impairment (1–3). Moreover, hypertension has been shown to induce cognitive decline and accelerate mild cognitive impairment (MCI), progressive deterioration, and dementia (4–7).
Hypertension-induced cognitive decline includes attention, memory, and decision-making impairments across all age groups from young individuals, middle-aged adults, and older adults (1, 8–11). Young hypertensive individuals can experience cognitive capacity-related changes (8). Cross-sectional studies in older adults have shown that hypertension contributes to cognitive decline, including simple attention, executive function, and psychomotor speed (11, 12). In older adults, hypertension, as a marker of cognitive impairment, affects cognitive performance (13, 14). Furthermore, studies have shown a relationship between blood pressure (BP) and cognitive function (15). Having an optimal BP range is associated with the maintenance of cognitive function (16). White coat hypertension and borderline hypertension groups exhibited poorer cognitive ability than the normal BP group (17). In addition, antihypertensive medications, such as calcium channel blockers, induce a protective effect against cognitive impairment in patients with hypertension (11, 18, 19).
Neuroimaging results have demonstrated that hypertension results in cognitive decline and neurostructural changes (20). The brains of hypertensive patients have higher amounts of amyloid-beta (Aβ) plaques, atrophy, and neurofibrillary tangles than healthy controls. Moreover, positron emission tomography (PET) studies have shown that hypertension is a risk factor for Aβ deposits and glucose metabolism disorders. Furthermore, numerous studies have used diffusion tensor imaging to show that hypertensive patients with small vessel disease have white matter changes (20–23). Hypertension is also related to cerebral microbleeds as demonstrated using brain magnetic resonance imaging (MRI) (24). A fluorodeoxyglucose-PET imaging study in older adult hypertensive patients revealed that high white matter signal is associated with low metabolism in gray matter, which may explain the memory impairment exhibited by these patients (25).
The renin–angiotensin–aldosterone system (RAAS) is central to BP regulation and plays an important role in the central nervous system. Inhibition of the RAAS can reduce the rate of cognitive decline in patients with MCI and dementia (26, 27). Angiotensin-converting enzyme (ACE) influences the metabolism of angiotensin in the RAAS system, and ACE inhibitors suppress microglial activation and preserve dendritic integrity and cognitive function (28).
Angiotensin II-treated mice exhibit short-term memory impairment and greater blood–brain barrier (BBB) leakage, microglial activation, and myelin loss than control adult mice (29). In addition, gradual induction of angiotensin II-dependent hypertension has been shown to produce significant spatial learning impairments in middle-aged cytochrome P450 family 1, subfamily A polypeptide 1 (Cyp1a1)-Ren2 transgenic rats (30). Furthermore, increased plasma aldosterone impairs cognitive function, whereas spironolactone and eplerenone, mineral corticoid receptor blockers, protect against cardiovascular mortality and cognitive impairment (31, 32). The activity of cytochrome c oxidase in various brain regions in mice with portal hypertension has been confirmed experimentally and has demonstrated that high pressure affects brain metabolic activity and spatial memory in varying degrees (33). Studies have also shown that the time of stimulus input in hippocampal slices of hypertensive mice correlates with decreased mRNA expression of several genes, such as brain-derived neurotrophic factor (BDNF), Homer1, and disks large homolog 4 (34).
Cognitive decline induced by hypertension
The supply of nutrients to the brain depends on dynamic blood flow. BP instability and abnormal blood flow and metabolism in patients with hypertension can damage neurons and impair cognitive function (35). There have been several advances in our understanding of the mechanisms underlying hypertension-induced cognitive decline, which include cerebral blood flow perfusion imbalance in response to cognitive and metabolic challenges, gray matter injury as reflected by changes in gray matter volume, cortical thinning, BBB dysfunction, Aβ accumulation, white matter injury, and genetic factors (7, 36). High homocysteine (HHcy) is one of the independent risk factors for hypertension that increases the risk of developing cognitive impairment (37).
Cerebral blood flow perfusion imbalance
In hypertensive patients, the cerebral blood supply can become damaged due to the destruction of the structural and functional integrity of the cerebral microcirculation, which results in greater sparsity of the microvasculature, endothelial dysfunction, and neurovascular uncoupling (7, 38, 39). Studies have suggested that disturbances in cerebral perfusion are one mechanism by which hypertension impacts cognitive decline. In the temporal and occipital lobes of hypertensive patients, both cerebral perfusion and total and local cortical thicknesses are decreased (39, 40). Elevated BP (both acute and sustained) results in the extravasation of fluid and damage to cerebral blood vessels (38). Moreover, endothelial dysfunction and abnormal cerebral microcirculation cause cognitive impairment in patients with hypertension (41). Furthermore, hypertension is associated with elevated levels of circulating endothelial microvesicles that contribute to BP-related endothelial dysfunction, which may serve as a biomarker for hypertension-induced cognitive impairment (41, 42).
Disruptions in neurovascular coupling, which forms the basis for the relationships among neuronal activity, hemodynamic factors, and cell-to-cell signaling, may lead to a decrease in vascular reserve capacity and cognitive decline in patients with either hyper- or hypotension (43). Elevated BP in hypertensive patients causes vascular dysfunction, which affects cerebrovascular blood regulation (especially microvascular circulation) and leads to cognitive decline (41). Hypertension can accelerate cerebral blood flow, reduce metabolism, and decrease glucose utilization (44). Accordingly, the interplay between functional blood flow reorganization and vascular brain damage results in hypertension-related cognitive decline. Indeed, microvascular dysfunction has been shown to be responsible for deficits in memory and processing speed and cognitive decline (45).
Gray matter injury
Gray matter disruptions are directly associated with cognitive impairment. Hypertension not only impairs the cardiovascular–renal axis but also impairs learning and memory (29). Spatial memory and cognition rely on the hippocampus, which is significantly smaller in hypertensive mice than in healthy mice (46). Hippocampal atrophy has been shown to be associated with hypertension; moreover, reduced dentate gyrus volume is associated with hypertension-induced cognitive impairment (47, 48). Hypertension impairs hippocampal neurogenesis in adult mice, affecting CA1 neurons, dendritic arborization, and long-term memory, which may be related to the down-regulation of the BDNF signaling pathway (49).
Hypertension damages the major arteries and capillaries in the brain, which, in turn, destroys the BBB and increases vascular permeability and accumulation of Aβ in the brain parenchyma (50, 51). Numerous studies have shown that MCI patients have accelerated destruction of the BBB and that blood vessel leakage accelerates hippocampal atrophy and neuronal loss, which leads to the onset of Alzheimer’s disease (AD) (52). A recent study showed that individuals with early cognitive impairment have brain capillary injuries. BBB destruction is an early biomarker of cognitive impairment that does not depend on Aβ or tau. Vascular contributions to cognitive impairment are increasingly being recognized (53, 54). Moreover, several angiodynamic changes can lead to cognitive decline. Studies in mice with cerebral venous congestion and BBB destruction have shown exacerbation of the neuroinflammatory response (51–54). Aβ promotes the overproduction of free radicals in endothelial cells, which results in neuronal cell necrosis. A study that explored the association between hypertension and AD using a hypertensive model of transverse aortic contraction found that the receptor for advanced cerebrovascular glycation end product activation is a key factor in the pathogenesis of AD. However, AD can be effectively suppressed by inhibiting this target, which offers potential as a new therapeutic option (50, 55). Another study showed that angiotensin II infusion causes learning and spatial memory deficits and anxiety, from the third week of perfusion, with Aβ deposition occurring in the fourth week (56).
White matter injury
In white matter, oligodendrocytes wrap the axons of neurons to form the myelin sheath to maintain appropriate nerve impulse conduction, which plays an important role in cognitive function (57). Endothelial cells or the permeability of brain cells produces change, which results in cerebral vascular injury or structural changes in the arterioles of white matter. In hypertensive patients, decreased cerebral blood flow is often followed by ischemic infarction, white matter damage, and brain atrophy (58–60). White matter lesions (WMLs), including subcortical and/or periventricular white matter loosening, are present in 85% of hypertensive patients (61).
A significant positive correlation exists between BP and white matter abnormalities in patients with hypertension. BP at baseline is closely related to WML volume, which is also associated with hypertension in most brain regions (62). Furthermore, white matter damage destroys cortical and subcortical connections, which leads to the disconnection of various functional areas, affecting the cognitive function of hypertensive patients (63). In a long-term study on white matter signal intensity and cognitive function in older adult hypertensive patients, white matter intensity was also positively correlated with cognitive function (64).
Genetic polymorphisms and variants
Studies on genetic polymorphisms and variants have helped improve our understanding of the relationship between hypertension and cognitive decline. High serum levels of the ACE, which regulates BP, may play an important role in the incidence of amnestic MCI (aMCI) (65, 66). Middle-aged and older adult patients with aMCI who carry the allele coding for the high-activity variant (D) of ACE show greater cognitive impairment, whereas those who carry the low-activity allele (I) have an increased risk of dementia (67–69). Furthermore, angiotensin II type 1 receptor polymorphisms play an important role in BP regulation and are related to reductions in prefrontal and hippocampal volumes, hippocampal volume over time, and memory loss in older adults (70, 71).
The methylenetetrahydrofolate reductase (MTHFR) C677T polymorphism has been reported to be associated with hypertension (72). Studies have shown that riboflavin, a cofactor of MTHFR, induces hypertension by regulating the methylation level of homozygous genes (73). The regulators of the G protein signaling 2 1891-1892del TC and cytochrome P450 family 4 subfamily A member 11 T8590C polymorphisms increased the risk of hypertension (74). Another study showed that hypertensive patients with cognitive impairment carry a copy of the apolipoprotein E4 (ApoE4) gene (75).
High homocysteine-induced cognitive decline in patients with hypertension
High homocysteine level promotes the occurrence of essential hypertension (EH) and cognitive impairment (76–78). It has been shown that the incidence of cognitive dysfunction increases as the concentration of homocysteine (Hcy) increases (79). Increased Hcy is likely to lead to vascular dementia, AD, and other pathological changes (78–80). Moreover, HHcy-stimulated vascular smooth muscle cell proliferation has been found to cause endothelial cell injury (81). The increase in Hcy leads to the oxidation of the vascular endothelium, which reduces vascular elasticity and increases susceptibility to hypertension (73, 79).
Cerebrovascular injury and neurotoxicity accelerate cognitive impairment. Hcy neurotoxicity manifests via the promotion of neuronal apoptosis, which affects nerve conduction and impairs cognitive function (79, 80, 82, 83). The connection between HHcy and cognitive decline can be explained by several mechanisms. HHcy, as a neurotoxin, promotes neurodegeneration via apoptosis and DNA breakage (82). Indeed, studies have shown that HHcy contributes to cognitive decline (83, 84). Additionally, recent studies have demonstrated that hypertensive patients with HHcy exhibit white matter hyperintensity and gray matter loss due to damaged cerebral vessels, which reflect the neurotoxic effects of HHcy (85). In mice, the Hcy level has been shown to affect the excitability of neurons and impair cognitive function. Moreover, long-term exposure to Hcy can induce changes in spatial learning, hippocampal signaling, and synaptic plasticity (84). Folic acid, vitamin B6, and vitamin B12 supplementation for 14 weeks significantly reduced the total serum Hcy level and improved cognitive function in middle-aged and older adult patients with HHcy (79, 86).
Mechanism of the mediation of cognitive decline induced by hypertension via high homocysteine-dependent DNA methylation
Homocysteine regulates the expression of genes related to cognitive function by interfering with methyl group metabolism and epigenetic regulation. HHcy mediates hypomethylation, which is caused by impaired DNA transmethylation, resulting in cognitive decline (82).
Homocysteine is an intermediate in the DNA methylation pathway
Methionine (Met) is a methyl donor that participates in the methylation of substrates, such as phospholipids, myelin, nucleic acids, choline, and catecholamine (79). Hcy is an intermediate product of the Met metabolism pathway. Met is converted into adenosylmethionine (SAM), which is catalyzed into S-adenosylhomocysteine (SAH) by methyltransferase. Subsequently, SAH is hydrolyzed to Hcy. Hcy can be remethylated into Met, and this reaction is dependent on folate as a substrate and vitamin B12 as a cofactor, as shown in Figure 1. Serum folate, Vitamin B12, and Hcy levels have been shown to correlate with cognitive function (79, 86). Moreover, HHcy is positively correlated with the prevalence of cognitive impairment and is considered a predictor of cognitive change (87, 88).
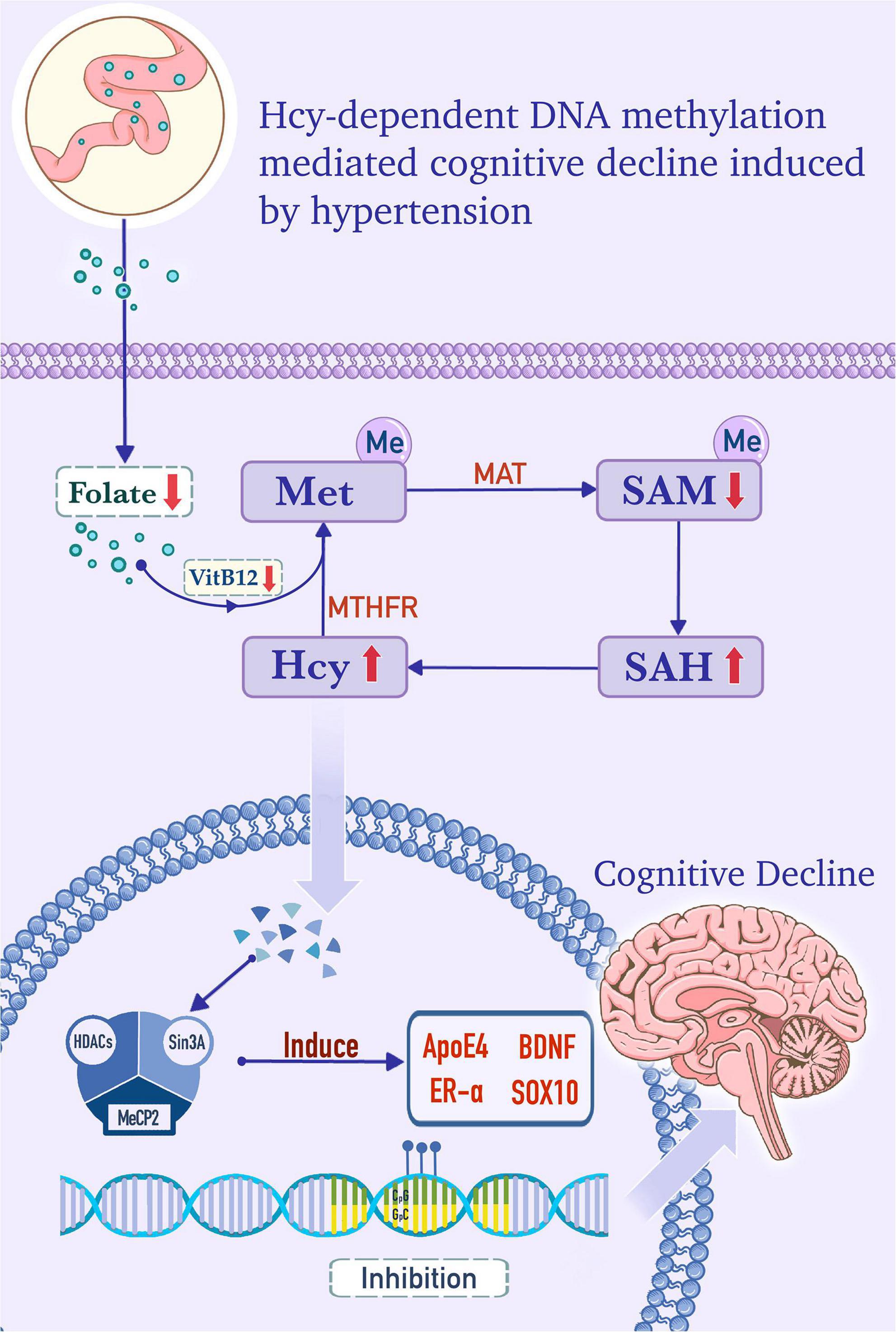
Figure 1. Homocysteine (Hcy)-dependent DNA methylation mediates cognitive decline induced by hypertension. In the cytoplasm, folate, from the serum as a substrate, and vitamin B12, as a cofactor, provide methyl for the conversion of homocysteine (Hcy) to methionine (Met). Met is converted into adenosylmethionine (SAM) by the catalysis of the Met adenosyltransferase (MAT). SAM is catalyzed into S-adenosylhomocysteine (SAH) by methyltransferase, and SAH is subsequently hydrolyzed into Hcy. Hcy is remethylated to form Met, and this step is dependent on methylenetetrahydrofolate reductase (MTHFR), folate, and vitamin B12. In hypertensive patients, folate and vitamin B12 deficiency, MTHFR deficiency, and/or MAT I/III deficiency, which are caused by MAT1A gene mutations, can increase the level of Hcy and promote the formation of the DNA methylation complex. The methylation complex is formed by methyl-CpG binding protein 2 (MeCP2), which recruits histone deacetylase (HDACs) and Sin3 transcription regulator family member A (Sin3A) under the catalysis of DNA methyltransferase (DNMT). The complex induces hypermethylation of apolipoprotein E4 (ApoE4), brain-derived neurotrophic factor (BDNF), estrogen receptor alpha (ER-α), and SOX10 genes, which is in accord with the lower expression levels of these factors and cognitive decline.
Homocysteine remethylation disorders are rare inherited disorders caused by deficient activity of the enzymes involved in the remethylation of Hcy to Met (89). Polymorphisms of the MTHFR gene are strongly associated with hypertension, and Met synthase 2756A > G and 5-methyltetrahydrofolate-homocysteine methyltransferase reductase 66A > G polymorphisms related to folate metabolism may serve as genetic markers for hypertension risk (90, 91). Patients with MTHFR deficiency exhibit cognitive deficits and psychiatric symptoms. In one study, the methylation rate of the methylenetetrahydrofolate dehydrogenase 1 (MTHFD1) promoter in patients with hypertension was shown to be significantly higher than that in the control group (92).
Homocysteine is a neurotoxic amino acid that induces calcium influx. Hcy-induced N-methyl-D-aspartic acid receptor channel activation can eventually lead to neuronal degeneration via glutamate excitotoxicity (93). HHcy reduces the cellular level of SAM, and co-treatment with SAM can antagonize apoptosis, which suggests that methylation mediates apoptosis (94). HHcy damages cerebral blood vessels via its neurotoxic effects and promotes the development of cognitive impairment and dementia. Some drug interventions for HHcy may improve and delay the progression of cognitive dysfunction and dementia. Patients with a folic acid deficiency with a normal Met level show impairments in spatial memory and learning. However, such deficits can be prevented by supplementation with Met in individuals with a folate-deficient diet (95). Furthermore, an increased serum Hcy level proportionally increases the risk of cognitive decline, whereas high levels of folic acid and B12 inhibit Hcy and serve as a protective factor for cognitive impairment. These findings suggest that increasing daily folic acid and vitamin B12 intake may normalize the Met cycle and methylation and protect the brain from functional damage (96). However, several studies have shown that vitamin supplements do not provide any benefit to patients with cognitive impairment (97). Thus, further investigations are necessary before interventions to reduce plasma Hcy levels are used to improve cognitive function.
Aberrant DNA methylation in neurons is involved in hypertension-related cognitive decline
The estrogen receptor alpha (ER-α) gene promoter is hypermethylated in EH patients and is positively correlated with plasma Hcy level (98). Increased plasma Hcy levels in EH patients occur via the hypermethylation of the ER-α gene promoter region. A plausible mechanism for this process is that high levels of plasma Hcy in EH patients increases the metabolite SAM, which provides more methyl groups for DNA methylation and increases the activity of DNA methyltransferase (DNMT). This leads to hypermethylation of the ER-α promoter region (98). In atheromatosis patients, the ER-α gene promoter is hypermethylated and is positively correlated to plasma Hcy levels (99). Moreover, the ER-α-A gene promoter has a high methylation rate in white matter-hyperintense patients with cognitive impairment and is associated with plasma Hcy levels. Methylation of the ER-α-A gene is a significant determining factor for cognitive impairment and is also significantly correlated with serum Hcy levels and white matter hyperintensity. In addition, ER-α-A gene promoter methylation is higher in patients with cognitive dysfunction and is also related to high plasma Hcy levels (100).
When the RAAS system is activated, angiotensin II plays an important role in cognitive decline resulting from chronic intermittent hypoxia-induced hypertension (101). Promoter methylation inhibits the expression of the angiotensin II type 2 receptor (AT2R) gene, which is related to increased cerebral hypoxic-ischemic injury caused by perinatal stress in neonatal rats (102). It has been shown that hypermethylation of CpG islands in the promoter region of the androgen receptor gene is significantly correlated with the level of HHcy in patients with vascular cognitive impairment (103).
The hippocampus plays a crucial role in spatial learning and memory. Hypertension impairs adult hippocampal neurogenesis, CA1 neuron dendritic arborization, and long-term memory. Aldosterone levels are increased in patients with hypertension, and it has been shown that aldosterone treatment significantly decreases DNA methylation and BDNF expression in the hippocampus (104). Demethylation of the BDNF exon IV promoter causes phosphorylation of methyl-CpG binding protein 2 (MeCP2), which can activate the transcription of BDNF in the rat hippocampus (105). Furthermore, elevated methylation of the BDNF promoter predicts conversion from aMCI to AD (106). However, the methylation rate of the BDNF promoter has been reported to vary among Xinjiang Uygur and Han populations with MCI (107).
The role of neurotrophic factors in the regulation of hippocampal long-term potentiation (LTP), which is the sustained increase in excitatory synaptic strength that is fundamental to learning and memory, has received considerable attention. Hypobaric hypoxia exposure increases the expression of DNMT1 and DNMT3b and significantly decreases the levels of pMeCP2 and BDNF (108). DNMT1 and DNMT3a affect learning and memory by regulating DNA methylation and neuronal gene expression (109).
Simulation experiments in zebrafish have shown that long-term exposure to Met significantly increases the activity of acetylcholinesterase, which is an enzyme that indirectly negatively impacts cognitive performance and contributes to the occurrence of neurodegenerative diseases (110). When Met is administrated to mice, the levels of Hcy and the induction of DNA hypermethylation increase, which leads to the down-regulation of several gamma-aminobutyric acidergic neuronal markers, such as reelin and glutamic acid decarboxylase 67 (GAD67); moreover, correlates with the onset of cognitive decline and schizophrenia-like behaviors (111). Met-induced hypermethylation of the reelin and GAD67 gene promoters is effectively reversed by valproate, histone deacetylase inhibitors, clozapine, and sulpiride (111–113). In addition, during fear conditioning, the synaptic plasticity gene reelin is demethylated and activated, and the memory suppressor gene protein phosphatase 1 is hypermethylated and inhibited (114). Studies have also shown that DNA activity-dependent methylation and demethylation are important substrates for reward-related experience-driven behavior and neuronal plasticity (115).
Homocysteine-dependent DNA methylation in oligodendroglial and/or myelin deficits
Genetic defects in enzymes involved in Hcy metabolism and folic acid, vitamin B6, or B12 deficiency elevate plasma Hcy levels, which increases the incidence of cardiovascular diseases (77, 79). The Met adenosyltransferase (MAT) 1a (MAT1A) gene, which encodes the major hepatic forms of the MAT protein, MATI and III, has been found to be strongly associated with hypertension (116). Patients with hypermethioninemia, which is caused by a MAT1A mutation, show neurological dysfunctions, cognitive impairment, learning impairment, and memory loss (117). MATI/III deficiency in mice due to a MAT1A gene mutation is characterized by high plasma Hcy levels and demyelination in the nervous system (118). The products of MAT and SAM are major methyl donors for myelin phospholipids, phosphatidylcholine, and sphingomyelin. Brain MRI studies have revealed periventricular hyperintensity and delayed or impaired myelination. Moreover, sural nerve biopsy studies have shown myelinated fiber loss (116–118).
Met is involved in the formation of the myelin sheath, which plays an important role in cognitive function. We found that Met-induced global DNA hypermethylation leads to oligodendroglial and/or myelin deficits and hypermethylation of the sex-determining region of the Y-chromosome (SRY)-related HMG-box 10 (SOX10) gene, which is an important factor for terminal differentiation of oligodendrocytes and cognitive behavioral changes (119). In addition, studies have demonstrated that Met can induce hypertension in mice (91, 120, 121). These findings suggest that DNA methylation may enable the regulation of cognitive dysfunction in patients with hypertension, although additional studies are needed to confirm this.
Hypertension induces cognitive decline and cerebral small vessel diseases, such as white matter hyperintensities, lacunar infarcts, and microhemorrhages. In white matter, DNA methylation deregulates ApoE4, huntingtin interacting protein 1, lectin mannose-binging 2, and myelin-associated oligodendrocytic basic protein (122, 123). DNA methylation of the SOX10 gene affects the gene expression of oligodendrocytes, which has become an epigenetic marker for schizophrenia (124).
The ApoE4 gene is associated with both hypertension and cognitive impairment induced by WMLs (75). MCI is part of the progression from normal aging to AD, and plasma Hcy and the ApoE4 gene are sensitive biomarkers for MCI in AD patients. Reduced ApoE methylation increases plasma ApoE levels, and increases in CPG165, CPG190, and CPG198 methylation are risk factors for MCI. Furthermore, increased CPG227 methylation decreases plasma ApoE levels, which results in a decreased risk of MCI (125).
Conclusion and future perspectives
Previous studies have established that hypertension induces cognitive decline via cerebral blood flow perfusion imbalance, white and gray matter injury with BBB disruption, neuroinflammation, and Aβ deposition. In addition, HHcy is an independent risk factor for hypertension-induced early cognitive impairment (126). However, the exact molecular mechanism underlying the effect of HHcy on cognitive impairment induced by hypertension remains unknown.
This review described changes in Hcy-dependent DNA methylation products, such as MAT, SAM, SAH, and MTHFR. Aldosterone treatment significantly decreases global DNA methylation, ER-α and ER-α-A gene promoter hypermethylation in EH patients, and white matter hyperintensity in patients with cognitive impairment, Furthermore, BDNF and ApoE methylation are involved in the MCI and AT2R receptor gene hypermethylation in the developing brain (98, 109). Met induces hypertension in mice, and we previously showed that DNA hypermethylation leads to cognitive behavioral changes with oligodendroglial and/or myelin deficits in Met-induced mice. These findings are shown in Tables 1, 2.
Widespread aberrant DNA methylation is present in patients with hypertension, and the level of 5-methylcytosine (5mC) and DNMTs, methylation of the MTHFD1 promoter, RAAS such as the ACE, angiotensin type 1 receptors AT1a and AT1b, endothelin-converting enzyme-1, adducin 1, the renal sodium retention system(e.g., 11 beta-hydroxysteroid dehydrogenase 2, and sodium-potassium-chloride cotransporter 1), the sympathetic nervous system(e.g., norepinephrine transporter), fatty acid binding protein 3, and glucokinase gene methylation are affected (92, 127). Moreover, DNA methylation mediates the process of cognitive decline. Furthermore, changes in DNMT1, DNMT3a2, DNMT3b, MeCP2, and 5mC correlate with cognitive decline (128).
Methylation regulates the expression of genes involved in the pathogenesis of hypertension (92, 127), some of which also mediate cognitive impairment (108, 128, 129). However, few studies have directly demonstrated that methylation regulates cognitive dysfunctions in patients with hypertension, such as learning, memory formation, and behavioral plasticity deficits. Methylation of numerous related genes, such as ACE, AT1a and AT1b in the RAAS, and SOX10, has positive effects on cognitive decline and provides a focus for future research on cognitive decline in patients with hypertension caused by DNA methylation. Furthermore, products of the Met metabolism pathway, such as SAM, SAH, Met, Hcy, folate, and vitamin B12, may be studied further in regard to cognitive decline induced by hypertension.
Author contributions
CW and R-YZ searched the literature and drafted the manuscript. X-SC critically revised the manuscript. All authors contributed to the article and approved the submitted version.
Funding
This work was supported by grants from Project of PLA (Nos. BLJ19J009 and 20QNPY006) and Open Project of Key Laboratory of Extreme Environmental Medicine of the Ministry of Education (No. KL2019GY005).
Acknowledgments
We thank Liwen Bianji (Edanz) (www.liwenbianji.cn/) for editing the English text of a draft of this manuscript and Gao Yang for drawing the figure.
Conflict of interest
The authors declare that the research was conducted in the absence of any commercial or financial relationships that could be construed as a potential conflict of interest.
Publisher’s note
All claims expressed in this article are solely those of the authors and do not necessarily represent those of their affiliated organizations, or those of the publisher, the editors and the reviewers. Any product that may be evaluated in this article, or claim that may be made by its manufacturer, is not guaranteed or endorsed by the publisher.
Abbreviations
ACE, angiotensin-converting enzyme; AD, Alzheimer’s disease; ApoE4, apolipoprotein E4; AT2R, angiotensin II type 2 receptor; Aβ, amyloid beta; BBB, blood–brain barrier; BDNF, brain-derived neurotrophic factor; BP, blood pressure; Dnmts, DNA methyltransferase; EH, essential hypertension; ER-α, estrogen receptor alpha; Hcy, homocysteine; HHcy, high homocysteine; MAT1A, methionine adenosyltransferase 1a; 5mC, 5-methylcytosine; MCI, mild cognitive impairment; MeCP2, methyl-CpG binding protein 2; Met, methionine; MRI, magnetic resonance imaging; MTHFD1, methylenetetrahydrofolate dehydrogenase 1; MTHFR, methylenetetrahydrofolate reductase; PET, positron emission tomography; RAAS, renin-angiotensin-aldosterone system; RAGE, receptor for advanced glycation end products; SAM, S-adenosyl methionine; SAH, S-adenosylhomocysteine; SOX10, sex determining region of the Y-chromosome (SRY)-related HMG-box 10; VCI, vascular cognitive impairment; WMLs, white matter lesions.
References
1. Skoog I. Hypertension and cognition. Int Psychogeriatr. (2003) 15(Suppl. 1):139–46. doi: 10.1017/S1041610203009104
2. Birns J, Kalra L. Cognitive function and hypertension. J Hum Hypertens. (2009) 23:86–96. doi: 10.1038/jhh.2008.80
3. Farmer ME, White LR, Abbott RD, Kittner SJ, Kaplan E, Wolz MM, et al. Blood pressure and cognitive performance. The Framingham study. Am J Epidemiol. (1987) 126:1103–14. doi: 10.1093/oxfordjournals.aje.a114749
4. Czuriga-Kovács KR, Czuriga D, Csiba L. Influence of hypertension, alone and in combination with other vascular risk factors on cognition. CNS Neurol Disord Drug Targets. (2016) 15:690–8. doi: 10.2174/1871527315666160518122721
5. Qin J, He Z, Wu L, Wang W, Lin Q, Lin Y, et al. Prevalence of mild cognitive impairment in patients with hypertension: a systematic review and meta-analysis. Hypertens Res. (2021) 44:1251–60. doi: 10.1038/s41440-021-00704-3
6. Sanchez Hoffmann S, Winkler A, Weimar C, Muller-Gerards D, Abramowski J, Moebus S, et al. Blood pressure and cognitive decline - the impact of hypertension over one decade. Neuropsychol Dev Cogn B Aging Neuropsychol Cogn. (2021) 28:528–42. doi: 10.1080/13825585.2020.1792403
7. Ungvari Z, Toth P, Tarantini S, Prodan CI, Sorond F, Merkely B, et al. Hypertension-induced cognitive impairment: from pathophysiology to public health. Nat Rev Nephrol. (2021) 17:639–54. doi: 10.1038/s41581-021-00430-6
8. Gupta R, Solanki RK, Pathak V. Blood pressure is associated with cognitive impairment in young hypertensives. World J Biol Psychiatry. (2008) 9:43–50. doi: 10.1080/15622970601187784
9. Kohler S, Baars MA, Spauwen P, Schievink S, Verhey FR, van Boxtel MJ. Temporal evolution of cognitive changes in incident hypertension: prospective cohort study across the adult age span. Hypertension. (2014) 63:245–51. doi: 10.1161/HYPERTENSIONAHA.113.02096
10. Paran E. Blood pressure and cognitive functioning among independent elderly. Am J Hypertens. (2003) 16:818–26. doi: 10.1016/s0895-7061(03)01005-7
11. Saxby BK, Harrington F, McKeith IG, Wesnes K, Ford GA. Effects of hypertension on attention, memory, and executive function in older adults. Health Psychol. (2003) 22:587–91. doi: 10.1037/0278-6133.22.6.587
12. Elias MF, Robbins MA, Schultz NR Jr., Pierce TW. Is blood pressure an important variable in research on aging and neuropsychological test performance? J Gerontol. (1990) 45:128–35. doi: 10.1093/geronj/45.4.p128
13. Scherr PA, Hebert LE, Smith LA, Evans DA. Relation of blood pressure to cognitive function in the elderly. Am J Epidemiol. (1991) 134:1303–15. doi: 10.1093/oxfordjournals.aje.a116033
14. van Boxtel MP, Gaillard C, Houx PJ, Buntinx F, de Leeuw PW, Jolles J. Can the blood pressure predict cognitive task performance in a healthy population sample? J Hypertens. (1997) 15:1069–76. doi: 10.1097/00004872-199715100-00004
15. Waldstein SR, Giggey PP, Thayer JF, Zonderman AB. Nonlinear relations of blood pressure to cognitive function: the Baltimore longitudinal study of aging. Hypertension. (2005) 45:374–9. doi: 10.1161/01.HYP.0000156744.44218.74
16. Obisesan TO, Obisesan OA, Martins S, Alamgir L, Bond V, Maxwell C, et al. High blood pressure, hypertension, and high pulse pressure are associated with poorer cognitive function in persons aged 60 and older: the Third National Health and Nutrition Examination Survey. J Am Geriatr Soc. (2008) 56:501–9. doi: 10.1111/j.1532-5415.2007.01592.x
17. Shehab A, Abdulle A. Cognitive and autonomic dysfunction measures in normal controls, white coat and borderline hypertension. BMC Cardiovasc Disord. (2011) 11:3. doi: 10.1186/1471-2261-11-3
18. McCaddon A, Hudson P, Davies G, Hughes A, Williams JH, Wilkinson C. Homocysteine and cognitive decline in healthy elderly. Dement Geriatr Cogn Disord. (2001) 12:309–13. doi: 10.1159/000051275
19. Tadic M, Cuspidi C, Hering D. Hypertension and cognitive dysfunction in elderly: blood pressure management for this global burden. BMC Cardiovasc Disord. (2016) 16:208. doi: 10.1186/s12872-016-0386-0
20. Chetouani A, Chawki MB, Hossu G, Kearney-Schwartz A, Chauveau-Beuret F, Bracard S, et al. Cross-sectional variations of white and grey matter in older hypertensive patients with subjective memory complaints. Neuroimage Clin. (2018) 17:804–10. doi: 10.1016/j.nicl.2017.12.024
21. Gons RA, de Laat KF, van Norden AG, van Oudheusden LJ, van Uden IW, Norris DG, et al. Hypertension and cerebral diffusion tensor imaging in small vessel disease. Stroke. (2010) 41:2801–6. doi: 10.1161/STROKEAHA.110.597237
22. Nitkunan A, Charlton RA, McIntyre DJO, Barrick TR, Howe FA, Markus HS. Diffusion tensor imaging and MR spectroscopy in hypertension and presumed cerebral small vessel disease. Magnetic Resonance Med. (2008) 59:528–34. doi: 10.1002/mrm.21461
23. Pantoni L. Cerebral small vessel disease: from pathogenesis and clinical characteristics to therapeutic challenges. Lancet Neurol. (2010) 9:689–701. doi: 10.1016/s1474-4422(10)70104-6
24. Uddin MN, Tivarus M, Adams H, Little E, Schifitto G, Lande MB. Magnetic resonance imaging in childhood primary hypertension: potential in the study of cognitive outcomes. Hypertension. (2021) 77:751–8. doi: 10.1161/hypertensionaha.120.15242
25. Verger A, Hossu G, Kearney-Schwartz A, Bracard S, Roch V, Van der Gucht A, et al. Grey-matter metabolism in relation with white-matter lesions in older hypertensive patients with subjective memory complaints: a pilot voxel-based analysis study. Cerebrovasc Dis. (2016) 42:106–9. doi: 10.1159/000445527
26. O’Caoimh R, Kehoe PG, Molloy DW. Renin Angiotensin aldosterone system inhibition in controlling dementia-related cognitive decline. J Alzheimers Dis. (2014) 42(Suppl. 4):S575–86. doi: 10.3233/jad-141284
27. Yagi S, Akaike M, Ise T, Ueda Y, Iwase T, Sata M. Renin-angiotensin-aldosterone system has a pivotal role in cognitive impairment. Hypertens Res. (2013) 36:753–8. doi: 10.1038/hr.2013.51
28. McHugh J. ACE inhibitors preserve cognitive function. Nat Rev Rheumatol. (2018) 14:623. doi: 10.1038/s41584-018-0102-9
29. Foulquier S, Namsolleck P, Van Hagen BT, Milanova I, Post MJ, Blankesteijn WM, et al. Hypertension-induced cognitive impairment: insights from prolonged angiotensin II infusion in mice. Hypertens Res. (2018) 41:817–27. doi: 10.1038/s41440-018-0090-9
30. Willeman MN, Chawla MK, Zempare MA, Biwer LA, Hoang LT, Uprety AR, et al. Gradual hypertension induction in middle-aged Cyp1a1-Ren2 transgenic rats produces significant impairments in spatial learning. Physiol Rep. (2019) 7:e14010. doi: 10.14814/phy2.14010
31. Sen S, Gürel N, Ufuktepe B, Ozünal ZG, Büyüklü C, Üresin Y. Evaluating the relationship of blood pressure, plasma angiotensin peptides and aldosterone with cognitive functions in patients with hypertension. Excli J. (2017) 16:245–55. doi: 10.17179/excli2016-725
32. Yagi S, Akaike M, Aihara K, Iwase T, Yoshida S, Sumitomo-Ueda Y, et al. High plasma aldosterone concentration is a novel risk factor of cognitive impairment in patients with hypertension. Hypertens Res. (2011) 34:74–8. doi: 10.1038/hr.2010.179
33. Arias N, Mendez M, Arias J, Arias JL. Brain metabolism and spatial memory are affected by portal hypertension. Metab Brain Dis. (2012) 27:183–91. doi: 10.1007/s11011-012-9276-z
34. Tucsek Z, Noa Valcarcel-Ares M, Tarantini S, Yabluchanskiy A, Fülöp G, Gautam T, et al. Hypertension-induced synapse loss and impairment in synaptic plasticity in the mouse hippocampus mimics the aging phenotype: implications for the pathogenesis of vascular cognitive impairment. GeroScience. (2017) 39:385–406. doi: 10.1007/s11357-017-9981-y
35. Faraco G, Sugiyama Y, Lane D, Garcia-Bonilla L, Chang H, Santisteban MM, et al. Perivascular macrophages mediate the neurovascular and cognitive dysfunction associated with hypertension. J Clin Invest. (2016) 126:4674–89. doi: 10.1172/jci86950
36. Dai HL, Guang XF, Zc X. Progress of hypertension and cognitive dysfunction. Chin J Hypertens. (2015) 23:1135–40.
37. Price BR, Wilcock DM, Weekman EM. Hyperhomocysteinemia as a risk factor for vascular contributions to cognitive impairment and dementia. Front Aging Neurosci. (2018) 10:350. doi: 10.3389/fnagi.2018.00350
38. Alosco ML, Gunstad J, Xu X, Clark US, Labbe DR, Riskin-Jones HH, et al. The impact of hypertension on cerebral perfusion and cortical thickness in older adults. J Am Soc Hypertens. (2014) 8:561–70. doi: 10.1016/j.jash.2014.04.002
39. Ogunniyi A, Talabi O. Cerebrovascular complications of hypertension. Niger J Med. (2001) 10:158–61.
40. De Silva TM, Faraci FM. Microvascular dysfunction and cognitive impairment. Cell Mol Neurobiol. (2016) 36:241–58. doi: 10.1007/s10571-015-0308-1
41. Konukoglu D, Uzun H. Endothelial dysfunction and hypertension. Adv Exp Med Biol. (2017) 956:511–40. doi: 10.1007/5584_2016_90
42. Stockelman KA, Hijmans JG, Bammert TD, Greiner JJ, Stauffer BL, DeSouza CA. Circulating endothelial cell derived microvesicles are elevated with hypertension and associated with endothelial dysfunction. Can J Physiol Pharmacol. (2020) 98:557–61. doi: 10.1139/cjpp-2020-0044
43. Shabir O, Berwick J, Francis SE. Neurovascular dysfunction in vascular dementia, Alzheimer’s and atherosclerosis. BMC Neurosci. (2018) 19:62. doi: 10.1186/s12868-018-0465-5
44. Fujishima M, Ibayashi S, Fujii K, Mori S. Cerebral blood flow and brain function in hypertension. Hypertens Res. (1995) 18:111–7. doi: 10.1291/hypres.18.111
45. Rensma SP, van Sloten TT, Houben A, Kohler S, van Boxtel MPJ, Berendschot T, et al. Microvascular dysfunction is associated with worse cognitive performance: the Maastricht study. Hypertension. (2020) 75:237–45. doi: 10.1161/hypertensionaha.119.13023
46. Wolters AF, Moonen AJH, Lopes R, Leentjens AFG, Duits AA, Defebvre L, et al. Grey matter abnormalities are associated only with severe cognitive decline in early stages of Parkinson’s disease. Cortex. (2020) 123:1–11. doi: 10.1016/j.cortex.2019.09.015
47. Korf ES, White LR, Scheltens P, Launer LJ. Midlife blood pressure and the risk of hippocampal atrophy: the Honolulu Asia Aging Study. Hypertension. (2004) 44:29–34. doi: 10.1161/01.HYP.0000132475.32317.bb
48. Li H, Sun D, Lu D, Zhang J, Zeng J. Low hippocampal dentate gyrus volume associated with hypertension-related cognitive impairment. Am J Alzheimers Dis Other Demen. (2020) 35:1533317520949782. doi: 10.1177/1533317520949782
49. Shih YH, Tsai SF, Huang SH, Chiang YT, Hughes MW, Wu SY. Hypertension impairs hippocampus-related adult neurogenesis, CA1 neuron dendritic arborization and long-term memory. Neuroscience. (2016) 322:346–57. doi: 10.1016/j.neuroscience.2016.02.045
50. Carnevale D, Mascio G, D’Andrea I, Fardella V, Bell RD, Branchi I, et al. Hypertension induces brain β-amyloid accumulation, cognitive impairment, and memory deterioration through activation of receptor for advanced glycation end products in brain vasculature. Hypertension. (2012) 60:188–97. doi: 10.1161/hypertensionaha.112.195511
51. Zlokovic BV. The blood-brain barrier in health and chronic neurodegenerative disorders. Neuron. (2008) 57:178–201. doi: 10.1016/j.neuron.2008.01.003
52. Montagne A, Barnes SR, Sweeney MD, Halliday MR, Sagare AP, Zhao Z, et al. Blood-brain barrier breakdown in the aging human hippocampus. Neuron. (2015) 85:296–302. doi: 10.1016/j.neuron.2014.12.032
53. Montagne A, Nation DA, Sagare AP, Barisano G, Sweeney MD, Chakhoyan A, et al. APOE4 leads to blood-brain barrier dysfunction predicting cognitive decline. Nature. (2020) 581:71–6. doi: 10.1038/s41586-020-2247-3
54. Nation DA, Sweeney MD, Montagne A, Sagare AP, D’Orazio LM, Pachicano M, et al. Blood-brain barrier breakdown is an early biomarker of human cognitive dysfunction. Nat Med. (2019) 25:270–6. doi: 10.1038/s41591-018-0297-y
55. Bueche CZ, Hawkes C, Garz C, Vielhaber S, Attems J, Knight RT. Hypertension drives parenchymal beta-amyloid accumulation in the brain parenchyma. Ann Clin Transl Neurol. (2014) 1:124–9. doi: 10.1002/acn3.27
56. Duchemin S, Belanger E, Wu R, Ferland G, Girouard H. Chronic perfusion of angiotensin II causes cognitive dysfunctions and anxiety in mice. Physiol Behav. (2013) 109:63–8. doi: 10.1016/j.physbeh.2012.10.005
57. Filley CM, Fields RD. White matter and cognition: making the connection. J Neurophysiol. (2016) 116:2093–104. doi: 10.1152/jn.00221.2016
58. Hiroki M, Miyashita K, Oda M. Tortuosity of the white matter medullary arterioles is related to the severity of hypertension. Cerebrovasc Dis. (2002) 13:242–50. doi: 10.1159/000057850
59. Yang Y, Kimura-Ohba S, Thompson JF, Salayandia VM, Cossé M, Raz L, et al. Vascular tight junction disruption and angiogenesis in spontaneously hypertensive rat with neuroinflammatory white matter injury. Neurobiol Dis. (2018) 114:95–110. doi: 10.1016/j.nbd.2018.02.012
60. Wilson C, Zhang X, Buckley C, Heathcote HR, Lee MD, McCarron JG. Increased vascular contractility in hypertension results from impaired endothelial calcium signaling. Hypertension. (2019) 74:1200–14. doi: 10.1161/hypertensionaha.119.13791
61. van Dijk EJ, Breteler MM, Schmidt R, Berger K, Nilsson LG, Oudkerk M. The association between blood pressure, hypertension, and cerebral white matter lesions: cardiovascular determinants of dementia study. Hypertension. (2004) 44:625–30. doi: 10.1161/01.Hyp.0000145857.98904.20
62. Kuller LH, Margolis KL, Gaussoin SA, Bryan NR, Kerwin D, Limacher M, et al. Relationship of hypertension, blood pressure, and blood pressure control with white matter abnormalities in the Women’s Health Initiative Memory Study (WHIMS)-MRI trial. J Clin Hypertens (Greenwich). (2010) 12:203–12. doi: 10.1111/j.1751-7176.2009.00234.x
63. Galluzzi S, Geroldi C, Benussi L, Ghidoni R, Testa C, Borsci G, et al. Association of blood pressure and genetic background with white matter lesions in patients with mild cognitive impairment. J Gerontol A Biol Sci Med Sci. (2008) 63:510–7. doi: 10.1093/gerona/63.5.510
64. Ferreira JP, Kearney Schwartz A, Watfa G, Zohra L, Felblinger J, Boivin JM, et al. Memory alterations and white matter hyperintensities in elderly patients with hypertension: the ADELAHYDE-2 study. J Am Med Dir Assoc. (2017) 18:451.e413–425. doi: 10.1016/j.jamda.2017.01.008
65. Pinheiro DS, Santos RS, Jardim P, Silva EG, Reis AAS, Pedrino GR, et al. The combination of ACE I/D and ACE2 G8790A polymorphisms revels susceptibility to hypertension: a genetic association study in Brazilian patients. PLoS One. (2019) 14:e0221248. doi: 10.1371/journal.pone.0221248
66. Sun F, He N, Zhang K, Wu N, Zhao J, Qiu C. Association of ACE gene A2350G and I/D polymorphisms with essential hypertension in the northernmost province of China. Clin Exp Hypertens. (2018) 40:32–8. doi: 10.1080/10641963.2017.1291659
67. Kölsch H, Jessen F, Freymann N, Kreis M, Hentschel F, Maier W, et al. ACE I/D polymorphism is a risk factor of Alzheimer’s disease but not of vascular dementia. Neurosci Lett. (2005) 377:37–9. doi: 10.1016/j.neulet.2004.11.062
68. Li Y, Zhang Z, Deng L, Bai F, Shi Y, Yu H, et al. Genetic variation in angiotensin converting-enzyme affects the white matter integrity and cognitive function of amnestic mild cognitive impairment patients. J Neurol Sci. (2017) 380:177–81. doi: 10.1016/j.jns.2017.06.026
69. Zhang Z, Deng L, Bai F, Shi Y, Yu H, Yuan Y, et al. ACE I/D polymorphism affects cognitive function and gray-matter volume in amnestic mild cognitive impairment. Behav Brain Res. (2011) 218:114–20. doi: 10.1016/j.bbr.2010.11.032
70. Taylor WD, Benjamin S, McQuoid DR, Payne ME, Krishnan RR, MacFall JR, et al. AGTR1 gene variation: association with depression and frontotemporal morphology. Psychiatry Res. (2012) 202:104–9. doi: 10.1016/j.pscychresns.2012.03.007
71. Zannas AS, McQuoid DR, Payne ME, MacFall JR, Ashley-Koch A, Steffens DC, et al. Association of gene variants of the renin-angiotensin system with accelerated hippocampal volume loss and cognitive decline in old age. Am J Psychiatry. (2014) 171:1214–21. doi: 10.1176/appi.ajp.2014.13111543
72. Amenyah SD, Ward M, McMahon A, Deane J, McNulty H, Hughes C, et al. DNA methylation of hypertension-related genes and effect of riboflavin supplementation in adults stratified by genotype for the MTHFR C677T polymorphism. Int J Cardiol. (2021) 322:233–9. doi: 10.1016/j.ijcard.2020.09.011
73. Wu YL, Hu CY, Lu SS, Gong FF, Feng F, Qian ZZ, et al. Association between methylenetetrahydrofolate reductase (MTHFR) C677T/A1298C polymorphisms and essential hypertension: a systematic review and meta-analysis. Metabolism. (2014) 63:1503–11. doi: 10.1016/j.metabol.2014.10.001
74. Zhang C, Wang L, Liao Q, Zhang L, Xu L, Chen C, et al. Genetic associations with hypertension: meta-analyses of six candidate genetic variants. Genet Test Mol Biomarkers. (2013) 17:736–42. doi: 10.1089/gtmb.2013.0080
75. de Leeuw FE, Richard F, de Groot JC, van Duijn CM, Hofman A, Van Gijn J. Interaction between hypertension, apoE, and cerebral white matter lesions. Stroke. (2004) 35:1057–60. doi: 10.1161/01.Str.0000125859.71051.83
76. Kumar A, Palfrey HA, Pathak R, Kadowitz PJ, Gettys TW, Murthy SN. The metabolism and significance of homocysteine in nutrition and health. Nutr Metab (Lond). (2017) 14:78. doi: 10.1186/s12986-017-0233-z
77. Miller AL. The methionine-homocysteine cycle and its effects on cognitive diseases. Altern Med Rev. (2003) 8:7–19.
78. Rodrigo R, Passalacqua W, Araya J, Orellana M, Rivera G. Homocysteine and essential hypertension. J Clin Pharmacol. (2003) 43:1299–306. doi: 10.1177/0091270003258190
79. Kim S, Choi BY, Nam JH, Kim MK, Oh DH, Yang YJ. Cognitive impairment is associated with elevated serum homocysteine levels among older adults. Eur J Nutr. (2018) 58:399–408. doi: 10.1007/s00394-017-1604-y
80. Lehmann M, Gottfries CG, Regland B. Identification of cognitive impairment in the elderly: homocysteine is an early marker. Dement Geriatr Cogn Disord. (1999) 10:12–20. doi: 10.1159/000017092
81. Hatefi M, Behzadi S, Dastjerdi MM, Ghahnavieh AA, Rahmani A, Mahdizadeh F, et al. Correlation of homocysteine with cerebral hemodynamic abnormality, endothelial dysfunction markers, and cognition impairment in patients with traumatic brain injury. World Neurosurg. (2017) 97:70–9. doi: 10.1016/j.wneu.2016.09.080
82. Jiang Y, Sun T, Xiong J, Cao J, Li G, Wang S. Hyperhomocysteinemia-mediated DNA hypomethylation and its potential epigenetic role in rats. Acta Biochim Biophys Sin (Shanghai). (2007) 39:657–67. doi: 10.1111/j.1745-7270.2007.00327.x
83. Ravaglia G, Forti P, Maioli F, Scali RC, Saccheitti L, Talerico T, et al. Homocysteine and cognitive performance in healthy elderly subjects. Arch Gerontol Geriatr Suppl. (2004) 9:349–57. doi: 10.1016/j.archger.2004.04.045
84. Algaidi SA, Christie LA, Jenkinson AM, Whalley L, Riedel G, Platt B. Long-term homocysteine exposure induces alterations in spatial learning, hippocampal signalling and synaptic plasticity. Exp Neurol. (2006) 197:8–21. doi: 10.1016/j.expneurol.2005.07.003
85. Kong Y, Li X, Chang L, Liu Y, Jia L, Gao L, et al. Hypertension with high homocysteine is associated with default network gray matter loss. Front Neurol. (2021) 12:740819. doi: 10.3389/fneur.2021.740819
86. Cai C, Xiao R, Van Halm-Lutterodt N, Zhen J, Huang X, Xu Y, et al. Association of MTHFR, SLC19A1 genetic polymorphism, serum folate, vitamin B(12) and HCY status with cognitive functions in Chinese adults. Nutrients. (2016) 8:665. doi: 10.3390/nu8100665
87. Lu ZH, Li J, Li XL, Ding M, Mao CJ, Zhu XY, et al. Hypertension with hyperhomocysteinemia increases the risk of early cognitive impairment after first-ever ischemic stroke. Eur Neurol. (2019) 82:75–85. doi: 10.1159/000504704
88. Tassino M, Campos TF, Guerra RO. Homocysteine (Hcy) and cognitive performance in a population sample of elderly Brazilians. Arch Gerontol Geriatr. (2009) 48:142–5. doi: 10.1016/j.archger.2007.12.002
89. Chang KJ, Zhao Z, Shen HR, Bing Q, Li N, Guo X, et al. Adolescent/adult-onset homocysteine remethylation disorders characterized by gait disturbance with/without psychiatric symptoms and cognitive decline: a series of seven cases. Neurol Sci. (2020) 42:1987–93. doi: 10.1007/s10072-020-04756-0
90. Hiraoka M, Kagawa Y. Genetic polymorphisms and folate status. Congenit Anom (Kyoto). (2017) 57:142–9. doi: 10.1111/cga.12232
91. Kim YR, Kang SH, Hong SH. Effects of methionine synthase and methionine synthase reductase polymorphisms on hypertension susceptibility. Genes Genomics. (2020) 42:477–83. doi: 10.1007/s13258-020-00917-9
92. Wang C, Xu G, Wen Q, Peng X, Chen H, Zhang J, et al. Significant association of methylenetetrahydrofolate dehydrogenase 1 promoter hypomethylation with stroke in a Chinese population with primary hypertension. Ann Clin Lab Sci. (2019) 49:112–8.
93. Ho PI, Ortiz D, Rogers E, Shea TB. Multiple aspects of homocysteine neurotoxicity: glutamate excitotoxicity, kinase hyperactivation and DNA damage. J Neurosci Res. (2002) 70:694–702. doi: 10.1002/jnr.10416
94. Kennedy BP, Bottiglieri T, Arning E, Ziegler MG, Hansen LA, Masliah E. Elevated S-adenosylhomocysteine in Alzheimer brain: influence on methyltransferases and cognitive function. J Neural Transm (Vienna). (2004) 111:547–67. doi: 10.1007/s00702-003-0096-5
95. Troen AM, Chao WH, Crivello NA, D’Anci KE, Shukitt-Hale B, Smith DE, et al. Cognitive impairment in folate-deficient rats corresponds to depleted brain phosphatidylcholine and is prevented by dietary methionine without lowering plasma homocysteine. J Nutr. (2008) 138:2502–9. doi: 10.3945/jn.108.093641
96. Meng H, Li Y, Zhang W, Zhao Y, Niu X, Guo J. The relationship between cognitive impairment and homocysteine in a B12 and folate deficient population in China: a cross-sectional study. Medicine (Baltimore). (2019) 98:e17970. doi: 10.1097/md.0000000000017970
97. Bhargava S, Bhandari A, Choudhury S. Role of homocysteine in cognitive impairement and Alzheimer’s disease. Indian J Clin Biochem. (2018) 33:16–20. doi: 10.1007/s12291-017-0646-5
98. Liu DF, Yang ZF, Liu D. Role of hcy in regulating methyl of estrogen receptor α gene promoter and its relationship with essential hypertension. Chin J Geriatric Heart Brain Vessel Dis. (2020) 22:20–3. doi: 10.3969/j.issn.1009-0126.2020.01.006
99. Huang YS, Zhi YF, Wang SR. Hypermethylation of estrogen receptor-alpha gene in atheromatosis patients and its correlation with homocysteine. Pathophysiology. (2009) 16:259–65. doi: 10.1016/j.pathophys.2009.02.010
100. Sun HY, Qu QM. Hypermethylation of ERsmall a, Cyrillic-A gene and high serum homocysteine level are correlated with cognitive impairment in white matter hyperintensity patients. QJM. (2019) 112:351–4. doi: 10.1093/qjmed/hcz031
101. Marciante AB, Shell B, Farmer GE, Cunningham JT. Role of angiotensin II in chronic intermittent hypoxia-induced hypertension and cognitive decline. Am J Physiol Regul Integr Comp Physiol. (2021) 320:R519–25. doi: 10.1152/ajpregu.00222.2020
102. Li Y, Xiao D, Yang S, Zhang L. Promoter methylation represses AT2R gene and increases brain hypoxic-ischemic injury in neonatal rats. Neurobiol Dis. (2013) 60:32–8. doi: 10.1016/j.nbd.2013.08.011
103. Fu ZH, Sun XY, Liu S, He SH. Association of vascular cognitive impairment with methylation status of the promoter region of androgen receptor gene and homocysteine level. J Int Neurol Neurosurg. (2016) 43:511–5. doi: 10.16636/j.cnki.jinn.2016.06.005
104. Buzgoova K, Graban J, Balagova L, Hlavacova N, Jezova D. Brain derived neurotrophic factor expression and DNA methylation in response to subchronic valproic acid and/or aldosterone treatment. Croat Med J. (2019) 60:71–7. doi: 10.3325/cmj.2019.60.71
105. Gomez-Pinilla F, Zhuang Y, Feng J, Ying Z, Fan G. Exercise impacts brain-derived neurotrophic factor plasticity by engaging mechanisms of epigenetic regulation. Eur J Neurosci. (2011) 33:383–90. doi: 10.1111/j.1460-9568.2010.07508.x
106. Xie B, Xu Y, Liu Z, Liu W, Jiang L, Zhang R, et al. Elevation of peripheral BDNF promoter methylation predicts conversion from amnestic mild cognitive impairment to Alzheimer’s disease: a 5-year longitudinal study. J Alzheimers Dis. (2017) 56:391–401. doi: 10.3233/JAD-160954
107. Ma W, Zhou X, Ji H, Luo M, Liu G, Li J, et al. Population difference in the association of BDNF promoter methylation with mild cognitive impairment in the Xinjiang Uygur and Han populations. Psychiatry Res. (2015) 229:926–32. doi: 10.1016/j.psychres.2015.07.017
108. Kumar R, Jain V, Kushwah N, Dheer A, Mishra KP, Prasad D, et al. Role of DNA methylation in hypobaric hypoxia-induced neurodegeneration and spatial memory impairment. Ann Neurosci. (2018) 25:191–200. doi: 10.1159/000490368
109. Feng J, Zhou Y, Campbell SL, Le T, Li E, Sweatt JD, et al. Dnmt1 and Dnmt3a maintain DNA methylation and regulate synaptic function in adult forebrain neurons. Nat Neurosci. (2010) 13:423–30. doi: 10.1038/nn.2514
110. Vuaden FC, Savio LE, Piato AL, Pereira TC, Vianna MR, Bogo MR, et al. Long-term methionine exposure induces memory impairment on inhibitory avoidance task and alters acetylcholinesterase activity and expression in zebrafish (Danio rerio). Neurochem Res. (2012) 37:1545–53. doi: 10.1007/s11064-012-0749-6
111. Dong E, Agis-Balboa RC, Simonini MV, Grayson DR, Costa E, Guidotti A. Reelin and glutamic acid decarboxylase67 promoter remodeling in an epigenetic methionine-induced mouse model of schizophrenia. Proc Natl Acad Sci U S A. (2005) 102:12578–83. doi: 10.1073/pnas.0505394102
112. Dong E, Nelson M, Grayson DR, Costa E, Guidotti A. Clozapine and sulpiride but not haloperidol or olanzapine activate brain DNA demethylation. Proc Natl Acad Sci U S A. (2008) 105:13614–9. doi: 10.1073/pnas.0805493105
113. Guidotti A, Grayson DR. DNA methylation and demethylation as targets for antipsychotic therapy. Dialogues Clin Neurosci. (2014) 16:419–29. doi: 10.31887/DCNS.2014.16.3/aguidotti
114. Miller CA, Sweatt JD. Covalent modification of DNA regulates memory formation. Neuron. (2007) 53:857–69. doi: 10.1016/j.neuron.2007.02.022
115. Day JJ, Childs D, Guzman-Karlsson MC, Kibe M, Moulden J, Song E, et al. DNA methylation regulates associative reward learning. Nat Neurosci. (2013) 16:1445–52. doi: 10.1038/nn.3504
116. Lai CQ, Parnell LD, Troen AM, Shen J, Caouette H, Warodomwichit D. MAT1A variants are associated with hypertension, stroke, and markers of DNA damage and are modulated by plasma vitamin B-6 and folate. Am J Clin Nutr. (2010) 91:1377–86. doi: 10.3945/ajcn.2009.28923
117. Korsisaari N, Rossi DJ, Paetau A, Charnay P, Henkemeyer M, Mäkelä TP. Conditional ablation of the Mat1 subunit of TFIIH in Schwann cells provides evidence that Mat1 is not required for general transcription. J Cell Sci. (2002) 115(Pt 22):4275–84. doi: 10.1242/jcs.00121
118. Furujo M, Kinoshita M, Nagao M, Kubo T. Methionine adenosyltransferase I/III deficiency: neurological manifestations and relevance of S-adenosylmethionine. Mol Genet Metab. (2012) 107:253–6. doi: 10.1016/j.ymgme.2012.08.002
119. Chen XJ, Huang NX, Cheng YJ, Cai QY, Tian YP, Chen XS, et al. DNA hypermethylation induced by L-Methionine leads to oligodendroglial and myelin deficits and schizophrenia-like behaviors in adolescent mice. Front Neurosci. (2021) 15:659853. doi: 10.3389/fnins.2021.659853
120. Bull C, Valverde JM, Berlet HH, Spaide JK, Tourlentes TT, Himwich HE. Hypertension with methionine in schizophrenic patients receiving tranylcypromine. Am J Psychiatry. (1964) 121:381–2. doi: 10.1176/ajp.121.4.381
121. Zhou Y, Zhao L, Zhang Z, Lu X. Protective effect of enalapril against methionine-enriched diet-induced hypertension: role of endoplasmic reticulum and oxidative stress. Biomed Res Int. (2015) 2015:724876. doi: 10.1155/2015/724876
122. Alelu-Paz R, Gonzalez-Corpas A, Ashour N, Escanilla A, Monje A, Guerrero Marquez C, et al. DNA methylation pattern of gene promoters of major neurotransmitter systems in older patients with schizophrenia with severe and mild cognitive impairment. Int J Geriatr Psychiatry. (2015) 30:558–65. doi: 10.1002/gps.4182
123. Bettencourt C, Foti SC, Miki Y, Botia J, Chatterjee A, Warner TT, et al. White matter DNA methylation profiling reveals deregulation of HIP1, LMAN2, MOBP, and other loci in multiple system atrophy. Acta Neuropathol. (2020) 139:135–56. doi: 10.1007/s00401-019-02074-0
124. Iwamoto K, Bundo M, Yamada K, Takao H, Iwayama-Shigeno Y, Yoshikawa T, et al. DNA methylation status of SOX10 correlates with its downregulation and oligodendrocyte dysfunction in schizophrenia. J Neurosci. (2005) 25:5376–81. doi: 10.1523/jneurosci.0766-05.2005
125. Mancera-Páez O, Estrada-Orozco K, Mahecha MF, Cruz F, Bonilla-Vargas K, Sandoval N, et al. Differential methylation in APOE (Chr19; Exon Four; from 44,909,188 to 44,909,373/hg38) and increased apolipoprotein E plasma levels in subjects with mild cognitive impairment. Int J Mol Sci. (2019) 20:1394. doi: 10.3390/ijms20061394
126. Iadecola C, Gottesman RF. Neurovascular and cognitive dysfunction in hypertension. Circ Res. (2019) 124:1025–44. doi: 10.1161/circresaha.118.313260
127. Smolarek I, Wyszko E, Barciszewska AM, Nowak S, Gawronska I, Jablecka A, et al. Global DNA methylation changes in blood of patients with essential hypertension. Med Sci Monit. (2010) 16:Cr149–55.
128. Morris MJ, Monteggia LM. Role of DNA methylation and the DNA methyltransferases in learning and memory. Dialogues Clin Neurosci. (2014) 16:359–71. doi: 10.31887/DCNS.2014.16.3/mmorris
129. Peter CJ, Fischer LK, Kundakovic M, Garg P, Jakovcevski M, Dincer A, et al. DNA methylation signatures of early childhood malnutrition associated with impairments in attention and cognition. Biol Psychiatry. (2016) 80:765–74. doi: 10.1016/j.biopsych.2016.03.2100
130. Hainsworth AH, Yeo NE, Weekman EM, Wilcock DM. Homocysteine, hyperhomocysteinemia and vascular contributions to cognitive impairment and dementia (VCID). Biochim Biophys Acta. (2016) 1862:1008–17. doi: 10.1016/j.bbadis.2015.11.015
131. Wu H, Coskun V, Tao J, Xie W, Ge W, Yoshikawa K, et al. Dnmt3a-dependent nonpromoter DNA methylation facilitates transcription of neurogenic genes. Science (2010) 329:444–8. doi: 10.1126/science.1190485
132. Martins E, Marcão A, Bandeira A, Fonseca H, Nogueira C, Vilarinho L. Methionine adenosyltransferase I/III deficiency in portugal: high frequency of a dominantly inherited form in a small area of douro high lands. JIMD Rep. (2012) 6:107–12. doi: 10.1007/8904_2011_124
133. Holmes L Jr., Lim A, Comeaux CR, Dabney KW, Okundaye O. DNA methylation of candidate genes (ACE II, IFN-ã, AGTR 1, CKG, ADD1, SCNN1B and TLR2) in essential hypertension: a systematic review and quantitative evidence synthesis. Int J Environ Res Public Health. (2019) 16:4829. doi: 10.3390/ijerph16234829
134. Zhi YF, Huang YS, Li ZH, Zhang R. Hypermethylation of estrogen receptor-á gene in atheromatosis patients and its correlation with hyperhomocysteinemia. Health Res. (2008) 37:314–7.
Keywords: hypertension, cognition decline, DNA methylation, Hcy, Met
Citation: Wan C, Zong R-Y and Chen X-S (2022) The new mechanism of cognitive decline induced by hypertension: High homocysteine-mediated aberrant DNA methylation. Front. Cardiovasc. Med. 9:928701. doi: 10.3389/fcvm.2022.928701
Received: 26 April 2022; Accepted: 30 September 2022;
Published: 24 October 2022.
Edited by:
Ertugrul Kilic, Istanbul Medipol University, TurkeyReviewed by:
Snigdha Mukerjee, Vanderbilt University, United StatesXiang Li, The Chinese University of Hong Kong, Hong Kong SAR, China
Copyright © 2022 Wan, Zong and Chen. This is an open-access article distributed under the terms of the Creative Commons Attribution License (CC BY). The use, distribution or reproduction in other forums is permitted, provided the original author(s) and the copyright owner(s) are credited and that the original publication in this journal is cited, in accordance with accepted academic practice. No use, distribution or reproduction is permitted which does not comply with these terms.
*Correspondence: Xing-Shu Chen, eGluZ3NodWNoZW4yMDExQDE2My5jb20=