- 1Section of Periodontics, School of Dentistry, University of California, Los Angeles, Los Angeles, CA, United States
- 2School of Dentistry, University of California, Los Angeles, Los Angeles, CA, United States
- 3Division of Advanced Prosthodontics, Weintraub Center for Reconstructive Biotechnology, School of Dentistry, University of California, Los Angeles, Los Angeles, CA, United States
- 4Laboratory for Digital Dental Technologies, Department of Reconstructive Dentistry and Gerodontology, School of Dental Medicine, University of Bern, Bern, Switzerland
- 5Department of Restorative, Preventive, and Pediatric Dentistry, School of Dental Medicine, University of Bern, Bern, Switzerland
- 6Department of Chemistry and Biochemistry, University of California, Los Angeles, Los Angeles, CA, United States
- 7Department of Pathology and Laboratory Medicine, David Geffen School of Medicine, University of California, Los Angeles, Los Angeles, CA, United States
- 8California NanoSystems Institute, University of California, Los Angeles, Los Angeles, CA, United States
- 9Jonsson Comprehensive Cancer Center, University of California, Los Angeles, Los Angeles, CA, United States
Implant-related oral diseases such as peri-implantitis and peri-mucositis are largely initiated by bacterial colonization on artificial implant surfaces. Therefore, implant and abutment material characteristics that minimize bacterial attachment and subsequent biofilm formation are important factors in reducing the risk of infection-related implant failure. This study compares the properties of two different titanium-based implant coating materials, titanium nitride (TiN) and titanium carbon nitride (TiCN). Surface hydrophilicity/ hydrophobicity and roughness were evaluated via contact angle measurements and surface profiling with white light interferometry, respectively. TiN-coated surfaces were hydrophobic according to its contact angle higher than 72.7°, whereas TiCN-coated surfaces were hydrophilic with its contact angle of 53.6°. The average roughness (Ra) was greater for TiCN than TiN with the root mean square roughness (Rq) being significantly higher. These findings are in contrast to the common understanding for titanium-based materials that surface roughness and hydrophobicity are positively correlated. A well-established saliva-based oral microbial biofilm model was employed to compare bacterial attachment and biofilm formation on TiN and TiCN. Growth conditions included relevant host components such as blood as well as the presence or absence of dietary carbohydrates. The accumulated biomass was measured by crystal violet staining and the bacterial community profiles of the attached biofilms were determined via 16S rRNA gene microbiome sequencing at different time points over a 7-day period. At all time points, TiCN showed significantly less bacterial attachment and biofilm formation compared to TiN. This implied the importance of the hydrophilic state over surface roughness as parameter for the prevention of oral microbial attachment. Although, the biofilm community composition was very similar on both materials, environmental growth conditions resulted in significantly different bacterial profiles independent of the surface. In conclusion, TiCN coating produced a unique titanium surface which is rougher but more hydrophilic. TiCN-coated surfaces exhibited reduced bacterial attachment and biofilm formation in comparison to TiN coating. This coating technique can be further explored to improve implant and abutment success.
Introduction
Dental implants and implant-supported dentures are widely accepted prosthetic replacements for untimely tooth loss in patients facing functional and esthetic problems. However, the long-term maintenance of dental implants has become a persistent challenge due to complications related to peri-implant tissue-associated diseases such as peri-mucositis and peri-implantitis (1–3). While peri-implant mucositis is a reversible condition involving inflammation of the soft tissues surrounding implant material, peri-implantitis results in irreversible loss of the bone supporting the implant due to inflammation (2, 4–6). The etiology of these diseases is multi-factorial involving host factors, implant material, as well as implant-associated plaque buildup and composition. Among these factors, biofilm accumulation around the implant is a leading cause of inflammation and eventual implant failure (7–9). This detrimental consequence highlights the significant need to identify dental implant materials with drastically reduced affinity for oral microbial colonization.
Mechanical and surface features are important factors in the clinical success of dental implants. Desirable properties of implant materials include being conducive to attachment of bone-generating cells for effective osseointegration and low adherence of microbial cells to reduce biofilm development and subsequent complications of peri-implant infections. Since the inception of dental implants in the 1960s by Branemark et al. (10), the field has focused on the development of implant materials that are biocompatible and resistant to fracture or corrosion (11, 12). To date, titanium and biomedical titanium alloys are the most preferred materials for the fabrication of dental implants (13–15). There are, however, controversial reports regarding the antibacterial nature of titanium. While some studies found that titanium exhibits antibacterial properties (16), other studies showed no inhibitory effect of titanium on oral bacteria (17, 18). Although titanium is considered a “gold standard” for the fabrication of implant materials, an ideal material with all of the desirable properties has not been established.
Surface coating is often employed to improve wear and corrosion resistance along with the hardness of the implant and abutment materials (19–23). Currently, several different types of surface modifications are being utilized for further improvement of titanium and titanium alloys (24). Of these, titanium nitride (TiN) (25, 26) and more recently diamond- like carbon coatings like titanium carbon nitride (TiCN) are gaining popularity due to their tribological properties that render them suitable for biomedical applications (27–31). In addition to favorable physical and chemical properties, ideal implant and abutment materials should also possess antibacterial activities to reduce the risk of infection and implant failure. Though widely investigated, contradictory reports exist for the antibacterial effect of TiN coatings. While several in vitro studies suggested that surface coatings like TiN enhance the antibacterial property of the material (32–34), others reported no significant difference (25, 35). Similarly, an enhanced antibacterial effect of TiCN coatings has been reported against Staphylococcus epidermidis and S. aureus (36), the main pathogens involved in orthopedic implant failure. Most of the aforementioned studies investigated the antibacterial effect of surface coatings against a single species or a consortium of multiple oral species (37). However, with over 700 microbial species in the oral cavity (38, 39) the in-vivo conditions are significantly different from in-vitro conditions in regards to their complexity and composition (40, 41). Although, an in-vitro study (42) used saliva as a source of oral community to study the density and morphology of biofilm formed on various surfaces, the composition of the oral community was not assessed. To date, there are very few reports that use oral community for comprehensive assessment of the implant or abutment surfaces including the TiCN and TiN surface coatings.
In this study, we explored the potential clinical effects of these two surface coatings for abutment materials by characterizing the material-bacterial interaction of TiCN and TiN with the oral bacterial community. Our comprehensive approach included the investigation of the material surface properties, biomass, architecture, and microbial composition of the oral biofilm formed on these two surfaces.
Materials and Methods
Surface Characterization
In this study, two titanium-based implant abutment coatings; titanium carbon nitride (TiCN) and titanium nitride (TiN) were compared. The two materials were tested in form of coated titanium discs (a kind gift from Zest Dental solutions), which were standardized to 7 mm in diameter and 2 mm in thickness.
Surface Hydrophilicity (Wettability) Test
To assess surface hydrophilic properties of the TiCN- and TiN-coated discs, a sessile drop method was used (43). Briefly, a drop of 10 μl deionized water was placed on the surface of the disc and the angle was measured by a contact angle meter (CA-X, Kyowa Interface Science, Tokyo, Japan). Three samples from each of the implant material groups were used and all procedures were performed in a clean room under controlled conditions of 20°C and 46% humidity.
Optical Profilometry
The surface roughness of TiCN- and TiN-coated discs was characterized with white light interferometry profiling, which has the capability to resolve, large surface areas with sub-nanometer resolution. To allow better positioning of the discs for imaging, the samples were mounted on a glass slide. Surface measurements were obtained using a WYKO NT-1100 white light interferometer (Bruker, Tucson, AZ) (44). All images were collected in Vertical Scanning Interferometry (VSI) mode sampling. Data analyses, including surface roughness measurements, were calculated according to the corresponding parameters in the Bruker, Wyko Optical Profilometry software. Average parameters for identically sized 4–5 different regions selected by the operator as representative of each quadrant were used. The calculated surface roughness average (Ra), and root mean square roughness (Rq), were defined as the main height calculated over the entire measured length or area and as the average between the height deviations and the mean surface taken over the evaluation area, respectively (as defined in the software parameters for the Veeco, Wyko Optical Profiler).
Oral Microbial Community and Culture Conditions
The TiCN- and TiN-coated discs were sterilized by autoclaving at 121 psi for 30 min and placed into sterile 24-well culture plates prior to inoculation for biofilm growth. Human saliva-derived oral community was grown anaerobically (10% CO2, 10% H2, and 80% N2) at 37°C according to previously described protocols (45, 46). Briefly, 100 μl of a pooled saliva stock that was collected under UCLA-IRB 11-002483 was grown in 1 ml of SHI medium (45) for 17–18 h under anaerobic conditions. Prior to use, the overnight grown oral community was pelleted and washed with 1XPBS (phosphate-buffered saline). For biofilm seeding, cells corresponding to an optical density of 0.1 at 600 nm were diluted in 50% SHI medium alone or 50% SHI medium supplemented with 0.5% sucrose (Scr) and 0.5% mannose (Man). One milliliters of this diluted oral community was seeded into sterile 24-well polystyrene plates (Fisher Scientific) containing the sterilized discs and incubated under anaerobic conditions (10% CO2, 10% H2, and 80% N2) at 37°C for up to 7 days. The biofilm communities were assessed after 1 (day1), 3 (day3), and 7 (day7) days. For all experiments, medium was carefully removed at the end of the respective incubation period; the discs were gently transferred into new 24-well-polystyrene plates and washed one time with 1 ml sterile PBS prior to further processing.
Crystal Violet Assay
The biomass of the biofilms accumulated on the titanium discs with TiCN and TiN coatings was assessed using the crystal violet assay as described earlier (47). Briefly, the PBS-washed discs were placed into a 24-well-polystyrene plate and incubated in 1 ml of methanol for 15 min for fixation of the biofilm. After removal of the methanol, the biofilms were submerged in 1 ml of 0.5% crystal violet aqueous solution for 15 min. The discs were then carefully transferred to a new 24-well-polystyrene plate and washed four times with distilled water to remove excess crystal violet and ensure complete removal of residual dye. After the final wash, 1 ml of 95% ethanol was added and the plate was incubated at room temperature on a rotatory shaker at 100 rpm for 15 min. The ethanol solution containing the crystal violet stain retained by the biofilms was transferred into 1.5 ml cuvettes (USA Scientific) and the optical density at 570 nm was determined for total biomass evaluation. All experiments were performed as biological and technical triplicates.
Scanning Electron Microscopy
Bacterial biofilm morphology formed on the TiN- and TiCN-coated discs after a 7-day incubation period in the presence of Scr and Man was visualized via scanning electron microscopy (SEM, ZEISS Supra 40 VP) at the Electron Imaging Center for Nano Machines (EICN), University of California Los Angeles (UCLA) according to previously published protocol (48). Briefly, after 7 days of biofilm growth, the discs were washed gently in 1XPBS and fixed with 2.5% glutaraldehyde for 1 h followed by dehydration in a graded ethanol series of 10, 30, 50, 70, 90, and 100% absolute ethanol. The dehydrated specimens were further dried with a critical point drying apparatus (Tousimis Autosamdry-810), sputter-coated with Iridium (South Bay Technology Ion Beam Sputtering / Etching System), and imaged with SEM (49) at 10.0 kV electron high tension (EHT). The working distance was (WD) 17 mm and the images were captured with secondary electron (SE) mode.
DNA Extraction, PCR, and Denaturing Gradient Gel-Electrophoresis
Microbial cells were harvested from the TiCN- and TiN-coated discs by scraping with a sterile pipette tip in 500 μl of 1X PBS. The microbial cells were then pelleted and total genomic DNA was isolated using the Master Pure™ DNA purification kit (Epicenter) with some minor modifications (50). The concentration of bacterial DNA was determined with a Nanodrop 2000 (Thermo Scientific).
For denaturing gradient gel electrophoresis (DGGE), a 300 bp region of the 16S ribosomal RNA encoding gene was amplified from the genomic DNA using the universal 16S rRNA specific primers Bac1 with a GC clamp (5′-CGC CCG CCG CGC CCC GCG CCC GTC CCG CCG CCC CCG CCC GAC TAC GTG CCA GCA GCC-3′) and Bac2 (5′-GGA CTA CCA GGG TAT CTA ATC C-3′) (51). The PCR was performed in a total reaction volume of 50 μl, containing 25 μl of 2X GoTaq® Green Master Mix (Promega), 0.5 μM each of forward and reverse primers and 50 ng of genomic DNA. The PCR conditions consisted of an initial denaturation for 3 min at 95°C followed by 35 cycles of denaturing at 95°C for 1 min, annealing at 56°C for 1 min, and elongation at 72°C for 30 s. After 35 cycles of amplification, an additional elongation step was performed at 72°C for 5 min. Before separating the PCR products via DGGE, PCR amplification was confirmed by electrophoresis on a 1.0% agarose gel. Further, the Bio-Rad DCode™Universal Mutation Detection System (Bio-Rad Laboratories, Inc., Hercules, CA) was used for running the DGGE. Before the electrophoresis, gradient gels ranging from 40 to 60% denaturant were prepared with denaturing urea and formamide. Approximately 45 μl of the PCR product were loaded into each well and the PCR products were separated by electrophoresis at 60 V for 17–18 h at 58°C. After electrophoresis, the gels were stained with SYBR safe and imaged with the Molecular Imager Gel Documentation system (Bio-Rad Laboratories).
16S rRNA Gene Sequencing and Data Analysis
Microbiome sequencing of the 16S rRNA gene was performed according to the earth-microbiome project protocols (https://earthmicrobiome.org/protocols-and-standards/16s/) at Laragen Sequencing and Genotyping (Culver City, CA, USA). Briefly, the modified 506f (52) and modified 806r primers (53) were used for amplification of the V4 region of the 16S rRNA gene (506f: GTGYCAGCMGCCGCGGTAA and 806r: GGACTACNVGGGTWTCTAAT).
Demutiplexed paired-end sequences were obtained from Laragen Inc and imported into Qiime2 v2020.11 (54). Low quality sequences containing bases with Phred quality values <20 were trimmed and denoised using the DADA2 package (55). The amplicon sequence variants (ASVs) generated after merging the paired ends were taxonomically assigned by comparison to the HOMD database (56). Alpha and beta diversity analyses were performed using the core metrics plugin in QIIME 2. The Shannon's index diversity measure was used for calculating alpha diversity, while weighted unifrac was used for assessing beta diversity. For calculations, the data were rarefied to 34,900 sampling depth, the lowest number of sequences found amongst all samples.
Statistical Analysis
Statistical comparisons were performed using GraphPad Prism (version 9.1.0, GraphPad Software, San Diego, California USA). The significance of alpha diversity measures was assessed by the Kruskal Wallis test, and beta diversity measures were evaluated using analysis of similarity (ANOSIM) with 999 permutations in Qiime2 (54).
Results
Surface Characterization
The surface properties of two different titanium-based implants coatings: TiCN and TiN, were analyzed for hydrophilicity and roughness. Wettability assessment revealed a larger spread area of the water droplet on TiCN-coated titanium discs compared to the firm semispherical shape of the water droplet formed on TiN (Figure 1A). This translated into average contact angles of 53.6 ± 1.6° and 72.7 ± 2.4°, respectively, and thus significantly higher hydrophilicity of the TiCN-coated surface compared to the TiN-coated one (Figure 1B).
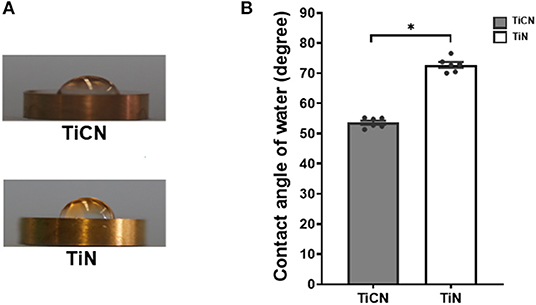
Figure 1. Hydrophilicity evaluation of titanium carbon nitride (TiCN) and titanium nitride (TiN) coatings by contact angle measurement of 10 μl ddH2O. (A) Representative images of 10 μl ddH2O placed on TiCN (upper panel) and TiN (lower panel) coatings. (B) A histogram representing the average contact angles with standard error of mean on TiCN (gray bars) and TiN (yellow bars) coatings. *indicates significant differences of p < 0.05.
Furthermore, the topography of the two different surface coatings was evaluated via interferometric analysis using white light. The measurements of Ra and Rq parameters revealed lower values for the TiN-coated discs in comparison to TiCN-coated discs (Figure 2A). While TiCN-coatings resulted in rougher surfaces (Ra: 0.94 ± 0.20 μm; Rq: 1.51 ± 0.38 μm) compared to TiN (Ra: 0.66 ± 0.02 μm; Rq: 0.88 ± 0.20 μm), only the difference in Rq was significant. Representative images are shown in Figure 2B.
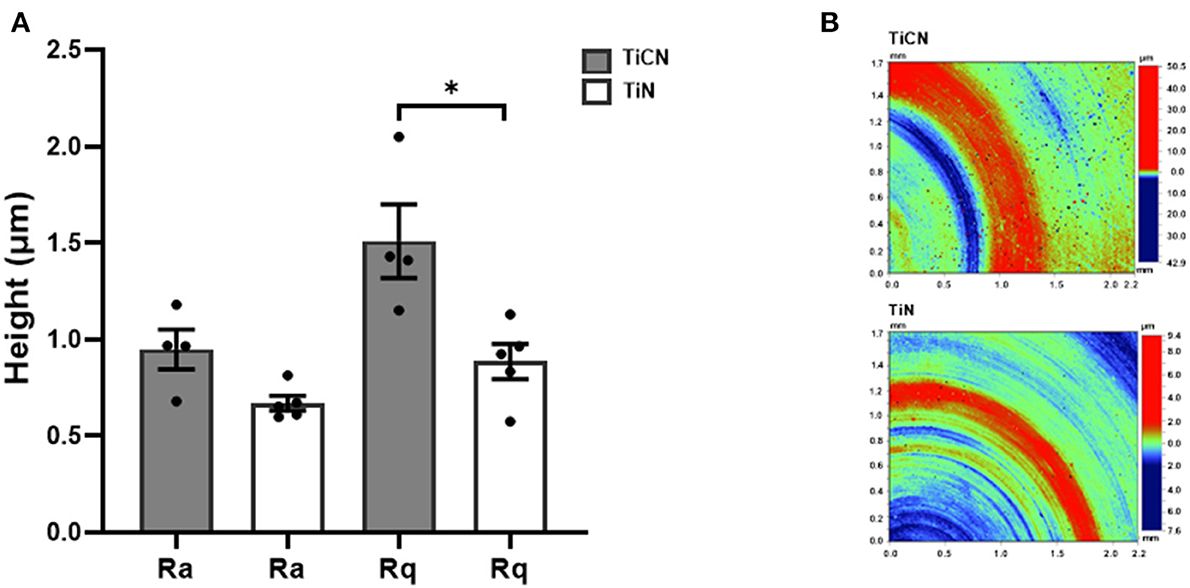
Figure 2. Roughness measurements of TiCN and TiN coatings by white light interferometry. (A) A histogram comparing the Ra (surface roughness average) and Rq (root mean square roughness) values between the two types of coatings. The average Ra and Rq values are shown with standard error of mean. (B) Representative images of TiCN (upper panel) and TiN (lower panel) coating measurements are shown. *indicates significant differences of p < 0.05.
Biofilm Formation
Next, the impact of differential surface properties of the coating materials on bacterial attachment and biofilm formation was investigated. Biofilm formation was assessed at three different time points (day1, day3, and day7) in the presence and absence of the carbohydrates Scr and Man (Figure 3). For the biofilm growth conditions without addition of Scr and Man only the day3 and day7 results are shown, since day1 biofilms were very loose and easily detached, which hampered accurate measurements (Figure 3A). Even though the biomass increased over time, biofilm accumulation overall remained significantly lower on the TiCN-coated discs compared to their TiN-coated counterparts independent of growth condition at all time points (Figure 3).
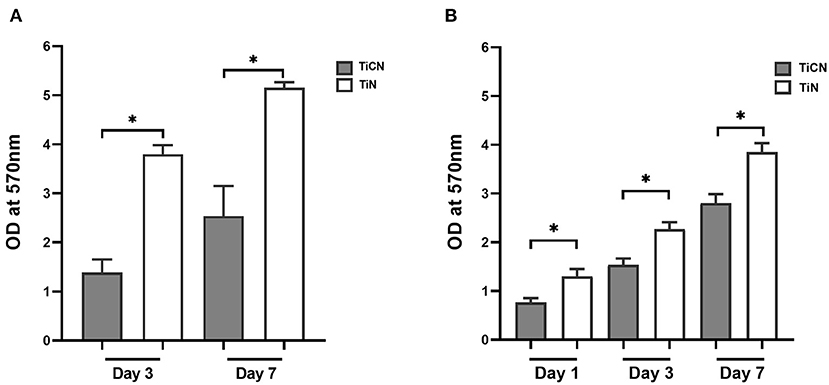
Figure 3. Biomass determination of oral microbial biofilm formed on the surfaces of TiCN- and TiN-coated discs using the crystal violet assay. Shown are the biomass of the biofilms formed on TiCN (gray bars) and TiN (yellow bars) in 50% SHI medium (A) without Scr and Man supplementation, and (B) in the presence of Scr and Man. *indicates significant differences of p < 0.05.
Further, the architecture of 7-day old biofilms grown in the presence of Scr and Man on both the TiCN- and TiN-coated discs was visualized with SEM (Figure 4). The biofilm architecture was distinct with long rod-shaped bacteria and cocci forming a loose network on the TiCN-coated discs (Figure 4A). In contrast, for the TiN-coated discs a dense mat of predominantly short rod-shaped bacteria was evident with an apparent lower number of cocci present (Figure 4B).
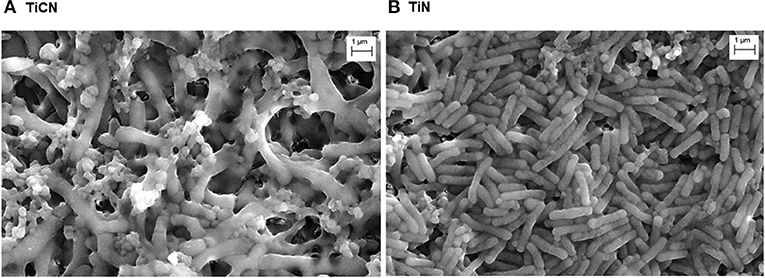
Figure 4. Visualization of oral microbial biofilms formed on (A) TiCN and (B) TiN coatings via scanning electron microscopy (SEM) after 7 days of biofilm growth in 50% SHI medium with Scr and Man.
Microbial Composition Analysis
Screening of biofilms by DGGE revealed that their overall community profile was very similar for both types of surface coating materials when grown under the same growth conditions (with/without Scr and Man) (Figure 5). However, the microbial community composition appeared to greatly vary depending on the presence or absence of Scr and Man. Addition of these carbohydrates resulted in lower diversity compared to biofilms grown in unsupplemented medium (Figures 5A,B).
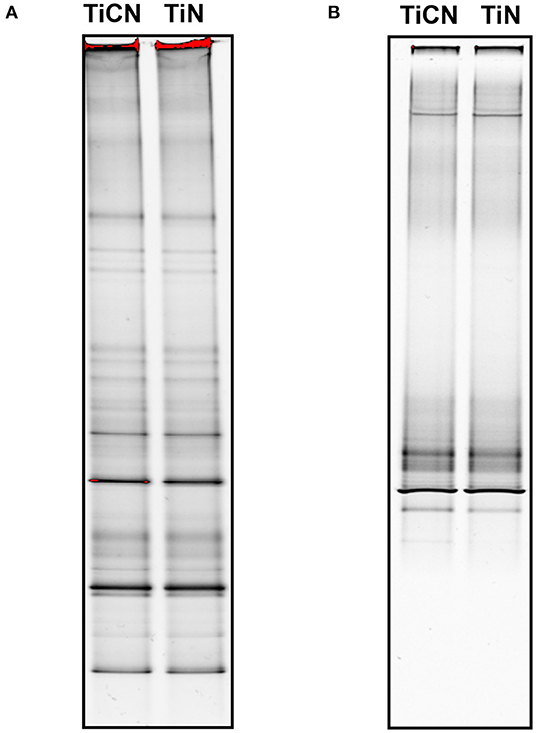
Figure 5. Denaturing gradient gel electrophoresis (DGGE) profiles of the oral microbial biofilms on TiCN and TiN coatings grown in 50% SHI medium (A) in the absence (B) and presence of Scr and Man.
Species-level microbial composition analysis via 16S rRNA gene sequencing further confirmed that the communities grown without Scr and Man were more diverse in contrast to the biofilms formed in their presence, independent of the type of disc surface coating (Figure 6). Under Scr and Man supplemented conditions, gram positive species such as members of the genus Streptococcus and Lactobacillus dominated the community. Specifically, on day3 the genus Streptococcus represented by S. agalactiae, S. salivarius, and S. anginosus comprised about 75% of the community on both TiCN and TiN, while the relative abundance of L. fermentum was 20.3% on TiCN and 22.3 % on TiN (Figure 6). Further incubation to day7 resulted in a decrease in relative abundance of streptococci to 42.3% on TiCN and 61.1% on TiN, which was accompanied by an increase of L. fermentum relative abundance to 37.9 and 35.1%, respectively. Although preferential attachment of Fusobacterium sp. HMT_203 and Porphyromonas pasteri was observed on TiCN-coated discs after 7 days of incubation compared to TiN, these differences were not significant. On the other hand, without addition of the carbohydrates, the community had a more diverse profile with comparable representation of both gram-positive and gram-negative species (Figure 6). Similar to biofilm growth in the presence of Scr and Man, no significant differences in microbial biofilm composition were observed between the two types of surface coatings. Although S. agalactiae was still the most dominant species at day3 (22.0–29.3% on TiCN and TiN, respectively) which reduced to 7.8 and 5.7%, respectively, on TiCN and TiN at day7, the levels of L. fermentum were drastically reduced both at the day3 and day7 time points.
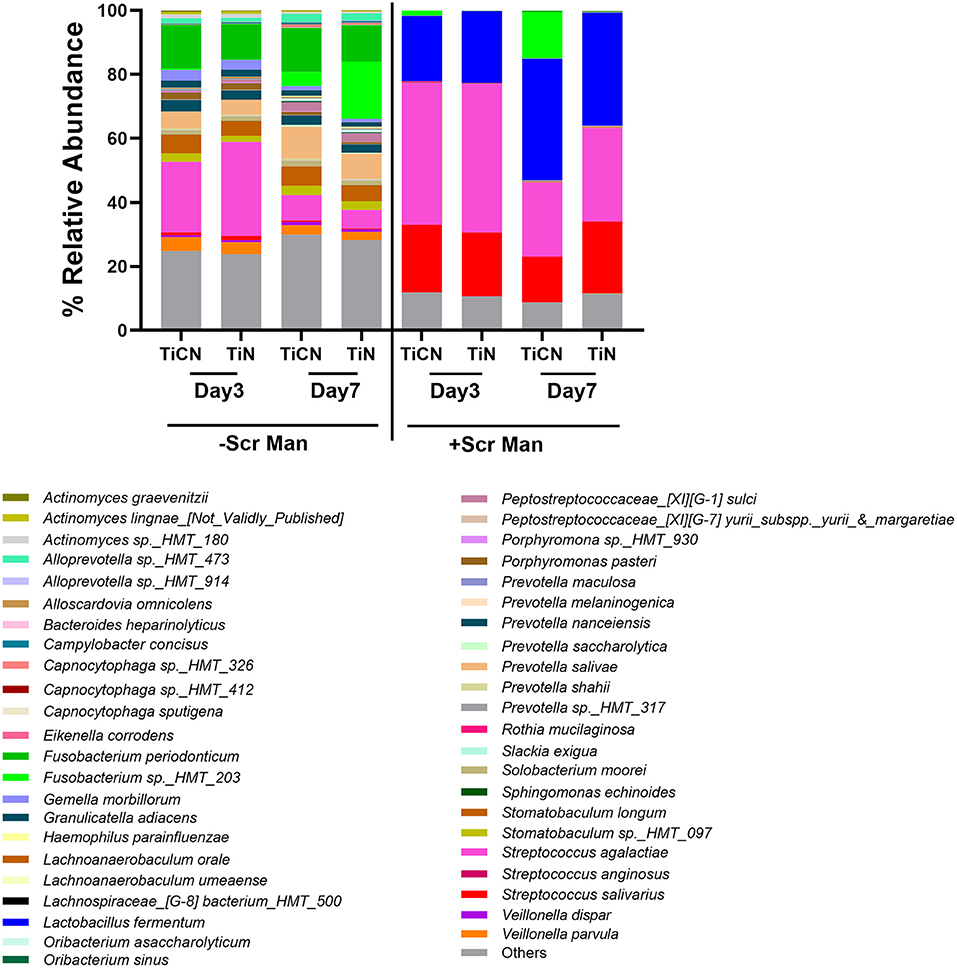
Figure 6. Species level microbial composition determination of TiCN and TiN surface-associated oral biofilms via 16S rRNA gene sequencing. Bar plots represent the relative abundance of species present in the oral biofilm communities formed on TiCN- and TiN-coated discs on day3 or day7 after growth in the absence or presence or of Scr and Man.
Alpha and Beta Diversity
Next, alpha diversity was compared between biofilm communities grown on TiCN and TiN surfaces in the presence and absence of Scr and Man by measuring the Shannon's index. While the biofilm growth conditions were a major driver of alpha diversity, the two different titanium-based surface coatings were not (Figure 7). Significantly lower alpha diversities were observed for biofilms grown in the presence of carbohydrates compared to those grown without, irrespective of the type of surface coating. In contrast, microbial communities that accumulated on TiCN- and TiN-coated discs under the same growth condition displayed no significant differences in alpha diversity.
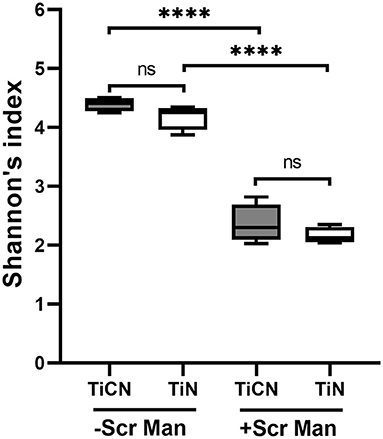
Figure 7. Alpha diversity analysis of TiCN and TiN surface coatings associated oral microbial biofilm communities. Box plots representing the alpha diversity of oral biofilms formed on TiCN (gray box plot) and TiN (yellow box plot) in the absence and presence of Scr and Man are shown. The whiskers represent minimum to maximum value and a line in the box represent the median. Alpha diversity is measured by Shannon's index representing mean species richness. ****indicates significant differences of p < 0.0001.
Further, the differences and similarities between the communities were assessed using the weighted unifrac distance measure. Similar to alpha diversity, no significant differences in beta diversity were observed for the biofilms based on the type of the titanium-based surface coating (TiCN or TiN) they were grown on (Figure 8A). The communities between the two different growth conditions, however, differed remarkably (Figure 8B). The biofilm communities grown with or without the addition of carbohydrates continued to change from day3 to day7 (Figure 8C) showing a significant shift in communities (p = 0.031 and 0.029, respectively).
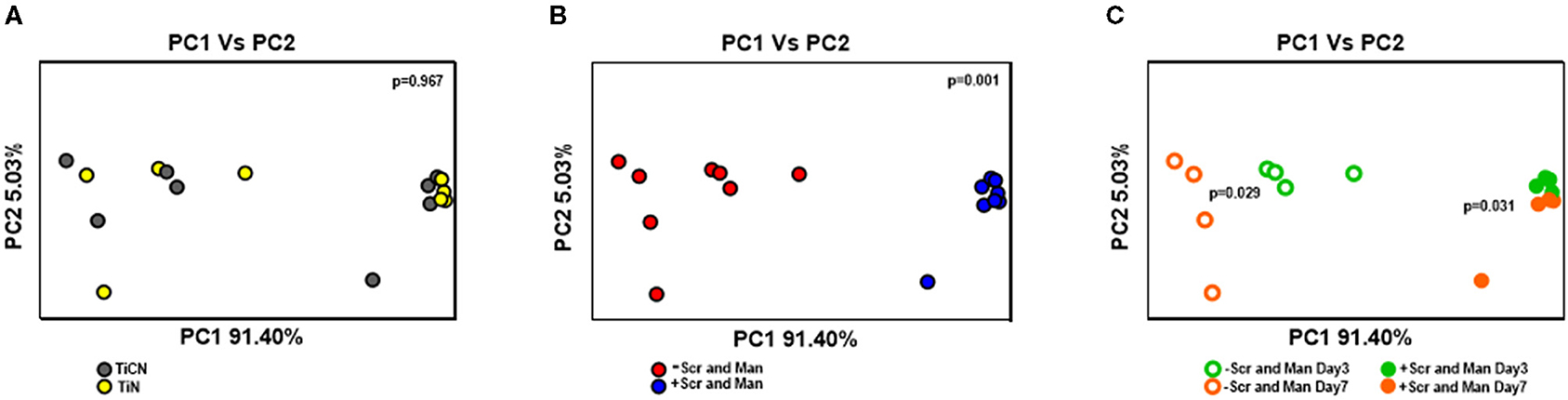
Figure 8. Beta diversity analysis of oral microbial biofilm communities formed on TiCN and TiN coatings evaluated by weighted unifrac. Principal coordinate analysis (PCoA) is plotted according to (A) the type of coating: TiCN (gray circles) and TiN (yellow circles) (B) growth conditions: without Scr and Man (red circles); with Scr and Man (blue circles) and (C) both growth conditions and time points: without and with Scr and Man day3 (open green circles and closed green circles, respectively) and without Scr and Man day7 (open orange circles and closed orange circles, respectively). The p-values obtained by analysis with 999 permutations in ANOSIM are shown with in the panels.
Discussion
Biofilm accumulation on implant and abutment materials remains a major cause of oral implant failure. In this study, the surface properties of two titanium-based implant abutment coatings, TiCN and TiN, and their effect on the biomass and composition of oral biofilms was investigated. The results of the study revealed that the TiCN-coated discs accumulated less oral biofilm biomass in comparison to the discs coated with TiN. However, the microbial composition of the community that attached to both these surfaces was very similar.
Microbial adhesion on a biomaterial surface can be attributed to several factors such as surface roughness, surface free energy (35) and chemical composition of the material (57–59). In this study, the surfaces of two titanium-based coatings were characterized using a wettability test and optical interferometry assays. While the wettability test revealed a more hydrophilic surface for the TiCN coating (Figure 1), the surface roughness parameters (Ra and Rq) suggested that TiCN was rougher than TiCN (Figure 2). This is an unusual observation for titanium-based materials, for which roughness is generally correlated with hydrophobicity instead of hydrophilicity and indicates that other factors may play a role. In addition, lower oral biofilm biomass accumulation was observed for TiCN-coated disc (Figure 3) suggesting that surface free energy/hydrophilicity and not surface roughness was the relevant factor in bacterial adhesion. Currently, there is no consensus regarding a lower biofilm accumulation to either hydrophilic or hydrophobic surfaces. In this study lower biofilm biomass was observed on a more hydrophilic surface. Similar to findings of this study, other studies have also demonstrated that hydrophilic surfaces accumulate less biofilm biomass (60–62).
Adhesion of bacteria on a surface is affected by a multitude of factors other than the physical and chemical properties of the material. In addition to the properties of the biomaterials, bacterial cell surface properties also affect adhesion (63), which can be very different in a heterogeneous microbial community. Therefore, it is necessary to assess the impact of an oral community that mimics the bacterial community present in in vivo conditions. In this study, we used well-established biofilm growth conditions that represented the diversity of oral bacterial community similar to the salivary microflora (45). Although in vitro conditions are significantly different than the in vivo conditions existing in the oral community (40, 41), investigation with oral community rather than single or multispecies biofilm is essential for getting relevant insight into the behavior of the dental materials.
The differential architecture of the biofilm grown on the TiCN and TiN coatings as observed via SEM further reveals the complexity of bacterial adhesion on a surface. Based on the surface properties, the initial bacterial population that adheres to the surface can be different resulting in a looser biofilm as seen on TiCN or a denser one as observed on TiN (Figure 4). However, a further investigation of the microbial composition, on the two titanium-based coatings revealed that the community that attached to both types of coatings was very similar (Figures 5, 6). The differences seen in the bacterial morphology can possibly be explained by the fact that the same bacterial species, based on the surface they attach to, can exhibit different morphological characteristics (64).
To further understand if microbial community differences can play a role in the bacterial attachment to the two different coatings, the oral biofilm was grown in the presence and absence of Scr and Man. As expected, 16S rRNA gene sequencing analysis revealed a less diverse community for biofilm growth conditions with carbohydrates which was more diverse in their absence. Addition of Scr and Man facilitate preferential growth of Streptococcus spp. while the absence results in a more diverse microbial community (65, 66). However, between the two different titanium-based coatings, there was no difference in the overall composition of the attached oral biofilm (Figure 6). A few other studies, utilizing single strand conformation polymorphism (SSCP) of the 16S rRNA gene have similarly reported that the oral biofilm composition did not change between various biomaterials tested (67, 68).
Alpha- and beta- diversity analyses (Figures 7, 8), further revealed that these measures were very similar between the two types of titanium-based coatings. Growth conditions, however, were the main drivers for diversity and dissimilarities/similarities between the communities. Interestingly, the oral communities in the absence of Scr and Man showed further diversification in bacterial composition between day3 and day7, while in the presence of Scr and Man the community differed mostly with respect to the abundance of Streptococcus and Lactobacillus species. This is in agreement with several other studies which suggest that addition of Scr and Man result in highly skewed communities with abundance of Streptococcus and Lactobacillus species (65, 66).
In conclusion, although both the titanium-based implant abutment coating surfaces showed similar composition of the bacterial species, biofilm accumulation on TiCN coating was significantly lower than on the TiN coating. For these surfaces, hydrophilicity seems to be the driving factor for the level of biofilm accumulation rather than the surface roughness. The TiCN coating tested in this study displayed the interesting characteristic of being more hydrophilic despite being rougher than the TiN-coated surface. To further establish possible clinical advantages of TiCN over TiN coating of implant abutment materials, in vivo assays are necessary including interaction with host environmental factors and tissue cells.
Data Availability Statement
The data presented in the study are deposited in the EBI (European Bioinformatics Institute) repository in ENA (European Nucleotide Archive) database. The accession numbers of the samples are ERS6646121 to ERS6646136 under the project PRJEB45983.
Author Contributions
BS: conception, methodology, data acquisition, analysis, interpretation, draft preparation, and critical revision of the manuscript. LZ, SZ, RO, and SL: data acquisition. SP: data acquisition and draft preparation. BY: conception. SS: data interpretation, drafting, and critical revision of the manuscript. TO: data analysis, interpretation, draft preparation, and critical revision of the manuscript. AM and RL: conception, methodology, data analysis, interpretation, draft preparation, and critical revision of the manuscript. All authors contributed to the article and approved the submitted version.
Conflict of Interest
The authors declare that the research was conducted in the absence of any commercial or financial relationships that could be construed as a potential conflict of interest.
Acknowledgments
The authors would like to thank Zest Dental Solutions for providing the TiCN- and TiN-coated titanium discs that were used as test specimen in this study. The authors would also like to acknowledge and recognize Drs. Nini Tran, Marcia Dinis, and Farnoosh Haghighi for critical review of the manuscript.
References
1. Mombelli A, Lang NP. The diagnosis and treatment of peri-implantitis. Periodontol 2000. (1998) 17:63–76. doi: 10.1111/j.1600-0757.1998.tb00124.x
2. Heitz-Mayfield LJA, Salvi GE. Peri-implant mucositis. J Clin Periodontol. (2018) 45(Suppl 20):S237–S45. doi: 10.1111/jcpe.12953
3. Ting M, Craig J, Balkin BE, Suzuki JB. Peri-implantitis: a comprehensive overview of systematic reviews. J Oral Implantol. (2018) 44:225–47. doi: 10.1563/aaid-joi-D-16-00122
4. Albrektsson TO, Johansson CB, Sennerby L. Biological aspects of implant dentistry: osseointegration. Periodontol 2000. (1994) 4:58–73. doi: 10.1111/j.1600-0757.1994.tb00006.x
5. Meffert RM. Maintenance and treatment of the ailing and failing implant. J Indiana Dent Assoc. (1994) 73:22–4; quiz 25.
6. Lindhe J, Meyle J, Group D of European Workshop on Periodontology. Peri-implant diseases: Consensus Report of the Sixth European Workshop on Periodontology. J Clin Periodontol. (2008) 35:282–5. doi: 10.1111/j.1600-051X.2008.01283.x
7. Busscher HJ, Rinastiti M, Siswomihardjo W, van der Mei HC. Biofilm formation on dental restorative and implant materials. J Dent Res. (2010) 89:657–65. doi: 10.1177/0022034510368644
8. Lee A, Wang HL. Biofilm related to dental implants. Implant Dent. (2010) 19:387–93. doi: 10.1097/ID.0b013e3181effa53
9. Lang NP, Berglundh T, Working Group 4 of Seventh European Workshop on P. Periimplant diseases: where are we now?–Consensus of the Seventh European Workshop on Periodontology. J Clin Periodontol. (2011) 38(Suppl 11):178–81. doi: 10.1111/j.1600-051X.2010.01674.x
10. Branemark PI, Hansson BO, Adell R, Breine U, Lindstrom J, Hallen O, et al. Osseointegrated implants in the treatment of the edentulous jaw. Experience from a 10-year period. Scand J Plast Reconstr Surg Suppl. (1977) 16:1–132.
11. Parr GR, Gardner LK, Toth RW. Titanium: the mystery metal of implant dentistry. Dental materials aspects. J Prosthet Dent. (1985) 54:410–4. doi: 10.1016/0022-3913(85)90562-1
12. Smith DC. Dental implants: materials and design considerations. Int J Prosthodont. (1993) 6:106–17.
13. Adell R, Lekholm U, Rockler B, Branemark PI, Lindhe J, Eriksson B, et al. Marginal tissue reactions at osseointegrated titanium fixtures (I). A 3-year longitudinal prospective study. Int J Oral Maxillofac Surg. (1986) 15:39–52. doi: 10.1016/S0300-9785(86)80010-2
14. Jemt T, Chai J, Harnett J, Heath MR, Hutton JE, Johns RB, et al. A 5-year prospective multicenter follow-up report on overdentures supported by osseointegrated implants. Int J Oral Maxillofac Implants. (1996) 11:291–8.
15. Niinomi M. Mechanical properties of biomedical titanium alloys. Mater Sci Eng A. (1998) 243:231–6. doi: 10.1016/S0921-5093(97)00806-X
16. Bundy KJ, Butler MF, Hochman RF. An investigation of the bacteriostatic properties of pure metals. J Biomed Mater Res. (1980) 14:653–63. doi: 10.1002/jbm.820140511
17. Joshi RI, Eley A. The in-vitro effect of a titanium implant on oral microflora: comparison with other metallic compounds. J Med Microbiol. (1988) 27:105–7. doi: 10.1099/00222615-27-2-105
18. Elagli K, Neut C, Romond C, Hildebrand HF. In vitro effects of titanium powder on oral bacteria. Biomaterials. (1992) 13:25–7. doi: 10.1016/0142-9612(92)90090-B
19. Kaestner P, Olfe J, He JW, Rie KT. Improvement in the load-bearing capacity and adhesion of TiC coatings on TiAl6V4 by duplex treatment. Surf Coat Technol. (2001) 142–4:928–33. doi: 10.1016/S0257-8972(01)01214-2
20. Zhecheva A, Sha W, Malinov S, Long A. Enhancing the microstructure and properties of titanium alloys through nitriding and other surface engineering methods. Surf Coat Technol. (2005) 200:2192–207. doi: 10.1016/j.surfcoat.2004.07.115
21. Cheng Y, Zheng YF. The corrosion behavior and hemocompatibility of TiNi alloys coated with DLC by plasma based ion implantation. Surf Coat Technol. (2006) 200:4543–8. doi: 10.1016/j.surfcoat.2005.03.039
22. Yildiz F, Yetim A, Alsaran A, Çelik A. Plasma nitriding behavior of Ti6Al4V orthopedic alloy. Surf Coat Technol. (2008) 202:2471–6. doi: 10.1016/j.surfcoat.2007.08.004
23. Huacho PMM, Nogueira MNM, Basso FG, Jafelicci Junior M, Francisconi RS, Spolidorio DMP. Analyses of biofilm on implant abutment surfaces coating with diamond-like carbon and biocompatibility. Braz Dent J. (2017) 28:317–23. doi: 10.1590/0103-6440201601136
24. Zhang L-C, Chen L-Y, Wang L. Surface modification of titanium and titanium alloys: technologies, developments, future interests. Adv Eng Mater. (2020) 22:1901258. doi: 10.1002/adem.201901258
25. Tamura Y, Yokoyama A, Watari F, Kawasaki T. Surface properties and biocompatibility of nitrided titanium for abrasion resistant implant materials. Dent Mater J. (2002) 21:355–72. doi: 10.4012/dmj.21.355
26. Gispert MP, Serro AP, Colaço R, do Rego AMB, Alves E, da Silva RC, et al. Tribological behaviour of Cl-implanted TiN coatings for biomedical applications. Wear. (2007) 262:1337–45. doi: 10.1016/j.wear.2007.01.017
27. Martinu L, Raveh A, Domingue A, Bertrand L, Klemberg-Sapieha JE, Gujrathi SC, et al. Hard carbon films deposited under high ion flux. Thin Solid Films. (1992) 208:42–7. doi: 10.1016/0040-6090(92)90945-8
28. Raveh A, Martinu L, Gujrathi SC, Klemberg-Sapieha JE, Wertheimer MR. Structure-property relationships in dual-frequency plasma deposited hard a-C: H films. Surf Coat Technol. (1992) 53:275–82. doi: 10.1016/0257-8972(92)90386-O
29. Snyders R, Bousser E, Amireault P, Klemberg-Sapieha JE, Park E, Taylor K, et al. Tribo-mechanical properties of DLC coatings deposited on nitrided biomedical stainless steel. Plasma Process Polym. (2007) 4:S640–S6. doi: 10.1002/ppap.200731601
30. Serro AP, Completo C, Colaço R, dos Santos F, da Silva CL, Cabral JMS, et al. A comparative study of titanium nitrides, TiN, TiNbN and TiCN, as coatings for biomedical applications. Surf Coat Technol. (2009) 203:3701–7. doi: 10.1016/j.surfcoat.2009.06.010
31. Camargo SEA, Roy T, Carey Iv PH, Fares C, Ren F, Clark AE, et al. Novel coatings to minimize bacterial adhesion and promote osteoblast activity for titanium implants. J Funct Biomater. (2020) 11:42. doi: 10.3390/jfb11020042
32. Grossner-Schreiber B, Griepentrog M, Haustein I, Muller WD, Lange KP, Briedigkeit H, et al. Plaque formation on surface modified dental implants. An in vitro study. Clin Oral Implants Res. (2001) 12:543–51. doi: 10.1034/j.1600-0501.2001.120601.x
33. Brunello G, Brun P, Gardin C, Ferroni L, Bressan E, Meneghello R, et al. Biocompatibility and antibacterial properties of zirconium nitride coating on titanium abutments: an in vitro study. PLoS One. (2018) 13:e0199591. doi: 10.1371/journal.pone.0199591
34. Chan C-W, Quinn J, Hussain I, Carson L, Smith GC, Lee S. A promising laser nitriding method for the design of next generation orthopaedic implants: cytotoxicity and antibacterial performance of titanium nitride (TiN) wear nano-particles, and enhanced wear properties of laser-nitrided Ti6Al4V surfaces. Surf Coat Technol. (2021) 405:126714. doi: 10.1016/j.surfcoat.2020.126714
35. Yoshinari M, Oda Y, Kato T, Okuda K, Hirayama A. Influence of surface modifications to titanium on oral bacterial adhesion in vitro. J Biomed Mater Res. (2000) 52:388–94. doi: 10.1002/1097-4636(200011)52:2<388::AID-JBM20>3.0.CO;2-E
36. Sáenz de Viteri V, Barandika G, Bayón R, Fernández X, Ciarsolo I, Igartua A, et al. Development of Ti-C-N coatings with improved tribological behavior and antibacterial properties. J Mech Behav Biomed Mater. (2016) 55:75–86. doi: 10.1016/j.jmbbm.2015.10.020
37. Almaguer-Flores A, Ximénez-Fyvie LA, Rodil SE. Oral bacterial adhesion on amorphous carbon and titanium films: effect of surface roughness and culture media. J Biomed Mater Res B Appl Biomater. (2010) 92B:196–204. doi: 10.1002/jbm.b.31506
38. Dewhirst FE, Chen T, Izard J, Paster BJ, Tanner AC, Yu WH, et al. The human oral microbiome. J Bacteriol. (2010) 192:5002–17. doi: 10.1128/JB.00542-10
39. Griffen AL, Beall CJ, Campbell JH, Firestone ND, Kumar PS, Yang ZK, et al. Distinct and complex bacterial profiles in human periodontitis and health revealed by 16S pyrosequencing. ISME J. (2012) 6:1176–85. doi: 10.1038/ismej.2011.191
40. Yao Y, Berg EA, Costello CE, Troxler RF, Oppenheim FG. Identification of protein components in human acquired enamel pellicle and whole saliva using novel proteomics approaches. J Biol Chem. (2003) 278:5300–8. doi: 10.1074/jbc.M206333200
41. Hannig C, Hannig M. The oral cavity-a key system to understand substratum-dependent bioadhesion on solid surfaces in man. Clin Oral Invest. (2009) 13:123–39. doi: 10.1007/s00784-008-0243-3
42. Souza JC, Mota RR, Sordi MB, Passoni BB, Benfatti CA, Magini RS. Biofilm formation on different materials used in oral rehabilitation. Braz Dent J. (2016) 27:141–7. doi: 10.1590/0103-6440201600625
43. Aita H, Hori N, Takeuchi M, Suzuki T, Yamada M, Anpo M, et al. The effect of ultraviolet functionalization of titanium on integration with bone. Biomaterials. (2009) 30:1015–25. doi: 10.1016/j.biomaterials.2008.11.004
44. Sharma S, Cross SE, Hsueh C, Wali RP, Stieg AZ, Gimzewski JK. Nanocharacterization in dentistry. Int J Mol Sci. (2010) 1(2013) 1:2523–45. doi: 10.3390/ijms11062523
45. Tian Y, He X, Torralba M, Yooseph S, Nelson KE, Lux R, et al. Using DGGE profiling to develop a novel culture medium suitable for oral microbial communities. Mol Oral Microbiol. (2010) 25:357–67. doi: 10.1111/j.2041-1014.2010.00585.x
46. Edlund A, Yang Y, Hall AP, Guo L, Lux R, He X, et al. An in vitro biofilm model system maintaining a highly reproducible species and metabolic diversity approaching that of the human oral microbiome. Microbiome. (2013) 1:25. doi: 10.1186/2049-2618-1-25
47. Zmantar T, Kouidhi B, Miladi H, Mahdouani K, Bakhrouf A. A microtiter plate assay for Staphylococcus aureus biofilm quantification at various pH levels and hydrogen peroxide supplementation. New Microbiol. (2010) 33:137–45.
48. Badihi Hauslich L, Sela MN, Steinberg D, Rosen G, Kohavi D. The adhesion of oral bacteria to modified titanium surfaces: role of plasma proteins and electrostatic forces. Clin Oral Implants Res. (2013) 24(Suppl A100):49–56. doi: 10.1111/j.1600-0501.2011.02364.x
49. Bray D. Critical point drying of biological specimens for scanning electron microscopy. In: Williams JR, Clifford AA, editors. Supercritical Fluid Methods and Protocols. Totowa, NJ: Humana Press (2000). p. 235–43.
50. Agnello M, Marques J, Cen L, Mittermuller B, Huang A, Chaichanasakul Tran N, et al. Microbiome associated with severe caries in Canadian First Nations Children. J Dent Res. (2017) 96:1378–85. doi: 10.1177/0022034517718819
51. Rupf S, Merte K, Eschrich K. Quantification of bacteria in oral samples by competitive polymerase chain reaction. J Dent Res. (1999) 78:850–6. doi: 10.1177/00220345990780040501
52. Parada AE, Needham DM, Fuhrman JA. Every base matters: assessing small subunit rRNA primers for marine microbiomes with mock communities, time series and global field samples. Environ Microbiol. (2016) 18:1403–14. doi: 10.1111/1462-2920.13023
53. Apprill A, McNally S, Parsons R, Weber L. Minor revision to V4 region SSU rRNA 806R gene primer greatly increases detection of SAR11 bacterioplankton. Aquat Microb Ecol. (2015) 75:129–37. doi: 10.3354/ame01753
54. Bolyen E, Rideout JR, Dillon MR, Bokulich NA, Abnet CC, Al-Ghalith GA, et al. Reproducible, interactive, scalable and extensible microbiome data science using QIIME 2. Nat Biotechnol. (2019) 37:852–7. doi: 10.1038/s41587-019-0209-9
55. Callahan BJ, McMurdie PJ, Rosen MJ, Han AW, Johnson AJA, Holmes SP. DADA2: high-resolution sample inference from Illumina amplicon data. Nat Methods. (2016) 13:581–3. doi: 10.1038/nmeth.3869
56. Chen T, Yu W-H, Izard J, Baranova OV, Lakshmanan A, Dewhirst FE. The Human Oral Microbiome Database: a web accessible resource for investigating oral microbe taxonomic and genomic information. Database. (2010) 2010:baq013. doi: 10.1093/database/baq013
57. Teughels W, Van Assche N, Sliepen I, Quirynen M. Effect of material characteristics and/or surface topography on biofilm development. Clin Oral Implants Res. (2006) 17(Suppl 2):68–81. doi: 10.1111/j.1600-0501.2006.01353.x
58. Barbour ME, O'Sullivan DJ, Jenkinson HF, Jagger DC. The effects of polishing methods on surface morphology, roughness and bacterial colonisation of titanium abutments. J Mater Sci Mater Med. (2007) 18:1439–47. doi: 10.1007/s10856-007-0141-2
59. Karygianni L, Jahnig A, Schienle S, Bernsmann F, Adolfsson E, Kohal RJ, et al. Initial bacterial adhesion on different Yttria-Stabilized Tetragonal Zirconia implant surfaces in vitro. Materials (Basel). (2013) 6:5659–74. doi: 10.3390/ma6125659
60. Yamada Y, Yamada M, Ueda T, Sakurai K. Reduction of biofilm formation on titanium surface with ultraviolet-C pre-irradiation. J Biomater Appl. (2014) 29:161–71. doi: 10.1177/0885328213518085
61. de Avila ED, Lima BP, Sekiya T, Torii Y, Ogawa T, Shi W, et al. Effect of UV-photofunctionalization on oral bacterial attachment and biofilm formation to titanium implant material. Biomaterials. (2015) 67:84–92. doi: 10.1016/j.biomaterials.2015.07.030
62. De-la-Pinta I, Cobos M, Ibarretxe J, Montoya E, Eraso E, Guraya T, et al. Effect of biomaterials hydrophobicity and roughness on biofilm development. J Mater Sci. (2019) 30:77. doi: 10.1007/s10856-019-6281-3
63. Vadillo-Rodriguez V, Busscher HJ, van der Mei HC, de Vries J, Norde W. Role of lactobacillus cell surface hydrophobicity as probed by AFM in adhesion to surfaces at low and high ionic strength. Colloids Surf B Biointerfaces. (2005) 41:33–41. doi: 10.1016/j.colsurfb.2004.10.028
64. Oh JK, Yegin Y, Yang F, Zhang M, Li J, Huang S, et al. The influence of surface chemistry on the kinetics and thermodynamics of bacterial adhesion. Sci Rep. (2018) 8:17247. doi: 10.1038/s41598-018-35343-1
65. Marsh PD. Dental plaque as a biofilm and a microbial community - implications for health and disease. BMC Oral Health. (2006) 6(Suppl 1):S14. doi: 10.1186/1472-6831-6-S1-S14
66. Du Q, Fu M, Zhou Y, Cao Y, Guo T, Zhou Z, et al. Sucrose promotes caries progression by disrupting the microecological balance in oral biofilms: an in vitro study. Sci Rep. (2020) 10:2961. doi: 10.1038/s41598-020-59733-6
67. Groessner-Schreiber B, Hannig M, Duck A, Griepentrog M, Wenderoth DF. Do different implant surfaces exposed in the oral cavity of humans show different biofilm compositions and activities? Eur J Oral Sci. (2004) 112:516–22. doi: 10.1111/j.1600-0722.2004.00171.x
Keywords: implant abutment, titanium nitride, titanium carbon nitride, oral biofilm, surface properties
Citation: Shokeen B, Zamani L, Zadmehr S, Pouraghaie S, Ozawa R, Yilmaz B, Lilak S, Sharma S, Ogawa T, Moshaverinia A and Lux R (2021) Surface Characterization and Assessment of Biofilm Formation on Two Titanium-Based Implant Coating Materials. Front. Dent. Med. 2:695417. doi: 10.3389/fdmed.2021.695417
Received: 15 April 2021; Accepted: 25 June 2021;
Published: 21 July 2021.
Edited by:
Jae-Sung Kwon, Yonsei University, South KoreaReviewed by:
Gülçin Akca, Gazi University, TurkeySamira Camargo, University of Florida, United States
Copyright © 2021 Shokeen, Zamani, Zadmehr, Pouraghaie, Ozawa, Yilmaz, Lilak, Sharma, Ogawa, Moshaverinia and Lux. This is an open-access article distributed under the terms of the Creative Commons Attribution License (CC BY). The use, distribution or reproduction in other forums is permitted, provided the original author(s) and the copyright owner(s) are credited and that the original publication in this journal is cited, in accordance with accepted academic practice. No use, distribution or reproduction is permitted which does not comply with these terms.
*Correspondence: Alireza Moshaverinia, YW1vc2hhdmVyaW5pYUBkZW50aXN0cnkudWNsYS5lZHU=; Renate Lux, cmx1eEBkZW50aXN0cnkudWNsYS5lZHU=
†Present address: Sara Zadmehr, Temple University, Kornberg School of Dentistry, Philadelphia, PA, United States
Ryotaro Ozawa, Department of Oral Interdisciplinary Medicine (Prosthodontics and Oral Implantology), Graduate School of Dentistry, Kanagawa Dental University, Yokosuka, Japan