- 1Department of Endodontics, University of Washington, Seattle, WA, United States
- 2Department of Restorative Dentistry, University of Washington, Seattle, WA, United States
Objective: Previous research has demonstrated that stem cells of the apical papilla (SCAP) have a lower differentiation potential and are less resistant to cell death as compared to other stem cells. N-acetyl cysteine (NAC) prevents apoptosis of the dental pulp stem cells (DPSCs) by inducing differentiation of these cells. The use of NAC with SCAP could possibly, enhance their differentiation and resistance to cytotoxicity. Hence, the aim of this study was to determine if NAC could prevent apoptosis of SCAP by promoting proliferation and differentiation of these cells thereby contributing to the success of Regenerative endodontic procedures (REPs).
Methods: Human SCAP were cultured with and without 2-hydroxyethyl methacrylate (HEMA), 20 mM NAC and Dexamethasone (Dex). Proliferation rates were analyzed at days 4 and 7. Flow cytometric analysis was used to analyze the levels of cell death. Differentiation of the cells was analyzed using Real-time PCR and an ALP assay. Data were analyzed using ANOVA with a post-hoc Tukey test.
Results: The NAC-treated cells had similar cell viability compared with the controls. The cells treated with NAC + HEMA had significantly higher rates of proliferation as compared to the HEMA only treated groups and displayed more cell viability when these groups were compared with flow cytometric analysis. Real-time PCR and the ALP assay demonstrated that the NAC group upregulated ALP, RUNX-2, and DSPP genes.
Conclusion: The data demonstrated that NAC protects the SCAP from apoptosis and enhances the proliferation and differentiation potential of these cells suggesting that NAC could be used effectively during REPs.
Introduction
Mesenchymal stem cells (MSCs) have attracted tremendous attention over the past years as a source of cells necessary for tissue regeneration, due to their multi-lineage differentiation capability. Dental derived MSCs have gained importance in the past several years because of their availability by non-invasive routine clinical procedures (e.g., extraction of 3rd molars). Furthermore, they have the potential to differentiate into several lineages including osteogenic, odontogenic, adipogenic, chondrogenic, myogenic, and neurogenic (1–5). Regenerative endodontics is defined by the AAE Glossary of terms as “Biologically-based procedures designed to physiologically replace damaged tooth structures, including dentin and root structures, as well as cells of the pulp-dentin complex”. To achieve this goal, Regenerative Endodontic procedures (REPs) utilize the stem cells of the apical papilla (SCAP), to allow teeth with pulp necrosis and immature apices to continue root development (6). SCAP are a unique population of dental stem cells (DSCs) located at the tips of growing tooth roots (3, 5). These stem cells have the potential to differentiate into several cell types, including cells of the pulp-dentin complex. This, combined with their anatomical position and relative resiliency in conditions of pulp necrosis and apical periodontitis, makes them ideal for REPs (5). The desired outcomes of these REPs include radiographic signs of healing, increased root length and thickness, and resolution of symptoms (7). However, the success rates of REPs vary considerably (7–9). This could be due to a variety of factors, which include etiology, the technique, inadequate disinfection, presence of bacteria in the canal, immunomodulatory effects on SCAP and properties of SCAP (10–13). REPs are dependent upon the survival and differentiation of the stem cells of the apical papilla (SCAP) (5). Previous studies have shown that SCAP are more susceptible to activated immune cells and have less differentiation potential, compared to other dental mesenchymal stem cells (12). This presents a challenge in endodontics because SCAP must be able to survive the canal environment and differentiate for the success of REPs, so, if the canal is adequately or inadequately disinfected, the SCAP still pose a challenge in this procedure due to their decreased differentiation capabilities. Hence, if their differentiation capacity could be enhanced along with adequate disinfection during the REP, there is a possibility that the success rates of these REPs would increase and be more consistent.
N-acetylcysteine (NAC) is a non-essential amino acid that has a variety of associated health benefits. NAC is a precursor to glutathione, a known antioxidant (14). The primary health benefit that has been associated with NAC is its antioxidant activity. However, studies have shown that NAC can aid in differentiation of dental pulp stromal cells (DPSCs), which protects these cells from undergoing apoptosis (15). Previous research has demonstrated and documented the beneficial effects of NAC on DPSCs, and SHED (16). NAC has also been shown to have anti-inflammatory properties and prevent apoptosis in dental pulp cells exposed to the cytotoxic agent 2-hydroxyethyl methacrylate (HEMA) or poly (methyl methacrylate) (PMMA) resin (17, 18). Various mechanisms have been discussed in the literature related to the anti-apoptotic and protective effects of NAC on various cells, including the inhibition of caspases, preventing the loss of mitochondrial membrane potential, inhibiting the decrease in NF-κB activity and helping with differentiation of cells (17). However, no studies have analyzed the effects of NAC on SCAP. We believe that adequate disinfection along with differentiation of SCAP in the presence of NAC could possibly increase the success rates of REPs and make these procedures more predictable. Hence, the aim of our study was to evaluate the effects of NAC on human SCAP survival, proliferation, and differentiation.
Materials and Methods
Cell Culture and Reagents
Human SCAP were obtained from Dr. Songtao Shi's lab at the University of Pennsylvania. These cells were maintained in Alpha-Minimum Essential Medium (Alpha MEM; Invitrogen, Carlsbad, CA, United States) supplemented with 10% fetal bovine serum (FBS), 1% penicillin-streptomycin and 1% L-glutamine (Invitrogen; Carlsbad, CA, United States). Cells were incubated at 37°C and 5% CO2. Cells were used only until passage five, since previous research has demonstrated that these stem cells demonstrate a more differentiated phenotype after passage five (19). For the experimental procedures, SCAP were cultured under the following conditions:
1) SCAP alone (negative control)
2) SCAP + 2-hydroxyethylmethacrylate (HEMA) 0.0082M (positive control)
3) SCAP +NAC (20Mm)
4) SCAP +NAC (20Mm) + 2-hydroxyethylmethacrylate (HEMA) 0.0082M
5) SCAP + 10−8M Dexamethasone (Dex) (positive control)
NAC was mixed in a HEPES buffer and sodium chloride prior to use. The cells were treated for the various time points and then analyzed as mentioned in the subsequent sections.
HEMA, NAC, HEPES, and Dex were purchased from Millipore Sigma (St. Louis, MO, United States).
Flow Cytometric Analysis
The SCAP were treated at 1 × 104 cells / well using the various conditions stated above in 12-well plates (n = 6) to analyze the effects of NAC under apoptotic conditions (Figure 1). After a 24-hour incubation period, the cells were analyzed using FITC-Annexin V and Propidium Iodide (PI) staining (Fisher Scientific, Pittsburg, PA, United States) to determine the levels of apoptosis. After treatment with HEMA, NAC, and Dex, the cells were washed twice with phosphate buffered saline (PBS) (Fisher Scientific, Pittsburg, PA, United States) and re-suspended in binding buffer containing FITC-Annexin V/ Propidium iodide (PI) as suggested by the manufacturer. After 15 min of incubation on ice, Annexin V/PI-stained cells were analyzed using a flow cytometry. Flow cytometric analysis was performed using LSR-2 (BD Biosciences, Franklin Lakes, NJ, United States). Dead cell fragments and debris were gated out by forward and side scatter analysis. Analysis included 10,000 events. The numbers in the quadrants in Figure 1 represent the percentages of the early and late apoptotic cells (Q3 and Q2 respectively), necrotic cells (Q1) and the live/ viable cells (Q4).
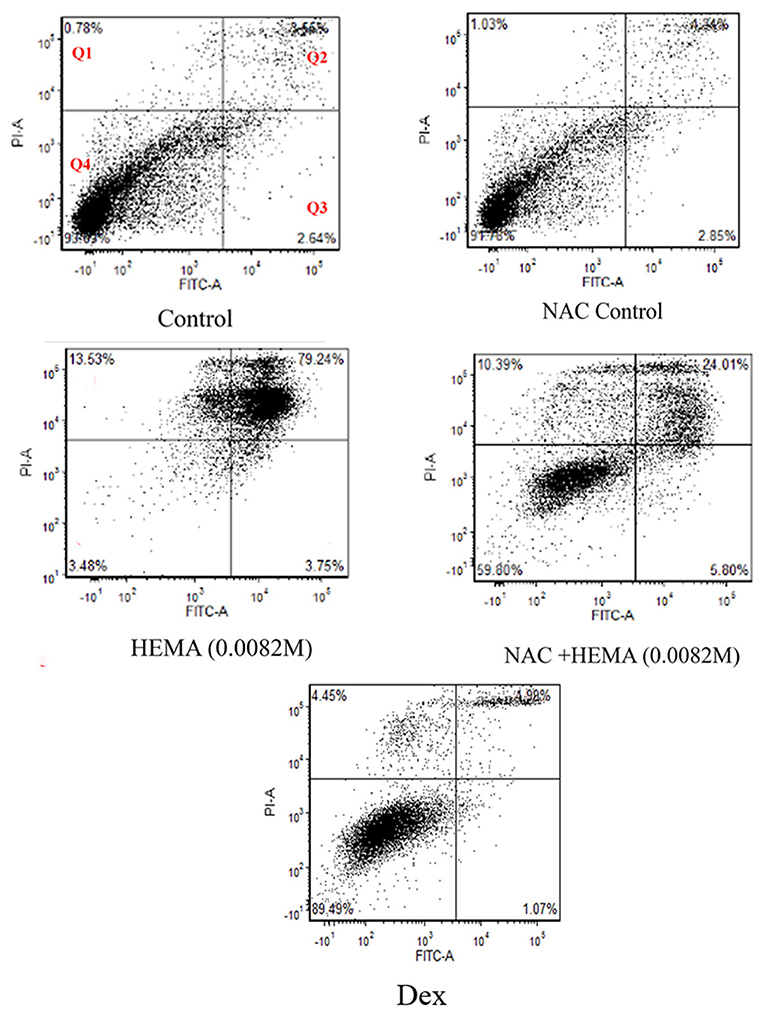
Figure 1. Flow cytometric analysis demonstrated that NAC did not affect cell viability. Cell viability was analyzed after a 24-h treatment using FITC-Annexin V and Propidium Iodide (PI). Cells treated with HEMA showed the highest percentages of late apoptotic and necrotic cells as compared to those treated with NAC and HEMA. The NAC, Dex and the untreated control cells demonstrated similar percentages of viable cells (91.7%, 89.49%, and 93.6% respectively). 10,000 events were analyzed for each group. Q1 are the cells undergoing necrosis, Q2 are the cells in the stage of late apoptosis, Q3 are the cells in early apoptosis, and Q4 represents the viable cells.
Cell Proliferation
SCAP were treated as mentioned previously and the cells were evaluated for proliferation at days 4 and 7. The assay was performed using the WST-1 Cell Proliferation Assay Kit from Millipore Sigma (St. Louis, MO, United States). This colorimetric assay is based on the cleavage of a water-soluble tetrazolium salt (WST-1) to formazan by cellular mitochondrial dehydrogenases. Cells (Control, HEMA, NAC, and Dex) were cultured in 24-well plates and proliferation rates were assessed at days 4 and 7 (n = 8). At the indicated time point, 550 μl of the kit reagent WST-1 with media was added to each well. After 90 min 100 μl was collected from each sample and placed in a 96-well plate. The optical density (OD) was measured using a micro-plate reader at an absorbance of 450nm (Figure 2).
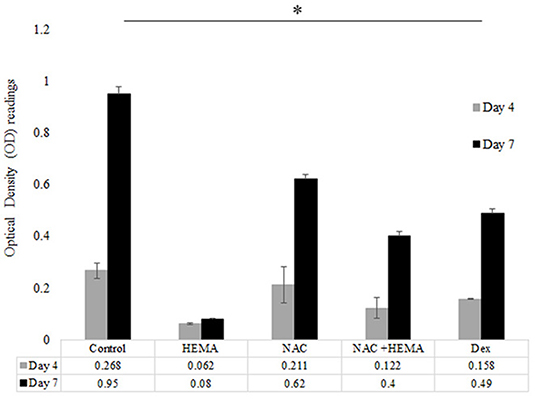
Figure 2. The HEMA and NAC treated groups showed higher proliferation rates as compared to the HEMA only group. Proliferation rates were analyzed at days 4 and 7. The proliferation rates of the HEMA group were low at both time points since most of these cells were apoptotic as seen with the flow cytometic data. However, the NAC and HEMA treated cells showed a statistically significant increase in proliferation rates at day 7. The Dex group had similar levels of proliferation at both times as the NAC treated group. At day 7 the untreated control showed statistically higher levels of proliferation as compared to the other groups (*p < 0.05).
Alkaline Phosphatase Assay
SCAP were treated with the conditions mentioned above (Control, HEMA, NAC, NAC +HEMA, and Dex) (Figure 3). Alkaline phosphatase (ALP) activity is upregulated in pluripotent stem cells undergoing differentiation (18, 20). Cells were treated with HEMA, NAC, and Dex at the same concentrations as in the previous assays and were cultured in 12-well culture plates in culture media for 14 days (n = 6). The ALP activity was assessed on day 14 using the TRACP and ALP assay kit from Takara-Bio (Mountain View, CA, United States). The cells were lysed and incubated with 0.5 mg/mL p-nitro-phenyl phosphate solution. The optical density (OD) was measured using a micro-plate reader at an absorbance of 405 nm. Concentrations were calculated from these OD readings based on the standards provided by the manufacturer.
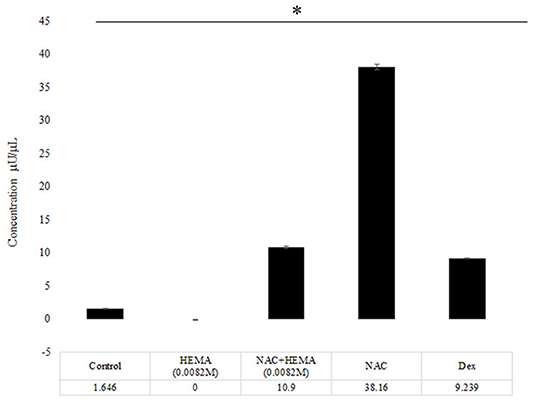
Figure 3. The NAC treated cells showed an increase in the Alkaline Phosphatase levels. At day 14, the NAC treated cells showed a statistically significant increase in the ALP activity as compared to the control cells, HEMA and Dex treated cells (*p < 0.05). The Dex treated cells demonstrated an increase in the ALP levels, but these levels were lower than the NAC group.
RNA Extraction
Human SCAP were treated with HEMA, NAC, and Dex for 24 h (similar concentrations as mentioned previously) in 12-well plates at a concentration of 1 × 105 cells/well and maintained in Dulbecco modified Eagle medium (Invitrogen, Carlsbad, CA, United States) with 10% fetal bovine serum. The total RNA was extracted using the RNAease Micro Kit from Qiagen (Valencia, CA, United States). The RNA was treated with DNase I (Qiagen, Valencia, CA, United States) to ensure the removal of the genomic DNA. The quality of RNA was measured using the NanoDrop Lite (Thermofisher Scientific; Waltham, MA, United States) (A260/A280 ratio).
Real-Time Reverse-Transcriptase Polymerase Chain Reaction
RNA was DNase treated as mentioned in the previous section, and complementary DNA was synthesized from 1.0 μg total RNA with a complementary DNA synthesis kit for reverse-transcriptase polymerase chain reaction (RT-PCR) (Bio-Rad Laboratories; Hercules, CA, United States). No RNA was obtained from the HEMA group due to the cells undergoing apoptosis, which corroborated the results from the Flow cytometric analysis and the Alkaline Phosphatase assay. Two microliters of the resulting complementary DNA product were used per 20 μL of reaction in the QuantStudio-3 Real-Time PCR system (Thermofisher Scientific; Waltham, MA, United States). Real-time PCR analysis was performed with the DNA Master SYBR Green I kit (Bio-Rad Laboratories; Hercules, CA, United States), with a total volume of 20 μL. Expression was analyzed for genes including Dentin Sialophosphoprotein (DSPP), Alkaline Phosphatase (ALP) and Runt-related transcription factor 2 (RUNX-2), with Glyceraldehyde 3-phosphate dehydrogenase (GAPDH) serving as a housekeeping/reference gene for normalization. Primer sequences used for this assay have been described previously (21). The comparative CT method () was used for performing relative quantification (RQ) analysis. The analysis was performed using the Expression Suite Software (version 1.3) from Thermofisher Scientific (Waltham, MA, USA).
Statistical Analysis
Statistical testing was performed using SigmaPlot 11.0 (Systat Software, Inc, San Jose, CA, USA). Data were analyzed by one-way analysis of variance (ANOVA) with a Tukey post-hoc test to analyze differences between the groups. Differences with p < 0.05 were considered significant (*, **, ***).
Results
Flow Cytometric Analysis Correlated Well With the Microscopic Analysis
Flow cytometric analysis revealed very similar cell viability between the untreated SCAP/control cells (93%) and the SCAP treated with NAC (91.78) and Dex (89.49%) as can been seen in Q4 in Figure 1. SCAP treated with HEMA (0.0082 m), displayed only 3.48% viable cells with 79.24% undergoing late apoptosis. SCAP treated with HEMA and NAC displayed 59.8% viable cells with 24% in late apoptosis.
Cell Proliferation Rates of the NAC Treated Cells Were Lower Than the Untreated Controls
Proliferation rates were examined at days 4 and 7 for all groups (Figure 2). At day 4, there was no significant difference in OD absorbance values for all analyzed groups. The HEMA treated group showed the lowest cell proliferation at days 4 and 7. At day 7, the NAC and Dex groups showed lower proliferation rates as compared to the control cells. At day 7, the differences in the proliferation rates between the control and the NAC and Dex treated cells were statistically significant (p < 0.05*). However, the differences in the proliferation rates between the NAC and Dex groups at day 7 were not statistically significant.
NAC Increased ALP Activity of the SCAP
The control group showed some ALP activity at day 14 (Figure 3). The HEMA-treated group showed the lowest amount of ALP activity. The HEMA+NAC group showed slightly higher levels of ALP activity as compared to the HEMA alone group. The NAC group showed a significantly higher levels of ALP activity as compared to all the other groups including the group treated with Dex (p < 0.05*).
NAC Induced Osteogenic and Dentinogenic Gene Expression in SCAP
SCAP were treated with NAC and Dex at the similar concentrations as the previous experiments for 24 h after which gene expression was analyzed. Genes analyzed were Alkaline Phosphatase (ALP), Runt-related transcription factor 2 (RUNX-2) and Dentin Sialophosphoprotein (DSPP) (Figure 4). The log 10 values were normalized to the GAPDH, the housekeeping gene for this assay. The NAC treated cells showed statistically significant higher gene expression levels for all genes tested as compared to the untreated/control cells. The levels of RUNX-2 were not statistically different for the NAC and Dex treated cells although these differences were significant for DSPP and ALP, which correlated well with the data from the ALP assay (Figure 3).
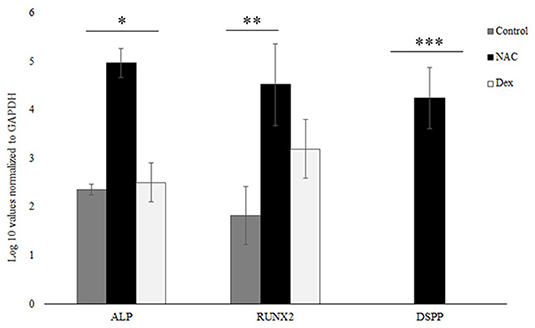
Figure 4. NAC upregulated ALP, RUNX-2, and DSPP. NAC treated upregulated the levels of various odontoblastic genes like ALP, RUNX-2, and DSPP. These values were normalized to the housekeeping gene GAPDH, and the log 10 values are shown here. The NAC treated cells had significantly higher expression levels of these genes as compared to the control cells. The gene expression levels similar for RUNX-2 between the NAC and Dex groups but statistically different for the ALP and the DSPP genes (*, **, *** p < 0.05).
Discussion
The use of dental stem cells has increased in the past decade. This is probably due to their ease of availability and their numbers. Even though these cells have shown a tremendous regenerative capacity, research data related to their characteristics is still lacking. A recent study demonstrated, the “double edged sword” uniqueness of dental stem cells in regulating inflammation (22). Other studies have demonstrated that some of the dental stem cells are more resistant and have varied differentiation potentials as compared to others (12, 23).
Previous research has demonstrated that SCAP are susceptible to apoptosis and the immune system and caused increased secretion of pro-inflammatory cytokines when cultured with activated immune cells (10, 12). This could be because the SCAP are more stem-like as compared to other dental stem cells, which makes them more susceptible to apoptosis (24, 25). Our data here corroborates the data from previous studies, wherein, SCAP was more susceptible to apoptosis and has a decreased differentiation potential. However, if the differentiation potential of these cells could be enhanced, this may lead to increased survival and decreased apoptotic levels.
N-acetyl cysteine (NAC) is a compound that has been shown to be effective in differentiating DPSCs, thereby, making them more resilient and less susceptible to apoptosis (15, 17, 26). However, there is no data available on the effects of NAC on SCAP. If NAC could differentiate SCAP, it could possibly make them less susceptible to apoptosis and may contribute to the success of REPs. A concentration of NAC (20 mM) was used in these experiments as lower concentrations were ineffective and previous studies have shown this concentration worked best with the DPSCs (15, 26). The data in this study demonstrated that this concentration worked well with the SCAP. Dexamethasone was used as another positive control used in this study, since previous studies have shown that this compound can differentiate dental pulp cells into an odontoblastic/osteoblastic phenotype (15, 27).
HEMA is a well-known inducer of apoptosis and was used as one of the positive controls for this study. It has been previous shown to be cytotoxic by increasing the reactive oxygen species (ROS) production; oxidative stress and DNA damage (26, 28).
The SCAP appeared to be susceptible to HEMA mediated apoptosis as seen with the flow cytometric analysis (Figure 1). The percentage of viable cells in the HEMA treated group were the lowest at 3.48%. The control and the NAC and the Dex treated cells showed similar percentages of viable cells 93.6, 91.7, and 89.49% respectively, which demonstrated that NAC does not affect the cells negatively. HEMA treated cells showed decreased levels of apoptosis when treated with NAC. The percentage of viable cells in the NAC+HEMA treated group was about 59.8% as compared to the 3.48% of the HEMA alone treated cells. This data demonstrated that NAC could protect the SCAP from undergoing apoptosis.
In addition to viability, cell functionality is an important factor to consider. Hence, the next step was to check the functionality of the SCAP when treated with NAC. The proliferation rates were analyzed at days 4 and 7. These times points were selected based off previous research studies and showed differences in proliferation over a period of 7 days (12, 29). The proliferation rates of the untreated control, NAC and Dex treated cells were similar at day 4. However, at day 7, the proliferation rates of the NAC and Dex treated groups were significantly lower than the untreated controls, which could be because the differentiating cells have lower proliferation rates (30). The HEMA treated cells had low proliferation rates since most cells were apoptotic, as seen with the flow cytometric analysis (Figures 2, 3). There were statistically significant differences between the control and the experimental groups at day 7 (p < 0.05 *). The differences between the NAC and Dex treated groups at both time points were not statistically significant (Figure 2).
The functionality and differentiation potential of the cells was analyzed using the ALP assay (Figure 3). Previous studies have shown that there is an increase in ALP secretion when stem cells differentiate into odontoblast or osteoblast like cells (15). The results indicated that at day 14, the NAC group had significantly higher levels of ALP as compared to the control, HEMA, HEMA +NAC and the Dex treated group (Figure 3) (p < 0.05*). Previous research has demonstrated that NAC stimulated the DPSCs to express ALP (31). The data here demonstrates that NAC has similar effects on SCAP. The Dex group showed higher levels than the control group but lower than the NAC group and this difference was statistically significant.
The Real-time PCR data demonstrated that NAC caused increased expression of ALP, RUNX-2 and DSPP (Figure 4). The ALP expression from the Real-time PCR data corroborated the data from the ALP assay. RUNX-2 is a transcription factor, which is associated with osteoblastic differentiation and has been shown to be upregulated in preodontoblasts and immature odontoblasts (32, 33). DSPP has been shown to be responsible for encoding dentin phosphoprotein (DPP) and dentin sialoprotein (DSP) and is expressed during odontoblastic differentiation (34, 35). Increase in the expression levels of all these genes suggests that NAC differentiates the SCAP into odontoblasts like cells. Dex also helps in differentiation of the SCAP. However, it did not upregulate the levels of DSPP but upregulated ALP and RUNX-2, suggesting possible differentiation of the SCAP into an osteoblastic phenotype. Furthermore, Dex has shown to have immunosuppressive effects, which could possibly affect the immune cells in the blood clot, which are necessary components for REPs (36). These immune cells are necessary for the differentiation of SCAP (37). Hence, any immunosuppression could adversely affect the outcome of a REP, which is why Dex would not be an ideal candidate for use during REPs and should be avoided. NAC does not have this effect on the immune cells (manuscript in preparation). These results correspond to the increased levels of differentiation of the cells when treated with NAC and Dex (Figures 3, 4). Furthermore, this differentiation aids the anti-apoptotic mechanism for SCAP as previous research has demonstrated that differentiated cells are less likely to undergo apoptosis (15). The HEMA group was not included in the Real-time PCR analysis since no RNA could be extracted from this group due to the apoptosis of the cells as seen from the flow cytometric analysis data (Figure 1).
The success of REPs requires a triad of factors which includes stem cells, growth factors and a scaffold (38). However, additional treatment factors need to be considered for a successful outcome. The tooth must be adequately disinfected to decrease the pro-inflammatory response and SCAP must be preserved (10, 11, 39). A scaffold or matrix must be present that allows for cellular proliferation and differentiation. Growth factors must be present in order to induce the differentiation of the stem cells into the proper cell type to reform the pulp-dentin complex (38). Finally, there must be a bacterial tight seal to prevent the canal space from being re-infected. The existing literature in regenerative endodontics lacks high level of evidence studies and studies with longer follow-up periods are needed (40). Hence, effective methods are needed to disinfect the canal space and promote the survival and differentiation of SCAP. A significant number of research articles have been published about disinfection and the importance of disinfection (11, 41). However, combining disinfection along with differentiation could be key to success in these procedures. The data from this study demonstrates that NAC could be effective in differentiating the SCAP, thereby making them more resilient to apoptosis (Figure 5). Further studies are necessary to delineate the pathway of this differentiation and survival, and, if these differentiated cells, interact differently with the immune system as compared to the undifferentiated SCAP.
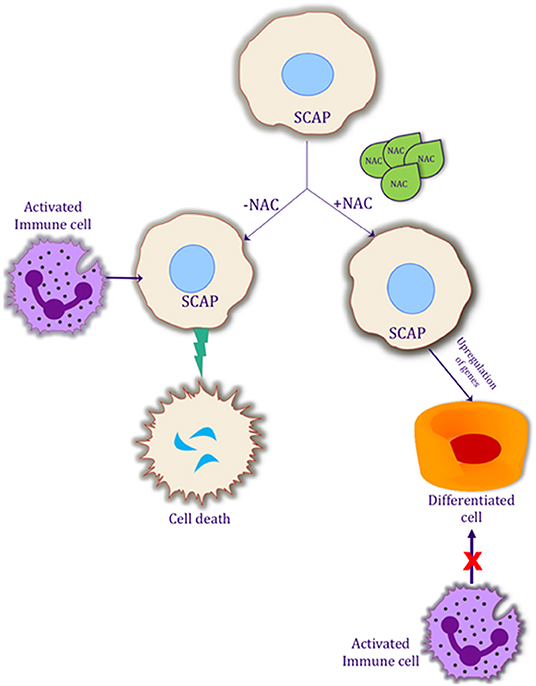
Figure 5. Possible mechanism of action of NAC on SCAP differentiation and its interaction with the activated immune system. Schematic representation of the effects of NAC on SCAP. Differentiation of SCAP helps it to be more resilient to an activated immune system, which in turn could possibly help the procedure of regenerative endodontics.
Conclusions
In summary, this research demonstrates for the first time the anti-apoptotic and differentiation effects of NAC on SCAP, which could be an important factor in the predictability of REPs. As more research continues with SCAP in a variety of medical and dental research, these findings could possibly have important implications in various treatment modalities in regenerative medicine and dentistry.
Data Availability Statement
The raw data supporting the conclusions of this article will be made available by the authors, without undue reservation.
Author Contributions
KC contributed to the drafting of the manuscript. AP contributed to the conception, designing, and the critical revision of the manuscript. Y-WC and JJ contributed to the critical revision of the manuscript. KC and AP contributed to the drawing of the figures. All authors contributed to the article and approved the submitted version.
Conflict of Interest
The authors declare that the research was conducted in the absence of any commercial or financial relationships that could be construed as a potential conflict of interest.
Publisher's Note
All claims expressed in this article are solely those of the authors and do not necessarily represent those of their affiliated organizations, or those of the publisher, the editors and the reviewers. Any product that may be evaluated in this article, or claim that may be made by its manufacturer, is not guaranteed or endorsed by the publisher.
References
1. About I. Dentin regeneration in vitro: the pivotal role of supportive cells. Adv Dent Res. (2011) 23:320-4. doi: 10.1177/0022034511405324
2. Bakopoulou A, About I. Stem cells of dental origin: current research trends and key milestones toward clinical application. Stem Cells Int. (2016) 2016:4209891. doi: 10.1155/2016/4209891
3. Huang GT, Sonoyama W, Liu Y, Liu H, Wang S, Shi S. The hidden treasure in apical papilla: the potential role in pulp/dentin regeneration and bioroot engineering. J Endod. (2008) 34:645-51. doi: 10.1016/j.Joen.2008.03.001
4. Mao JJ, Giannobile WV, Helms JA, Hollister SJ, Krebsbach PH, Longaker MT, et al. Craniofacial tissue engineering by stem cells. J Dent Res. (2006) 85:966-79. doi: 10.1177/154405910608501101
5. Sonoyama W, Liu Y, Yamaza T, Tuan RS, Wang S, Shi S, et al. Characterization of the apical papilla and its residing stem cells from human immature permanent teeth: a pilot study. J Endod. (2008) 34:166-71. doi: 10.1016/j.Joen.2007.11.021
6. Banchs F, Trope M. Revascularization of immature permanent teeth with apical periodontitis: new treatment protocol? J Endod. (2004) 30:196-200. doi: 10.1097/00004770-200404000-00003
7. Jeeruphan T, Jantarat J, Yanpiset K, Suwannapan L, Khewsawai P, Hargreaves MK. Mahidol study 1: comparison of radiographic and survival outcomes of immature teeth treated with either regenerative endodontic or apexification methods: a retrospective study. J Endod. (2012) 38:1330-6. doi: 10.1016/j.Joen.2012.06.028
8. Bose R, Nummikoski P, Hargreaves K. A retrospective evaluation of radiographic outcomes in immature teeth with necrotic root canal systems treated with regenerative endodontic procedures. J Endod. (2009) 35:1343-9. doi: 10.1016/j.Joen.2009.06.021
9. Kahler B, Mistry S, Moule A, Ringsmuth AK, Case P, Thomson A, et al. Revascularization outcomes: a prospective analysis of 16 consecutive cases. J Endod. (2014) 40:333-8. doi: 10.1016/j.Joen.2013.10.032
10. Latham J, Fong H, Jewett A, Johnson JD, Paranjpe A. Disinfection efficacy of current regenerative endodontic protocols in simulated necrotic immature permanent teeth. J Endod. (2016) 42:1218-25. doi: 10.1016/j.Joen.2016.05.004
11. Verma P, Nosrat A, Kim JR, Price JB, Wang P, Bair E, et al. Effect of residual bacteria on the outcome of pulp regeneration in vivo. J Dent Res. (2017) 96:100-6. doi: 10.1177/0022034516671499
12. Whiting D, Chung WO, Johnson JD, Paranjpe A. Characterization of the cellular responses of dental mesenchymal stem cells to the immune system. J Endod. (2018) 44:1126-31. doi: 10.1016/j.Joen.2018.03.018
13. Botero TM, Tang X, Gardner R, Hu JCC, Boynton JR, Holland RG. Clinical evidence for regenerative endodontic procedures: immediate vs. delayed induction? J Endod. (2017) 43:S75-81. doi: 10.1016/j.Joen.2017.07.009
14. Aldini G, Altomare A, Baron G, Vistoli G, Carini M, Borsani L, et al. N-acetylcysteine as an antioxidant and disulphide breaking agent: the reasons why. Free Radic Res. (2018) 52:751-62. doi: 10.1080/10715762.2018.1468564
15. Paranjpe A, Cacalano NA, Hume WR, Jewett A. N-acetylcysteine protects dental pulp stromal cells from hema-induced apoptosis by inducing differentiation of the cells. Free Radic Biol Med. (2007) 43:1394-408. doi: 10.1016/j.Freeradbiomed.2007.07.011
16. Martacic J, Filipovic MK, Borozan S, Cvetkovic Z, Popovic T, Arsic A, et al. N-acetyl-L-Cysteine protects dental tissue stem cells against oxidative stress in vitro. Clin Oral Investig. (2018) 22:2897-2903. doi: 10.1007/S00784-018-2377-2
17. Paranjpe A, Cacalano NA, Hume WR, Jewett A. Mechanisms of N-acetyl cysteine-mediated protection from 2-hydroxyethyl methacrylate-induced apoptosis. J Endod. (2008) 34:1191-7. doi: 10.1016/j.Joen.2008.06.011
18. Yamada M, Kojima N, Paranjpe A, Att W, Aita H, Jewett A, et al. N-Acetyl cysteine (NAC)-assisted detoxification of PMMA resin. J Dent Res. (2008) 87:372-7. doi: 10.1177/154405910808700417
19. Schneider R, Holland GR, Chiego D Jr, Hu JC, Nor JE, Botero MT. White mineral trioxide aggregate induces migration and proliferation of stem cells from the apical papilla. J Endod. (2014) 40:931-6. doi: 10.1016/j.Joen.2013.11.021
20. Kojima N, Yamada M, Paranjpe A, Tsukimura N, Kubo K, Jewett A, et al. Restored viability and function of dental pulp cells on poly-methylmethacrylate (PMMA)-based dental resin supplemented with N-acetyl cysteine (NAC). Dent Mater. (2008) 24:1686-93. doi: 10.1016/j.Dental.2008.04.008
21. Foster BL, Nociti FH Jr, Swanson EC, Matsa-Dunn D, Berry JE, Cupp CJ, et al. Somerman: regulation of cementoblast gene expression by inorganic phosphate in vitro. Calcif Tissue Int. (2006) 78:103-12. doi: 10.1007/S00223-005-0184-7
22. Zhou LL, Liu W, Wu YM, Sun WL, Dorfer CE, Fawzy El-Sayed MK. Oral mesenchymal stem/progenitor cells: the immunomodulatory masters. Stem Cells Int. (2020) (2020):1327405. doi: 10.1155/2020/1327405
23. Hu L, Zhao B, Gao Z, Xu J, Fan Z, Zhang C, et al. Regeneration characteristics of different dental derived stem cell sheets. J Oral Rehabil. (2019) 47:66-72. doi: 10.1111/joor.12839
24. Jewett A, Arasteh A, Tseng HC, Behel A, Arasteh H, Yang W, et al. Strategies to rescue mesenchymal stem cells (MSCs) and dental pulp stem cells (DPSCs) from NK cell mediated cytotoxicity. PLoS ONE. (2010) 5:e9874. doi: 10.1371/Journal.Pone.0009874
25. Tseng HC, Arasteh A, Paranjpe A, Teruel A, Yang W, Behel A, et al. Increased lysis of stem cells but not their differentiated cells by natural killer cells; de-differentiation or reprogramming activates NK Cells. PLoS ONE. (2010) 5:e11590 doi: 10.1371/Journal.Pone.0011590
26. Paranjpe A, Bordador LC, Wang MY, Hume WR, Jewett A. Resin monomer 2-hydroxyethyl methacrylate (HEMA) is a potent inducer of apoptotic cell death in human and mouse cells. J Dent Res. (2005) 84:172-7. doi: 10.1177/154405910508400212
27. Nakamura H, Saruwatari L, Aita H, Takeuchi K, Ogawa T. Molecular and biomechanical characterization of mineralized tissue by dental pulp cells on titanium. J Dent Res. (2005) 84:515-20. doi: 10.1177/154405910508400606
28. Schweikl H, Gallorini M, Poschl G, Urmann V, Petzel C, Bolay C, et al. Buchalla: Functions of transcription factors NF-KappaB and Nrf2 in the inhibition of LPS-stimulated cytokine release by the resin monomer hema. Dent Mater. (2018) 34:1661-78. doi: 10.1016/j.Dental.2018.08.292
29. Machado J, Johnson JD, Paranjpe A. The effects of endosequence root repair material on differentiation of dental pulp cells. J Endod. (2016) 42:101-5. doi: 10.1016/j.Joen.2015.08.007
30. Ruijtenberg S, van den Heuvel S. Coordinating cell proliferation and differentiation: antagonism between cell cycle regulators and cell type-specific gene expression. Cell Cycle. (2016) 15:196-212. doi: 10.1080/15384101.2015.1120925
31. Paranjpe A, Smoot T, Zhang H, Johnson DJ. Direct contact with mineral trioxide aggregate activates and differentiates human dental pulp cells. J Endod. (2011) 37:1691-5 doi: 10.1016/j.Joen.2011.09.012
32. Lei S, Liu XM, Liu Y, Bi J, Zhu S, Chen X. Lipopolysaccharide downregulates the osteo-/odontogenic differentiation of stem cells from apical papilla by inducing autophagy. J Endod. (2020) 46:502-8. doi: 10.1016/j.Joen.2020.01.009
33. Vanacker J, Viswanath A, De Berdt P, Everard A, Cani PD, Bouzin C, et al. Hypoxia modulates the differentiation potential of stem cells of the apical papilla. J Endod. (2014) 40:1410-8. doi: 10.1016/j.Joen.2014.04.008
34. Xiao M, Yao B, Zhang BD, Bai Y, Sui W, Wang W, et al. Stromal-derived factor-1alpha signaling is involved in bone morphogenetic protein-2-induced odontogenic differentiation of stem cells from apical papilla via the Smad and Erk signaling pathways. Exp Cell Res. (2019) 381:39-49. doi: 10.1016/j.Yexcr.2019.04.036
35. Zhu Q, Gibson MP, Liu Q, Liu Y, Lu Y, Wang X, et al. Proteolytic processing of dentin sialophosphoprotein (DSPP) is essential to dentinogenesis. J Biol Chem. (2012) 287:30426-35. doi: 10.1074/jbc.M112.388587
36. Giles AJ, Hutchinson MND, Sonnemann HM, Jung J, Fecci PE, Ratnam NM, et al. Dexamethasone-induced immunosuppression: mechanisms and implications for immunotherapy. J Immunother Cancer. (2018) 6:51. doi: 10.1186/S40425-018-0371-5
37. Liu XM, Liu Y, Yu S, Jiang LM, Song B, Chen X. Potential immunomodulatory effects of stem cells from the apical papilla on treg conversion in tissue regeneration for regenerative endodontic treatment. Int Endod J. (2019) 52:1758-67. doi: 10.1111/iej.13197
38. Murray PE, Garcia-Godoy F, Hargreaves MK. Regenerative endodontics: a review of current status and a call for action. J Endod. (2007) 33:377-90. doi: 10.1016/j.Joen.2006.09.013
39. Tan EE, Quah SY, Bergenholtz G, Rosa V, Hoon Yu VS, Tan KS. Antibiotics used in regenerative endodontics modify immune response of macrophages to bacterial infection. J Endod. (2019) 45:1349-56 doi: 10.1016/j.Joen.2019.08.001
40. Tong HJ, Rajan S, Bhujel N, Kang J, Duggal M, Nazzal H. Regenerative endodontic therapy in the management of non-vital immature permanent teeth: a systematic review-outcome evaluation and meta-analysis. J Endod. (2017) 43:1453-64 doi: 10.1016/j.Joen.2017.04.018
Keywords: apoptosis, differentiation, N-acetylcysteine (NAC), proliferation, SCAP
Citation: Countryman K, Chen Y-W, Johnson JD and Paranjpe A (2022) N-Acetylcysteine Protects the Stem Cells of the Apical Papilla. Front. Dent. Med. 3:848081. doi: 10.3389/fdmed.2022.848081
Received: 03 January 2022; Accepted: 10 May 2022;
Published: 30 May 2022.
Edited by:
Bruno Cavalcanti, University of Michigan, United StatesReviewed by:
Mohammed El-Awady Grawish, Mansoura University, EgyptEhsan Taghiabadi, Tehran University of Medical Sciences, Iran
Copyright © 2022 Countryman, Chen, Johnson and Paranjpe. This is an open-access article distributed under the terms of the Creative Commons Attribution License (CC BY). The use, distribution or reproduction in other forums is permitted, provided the original author(s) and the copyright owner(s) are credited and that the original publication in this journal is cited, in accordance with accepted academic practice. No use, distribution or reproduction is permitted which does not comply with these terms.
*Correspondence: Avina Paranjpe, YXZpbmFAdXcuZWR1