- 1Department of Dentistry, Division of Pediatric Dentistry, University of Toledo, Toledo, OH, United States
- 2Department of Biological Sciences, University of Toledo, Toledo, OH, United States
- 3Department of Mechanical, Industrial and Manufacturing Engineering (MIME), University of Toledo, Toledo, OH, United States
- 4Department of Cariology, Restorative Sciences & Endodontics, University of Michigan, Ann Arbor, MI, United States
- 5Department of Orthodontics and Dentofacial Orthopedics, The Eastman Institute for Oral Health, University of Rochester, Rochester, NY, United States
- 6Department of Medicine, University of Toledo, Toledo, OH, United States
- 7Mulford Health Sciences Library, University of Toledo, Toledo, OH, United States
- 8Department of Medical Microbiology and Immunology, University of Toledo, Toledo, OH, United States
The COVID-19 pandemic pushed dental health officials around the world to reassess and adjust their existing healthcare practices. As studies on controlled COVID-19 transmission remain challenging, this review focuses on particles that can carry the virus and relevant approaches to mitigate the risk of pathogen transmission in dental offices. This review gives an overview of particles generated in clinical settings and how size influences their distribution, concentration, and generation route. A wide array of pertinent particle characterization and counting methods are reviewed, along with their working range, reliability, and limitations. This is followed by a focus on the effectiveness of personal protective equipment (PPE) and face shields in protecting patients and dentists from aerosols. Direct studies on severe acute respiratory syndrome coronavirus 2 (SARS-CoV-2) are still limited, but the literature supports the use of masks as an important and effective non-pharmaceutical preventive measure that could reduce the risk of contracting a respiratory infection by up to 20%. In addition to discussing about PPE used by most dental care professionals, this review describes other ways by which dental offices can protect patients and dental office personnel, which includes modification of the existing room design, dental equipment, and heating, ventilation, and air conditioning (HVAC) system. More affordable modifications include positioning a high-efficiency particulate air (HEPA) unit within proximity of the patient's chair or using ultraviolet germicidal irradiation in conjunction with ventilation. Additionally, portable fans could be used to direct airflow in one direction, first through the staff working areas and then through the patient treatment areas, which could decrease the number of airborne particles in dental offices. This review concludes that there is a need for greater awareness amongst dental practitioners about the relationship between particle dynamics and clinical dentistry, and additional research is needed to fill the broad gaps of knowledge in this field.
Introduction
Severe acute respiratory syndrome coronavirus 2 (SARS-CoV-2), which is the causative virus of coronavirus disease-2019 (COVID-19), presents challenges greater than that posed by seasonal influenza (R0 ~ 1.2), Middle East respiratory syndrome (MERS) (R0 ~ 1.4), or severe acute respiratory syndrome (SARS) (R0 ~ 3) due to its high reproductive number (R0 = 1.4–3.9) (1–3). The most recent global assessment of R0 for COVID-19 is 4.08. This high reproductive number contributed to the unprecedented global spread of SARS-CoV-2 (4).
According to a scientific brief published by the WHO on March 29, 2020, the primary transmission mode of SARS-CoV-2 is through respiratory droplets and contact routes (i.e., oral secretions) with a diameter >5 μm (5, 6). These droplets enter the air through speaking, coughing, and sneezing by individuals in close contact with each other (5–8). Short-range inhalation of aerosols can be a route of COVID-19 transmission as with many respiratory pathogens (9). Oral cavity is another important site for SARS-CoV-2 infection (10). The most reported mode of pathogen spread is through respiratory droplets. Individuals that are pre-symptomatic or asymptomatic do not significantly cough or sneeze, yet are responsible for more than 50% of COVID-19 transmission (11), and hence it is important to study the effect of particles expired through normal breathing and oral cavity secretions (10, 12).
Dental practices across the globe closed down for non-emergency dental care during peak COVID-19 periods because of the higher risk of virus transmission during dental appointment due to the close proximity of patient–provider and the use of modern dental tools, such as high-speed handpieces, ultrasonic scalers, air turbines, and air-water syringes, in the presence of contaminated salivary secretions (5, 10, 13–15). It is important to note that dental professionals demonstrated high compliance (72.8%) to CDC guidelines during the COVID-19 pandemic, which has led to very low cases of COVID-19 transmission in dental settings (16). In this review, the authors present studies focused on the transport of particles, the various methods of characterizing the particles, bioaerosols in dentistry, and finally recommendations for reducing the transmission of potentially virus-laden droplets generated during routine dental treatments.
Particles
To better understand the modifications that could be adopted by dental offices to minimize transmission of SARS-CoV-2, the team elaborates on the definition of particle and droplet, transmission of particles via bioaerosols, and the natural production and dissemination of aerosols produced during cleaning or treatment at a dental office.
Definitions of Particle and Droplet
In dental settings, particles are generated from patient–provider interactions, dental equipment, and dental procedures. Our review defines droplets as water-based with a mean diameter >5 μm. Aerosols (or airborne nuclei) are defined as liquid or solid particles typically <5 μm in diameter. Particulate matters (PMs) are arguably interchangeable with aerosols and typically refer to solid (or liquid) particles in the size range of sub-micron to 10 μm. Particle concentration refers to the count (or particle mass) per unit volume, e.g., count/m3 (or μg/L).
Size influences the behavior and trajectory of droplets and aerosols/particles. Within a 1 m radius, droplets larger than 50 μm display ballistic or jet-like movements. Intermediate size droplets can either fall on the surface or can stay in the air and travel approximately 2 m before settling down. Smaller droplets and aerosols are the least impacted by gravity and can stay airborne for long durations. For example, a dust particle with a diameter of 10 μm falls 1 m in air at rest in ~5.5 min, whereas a 1 μm particle takes over 9 h to travel the same distance. Owing to air currents, such small particles may spread throughout the room, especially after the volatile liquid in the droplet dries out (17).
Bioaerosols Production and the Influencing Factors
Human lungs inspire approximately 0.5–0.75 L per breath during rest. Air expelled through nasal respiration produces an exit velocity of ~0.5 m/s, whereas the exit velocity while speaking with a normal volume and pace exhibits ~0.3 m/s. In contrast, during periodic coughing, the exit velocity dramatically increases to 4–5 m/s (18). Direct observations of human sneezing and coughing reveal that these airflows consist of initially hot and moist turbulence followed by cool and buoyant clouds containing droplets of varying sizes. A single cough may expel ~700 particles as compared with a sneeze that may produce over 40,000 particles (19). In this regard, most of the particles and droplets produced from a sneeze are relatively large and may be easily blocked by the use of a simple mask. In addition to coughing and sneezing, speaking can generate droplets of size ranging from 20 to 500 μm (5). However, the estimated concentration of droplets per cough is 2.4–5.2 cm−3, which is significantly more than that of speech, 0.004–0.223 cm−3 (20).
In addition to particle size, environmental factors such as temperature and relative humidity influence the potency and distribution of infectious respiratory droplets and particles. For example, a 100 μm droplet will evaporate in approximately 10 s after expulsion while a 1 μm droplet will evaporate within 0.001 s (19). Increased air temperature, however, leads to an immediate decrease in particles post expulsion. By contrast, however, in an environment of elevated humidity, these numbers increase. Regardless of humidity or temperature, particles or droplets with a diameter of <0.1 μm evaporate almost immediately or cannot contain enough viral material to be infectious (9).
Viruses can be transmitted via droplets produced by sneezing and coughing, with diameters varying in the range of 0.1 μm-0.9 mm (21). Once an individual begins coughing, the duration and position of the mouth influence the area covered by the expelled droplets, which can degrade into categories of smaller size (9). Viral, fungal, and bacterial particles react in different ways depending on changes in temperature or humidity of the environment. The maximum stability of influenza occurs at 20–40% humidity and has a minimum stability at 50% humidity (22). Studies have suggested that SARS-CoV-2 exhibits similar survival curves in response to increases in temperature and humidity (23). With respect to coronaviruses at room temperature, they remain viable on surfaces for up to 9 days (24). At temperatures >30°C, the survival of these viruses decreases dramatically (24).
Bioaerosols in the Practice of Dentistry
Dissemination of Microorganisms
Dissemination of microorganisms in dental operatories can occur directly, by contact with bacteria on the surfaces of dental instruments and dental providers, or indirectly, via splatter of droplets larger than 100 μm in diameter or by particles <100 μm in diameter suspended in air (25). Most dental bioaerosol studies have investigated bacterial colonies on the surfaces of dental instruments as the main pathogen. The potential for disease transmission of airborne bacteria (e.g., tuberculosis), viruses (e.g., measles and SARS), and bloodborne viruses, which can become aerosolized by blood splatter via high-speed handpieces used in dentistry and orthodontics, is not known (26–28). COVID-19 transmission is primarily through respiratory droplets and less likely through fomite transmission (29). The two most notable sources of droplet and aerosol generation in dental settings are procedures involving air turbine handpieces and ultrasonic instruments.
Air Turbine Handpieces
Studies have demonstrated that air turbine handpieces atomize 20 times more bacteria when compared to air spray. This production of bioaerosols is equivalent to the concentration produced by biological motions such as sneezing (30). A prophylactic hygiene handpiece with a pumice cup and pumice is often used for cleaning teeth. This common dental procedure produces a volume of aerosolized bacteria comparable to that resulting from a cough (31). Tubercle bacilli were found in droplet scatterings generated by dental air turbine handpieces within a range of 6 inches to over 4 feet from the patient's mouth (26). This distance is larger than the distance between providers and patients, indicating a working dentist/assistant will undoubtedly be affected (30).
Ultrasonic Instruments
Ultrasonic instruments also produce significant amounts of aerosols and the vibration of the tip generates significant amounts of heat. Water is used to cool the instrument, which results in the generation of significant splatter. When mixed with saliva and plaque from the oral cavity, aerosolized splatter from ultrasonic instruments has the potential to become highly infectious and a major risk factor for disease transmission (31). Moreover, ultrasonic instrumentation can transmit 100,000 microbes/ft3 with aerosolization of up to 6 ft (32). In the absence of a favorable air current, microbes can survive for a period ranging from 35 min to 17 h. Using microbiological analysis, significantly higher bacterial counts were detected after scaling treatments, with the presence of Staphylococcus and Streptococcus species being the most notable (26). Results also showed high numbers of colony forming units (CFUs) and identified strictly oral anaerobes on all microbial plates from both groups, which meant that a significant amount of contamination occurred during ultrasonic scaling (26).
Methods of Characterizing Bioaerosols
Aerosolized particles can be classified into three categories: natural (e.g., fog, dust, and mist), anthropogenic (e.g., air pollution and smoke), and biological (e.g., bioaerosols) which are primarily released by humans and animals. These particles are carried through natural and anthropogenic means (33, 34). Bioaerosols contain both volatile and non-volatile material and their behavior and transmissibility depend on their size. Smaller bioaerosol particles penetrate more easily and go farther into the respiratory tract, which means they are more likely to transmit diseases compared to the larger particles (19).
Over the years, researchers have developed a variety of instruments to assist with particle sizing and classification. A broad list of instruments used in bioaerosol particle counting and sizing is presented in Table 1, and a summary of measured particle size distributions is shown in Figure 1. More recently, researchers have started using machine learning to analyze SARS-CoV-2 infected particles (44).
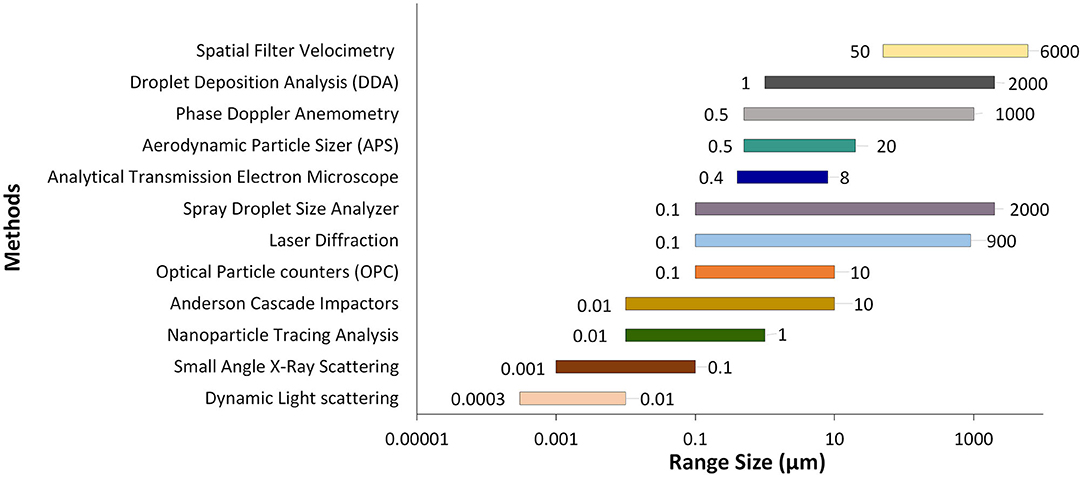
Figure 1. A summary of identified bioaerosol particle size distributions measured using select methods.
Recommendations to Minimize COVID-19 Transmission in Dental Settings
Patient/Staff as Source of Particle
Although there are differences in aerodynamic behavior and properties between droplets and particles, both provide mechanisms for transmitting pathogenic microorganisms between patient and dental personnel. Human interactions (speaking, sneezing, and coughing), even without any symptom, can be a source of respiratory pathogen transmission in an indoor setting (5, 20), such as in dental offices. Dental providers working with high-speed handpieces have routine exposure to bodily fluids including respiratory particles and oral secretions (10, 34). Ultrasonic scalers, air turbines, three-in-one syringes, and air-water syringes are also significant contributors to bioaerosol generation (31).
Personal Protective Equipment
Personal protective equipment (PPE) is an important mitigation strategy (45). Global sources for producing PPE continue to be insufficient due to a large number of COVID-19 cases, misinformation, panic buying, and stockpiling (6). It is imperative to revisit the current and developing PPE options with respect to their efficacy. The WHO has listed the following PPEs as necessary for healthcare workers: medical masks, N95 respirators, filtering facepiece respirators-2 (FFP2) standard or equivalent, gowns, gloves, aprons, and eye protection (goggles or face shields) (6). A recent study on COVID-19 prevalence among dentists, while adhering to the listed PPEs, indicated a very low percentage of only 0.9% (16). While combinations of PPE are recommended, the effectiveness of specific PPE at preventing SARS-CoV-2 infection has not been quantified. Determining the effectiveness of PPE is complicated due to the limited controlled human infection studies. “Silent spreaders” may expose healthcare workers at work and elsewhere if basic non-pharmaceutical interventions are not universally adopted and enforced (46, 47). In the absence of PPE measures, historical data from similarly transmitted respiratory diseases, such as tuberculosis in dental settings showed a transmission of 10%, which was more than double the reported data from the National Health and Nutrition Examination Survey (NHANES) in the year 2000 (48).
Face Shields
Most harmful particles are generated during the initial part of a cough (49). Healthcare providers working at a distance of 46 cm from an infected patient may inhale 0.9% of these harmful particles. Wearing a face shield can reduce the inhalation of aerosols by 96% and surface contamination by 97% for droplets with a volume median diameter (VMD) of 8.5 μm. However, for smaller droplets with a VMD of 3.4 μm, the reduction rates are lower with 68 and 76% for aerosols and surface contamination, respectively (45). Face shields may reduce the inhalation of large harmful aerosol particles for a short time. However, smaller particles remain in the air for longer periods and could bypass the face shield. These particles pose inhalation risks for healthcare providers and patients (50).
Face Masks
A study conducted on 47 human subjects with influenza showed that the average cough profiles had a volume of 4.16 L with a peak flow rate of 11.1 L/s (22). A mask designed to suppress droplets at this volume and flow rate could be an effective inhibitor for smaller cough volumes and lesser peak flow rates (18). A different study reported that each patient suffering from influenza released ~38 pL of particles in the size range of <10 μm. After being diagnosed and receiving treatment, the study participants released ~26 pL of particles per cough. Droplets become infectious when they encounter the mucous membranes, e.g., oral and nasal cavities of the body (51). Studies on influenza-related diseases support the use of surgical masks for effective reduction of infection (47). Using masks in crowded places could reduce the risk of contracting influenza-like respiratory infections by 20% (52). Masks made of foam, cloth, or paper are less effective at filtering bacterial aerosols (50).
Masks vs. Respirators
According to the CDC, the N95 FFP can block at least 95% of 0.3 μm particles. Most research pertaining to the efficacy of face masks has been done by quantifying the number of respiratory viruses in exhaled breaths of participants with acute respiratory virus illness (17). Surgical masks can effectively reduce the emission of influenza-laden respiratory droplets but not particles (53). When subjects cough while wearing a surgical mask or N95, the dispersion of forward moving viral aerosol particles decreases but the lateral dispersion patterns of particles increases (54). N95s and surgical masks offer similar levels of protection against viral infection of respiratory diseases in non-aerosol producing environments (55).
Equipment as Source of Particles (Room Design/Equipment Modification)
Dental buildings can contain high levels of circulating bioaerosols. Air-conditioning and ventilation systems in these settings should be maintained on a regular basis to minimize recirculation of contaminants (56). Cooling towers, air-conditioning, and mechanical ventilation systems are known sources of Legionella pneumophila (57). The risks to dentists, patients, and others who are routinely exposed to bioaerosols remain unclear, prompting the need for further research (14).
Office or Clinic Design
Multiple actions can be taken to decrease the transmission of infectious particles in healthcare settings. Electronic-based patient triages and check-ins, automatic doors, motion-sensing lights, and hand-sanitizer dispensers reduce the physical interface among patients, physicians, and interior surfaces. The use of thermal imaging to screen for elevated body temperatures ensures a safe distance between ingress patients and office staff while shortening the initial screening process. Since dental procedures generate mists and aerosols, local exhaust ventilation should be positioned with consideration of the aerosol flow direction as well as the location of the physician and the patient (9, 58–61).
Heating, Ventilation, and Air Conditioning System Design
Dental offices are advised to use a systems-based approach with engineering controls to minimize cross contamination. For example, fans could be used to direct the airflow first through the staff working areas and then through the patient treatment areas, thereby reducing workplace risks involving airborne particles or droplets (62). Positioning of patients in front of each other should be avoided whenever possible (9). Operatories should be oriented parallel to the direction of airflow, which will assist in directing the flow of airborne contaminants (62).
The CDC also recommends positioning the heads of patients away from pedestrian corridors and closer to the back wall and return air vents (9, 63). Several studies have evaluated the characteristics of plumes generated by exhaled droplets and noted that a top exhaust system is more efficient than the traditional air conditioning systems (64). Ultraviolet (UV) germicidal irradiation, including UV-C at 254 nm, in conjunction with ventilation is emerging as a cost-effective tool for reducing viral aerosols (52, 65, 66). In the design of future dental offices, the designation of negative-pressure isolation rooms, antechambers, and 24/7 HVAC systems could improve aerobiological controls (63).
Interior Design
It is important to consider an interior design that prompts safe conduct of ordinary activities. Visual signage is a good option to communicate instructions clearly to the general public. For example, clear marking of risk zones with visual aids or creating visual cues for specific activities raises awareness and allows policies to be followed easily (58). Additionally, creating signage that indicates “clean” areas around donning rooms and PPE carts can establish easy-to-follow protocols. Efficient and regimented routines create less interaction and reduce cross-contamination. The efficacy of visual signage is increased if supplemented with an office culture based on safety and education. Additionally, anti-bacterial surface coatings reduce healthcare-associated infections (HAIs) by 36% while also decreasing CFUs and clinically relevant pathogens by ~59–75% (67). Other design initiatives worth considering are sanitizing stations, maintaining social distancing whenever possible in the reception and treatment rooms, and dedicated PPE recycling bins in each room (58).
Conclusions
Respiratory droplets are the primary mode of SARS-CoV-2 transmission. Human and simulated studies have demonstrated that sneezing and high-volume coughs in patients pose a significant risk for viral transmission. Environmental conditions effect the potency of viruses shed from human coughing and sneezing as well as dental procedures. SARS-CoV-2 reacts inversely to temperature and humidity, which are factors that can be controlled in a closed dental setting. One of the biggest concerns for dental providers is the working proximity to potentially active SARS-CoV-2 sources, as intermediate size (10–50 μm) droplets can travel as far as 2 m away from the source. Furthermore, these droplets are easily disseminated by air currents. Surgical masks and respirators appear to provide significant protection against viral particle transmission from infected individuals, although the risk is not completely eliminated. While we highlighted various methods used to study the size and distribution of airborne droplets and particles, these methods for the most part do not measure the viral load and more specialized tools need to be developed. Implementing some of the recommendations we proposed and a greater awareness amongst dental practitioners about the relationship between particle dynamics and clinical dentistry, can help improve safety in dental practice. Finally, while this review provides a broad overview of past and current studies related to pathogen and particle transmission in dental settings, there is a clear gap in our understanding of how dental practices specifically affect the transmission of SARS-CoV-2; therefore, much more research is needed in this area. This knowledge is imperative to addressing the current crisis, and those that might be faced in the future.
Author Contributions
All authors gave their final approval and agreed to be accountable for all aspects of the work.
Conflict of Interest
The authors declare that the research was conducted in the absence of any commercial or financial relationships that could be construed as a potential conflict of interest.
Publisher's Note
All claims expressed in this article are solely those of the authors and do not necessarily represent those of their affiliated organizations, or those of the publisher, the editors and the reviewers. Any product that may be evaluated in this article, or claim that may be made by its manufacturer, is not guaranteed or endorsed by the publisher.
Acknowledgments
The authors thank the National Institutes of Health (grant number: X01 DE030405-01) for the partial financial support for this article through the National Dental PBRN collaborative group funding U19-DE-028717 and U01-DE-028727. The authors thank Mohammad Reza D. Jahanshahi, Armon Jahanshahi, Dr. Michael Nedley, Dr. Mel Krajden, Dr. Yvonne Kapila, and Dr. Daniel Clauw for their expertise and guidance.
References
1. Coburn BJ, Wagner BG, Blower S. Modeling influenza epidemics and pandemics: insights into the future of swine flu (h1n1). BMC Med. (2009) 7:30. doi: 10.1186/1741-7015-7-30
2. Yin Y, Wunderink RG. Mers, sars and other coronaviruses as causes of pneumonia. Respirology. (2018) 23:130–7. doi: 10.1111/resp.13196
3. Wang J, Pan L, Tang S, Ji JS, Shi X. Mask use during covid-19: a risk adjusted strategy. Environ Pollut. (2020) 266:115099. doi: 10.1016/j.envpol.2020.115099
4. Yu CJ, Wang ZX, Xu Y, Hu MX, Chen K, Qin G. Assessment of basic reproductive number for covid-19 at global level: a meta-analysis. Medicine. (2021) 100:e25837. doi: 10.1097/MD.0000000000025837
5. Bax A, Bax CE, Stadnytskyi V, Anfinrud P. Sars-cov-2 transmission via speech-generated respiratory droplets. Lancet Infect Dis. (2021) 21:318. doi: 10.1016/S1473-3099(20)30726-X
6. Modes of Transmission of Virus Causing Covid-19: Implications for IPC Precaution Recommendations: Scientific Brief. Geneva: World Health Organization (2020).
7. Peng X, Xu X, Li Y, Cheng L, Zhou X, Ren B. Transmission routes of 2019-ncov and controls in dental practice. Int J Oral Sci. (2020) 12:9. doi: 10.1038/s41368-020-0075-9
8. Sun P, Lu X, Xu C, Sun W, Pan B. Understanding of covid-19 based on current evidence. J Med Virol. (2020) 92:548–51. doi: 10.1002/jmv.25722
9. Covid-19 Overview and Infection Prevention and Control Priorities in Non-US Healthcare Settings. Atlanta, GA: Centers for Disease Control and Prevention (2021).
10. Huang N, Pérez P, Kato T, Mikami Y, Okuda K, Gilmore RC, et al. Sars-cov-2 infection of the oral cavity and saliva. Nat Med. (2021) 27:892–903. doi: 10.1038/s41591-021-01296-8
11. Johansson MA, Quandelacy TM, Kada S, Prasad PV, Steele M, Brooks JT, et al. Sars-cov-2 transmission from people without covid-19 symptoms. JAMA Netw Open. (2021) 4:e2035057. doi: 10.1001/jamanetworkopen.2020.35057
12. Scheuch G. Breathing is enough: for the spread of influenza virus and sars-cov-2 by breathing only. J Aerosol Med Pulm Drug Deliv. (2020) 33:230–4. doi: 10.1089/jamp.2020.1616
13. Ather A, Patel B, Ruparel NB, Diogenes A, Hargreaves KM. Coronavirus disease 19 (covid-19): implications for clinical dental care. J Endod. (2020) 46:584–95. doi: 10.1016/j.joen.2020.03.008
14. Bahador M, Alfirdous RA, Alquria TA, Griffin IL, Tordik PA, Martinho FC. Aerosols generated during endodontic treatment: a special concern during the coronavirus disease 2019 pandemic. J Endod. (2021) 47:732–9. doi: 10.1016/j.joen.2021.01.009
15. Caprioglio A, Pizzetti GB, Zecca PA, Fastuca R, Maino G, Nanda R. Management of orthodontic emergencies during 2019-ncov. Prog Orthod. (2020) 21:10. doi: 10.1186/s40510-020-00310-y
16. Estrich CG, Mikkelsen M, Morrissey R, Geisinger ML, Ioannidou E, Vujicic M, et al. Estimating covid-19 prevalence and infection control practices among us dentists. J Am Dent Assoc. (2020) 151:815–24. doi: 10.1016/j.adaj.2020.09.005
17. Interim Guidance on Planning for the Use of Surgical Masks and Respirators in Health Care Settings During an Influenza Pandemic. Atlanta, GA: Centers for Disease Control and Prevention (2006).
18. Kähler CJ, Hain R. Fundamental protective mechanisms of face masks against droplet infections. J Aerosol Sci. (2020) 148:105617. doi: 10.1016/j.jaerosci.2020.105617
19. Fernstrom A, Goldblatt M. Aerobiology and its role in the transmission of infectious diseases. J Pathog. (2013) 2013:493960. doi: 10.1155/2013/493960
20. Chao CYH, Wan MP, Morawska L, Johnson GR, Ristovski ZD, Hargreaves M, et al. Characterization of expiration air jets and droplet size distributions immediately at the mouth opening. J Aerosol Sci. (2009) 40:122–33. doi: 10.1016/j.jaerosci.2008.10.003
21. Zayas G, Chiang MC, Wong E, MacDonald F, Lange CF, Senthilselvan A, et al. Cough aerosol in healthy participants: fundamental knowledge to optimize droplet-spread infectious respiratory disease management. BMC Pulm Med. (2012) 12:11. doi: 10.1186/1471-2466-12-11
22. Lindsley WG, Reynolds JS, Szalajda JV, Noti JD, Beezhold DH. A cough aerosol simulator for the study of disease transmission by human cough-generated aerosols. Aerosol Sci Technol. (2013) 47:937–44. doi: 10.1080/02786826.2013.803019
23. van Doremalen N, Bushmaker T, Morris DH, Holbrook MG, Gamble A, Williamson BN, et al. Aerosol and surface stability of sars-cov-2 as compared with sars-cov-1. N Engl J Med. (2020) 382:1564–7. doi: 10.1056/NEJMc2004973
24. Kampf G, Todt D, Pfaender S, Steinmann E. Persistence of coronaviruses on inanimate surfaces and their inactivation with biocidal agents. J Hosp Infect. (2020) 104:246–51. doi: 10.1016/j.jhin.2020.01.022
25. Miller RL, Micik RE, Abel C, Ryge G. Studies on dental aerobiology. II. Microbial splatter discharged from the oral cavity of dental patients. J Dent Res. (1971) 50:621–5. doi: 10.1177/00220345710500031701
26. Belting CM, Haberfelde GC, Juhl LK. Spread of organisms from dental air rotor. J Am Dent Assoc. (1964) 68:648–51. doi: 10.14219/jada.archive.1964.0145
27. Bennett AM, Fulford MR, Walker JT, Bradshaw DJ, Martin MV, Marsh PD. Microbial aerosols in general dental practice. Br Dent J. (2000) 189:664–7. doi: 10.1038/sj.bdj.4800859
28. Harrel SK, Molinari J. Aerosols and splatter in dentistry: a brief review of the literature and infection control implications. J Am Dent Assoc. (2004) 135:429–37. doi: 10.14219/jada.archive.2004.0207
29. CDC. Science Brief: Sars-Cov-2 and Surface (fomite) Transmission for Indoor Community Environments. CDC (2021). Available online at: https://www.cdc.gov/coronavirus/2019-ncov/more/science-and-research/surface-transmission.html
30. Pelzner RB, Kempler D, Stark MM, Barkin PR, Graham DA. Laser evaluation of handpiece contamination. J Dent Res. (1977) 56:1629–34. doi: 10.1177/00220345770560123601
31. Micik RE, Miller RL, Mazzarella MA, Ryge G. Studies on dental aerobiology. I. Bacterial aerosols generated during dental procedures. J Dent Res. (1969) 48:49–56. doi: 10.1177/00220345690480012401
32. Nejatidanesh F, Khosravi Z, Goroohi H, Badrian H, Savabi O. Risk of contamination of different areas of dentist's face during dental practices. Int J Prev Med. (2013) 4:611–5.
33. Asadi S, Bouvier N, Wexler AS, Ristenpart WD. The coronavirus pandemic and aerosols: does covid-19 transmit via expiratory particles? Aerosol Sci Technol. (2020) 0:1–4. doi: 10.1080/02786826.2020.1749229
34. Ge ZY, Yang LM, Xia JJ, Fu XH, Zhang YZ. Possible aerosol transmission of covid-19 and special precautions in dentistry. J Zhejiang Univ Sci B. (2020) 21:361–8. doi: 10.1631/jzus.B2010010
35. Morawska L, Johnson G, Ristovski Z, Hargreaves M, Mengersen K, Corbett S, et al. Size distribution and sites of origin of droplets expelled from the human respiratory tract during expiratory activities. J Aerosol Sci. (2009) 40:256–69. doi: 10.1016/j.jaerosci.2008.11.002
36. Andersen AA. New sampler for the collection, sizing, and enumeration of viable airborne particles. J Bacteriol. (1958) 76:471–84. doi: 10.1128/jb.76.5.471-484.1958
37. Duguid JP. The size and the duration of air-carriage of respiratory droplets and droplet-nuclei. J Hyg. (1946) 44:471–9. doi: 10.1017/S0022172400019288
38. Kohli R, Mittal KL. Developments in Surface Contamination and Cleaning: Detection, Characterization, and Analysis of Contaminants. Oxford: Elsevier Inc (2012).
39. Ragucci R, Cavaliere A, Massoli P. Drop sizing by laser light scattering exploiting intensity angular oscillation in the mie regime. Particle Particle Systems Characterization. (1990) 7:221–5. doi: 10.1002/ppsc.19900070136
40. VanSciver M, Miller S, Hertzberg J. Particle image velocimetry of human cough. Aerosol Sci Technol. (2011) 45:415–22. doi: 10.1080/02786826.2010.542785
41. Zhu S, Kato S, Yang J-H. Study on transport characteristics of saliva droplets produced by coughing in a calm indoor environment. Build Environ. (2006) 41:1691–702. doi: 10.1016/j.buildenv.2005.06.024
42. Papineni RS, Rosenthal FS. The size distribution of droplets in the exhaled breath of healthy human subjects. J Aerosol Med. (1997) 10:105–16. doi: 10.1089/jam.1997.10.105
43. Edwards DA, Man JC, Brand P, Katstra JP, Sommerer K, Stone HA, et al. Inhaling to mitigate exhaled bioaerosols. Proc Natl Acad Sci USA. (2004) 101:17383–8. doi: 10.1073/pnas.0408159101
44. Nemati M, Ansary J, Nemati N. Machine-learning approaches in covid-19 survival analysis and discharge-time likelihood prediction using clinical data. Patterns. (2020) 1:100074. doi: 10.1016/j.patter.2020.100074
45. Bouadma L, Barbier F, Biard L, Esposito-Farèse M, Le Corre B, Macrez A, et al. Personal decision-making criteria related to seasonal and pandemic a(h1n1) influenza-vaccination acceptance among french healthcare workers. PLoS ONE. (2012) 7:e38646. doi: 10.1371/journal.pone.0038646
46. Froum SH, Froum SJ. Incidence of covid-19 virus transmission in three dental offices: A 6-month retrospective study. Int J Periodontics Restorative Dent. (2020) 40:853–9. doi: 10.11607/prd.5455
47. Jefferson T, Del Mar C, Dooley L, Ferroni E, Al-Ansary LA, Bawazeer GA, et al. Physical interventions to interrupt or reduce the spread of respiratory viruses: systematic review. BMJ. (2009) 339:b3675. doi: 10.1136/bmj.b3675
48. Merte JL, Kroll CM, Collins AS, Melnick AL. An epidemiologic investigation of occupational transmission of mycobacterium tuberculosis infection to dental health care personnel: infection prevention and control implications. J Am Dent Assoc. (2014) 145:464–71. doi: 10.14219/jada.2013.52
49. Day JB, Jones BM, Afshari AA, Frazer DG, Goldsmith W. Temporal characteristics of aerosol generation during a voluntary cough. A67 airway function and aerosols in children, adults and mice. Am Thorac Soc. (2010). A2183. doi: 10.1164/ajrccm-conference.2010.181.1_MeetingAbstracts.A2183
50. Balachandar S, Zaleski S, Soldati A, Ahmadi G, Bourouiba L. Host-to-host airborne transmission as a multiphase flow problem for science-based social distance guidelines. Int J Multiphase Flow. (2020) 132:103439. doi: 10.1016/j.ijmultiphaseflow.2020.103439
51. Harris C, Carson G, Baillie JK, Horby P, Nair H. An evidence-based framework for priority clinical research questions for covid-19. J Glob Health. (2020) 10:011001. doi: 10.7189/jogh.10.011001
52. Barasheed O, Alfelali M, Mushta S, Bokhary H, Alshehri J, Attar AA, et al. Uptake and effectiveness of facemask against respiratory infections at mass gatherings: a systematic review. Int J Infect Dis. (2016) 47:105–11. doi: 10.1016/j.ijid.2016.03.023
53. Leung NHL, Chu DKW, Shiu EYC, Chan KH, McDevitt JJ, Hau BJP, et al. Respiratory virus shedding in exhaled breath and efficacy of face masks. Nat Med. (2020) 26:676–80. doi: 10.1038/s41591-020-0843-2
54. Bartoszko JJ, Farooqi MAM, Alhazzani W, Loeb M. Medical masks vs n95 respirators for preventing covid-19 in healthcare workers: a systematic review and meta-analysis of randomized trials. Influenza Other Respir Viruses. (2020) 14:365–73. doi: 10.1111/irv.12745
55. Noti JD, Lindsley WG, Blachere FM, Cao G, Kashon ML, Thewlis RE, et al. Detection of infectious influenza virus in cough aerosols generated in a simulated patient examination room. Clin Infect Dis. (2012) 54:1569–77. doi: 10.1093/cid/cis237
56. Leggat PA, Kedjarune U. Bacterial aerosols in the dental clinic: a review. Int Dent J. (2001) 51:39–44. doi: 10.1002/j.1875-595X.2001.tb00816.x
57. Zemouri C, de Soet H, Crielaard W, Laheij A. A scoping review on bio-aerosols in healthcare and the dental environment. PLoS ONE. (2017) 12:e0178007. doi: 10.1371/journal.pone.0178007
58. Ashrae Position Document on Infectious Aerosols. Atlanta, GA: American Society for Heating, Ventilating and Air-Conditioning Engineers (2020).
59. Novoselac A, Siegel JA. Impact of placement of portable air cleaning devices in multizone residential environments. Build Environ. (2009) 44:2348–56. doi: 10.1016/j.buildenv.2009.03.023
60. Qian H, Zheng X. Ventilation control for airborne transmission of human exhaled bio-aerosols in buildings. J Thorac Dis. (2018) 10(Suppl 19):S2295–304. doi: 10.21037/jtd.2018.01.24
61. Yang J, Sekhar C, Wai DCK, Raphael B. Computational fluid dynamics study and evaluation of different personalized exhaust devices. HVACandR Res. (2013) 19:934–46. doi: 10.1080/10789669.2013.826066
62. Olmedo I, Nielsen PV, Ruiz de Adana M, Jensen RL, Grzelecki P. Distribution of exhaled contaminants and personal exposure in a room using three different air distribution strategies. Indoor Air. (2012) 22:64–76. doi: 10.1111/j.1600-0668.2011.00736.x
63. Kurnitski J, Boerstra A, Franchimon F, Mazzarella L, Hogeling J, Hovorka F. How to Operate and Use Building Services in Order to Prevent the Spread of the Coronavirus Disease (covid-19) Virus (sars-cov-2) in Workplaces. REHVA European Federation of Heating, Ventilation and Air Conditioning Association (2020). Available online at: https://www.rehva.eu/fileadmin/user_upload/REHVA_COVID-19_guidance_document_V4_23112020.pdf
64. Dentistry Workers and Employers. Washington, DC: Department of Labor - Occupational Safety and Health Administration (2020).
65. Bedell K, Buchaklian AH, Perlman S. Efficacy of an automated multiple emitter whole-room ultraviolet-c disinfection system against coronaviruses MHV and mers-cov. Infect Control Hosp Epidemiol. (2016) 37:598–9. doi: 10.1017/ice.2015.348
66. Walker CM, Ko G. Effect of ultraviolet germicidal irradiation on viral aerosols. Environ Sci Technol. (2007) 41:5460–5. doi: 10.1021/es070056u
Keywords: COVID-19, particle measurement, bioaerosol, dental procedures, particle topography
Citation: Dabiri D, Conti SR, Sadoughi Pour N, Chong A, Dadjoo S, Dabiri D, Wiese C, Badal J, Hoogland MA, Conti HR, Taylor TR, Choueiri G and Amili O (2021) A Multi-Disciplinary Review on the Aerobiology of COVID-19 in Dental Settings. Front. Dent. Med. 2:726395. doi: 10.3389/fdmed.2021.726395
Received: 16 June 2021; Accepted: 24 August 2021;
Published: 29 September 2021.
Edited by:
Martha J. Somerman, Frontiers in Dental Medicine, United StatesReviewed by:
Gehoon Chung, Seoul National University, South KoreaBlake Matthew Warner, National Institutes of Health (NIH), United States
Copyright © 2021 Dabiri, Conti, Sadoughi Pour, Chong, Dadjoo, Dabiri, Wiese, Badal, Hoogland, Conti, Taylor, Choueiri and Amili. This is an open-access article distributed under the terms of the Creative Commons Attribution License (CC BY). The use, distribution or reproduction in other forums is permitted, provided the original author(s) and the copyright owner(s) are credited and that the original publication in this journal is cited, in accordance with accepted academic practice. No use, distribution or reproduction is permitted which does not comply with these terms.
*Correspondence: Darya Dabiri, ZGFyeWEuZGFiaXJpQHV0b2xlZG8uZWR1