- 1Department of Cardiology, University Hospitals Leuven, Leuven, Belgium
- 2Department of Cardiovascular Sciences, KU Leuven, Leuven, Belgium
- 3Department of Cardiac Sciences, Cumming School of Medicine, Libin Cardiovascular Institute, University of Calgary, Calgary, AB, Canada
The autonomic nervous system plays a crucial role in atrial fibrillation pathophysiology. Parasympathetic hyperactivity result in a shortening of the action potential duration, a reduction of the conduction wavelength, and as such facilitates reentry in the presence of triggers. Further, autonomic remodeling of atrial myocytes in AF includes progressive sympathetic hyperinnervation by increased atrial sympathetic nerve density and sympathetic atrial nerve sprouting. Knowledge on the pathophysiological process in AF, including the contribution of the autonomic nervous system, may in the near future guide personalized AF management. This review focuses on the role of the autonomic nervous system in atrial fibrillation pathophysiology and non-invasive assessment of the autonomic nervous system.
1 Introduction
Atrial fibrillation (AF) is the most prevalent cardiac arrhythmia and is associated with a significant burden to patients and health care (1). While clinical research on AF pathophysiology is focusing mostly on structural and electrical remodeling, there has been significantly less attention for the role of the autonomic function. Increasing knowledge on the pathophysiological process in AF, including the contribution of the autonomic nervous system (ANS) and non-invasive substrate determination, may in the near future guide personalized AF management (1). In this review, we focus on the contribution of the ANS in AF pathophysiology, non-invasive assessment of the ANS, and briefly discuss the evidence and knowledge gaps in vagally-mediated AF.
2 Vagal and adrenergic atrial fibrillation subtypes
In 1978 Coumel et al. described an atrial arrhythmia syndrome of vagal origin (2). They reported on 18 cases, predominantly middle-aged men without underlying heart disease, who developed progressive deterioration of AF paroxysms precipitated by vagal overactivity. They noted a particular resistance to digitalis and beta-blocking agents, while in 5 cases the arrhythmia was managed successfully by implanting an atrial pacemaker for maintaining the atrial rate (2). Historically, in prior guidelines, paroxysmal AF was classified into three types based on the involvement of the ANS (3, 4). These included vagally-mediated AF, adrenergic-mediated AF, and mixed AF. It was promptly acknowledged that the impact of such classification had limited impact on AF management (3).
The clinical characteristics of patients with vagal and adrenergic AF subtypes differ significantly. Patients with vagally-mediated AF are typically younger, more often male patients without structural heart disease and in the absence of significant cardiovascular comorbidities (5). In contrast, adrenergic AF is believed to manifest in patients by a combination of triggers and atrial remodeling due to underlying structural heart disease and risk factors such as arterial hypertension, diabetes, and heart failure (5). For example, the suggestion that nocturnal AF episodes are predominantly vagally-mediated does not take into account episodes related to obstructive sleep apnea (6). Studies reporting self-reported triggers of AF paroxysms observed that vagal triggers represented between 6% and 38% of triggers (7, 8). These studies typically limited vagal triggers to postprandial AF paroxysms, nocturnal episodes, and in the absence of any acute adrenergic trigger. Further, some patients exhibit episodes compatible with both vagal and adrenergic AF (9). A specific subgroup of patients with vagal AF are endurance athletes, where the pathophysiology of AF is hypothesized to be chronic inflammation, structural remodeling due to long periods of increased atrial pressure, and an altered sympathetic-parasympathetic balance with higher vagal tone (10). The prevalence of AF in endurance athletes have been reported to be up to 10 times higher compared to controls and are predominantly vagally-mediated (11–14).
3 The autonomic nervous system in atrial fibrillation
AF results from a complex interplay of triggers, substrate, and remodeling. This pathophysiological triad interacts with several anatomical structures, which can be targeted during ablation procedures.
3.1 Triggers
AF triggers are focal ectopic activity manifesting as atrial ectopic beats or micro-reentrant circuits (15, 16). At the cellular level, ectopic firing arises from enhanced automaticity or triggered activity. While early afterdepolarizations are related to atrial action potential prolongation with reactivation of L-type calcium channels, delayed afterdepolarizations are related to calcium overload and ryanodine receptor dysfunction of the sarcoplasmic reticulum (16). Particularly delayed afterdepolarizations are of pathophysiological importance in AF as cardiovascular diseases, such as heart failure, are associated with altered atrial calcium handling (17). Similar cellular calcium abnormalities have been identified in paroxysmal and persistent AF (18, 19). Triggered activity can result in local micro-reentry due to spatial differences in action potential duration and myofibril arrangements, such as the complexity of the muscular sleeves in the pulmonary veins (20).
In AF, adrenergic activation mediated by the sympathetic nervous system strongly promote arrhythmogenesis by boosting the calcium-dependent cardiac function and calcium-dependent triggered activity (21). Further, parasympathetic hyperactivity result in a shortening of the action potential duration by activation of the acetylcholine-activated outward potassium current (22). These acetylcholine-activated potassium channels have a higher density in the left atrium when compared to the right atrium (23). Shortening of action potential duration reduces the conduction wavelength, refractory period, and as such facilitates reentry in the presence of triggers (22, 24). In animal models, the shortening of the effective refractory period was highest in the pulmonary veins and posterior left atrium (24). This heterogeneity in response to parasympathetic nerve stimulation is attributed to both differences in nerve supply and distribution of acetylcholine-activated potassium channels (22, 25).
3.2 Substrate
The substrate can be defined as specific conditions that favor initiation and maintenance of AF (15). AF substrate can be divided in three types based on their etiology and eligibility for intervention. The non-modifiable substrate includes static risk factors such as age, sex, and genetic predisposition (15). The modifiable substrate are dynamic risk factors that can be targets in the management of AF with medical or lifestyle interventions, such as comorbidities as obesity, obstructive sleep apnea, and alcohol use (15). Lastly, the AF-induced substrate was introduced in 1995 as the “AF begets AF” concept as research showed that the presence of AF episodes augments the susceptibility for future AF episodes (26). In the current era this is often referred to as atrial remodeling, which are time-dependent changes of atrial myocytes and the extracellular matrix to various external stressors or risk factors resulting in persistent changes in left atrial size or function (27). Typically, the AF substrate refers to abnormalities predisposing to re-entrant circuits with areas of slow conduction prone to unilateral conduction block and altered refractoriness, such as myocardial fibrosis (28).
3.3 Remodeling
While structural and electrical remodeling receive most attention in research, autonomic remodeling promoting the sympathetic nervous system also plays a crucial role in AF pathophysiology. AF is associated with progressive sympathetic hyperinnervation by increased atrial sympathetic nerve density and sympathetic atrial nerve sprouting in both animal models and patients with AF (21). The coinciding adrenergic overstimulation promotes the occurrence of delayed afterdepolarization (16). Chronic adrenergic stimulation stimulates structural remodeling by increased oxidative stress, amongst others (29). This sympathetic hyperinnervation can typically be observed in patients with obesity, diabetes mellitus, heart failure, and persistent AF (30–33). However, also the extracardiac sympathetic nervous system shows clear signs of remodeling in chronic cardiovascular diseases with neuronal hypertrophy and increase in the synaptic density of the stellate ganglia (34, 35).
3.4 Anatomic structures
Throughout the right and left atrium, there are anatomical structures that are well-known for their predisposition to AF triggers and their specific role in the perpetuation of AF. In paroxysmal AF, up to 94% of AF triggers originate inside the pulmonary veins and may induce AF by interacting with the complexity of the myocardial sleeves at the veno-atrial junction or the local atrial substrate (36, 37). While targeting the triggers originating from the pulmonary veins is the cornerstone for patients with paroxysmal AF, in persistent and long-standing persistent AF the triggers and re-entry sites are more frequently dependent of the atrial remodeling and the myocardial substrate (1, 15, 38).
Additional relevant anatomical structures are the ganglionated plexi. In 2004 Pachon et al. described the AF-Nest ablation technique (39). They defined two types of atrial tissue by using spectral analysis of atrial electrograms by applying fast Fourier transformation (39). First, a compact myocardium which shows classical myocardial behavior with fast conduction. Second, a fibrillar myocardium which they compared to a group of nerve cells, characterized by heterogeneous conduction and short refractory periods. They reported clustering of fibrillar myocardium in small areas, which they called AF nests as upon stimulation of these areas ectopy and AF could be induced (39). Later on, they correlated these AF nests with vagal innervation by assessing the response upon extracardiac vagal stimulation before and after ablation of these fibrillar regions (40). In 2007, Lellouche et al. described that a specific pattern of local electrograms, so-called high amplitude fractionated electrograms, prior to ablation was associated with parasympathetic responses defined as an increase in AH-interval with ≥10 ms or a decrease in heart rate ≥20% (41). In retrospect, both Pachon et al. and Lellouche et al. described techniques to determine the endocardial location of the ganglionated plexi.
Lastly, while the ligament of Marshall is the remaining structure after involution of the left superior caval vein, the vein of Marshall remains an active vein with abundant parasympathetic and sympathetic innervation and myocardial bundles (42). Due to its size, the vein of Marshall is targeted using ethanol infusion. While the vein of Marshall has been an origin of triggers and reentrant activity in the AF pathogenesis, it is most commonly targeted to facilitate or complete mitral isthmus block given its posterior epicardial location between the left inferior pulmonary vein and the coronary sinus (42, 43).
4 Assessing the autonomic nervous system in atrial fibrillation
The gold standard for assessing cardiac ANS activity is the direct measurement of action potentials, nerve activity, or changes in neurotransmitter concentrations in the sympathetic and parasympathetic nerves (44). The invasiveness of these techniques limits their use in clinical practice. Further, the robustness of measurement of venous and urinary neurotransmitter levels or their spillover is limited due to the rapid clearance of acetylcholine and the fact that circulating noradrenaline represents only a minor fraction of the neurotransmitter secreted from nerve terminals (44, 45). ANS activity is typically assessed by measuring its effect on the innervated organs, therefore ANS response, rather than true activity (46).
4.1 Heart rate variability
The most commonly applied methods to assess ANS response is heart rate variability (HRV) (47). These sets of measurements describe the fluctuations in time intervals on a beat-to-beat basis. While a higher HRV is typically considered beneficial, it should be differentiated from pathological conditions where an irregular heart rate mimics a large variability in cycle length, such as AF (48). HRV measures can largely be divided into three groups (Table 1 and Figure 1): time domain, frequency domain, and nonlinear measurements (47). In brief, the time domain indices quantify the variability in cycle length on a beat-to-beat basis, while the frequency domain indices are based on a fast fourier transformation or autoregressive models resulting in the variance of all normal-to-normal beats in predefined frequency bands (47). Nonlinear measurements, such as detrended fluctuation analysis and poincaré plots, assess the complexity, repeatability, and predictability of patterns in the normal-to-normal intervals (47). The standard deviation of normal-to-normal intervals (SDNN) is the standard of time domain measurements and reflects the sum of both sympathetic and parasympathetic activity (49). The root-mean-square of successive differences (RMSSD) is the best time domain measure to assess vagally-mediated changes in HRV and is highly correlated with the high frequency (HF) component in frequency domain analysis. The low frequency (LF) component in frequency domain analysis reflects both sympathetic and parasympathetic nervous system activity. Compared to fast fourier transformation, autoregressive models have several advantages including smoother spectral components, easy post-processing of the spectrum, and lower requirements with regards to the length of the data (50). Further, it should be noted that both short-term and 24-h measurements are reported, where the 24-h measurements are the gold standard and often show a higher predictive power (51).
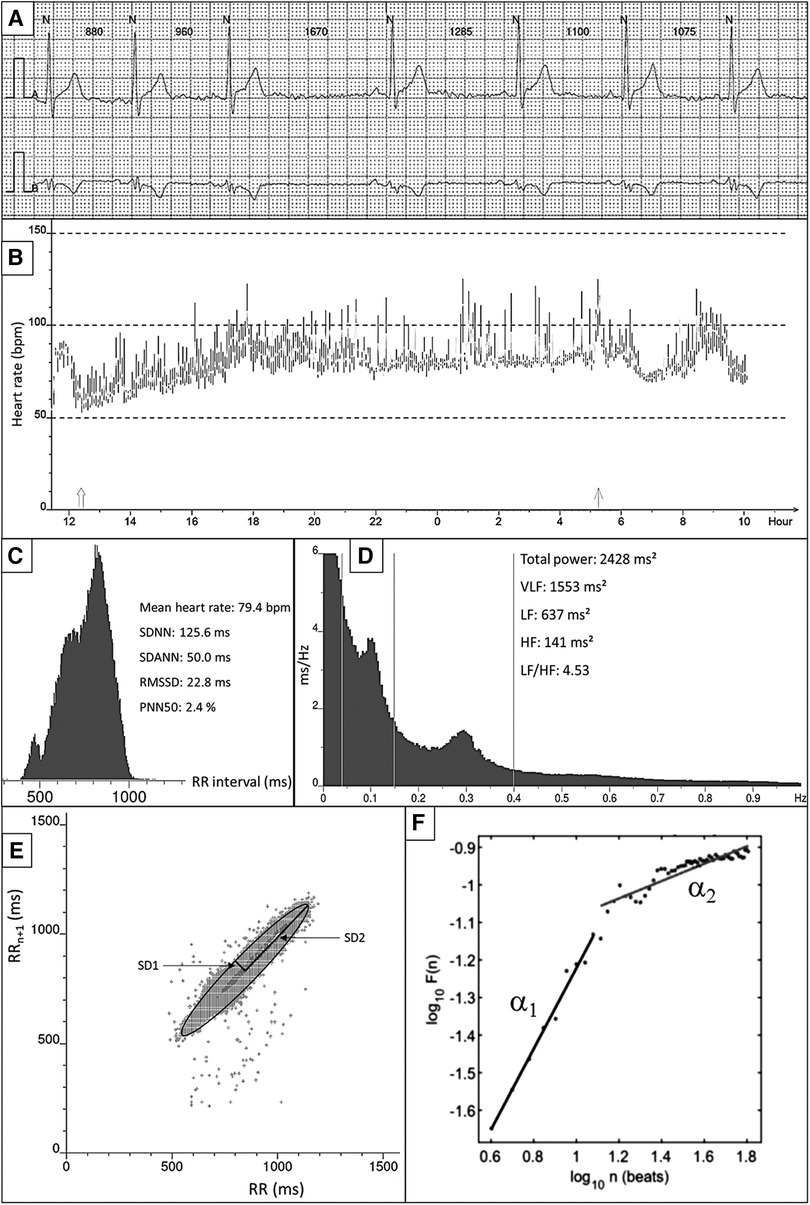
Figure 1. Overview of HRV measurements and techniques. (A) Example of raw ECG. All QRS complexes are marked with N, identifying normal heartbeats. The RR interval between consecutive beats is shown in milliseconds. (B) 24-h tachogram presenting the heart rate on the Y-axis and the time on the X-axis. (C) 24-h RR histogram presenting results of the time domain HRV analysis. (D) 24 h spectral analysis of the heart rate using a Fast Fourier Transformation with results of the frequency domain HRV analysis. (E) Poincaré plot presenting the RR interval between consecutive normal heartbeats. (F) Detrended fluctuation analysis.
Table 2 presents an overview on the findings of relevant studies regarding HRV in AF. Overall, these reports show that persistent AF is associated with lower HRV measures and that HRV shows distinct patterns to differentiate between vagal and adrenergic subtypes (9, 52, 54–56, 58, 62). However, there is an overlap where patients have both distinct vagal and adrenergic onset of AF (9, 56). Typically, HRV is reduced after AF catheter ablation, but patients with AF recurrence either show less pronounced reduction or recovery or HRV measures (53, 57, 59, 60). These associations were also present in a meta-analysis including 16 studies and a total of 2,352 patients, albeit significant publication bias was detected (63).
4.2 Heart rate turbulence
Heart rate turbulence (HRT) describes the perturbation of sinus rhythm cycle length after isolated extrasystoles (64). It provides measurements of the brief acceleration of the heart rate, turbulence onset, and the subsequent gradual deceleration of the heart rate, known as turbulence slope. While HRT is best known for risk stratification of sudden cardiac death where it is based on ventricular extrasystoles, one can also use atrial HRT by using atrial extrasystoles (64–66). Ventricular-based HRT was associated with the incidence and burden of postoperative AF (67). Atrial-based HRT onset has shown clear dynamics before the onset of AF suggesting an important role for transient enhancement of vagal tone (68). When inspecting the graphs, however, one can appreciate that most patients show a significant increase in vagal outflow, but that a smaller subset show the exact opposite suggestive of an adrenergic phenotype. It should be noted, however, that for ventricular-based HRT the site of origin does not influence its results, while for atrial-based HRT the result is highly dependent on the coupling interval and atrioventricular nodal conduction (65, 69). Therefore, instead of truly reflecting autonomic function, the potential of HRT in AF could rather be a surrogate of the pro-arrhythmic potential of short-coupled atrial extrasystoles with delayed AV conduction.
4.3 Baroreflex sensitivity
Baroreflex sensitivity (BRS) reflects acute changes in sympathetic-parasympathetic balance during blood pressure variability and is a potent risk stratification tool in cardiovascular diseases (70–72). Baroreceptors located on the aortic and carotid wall sense acute changes in blood pressure and counteract with changes in heart rate and systemic vascular resistance. As the acute changes in cardiac sympathetic activity are too slow to respond to beat-to-beat changes in blood pressure, BRS is considered to reflect vagal activity. BRS is expressed as the change in interbeat intervals in milliseconds per unit change in blood pressure (70). Higher values indicate more pronounced vagal activity. However, it should be noted that BRS does not represent the blood pressure buffering capacity but only measures the reflex effect on the sinus node, and BRS does not take into account the direction of the effects (increase or decrease). Acute increases in BRS preceding onset of AF has been described, suggesting a typical vagally-mediated AF subtype (73). Further, BRS was severely impaired during AF, but could be restored by maintaining rhythm control (74). Before catheter ablation, but measured during sinus rhythm, patients with persistent AF had a significant lower BRS when compared to those with paroxysmal AF (75).
Data on BRS after catheter ablation is limited. Both Kondo et al. and Miyoshi et al. described a significant reduction in BRS after radiofrequency catheter ablation (75, 76). Further, both described a significant difference in change between patients with AF recurrence and those without AF recurrence (75, 76). In the study by Kondo et al. the reduction in BRS was 5.0 ± 4.0 ms/mmHg in patients without recurrence, which was significantly larger than the 1.9 ± 1.4 ms/mmHg observed in patients with AF recurrence (p = 0.019) (76). Miyoshi et al. observed a slightly lower BRS in patients with persistent AF when compared to paroxysmal AF [2.97 (IQR 0.52–6.62) vs. 4.70 (IQR 2.36–8.37) ms/mmHg, p = 0.047] (75). Further, the reduction in BRS was more pronounced in patients with paroxysmal AF when compared to those with persistent AF. The reduction in BRS was significantly smaller in paroxysmal AF patients with recurrence, when compared to those without recurrence [4.21 (IQR2.50–8.19) vs. 1.97 (IQR 0.46–2.88) ms/mmHg, p = 0.011] (75). The latter was not present in patients with persistent AF and recurrence.
4.4 Other
The concept of P-wave alternans or atrial alternans is currently under investigation in the setting of AF (77). Similar to the well-known albeit nearly abandoned T-wave alternans, atrial repolarization dynamics reflect sympathetic nervous system activity by measuring beat-to-beat differences in action potential dynamics due to changes in cytosolic calcium levels (77). Distinct differences in action potential restitution curves have been observed when comparing vagally-mediated and adrenergic AF models (78). Hence, atrial alternans could be of interest to differentiate these AF subtypes, but most contemporary data is based on in-silico or animal models (77). This is partly due to the challenging technical requirements to measure atrial alternans.
Alternative measures of ANS activity, such as post-extrasystolic potentiation, self similarity, deceleration capacity, salivary gland activity, and sudomotor function, have been poorly or not studied in the setting of AF (79, 80).
5 Discussion
Over time, the role of the autonomic nervous system and its role in modulating AF pathophysiology has been consistently documented. Surges of both sympathetic and parasympathetic activity have been associated with the onset of AF. Differentiating these vagal and adrenergic AF paroxysms is however challenging as patients may exhibit both subtypes. How this knowledge can be applied to improve AF catheter ablation outcomes requires further investigation. Based on previous randomized clinical trials, some patients might benefit from autonomic denervation. Given the wide range of therapeutic interventions, the question is how can we identify which patients will best benefit from a certain intervention and evolve towards personalized AF treatment strategies. Identifying patients who might benefit from parasympathetic denervation may be challenging due to the overlap phenomenon. Also, rather than detecting AF current research is focusing on prediction of AF burden, while patients with vagal subtypes may have less frequent episodes when linked to specific triggers (81–83). Novel artificial intelligence algorithms based on HRV features have high accuracy in predicting AF based on the ECG signal just a few minutes before AF onset (82, 83). Hence, in the era of digital health and wearables there may be an opportunity to collect data and improve our understanding on how we can differentiate vagal and adrenergic AF subtypes and guide treatment strategies in future randomized clinical trials.
6 Conclusion
While the existence of a vagal AF subtype cannot be denied, there is no universal definition of a vagal AF. This is mainly due to the fact that vagal and adrenergic AF subtypes represent the extremes of a spectrum where most of the patients will present with a mixed subtype. Therefore, it is important to note that the described characteristics are not definitive markers for either subtype or that there may be significant overlap. Differentiation of vagal and adrenergic AF subtypes may have implications for the treatment options as certain interventions have shown not to modify the autonomic innervation of the heart. Non-invasive methods for identifying patients with vagally-mediated AF, such as ambulatory HRV, may have potential to guide ablation strategy. However, further research is needed to better understand the mechanisms underlying the relationship between autonomic tone and AF, and to validate the use of measures such as HRV, baroreflex sensitivity, or novel artificial intelligence algorithms, as tool for patient selection.
Author contributions
BV: Conceptualization, Formal Analysis, Methodology, Writing – original draft, Writing – review & editing. PH: Conceptualization, Formal Analysis, Methodology, Writing – review & editing. CM: Conceptualization, Formal Analysis, Methodology, Writing – review & editing.
Funding
The author(s) declare financial support was received for the research, authorship, and/or publication of this article.
BV is supported by a research grant of the Frans Van de Werf Fund for Clinical Cardiovascular Research (Leuven, Belgium).
Conflict of interest
The authors declare that the research was conducted in the absence of any commercial or financial relationships that could be construed as a potential conflict of interest.
Publisher's note
All claims expressed in this article are solely those of the authors and do not necessarily represent those of their affiliated organizations, or those of the publisher, the editors and the reviewers. Any product that may be evaluated in this article, or claim that may be made by its manufacturer, is not guaranteed or endorsed by the publisher.
References
1. Hindricks G, Potpara T, Dagres N, Arbelo E, Bax JJ, Blomstrom-Lundqvist C, et al. 2020 ESC guidelines for the diagnosis and management of atrial fibrillation developed in collaboration with the European association of cardio-thoracic surgery (EACTS). Eur Heart J. (2021) 42(5):373–498. doi: 10.1093/eurheartj/ehaa612
2. Coumel P, Attuel P, Lavallee J, Flammang D, Leclercq JF, Slama R. The atrial arrhythmia syndrome of vagal origin. Arch Mal Coeur Vaiss. (1978) 71(6):645–56.28709
3. Fuster V, Ryden LE, Cannom DS, Crijns HJ, Curtis AB, Ellenbogen KA, et al. ACC/AHA/ESC 2006 guidelines for the management of patients with atrial fibrillation: a report of the American college of cardiology/American heart association task force on practice guidelines and the European society of cardiology committee for practice guidelines (writing committee to revise the 2001 guidelines for the management of patients with atrial fibrillation): developed in collaboration with the European heart rhythm association and the heart rhythm society. Circulation. (2006) 114(7):e257–354. doi: 10.1161/CIRCULATIONAHA.106.177292
4. Camm AJ, Kirchhof P, Lip GY, Schotten U, Savelieva I, Ernst S, et al. Guidelines for the management of atrial fibrillation: the task force for the management of atrial fibrillation of the European society of cardiology (ESC). Europace. (2010) 12(10):1360–420. doi: 10.1093/europace/euq350
5. Coumel P. Paroxysmal atrial fibrillation: a disorder of autonomic tone? Eur Heart J. (1994) 15(Suppl A):9–16. doi: 10.1093/eurheartj/15.suppl_a.9
6. Lin CH, Timofeeva M, O'Brien T, Lyons OD. Obstructive sleep apnea and nocturnal attacks of paroxysmal atrial fibrillation. J Clin Sleep Med. (2022) 18(5):1279–86. doi: 10.5664/jcsm.9840
7. Hansson A, Madsen-Hardig B, Olsson SB. Arrhythmia-provoking factors and symptoms at the onset of paroxysmal atrial fibrillation: a study based on interviews with 100 patients seeking hospital assistance. BMC Cardiovasc Disord. (2004) 4:13. doi: 10.1186/1471-2261-4-13
8. de Vos CB, Nieuwlaat R, Crijns HJ, Camm AJ, LeHeuzey JY, Kirchhof CJ, et al. Autonomic trigger patterns and anti-arrhythmic treatment of paroxysmal atrial fibrillation: data from the euro heart survey. Eur Heart J. (2008) 29(5):632–9. doi: 10.1093/eurheartj/ehn025
9. Fioranelli M, Piccoli M, Mileto GM, Sgreccia F, Azzolini P, Risa MP, et al. Analysis of heart rate variability five minutes before the onset of paroxysmal atrial fibrillation. Pacing Clin Electrophysiol. (1999) 22(5):743–9. doi: 10.1111/j.1540-8159.1999.tb00538.x
10. Carpenter A, Frontera A, Bond R, Duncan E, Thomas G. Vagal atrial fibrillation: what is it and should we treat it? Int J Cardiol. (2015) 201:415–21. doi: 10.1016/j.ijcard.2015.08.108
11. Abdulla J, Nielsen JR. Is the risk of atrial fibrillation higher in athletes than in the general population? A systematic review and meta-analysis. Europace. (2009) 11(9):1156–9. doi: 10.1093/europace/eup197
12. Molina L, Mont L, Marrugat J, Berruezo A, Brugada J, Bruguera J, et al. Long-term endurance sport practice increases the incidence of lone atrial fibrillation in men: a follow-up study. Europace. (2008) 10(5):618–23. doi: 10.1093/europace/eun071
13. Mont L, Sambola A, Brugada J, Vacca M, Marrugat J, Elosua R, et al. Long-lasting sport practice and lone atrial fibrillation. Eur Heart J. (2002) 23(6):477–82. doi: 10.1053/euhj.2001.2802
14. Fragakis N, Vicedomini G, Pappone C. Endurance sport activity and risk of atrial fibrillation—epidemiology, proposed mechanisms and management. Arrhythm Electrophysiol Rev. (2014) 3(1):15–9. doi: 10.15420/aer.2011.3.1.15
15. Andrade JG, Aguilar M, Atzema C, Bell A, Cairns JA, Cheung CC, et al. The 2020 Canadian cardiovascular society/Canadian heart rhythm society comprehensive guidelines for the management of atrial fibrillation. Can J Cardiol. (2020) 36(12):1847–948. doi: 10.1016/j.cjca.2020.09.001
16. Iwasaki YK, Nishida K, Kato T, Nattel S. Atrial fibrillation pathophysiology: implications for management. Circulation. (2011) 124(20):2264–74. doi: 10.1161/CIRCULATIONAHA.111.019893
17. Yeh YH, Wakili R, Qi XY, Chartier D, Boknik P, Kaab S, et al. Calcium-handling abnormalities underlying atrial arrhythmogenesis and contractile dysfunction in dogs with congestive heart failure. Circ Arrhythm Electrophysiol. (2008) 1(2):93–102. doi: 10.1161/CIRCEP.107.754788
18. Voigt N, Heijman J, Wang Q, Chiang DY, Li N, Karck M, et al. Cellular and molecular mechanisms of atrial arrhythmogenesis in patients with paroxysmal atrial fibrillation. Circulation. (2014) 129(2):145–56. doi: 10.1161/CIRCULATIONAHA.113.006641
19. Voigt N, Li N, Wang Q, Wang W, Trafford AW, Abu-Taha I, et al. Enhanced sarcoplasmic reticulum Ca2+ leak and increased Na+-Ca2+ exchanger function underlie delayed afterdepolarizations in patients with chronic atrial fibrillation. Circulation. (2012) 125(17):2059–70. doi: 10.1161/CIRCULATIONAHA.111.067306
20. Nishida K, Datino T, Macle L, Nattel S. Atrial fibrillation ablation: translating basic mechanistic insights to the patient. J Am Coll Cardiol. (2014) 64(8):823–31. doi: 10.1016/j.jacc.2014.06.1172
21. Chen PS, Chen LS, Fishbein MC, Lin SF, Nattel S. Role of the autonomic nervous system in atrial fibrillation: pathophysiology and therapy. Circ Res. (2014) 114(9):1500–15. doi: 10.1161/CIRCRESAHA.114.303772
22. Kharbanda RK, van der Does WFB, van Staveren LN, Taverne Y, Bogers A, de Groot NMS. Vagus nerve stimulation and atrial fibrillation: revealing the paradox. Neuromodulation. (2022) 25(3):356–65. doi: 10.1016/j.neurom.2022.01.008
23. Sarmast F, Kolli A, Zaitsev A, Parisian K, Dhamoon AS, Guha PK, et al. Cholinergic atrial fibrillation: I(K,ACh) gradients determine unequal left/right atrial frequencies and rotor dynamics. Cardiovasc Res. (2003) 59(4):863–73. doi: 10.1016/s0008-6363(03)00540-6
24. Arora R, Ng J, Ulphani J, Mylonas I, Subacius H, Shade G, et al. Unique autonomic profile of the pulmonary veins and posterior left atrium. J Am Coll Cardiol. (2007) 49(12):1340–8. doi: 10.1016/j.jacc.2006.10.075
25. Tan AY, Li H, Wachsmann-Hogiu S, Chen LS, Chen PS, Fishbein MC. Autonomic innervation and segmental muscular disconnections at the human pulmonary vein-atrial junction: implications for catheter ablation of atrial-pulmonary vein junction. J Am Coll Cardiol. (2006) 48(1):132–43. doi: 10.1016/j.jacc.2006.02.054
26. Wijffels MC, Kirchhof CJ, Dorland R, Allessie MA. Atrial fibrillation begets atrial fibrillation. A study in awake chronically instrumented goats. Circulation. (1995) 92(7):1954–68. doi: 10.1161/01.cir.92.7.1954
27. Thomas L, Abhayaratna WP. Left atrial reverse remodeling: mechanisms, evaluation, and clinical significance. JACC Cardiovasc Imaging. (2017) 10(1):65–77. doi: 10.1016/j.jcmg.2016.11.003
28. Andrade J, Khairy P, Dobrev D, Nattel S. The clinical profile and pathophysiology of atrial fibrillation: relationships among clinical features, epidemiology, and mechanisms. Circ Res. (2014) 114(9):1453–68. doi: 10.1161/CIRCRESAHA.114.303211
29. Lymperopoulos A, Rengo G, Koch WJ. Adrenergic nervous system in heart failure: pathophysiology and therapy. Circ Res. (2013) 113(6):739–53. doi: 10.1161/CIRCRESAHA.113.300308
30. Ng J, Villuendas R, Cokic I, Schliamser JE, Gordon D, Koduri H, et al. Autonomic remodeling in the left atrium and pulmonary veins in heart failure: creation of a dynamic substrate for atrial fibrillation. Circ Arrhythm Electrophysiol. (2011) 4(3):388–96. doi: 10.1161/CIRCEP.110.959650
31. Stevens MJ, Raffel DM, Allman KC, Dayanikli F, Ficaro E, Sandford T, et al. Cardiac sympathetic dysinnervation in diabetes: implications for enhanced cardiovascular risk. Circulation. (1998) 98(10):961–8. doi: 10.1161/01.cir.98.10.961
32. Gould PA, Yii M, McLean C, Finch S, Marshall T, Lambert GW, et al. Evidence for increased atrial sympathetic innervation in persistent human atrial fibrillation. Pacing Clin Electrophysiol. (2006) 29(8):821–9. doi: 10.1111/j.1540-8159.2006.00447.x
33. McCully BH, Hasan W, Streiff CT, Houle JC, Woodward WR, Giraud GD, et al. Sympathetic cardiac hyperinnervation and atrial autonomic imbalance in diet-induced obesity promote cardiac arrhythmias. Am J Physiol Heart Circ Physiol. (2013) 305(10):H1530–7. doi: 10.1152/ajpheart.00196.2013
34. Han S, Kobayashi K, Joung B, Piccirillo G, Maruyama M, Vinters HV, et al. Electroanatomic remodeling of the left stellate ganglion after myocardial infarction. J Am Coll Cardiol. (2012) 59(10):954–61. doi: 10.1016/j.jacc.2011.11.030
35. Ajijola OA, Wisco JJ, Lambert HW, Mahajan A, Stark E, Fishbein MC, et al. Extracardiac neural remodeling in humans with cardiomyopathy. Circ Arrhythm Electrophysiol. (2012) 5(5):1010–116. doi: 10.1161/CIRCEP.112.972836
36. Corradi D, Callegari S, Gelsomino S, Lorusso R, Macchi E. Morphology and pathophysiology of target anatomical sites for ablation procedures in patients with atrial fibrillation: part ii: pulmonary veins, caval veins, ganglionated plexi, and ligament of Marshall. Int J Cardiol. (2013) 168(3):1769–78. doi: 10.1016/j.ijcard.2013.06.141
37. Haissaguerre M, Jais P, Shah DC, Takahashi A, Hocini M, Quiniou G, et al. Spontaneous initiation of atrial fibrillation by ectopic beats originating in the pulmonary veins. N Engl J Med. (1998) 339(10):659–66. doi: 10.1056/NEJM199809033391003
38. Verma A. The techniques for catheter ablation of paroxysmal and persistent atrial fibrillation: a systematic review. Curr Opin Cardiol. (2011) 26(1):17–24. doi: 10.1097/HCO.0b013e3283413925
39. Pachon MJ, Pachon ME, Pachon MJ, Lobo TJ, Pachon MZ, Vargas RN, et al. A new treatment for atrial fibrillation based on spectral analysis to guide the catheter RF-ablation. Europace. (2004) 6(6):590–601. doi: 10.1016/j.eupc.2004.08.005
40. Pachon ME, Pachon-Mateos JC, Higuti C, Santillana PT, Lobo T, Pachon C, et al. Relation of fractionated atrial potentials with the vagal innervation evaluated by extracardiac vagal stimulation during cardioneuroablation. Circ Arrhythm Electrophysiol. (2020) 13(4):e007900. doi: 10.1161/CIRCEP.119.007900
41. Lellouche N, Buch E, Celigoj A, Siegerman C, Cesario D, De Diego C, et al. Functional characterization of atrial electrograms in sinus rhythm delineates sites of parasympathetic innervation in patients with paroxysmal atrial fibrillation. J Am Coll Cardiol. (2007) 50(14):1324–31. doi: 10.1016/j.jacc.2007.03.069
42. Valderrabano M. Vein of Marshall ethanol infusion in the treatment of atrial fibrillation: from concept to clinical practice. Heart Rhythm. (2021) 18(7):1074–82. doi: 10.1016/j.hrthm.2021.03.032
43. Gillis K, O'Neill L, Wielandts JY, Hilfiker G, Almorad A, Lycke M, et al. Vein of Marshall ethanol infusion as first step for mitral isthmus linear ablation. JACC Clin Electrophysiol. (2022) 8(3):367–76. doi: 10.1016/j.jacep.2021.11.019
44. Zygmunt A, Stanczyk J. Methods of evaluation of autonomic nervous system function. Arch Med Sci. (2010) 6(1):11–8. doi: 10.5114/aoms.2010.13500
45. Sinski M, Lewandowski J, Abramczyk P, Narkiewicz K, Gaciong Z. Why study sympathetic nervous system? J Physiol Pharmacol. (2006) 57(Suppl 11):79–92.17244940
46. Malik M, Hnatkova K, Huikuri HV, Lombardi F, Schmidt G, Zabel M. Rebuttal from Marek Malik, Katerina Hnatkova, Heikki V. Huikuri, Federico Lombardi, Georg Schmidt and Markus Zabel. J Physiol. (2019) 597(10):2603–4. doi: 10.1113/JP277962
47. Sassi R, Cerutti S, Lombardi F, Malik M, Huikuri HV, Peng CK, et al. Advances in heart rate variability signal analysis: joint position statement by the E-cardiology esc working group and the European heart rhythm association co-endorsed by the Asia pacific heart rhythm society. Europace. (2015) 17(9):1341–53. doi: 10.1093/europace/euv015
48. Stein PK, Domitrovich PP, Hui N, Rautaharju P, Gottdiener J. Sometimes higher heart rate variability is not better heart rate variability: results of graphical and nonlinear analyses. J Cardiovasc Electrophysiol. (2005) 16(9):954–9. doi: 10.1111/j.1540-8167.2005.40788.x
49. Khan AA, Lip GYH, Shantsila A. Heart rate variability in atrial fibrillation: the balance between sympathetic and parasympathetic nervous system. Eur J Clin Invest. (2019) 49(11):e13174. doi: 10.1111/eci.13174
50. Li K, Rudiger H, Ziemssen T. Spectral analysis of heart rate variability: time window matters. Front Neurol. (2019) 10:545. doi: 10.3389/fneur.2019.00545
51. Shaffer F, Ginsberg JP. An overview of heart rate variability metrics and norms. Front Public Health. (2017) 5:258. doi: 10.3389/fpubh.2017.00258
52. van den Berg MP, Haaksma J, Brouwer J, Tieleman RG, Mulder G, Crijns HJ. Heart rate variability in patients with atrial fibrillation is related to vagal tone. Circulation. (1997) 96(4):1209–16. doi: 10.1161/01.cir.96.4.1209
53. Akyürek O, Diker E, Guldal M, Oral D. Predictive value of heart rate variability for the recurrence of chronic atrial fibrillation after electrical cardioversion. Clin Cardiol. (2003) 26(4):196–200. doi: 10.1002/clc.4960260411
54. Vikman S, Makikallio TH, Yli-Mayry S, Nurmi M, Airaksinen KE, Huikuri HV. Heart rate variability and recurrence of atrial fibrillation after electrical cardioversion. Ann Med. (2003) 35(1):36–42. doi: 10.1080/07853890310004110
55. Friedman HS. Heart rate variability in atrial fibrillation related to left atrial size. Am J Cardiol. (2004) 93(6):705–9. doi: 10.1016/j.amjcard.2003.11.052
56. Lombardi F, Tarricone D, Tundo F, Colombo F, Belletti S, Fiorentini C. Autonomic nervous system and paroxysmal atrial fibrillation: a study based on the analysis of RR interval changes before, during and after paroxysmal atrial fibrillation. Eur Heart J. (2004) 25(14):1242–8. doi: 10.1016/j.ehj.2004.05.016
57. Seaborn GE, Todd K, Michael KA, Baranchuk A, Abdollah H, Simpson CS, et al. Heart rate variability and procedural outcome in catheter ablation for atrial fibrillation. Ann Noninvasive Electrocardiol. (2014) 19(1):23–33. doi: 10.1111/anec.12098.
58. Perkiomaki J, Ukkola O, Kiviniemi A, Tulppo M, Ylitalo A, Kesaniemi YA, et al. Heart rate variability findings as a predictor of atrial fibrillation in middle-aged population. J Cardiovasc Electrophysiol. (2014) 25(7):719–24. doi: 10.1111/jce.12402
59. Vesela J, Osmancik P, Herman D, Prochazkova R. Changes in heart rate variability in patients with atrial fibrillation after pulmonary vein isolation and ganglionated plexus ablation. Physiol Res. (2019) 68(1):49–57. doi: 10.33549/physiolres.933710
60. Marinkovic M, Mujovic N, Vucicevic V, Steffel J, Potpara TS. A square root pattern of changes in heart rate variability during the first year after circumferential pulmonary vein isolation for paroxysmal atrial fibrillation and their relation with long-term arrhythmia recurrence. Kardiol Pol. (2020) 78(3):209–18. doi: 10.33963/KP.15187
61. Khan AA, Junejo RT, Thomas GN, Fisher JP, Lip GYH. Heart rate variability in patients with atrial fibrillation and hypertension. Eur J Clin Invest. (2021) 51(1):e13361. doi: 10.1111/eci.13361
62. Kim SH, Lim KR, Seo JH, Ryu DR, Lee BK, Cho BR, et al. Higher heart rate variability as a predictor of atrial fibrillation in patients with hypertension. Sci Rep. (2022) 12(1):3702. doi: 10.1038/s41598-022-07783-3
63. Zhang E, Liang S, Sun T, Xu J, Lu F, Wu D, et al. Prognostic value of heart rate variability in atrial fibrillation recurrence following catheter ablation: a systematic review and meta-analysis. Front Cardiovasc Med. (2022) 9:1048398. doi: 10.3389/fcvm.2022.1048398
64. Bauer A, Malik M, Schmidt G, Barthel P, Bonnemeier H, Cygankiewicz I, et al. Heart rate turbulence: standards of measurement, physiological interpretation, and clinical use: international society for holter and noninvasive electrophysiology consensus. J Am Coll Cardiol. (2008) 52(17):1353–65. doi: 10.1016/j.jacc.2008.07.041
65. Wichterle D, Bulkova V, Fikar M, Havranek S, Stovicek P. Heart rate turbulence after atrial premature complexes depends on coupling interval and atrioventricular nodal conduction. Pacing Clin Electrophysiol. (2007) 30(Suppl 1):S174–7. doi: 10.1111/j.1540-8159.2007.00632.x
66. Bauer A, Barthel P, Muller A, Ulm K, Huikuri H, Malik M, et al. Risk prediction by heart rate turbulence and deceleration capacity in postinfarction patients with preserved left ventricular function retrospective analysis of 4 independent trials. J Electrocardiol. (2009) 42(6):597–601. doi: 10.1016/j.jelectrocard.2009.07.013
67. Park SJ, On YK, Kim JS, Jeong DS, Kim WS, Lee YT. Heart rate turbulence for predicting new-onset atrial fibrillation in patients undergoing coronary artery bypass grafting. Int J Cardiol. (2014) 174(3):579–85. doi: 10.1016/j.ijcard.2014.04.130
68. Vikman S, Lindgren K, Makikallio TH, Yli-Mayry S, Airaksinen KE, Huikuri HV. Heart rate turbulence after atrial premature beats before spontaneous onset of atrial fibrillation. J Am Coll Cardiol. (2005) 45(2):278–84. doi: 10.1016/j.jacc.2004.10.033
69. Schwab JO, Shlevkov N, Grunwald K, Schrickel JW, Yang A, Lickfett L, et al. Influence of the point of origin on heart rate turbulence after stimulated ventricular and atrial premature beats. Basic Res Cardiol. (2004) 99(1):56–60. doi: 10.1007/s00395-003-0439-2
70. Swenne CA. Baroreflex sensitivity: mechanisms and measurement. Neth Heart J. (2013) 21(2):58–60. doi: 10.1007/s12471-012-0346-y
71. La Rovere MT, Maestri R, Robbi E, Caporotondi A, Guazzotti G, Febo O, et al. Comparison of the prognostic values of invasive and noninvasive assessments of baroreflex sensitivity in heart failure. J Hypertens. (2011) 29(8):1546–52. doi: 10.1097/HJH.0b013e3283487827
72. Garcia R, Degand B, Fraty M, Le Marcis V, Bidegain N, Laude D, et al. Baroreflex sensitivity assessed with the sequence method is associated with ventricular arrhythmias in patients implanted with a defibrillator for the primary prevention of sudden cardiac death. Arch Cardiovasc Dis. (2019) 112(4):270–7. doi: 10.1016/j.acvd.2018.11.009
73. de Castro RR, Mesquita ET, da Nobrega AC. Parasympathetic-mediated atrial fibrillation during tilt test associated with increased baroreflex sensitivity. Europace. (2006) 8(5):349–51. doi: 10.1093/europace/eul024
74. Field ME, Wasmund SL, Page RL, Hamdan MH. Restoring sinus rhythm improves baroreflex function in patients with persistent atrial fibrillation. J Am Heart Assoc. (2016) 5(2). doi: 10.1161/JAHA.115.002997
75. Miyoshi M, Kondo H, Ishii Y, Shinohara T, Yonezu K, Harada T, et al. Baroreflex sensitivity in patients with atrial fibrillation. J Am Heart Assoc. (2020) 9(24):e018019. doi: 10.1161/JAHA.120.018019
76. Kondo H, Shinohara T, Fukui A, Miyoshi M, Ishii Y, Otsubo T, et al. Possible role of baroreflex sensitivity in patients with paroxysmal atrial fibrillation. JACC Clin Electrophysiol. (2019) 5(4):523–5. doi: 10.1016/j.jacep.2019.01.009
77. Muthavarapu N, Mohan A, Manga S, Sharma P, Bhanushali AK, Yadav A, et al. Targeted atrial fibrillation therapy and risk stratification using atrial alternans. J Cardiovasc Dev Dis. (2023) 10(2). doi: 10.3390/jcdd10020036
78. Lu Z, Cui B, He B, Hu X, Wu W, Wu L, et al. Distinct restitution properties in vagally mediated atrial fibrillation and six-hour rapid pacing-induced atrial fibrillation. Cardiovasc Res. (2011) 89(4):834–42. doi: 10.1093/cvr/cvq334
79. Vinik AI, Nevoret ML, Casellini C. The new age of sudomotor function testing: a sensitive and specific biomarker for diagnosis, estimation of severity, monitoring progression, and regression in response to intervention. Front Endocrinol (Lausanne). (2015) 6:94. doi: 10.3389/fendo.2015.00094
80. Hardman SM, Noble MI, Seed WA. Postextrasystolic potentiation and its contribution to the beat-to-beat variation of the pulse during atrial fibrillation. Circulation. (1992) 86(4):1223–32. doi: 10.1161/01.cir.86.4.1223
81. Attia ZI, Noseworthy PA, Lopez-Jimenez F, Asirvatham SJ, Deshmukh AJ, Gersh BJ, et al. An artificial intelligence-enabled ECG algorithm for the identification of patients with atrial fibrillation during sinus rhythm: a retrospective analysis of outcome prediction. Lancet. (2019) 394(10201):861–7. doi: 10.1016/S0140-6736(19)31721-0
82. Parsi A, Glavin M, Jones E, Byrne D. Prediction of paroxysmal atrial fibrillation using new heart rate variability features. Comput Biol Med. (2021) 133:104367. doi: 10.1016/j.compbiomed.2021.104367
83. Ebrahimzadeh E, Kalantari M, Joulani M, Shahraki RS, Fayaz F, Ahmadi F. Prediction of paroxysmal atrial fibrillation: a machine learning based approach using combined feature vector and mixture of expert classification on HRV signal. Comput Methods Programs Biomed. (2018) 165:53–67. doi: 10.1016/j.cmpb.2018.07.014
Keywords: atrial fibrillation, autonomic nervous system, pathophysiology, heart rate variability, heart rate turbulence, non-invasive assessment
Citation: Vandenberk B, Haemers P and Morillo C (2024) The autonomic nervous system in atrial fibrillation—pathophysiology and non-invasive assessment. Front. Cardiovasc. Med. 10:1327387. doi: 10.3389/fcvm.2023.1327387
Received: 24 October 2023; Accepted: 13 December 2023;
Published: 4 January 2024.
Edited by:
Carlo de Asmundis, University Hospital Brussels, BelgiumReviewed by:
Daniela Lucini, University of Milan, Italy© 2024 Vandenberk, Haemers and Morillo. This is an open-access article distributed under the terms of the Creative Commons Attribution License (CC BY). The use, distribution or reproduction in other forums is permitted, provided the original author(s) and the copyright owner(s) are credited and that the original publication in this journal is cited, in accordance with accepted academic practice. No use, distribution or reproduction is permitted which does not comply with these terms.
*Correspondence: Bert Vandenberk dmFuZGVuYmVya2JlcnRAZ21haWwuY29t