- 1Department of Cardiology, Royal Prince Alfred Hospital, Sydney, NSW, Australia
- 2Department of Cardiology, Concord Hospital, Sydney, NSW, Australia
- 3Sydney Medical School, University of Sydney, Darlington, NSW, Australia
The coronary microcirculation plays a cardinal role in regulating coronary blood flow to meet the changing metabolic demands of the myocardium. Coronary microvascular dysfunction (CMD) refers to structural and functional remodeling of the coronary microcirculation. CMD plays a role in the pathogenesis of obstructive and non-obstructive coronary syndromes as well as myocardial diseases, including heart failure with preserved ejection fraction (HFpEF). Despite recent diagnostic advancements, CMD is often under-appreciated in clinical practice, and may allow for the development of novel therapeutic targets. This review explores the diagnosis and pathogenic role of CMD across a range of cardiovascular diseases, its prognostic significance, and the current therapeutic landscape.
Introduction
Coronary Microvascular Dysfunction (CMD) is characterized by the remodeling of the coronary microcirculation in response to various pathogenic stimuli. The evolving use of invasive and non-invasive diagnostic modalities has enabled understanding of the role of CMD across a range of cardiovascular conditions, including atherosclerotic epicardial coronary disease, coronary syndromes with no obstructive coronary arteries and primary myocardial pathologies. Furthermore, the presence of CMD is an early marker of cardiovascular disease and is associated with poor clinical outcomes (1). This review aims to describe the mechanistic and prognostic role of CMD across a spectrum of cardiovascular conditions. In addition, we discuss currently available diagnostic modalities to identify CMD, evaluate current treatment strategies and highlight future clinical trials exploring novel therapeutic targets.
Role of the coronary microcirculation
The coronary arterial bed contains three structurally and functionally distinct compartments (2). The large epicardial coronary arteries (500 μm–5 mm in diameter) function as conduit vessels that offer little resistance to coronary blood flow (CBF), in the absence of obstructive atheroma (3–5). The epicardial pre-arterioles (500–100 μm) account for most of the resistance and respond to flow-related stimuli. The proximal component is more responsive to shifts in flow, whilst the distal is more sensitive to pressure variations (3–5). The intramyocardial arterioles (<100 μm) have the highest resistance and are responsible for the metabolic regulation of CBF in response to myocardial oxygen demand (autoregulation). Lastly, the capillaries (<10 μm) function as exchange vessels, given their large surface area and relatively high permeability (3–5). The coronary microcirculation refers to the epicardial pre-arterioles, intramyocardial arterioles, and capillaries.
Coronary microvascular dysfunction is characterized by structural and functional remodeling of the microcirculation leading to impaired coronary blood flow autoregulation (4). Potential mechanisms include dysfunctional coronary vasodilator capacity and/or enhanced reactivity to microvascular vasoconstriction. The endothelium is fundamental in regulating CBF through the modulation of vasorelaxant substances, including nitric oxide (NO), prostacyclin (PGI2), and endothelium-derived hyperpolarizing factors (EDHF) (6, 7). Dysfunctional endothelium results from pathological vasoconstrictive substances (such as endothelin-1, superoxide, hydrogen peroxide, and thromboxane) overhauling the vascular steady state (8, 9). In addition, microvascular spasm involves the impairment of vasomotion (physiological rhythmical contractions) and is closely linked to the presence of endothelial dysfunction (10, 11). Its complex pathophysiology is characterized by vascular smooth muscle cell (VSMC) dysfunction coupled with a predominance of vasoconstrictive metabolites in the setting of enhanced Rho-kinase activity (12–14). Enhanced Rho-kinase activity results in excessive myosin light chain phosphorylation by inhibiting the myosin-binding submit (MBS), leading to a state of hyper-contractility (15). In addition, chronic low-grade inflammation and overactivity of the autonomic nervous system may also contribute (16, 17).
The primary structural alternations in CMD include luminal narrowing of the intramural arterioles and capillaries, perivascular fibrosis and capillary rarefaction (4). These pathological changes often occur in the context of left ventricular hypertrophy. In addition, conditions such as hypertrophic cardiomyopathy (HCM) and hypertensive heart disease result in the thickening of medial and intimal vessel walls, leading to impaired CBF (18). Such remodeling of the microcirculation is associated with various comorbidities, including diabetes, hypertension, renal impairment, and diffuse epicardial atherosclerosis (9). Figure 1 summaries the pathogenic abnormalities that characterize CMD.
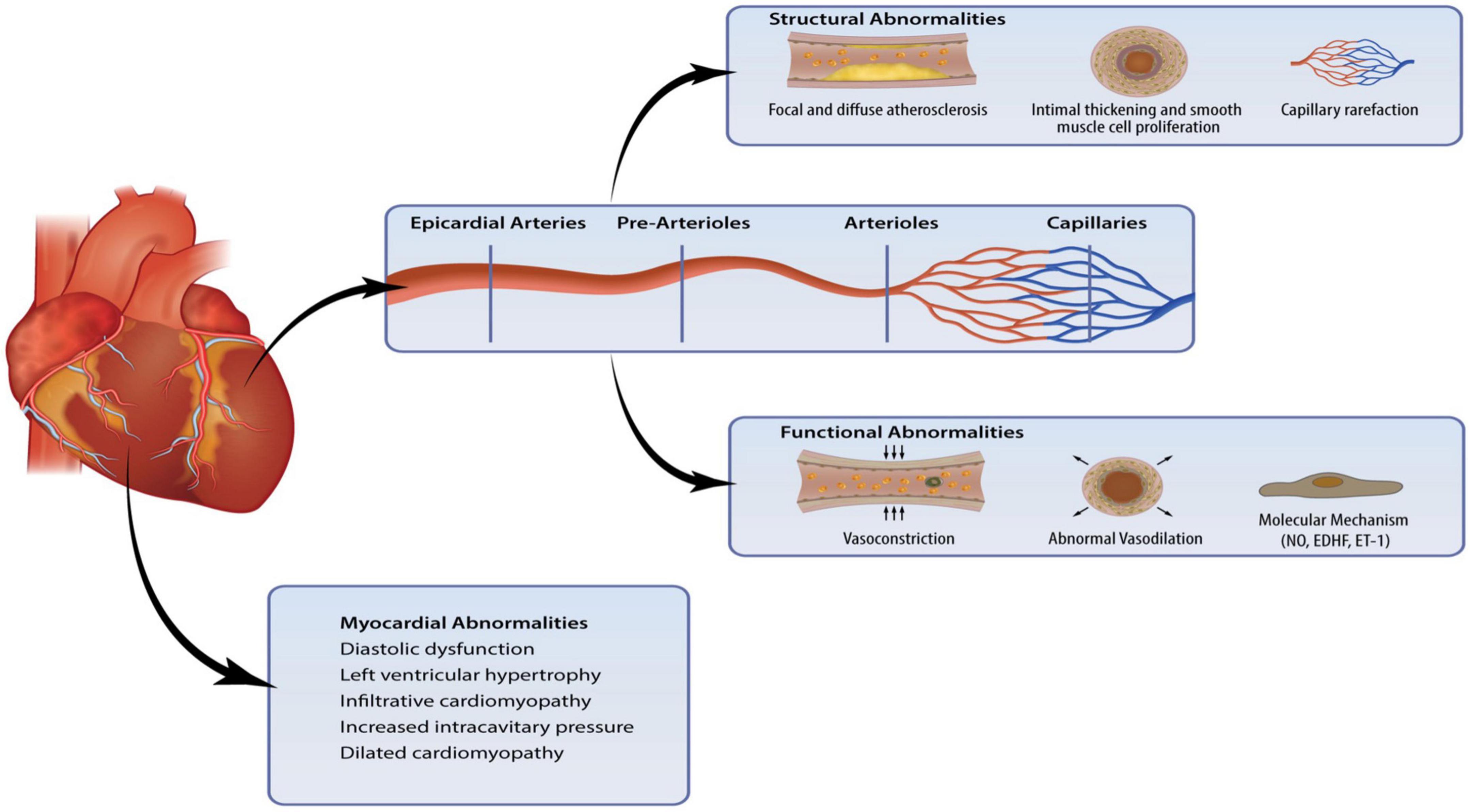
Figure 1. Coronary microvascular dysfunction (CMD) is a result of pathogenic changes in the coronary microcirculation and myocardium. Structural and functional abnormalities lead to endothelial dysfunction and myocardial abnormalities can affect the intramural microvasculature.
Methods of assessing CMD
Assessment of the coronary microcirculation requires functional evaluation of the coronary arteries. Invasive and non-invasive methods can be considered though each has its own advantages and limitations (Tables 1, 2). The optimal method for evaluation depends on patient profile, nature of symptoms (acute vs. chronic), comorbidities, and requirement of serial assessments. Despite growing evidence highlighting the prognostic significance of CMD, European Society of Cardiology (ESC) guidelines currently provide a class IIa recommendation for invasive testing and class IIb recommendation for non-invasive testing (19). In addition, protocols for invasive measurements vary between institutions world-wide, creating difficulties for international comparisons and guidelines.
Invasive
Comprehensive functional angiography allows clinicians to investigate the coronary microcirculation and exclude the presence of obstructive CAD (20). Coronary flow reserve (CFR) and measurements of microvascular resistance identify an impaired vasodilatory response (21). In addition, intracoronary provocation testing can identify a microvascular hypercontractive response (22).
Coronary flow reserve is defined as the ratio of maximum hyperemic to basal coronary flow velocity (23). It is a surrogate marker for the vasodilator capacity of the coronary microcirculation and can be assessed via an intracoronary Doppler flow wire (CFRDoppl) or temperature sensor-tipped guidewire (CFRthermo) (24). Both techniques have evidence to predict clinical events and can simultaneously measure fractional flow reserve (FFR) to exclude or confirm the presence of hemodynamically significant epicardial stenosis (25, 26). This is crucial as CFR is reduced in patients with epicardial coronary artery disease (CAD) and an impaired hyperemic microvascular dilatory response. CFR is considered normal when values are >2.5 (4, 7). The index of microvascular resistance (IMR) is a marker of minimal achievable resistance of the microcirculation measured using a thermodilution technique (27). It is defined as the product of the distal coronary pressure and mean transit time of a saline bolus during maximal hyperemia. Notably, it is independent of epicardial vascular function, heart rate, blood pressure and ventricular contractility (28). Compared with CFR, IMR provides a more reproducible assessment of the microcirculation, which is independent of hemodynamic perturbations (27). Alternatively, hyperemic microvascular resistance (HMR) using Doppler flow velocity can also be considered.
The diagnosis of coronary spasm (epicardial or microvascular) requires intracoronary provocation testing with acetylcholine (ACh) or ergonovine (ER). Acetylcholine (ACh) is a vasoactive substance that provokes vasospasm via cholinergic receptors on vascular smooth muscle cells (29). Healthy endothelium responds to ACh through vasodilatation via nitric oxide (NO) release (30). In contrast, dysfunctional endothelium cannot release enough NO to counteract the stimulated muscarinic receptors leading to vasoconstriction (30). In the context of microvascular spasm, the patient will demonstrate ischemic electrocardiographic changes along with reproduction of the typical symptoms (e.g., angina) without angiographic evidence of epicardial vasoconstriction. The safety of intracoronary provocation testing is excellent with similar rates of serious adverse events as routine diagnostic coronary angiography (31, 32). A large retrospective study from Japan of 21,512 patients undergoing pharmacological intracoronary provocation testing demonstrated < 1% serious cardiac complications (33). This was reiterated by Ciliberti et al. who published a systematic review demonstrating a low overall occurrence of major and minor adverse events (0.8 and 4.7%, respectively) (34). Predictors for complications during ACh provocation testing included a history of paroxysmal AF, moderate-to-severe LV diastolic dysfunction and higher QT dispersion at baseline ECG (35). A recent prospective analysis by Montone et al. demonstrated that these complications are not associated with a worse prognosis at a medium-long term follow-up (35). The authors also highlighted the prognostic significance of a positive ACh provocation test, especially in patients with myocardial infarction and no obstructive coronary artery disease (MINOCA) (35).
Non-invasive
Non-invasive methods to diagnose CMD should only be used after epicardial coronary disease has been excluded. Such techniques can only assess the vasodilator capacity of the coronary microvasculature and provide an estimate of myocardial blood flow. As mentioned above, assessment of endothelial-dependent microvascular dysfunction requires invasive provocation testing.
Transthoracic Doppler echocardiography (TTDE) of the LAD coronary artery is a widely applicable method to assess coronary flow velocity reserve (CFVR) (36). Nevertheless, technical issues, reduced spatial resolution and operator variability limit its use (24). On the other hand, myocardial contrast echocardiography is a promising method that provides a more objective assessment of coronary vasodilator capacity. Its use has also been limited, given the lack of validation in large scale studies (37). Positron emission tomography (PET) and cardiac magnetic resonance imaging (CMR) are two imaging modalities that provide a valid global and regional myocardial blood flow estimate. PET calculates the rate of radioactive tracer uptake into the left ventricular myocardium, allowing for automated quantification of absolute global myocardial blood flow (mL/min/g) at rest and peak hyperemia (38, 39). Alternatively, CMR measures myocardial signal intensity changes between rest and stress images to assess myocardial perfusion reserve (MPR). It can also calculate MPR quantitatively as a ratio of absolute myocardial blood flow (mL/min/g) at peak hyperemia and rest (40). Higher spatial resolution and lack of ionizing radiation make CMR beneficial, though lack of availability and higher costs have limited its use in clinical settings.
CMD in obstructive and non-obstructive coronary syndromes
CMD in acute obstructive coronary syndromes
Despite restoring patency of the epicardial coronary vessels in acute coronary syndrome, structural and functional abnormalities of the microvasculature result in suboptimal myocardial reperfusion. This phenomenon, known as coronary microvascular obstruction (CMVO), is an important predictor of left ventricular remodeling, HF, and death in patients with ST-segment elevation acute myocardial infarction (STEMI) (41–43). It’s pathogenesis involves four interacting mechanisms: distal atherothrombotic embolization, ischemia-related injury, reperfusion-related injury, and individual susceptibility (44).
Distal atherothrombotic embolization results in mechanical obstruction and functional impairment via biologically active substances promoting a procoagulant state and vasoconstriction (45). Ischemia-related injury is characterized by capillary damage determined by the duration and extent of ischemia (46). Despite restoration of epicardial vessel patency, reperfusion itself can aggravate microvascular injury (MVI) by inducing a vigorous inflammatory response with subsequent leucocyte and platelet plugging, the release of free oxygen radicals and a reduction in nitric oxide bioavailability (47). A subset of patients may develop intramyocardial hemorrhage (IMH), an irreversible pathological consequence of severe MVI (48). Lastly, individual susceptibility to CMVO is characterized by genetic polymorphisms (e.g., 1976T.C mutation of the adenosine 2A receptor gene), diabetes, acute hyperglycemia, hypercholesterolemia, and lack of pre-conditioning (44, 49). Despite its prognostic significance, an optimal treatment strategy for CMVO is still under debate, with numerous RCTs exploring the role of intracoronary thrombolysis illustrating inconsistent results (50–55).
CMD in chronic obstructive coronary syndrome
The synergistic effect of CMD and obstructive CAD leads to the progression of myocardial ischemia. Epicardial CAD can result in low perfusion pressures distal to the stenosis, which results in abnormal microvascular remodeling and a reduction in coronary vasodilator capacity (56). These pathological changes hinder the development of collateral flow, a critical response to stress-induced myocardial ischemia (56). In addition, the presence of CMD can lead to an underestimation of the degree of epicardial stenosis measured by FFR (57). The prognostic implications of CMD have been highlighted in recent literature (58–60). Van de Hoef et al. demonstrated that an invasively derived CFR < 2.7 in patients with obstructive CAD was associated with increased all-cause mortality (61). Similar findings were derived by Gupta and Cortigiani, who used PET and TTDE, respectively (58, 62). Interestingly, Taqueti et al. confirmed a reduced CFR modified the effect of early revascularization (63). In 329 patients referred for invasive coronary angiography after stress testing, only those with a low CFR appeared to benefit from revascularization, and solely if the revascularization included CABG (63). Hence, CMD in obstructive CAD is not only a prognostic marker but can also guide a successful treatment strategy.
CMD post-revascularization
Coronary microvascular dysfunction following successful PCI can lead to persistent angina representing a sub-optimal clinical result. The underlying pathophysiology is characterized by distal plaque embolization, neo-atherosclerosis, incomplete revascularization, and vasomotor abnormalities (64, 65). Notably, the pharmacological coating of drug-eluting stents (DES) may enhance coronary vasoconstrictive reactivity and promote neo-atherosclerosis via the interaction of the canonical mTOR pathway and FK506-binding protein 12 (66). This concept was emphasized by Ong et al., who illustrated the presence of vasomotor abnormalities in almost half the patients undergoing coronary angiography for recurrent angina after PCI (31). Furthermore, the prognostic significance of CMD post-revascularization was demonstrated by a study of 570 patients that found a correlation between impaired IMR (>25) post-PCI and adverse clinical events in patients with stable CAD (67). This association was independent of the final FFR value suggesting the prognostic significance of CMD despite successful revascularization of epicardial vessels. Likewise, Li et al. and Milo et al. highlighted that a reduction in CFR post-PCI is associated with recurrent angina and abnormal functional stress testing (measured by intracoronary thermodilution and TTDE, respectively) (68, 69). These studies reiterate the clinical significance of residual CMD post-revascularization.
CMD in ischemia with no obstructive coronary artery disease
Coronary microvascular dysfunction characterizes the mechanistic abnormalities in microvascular angina (MVA), a prevalent endotype in patients with ischemia and no obstructive coronary artery disease (INOCA). CMD is present in almost half of patients with INOCA (70). The abnormal vasomotor function of the coronary microvasculature leads to recurrent angina and a poor quality of life (71). Metabolic risk factors such as, dyslipidemia, obesity and T2DM are highly prevalent in INOCA (72). These comorbidities contribute to endothelial dysfunction by promoting a pro-inflammatory state and increased adrenergic activity.
Coronary microvascular dysfunction is an important prognostic marker for this cohort of patients. In the WISE study, a prospective cohort study of women with symptoms of ischemia undergoing coronary angiography, reduced CFR was a powerful incremental predictor of MACE (73). Likewise, subsequent studies using non-invasive measures of coronary vasodilatory capacity demonstrated similar results (62, 74). Murthy et al. provided the most definitive data highlighting the association between CFR < 2.0 (measured by PET) with a 7.8 and 5.6% annualized rate of MACE among symptomatic men and women with INOCA (compared to 3.3 and 1.7%, respectively, for CFR ≥ 2.0) (75).
Classifying patients into specific INOCA endotypes (microvascular angina, epicardial vasospastic angina, non-cardiac chest pain) can allow clinicians to prescribe targeted pharmacological treatment. For example, the CorMica trial demonstrated that functional angiography for correct classification of INOCA endotypes leads to improved patient outcomes, including a reduction in angina severity and better quality of life (20).
CMD in myocardial infarction with no obstructive coronary artery disease
Myocardial infarction with non-obstructive coronary artery disease (MINOCA) accounts for approximately 6–14% of patients with acute MI cases and represents a myriad of underlying etiologies (76). These patients have a 1-year mortality and rehospitalization rates comparable to those with MI and obstructive coronary artery disease (MI-CAD) (77, 78). Despite the poor understanding of the underlying pathophysiological mechanism in MINOCA, over 20% of cases are associated with CMD (79). CMD develops due to two separate mechanistic pathways: acute hyper-adrenergic tone with a rapid rise in microvascular resistance leading to cardiomyocyte death or chronic microvascular ischemia coupled with an acute trigger causing myocardial necrosis. The prognostic significance of CMD in patients with MINOCA was demonstrated by Abdu and colleagues’ evaluation of 109 patients (80). Microvascular function was assessed via coronary angiography-derived index of microvascular resistance (caIMR), a novel non-invasive method based on quantitative flow ratio (QFR). Over half (50.5%) of the patients were classified into the high caIMR group, and the rate of MACE was significantly higher in this cohort (80). In some cases, CMD may not be the sole cause of MINOCA but simply an “innocent” bystander playing a pathogenic role. For example, using stress CMR, Mauricio et al. demonstrated abnormal stress perfusion in 63% of females with MINOCA, however, this only corresponded with a myocardial scar in 75% of cases (81). These findings suggest that further mechanistic studies are required to elucidate the precise contribution of CMD in MINOCA.
CMD in myocardial pathologies
CMD in heart failure with preserved ejection fraction
Heart failure with preserved ejection fraction (HFpEF) refers to a heterogeneous syndrome characterized by diastolic dysfunction secondary to adverse LV remodeling, cardiometabolic dysfunction, and extracellular fibrosis (82–84). In recent times, our understanding of this clinical entity has evolved. We now acknowledge HFpEF is a systemic disease characterized by inflammation and microvascular endothelial dysfunction. It is hypothesized that the interplay between immunoregulatory cytokines and reduced NO results in an inflammatory milieu leading to CMD (85). Comorbidities such as hypertension, type II diabetes, chronic kidney disease, and obesity contribute to and accelerate this process, ultimately leading to adverse clinical outcomes (86).
Over the last decade, research has provided insights to the links between CMD and HFpEF. The PROMIS-HFpEF study demonstrated the presence of CMD (as per Doppler echocardiography) in 75% of HFpEF patients and its association with markers of HF severity (87). In addition, a recent cohort study confirmed similar findings in 106 patients who underwent functional angiography and CMR. Overall, 85% had evidence of CMD, with the majority illustrating endothelial-independent dysfunction (88). These results are consistent with older retrospective studies that suggested this association (87, 89). Taqueti et al. not only confirmed the presence of CMD in HFpEF but highlighted its prognostic significance. CMD was associated with a > 5-fold risk of HFpEF hospitalization, higher rate of MACE and mortality (90). Despite the overwhelming evidence confirming the presence of CMD in HFpEF, the question of cause or effect remains. Does CMD lead to HFpEF, or does myocardial remodeling in HFpEF lead to coronary microvascular ischemia and subsequent dysfunction? Nonetheless, targeting CMD in HFpEF patients might be a promising therapeutic strategy in years to come.
CMD takotsubo cardiomyopathy
Takotsubo Cardiomyopathy (TTC) is an acute and reversible form of myocardial dysfunction. Despite several etiopathogenetic mechanisms proposed, most believe it is characterized by a catecholaminergic surge following an emotional or psychological stressor leading to coronary microvascular vasoconstriction (91). Numerous diagnostic indices have confirmed the presence of CMD in this syndrome. Non-invasive modalities demonstrated reduced myocardial blood flow and perfusion defects within dysfunctional segments (92–94). In addition, numerous groups confirmed an elevated IMR in such patients, suggesting impaired microcirculatory resistance (95–97). The degree of CMD may have prognostic significance in this cohort. Montone et al. prospectively evaluated 101 patients and demonstrated a correlation between coronary slow flow on angiography and poor long-term clinical outcomes (98). Such measures may allow clinicians to risk stratify patients and consider aggressive medical therapy with close follow-up.
CMD in miscellaneous cardiomyopathies
Hypertrophic cardiomyopathy (HCM) is a genetic disorder that involves a complex interplay of myocyte disarray, interstitial fibrosis, and coronary microvascular remodeling (84, 99). In the presence of increased oxygen demand, such structural changes predispose the myocardium to ischemia leading to clinical sequelae of angina, syncope, and sudden death (100). Multiple coronary microvascular indices have demonstrated the presence of CMD in HCM. Camici et al. used PET to quantify MBF and demonstrate the presence of CMD in both hypertrophied and non-hypertrophied myocardium (101). Further studies highlighted a reduced CFR in the sub-endocardium secondary to elevated extravascular compressive forces. The presence of CMD in HCM has important prognostic implications. Cecchi et al. found that the severity of CMD (as assessed by PET) was an independent predictor of cardiovascular morbidity and mortality (102). Moreover, they highlighted that severe CMD was even present in many asymptomatic patients, preceding adverse cardiovascular outcomes by several years.
Infiltrative cardiomyopathies represent a group of disorders characterized by changes in the myocardium and coronary microvasculature leading to significant remodeling and mechanical dysfunction. Over the past decade, clinical research has confirmed the presence of CMD in cardiac amyloidosis, Anderson-Fabry disease and sarcoidosis. Contemporary literature employing non-invasive imaging demonstrated a significant reduction in hyperemic blood flow and reserve in this cohort (103–105). Interestingly, the presence of CMD can also be an early marker of disease activity and may denote a favorable window for an aggressive therapy (104, 106). Despite these efforts, there is minimal literature available to explain the prognostic impact of CMD in these patients.
Treatment and future directions
At this stage, there is no specific therapy for CMD. Current practice is primarily based on studies with heterogeneous populations and varied diagnostic techniques. Current therapeutic strategies focus on optimizing risk factors, lifestyle modifications, and conventional pharmacological therapy (Figure 2). Risk factors for CMD overlap with traditional cardiac risk factors. Management of diabetes, hypertension and hypercholesterolemia is essential in this cohort. In addition, lifestyle modifications, including physical exercise, smoking cessation and weight loss, contribute to improvements in cardiorespiratory fitness and CVD outcomes (107–110). Recently, Camilli et al. demonstrated an association between ambient air pollution and coronary vasomotor abnormalities (111). In particular, higher levels of airborne particular matter (PM) < 2.5 μm was an independent risk factor for the occurrence of epicardial spasm and MINOCA (111). These findings suggest that reducing air pollution levels may improve ischemic symptoms.
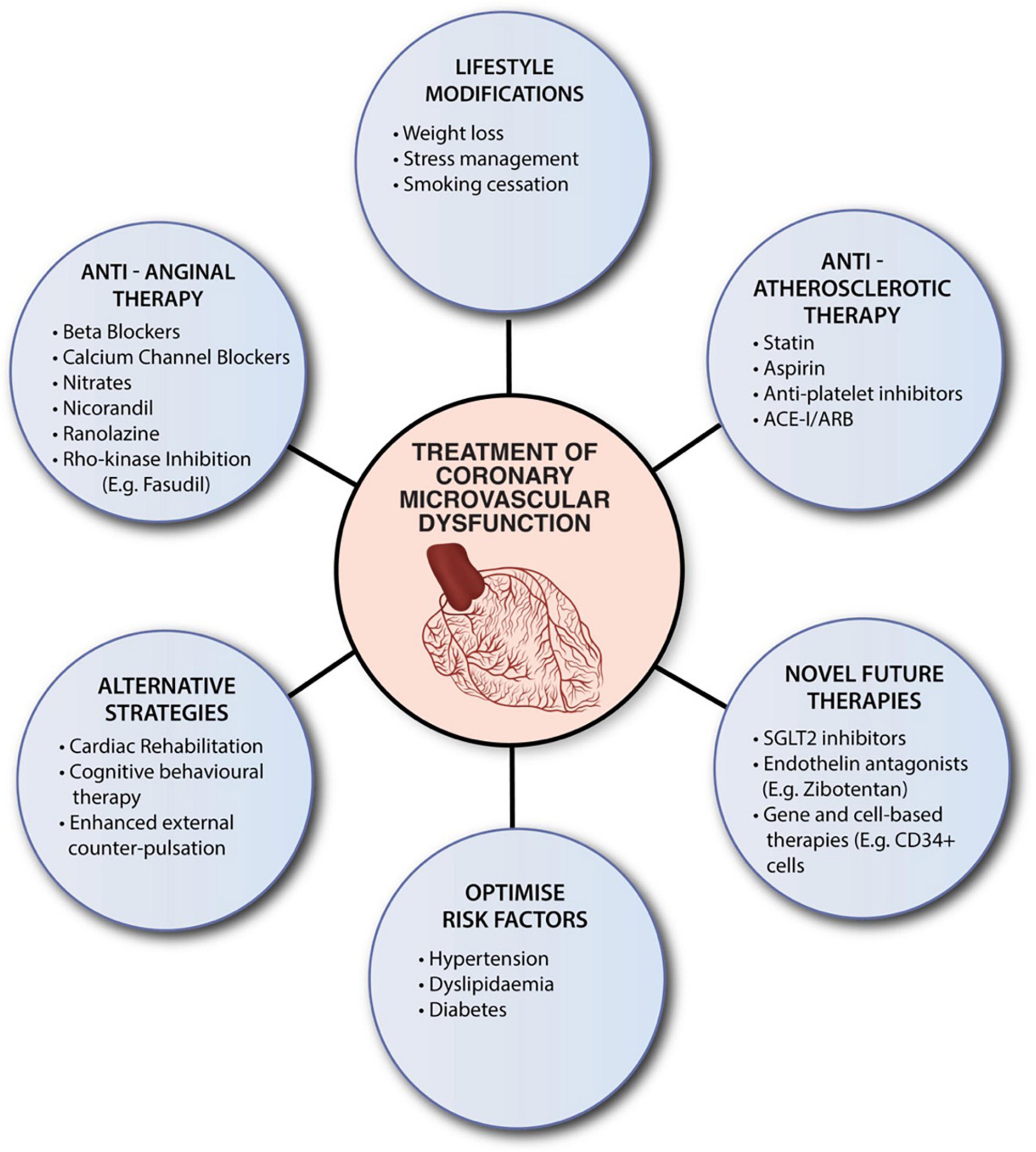
Figure 2. Summaries current therapeutic strategies for CMD. Despite the absence of specific evidenced-based therapies, clinicians should consider optimizing risk factors, encourage lifestyle modifications, and utilize conventional pharmacological therapy. ACE-1, angiotensin-converting enzyme inhibitors (ACE-I); ARB, angiotensin receptor blockers; SGLT2, sodium-glucose cotransporter-2 inhibitor.
Conventional pharmacotherapy includes anti-atherosclerotic and anti-anginal and drugs. Anti-atherosclerotic therapy consists of angiotensin-converting enzyme inhibitors (ACE-I) or receptor blockers (ARB), statins and thromboxane A2 inhibitors (TXA2). ACE-I elicit vasoprotective effects through inhibition of the renin-angiotensin axis. The TREND data (Trial on Reversing Endothelial Dysfunction) demonstrated the ability of ACE-I, particularly quinapril, to reverse endothelial dysfunction (112). Subsequent studies have emulated similar results for ARB (113). Statins are primarily HMG-CoA reductase inhibitors that reduce plaque, macrophage and foam cell formation. Their off-target anti-inflammatory effects play a prominent role in CMD (114). TXA2 inhibitors, such as aspirin, minimize platelet-rich-microemboli, which confers microvascular protection against oxidative injury. Together, these agents’ anti-inflammatory and antioxidant properties improve myocardial perfusion and coronary endothelial function.
Regarding anti-anginal pharmacotherapy, beta-blockers are first line for patients with CMD. They primarily improve symptoms of effort angina in patients with elevated adrenergic activity. Notably, third-generation beta-blockers (e.g., nebivolol and carvedilol) can reduce myocardial oxygen demand through their adjunctive NO-mediated vasodilatory effect (115). Short-acting nitrates can also be considered in patients with anginal attacks with an abnormal vasodilatory reserve (116). On the other hand, non-dihydropyridine calcium-channel blockers are preferred in patients with vasospasm-mediated CMD (116). Ranolazine and Fasudil are novel agents that may provide concurrent benefits. Ranolazine is a piperazine derivative that selectively inhibits the late sodium current and reduces intracellular calcium in cardiomyocytes, leading to improved ventricular relaxation and facilitating microvascular function (117). Small RCT’s have demonstrated varied efficacy, though patient stratification via invasive CFR may identify a beneficial population for this agent (118, 119). Fasudil is a specific Rho-kinase inhibitor highly effective in preventing ACh-induced coronary spasm (120, 121). The Rho-kinase pathway is involved in endothelial dysfunction, vasospasm and inflammatory cell accumulation. Fasudil can target these pathogenic mechanisms leading to a reduction in myocardial ischemia and symptoms of angina (120, 121).
Fortunately, multiple randomized controlled trials are underway to elucidate specific therapies for CMD. For example, the WARRIOR trial (Women’s Ischemia Treatment Reduces Events In Non-Obstructive CAD–NCT03417388) is a prospective, randomized trial evaluating intensive medical therapy (high-intensity statin, ACE-Is or ARBs, and aspirin) vs. usual care in 4,422 symptomatic women with INOCA (122). Furthermore, a randomized controlled trial (RCT) assessing Zibotentan (NCT04097314), a potent and selective antagonist of Endothelin-A, in MVA is near completion (123). As previously mentioned, CMVO is an essential predictor of left ventricular remodeling, HF, and death in patients with STEMI. The RESTORE-MI (Restoring Microcirculatory Perfusion in STEMI) trial is currently recruiting patients with STEMI and objective evidence of microvascular dysfunction (IMR value > 32) following reperfusion. This multi-center RCT assesses the efficacy of intracoronary tenecteplase, a fibrin specific thrombolytic, as a novel therapy for these patients.
Sodium-glucose Cotransport-2 Inhibitors (SGLT2i) is another drug class that may show hope for patients with CMD. Empagliflozin was shown to restore the beneficial effect of cardiac microvascular endothelial cells on cardiomyocyte function and demonstrate an improvement in CFVR in a prediabetic mouse model (124). Furthermore, the positive results of both the EMPEROR-PRESERVED (Empagliflozin Outcome Trial in Patients with Chronic Health Failure with Preserved Ejection Fraction) and DELIVER (Dapagloflozin Evaluation to Improve the LIVEs of Patients with Preserved Ejection Fraction Heart Failure) trials highlight the need for further studies on a homogenous patient population with CMD (125, 126).
Concentrating on MINOCA, current prospective trials focus on secondary preventive treatments and precision therapy. The MINOCA-BAT (Randomized Evaluation of Beta Blocker and ACEI/ARB Treatment in MINOCA Patients) trial aims to determine whether beta-blocker and/or ACE-I/ARBs reduce the composite end-point of all-cause mortality, readmission for MI, ischemic stroke or heart failure (NCTO3686696) (127). Likewise, the StratMEd-MINOCA (Stratified Medicine of Eplerenone in Acute MI/Injury) trial will assess if a stratified medical approach with early risk stratification by CMD (defined as IMR ≥ 25) coupled with mineralocorticoid antagonist therapy will limit myocardial damage (NCT05198791). Lastly, the PROMISE trial (Prognostic Value of Precision Medicine in Patients With MINOCA) will evaluate whether a precision-medicine approach with a specific therapy tailored to the underlying pathogenic mechanism will improve the quality of life in MINOCA patients (128).
Summary and perspectives
Coronary microvascular dysfunction is prevalent across various cardiac conditions and characterized by structural and functional abnormalities of the coronary microcirculation. Its pathogenic role in myocardial ischemia contributes to clinical manifestations across these disorders and is associated with an adverse prognosis. Notably, the presence of CMD is often detectable prior to clinical symptoms and may support the potential for early risk stratification and treatment. Unfortunately, despite significant advances over the past decade, clinicians do not have a specific agent for treating this condition. Clarifying the mechanistic role of CMD across clinical phenotypes and identification of novel therapeutic targets requires further research. In line with ongoing RCTs, developing personalized treatments across this heterogenous cohort is the next challenge in cardiovascular medicine.
Author contributions
RR, AY, JW, and RP: conceptualization. RR and RP: methodology. RR: investigation, resources, and writing—original draft preparation. RR, AY, MN, JW, and RP: writing—review and editing. JW and RP: supervision. All authors have read and agreed to the published version of the manuscript and fulfill relevant criteria for authorship.
Conflict of interest
AY was received honoraria and research support from Abbott Vascular and Philips Healthcare. MN was received research support from Abbott Vascular.
The remaining authors declare that the research was conducted in the absence of any commercial or financial relationships that could be construed as a potential conflict of interest.
Publisher’s note
All claims expressed in this article are solely those of the authors and do not necessarily represent those of their affiliated organizations, or those of the publisher, the editors and the reviewers. Any product that may be evaluated in this article, or claim that may be made by its manufacturer, is not guaranteed or endorsed by the publisher.
References
1. Radico F, Zimarino M, Fulgenzi F, Ricci F, Di Nicola M, Jespersen L, et al. Determinants of long-term clinical outcomes in patients with angina but without obstructive coronary artery disease: a systematic review and meta-analysis. Eur Heart J. (2018) 39:2135–46. doi: 10.1093/eurheartj/ehy185
2. Del Buono M, Montone R, Camilli M, Carbone S, Narula J, Lavie C, et al. Coronary microvascular dysfunction across the spectrum of cardiovascular diseases: JACC state-of-the-art review. J Am Coll Cardiol. (2021) 78:1352–71. doi: 10.1016/j.jacc.2021.07.042
3. Komaru T, Kanatsuka H, Shirato K. Coronary microcirculation: physiology and pharmacology. Pharmacol Ther. (2000) 86:217–61. doi: 10.1016/S0163-7258(00)00057-7
4. Camici P, Crea F. Coronary microvascular dysfunction. N Engl J Med. (2007) 356:830–40. doi: 10.1056/NEJMra061889
5. Kuo L, Chilian W, Davis M. Coronary arteriolar myogenic response is independent of endothelium. Circ Res. (1990) 66:860–6. doi: 10.1161/01.RES.66.3.860
6. Pries A, Badimon L, Bugiardini R, Camici P, Dorobantu M, Duncker D, et al. Coronary vascular regulation, remodelling, and collateralization: mechanisms and clinical implications on behalf of the working group on coronary pathophysiology and microcirculation. Eur Heart J. (2015) 36:3134–46. doi: 10.1093/eurheartj/ehv100
7. Kaski J, Crea F, Gersh B, Camici P. Reappraisal of ischemic heart disease: fundamental role of coronary microvascular dysfunction in the pathogenesis of angina pectoris. Circulation. (2018) 138:1463–80. doi: 10.1161/CIRCULATIONAHA.118.031373
8. Tsai S, Lu G, Xu X, Ren Y, Hein T, Kuo L. Enhanced endothelin-1/Rho-kinase signalling and coronary microvascular dysfunction in hypertensive myocardial hypertrophy. Cardiovasc Res. (2017) 113:1329–37. doi: 10.1093/cvr/cvx103
9. Crea F, Camici P, Bairey Merz C. Coronary microvascular dysfunction: an update. Eur Heart J. (2014) 35:1101–11. doi: 10.1093/eurheartj/eht513
10. Takahashi J, Nihei T, Takagi Y, Miyata S, Odaka Y, Tsunoda R, et al. Prognostic impact of chronic nitrate therapy in patients with vasospastic angina: multicentre registry study of the Japanese coronary spasm association. Eur Heart J. (2014) 36:228–37. doi: 10.1093/eurheartj/ehu313
11. Haddock R, Hill C. Rhythmicity in arterial smooth muscle. J Physiol. (2005) 566(Pt 3):645–56. doi: 10.1113/jphysiol.2005.086405
12. Nakano T, Osanai T, Tomita H, Sekimata M, Homma Y, Okumura K. Enhanced activity of variant phospholipase C-delta1 protein (R257H) detected in patients with coronary artery spasm. Circulation. (2002) 105:2024–9. doi: 10.1161/01.CIR.0000014613.36469.3F
13. Kakkar R, Ye B, Stoller D, Smelley M, Shi N, Galles K, et al. Spontaneous coronary vasospasm in KATP mutant mice arises from a smooth muscle-extrinsic process. Circ Res. (2006) 98:682–9. doi: 10.1161/01.RES.0000207498.40005.e7
14. Büssemaker E, Pistrosch F, Förster S, Herbrig K, Gross P, Passauer J, et al. Rho kinase contributes to basal vascular tone in humans: role of endothelium-derived nitric oxide. Am J Physiol Heart Circ Physiol. (2007) 293:H541–7. doi: 10.1152/ajpheart.00770.2006
15. Lanza G, Careri G, Crea F. Mechanisms of coronary artery spasm. Circulation. (2011) 124:1774–82. doi: 10.1161/CIRCULATIONAHA.111.037283
16. Yasue H, Hirai N, Mizuno Y, Harada E, Itoh T, Yoshimura M, et al. Low-grade inflammation, thrombogenicity, and atherogenic lipid profile in cigarette smokers. Circ J. (2006) 70:8–13. doi: 10.1253/circj.70.8
17. Takaoka K, Yoshimura M, Ogawa H, Kugiyama K, Nakayama M, Shimasaki Y, et al. Comparison of the risk factors for coronary artery spasm with those for organic stenosis in a Japanese population: role of cigarette smoking. Int J Cardiol. (2000) 72:121–6. doi: 10.1016/S0167-5273(99)00172-2
18. Camici P, Tschöpe C, Di Carli M, Rimoldi O, Van Linthout S. Coronary microvascular dysfunction in hypertrophy and heart failure. Cardiovasc Res. (2020) 116:806–16. doi: 10.1093/cvr/cvaa023
19. Knuuti J, Wijns W, Saraste A, Capodanno D, Barbato E, Funck-Brentano C, et al. 2019 ESC Guidelines for the diagnosis and management of chronic coronary syndromes: the task force for the diagnosis and management of chronic coronary syndromes of the European Society of Cardiology (ESC). Eur Heart J. (2019) 41:407–77. doi: 10.15829/1560-4071-2020-2-3757
20. Ford T, Stanley B, Good R, Rocchiccioli P, McEntegart M, Watkins S, et al. Stratified medical therapy using invasive coronary function testing in angina: the CorMicA trial. J Am Coll Cardiol. (2018) 72(23 Pt A):2841–55. doi: 10.1016/j.jacc.2018.09.006
21. Gould K, Lipscomb K. Effects of coronary stenoses on coronary flow reserve and resistance. Am J Cardiol. (1974) 34:48–55. doi: 10.1016/0002-9149(74)90092-7
22. Ong P, Athanasiadis A, Sechtem U. Intracoronary acetylcholine provocation testing for assessment of coronary vasomotor disorders. J Vis Exp. (2016) doi: 10.3791/54295
23. Fearon W. Chapter 14 - Invasive testing. In: de Lemos J, Omland T editors. Chronic Coronary Artery Disease. Amsterdam: Elsevier (2018). p. 194–203. doi: 10.1016/B978-0-323-42880-4.00014-5
24. Ong P, Safdar B, Seitz A, Hubert A, Beltrame J, Prescott E. Diagnosis of coronary microvascular dysfunction in the clinic. Cardiovasc Res. (2020) 116:841–55. doi: 10.1093/cvr/cvz339
25. Fearon W, Farouque H, Balsam L, Caffarelli A, Cooke D, Robbins R, et al. Comparison of coronary thermodilution and doppler velocity for assessing coronary flow reserve. Circulation. (2003) 108:2198–200. doi: 10.1161/01.CIR.0000099521.31396.9D
26. Vink C, van de Hoef T, Götte M, Eringa E, Appelman Y. Reduced microvascular blood volume as a driver of coronary microvascular disease in patients with non-obstructive coronary artery disease: rationale and design of the MICORDIS study. Front Cardiovasc Med. (2021) 8:730810. doi: 10.3389/fcvm.2021.730810
27. Ng M, Yeung A, Fearon W. Invasive assessment of the coronary microcirculation. Circulation. (2006) 113:2054–61. doi: 10.1161/CIRCULATIONAHA.105.603522
28. Kern M. Coronary physiology revisited : practical insights from the cardiac catheterization laboratory. Circulation. (2000) 101:1344–51. doi: 10.1161/01.CIR.101.11.1344
29. Vanhoutte P. Endothelium and control of vascular function. State of the art lecture. Hypertension. (1989) 13(6 Pt 2):658–67. doi: 10.1161/01.HYP.13.6.658
30. Sandoo A, van Zanten J, Metsios G, Carroll D, Kitas G. The endothelium and its role in regulating vascular tone. Open Cardiovasc Med J. (2010) 4:302–12. doi: 10.2174/1874192401004010302
31. Ong P, Athanasiadis A, Borgulya G, Vokshi I, Bastiaenen R, Kubik S, et al. Clinical usefulness, angiographic characteristics, and safety evaluation of intracoronary acetylcholine provocation testing among 921 consecutive white patients with unobstructed coronary arteries. Circulation. (2014) 129:1723–30. doi: 10.1161/CIRCULATIONAHA.113.004096
32. Reriani M, Sara J, Flammer A, Gulati R, Li J, Rihal C, et al. Coronary endothelial function testing provides superior discrimination compared with standard clinical risk scoring in prediction of cardiovascular events. Coron Artery Dis. (2016) 27:213–20. doi: 10.1097/MCA.0000000000000347
33. Isogai T, Yasunaga H, Matsui H, Tanaka H, Ueda T, Horiguchi H, et al. Serious cardiac complications in coronary spasm provocation tests using acetylcholine or ergonovine: analysis of 21 512 patients from the diagnosis procedure combination database in Japan. Clin Cardiol. (2015) 38:171–7. doi: 10.1002/clc.22369
34. Ciliberti G, Seshasai S, Ambrosio G, Kaski J. Safety of intracoronary provocative testing for the diagnosis of coronary artery spasm. Int J Cardiol. (2017) 244:77–83. doi: 10.1016/j.ijcard.2017.05.109
35. Montone R, Rinaldi R, Del Buono M, Gurgoglione F, La Vecchia G, Russo M, et al. Safety and prognostic relevance of acetylcholine testing in patients with stable myocardial ischaemia or myocardial infarction and non-obstructive coronary arteries. Eurointervention. (2022) 18:e666–76. doi: 10.4244/EIJ-D-21-00971
36. Vegsundvåg J, Holte E, Wiseth R, Hegbom K, Hole T. Coronary flow velocity reserve in the three main coronary arteries assessed with transthoracic doppler: a comparative study with quantitative coronary angiography. J Am Soc Echocardiogr. (2011) 24:758–67. doi: 10.1016/j.echo.2011.03.010
37. Vogel R, Indermühle A, Reinhardt J, Meier P, Siegrist P, Namdar M, et al. The quantification of absolute myocardial perfusion in humans by contrast echocardiography: algorithm and validation. J Am Coll Cardiol. (2005) 45:754–62. doi: 10.1016/j.jacc.2004.11.044
38. Schindler T, Schelbert H, Quercioli A, Dilsizian V. Cardiac PET imaging for the detection and monitoring of coronary artery disease and microvascular health. JACC Cardiovasc Imaging. (2010) 3:623–40. doi: 10.1016/j.jcmg.2010.04.007
39. Leung D, Leung M. Non-invasive/invasive imaging: significance and assessment of coronary microvascular dysfunction. Heart. (2011) 97:587–95. doi: 10.1136/hrt.2009.183327
40. Morton G, Chiribiri A, Ishida M, Hussain S, Schuster A, Indermuehle A, et al. Quantification of absolute myocardial perfusion in patients with coronary artery disease: comparison between cardiovascular magnetic resonance and positron emission tomography. J Am Coll Cardiol. (2012) 60:1546–55. doi: 10.1016/S0735-1097(12)61065-1
41. Bax M, de Winter R, Schotborgh C, Koch K, Meuwissen M, Voskuil M, et al. Short-and long-term recovery of left ventricular function predicted at the time of primary percutaneous coronary intervention in anterior myocardial infarction. J Am Coll Cardiol. (2004) 43:534–41. doi: 10.1016/j.jacc.2003.08.055
42. Henriques J, Zijlstra F, van ‘t Hof A, de Boer M, Dambrink J, Gosselink M, et al. Angiographic assessment of reperfusion in acute myocardial infarction by myocardial blush grade. Circulation. (2003) 107:2115–9. doi: 10.1161/01.CIR.0000065221.06430.ED
43. Ndrepepa G, Tiroch K, Fusaro M, Keta D, Seyfarth M, Byrne R, et al. 5-year prognostic value of no-reflow phenomenon after percutaneous coronary intervention in patients with acute myocardial infarction. J Am Coll Cardiol. (2010) 55:2383–9. doi: 10.1016/j.jacc.2009.12.054
44. Niccoli G, Scalone G, Lerman A, Crea F. Coronary microvascular obstruction in acute myocardial infarction. Eur Heart J. (2015) 37:1024–33. doi: 10.1093/eurheartj/ehv484
45. Heusch G, Kleinbongard P, Böse D, Levkau B, Haude M, Schulz R, et al. Coronary microembolization: from bedside to bench and back to bedside. Circulation. (2009) 120:1822–36. doi: 10.1161/CIRCULATIONAHA.109.888784
46. Iwakura K, Ito H, Kawano S, Okamura A, Tanaka K, Nishida Y, et al. Prediction of the no-reflow phenomenon with ultrasonic tissue characterization in patients with anterior wall acute myocardial infarction. Am J Cardiol. (2004) 93:1357–61,A5. doi: 10.1016/j.amjcard.2004.02.030
47. Yellon D, Hausenloy D. Myocardial reperfusion injury. N Engl J Med. (2007) 357:1121–35. doi: 10.1056/NEJMra071667
48. Betgem R, De Waard G, Nijveldt R, Beek A, Escaned J, Van Royen N. Intramyocardial haemorrhage after acute myocardial infarction. Nat Rev Cardiol. (2015) 12:156–67. doi: 10.1038/nrcardio.2014.188
49. Vignali L, Talanas G, Saia F, Menozzi A, Cattabiani M, Solinas E, et al. Genetic association between the 1976T_C polymorphism in the adenosine A2 receptor and angiographic no-reflow phenomenon (abstr). Il Giornale Ital Cardiol Invasiva. (2007) 3(Suppl. 1):109.
50. Wang X, Liu H, Wu H, Xiao Y, Bai S, Li X, et al. Safety and efficacy of intracoronary prourokinase administration in patients with high thrombus burden. Coronary Artery Dis. (2020) 31:493–9. doi: 10.1097/MCA.0000000000000853
51. Pelliccia F, Greco C, Tanzilli G, Viceconte N, Schiariti M, Gaudio C. Long-term outcome of patients with ST-segment elevation myocardial infarction treated with low-dose intracoronary thrombolysis during primary percutaneous coronary intervention: the 5-year results of the DISSOLUTION trial. J Thromb Thrombolys. (2021) 51:212–6. doi: 10.1007/s11239-020-02157-w
52. Jiang W, Xiong X, Du X, Ma H, Li W, Cheng F. Safety and efficacy study of prourokinase injection during primary percutaneous coronary intervention in acute ST-segment elevation myocardial infarction. Coronary Artery Dis. (2021) 32:25–30. doi: 10.1097/MCA.0000000000000898
53. Huang D, Qian J, Liu Z, Xu Y, Zhao X, Qiao Z, et al. Effects of intracoronary pro-urokinase or tirofiban on coronary flow during primary percutaneous coronary intervention for acute myocardial infarction: a multi-center, placebo-controlled, single-blind, randomized clinical trial. Front Cardiovasc Med. (2021) 8:710994. doi: 10.3389/fcvm.2021.710994
54. Chen L, Shi L, Tian W, Zhao S. Intracoronary thrombolysis in patients with ST-segment elevation myocardial infarction: a meta-analysis of randomized controlled trials. Angiology. (2021) 72:679–86. doi: 10.1177/0003319721995039
55. Alyamani M, Campbell S, Navarese E, Welsh R, Bainey K. Safety and efficacy of intracoronary thrombolysis as adjunctive therapy to primary PCI in STEMI. A systematic review and meta-analysis. Can J Cardiol. (2020) 37:339–46. doi: 10.1016/j.cjca.2020.03.034
56. Duncker D, Koller A, Merkus D, Canty J Jr. Regulation of coronary blood flow in health and ischemic heart disease. Prog Cardiovasc Dis. (2015) 57:409–22. doi: 10.1016/j.pcad.2014.12.002
57. Taqueti V, Di Carli M. Coronary microvascular disease pathogenic mechanisms and therapeutic options: JACC state-of-the-art review. J Am Coll Cardiol. (2018) 72:2625–41. doi: 10.1016/j.jacc.2018.09.042
58. Gupta A, Taqueti V, van de Hoef T, Bajaj N, Bravo P, Murthy V, et al. Integrated noninvasive physiological assessment of coronary circulatory function and impact on cardiovascular mortality in patients with stable coronary artery disease. Circulation. (2017) 136:2325–36. doi: 10.1161/CIRCULATIONAHA.117.029992
59. Sechtem U, Brown D, Godo S, Lanza G, Shimokawa H, Sidik N. Coronary microvascular dysfunction in stable ischaemic heart disease (non-obstructive coronary artery disease and obstructive coronary artery disease). Cardiovasc Res. (2020) 116:771–86. doi: 10.1093/cvr/cvaa005
60. Stenström I, Maaniitty T, Uusitalo V, Pietilä M, Ukkonen H, Kajander S, et al. Frequency and angiographic characteristics of coronary microvascular dysfunction in stable angina: a hybrid imaging study. Eur Hear J Cardiovasc Imaging. (2017) 18:1206–13. doi: 10.1093/ehjci/jex193
61. van de Hoef T, Bax M, Damman P, Delewi R, Hassell M, Piek M, et al. Impaired coronary autoregulation is associated with long-term fatal events in patients with stable coronary artery disease. Circ Cardiovasc Interv. (2013) 6:329–35. doi: 10.1161/CIRCINTERVENTIONS.113.000378
62. Cortigiani L, Rigo F, Gherardi S, Galderisi M, Bovenzi F, Picano E, et al. Prognostic effect of coronary flow reserve in women versus men with chest pain syndrome and normal dipyridamole stress echocardiography. Am J Cardiol. (2010) 106:1703–8. doi: 10.1016/j.amjcard.2010.08.011
63. Taqueti V, Hachamovitch R, Murthy V, Naya M, Foster C, Hainer J, et al. Global coronary flow reserve is associated with adverse cardiovascular events independently of luminal angiographic severity and modifies the effect of early revascularization. Circulation. (2015) 131:19–27. doi: 10.1161/CIRCULATIONAHA.114.011939
64. Bolognese L, Carrabba N, Parodi G, Santoro G, Buonamici P, Cerisano G, et al. Impact of microvascular dysfunction on left ventricular remodeling and long-term clinical outcome after primary coronary angioplasty for acute myocardial infarction. Circulation. (2004) 109:1121–6. doi: 10.1161/01.CIR.0000118496.44135.A7
65. Kern M, Dupouy P, Drury J, Aguirre F, Aptecar E, Bach R, et al. Role of coronary artery lumen enlargement in improving coronary blood flow after balloon angioplasty and stenting: a combined intravascular ultrasound doppler flow and imaging study. J Am Coll Cardiol. (1997) 29:1520–7. doi: 10.1016/S0735-1097(97)00082-X
66. Harari E, Guo L, Smith S, Paek K, Fernandez R, Sakamoto A, et al. Direct targeting of the mTOR (Mammalian Target of Rapamycin) kinase improves endothelial permeability in drug-eluting stents-brief report. Arterioscler Thromb Vasc Biol. (2018) 38:2217–24. doi: 10.1161/ATVBAHA.118.311321
67. Nishi T, Murai T, Ciccarelli G, Shah S, Kobayashi Y, Derimay F, et al. Prognostic value of coronary microvascular function measured immediately after percutaneous coronary intervention in stable coronary artery disease: an international multicenter study. Circ Cardiovasc Interv. (2019) 12:e007889. doi: 10.1161/CIRCINTERVENTIONS.119.007889
68. Li Y, Yang D, Lu L, Wu D, Yao J, Hu X, et al. Thermodilutional confirmation of coronary microvascular dysfunction in patients with recurrent angina after successful percutaneous coronary intervention. Can J Cardiol. (2015) 31:989–97. doi: 10.1016/j.cjca.2015.03.004
69. Milo M, Nerla R, Tarzia P, Infusino F, Battipaglia I, Sestito A, et al. Coronary microvascular dysfunction after elective percutaneous coronary intervention: correlation with exercise stress test results. Int J Cardiol. (2013) 168:121–5. doi: 10.1016/j.ijcard.2012.09.059
70. Reis S, Holubkov R, Smith A, Kelsey S, Sharaf B, Reichek N, et al. Coronary microvascular dysfunction is highly prevalent in women with chest pain in the absence of coronary artery disease: results from the NHLBI WISE study. Am Heart J. (2001) 141:735–41. doi: 10.1067/mhj.2001.114198
71. Kunadian V, Chieffo A, Camici P, Berry C, Escaned J, Maas A, et al. An EAPCI Expert consensus document on ischaemia with non-obstructive coronary arteries in collaboration with European society of cardiology working group on coronary pathophysiology & microcirculation endorsed by coronary vasomotor disorders international study group. Eur Heart J. (2020) 41:ehaa503. doi: 10.1093/eurheartj/ehaa503
72. Suda A, Takahashi J, Hao K, Kikuchi Y, Shindo T, Ikeda S, et al. Coronary functional abnormalities in patients with angina and nonobstructive coronary artery disease. J Am Coll Cardiol. (2019) 74:2350–60. doi: 10.1016/j.jacc.2019.08.1056
73. Kenkre T, Malhotra P, Johnson B, Handberg E, Thompson D, Marroquin O, et al. Ten-year mortality in the WISE study (Women’s Ischemia Syndrome Evaluation). Circ Cardiovasc Qual Outcomes. (2017) 10:e003863. doi: 10.1161/CIRCOUTCOMES.116.003863
74. Balázs E, Pintér K, Egyed Á, Csanády M, Forster T, Nemes A. The independent long-term prognostic value of coronary flow velocity reserve in female patients with chest pain and negative coronary angiograms (results from the SZEGED study). Int J Cardiol. (2011) 146:259–61. doi: 10.1016/j.ijcard.2010.10.071
75. Murthy V, Naya M, Taqueti V, Foster C, Gaber M, Hainer J, et al. Effects of sex on coronary microvascular dysfunction and cardiac outcomes. Circulation. (2014) 129:2518–27. doi: 10.1161/CIRCULATIONAHA.113.008507
76. Tamis-Holland J, Jneid H, Reynolds H, Agewall S, Brilakis E, Brown T, et al. Contemporary diagnosis and management of patients with myocardial infarction in the absence of obstructive coronary artery disease: a scientific statement from the American heart association. Circulation. (2019) 139:e891–908. doi: 10.1161/CIR.0000000000000670
77. Pasupathy S, Air T, Dreyer R, Tavella R, Beltrame J. Systematic review of patients presenting with suspected myocardial infarction and nonobstructive coronary arteries. Circulation. (2015) 131:861–70. doi: 10.1161/CIRCULATIONAHA.114.011201
78. Choo E, Chang K, Lee K, Lee D, Kim J, Ahn Y, et al. Prognosis and predictors of mortality in patients suffering myocardial infarction with non-obstructive coronary arteries. J Am Heart Assoc. (2019) 8:e011990. doi: 10.1161/JAHA.119.011990
79. Vancheri F, Longo G, Vancheri S, Henein M. Coronary microvascular dysfunction. J Clin Med. (2020) 9:2880. doi: 10.3390/jcm9092880
80. Ai H, Feng Y, Gong Y, Zheng B, Jin Q, Zhang H, et al. Coronary angiography-derived index of microvascular resistance. Front Physiol. (2020) 11:605356. doi: 10.3389/fphys.2020.605356
81. Mauricio R, Srichai M, Axel L, Hochman J, Reynolds H. Stress cardiac MRI in women with myocardial infarction and nonobstructive coronary artery disease. Clin Cardiol. (2016) 39:596–602. doi: 10.1002/clc.22571
82. Elliott P, Andersson B, Arbustini E, Bilinska Z, Cecchi F, Charron P, et al. Classification of the cardiomyopathies: a position statement from the European society of cardiology working group on myocardial and pericardial diseases. Eur Heart J. (2007) 29:270–6. doi: 10.1093/eurheartj/ehm342
83. Neglia D, L’Abbate A. Coronary microvascular dysfunction and idiopathic dilated cardiomyopathy. Pharmacol Rep. (2005) 57:151–5.
84. Schwartzkopff B, Mundhenke M, Strauer B. Alterations of the architecture of subendocardial arterioles in patients with hypertrophic cardiomyopathy and impaired coronary vasodilator reserve: a possible cause for myocardial ischemia. J Am Coll Cardiol. (1998) 31:1089–96. doi: 10.1016/S0735-1097(98)00036-9
85. Schulz E, Jansen T, Wenzel P, Daiber A, Münzel T. Nitric oxide, tetrahydrobiopterin, oxidative stress, and endothelial dysfunction in hypertension. Antioxid Redox Sign. (2008) 10:1115–26. doi: 10.1089/ars.2007.1989
86. Redfield M. Understanding “diastolic” heart failure. N Engl J Med. (2004) 350:1930–1. doi: 10.1056/NEJMp048064
87. Shah S, Lam C, Svedlund S, Saraste A, Hage C, Tan R, et al. Prevalence and correlates of coronary microvascular dysfunction in heart failure with preserved ejection fraction: PROMIS-HFpEF. Eur Heart J. (2018) 39:3439–50. doi: 10.1093/eurheartj/ehy531
88. Rush C, Berry C, Oldroyd K, Rocchiccioli J, Lindsay M, Touyz R, et al. Prevalence of coronary artery disease and coronary microvascular dysfunction in patients with heart failure with preserved ejection fraction. JAMA Cardiol. (2021) 6:1130–43. doi: 10.1001/jamacardio.2021.1825
89. Dryer K, Gajjar M, Narang N, Lee M, Paul J, Shah A, et al. Coronary microvascular dysfunction in patients with heart failure with preserved ejection fraction. Am J Physiol Heart Circ Physiol. (2018) 314:H1033–42. doi: 10.1152/ajpheart.00680.2017
90. Taqueti V, Solomon S, Shah A, Desai A, Groarke J, Osborne M, et al. Coronary microvascular dysfunction and future risk of heart failure with preserved ejection fraction. Eur Heart J. (2018) 39:840–9. doi: 10.1093/eurheartj/ehx721
91. Ghadri J, Wittstein I, Prasad A, Sharkey S, Dote K, Akashi Y, et al. International expert consensus document on takotsubo syndrome (Part I): clinical characteristics, diagnostic criteria, and pathophysiology. Eur Heart J. (2018) 39:2032–46. doi: 10.1093/eurheartj/ehy076
92. Abdelmoneim S, Mankad S, Bernier M, Dhoble A, Hagen M, Ness S, et al. Microvascular function in Takotsubo cardiomyopathy with contrast echocardiography: prospective evaluation and review of literature. J Am Soc Echocardiogr. (2009) 22:1249–55. doi: 10.1016/j.echo.2009.07.012
93. Cimarelli S, Sauer F, Morel O, Ohlmann P, Constantinesco A, Imperiale A. Transient left ventricular dysfunction syndrome: patho-physiological bases through nuclear medicine imaging. Int J Cardiol. (2010) 144:212–8. doi: 10.1016/j.ijcard.2009.04.025
94. Ito K, Sugihara H, Katoh S, Azuma A, Nakagawa M. Assessment of Takotsubo (ampulla) cardiomyopathy using 99mTc-tetrofosmin myocardial SPECT–comparison with acute coronary syndrome. Ann Nucl Med. (2003) 17:115–22. doi: 10.1007/BF02988449
95. Daniels D, Fearon W. The index of microcirculatory resistance (IMR) in takotsubo cardiomyopathy. Catheter Cardiovasc Interv. (2011) 77:128–31. doi: 10.1002/ccd.22599
96. Warisawa T, Naganuma T, Nakamura S. Reversible microvascular dysfunction in takotsubo syndrome shown using index of microcirculatory resistance. Circ J. (2016) 80:750–2. doi: 10.1253/circj.CJ-15-1283
97. Cuisset T, Quilici J, Pankert M, Fourcade L, Poyet R, Lambert M, et al. Usefulness of index of microcirculatory resistance to detect microvascular dysfunction as a potential mechanism of stress-induced cardiomyopathy (Tako-tsubo syndrome). Int J Cardiol. (2011) 153:e51–3. doi: 10.1016/j.ijcard.2011.02.028
98. Montone R, Galiuto L, Meucci M, Del Buono M, Vergni F, Camilli M, et al. Coronary slow flow is associated with a worse clinical outcome in patients with Takotsubo syndrome. Heart. (2020) 106:923–30. doi: 10.1136/heartjnl-2019-315909
99. Ariga R, Tunnicliffe E, Manohar S, Mahmod M, Raman B, Piechnik S, et al. Identification of myocardial disarray in patients with hypertrophic cardiomyopathy and ventricular arrhythmias. J Am Coll Cardiol. (2019) 73:2493–502. doi: 10.1016/j.jacc.2019.02.065
100. Maron M, Olivotto I, Maron B, Prasad S, Cecchi F, Udelson J, et al. The case for myocardial ischemia in hypertrophic cardiomyopathy. J Am Coll Cardiol. (2009) 54:866–75. doi: 10.1016/j.jacc.2009.04.072
101. Camici P, Chiriatti G, Lorenzoni R, Bellina R, Gistri R, Italiani G, et al. Coronary vasodilation is impaired in both hypertrophied and nonhypertrophied myocardium of patients with hypertrophic cardiomyopathy: a study with nitrogen-13 ammonia and positron emission tomography. J Am Coll Cardiol. (1991) 17:879–86. doi: 10.1016/0735-1097(91)90869-B
102. Cecchi F, Olivotto I, Gistri R, Lorenzoni R, Chiriatti G, Camici P. Coronary microvascular dysfunction and prognosis in hypertrophic cardiomyopathy. N Engl J Med. (2003) 349:1027–35. doi: 10.1056/NEJMoa025050
103. Kalliokoski R, Kalliokoski K, Sundell J, Engblom E, Penttinen M, Kantola I, et al. Impaired myocardial perfusion reserve but preserved peripheral endothelial function in patients with Fabry disease. J Inherit Metab Dis. (2005) 28:563–73. doi: 10.1007/s10545-005-0563-2
104. Suwaidi J, Velianou J, Gertz M, Cannon R, Higano S, Holmes D, et al. Systemic amyloidosis presenting with angina pectoris. Ann Intern Med. (1999) 131:838–41. doi: 10.7326/0003-4819-131-11-199912070-00007
105. Kruse M, Kovell L, Kasper E, Pomper M, Moller D, Solnes L, et al. Myocardial blood flow and inflammatory cardiac sarcoidosis. JACC Cardiovasc Imaging. (2017) 10:157–67.
106. Tomberli B, Cecchi F, Sciagrà R, Berti V, Lisi F, Torricelli F, et al. Coronary microvascular dysfunction is an early feature of cardiac involvement in patients with Anderson-Fabry disease. Eur J Heart Fail. (2013) 15:1363–73. doi: 10.1093/eurjhf/hft104
107. Hambrecht R, Gielen S, Linke A, Fiehn E, Yu J, Walther C, et al. Effects of exercise training on left ventricular function and peripheral resistance in patients with chronic heart failure: a randomized trial. JAMA. (2000) 283:3095–101. doi: 10.1001/jama.283.23.3095
108. Rooks C, Faber T, Votaw J, Veledar E, Goldberg J, Raggi P, et al. Effects of smoking on coronary microcirculatory function: a twin study. Atherosclerosis. (2011) 215:500–6. doi: 10.1016/j.atherosclerosis.2011.01.012
109. Olsen R, Pedersen L, Jürs A, Snoer M, Haugaard S, Prescott E. A randomised trial comparing the effect of exercise training and weight loss on microvascular function in coronary artery disease. Int J Cardiol. (2015) 185:229–35. doi: 10.1016/j.ijcard.2015.03.118
110. Carbone S, Del Buono M, Ozemek C, Lavie C. Obesity, risk of diabetes and role of physical activity, exercise training and cardiorespiratory fitness. Prog Cardiovasc Dis. (2019) 62:327–33. doi: 10.1016/j.pcad.2019.08.004
111. Camilli M, Russo M, Rinaldi R, Caffè A, La Vecchia G, Bonanni A, et al. Air pollution and coronary vasomotor disorders in patients with myocardial ischemia and unobstructed coronary arteries. J Am Coll Cardiol. (2022) 80:1818–28. doi: 10.1016/j.jacc.2022.08.744
112. Mancini G, Henry G, Macaya C, O’Neill B, Pucillo A, Carere R, et al. Angiotensin-converting enzyme inhibition with quinapril improves endothelial vasomotor dysfunction in patients with coronary artery disease: the TREND (Trial on Reversing ENdothelial Dysfunction) study. Circulation. (1996) 94:258–65. doi: 10.1161/01.CIR.94.3.258
113. Li S, Wu Y, Yu G, Xia Q, Xu Y. Angiotensin II receptor blockers improve peripheral endothelial function: a meta-analysis of randomized controlled trials. PLoS One. (2014) 9:e90217. doi: 10.1371/journal.pone.0090217
114. Eshtehardi P, McDaniel M, Dhawan S, Binongo J, Krishnan S, Golub L, et al. Effect of intensive atorvastatin therapy on coronary atherosclerosis progression, composition, arterial remodeling, and microvascular function. J Invasive Cardiol. (2012) 24:522–9.
115. Lanza G, Crea F. Primary coronary microvascular dysfunction: clinical presentation, pathophysiology, and management. Circulation. (2010) 121:2317–25. doi: 10.1161/CIRCULATIONAHA.109.900191
116. Ong P, Athanasiadis A, Sechtem U. Pharmacotherapy for coronary microvascular dysfunction. Eur Heart J Cardiovasc Pharmacother. (2015) 1:65–71. doi: 10.1093/ehjcvp/pvu020
117. Hasenfuss G, Maier L. Mechanism of action of the new anti-ischemia drug ranolazine. Clin Res Cardiol. (2008) 97:222–6. doi: 10.1007/s00392-007-0612-y
118. Mehta P, Goykhman P, Thomson L, Shufelt C, Wei J, Yang Y, et al. Ranolazine improves angina in women with evidence of myocardial ischemia but no obstructive coronary artery disease. JACC Cardiovasc Imaging. (2011) 4:514–22. doi: 10.1016/j.jcmg.2011.03.007
119. Villano A, Di Franco A, Nerla R, Sestito A, Tarzia P, Lamendola P, et al. Effects of ivabradine and ranolazine in patients with microvascular angina pectoris. Am J Cardiol. (2013) 112:8–13. doi: 10.1016/j.amjcard.2013.02.045
120. Mohri M, Shimokawa H, Hirakawa Y, Masumoto A, Takeshita A. Rho-kinase inhibition with intracoronary fasudil prevents myocardial ischemia in patients with coronary microvascular spasm. J Am Coll Cardiol. (2003) 41:15–9. doi: 10.1016/S0735-1097(02)02632-3
121. Masumoto A, Mohri M, Shimokawa H, Urakami L, Usui M, Takeshita A. Suppression of coronary artery spasm by the Rho-kinase inhibitor fasudil in patients with vasospastic angina. Circulation. (2002) 105:1545–7. doi: 10.1161/hc1002.105938
122. Handberg E, Merz C, Cooper-Dehoff R, Wei J, Conlon M, Lo M, et al. Rationale and design of the Women’s ischemia trial to reduce events in nonobstructive CAD (WARRIOR) trial. Am Heart J. (2021) 237:90–103. doi: 10.1016/j.ahj.2021.03.011
123. Morrow A, Ford T, Mangion K, Kotecha T, Rakhit R, Galasko G, et al. Rationale and design of the medical research council’s precision medicine with Zibotentan in Microvascular angina (PRIZE) trial. Am Heart J. (2020) 229:70–80. doi: 10.1016/j.ahj.2020.07.007
124. Adingupu D, Göpel S, Grönros J, Behrendt M, Sotak M, Miliotis T, et al. SGLT2 inhibition with empagliflozin improves coronary microvascular function and cardiac contractility in prediabetic ob/ob-/- mice. Cardiovasc Diabetol. (2019) 18:16. doi: 10.1186/s12933-019-0820-6
125. Solomon S, de Boer R, DeMets D, Hernandez A, Inzucchi S, Kosiborod M, et al. Dapagliflozin in heart failure with preserved and mildly reduced ejection fraction: rationale and design of the DELIVER trial. Eur J Heart Fail. (2021) 23:1217–25. doi: 10.1002/ejhf.2249
126. Anker S, Butler J, Filippatos G, Ferreira J, Bocchi E, Böhm M, et al. Empagliflozin in heart failure with a preserved ejection fraction. N Engl J Med. (2021) 385:1451–61.
127. Nordenskjöld A, Agewall S, Atar D, Baron T, Beltrame J, Bergström O, et al. Randomized evaluation of beta blocker and ACE-inhibitor/angiotensin receptor blocker treatment in patients with myocardial infarction with non-obstructive coronary arteries (MINOCA-BAT): rationale and design. Am Heart J. (2021) 231:96–104. doi: 10.1016/j.ahj.2020.10.059
128. Montone R, Cosentino N, Graziani F, Gorla R, Del Buono M, La Vecchia G, et al. Precision medicine versus standard of care for patients with myocardial infarction with non-obstructive coronary arteries (MINOCA): rationale and design of the multicentre, randomised PROMISE trial. Eurointervention. (2022) 18:e933–9. doi: 10.4244/EIJ-D-22-00178
Keywords: coronary microcirculation, microvascular angina, endothelial dysfunction, inflammation, INOCA
Citation: Rehan R, Yong A, Ng M, Weaver J and Puranik R (2023) Coronary microvascular dysfunction: A review of recent progress and clinical implications. Front. Cardiovasc. Med. 10:1111721. doi: 10.3389/fcvm.2023.1111721
Received: 30 November 2022; Accepted: 09 January 2023;
Published: 26 January 2023.
Edited by:
Giovanni Luigi De Maria, University of Oxford, United KingdomReviewed by:
Shmuel Banai, Tel Aviv Sourasky Medical Center, IsraelRocco Antonio Montone, Agostino Gemelli University Polyclinic (IRCCS), Italy
Copyright © 2023 Rehan, Yong, Ng, Weaver and Puranik. This is an open-access article distributed under the terms of the Creative Commons Attribution License (CC BY). The use, distribution or reproduction in other forums is permitted, provided the original author(s) and the copyright owner(s) are credited and that the original publication in this journal is cited, in accordance with accepted academic practice. No use, distribution or reproduction is permitted which does not comply with these terms.
*Correspondence: Rajesh Puranik, cmFqLnB1cmFuaWtAY21ycy5vcmcuYXU=