- Department of Medicine, College of Medicine, Umm Al-Qura University, Makkah, Saudi Arabia
Introduction: Glucagon-like peptide-1 receptor agonists (GLP-1 RA) are novel agents with proven cardiovascular (CV) benefits. GLP-1 RAs have been used for diabetes and found to improve CV outcomes in diabetic and nondiabetic patients. They are authorized for treating obesity. Our narrative review discussed the CV benefits of GLP-1 RAs in terms of controlling CV risk factors and improving CV outcomes in diabetic and nondiabetic patients regardless of their CV history, and the CV perspectives related to their use in clinical practice.
Areas covered: Literature was searched with no limits on date or language, using various combinations of keywords. Data on the CV benefits of GLP-1 RAs and their use in clinical practice were summarized.
Results: Several studies have discussed the CV beneficial effects of GLP-1 RAs in terms of reducing blood pressure, lipid levels, body weight, risk for arrhythmias, reducing the risk of major adverse CV events, and hospital admission for heart failure.
Conclusion: The cardioprotective effects and low risk of hypoglycemia of GLP-1 RAs make them preferred agents in any multidisciplinary approach aiming to reduce CV disease burden and improve prognosis. Cardiologists are encouraged to strongly consider the CV benefits of GLP-1 RAs in their risk-reduction strategies.
1 Introduction
Besides the traditional standard-of-care agents, newer classes of glucose-lowering agents have been introduced and changed the landscape of type 2 diabetes mellitus (T2DM) management (1). Glucagon-like peptide-1 receptor agonists (GLP-1 RAs) are novel glucose-lowering agents that activate GLP-1 receptors leading to blood glucose reduction, postprandial glucose metabolism improvement, and gastric emptying delay (2). In response to ingested meals, GLP-1, an incretin hormone, stimulate glucose-dependent insulin secretion, suppress glucagon, prolong gastric emptying, and promote glucose uptake into muscles and adipose tissues (3). Consequently, the feeling of satiety is promoted and associated with a decrease of craving and food intake leading to weight loss. In addition, GLP-1 RAs decrease the glycated hemoglobin by 0.8%–1.5%, making them superior to other anti-diabetic drugs and a good choice for diabetes management (2). Currently, there are six FDA-approved GLP-1 RAs that have different properties, particularly in terms of administration, weight loss, and cardiovascular (CV) benefits: exenatide, liraglutide, dulaglutide, lixisenatide, semaglutide, and tirzepatide (4). Liraglutide, semaglutide, and tirzepatide are FDA approved for chronic weight management in patients with obesity or overweight with comorbidities (5).
The CV benefits were variable among the different available GLP-1 RAs; some randomized controlled trials demonstrated that liraglutide, dulaglutide, semaglutide, and albiglutide reduced the rate of CV events in T2DM patients (6–9), while other clinical trials concluded that lixisenatide and exenatide did not significantly improve CV outcomes in such patients (10, 11). In its most recent guidelines, the American Diabetes Association (ADA) established a comprehensive clinical approach to reduce CV risks in T2DM patients. This approach emphasized the use of cardioprotective GLP-1 RAs [liraglutide, dulaglutide, semaglutide, and albiglutide (withdrawn from the market for business reasons)] in T2DM patients with or at high risk for atherosclerotic CVD (ASCVD), heart failure (HF), or chronic kidney disease (12).
Another novel glucose-lowering agent is tirzepatide which has a dual mechanism of action: a GLP-1 and a glucose-dependent insulinotropic polypeptide (GIP) co-agonist. Both the GLP-1 and GIP receptors are expressed on neurons in the arcuate nucleus and other parts of the hypothalamus, and their activation reduce food intake and body weight (13, 14). A pre-specified meta-analysis including all the seven clinical trials that constituted the clinical development program of tirzepatide (SURPASS) demonstrated its CV safety in patients with T2DM in terms of not increasing the risk of major adverse CV events (MACE). This meta-analysis also suggested a non-significant trend toward potential CV benefits of tirzepatide (hazard ratios 0.8, [95% confidence interval (CI), 0.57–1.11] for MACE; 0.9 [95% CI, 0.50–1.61] for CV death; 0.8 [95% CI, 0.51–1.25] for all-cause death) in line with what was demonstrated for GLP-1 RAs (15).
These findings have promoted an interest to discover the CV benefits of this pharmacological class extending beyond its glycemic effect. GLP-1 RAs have been found to improve body weight, blood pressure (BP), lipid profile levels, and myocardial infarction (MI) outcomes even in nondiabetic patients without increasing the risk of hypoglycemic events (11, 16–20). Similarly, GLP-1 RAs have significantly reduced the risk of individual MACE components, all-cause mortality, and hospital admission for HF (21). The mechanisms underlying these CV benefits include promoting myocardial glucose uptake and utilization, reducing oxidative stress, inhibiting cardiomyocyte apoptosis, vasodilation, improving coronary blood flow, natriuresis, anti-inflammatory effects, renal protection, reducing plaque formation, and neurohormonal regulation (22). The anti-atherosclerosis and attenuated plaque formation effects of GLP-1 RAs are mediated through inhibiting the overall inflammatory response by suppressing the expression and release of proinflammatory cytokines (such as interleukin-6 and tumor necrosis factor-α) and suppressing the activation of nuclear factor-kappa B signaling pathway (23, 24). Another suggested mechanism for CV benefit is the reduction of epicardial adipose tissue which is involved in the development and progression of coronary artery disease, atrial fibrillation (AF), and HF. Epicardial adipose tissue responds to GLP-1 RAs in a mechanism that contributes to their CV benefits (25–28).
Our narrative review aimed to discuss the CV benefits of GLP-1 RAs in terms of controlling CV risk factors and improving HF outcomes, and the CV perspectives related to their use in clinical practice.
2 Methods
The authors conducted a literature search, with no limits on date or language, using various combinations of keywords, including “glucagon-like peptide-1”, “cardiovascular diseases”, “cardiovascular diseases prevention”, “cardiovascular outcome trial”, and “cardiovascular”. Further references were identified by searching the reference lists of retrieved articles and from the authors' knowledge of the field.
3 Results
3.1 Effects on overweight/obesity
Obesity has been identified as a chronic disease linked to increased mortality, coronary artery disease, T2DM, some types of cancer, and many other complications (29–31). A systematic review has showed that a 5%–10% weight reduction improves cardiometabolic parameters, including BP, blood glucose level and dyslipidemia (32).
A randomized controlled trial involving 3,731 patients who did not have T2DM showed that 3 mg of subcutaneous liraglutide was associated with body weight reduction. A total of 63.2% of the patients in the liraglutide arm lost at least 5% of their body weight compared with 27.1% in the placebo arm (P < 0.001). A weight loss difference of −5.6 kg (95% CI, −6.0 to −5.1; P < 0.001) was demonstrated at Week 56 in favor of liraglutide (33). These findings were further demonstrated in different meta-analyses (34–36). Liraglutide was well-tolerated, and the associated gastrointestinal adverse events were of mild to moderate severity. It is a short-acting GLP-1 RA and should be administered once daily.
Regarding exenatide, randomized trials reported that subcutaneous exenatide (10 mcg with a 4-week 5-mcg dose-initiation period) plus lifestyle modification resulted in weight reduction in nondiabetic obese patients (37). Meta-analyses of randomized controlled trials also showed that exenatide (10–20 mcg/day) could significantly reduce body weight in obese or overweight nondiabetic participants (34, 38). However, exenatide had the modest effect on weight reduction (39).
In the STEP-1 trial (phase 3, randomized, placebo-controlled), once-weekly injection of 2.4 mg semaglutide plus lifestyle modification were associated with sustained and clinically-relevant reduction in body weight in overweight or obese participants (40). A meta-analysis of 4 randomized controlled trials including a total of 3,613 nondiabetic individuals with obesity, reported a mean difference for weight reduction of −11.85% favoring semaglutide (95% CI, −12.81 to −10.90; P < 0.001). Most of the studies used once weekly subcutaneous injection of semaglutide starting at a dose of 0.25 mg and escalated every 4 weeks. Semaglutide was associated with gastrointestinal adverse events (nausea, vomiting, diarrhea, constipation) which were of mild to moderate severity, short duration, and resolved without treatment discontinuation (41).
Notably, a recent systematic review showed that compared to other semaglutide doses and GLP-1 RAs, 2.4 mg semaglutide had the greatest effect on weight reduction, controlling glucose level, and reducing BP; however, it was associated with the highest rate of gastrointestinal adverse events (39).
A systematic review and meta-analysis conducted to investigate the efficacy of tirzepatide on glycated hemoglobin, fasting serum glucose, and body weight in patients with uncontrolled T2DM reported that tirzepatide significantly improved glycemic control and body weight with an acceptable safety profile (42). In addition, SURMOUNT-1, a phase 3 clinical trial, evaluated the efficacy and safety of tirzepatide in nondiabetic adults with obesity or overweight. Three doses of once-weekly tirzepatide (5, 10, 15 mg) provided significant and sustained reduction in body weight (43).
The FDA has approved liraglutide (a maintenance dose of 3 mg injected daily), subcutaneous semaglutide (2.4 mg injected once weekly), and tirzepatide (5–15 mg injected once weekly) for chronic weight management in adults with body mass index (BMI) of 30 kg/m2 or greater, or with a BMI of 27 kg/m2 or greater with at least one weight-related condition (such as high BP, T2DM, or high cholesterol) to be used in addition to a reduced calorie diet and increased physical activity (44–46). However, exenatide was not approved for this indication.
Overall, robust findings have showed that GLP-1 RAs effectively promote weight loss. Weight reduction has been linked to improved CV risk factors and complications, particularly hypertension, ASCVD, HF, AF, pulmonary hypertension, and left ventricular hypertrophy (47, 48). In addition, weight reduction induced by GLP-1 RAs improved obstructive sleep apnea (another CV risk factor) severity and related outcomes (49, 50). Furthermore, GLP-RAs may be considered strong candidates for the management of nonalcoholic fatty liver disease looking at their promising results in reducing liver steatosis (51).
3.2 Effects on hypertension and hyperlipidemia
Besides their contribution to weight reduction and glycemic control, GLP-RAs were associated with BP and lipid levels reduction (52). The results of a recent meta-analysis showed that semaglutide, exenatide, and liraglutide were associated with a systolic BP (SBP) decrease ranging from—4.89 mm Hg to—2.68 mm Hg. Notably, only 2.4 mg subcutaneous semaglutide was associated with diastolic BP (DBP) decrease of 1.61 mm Hg (39). BP reduction has been shown to be associated with significant reduction in MACE (2). Moreover, hypertension is a common preventable risk factor for CVDs including coronary artery diseases, AF, and HF (53). However, a clinically meaningful reduction in BP which can be associated with lower risk of CV events was defined as a decrease of at least 5 mm Hg in SBP or 3 mm Hg in DBP (54).
Effects on lipid profile were collected as secondary outcomes from several clinical trials. GLP-1 RAs were associated with favorable effects on lipoprotein metabolism manifested as modest lowering of low-density lipoprotein, total cholesterol, and fasting triglyceride levels. Moreover, suppression of postprandial hypertriglyceridemia, a potential risk predictor of ASCVD, has been reported (20, 55, 56). Hence, GLP-1 RAs can alter the process of atherosclerosis and show atherothrombotic benefits; consequently, they decrease the risk of CVDs (2, 57, 58).
3.3 Effects on major adverse cardiovascular events (MACE)
GLP-1 RAs showed significant outcome reduction in terms of MACE reduction in both diabetic and nondiabetic patients regardless of their history of established CVD.
The CV outcome trials (CVOT) (Figure 1) of all the GLP-1 RAs (liraglutide, semaglutide, dulaglutide, albiglutide, extended-release exenatide, and lixisenatide) (6–11, 59) have demonstrated non-inferiority (CV safety). On the other hand, to date, three GLP-1 RAs have demonstrated a reduction in the MACE in patients with T2DM (liraglutide, subcutaneous semaglutide, dulaglutide) (60) (Table 1).
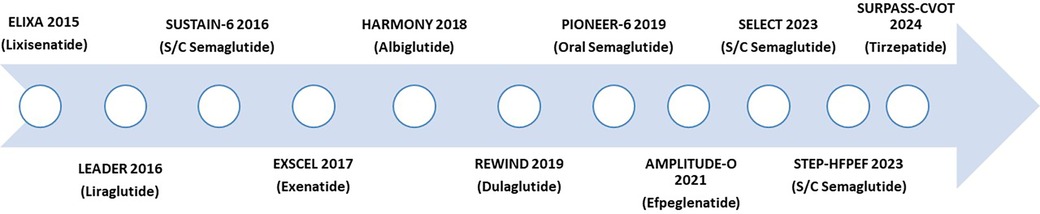
Figure 1. Timeline of GLP-1 RAs cardiovascular outcomes trials. GLP1 RA, glucagon-like peptide 1 receptor agonist; s/c, subcutaneous.
The LEADER trial (6) investigated the CV effects of liraglutide in 9,340 T2DM patients who were randomized to receive either liraglutide (up to 1.8 mg once daily) or placebo. Patients included were either ≥ 50 or ≥60 years old with known CVD or multiple CV risk factors, respectively. The primary endpoint was three-point MACE composite outcome (first occurrence of death from CV causes, nonfatal MI, or nonfatal stroke) which occurred in 13% of the treatment arm vs. 14.9% of the placebo arm with a 13% relative risk reduction. Both non-inferiority (P < 0.001) and superiority (P = 0.01) to placebo were demonstrated for the primary composite MACE endpoint. The number needed to treat to prevent a MACE event was 53 over 3.8 years. The rates of nonfatal MI, nonfatal stroke, and hospitalization for HF were numerically but non-significantly lower in the liraglutide group vs. the placebo group.
Based on the demonstrated superiority, the FDA approved an additional labeled indication for liraglutide “to reduce the risk of major cardiovascular events in adults with type 2 diabetes mellitus and established cardiovascular disease” (61).
The CV outcomes of semaglutide in T2DM patients were evaluated in the SUSTAIN-6 trial (7). It included 3,297 T2DM patients who were on standard-of-care treatment and randomly assigned to receive once-weekly subcutaneous semaglutide (0.5 mg or 1.0 mg), or placebo for 104 weeks. Inclusion criteria were an age of ≥50 years with established CVD or an age of ≥60 years with at least one CV risk factor. The primary endpoint (three-point MACE composite outcome) was 6.6% in the semaglutide arm compared to 8.9% in the placebo arm (P < 0.001 for non-inferiority) resulting in a risk reduction of 26%. Semaglutide was significantly associated with a lower rate of nonfatal stroke (P = 0.04) and a numerically but non-significantly lower rate of nonfatal MI. Rate of CV death was similar in both arms. The number needed to treat to prevent a MACE event was 45 for 2 years. The superiority of subcutaneous semaglutide was demonstrated in a post hoc analysis for the primary composite MACE endpoint (P = 0.02). Hence, subcutaneous semaglutide labeling was updated to include an indication for reducing the risk of MACE in patients with T2DM and established CVD (61).
On the other hand, the PIONEER-6 trial aimed to evaluate the CV safety of once-daily oral semaglutide (the only oral GLP-1 RA) (59). It included 3,183 T2DM patients who were assigned to receive, in addition to standard of care, oral semaglutide (3–7 mg daily) or placebo with a median follow-up of 1.3 years. This trial showed that oral semaglutide was non-inferior to placebo and associated with a reduced risk of MACE composite outcome by 21% (P < 0.001 for non-inferiority) which was comparable to that reported by subcutaneous semaglutide in the SUSTAIN-6 trial. However, superiority with oral semaglutide was not achieved (P = 0.17). In both trails, sample size, discontinuation rate, mean diabetes duration, and percentage of patients with established ASCVD were comparable. The proportion of patients with chronic kidney disease was greater in the SUSTAIN-6 trial. Unlike subcutaneous semaglutide, more patients experienced nonfatal MI with oral semaglutide, but the oral formulation showed a statistically significant reduction in CV death and all-cause mortality. As a conclusion, oral semaglutide has a CV safety profile similar to that of the subcutaneous form, as shown in SUSTAIN-6 trial. Hence, it is unclear whether the lack of CV benefit with oral semaglutide is attributed to its formulation or secondary to selection bias. However, PIONEER-6 study was neither powered for superiority (CV efficacy) nor designed to determine statistical significance for secondary outcomes. In an attempt to assess CV efficacy of oral semaglutide after demonstrating its CV safety in the PIONEER-6 trial, a large CVOT (N = 9,650) is currently ongoing (SOUL trial) to compare the risk of MACE composite outcome with oral semaglutide vs. placebo in subjects with T2DM and established ASCVD and/or chronic kidney disease (62).
The CV outcomes of dulaglutide in T2DM patients were assessed in the REWIND trial (8). It included 9,901 patients with T2DM aged at least 50 years who had either an established CV event or CV risk factors. They were randomly assigned to receive either a weekly subcutaneous injection of dulaglutide (1.5 mg) or placebo. The primary endpoint was a three-point MACE composite outcome, and the tested hypothesis was superiority to placebo in terms of CV benefits. The primary composite outcome occurred in 12% of the dulaglutide group participants vs. 13.4% of the placebo group participants (P = 0·026). All-cause and CV mortality did not differ between groups. Dulaglutide was additionally approved for reducing the risk of MACE outcomes in T2DM adults with established CVD or multiple CV risk factors (61).
Several meta-analyses of the GLP-1 RAs CVOTs have been published (21, 63–65). A meta-analysis of eight GLP-1 RAs CVOTs demonstrated that GLP-1 RAs reduced the risk of MACE by 14% (P = 0.006) in the overall T2DM population. At the level of individual MACE components, GLP-1 RAs also reduced CV mortality (by 13%, P = 0.016), nonfatal stroke (by 16%, P = 0.007), and nonfatal MI (by 9%, but statistically non-significant).
On the other hand, another meta-analysis showed that GLP-1 RAs reduced the risk of CV death [Risk Ratio (RR), 0.90; 95% CI, 0.83–0.97; P = 0.004], and fatal or nonfatal stroke (RR, 0.85; 95% CI, 0.77–0.94; P = 0.001). However, GLP-1 RAs failed to reduce the risk of fatal or nonfatal MI (RR, 0.91; 95% CI, 0.82–1.01; P = 0.06) (66).
The reported CV benefits were not associated with an increase in risk of severe hypoglycemia, retinopathy, or pancreatic adverse effects (21).
Moreover, a very recent multicenter, double-blind, randomized, placebo-controlled trial (the SELECT trial), showed that weekly 2.4 mg subcutaneous semaglutide was superior to placebo in reducing the risk of CV death, nonfatal MI, or nonfatal stroke at a mean follow-up of 39.8 months. This trial included 17,604 nondiabetic overweight or obesity patients with preexisting CVD. The primary endpoint (a composite of death from CV causes, nonfatal MI, or nonfatal stroke) occurred in 6.5% of the semaglutide group vs. 8% of the placebo group (hazard ratio, 0.8; 95% CI, 0.72–0.90; P < 0.001) (67).
Based on the improved CV outcomes of GLP-1 RAs, the novel combination of GIP and GLP-1 RA may be another promising component in approaches aiming to reduce CV events, particularly in high-risk patients. A phase 3, randomized, double-blind CVOT, SURPASS-CVOT, is currently ongoing and aiming to assess tirzepatide vs. dulaglutide (1.5 mg weekly) for both non-inferiority and superiority in terms of CV outcomes (68). SURPASS-CVOT will provide definitive evidence on the CV safety and efficacy of tirzepatide.
3.4 Effects on heart failure
In addition to their demonstrated CV benefits, GLP-1 RAs have showed promising signals for their potential benefits in HF management. Two small clinical trials, the FIGHT and LIVE, evaluated the effect of liraglutide on patients with acute or chronic HF with reduced ejection fraction (HFrEF) (69, 70). Both trials failed to show any benefit of liraglutide in terms of HF outcomes.
On the other hand, the STEP-HFpEF trial evaluated the effect of semaglutide on nondiabetic obese patients with HF with preserved left ventricular EF (HFpEF) and obesity. Compared to placebo, semaglutide led to greater weight loss, HF-related symptoms improvement, reduced physical limitations, and significant difference in 6-minute walk distance (71). Of interest, the STEP-HFpEF trial has demonstrated benefits of semaglutide in nondiabetic patients with HFpEF and obesity. The effect of 2.4 mg once-weekly injected semaglutide in obese diabetic patients with HFpEF is currently investigated in an ongoing trial (STEP-HFpEF DM) (72).
No benefits in terms of HF outcomes were observed in the individual CVOTs of the GLP-1 RAs (22). However, Sattar et al. meta-analysis of the eight CVOTs including more than 60,000 patients with T2DM showed positive effect of GLP-1 RAs on hospital admission for HF (21). Similar results were also reported by the meta-analysis of Giugliano et al. (63). Overall, GLP-1 RAs showed a significant reduction in the risk of HF hospitalization by 10% (P = 0.023) to 11% (P = 0.013) (21, 63, 64).
On the other hand, a recent meta-analysis focusing on the effect of GLP-1 RAs on prognosis in patients with HF showed that GLP-1 RAs significantly reduced the risk of MACE compared to placebo in HF coexisting with T2DM. However, this benefit was not observed in all-cause death, hospitalization for HF, CV death, MI, stroke, and death or hospitalization for HF (65).
The benefits of GLP-1 RAs in HF may be explained in terms of their weight reduction properties. Obesity is linked to increased risk of HF incidence, particularly HFpEF (73). Moreover, the ability of GLP-1 RAs to reduce the generation of reactive oxygen species and systemic inflammation may be considered in this context (74).
3.5 Effects on arrythmia
GLP-1 RAs were associated with a significant reduction in all-cause mortality and CV mortality, and did not increase the risk of sudden cardiac death, atrial arrythmias, or ventricular arrhythmias in T2DM patients (75, 76). Although a meta-analysis including all the GLP-1 RAs CVOTs has showed that this group has no effect on the risk of various types of arrhythmias (77), two meta-analyses have demonstrated a reduced risk of atrial arrhythmias (78, 79). One of these meta-analyses compared the effect of different glucose-lowering agents on the risk of AF and included only studies that reported AF or atrial flutter as clinical end points with a follow-up period of at least 12 months (78). The second meta-analysis included randomized controlled trials (ELIXA, HARMONY OUTCOMES, PIONEER-6, SUSTAIN-6, LEADER) that compared GLP-1 RAs with placebo and met the critical criterion of a proportion of patients with T2DM and MI > 30% (79). Of interest, the subgroup analysis showed that semaglutide reduced the risk of atrial arrhythmias and AF by 36% and 38%, respectively, while no anti-arrhythmic effect was observed among the other GLP-1RAs (79). Hence, the anti-arrhythmic effects of semaglutide must be considered in the relevant clinical context i.e., in T2DM patient with MI. The favorable outcomes of semaglutide on AF was also emphasized by the findings of recent meta-analyses (80, 81), whether in patients with or without diabetes (81).
3.6 GLP-1 RAs in clinical practice
The cardioprotective properties of GLP-1 RAs seem to be mediated through CV risk factors modification. GLP-1 RAs have been shown to improve glycemic control and insulin resistance; lower blood pressure; reduce body weight; and moderately reduce total cholesterol, low-density lipoprotein cholesterol, and triglycerides (82, 83) (Figure 2). However, the cardioprotective effects of GLP-1 RAs may be explained beyond traditional risk factors modification. GLP-1 RAs exert direct and indirect effects on the CV system including vasodilation, natriuresis anti-atherosclerotic effects, improvements in endothelial function, anti-inflammatory effects (decrease cytokines level such as tumor necrosis factor, interlukin-1, interlukin-6) and a consequent decrease in C-reactive protein, and reductions in infarct size (60, 84, 85).
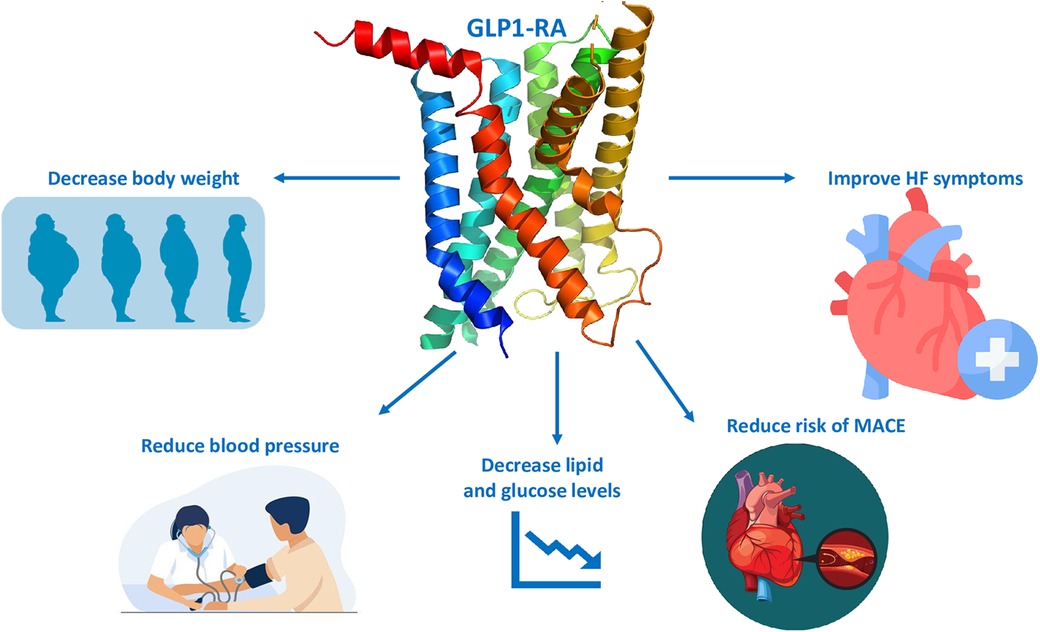
Figure 2. GLP-1 RAs cardiovascular benefits. GLP1 RA, glucagon-like peptide 1 receptor agonist; HF, heart failure; MACE, major adverse cardiovascular events.
Recently, GLP-1 RAs have been recommended by different guidelines. According to the 2023 ADA standards of care in diabetes (12), GLP-1 RAs with demonstrated CV benefit are considered for T2DM patients with established ASCVD or at high risk for ASCVD to reduce the risk of MACE. MACE reduction has been driven by the reduction of CV deaths as demonstrated with liraglutide, or reduction of nonfatal strokes as demonstrated with dulaglutide or semaglutide (6, 8, 62). Established ASCVD was defined as MI, stroke, any revascularization procedure, transient ischemic attack, unstable angina, amputation, symptomatic or asymptomatic coronary artery disease. Indicators of high CV risk included an age of >55 years, with two or more additional risk factors (obesity, hypertension, smoking, dyslipidemia, or albuminuria) (1). Similarly, the 2024 European Society of Cardiology (ESC) guidelines for the management of chronic coronary syndrome has recommended semaglutide for overweight (BMI >27 kg/m2) or obese chronic coronary syndrome patients without diabetes to reduce CV mortality, MI, or stroke. Moreover, GLP-1 RAs with proven CV benefits are recommended in patients with T2DM and chronic coronary syndrome to reduce CV event (86).
Cardiologists are invited to be more familiar with the members of this pharmacological family. All GLP-1 RAs including the dual agent tirzepatide and oral semaglutide demonstrated CV safety. Only liraglutide, subcutaneous semaglutide, and dulaglutide have showed CV efficacy. Furthermore, liraglutide, subcutaneous semaglutide, and tirzepatide are approved for chronic weight management regardless of diabetes status. These outcomes were further endorsed by the recent ESC Clinical Consensus Statement on Obesity and Cardiovascular Disease (87).
In addition, a recent analysis of the SELECT trial results showed that semaglutide use was associated with a substantial reduction in non-CV death. The lower rate of non-CV deaths was because of lower infectious deaths, in particular COVID-19–related deaths, suggesting that semaglutide has a favorable outcome in terms of mortality across a broad population of patients with CV disease and obesity (88).
Besides HbA1c and weight reduction, the criteria for initiating a GLP-1 RA with proven CV benefit should include an estimation of the patient's CV risk. Cardiologists may consider making an informed clinical decision by using reliable CV risk scores in addition to the judgment of available clinical evidence. The best-fit patients for GLP-1 RAs are those with ASCVD with a high risk of future stroke (e.g., with history of stroke or revascularization, or with evidence of significant artery narrowing). They may also be preferred in patients with elevated glycemia levels and high BMI aiming at modifying CV risk factors (2). Of note, GLP-1 RAs are not recommended for patients with T1DM.
GLP-1 RAs have a favorable safety profile. Gastrointestinal side effects are the most common including nausea, vomiting, and diarrhea. Unless combined with other agents that cause hypoglycemia, such risk is minimal with GLP-1 RAs. Pruritis and erythema at injection site may occur. Pancreatitis and pancreatic cancer were reported in some studies but not in large CVOTs with relatively long duration of follow-up. However, GLP-1 RAs are still not recommended in patients with a history of pancreatitis (2). Furthermore, GLP-1 RAs are not recommended for patients with severe gastrointestinal diseases (e.g., gastroparesis and inflammatory bowel disease); with a personal or family history of multiple endocrine neoplasia 2A, multiple endocrine neoplasia 2B, or medullary thyroid cancer (89). Subcutaneous semaglutide should be used cautiously in patients with pre-existing diabetic retinopathy (90, 91). It is noteworthy that GLP-1 RAs are contraindicated in pregnancy and breastfeeding; women who are planning to get pregnant should stop the medication 2 months before planned pregnancy (84). In addition, GLP-1 RAs were not associated with increased risk of suicide death, self-harm, or incident depression and anxiety-related disorders; suicide death among GLP-1 RAs users was rare (92).
Except oral semaglutide, GLP-1 RAs are administered subcutaneously, particularly those with proven CV benefits. Hence, treatment compliance can be improved by selecting agents that are injected once weekly such as semaglutide or dulaglutide. Gastrointestinal side effects are reduced by starting the GLP-1 RA at a low dose and titrating up over a certain duration according to each product labelling. Up-titration can be slowed to limit severity and frequency of side effects. Doses may be lowered temporarily until symptoms resolve. Upon initiation of a GLP-1 RAs and during up-titration, it is advisable for cardiologists to counsel their patients to take smaller meals, eat slowly and stop before feeling full, and avoid fatty or spicy foods (2).
4 Conclusion
GLP-1 RAs seem to emulate the evolution of statins and sodium-glucose co-transporter-2 inhibitors in terms of being approved for indications other than their initial indication and becoming promising agents in CV medicine which will continue to grow. They have emerged as a valuable therapeutic option for diabetic and nondiabetic individuals, particularly those at high risk of CVD.
GLP-1 RAs are the first weight-reduction medications that are safe and improve CV outcomes. The CV benefits of these agents are evident at the level of primary prevention of CVDs through controlling CV risk factors, particularly overweight and obesity. Moreover, the cardioprotective properties of GLP-1 RAs have been manifested in terms of reducing hypertension, hyperlipidemia, and cardiac arrythmias; controlling these factors plays a key role in the CV risk mitigating strategies. In addition to cardiometabolic risk factor reduction, their additional effect at the cellular and cytokines levels is likely to contribute to reduction of inflammation, atherosclerosis, and plaque formation.
The CV benefits extend to the promising effects in improving HF outcomes. Emerging evidence from clinical trials suggested a positive impact of GLP-1 RAs on nondiabetic obese HFpEF in terms of improving physical limitations, symptoms, and exercise function. These interesting findings may be also attributed to the weight reduction properties. Several CVOTs have demonstrated that GLP-1 RAs reduced CV risk in T2DM patients with established ASCVD or high CV risk independent of HbA1c levels.
Considering the reported CV findings, cardiologists are qualified to take the lead in adopting CV risk mitigations strategies as a part of comprehensive management strategies in collaboration with endocrinologists/diabetologists. GLP-1 RAs initiation by cardiologists in the appropriate clinical context may be considered a key factor in such strategies. Hence, implementing evidence-based agents with CV benefits by cardiologists is crucial for mitigating the CV risks in diabetic and nondiabetic patients. The cardioprotective effects of GLP-1 RAs along with their low risk of hypoglycemia make them preferred agents in any multidisciplinary approach for prevention of CVDs, reduction of the disease burden and improvement of prognosis. In this context, cardiologists are required to discuss with insurance companies the prescription of GLP-1 RAs and to advocate their use as cardioprotective gents in the presence of robust body of evidence. We are looking forward for the results of the SOUL and SURPASS-CVOT trials which, in case concluded with positive results, will add two new GLP-1RAs with CV efficacy.
Author contributions
YT: Conceptualization, Supervision, Validation, Writing – original draft, Writing – review & editing.
Funding
The author(s) declare that no financial support was received for the research and/or publication of this article.
Acknowledgments
The authors wish to thank Mohammad Yassine, BPharm, MSc and Racha Aaraj, Pharm D, MSc, MPH from Phoenix Clinical Research for the support in the preparation of this manuscript. This assistance was carried out in accordance with Good Publication Practice (GPP 2022) and the ICMJE requirements. The views and opinions expressed are those of the authors.
Conflict of interest
The author declares that the research was conducted in the absence of any commercial or financial relationships that could be construed as a potential conflict of interest.
Generative AI statement
The author(s) declare that no Generative AI was used in the creation of this manuscript.
Publisher's note
All claims expressed in this article are solely those of the authors and do not necessarily represent those of their affiliated organizations, or those of the publisher, the editors and the reviewers. Any product that may be evaluated in this article, or claim that may be made by its manufacturer, is not guaranteed or endorsed by the publisher.
Abbreviations
ADA, American diabetes association; AF, atrial fibrillation; ASCVD, atherosclerotic cardiovascular disease; BP, blood pressure; CI, confidence interval; CV, cardiovascular; CVD, cardiovascular disease; CVOT, cardiovascular outcome clinical trial; DBP, diastolic blood pressure; EMA, European medicines agency; ESC, European society of cardiology; FDA, food and drug administration; GLP-1 RA, glucagon-like peptide-1 receptor agonist; HF, heart failure; HFpEF, heart failure with preserved ejection fraction; HFrEF, heart failure with reduced ejection fraction; MACE, major adverse cardiovascular events; MI, myocardial infarction; RR, risk ratio; SBP, systolic blood pressure; T2DM, type 2 diabetes mellitus.
References
1. ElSayed NA, Aleppo G, Aroda VR, Bannuru RR, Brown FM, Bruemmer D, et al. 9. Pharmacologic approaches to glycemic treatment: standards of care in diabetes-2023. Diabetes Care. (2023) 46(1):S140–57. doi: 10.2337/dc23-S009
2. Marx N, Husain M, Lehrke M, Verma S, Sattar N. GLP-1 Receptor agonists for the reduction of atherosclerotic cardiovascular risk in patients with type 2 diabetes. Circulation. (2022) 146(24):1882–94. doi: 10.1161/CIRCULATIONAHA.122.059595
3. Holst JJ. The physiology of glucagon-like peptide 1. Physiol Rev. (2007) 87(4):1409–39. doi: 10.1152/physrev.00034.2006
4. Collins L, Costello RA. Glucagon-Like peptide-1 receptor agonists. In: StatPearls. Treasure Island (FL): StatPearls Publishing (2025). Available online at: http://www.ncbi.nlm.nih.gov/books/NBK551568/ (cited March 17, 2025).
5. Alfaris N, Waldrop S, Johnson V, Boaventura B, Kendrick K, Stanford FC. GLP-1 single, dual, and triple receptor agonists for treating type 2 diabetes and obesity: a narrative review. EClinicalMedicine. (2024) 75:102782. doi: 10.1016/j.eclinm.2024.102782
6. Marso SP, Daniels GH, Brown-Frandsen K, Kristensen P, Mann JFE, Nauck MA, et al. Liraglutide and cardiovascular outcomes in type 2 diabetes. N Engl J Med. (2016) 375(4):311–22. doi: 10.1056/NEJMoa1603827
7. Marso SP, Bain SC, Consoli A, Eliaschewitz FG, Jódar E, Leiter LA, et al. Semaglutide and cardiovascular outcomes in patients with type 2 diabetes. N Engl J Med. (2016) 375(19):1834–44. doi: 10.1056/NEJMoa1607141
8. Gerstein HC, Colhoun HM, Dagenais GR, Diaz R, Lakshmanan M, Pais P, et al. Dulaglutide and cardiovascular outcomes in type 2 diabetes (REWIND): a double-blind, randomised placebo-controlled trial. Lancet Lond Engl. (2019) 394(10193):121–30. doi: 10.1016/S0140-6736(19)31149-3
9. Hernandez AF, Green JB, Janmohamed S, Agostino D, Granger RB, Jones CB, et al. Albiglutide and cardiovascular outcomes in patients with type 2 diabetes and cardiovascular disease (harmony outcomes): a double-blind, randomised placebo-controlled trial. Lancet Lond Engl. (2018) 392(10157):1519–29. doi: 10.1016/S0140-6736(18)32261-X
10. Pfeffer MA, Claggett B, Diaz R, Dickstein K, Gerstein HC, Køber LV, et al. Lixisenatide in patients with type 2 diabetes and acute coronary syndrome. N Engl J Med. (2015) 373(23):2247–57. doi: 10.1056/NEJMoa1509225
11. Holman RR, Bethel MA, Mentz RJ, Thompson VP, Lokhnygina Y, Buse JB, et al. Effects of once-weekly exenatide on cardiovascular outcomes in type 2 diabetes. N Engl J Med. (2017) 377(13):1228–39. doi: 10.1056/NEJMoa1612917
12. ElSayed NA, Aleppo G, Aroda VR, Bannuru RR, Brown FM, Bruemmer D, et al. 10. Cardiovascular disease and risk management: standards of care in diabetes-2023. Diabetes Care. (2023) 46(1):S158–90. doi: 10.2337/dc23-S010
13. Adriaenssens AE, Gribble FM, Reimann F. The glucose-dependent insulinotropic polypeptide signaling axis in the central nervous system. Peptides. (2020) 125:170194. doi: 10.1016/j.peptides.2019.170194
14. Zhang Q, Delessa CT, Bakhti AR, Colldén M, Drucker G, J D, et al. The glucose-dependent insulinotropic polypeptide (GIP) regulates body weight and food intake via CNS-GIPR signaling. Cell Metab. (2021) 33(4):833–844.e5. doi: 10.1016/j.cmet.2021.01.015
15. Sattar N, McGuire DK, Pavo I, Weerakkody GJ, Nishiyama H, Wiese RJ, et al. Tirzepatide cardiovascular event risk assessment: a pre-specified meta-analysis. Nat Med. (2022) 28(3):591–8. doi: 10.1038/s41591-022-01707-4
16. Blonde L, Pencek R, MacConell L. Association among weight change, glycemic control, and markers of cardiovascular risk with exenatide once weekly: a pooled analysis of patients with type 2 diabetes. Cardiovasc Diabetol. (2015) 14:12. doi: 10.1186/s12933-014-0171-2
17. Bethel MA, Patel RA, Merrill P, Lokhnygina Y, Buse JB, Mentz RJ, et al. Cardiovascular outcomes with glucagon-like peptide-1 receptor agonists in patients with type 2 diabetes: a meta-analysis. Lancet Diabetes Endocrinol. (2018) 6(2):105–13. doi: 10.1016/S2213-8587(17)30412-6
18. Xu D, Nair A, Sigston C, Ho C, Li J, Yang D, et al. Potential roles of glucagon-like peptide 1 receptor agonists (GLP-1 RAs) in nondiabetic populations. Cardiovasc Ther. (2022) 2022:6820377. doi: 10.1155/2022/6820377
19. Chen WR, Hu SY, Chen YD, Zhang Y, Qian G, Wang J, et al. Effects of liraglutide on left ventricular function in patients with ST-segment elevation myocardial infarction undergoing primary percutaneous coronary intervention. Am Heart J. (2015) 170(5):845–54. doi: 10.1016/j.ahj.2015.07.014
20. Iqbal J, Wu HX, Hu N, Zhou YH, Li L, Xiao F, et al. Effect of glucagon-like peptide-1 receptor agonists on body weight in adults with obesity without diabetes mellitus-a systematic review and meta-analysis of randomized control trials. Obes Rev Off J Int Assoc Study Obes. (2022) 23(6):e13435. doi: 10.1111/obr.13435
21. Sattar N, Lee MMY, Kristensen SL, Branch KRH, Prato D, Khurmi S, et al. Cardiovascular, mortality, and kidney outcomes with GLP-1 receptor agonists in patients with type 2 diabetes: a systematic review and meta-analysis of randomised trials. Lancet Diabetes Endocrinol. (2021) 9(10):653–62. doi: 10.1016/S2213-8587(21)00203-5
22. Ferhatbegović L, Mršić D, Macić-Džanković A. The benefits of GLP1 receptors in cardiovascular diseases. Front Clin Diabetes Healthc. (2023) 4:1293926. doi: 10.3389/fcdhc.2023.1293926
23. Esser N, Legrand-Poels S, Piette J, Scheen AJ, Paquot N. Inflammation as a link between obesity, metabolic syndrome and type 2 diabetes. Diabetes Res Clin Pract. (2014) 105(2):141–50. doi: 10.1016/j.diabres.2014.04.006
24. Nikolaidis LA, Doverspike A, Hentosz T, Zourelias L, Shen YT, Elahi D, et al. Glucagon-like peptide-1 limits myocardial stunning following brief coronary occlusion and reperfusion in conscious canines. J Pharmacol Exp Ther. (2005) 312(1):303–8. doi: 10.1124/jpet.104.073890
25. Dutour A, Abdesselam I, Ancel P, Kober F, Mrad G, Darmon P, et al. Exenatide decreases liver fat content and epicardial adipose tissue in patients with obesity and type 2 diabetes: a prospective randomized clinical trial using magnetic resonance imaging and spectroscopy. Diabetes Obes Metab. (2016) 18(9):882–91. doi: 10.1111/dom.12680
26. Iacobellis G, Mohseni M, Bianco SD, Banga PK. Liraglutide causes large and rapid epicardial fat reduction. Obes Silver Spring Md. (2017) 25(2):311–6. doi: 10.1002/oby.21718
27. Iacobellis G, Baroni MG. Cardiovascular risk reduction throughout GLP-1 receptor agonist and SGLT2 inhibitor modulation of epicardial fat. J Endocrinol Invest. (2022) 45(3):489–95. doi: 10.1007/s40618-021-01687-1
28. Malavazos AE, Iacobellis G, Dozio E, Basilico S, Di Vincenzo A, Dubini C, et al. Human epicardial adipose tissue expresses glucose-dependent insulinotropic polypeptide, glucagon, and glucagon-like peptide-1 receptors as potential targets of pleiotropic therapies. Eur J Prev Cardiol. (2023) 30(8):680–93. doi: 10.1093/eurjpc/zwad050
29. Bogers RP, Bemelmans WJE, Hoogenveen RT, Boshuizen HC, Woodward M, Knekt P, et al. Association of overweight with increased risk of coronary heart disease partly independent of blood pressure and cholesterol levels: a meta-analysis of 21 cohort studies including more than 300 000 persons. Arch Intern Med. (2007) 167(16):1720–8. doi: 10.1001/archinte.167.16.1720
30. Guh DP, Zhang W, Bansback N, Amarsi Z, Birmingham CL, Anis AH. The incidence of co-morbidities related to obesity and overweight: a systematic review and meta-analysis. BMC Public Health. (2009) 9:88. doi: 10.1186/1471-2458-9-88
31. Renehan AG, Tyson M, Egger M, Heller RF, Zwahlen M. Body-mass index and incidence of cancer: a systematic review and meta-analysis of prospective observational studies. Lancet Lond Engl. (2008) 371(9612):569–78. doi: 10.1016/S0140-6736(08)60269-X
32. Leblanc ES, O’Connor E, Whitlock EP, Patnode CD, Kapka T. Effectiveness of primary care-relevant treatments for obesity in adults: a systematic evidence review for the U.S. preventive services task force. Ann Intern Med. (2011) 155(7):434–47. doi: 10.7326/0003-4819-155-7-201110040-00006
33. Pi-Sunyer X, Astrup A, Fujioka K, Greenway F, Halpern A, Krempf M, et al. A randomized, controlled trial of 3.0 mg of liraglutide in weight management. N Engl J Med. (2015) 373(1):11–22. doi: 10.1056/NEJMoa1411892
34. Vilsbøll T, Christensen M, Junker AE, Knop FK, Gluud LL. Effects of glucagon-like peptide-1 receptor agonists on weight loss: systematic review and meta-analyses of randomised controlled trials. Br Med J. (2012) 344:d7771. doi: 10.1136/bmj.d7771
35. Zhang P, Liu Y, Ren Y, Bai J, Zhang G, Cui Y. The efficacy and safety of liraglutide in the obese, non-diabetic individuals: a systematic review and meta-analysis. Afr Health Sci. (2019) 19(3):2591–9. doi: 10.4314/ahs.v19i3.35
36. Mehta A, Marso SP, Neeland IJ. Liraglutide for weight management: a critical review of the evidence. Obes Sci Pract. (2017) 3(1):3–14. doi: 10.1002/osp4.84
37. Rosenstock J, Klaff LJ, Schwartz S, Northrup J, Holcombe JH, Wilhelm K, et al. Effects of exenatide and lifestyle modification on body weight and glucose tolerance in obese subjects with and without pre-diabetes. Diabetes Care. (2010) 33(6):1173–5. doi: 10.2337/dc09-1203
38. Su N, Li Y, Xu T, Li L, Kwong JSW, Du H, et al. Exenatide in obese or overweight patients without diabetes: a systematic review and meta-analyses of randomized controlled trials. Int J Cardiol. (2016) 219:293–300. doi: 10.1016/j.ijcard.2016.06.028
39. Ma H, Lin YH, Dai LZ, Lin CS, Huang Y, Liu SY. Efficacy and safety of GLP-1 receptor agonists versus SGLT-2 inhibitors in overweight/obese patients with or without diabetes mellitus: a systematic review and network meta-analysis. BMJ Open. (2023) 13(3):e061807. doi: 10.1136/bmjopen-2022-061807
40. Wilding JPH, Batterham RL, Calanna S, Davies M, Van Gaal LF, Lingvay I, et al. Once-weekly semaglutide in adults with overweight or obesity. N Engl J Med. (2021) 384(11):989–1002. doi: 10.1056/NEJMoa2032183
41. Tan HC, Dampil OA, Marquez MM. Efficacy and safety of semaglutide for weight loss in obesity without diabetes: a systematic review and meta-analysis. J ASEAN Fed Endocr Soc. (2022) 37(2):65–72. doi: 10.15605/jafes.037.02.14
42. Bhagavathula AS, Vidyasagar K, Tesfaye W. Efficacy and safety of tirzepatide in patients with type 2 diabetes mellitus: a systematic review and meta-analysis of randomized phase II/III trials. Pharm Basel Switz. (2021) 14(10):991. doi: 10.3390/ph14100991
43. Jastreboff AM, Aronne LJ, Ahmad NN, Wharton S, Connery L, Alves B, et al. Tirzepatide once weekly for the treatment of obesity. N Engl J Med. (2022) 387(3):205–16. doi: 10.1056/NEJMoa2206038
44. Research C for DE and FDA. FDA. (2021). FDA approves weight management drug for patients aged 12 and older. Available from: Available online at: https://www.fda.gov/drugs/news-events-human-drugs/fda-approves-weight-management-drug-patients-aged-12-and-older (cited May 5, 2024).
45. Commissioner O of the FDA. FDA. (2021). FDA Approves New Drug Treatment for Chronic Weight Management, First Since 2014. Available from: Available online at: https://www.fda.gov/news-events/press-announcements/fda-approves-new-drug-treatment-chronic-weight-management-first-2014 (cited May 5, 2024).
46. Commissioner O of the FDA. FDA. (2023). FDA Approves New Medication for Chronic Weight Management. Available from: Available online at: https://www.fda.gov/news-events/press-announcements/fda-approves-new-medication-chronic-weight-management (cited May 5, 2024).
47. Powell-Wiley TM, Poirier P, Burke LE, Després JP, Gordon-Larsen P, Lavie CJ, et al. Obesity and cardiovascular disease: a scientific statement from the American Heart Association. Circulation. (2021) 143(21):e984–1010. doi: 10.1161/CIR.0000000000000973
48. Wilding JPH, Jacob S. Cardiovascular outcome trials in obesity: a review. Obes Rev Off J Int Assoc Study Obes. (2021) 22(1):e13112. doi: 10.1111/obr.13112
49. Malhotra A, Grunstein RR, Fietze I, Weaver TE, Redline S, Azarbarzin A, et al. Tirzepatide for the treatment of obstructive sleep apnea and obesity. N Engl J Med. (2024) 391(13):1193–205. doi: 10.1056/NEJMoa2404881
50. Blackman A, Foster GD, Zammit G, Rosenberg R, Aronne L, Wadden T, et al. Effect of liraglutide 3.0 mg in individuals with obesity and moderate or severe obstructive sleep apnea: the SCALE sleep apnea randomized clinical trial. Int J Obes. (2016) 40(8):1310–9. doi: 10.1038/ijo.2016.52
51. Patel Chavez C, Cusi K, Kadiyala S. The emerging role of glucagon-like peptide-1 receptor agonists for the management of NAFLD. J Clin Endocrinol Metab. (2022) 107(1):29–38. doi: 10.1210/clinem/dgab578
52. Ussher JR, Drucker DJ. Glucagon-like peptide 1 receptor agonists: cardiovascular benefits and mechanisms of action. Nat Rev Cardiol. (2023) 20(7):463–74. doi: 10.1038/s41569-023-00849-3
53. GBD 2015 Risk Factors Collaborators. Global, regional, and national comparative risk assessment of 79 behavioural, environmental and occupational, and metabolic risks or clusters of risks, 1990–2015: a systematic analysis for the global burden of disease study 2015. Lancet Lond Engl. (2016) 388(10053):1659–724. doi: 10.1016/S0140-6736(16)31679-8
54. Blood Pressure Lowering Treatment Trialists’ Collaboration. Age-stratified and blood-pressure-stratified effects of blood-pressure-lowering pharmacotherapy for the prevention of cardiovascular disease and death: an individual participant-level data meta-analysis. Lancet Lond Engl. (2021) 398(10305):1053–64. doi: 10.1016/S0140-6736(21)01921-8
55. Kolovou GD, Watts GF, Mikhailidis DP, Pérez-Martínez P, Mora S, Bilianou H, et al. Postprandial hypertriglyceridaemia revisited in the era of non-fasting lipid profile testing: a 2019 expert panel statement, main text. Curr Vasc Pharmacol. (2019) 17(5):498–514. doi: 10.2174/1570161117666190507110519
56. Berberich AJ, Hegele RA. Lipid effects of glucagon-like peptide 1 receptor analogs. Curr Opin Lipidol. (2021) 32(3):191–9. doi: 10.1097/MOL.0000000000000750
57. Papazafiropoulou AK, Melidonis A, Antonopoulos S. Effects of glucagon-like peptide-1 receptor agonists and sodium-glucose cotransporter 2 inhibitors on cardiorenal and metabolic outcomes in people without diabetes. Curr Pharm Des. (2021) 27(8):1035–42. doi: 10.2174/1381612826666200909142126
58. Song X, Jia H, Jiang Y, Wang L, Zhang Y, Mu Y, et al. Anti-atherosclerotic effects of the glucagon-like peptide-1 (GLP-1) based therapies in patients with type 2 diabetes Mellitus: a meta-analysis. Sci Rep. (2015) 5:10202. doi: 10.1038/srep10202
59. Husain M, Birkenfeld AL, Donsmark M, Dungan K, Eliaschewitz FG, Franco DR, et al. Oral semaglutide and cardiovascular outcomes in patients with type 2 diabetes. N Engl J Med. (2019) 381(9):841–51. doi: 10.1056/NEJMoa1901118
60. Boyle JG, Livingstone R, Petrie JR. Cardiovascular benefits of GLP-1 agonists in type 2 diabetes: a comparative review. Clin Sci Lond Engl. (2018) 132(15):1699–709. doi: 10.1042/CS20171299
61. Urquhart S, Willis S. Long-acting GLP-1 receptor agonists: findings and implications of cardiovascular outcomes trials. JAAPA Off J Am Acad Physician Assist. (2020) 33(8):19–30. doi: 10.1097/01.JAA.0000669452.63883.45
62. McGuire DK, Busui RP, Deanfield J, Inzucchi SE, Mann JFE, Marx N, et al. Effects of oral semaglutide on cardiovascular outcomes in individuals with type 2 diabetes and established atherosclerotic cardiovascular disease and/or chronic kidney disease: design and baseline characteristics of SOUL, a randomized trial. Diabetes Obes Metab. (2023) 25(7):1932–41. doi: 10.1111/dom.15058
63. Giugliano D, Scappaticcio L, Longo M, Caruso P, Maiorino MI, Bellastella G, et al. GLP-1 receptor agonists and cardiorenal outcomes in type 2 diabetes: an updated meta-analysis of eight CVOTs. Cardiovasc Diabetol. (2021) 20(1):189. doi: 10.1186/s12933-021-01366-8
64. Giugliano D, Longo M, Signoriello S, Maiorino MI, Solerte B, Chiodini P, et al. The effect of DPP-4 inhibitors, GLP-1 receptor agonists and SGLT-2 inhibitors on cardiorenal outcomes: a network meta-analysis of 23 CVOTs. Cardiovasc Diabetol. (2022) 21(1):42. doi: 10.1186/s12933-022-01474-z
65. Zhao H, Liu Y, Liu M, Xu Y, Ling Q, Lin W, et al. Clinical outcomes with GLP-1 receptor agonists in patients with heart failure: a systematic review and meta-analysis of randomized controlled trials. Drugs. (2023) 83(14):1293–307. doi: 10.1007/s40265-023-01932-2
66. Qin J, Song L. Glucagon-like peptide-1 (GLP-1) receptor agonists and cardiovascular events in patients with type 2 diabetes mellitus: a meta-analysis of double-blind, randomized, placebo-controlled clinical trials. BMC Endocr Disord. (2022) 22(1):125. doi: 10.1186/s12902-022-01036-0
67. Lincoff AM, Brown-Frandsen K, Colhoun HM, Deanfield J, Emerson SS, Esbjerg S, et al. Semaglutide and cardiovascular outcomes in obesity without diabetes. N Engl J Med. (2023) 389(24):2221–32. doi: 10.1056/NEJMoa2307563
68. Nicholls SJ, Bhatt DL, Buse JB, Prato SD, Kahn SE, Lincoff AM, et al. Comparison of tirzepatide and dulaglutide on major adverse cardiovascular events in participants with type 2 diabetes and atherosclerotic cardiovascular disease: SURPASS-CVOT design and baseline characteristics. Am Heart J. (2024) 267:1–11. doi: 10.1016/j.ahj.2023.09.007
69. Margulies KB, Hernandez AF, Redfield MM, Givertz MM, Oliveira GH, Cole R, et al. Effects of liraglutide on clinical stability among patients with advanced heart failure and reduced ejection fraction: a randomized clinical trial. JAMA. (2016) 316(5):500–8. doi: 10.1001/jama.2016.10260
70. Jorsal A, Kistorp C, Holmager P, Tougaard RS, Nielsen R, Hänselmann A, et al. Effect of liraglutide, a glucagon-like peptide-1 analogue, on left ventricular function in stable chronic heart failure patients with and without diabetes (LIVE)-a multicentre, double-blind, randomised, placebo-controlled trial. Eur J Heart Fail. (2017) 19(1):69–77. doi: 10.1002/ejhf.657
71. Kosiborod MN, Abildstrøm SZ, Borlaug BA, Butler J, Rasmussen S, Davies M, et al. Semaglutide in patients with heart failure with preserved ejection fraction and obesity. N Engl J Med. (2023) 389(12):1069–84. doi: 10.1056/NEJMoa2306963
72. Kosiborod MN, Abildstrøm SZ, Borlaug BA, Butler J, Christensen L, Davies M, et al. Design and baseline characteristics of STEP-HFpEF program evaluating semaglutide in patients with obesity HFpEF phenotype. JACC Heart Fail. (2023) 11(8 Pt 1):1000–10. doi: 10.1016/j.jchf.2023.05.010
73. Kato ET, Lewis BS, Ono K. GLP-1 receptor agonists: new game changing drugs in patients with heart failure with preserved ejection fraction and obesity. Eur Heart J Cardiovasc Pharmacother. (2023) 9(8):679–80. doi: 10.1093/ehjcvp/pvad072
74. Chaudhuri A, Ghanim H, Vora M, Sia CL, Korzeniewski K, Dhindsa S, et al. Exenatide exerts a potent antiinflammatory effect. J Clin Endocrinol Metab. (2012) 97(1):198–207. doi: 10.1210/jc.2011-1508
75. Al-Sadawi M, Aslam F, Aleem S, Alsadaoee M, Stevens GA, Singh A, et al. Association of glp-1 agonists with arrhythmias and sudden cardiac death in patients with type 2 diabetes: a meta-analysis of 27 randomized controlled trials. J Am Coll Cardiol. (2022) 79(9):1525. doi: 10.1016/S0735-1097(22)02516-5
76. Al-Sadawi MA, Aslam FM, Tao M, Alsaiqali M, Almasry IO, Fan R, et al. Effects of GLP-1 agonists on mortality and arrhythmias in patients with type II diabetes. Int J Cardiol Heart Vasc. (2023) 47:101218. doi: 10.1016/j.ijcha.2023.101218
77. Wei J, Wang R, Ye H, Wang Y, Wang L, Zhang X. Effects of GLP-1 receptor agonists on arrhythmias and its subtypes in patients with type 2 diabetes: a systematic review and meta-analysis. Front Endocrinol. (2022) 13:910256. doi: 10.3389/fendo.2022.910256
78. Shi W, Zhang W, Zhang D, Ren G, Wang P, Gao L, et al. Comparison of the effect of glucose-lowering agents on the risk of atrial fibrillation: a network meta-analysis. Heart Rhythm. (2021) 18(7):1090–6. doi: 10.1016/j.hrthm.2021.03.007
79. Liu Z, Bian N, Wu S, Fan Y, Li H, Yu J, et al. A meta-analysis evaluating indirectly GLP-1 receptor agonists and arrhythmias in patients with type 2 diabetes and myocardial infarction. Front Cardiovasc Med. (2022) 9:1019120. doi: 10.3389/fcvm.2022.1019120
80. Zhang HD, Ding L, Liu K, Mi LJ, Zhang AK, Yu FY, et al. Semaglutide for the prevention of atrial fibrillation: a systematic review and meta-analysis. Diabetes Metab Syndr. (2024) 18(6):103067. doi: 10.1016/j.dsx.2024.103067
81. Costa G, Resende B, Cunha G, Guimaraes J, Fernandes D, Monteiro R, et al. Semaglutide and atrial fibrillation: a systematic review and meta-analysis of randomized clinical trials. Eur Heart J. (2024) 45(Supplement_1):ehae666.442. doi: 10.1093/eurheartj/ehae666.442
82. Muzurović E, Mikhailidis DP. Impact of glucagon-like peptide 1 receptor agonists and sodium-glucose transport protein 2 inhibitors on blood pressure and lipid profile. Expert Opin Pharmacother. (2020) 21(17):2125–35. doi: 10.1080/14656566.2020.1795132
83. Jia X, Alam M, Ye Y, Bajaj M, Birnbaum Y. GLP-1 receptor agonists and cardiovascular disease: a meta-analysis of recent cardiac outcome trials. Cardiovasc Drugs Ther. (2018) 32(1):65–72. doi: 10.1007/s10557-018-6773-2
84. Andrikou E, Tsioufis C, Andrikou I, Leontsinis I, Tousoulis D, Papanas N. GLP-1 receptor agonists and cardiovascular outcome trials: an update. Hell J Cardiol. (2019) 60(6):347–51. doi: 10.1016/j.hjc.2018.11.008
85. Sposito AC, Berwanger O, de Carvalho LSF, Saraiva JFK. GLP-1RAs in type 2 diabetes: mechanisms that underlie cardiovascular effects and overview of cardiovascular outcome data. Cardiovasc Diabetol. (2018) 17(1):157. doi: 10.1186/s12933-018-0800-2
86. Vrints C, Andreotti F, Koskinas KC, Rossello X, Adamo M, Ainslie J, et al. 2024 ESC guidelines for the management of chronic coronary syndromes. Eur Heart J. (2024) 45(36):3415–537. doi: 10.1093/eurheartj/ehae177
87. Koskinas KC, Van Craenenbroeck EM, Antoniades C, Blüher M, Gorter TM, Hanssen H, et al. Obesity and cardiovascular disease: an ESC clinical consensus statement. Eur Heart J. (2024) 45:ehae508. doi: 10.1093/eurheartj/ehae508
88. Scirica BM, Lincoff AM, Lingvay I, Bogdanski P, Buscemi S, Colhoun H, et al. The effect of semaglutide on mortality and COVID-19-related deaths: an analysis from the SELECT trial. J Am Coll Cardiol. (2024) 84(17):1632–42. doi: 10.1016/j.jacc.2024.08.007
89. Prasad-Reddy L, Isaacs D. A clinical review of GLP-1 receptor agonists: efficacy and safety in diabetes and beyond. Drugs Context. (2015) 4:212283. doi: 10.7573/dic.212283
90. Sharma A, Parachuri N, Kumar N, Saboo B, Tripathi HN, Kuppermann BD, et al. Semaglutide and the risk of diabetic retinopathy-current perspective. Eye Lond Engl. (2022) 36(1):10–1. doi: 10.1038/s41433-021-01741-5
91. Cigrovski Berkovic M, Strollo F. Semaglutide-eye-catching results. World J Diabetes. (2023) 14(4):424–34. doi: 10.4239/wjd.v14.i4.424
Keywords: cardiovascular disease, cardiovascular outcome, glucagon-like peptide-1 receptor agonists, heart failure, obesity
Citation: Turkistani Y (2025) Glucagon-like peptide-1 receptor agonists: a review from a cardiovascular perspective. Front. Cardiovasc. Med. 12:1535134. doi: 10.3389/fcvm.2025.1535134
Received: 26 November 2024; Accepted: 28 March 2025;
Published: 24 April 2025.
Edited by:
Preetha Shridas, University of Kentucky, United StatesReviewed by:
Aneesh Dhore-patil, Houston Methodist Hospital, United StatesRaffaele Carraro, La Princesa University Hospital, Spain
Copyright: © 2025 Turkistani. This is an open-access article distributed under the terms of the Creative Commons Attribution License (CC BY). The use, distribution or reproduction in other forums is permitted, provided the original author(s) and the copyright owner(s) are credited and that the original publication in this journal is cited, in accordance with accepted academic practice. No use, distribution or reproduction is permitted which does not comply with these terms.
*Correspondence: Yosra Turkistani, WW9zcmEuVHVya2lzdGFuaUBvdXRsb29rLmNvbQ==