- 1Laboratory of Characterization and Analysis of Biomaterials, Faculty of Dentistry, Federal University of Alagoas, Brazil
- 2Laboratory of Quality Control of Drugs, Medicines, Foods and Biomaterials, Institute of Pharmaceutical Sciences, Federal University of Alagoas, Brazil
Despite the huge improvements made in adhesive technology over the past 50 years, there are still some unresolved issues regarding the durability of the adhesive interface. A complete sealing of the interface between the resin and the dentin substrate remains difficult to achieve, and it is doubtful whether an optimal interdiffusion of the adhesive system within the demineralized collagen framework can be produced in a complete and homogeneous way. In fact, it is suggested that hydrolytic degradation, combined with the action of dentin matrix enzymes, destabilizes the tooth-adhesive bond and disrupts the unprotected collagen fibrils. While a sufficient resin–dentin adhesion is usually achieved immediately, bonding efficiency declines over time. Thus, here, a review will be carried out through a bibliographic survey of scientific articles published in the last few years to present strategies that have been proposed to improve and/or develop new adhesive systems that can help prevent degradation at the adhesive interface. It will specially focus on new clinical techniques or new materials with characteristics that contribute to increasing the durability of adhesive restorations and avoiding the recurrent replacement restorative cycle and the consequent increase in damage to the tooth.
Introduction
To achieve an effective and complete marginal sealing and clinical longevity, an ideal tooth–restoration interface must have long-lasting durability. In enamel, adhesion is formed by resinous monomers that polymerize in demineralized areas, creating a more stable and stronger adhesive interface. The durability of the resin–dentin bond depends on the quality of a microinterface, the so-called hybrid layer, which is achieved by the infiltration and polymerization of resinous monomers of the adhesive system through a network of collagen fibrils exposed by the action of acids on the dentin surface and into dentinal tubules and, consequently, by the resistance to degradation of each one of the bond components. While the enamel is a dry substrate, with hydroxyapatite (92% v.) being the main component of its mineral phase, the dentin is the opposite, which is a complex and wet substrate with an average 45% (v) of hydroxyapatite crystals, 33% (v.) of organic matrix, mostly collagen type I, and 22% of water by volume (1, 2).
Although the exact mechanism responsible for hybrid layer degradation has not been completely understood, this process is thought to involve the hydrolytic degradation of monomers in water-rich zones within the hybrid layer, followed by enzymatic action on exposed collagen fibrils with relevant implications for the maintenance of the integrity of the adhesive interface (3). Many attempts to develop new functional adhesives that limit enzymatic degradation and polymer damage and have antimicrobial action have been presented (1, 4). Some strategies to modify the dentin surface, such as reducing the remaining demineralized collagen and applying ethanol to reduce water, seem to help increase the immediate bond strength. However, no progress has been made toward a synthesis of new materials as well as improving or modifying techniques in order to achieve good long-term results.
This review discusses recent clinical techniques and materials with characteristics that contribute to increasing the durability of adhesive restorations. The objective of this study is to present strategies that have been proposed in recent years for the improvement and/or development of new adhesive systems that can help prevent degradation at the adhesive interface.
Matrix metalloproteinase inhibitors and collagen cross-linking agents
Matrix metalloproteinases (MMPs) are a group of more than 25 secreted and membrane-bound enzymes responsible for damage to the pericellular substrate. The main organic component of the tooth structure is collagen, and MMPs that degrade collagen and the extracellular matrix have been held responsible for the degradation of the hybrid layer (5).
The organic constituents of the hybrid layer, especially collagen, undergo degradation because of the enzymatic activity of MMPs, among other proteases present in the dentin matrix (6, 7). When the pH of the environment decreases to 4.5 or less, such as during the carious process and during the acid etching of the dentin surface that is carried out during the adhesive procedure, the MMPs that are in a latent state are activated and their action triggered by way of degrading the collagen that may have remained exposed underneath the formed hybrid layer. On the other hand, the acidic adhesive systems can also induce the activation of MMPs in the dentinal substrate. Thus, even if there is an inhibition of a part of the MMPs exposed by the demineralization of the dentin tissue, other MMPs would be activated in the presence of acidic adhesives (6, 8).
Once activated, collagenases cleave triple helical collagen molecules into two fragments of one-quarter and three-quarter length. The first fragment of one-quarter contains the C-terminal part of the collagen molecule and the second one of three-quarter contains the N-terminal portion (9). Collagen loses its triple helical conformation and can then be degraded by gelatinases MMP-2 and MMP-9 (10). MMP-2 and MMP-9 damage the non-helical fragments and reduce them to even smaller peptide fragments. The binding sites of collagen to MMPs are very similar to the catalytic sites of these enzymes. Thus, the collagen-binding domain of MMP-2 and MMP-9 binds to a short segment of the collagen α1 chain (11). After binding to the substrate, the water molecule that is bound to the zinc of the catalytic domain attacks the substrate and, through the transfer of protons, promotes a cleavage of the peptides (12). Endogenous MMPs in the dentin matrix are presumed to bind to collagen peptides via their hemopexin-like domains (MMP-1, MMP-8) and/or their fibronectin-like domains (MMP-2, MMP-9) (13).
Studies have been carried out with several substances in an attempt to inhibit MMPs, aiming to improve the durability of the adhesive interface (14). However, issues such as the substantivity of MMP inhibitors and their release from polymer matrices remain (15). Synthetic or natural bioactive agents that inhibit the endogenous enzymes of the organic collagen matrix have been used in an attempt to produce a strong and durable bonding interface (14), and chlorhexidine (CHX) is the most widely used (16). These compounds can be applied in different ways; for example, they can be incorporated into the acid-etching gel, added into the adhesive system, or applied directly on the dentin surface after acid etching, while remaining in contact with the dentin surface (14, 17–27).
MMP inhibitors act through different mechanisms such as by chelating cations (17, 28, 29), collagen cross-linking (30–32), and competitive inhibition for active sites of the collagen molecule (12, 33, 34). In Table 1, which is modified from de Moraes et al. (35), different techniques and materials using various types of MMP inhibitors and their mechanisms of action are presented. Many studies have applied CHX as a potential inhibitor of MMPs (36–45). CHX can interact with the organic components of the dentin matrix. This interaction occurs through electrostatic forces, between CHX-protonated amine groups, mineral phosphates, and non-collagenous phosphoproteins, which, in turn, are closely related to collagen fibrils (46). The preservation of collagen integrity in the hybrid layer occurs through the interaction of CHX with the sulfhydryl group on the domain or cysteine located at the active site of the MMPs. This may distort MMP molecules and prevent them from binding to substrates, thus competing for the binding of calcium and zinc ions to MMPs because of their chelating action. Without these ions, MMPs will lose their catalytic activities (47). In vitro studies have shown that CHX can preserve the bond durability of adhesives up to 12 months (37, 38, 44). Nonetheless, the clinical trials carried out by Araújo et al. (41), Sartori et al. (42), and Valério et al. (40) concluded, after a 2-, 3-, and 4-year follow-up, respectively, that the addition of CHX into the primer of the self-etch adhesive or the application of CHX on the etched dentin did not provide any clinical advantages nor did it improve the clinical durability of adhesive restorations, compared with the groups without CHX.
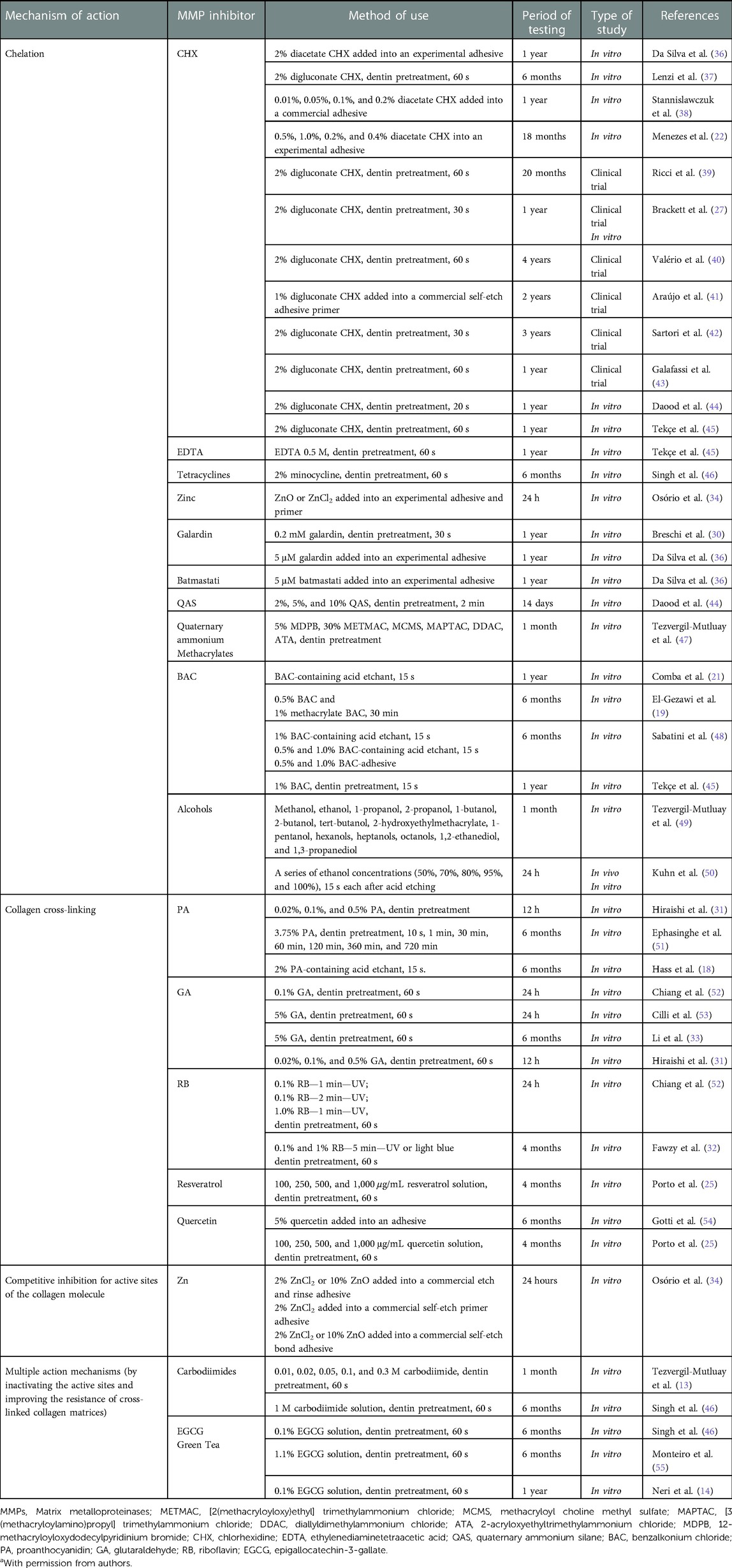
Table 1 Matrix metalloproteinases inhibitors and strategies to improve the stability of the dentin/resin bonding [Moraes et al (35)]a.
Of late, other compounds have been presented, such as 2% doxycycline (56), 1,10-phenanthroline (57), captopril (58), ion-releasing filler (59), and Emblica officinalis (Indian gooseberry or amla) as an acid etchant and MMP inhibitor. Amla juice has a similar etching effect as a self-etch adhesive in SEM and 100% amla extract has been found to inhibit MMP-9 by gelatin zymography (60) and a novel mussel-inspired monomer [N-(3,4-dihydroxyphenethyl) methacrylamide] (61) with the aim of preserving the bonding between the restorative material and the tooth wall.
Polymer hydrolysis
Although several studies consider adhesion to enamel to be a reliable approach, adhesion to dentin remains a crucial challenge (62). Differences in hydrophilicity and water content of adhesives directly influence the durability of interfaces. The presence of water plays an important role both in the prior acid-etching technique and in the self-etching technique. Water is an essential component in self-etching systems, as it enables the ionization of acidic monomers that demineralize the underlying enamel and/or dentin (63). In addition to having water in their composition, the ionizable groups of acidic monomers are hydrophilic. Therefore, different water sorption rates can be expected for the bonding interfaces produced by different bonding systems (64). Newer systems such as Universal or Multi-mode adhesives offer the option of selecting a conventional or self-etching bonding strategy, or an alternate strategy of “selective enamel etching” and self-etching into dentin. Despite the composition being similar to that of older self-etching adhesives, universal ones contain specific functional monomers of carboxylate and/or phosphate, with one of the main adhesives being 10-methacryloyloxydecyl dihydrogen phosphate (10-MDP), which ionically binds to dentin and is more effective and stable in water than that provided by other functional monomers (65).
There are several factors involved in the sorption of water and degradation of polymers, such as the following: the pH of the storage medium (66); conversion degree (67); the polarity of the molecular structure and the presence of hydroxyl groups able to form hydrogen bonds with water and the number of crosslinks (68); the presence of residual water and the presence and type of filler particles (69).
Hydrolysis is considered the main reason for resin degradation within the hybrid layer, contributing to a reduction in bond strength over time. After penetrating the polymer matrix, the water triggers the process of chemical degradation, resulting in the generation of oligomers and monomers. Water sorption and solubility rates presented by adhesive systems after polymerization are important for indirectly determining the longevity and marginal quality of the restoration (28). It has long been known that moisture present in the oral or storage environment plays an important role in the process of chemical degradation of polymers and has a deleterious effect on the resin–dentin interface (70).
Leaching is facilitated by the penetration of water into weakly cross-linked bonds or hydrophilic groups in the adhesive. Hydrophilic groups show limited monomer/polymer conversion because of adhesive phase separation (71) and a lack of compatibility between the hydrophobic photoinitiator and the hydrophilic phase (72). The poorly polymerized hydrophilic phase degrades rapidly in aqueous media (73). The structure of methacrylate adhesives, featuring hydrolytically susceptible groups such as ester and urethane, as well as hydroxyl, carboxyl, and phosphate groups (74), can be hydrolyzed by chemical and enzymatic degradation in an oral environment (75), and the collagen matrix previously infiltrated by resin becomes susceptible to attack by proteolytic enzymes (73).
Adhesive systems typically employ 2-bis[4-(2-hydroxy-3-methacryloxypropoxy)-phenyl]-propane (Bis-GMA) as the base monomer and low-viscosity 2-hydroxyethyl methacrylate (HEMA) as a monomer thinner to improve material quality and handling properties and to ensure proper dentin infiltration (76). However, adhesive durability and protective ability are often compromised by a failure of the tooth/restoration interface (77, 78). The issue of increasing durability and that of toxicity require the development of longer-lasting dental products without Bis-GMA/HEMA in adhesive systems.
New monomers and polymerization systems have been suggested to bypass the process of hydrolysis of the Bis-GMA/HEMA system (79), for example, triethylene glycol vinyl-benzyl-ether (TEGBE) monomer, which is a hydrolytically stable ether-based monomer (80). This compound has a dumbbell-shaped amphiphilic structure with a hydrophilic core and two hydrophobic vinyl-benzyl groups. Its polymerization mechanism does not show the limitation of diffusion and provides a higher degree of conversion (81). Song et al. (68). showed that the functional amino silane methacrylate monomer (ASMA) is able to act simultaneously as a co-monomer and co-initiator with the ability to develop a simplified adhesive that achieves greater durability and mechanical properties.
Adhesive systems with antimicrobial activity
Adding antimicrobial substances to dental adhesives has been shown to improve tooth–restoration bonding (82), inhibiting the growth and multiplication of remaining bacteria (82, 83), while preventing the entry of new microorganisms and even limiting the influence of the factors that initiate the chemical and enzymatic degradation of dental adhesives (84). As a result, the durability of the tooth–restoration interface increases and there is a reduction in secondary caries.
The search for materials with antibacterial properties resulted in the development of new dental adhesives with antimicrobial agents, with inorganic fillers, modified monomers, or additives incorporated both in the polymeric matrix and in the filler particles (85–87) such as zinc oxide nanoparticles (88), silica nanoparticles functionalized with amphotericin B (89), silver nanoparticles (90–92), modified monomers containing quaternary ammonium (93), flavonoids (94, 95), plant extracts (94, 96), gold nanoparticles (97), calcium phosphate (87, 98), zinc oxide (99), and titanium dioxide (100, 101). Aguiar et al. (102) compiled various studies wherein commercially available antimicrobial adhesives (Clearfil™ SE Protect Bond/MDPB, Gluma 2 Bond/glutaraldehyde, Peak Universal Bond/chlorhexidine) and experimental materials or commercial adhesives modified with antimicrobial agents, including materials with quaternary ammonium methacrylate (QAM), dimethylamine dodecyl methacrylate (DMADDM), and dimethylamine hexa-decyl methacrylate (DMAHDM), silver nanoparticles (NAg), titanium dioxide (TiO2), zinc oxide (ZnO2)], silver- or zinc-doped bioactive active glass (BAG), titanium, and copper iodide, and compounds such as triclosan, quercetin, and grape seed extract, were analyzed. Compared with control groups, all these tested compounds showed the best results of inhibition for all tested pathogens, both single-layer bacteria and biofilm, without any adverse effects on the physicochemical and mechanical properties of adhesive systems.
Silver nanoparticles, either pure or mixed with other substances, were tested. The addition of silver nanoparticles in dental adhesives demonstrated specific antibacterial activity at the tooth–restoration interface (103, 104). In 2020, Münchow et al. (83) combined two processing methods (electrospinning and cryomilling) to obtain bioactive fillers based on fibers containing a strong MMP inhibitor, doxycycline, with antimicrobial and metalloproteinase inhibitor properties. The filler was used to obtain bioactive adhesives with the potential to inhibit MMPs and antibacterial activity against S. mutans and Lactobacillus, without compromising the physical–mechanical properties or the bond strength (up to 12 months).
Bacterial resistance against silver is difficult to achieve because of its multiple antibacterial mechanisms (105): (a) interaction with life-sustaining enzymes and blocking of the electron transport system in bacteria (106) and the thiol group of enzymes deactivating them, leading to bacterial cell death (107); (b) binding to bacterial cells by interacting with their cell membranes or cell walls. This can inhibit the movement of the organism or trigger leakage or rupture of the membrane (107, 108) (c) penetration inside the cell and the damage to intracellular structures (mitochondria, vacuoles, ribosomes) and biomolecules (protein, lipids, and DNA) (109); and (d) the formation of an organometallic complex when silver ions bind to the amino acids. Thus, silver ions can be generated inside the bacterial cell when this organometallic complex breaks down. The accumulation of silver ions in the cell can inactivate bacterial DNA and RNA, and it can damage and rupture the cell membrane, causing cell death (110).
Bonding agents with the potential for biomimetic remineralization
To help remineralization of hard dental tissue is a desirable property in dentin bonding agents. Biomimetic remineralization is being considered a replacement for the restorative approach, which currently follows a minimally invasive procedure. Thus, selective caries tissue removal has become a clinical reality in deep cavities where demineralized dentin is left on the pulp areas and sealed with restoration (111).
In this sense, adhesion is a clinical challenge because conventional adhesives have low bond strength when applied to demineralized or contaminated dentin, as this dentin layer can be only partially infiltrated by resin monomers (112), becoming more prone to hydrolytic and/or enzymatic degradation, which may compromise the durability of composite resin restorations (113, 114).
Thus, the development of bioactive restorative materials capable of remineralizing mineral-depleted sites at the dentin–adhesive interface is one of the main aims of dental research (112, 115–117).
Studies using bioactive glasses incorporated in experimental adhesives provided mineral gain, increased microhardness, and better sealing of the resin–dentin interface (112, 115). The mechanism is based on the ability to release Si(OH)4 from the bioactive glasses, which binds to collagen exposed by acid etching and polymerizes into an absorbent SiO2-rich layer. This layer can aid in the precipitation of amorphous calcium phosphate (118) and subsequent conversion to nonstoichiometric apatite (119). In addition, a mineral-rich alkaline environment can allow a condensation of Si(OH)4 and precipitation of Ca2+ and PO4−3, and this can help fossilize metalloproteinases and cathepsins and reduce their proteolytic activity (28, 120).
It was also found that the use of materials with zinc can reduce metalloproteinase-mediated collagen degradation within poorly infiltrated hybrid layers and decayed dentin, protecting the sensitive collagen cleavage sites within demineralized dentin (121, 122), as zinc ions act as inhibitors of MMP-2 and MMP-9 (123).
The use of casein phosphopeptide-amorphous calcium phosphate (CPP-ACP) may be associated with an increased longevity of the dentin–resin interface (124–126) through the release, deposition, and stabilization of gradients with a high concentration of calcium and phosphate ions on the tooth (127).
The self-assembling peptide P11−4 (CH3CO-QQRFEWEFEQQ-NH3) was designed as a β-sheet-forming peptide that assembles into hierarchical structures in response to environmental triggers, resulting in the formation of a 3D biomimetic scaffold that is capable of nucleating hydroxyapatite, since the anionic groups present in P11−4 side chains attract calcium ions, inducing a hierarchical precipitation of calcium phosphate salts onto the preformed scaffold (128, 129). The self-assembled peptide possibly controls the deposition and growth of hydroxyapatite crystals on enamel (130–132). The behavior and performance of P11−4 in dentin has already been described (125, 126, 133) and may be a promising method in the treatment of caries-affected dentin, increasing the longevity of bonded restorations.
Sodium fluoride has also been shown to be a possible chemical inhibitor of MMPs (134, 135). However, Moreira et al. (126) did not detect an increase in bond strength when using sodium fluoride compared with CPP-ACP and peptide P11−4.
A complete remineralization of caries-affected dentin remains a challenge, but biomimetic remineralization strategies suggest that it is possible to promote mineral precipitation within the hybrid layer and reduce collagen degradation. However, more studies are needed to define viable clinical protocols and ascertain their long-term efficacy.
Removal of residual water not bound to collagen
It is common knowledge that the adhesion of resin materials to conditioned enamel is significantly higher than that of dentin, which is mainly attributed to the characteristics of each substrate (136). The presence of water in the dentin hybrid layer during polymerization interferes with the quality of hybridization in this layer (137) and can cause hydrolytic degradation (138), a low-quality mechanical property (138), and nanoleakage (139).
Due to these problematics related to the presence of water in the hybrid layer, adhesive systems containing solvent and less hydrophilic monomers are preferred for residual water removal (139, 140). The types of solvent most commonly used in these systems are ethanol, acetone, and water. Ethanol binds to residual water through hydrogen bonds, increasing evaporation rates, and is more effective than water as a solvent. On the other hand, solvents containing acetone show promising performance in a simplified adhesive system, which uses a single bottle containing hydrophilic and hydrophobic monomers together (141). In addition, acetone contains a higher vapor pressure in addition to the water-chasing effect, which is believed to induce a greater removal of residual water (142). However, the application of both types of solvents in challenging clinical situations, as in non-carious cervical lesions, yields similar clinical performance and the same survival rates (143).
An alternate technique to remove residual water is called “ethanol wet bonding,” which is applied on etched and rinsed dentin, inducing the replacement of water by ethanol. In this way, ethanol carries out the process of chemical dehydration, increasing the interfibrillar spaces because of the additional interfibrillar shrinkage and reducing the hydrophilicity of the collagen matrix (139). However, it is necessary to point out that this technique is not a new one (144). Over a period of time, the scientific literature presents in vitro studies evaluating this technique, showing favorable and promising results (144, 145). However, when ethanol wet bonding is applied in in vivo studies or clinical trials, the results are not promising (50). Thus, the presence of sound dentin with a constant permeability through the dentinal tubules is a persistent challenge for the complete removal of residual water by ethanol (50, 147).
Other adhesive techniques with the same objective are proposed: the application of multiple adhesive layers or a layer of a hydrophobic resin agent and air-blowing the adhesive with high intensity are possibilities reported in the literature as viable (147). Likewise, a polar aprotic solvent, dimethyl sulfoxide (DMSO), was proposed as a component that improves adhesive infiltration and reduces residual water from the resin–dentin interface, thus improving the bond strength between resin-based materials and etched dentin (148).
It is relevant to emphasize the evaluation of adhesive systems and application techniques, from etch-and-rinse ones (by three or two steps) with a wet dentin substrate, as already mentioned, to a self-etch adhesive system (by two or single step) and a universal one, which allow working on a dry substrate, free from moisture (149, 150). Therefore, based on short-term randomized clinical trials, self-etch and etch-and-rinse adhesive systems applied with multiple coats appear to yield satisfactory clinical performance (149, 151). Also, as observed in a long-term clinical trial (13 years), according to a van Djiken study, a continuous and similar bonding degradation for all adhesive systems evaluated in non-carious cervical lesions was demonstrated (152).
Conclusion and points of view for further research
Adhesive systems have improved over the years with regard to their interaction with the dental substrate, material composition, and technique. Even so, to increase the durability of the adhesive interface remains a challenge. The measures to improve the dental bonding systems presented in this review have been driven by the need to make the best use of the material to resolve issues such as enzymatic and hydrolytic degradation, preservation of the adhesive interface bonding strength over time, and the development of new bioactive products.
There is huge potential for the development of better, stronger, and bio-based materials purposely made from chemicals derived from renewable biological resources, such as natural polymers, propolis, vegetable oils, plant extracts, resins, and bioactive compounds. However, the accessed studies were done in a laboratory environment and in a short period of time. Even with excellent laboratorial results, the materials require further clinical investigation in different substrates (sound and carious dentin, abraded and sclerotic dentin) over a longer period of time in a challenging oral environment so that better results can be achieved and the presented evidence validated.
Author contributions
ICCMP conceived the original draft. ICCMP, TLGFL, RFR, RBEL, and MABS discussed the original draft and wrote the manuscript, and all authors dealt with a specific topic in the article and contributed to the literature searches, analysis, interpretation, and writing of the manuscript. All authors contributed to the article and approved the submitted version.
Conflict of interest
The authors declare that the research was conducted in the absence of any commercial or financial relationships that could be construed as a potential conflict of interest.
Publisher's note
All claims expressed in this article are solely those of the authors and do not necessarily represent those of their affiliated organizations or those of the publisher, the editors, and the reviewers. Any product that may be evaluated in this article, or claim that may be made by its manufacturer, is not guaranteed or endorsed by the publisher.
References
1. Perdigão J. Current perspectives on dental adhesion: (1) dentin adhesion—not there yet. Jpn Dent Sci Rev. (2020) 56:190–207. doi: 10.1016/j.jdsr.2020.08.004
2. Tjäderhane L, Carrilho MR, Breschi L, Tay FR, Pashley DH. Dentin basic structure and composition—an overview. Endod Topics. (2012) 20:3–29. .org/doi: 10.1111/j.1601-1546.2012.00269.x
3. Tjäderhane L, Buzalaf MA, Carrilho M, Chaussain C. Matrix metalloproteinases and other matrix proteinases in relation to cariology: the era of ‘dentin degradomics’. Caries Res. (2015) 49:193–208. doi: 10.1159/000363582
4. Melo MA, Orrego S, Weir MD, Xu HHK, Arola DD. Designing multiagent dental materials for enhanced resistance to biofilm damage at the bonded interface. ACS Appl Mater Interfaces. (2016) 8:11779–87. doi: 10.1021/acsami.6b01923
5. Anshida VP, Kumari RA, Murthy CS, Samuel A. Extracellular matrix degradation by host matrix metalloproteinases in restorative dentistry and endodontics: an overview. J Oral Maxillofac Pathol. (2020) 24:352–60. doi: 10.4103/jomfp.JOMFP_34_20
6. Almahdy A, Koller G, Sauro S, Bartsch JW, Sherriff M, Watson TF, et al. Effects of MMP inhibitors incorporated within dental adhesives. J Dent Res. (2012) 91:605–11. doi: 10.1177/0022034512446339
7. Epasinghe DJ, Yiu CKY, Burrow MF, Hiraishi N, Tay FR. The inhibitory effect of proanthocyanidin on soluble and collagen-bound proteases. J Dent. (2013) 41:832–39. doi: 10.1016/j.jdent.2013.06.002
8. Niu LN, Zhang W, Pashley DH, Breschi L, Mao J, Chen JH, et al. Biomimetic remineralization of dentin. Dent Mater. (2014) 30:77–96. doi: 10.1016/j.dental.2013.07.013
9. Seseogullari-Dirihan R, Mutluay MM, Pashley DH, Tezvergil-Mutluay A. Is the inactivation of dentin proteases by crosslinkers reversible? Dent Mater. (2017) 33:e62–8. doi: 10.1016/j.dental.2016.09.036
10. Pessoa JI, Guimarães GN, Viola NV, da Silva WJ, de Souza AP, Tjäderhane L, et al. In situ study of the gelatinase activity in demineralized dentin from rat molar teeth. Acta Histochem. (2013) 115:245–51. doi: 10.1016/j.acthis.2012.07.002
11. Fields GB. New strategies for targeting matrix metalloproteinases. Matrix Biol. (2015) 44–46:239–46. doi: 10.1016/j.matbio.2015.01.002
12. Jacobsen JA, Jourden JLM, Miller MT, Cohen SM. To bind zinc or not to bind zinc: an examination of innovative approaches to improved metalloproteinase inhibition. Biochim Biophys Acta Mol Cell Res. (2010) 1803:1872–94. doi: 10.1016/j.bbamcr.2009.08.006
13. Tezvergil-Mutluay A, Mutluay M, Seseogullari-Dirihan R, Agee KA, Key WO, Scheffel DL, et al. Effect of phosphoric acid on the degradation of human dentin matrix. J Dent Res. (2013) 92:87–91. doi: 10.1177/0022034512466264
14. Neri R, Yamauti M, Dasilveira FD, Mendonça JS, De Carvalho RM, Santiago SL. Influence of dentin biomodification with epigallocatechin-3-gallate on the bond strength of self-etch adhesive: twelve-month results. Int J Adhes Adhes. (2016) 71:81–6. .org/doi: 10.1016/j.ijadhadh.2016.08.007
15. Liu Y, Tjäderhane L, Breschi L, Mazzoni A, Li N, Mao J, et al. Limitations in bonding to dentin and experimental strategies to prevent bond degradation. J Dental Res. (2011) 90:953–68. doi: 10.1177/0022034510391799
16. Kiuru O, Sinervo J, Vähänikkilä H, Anttonen V, Tjäderhane L. MMP inhibitors and dentin bonding: systematic review and meta-analysis. Int J Dent. (2021) 27:9949699. doi: 10.1155/2021/9949699
17. Montagner F, Sarkis-Onofre R, Pereira-Cenci T, Cenci MS. MMP inhibitors on dentin stability: a systematic review and meta-analysis. J Dent Res. (2014) 93:733–43. doi: 10.1177/0022034514538046
18. Hass V, De Paula AM, Parreiras S, Gutiérrez MF, Luque-Martinez I, De Paris Matos T, et al. Degradation of dentin-bonded interfaces treated with collagen cross-linking agents in a cariogenic oral environment: an in situ study. J Dent. (2016) 49:60–7. doi: 10.1016/j.jdent.2016.02.009
19. El Gezawi M, Haridy R, Elazm EA, Al-Harbi F, Zouch M, Kaisarly D. Microtensile bond strength, 4-point bending and nanoleakage of resin dentin interfaces: effects of two matrixmetalloproteinase inhibitors. J Mech Behav Biomed Mater. (2018) 78:206–13. doi: 10.1016/j.jmbbm.2017.11.024
20. Siqueira FSF, Hilgemberg B, Araujo LCR, Hass V, Bandeca MC, Reis A, et al. Effect of phosphoric acid containing MMP-inactivator on the properties of resin bonding to eroded dentin. J Adhes Dent. (2019) 21:149–58. doi: 10.3290/j.jad.a42324
21. Comba A, Maravic T, Valente L, Girlando M, Cunha SR, Checchi V, et al. Effect of benzalkonium chloride on dentin bond strength and endogenous enzymatic activity. J Dent. (2019) 85:25–32. doi: 10.1016/j.jdent.2019.04.008
22. Menezes LR, Silva EO, Rocha LVM, Barbosa IF, Tavares MR. The use of clays for chlorhexidine-controlled release as a new perspective for longer durability of dentin adhesion. J Mater Sci Mater Med. (2019) 30:132. doi: 10.1007/s10856-019-6344-5
23. Simmer FS, Silva EM, Bezerra RSG, Miranda MESNG, Noronha-Filho JD, Amaral CM. Bond stability of conventional adhesive system with MMP inhibitors to superficial and deep dentin. J Mech Behav Biomed Mater. (2019) 100:103402. doi: 10.1016/j.jmbbm.2019.103402
24. Islam MS, Hiraishi N, Nassar M, Sono R, Otsuki M, Takatsura T, et al. In vitro effect of hesperidin on root dentin collagen and de/re-mineralization. Dent Mater J. (2012) 31:362–67. doi: 10.4012/dmj.2011-203
25. Porto ICCMP, Nascimento TG, Oliveira JMS, Freitas PH, Haimeur A, França R. Use of polyphenols as a strategy to prevent bond degradation in the dentin-resin interface. Eur J Oral Sci. (2018) 126:1–13. doi: 10.1111/eos.12403
26. Huang B, Cvitkovitch DG, Santerre JP, Finer Y. Biodegradation of resin-dentin interfaces is dependent on the restorative material, mode of adhesion, esterase or MMP inhibition. Dent Mater. (2018) 34:1253–62. doi: 10.1016/j.dental.2018.05.008
27. Brackett MG, Li N, Brackett WW, Sword RJ, Qi YP, Niu LN, et al. The critical barrier to progress in dentine bonding with the etch-and-rinse technique. J Dent. (2011) 39:238–48. doi: 10.1016/j.jdent.2010.12.009
28. Tjäderhane L, Nascimento FD, Breschi L, Mazzoni A, Tersariol IL, Geraldeli S, et al. Optimizing dentin bond durability: control of collagen degradation by matrix metalloproteinases and cysteine cathepsins. Dent Mater. (2013) 29:116–35. doi: 10.1016/j.dental.2012.08.004
29. Moon P, Weaver J, Brooks C. Review of matrix metalloproteinases’ effect on the hybrid dentin bond layer stability and chlorhexidine clinical use to prevent bond failure. Open Dent J. (2010) 4:147–52. doi: 10.2174/1874210601004010147
30. Breschi L, Martin P, Mazzoni A, Nato F, Carrilho M, Tjäderhane L, et al. Use of a specific MMP-inhibitor (galardin) for preservation of hybrid layer. Dent Mater. (2010) 26:571–8. doi: 10.1016/j.dental.2010.02.007
31. Hiraishi N, Sono R, Sofiqul I, Yiu C, Nakamura H, Otsuki M, et al. In vitro evaluation of plant-derived agents to preserve dentin collagen. Dent Mater. (2013) 29:1048–54. doi: 10.1016/j.dental.2013.07.015
32. Fawzy S, Nitisusanta LI, Iqbal K, Daood U, Neo J. Riboflavin as a dentin crosslinking agent: ultraviolet A versus blue light. Dent Mater. (2012) 28:1284–91. doi: 10.1016/j.dental.2012.09.009
33. Li J, Chen B, Hong N, Wu S, Li Y. Effect of baicalein on matrix metalloproteinases and durability of resin-dentin bonding. Oper Dent. (2018) 43:426–36. doi: 10.2341/17-097-L
34. Osorio R, Yamauti M, Osorio E, Román JS, Toledano M. Zinc-doped dentin adhesive for collagen protection at the hybrid layer. Eur J Oral Sci. (2011) 119:401–10. doi: 10.1111/j.1600-0722.2011.00853.x
35. de Moraes IQS, do Nascimento TG, da Silva AT, de Lira LMSS, Parolia A, Porto ICCM. Inhibition of matrix metalloproteinases: a troubleshooting for dentin adhesion. Restor Dent Endod. (2020) 45:e31. doi: 10.5395/rde.2020.45.e31
36. Da Silva EM, De Sá Rodrigues CU, De Oliveira Matos MP, De Carvalho TR, Dos Santos GB, Amaral CM. Experimental etch-and-rinse adhesive systems containing MMP-inhibitors: physicochemical characterization and resin-dentin bonding stability. J Dent. (2015) 43:1491–7. doi: 10.1016/j.jdent.2015.10.004
37. Lenzi T, Tedesco TK, Soares FZM, Loguercio AD, Rocha RO. Chlorhexidine application for bond strength preservation in artificially-created caries-affected primary dentin. Int J Adhes Adhes. (2014) 54:51–6. .org/doi: 10.1016/j.ijadhadh.2014.04.007
38. Stanislawczuk R, Pereira F, Muñoz MA, Luque I, Farago PV, Reis A, et al. Effects of chlorhexidine-containing adhesives on the durability of resin-dentin interfaces. J Dent. (2014) 42:39–47. doi: 10.1016/j.jdent.2013.11.002
39. Ricci HA, Sanabe ME, de Souza Costa CA, Pashley DH, Hebling J. Chlorhexidine increases the longevity of in vivo resin-dentin bonds. Euro J Oral Sci. (2010) 118:411–6. doi: 10.1111/j.1600-0722.2010.00754.x
40. Valério RA, Galo R, Galafassi D. Four-year clinical prospective follow-up of resin composite restoration after selective caries removal using Er:YAG laser. Clin Oral Invest. (2020) 24:2271–83. doi: 10.1007/s00784-019-03082-w
41. Araújo MS, Souza LC, Apolonio FM, Barros LO, Reis A, Loguercio AD, et al. Two-year clinical evaluation of chlorhexidine incorporation in two-step self-etch adhesive. J Dent. (2015) 43:140–8. doi: 10.1016/j.jdent.2014.07.010
42. Sartori N, Stolf SC, Silva SB, Lopes GC, Carrilho M. Influence of chlorhexidine digluconate on the clinical performance of adhesive restorations: a 3-year follow-up. J Dent. (2013) 41:1188–95. doi: 10.1016/j.jdent.2013.09.004
43. Galafassi D, Scatena C, Galo R, Cury-Zotti FA, Corona SAM, Borsatto MC. Clinical evaluation of composite restorations in Er:YAG laser-prepared cavities re-wetting with chlorhexidine. Clin Oral Invest. (2017) 21:1231–41. doi: 10.1007/s00784-016-1897-x
44. Daood D, Yiu CKY, Burrow MF, Niu LN, Tay FR. Effect of a novel quaternary ammonium silane cavity disinfectant on durability of resin-dentine bond. J Dent. (2017) 60:77–86. doi: 10.1016/j.jdent.2017.03.003
45. Tekçe N, Tuncer S, Bemirci M, Balci S. Do matrix metalloproteinase inhibitors improve the bond durability of universal dental adhesives? Scanning. (2016) 38:535–44. doi: 10.1002/sca.21293
46. Singh P, Nagpal R, Singh UP. Effect of dentin biomodifiers on the immediate and long-term bond strengths of a simplified etch and rinse adhesive to dentin. Restor Dent Endod. (2017) 42:188–99. doi: 10.5395/rde.2017.42.3.188
47. Tezvergil-Mutluay A, Agee KA, Uchiyama T, Imazato S, Mutluay MM, Cadenaro M, et al. The inhibitory effects of quaternary ammonium methacrylates on soluble and matrix-bound MMPs. J Dent Res. (2011) 90:535–40. doi: 10.1177/0022034510389472
48. Sabatini C, Kim JH, Ortiz Alias P. In vitro evaluation of benzalkonium chloride in the preservation of adhesive interfaces. Oper Dent. (2014) 39:283–90. doi: 10.2341/13-131-LR
49. Tezvergil-Mutluay A, Agee KA, Hoshita T, Uchiyama T, Tjaderhane L, Breschi L, et al. Inhibition of MMPs by alcohols. Dent Mater. (2011) 27:926–33. doi: 10.1016/j.dental.2011.05.004
50. Kuhn E, Farhat P, Teitebaum AP, Mena-Serrano A, Loguercio AD, Reis A, et al. Ethanol-wet bonding technique: clinical versus laboratory findings. Dent Mater. (2015) 31:1030–37. doi: 10.1016/j.dental.2015.05.010
51. Epasinghe D, Yiu C, Burrow M. Effect of proanthocyanidin incorporation into dental adhesive on durability of resin–dentin bond. Int J Adhes Adhes. (2015) 63:145–51. doi: 10.1016/j.dental.2014.08.371
52. Chiang YS, Chen YL, Chuang SF, Wu CM, Wei PJ, Han CF, et al. Riboflavin-ultraviolet-A-induced collagen cross-linking treatments in improving dentin bonding. Dent Mater. (2013) 29:682–92. doi: 10.1016/j.dental.2013.03.015
53. Cilli R, Prakki A, De Araújo PA, França FMG, Amaral FLB. Influence of glutaraldehyde priming on bond strength of an experimental adhesive system applied to wet and dry dentine. J Dent. (2009) 37:212–18. doi: 10.1016/j.jdent.2008.11.017
54. Gotti VB, Feitosa VP, Sauro S, Correr-Sobrinho L, Leal FB, Stansbury JW, et al. Effect of antioxidants on the dentin interface bond stability of adhesives exposed to hydrolytic degradation. J Adhes Dent. (2015) 17:35–44. doi: 10.3290/j.jad.a33515
55. Monteiro TMA, Basting RT, Turssi CP, França FGM, Amaral FLB. Influence of natural and synthetic metalloproteinase inhibitors on bonding durability of an etch-and-rinse adhesive to dentin. Int J Adhes Adhes. (2013) 47:83–8. .org/doi: 10.1016/j.ijadhadh.2013.09.020
56. Parsaie Z, Firouzmandi M, Mohammadi N. Evaluating the effect of pretreatment with matrix metalloproteinase inhibitors on the shear bond strength of composite resin to primary teeth dentin: a 6-month in vitro study. Contemp Clin Dent. (2021) 12:408–13. doi: 10.4103/ccd.ccd_662_20
57. Yang H, Lin XJ, Liu Q, Yu H. Effects of protease inhibitors on dentin erosion: an in situ study. Clin Oral Investig. (2022). doi: 10.1007/s00784-022-04657-w
58. Shu C, Zheng X, Wang Y, Xu Y, Zhang D, Deng S. Captopril inhibits matrix metalloproteinase activity and improves dentin bonding durability. Clin Oral Investig. (2022) 26:3213–25. doi: 10.1007/s00784-021-04303-x
59. Mendes Soares IP, Anselmi C, Guiné I, Fernandes LO, Pires MLBA, de Souza Costa CA, et al. Inhibitory activity of S-PRG filler on collagen-bound MMPs and dentin matrix degradation. J Dent. (2022) 124:104237. doi: 10.1016/j.jdent.2022.104237
60. Rajkumar DS, Mariswamy AB. Comparative evaluation of Emblica officinalis as an etchant and an MMP inhibitor with orthophosphoric acid and chlorhexidine on the microshear bond strength of composite resin: an ex vivo study. Restor Dent Endod. (2021) 46:e36. doi: 10.5395/rde.2021.46.e36
61. Li K, Ngo FM, Yau AYL, Tam WWL, Tse ECM, Tsoi JKH, et al. Mussel-inspired monomer—a new selective protease inhibitor against dentine collagen degradation. Dent Mater. (2022) 38:1149–61. doi: 10.1016/j.dental.2022.05.002
62. Frassetto A, Breschi L, Turco G, Marchesi G, Di Lenarda R, Tay FR, et al. Mechanisms of degradation of the hybrid layer in adhesive dentistry and therapeutic agents to improve bond durability—a literature review. Dent Mater. (2016) 32:e41–53. doi: 10.1016/j.dental.2015.11.007
63. Rosa WL, Piva E, Silva AF. Bond strength of universal adhesives: a systematic review and meta-analysis. J Dent. (2015) 43:765–76. https://doi.org/doi: 10.1016/j.jdent.2015.04.003
64. Mortier E, Gerdolle DA, Jacquot B, Panighi MM. Importance of water sorption and solubility studies for couple bonding agent/resin-based filling material. Oper Dent. (2004) 29:669–76.15646223
65. Nagarkar S, Theis-Mahon N, Perdigão J. Universal dental adhesives: current status, laboratory testing, and clinical performance. J Biomed Mater Res B Appl Biomater. (2019) 107:2121–31. doi: 10.1002/jbm.b.34305
66. Prakki A, Cilli R, Mondelli RF, Kalachandra S, Pereira JC. Influence of pH environment on polymer based dental material properties. J Dent. (2005) 33:91–8.15683889
67. Ge XP, Ye Q, Song LY, Laurence JS, Spencer P. Synthesis and evaluation of a novel co-initiator for dentin adhesives: polymerization kinetics and leachables study. JOM. (2015) 67:796–803. doi: 10.1007/s11837-015-1335-6
68. Song L, Sarikaya R, Ye Q, Misra A, Tamerler C, Spencer P. Multifunctional monomer acts as co-initiator and crosslinker to provide autonomous strengthening with enhanced hydrolytic stability in dental adhesives. Dent Mater. (2020) 36:284–95. doi: 10.1016/j.dental.2019.11.007
69. Tay FR, Pashley DH, Yiu C, Cheong C, Hashimoto M, Itou K, et al. Nanoleakage types and potential implications: evidence from unfilled and filled adhesives with the same resin composition. Am J Dent. (2004) 17:182–90. doi: 10.1016/j.dental.2008.09.011
70. Reis A, Grande RH, Oliveira GM, Lopes GC, Loguercio AD. A 2-year evaluation of moisture on microtensile bond strength and nanoleakage. Dent Mater. (2007) 23:862–70. .org/doi: 10.1016/j.dental.2006.05.005
71. Spencer P, Wang Y. Adhesive phase separation at the dentin interface under wet bonding conditions. J Biomed Mater Res. (2002) 62:447–56. doi: 10.1002/jbm.10364
72. Ye Q, Park J, Topp E, Spencer P. Effect of photoinitiators on the in vitro performance of a dentin adhesive exposed to simulated oral environment. Dent Mater. (2009) 25:452–8. doi: 10.1016/j.dental.2008.09.011
73. Perdigão J, Reis A, Loguercio AD. Dentin adhesion and MMPs: a comprehensive review. J Esthet Restor Dent. (2013) 25:219–41. .org/doi: 10.1111/jerd.12016
74. Feitosa VP, Ogliari FA, Van Meerbeek B, Watson TF, Yoshihara K, Ogliari AO, et al. Can the hydrophilicity of functional monomers affect chemical interaction? J Dent Res. (2014) 93:201–6. doi: 10.1177/0022034513514587
75. Ferracane JL. Hygroscopic and hydrolytic effects in dental polymer networks. Dent Mater. (2006) 22:211–22. doi: 10.1016/j.dental.2005.05.005
76. Spencer P, Wang Y, Walker MP, Wieliczka DM, Swafford JR. Interfacial chemistry of the dentin/adhesive bond. J Dent Res. (2000) 79:1458–63. doi: 10.1177/00220345000790070501
77. Tjäderhane L. Dentin bonding: can we make it last? Oper Dent. (2015) 40:4–18. doi: 10.2341/14-095-BL
78. Wang Y, Spencer P, Walker MP. Chemical profile of adhesive/caries-affected dentin interfaces using Raman microspectroscopy. J Biomed Mater Res Part A. (2007) 81(2):279–86. doi: 10.1002/jbm.a.30981
79. Song LY, Ye Q, Ge XP, Misra A, Tamerler C, Spencer P. New silyl-functionalized BisGMA provides autonomous strengthening without leaching for dental adhesives. Acta Biomater. (2019) 83:130–9. doi: 10.1016/j.actbio.2018.10.033
80. Gonzalez-Bonet A, Kaufman G, Yang Y, Wong C, Jackson A, Huyang G, et al. Preparation of dental resins resistant to enzymatic and hydrolytic degradation in oral environments. Biomacromolecules. (2015) 16:3381–8. doi: 10.1021/acs.biomac.5b01069
81. Yang Y, Urbas A, Gonzalez-Bonet A, Sheridan RJ, Seppala JE, Beers KL, et al. A composition-controlled cross-linking resin network through rapid visible-light photo-copolymerization. Polym Chem. (2016) 7:5023–30. doi: 10.1038/s41598-018-23939-6
82. Osorio R, Osorio E, Aguilera FS, Medina-Castillo AL, Toledano M, Toledano-Osorio M. Silver improves collagen structure and stability at demineralized dentin: a dynamic-mechanical and Raman analysis. J Dent. (2018) 79:61–7. doi: 10.1016/j.jdent.2018.10.003
83. Münchow EA, da Silva AF, Piva E, Cuevas-Suárez CE, de Albuquerque MTP, Pinal R, et al. Development of an antibacterial and anti-metalloproteinase dental adhesive for long-lasting resin composite restorations. J Mater Chem B. (2020) 8:10797–811. doi: 10.1039/d0tb02058c
84. Jun SK, Yang SA, Kim YJ, El-Fiqi A, Mandakhbayar N, Kim DS, et al. Multi-functional nano-adhesive releasing therapeutic ions for MMP-deactivation and remineralization. Sci Rep. (2018) 8:5663. doi: 10.1038/s41598-018-23939-6
85. Almaroof A, Niazi SA, Rojo L, Mannocci F, Deb S. Evaluation of dental adhesive systems incorporating an antibacterial monomer eugenyl methacrylate (EgMA) for endodontic restorations. Dent Mater. (2017) 33:e239–54. doi: 10.1016/j.dental.2017.01.016
86. André CB, Gomes BPFA, Duque TM, Rosalen PL, Chan DCN, Ambrosano GMB, et al. Antimicrobial activity, effects on Streptococcus mutans biofilm and interfacial bonding of adhesive systems with and without antibacterial agent. Int J Adhes Adhes. (2017) 72:123–9. doi: 10.1016/j.ijadhadh.2016.10.011
87. Li Y, Hu X, Ruan J, Arola DD, Ji C, Weir MD, et al. Bonding durability, antibacterial activity and biofilm pH of novel adhesive containing antibacterial monomer and nanoparticles of amorphous calcium phosphate. J Dent. (2019) 81:91–101. doi: 10.1016/j.jdent.2018.12.013
88. Gutiérrez MF, Alegría-Acevedo LF, Méndez-Bauer L, Bermudez J, Dávila-Sánchez A, Buvinic S, et al. Biological, mechanical and adhesive properties of universal adhesives containing zinc and copper nanoparticles. J Dent. (2019) 82:45–55. doi: 10.1016/j.jdent.2019.01.012
89. Lino MM, Paulo CS, Vale AC, Vaz MF, Ferreira LS. Antifungal activity of dental resins containing amphotericin B-conjugated nanoparticles. Dent Mater. (2013) 29:e252–62. doi: 10.1016/j.dental.2013.07.023
90. Degrazia FW, Leitune VC, Garcia IM, Arthur RA, Samuel SM, Collares FM. Effect of silver nanoparticles on the physicochemical and antimicrobial properties of an orthodontic adhesive. J Appl Oral Sci. (2016) 24:404–10. doi: 10.1590/1678-775720160154
91. Dutra-Correa M, Leite AABV, de Cara SPHM, Diniz IMA, Marques MM, Suffredini IB, et al. Antibacterial effects and cytotoxicity of an adhesive containing low concentration of silver nanoparticles. J Dent. (2018) 77:66–71. doi: 10.1016/j.jdent.2018.07.010
92. Thomas R, Snigdha S, Bhavitha KB, Babu S, Ajith A, Radhakrishnan EK. Biofabricated silver nanoparticles incorporated polymethyl methacrylate as a dental adhesive material with antibacterial and antibiofilm activity against Streptococcus mutans. 3 Biotech. (2018) 8:404. doi: 10.1007/s13205-018-1420-y
93. Wang W, Zhu S, Zhang G, Wu F, Ban J, Wang L. Antibacterial and thermomechanical properties of experimental dental resins containing quaternary ammonium monomers with two or four methacrylate groups. RSC Adv. (2019) 9:40681–88. doi: 10.1039/c9ra07788j
94. Yang H, Li K, Yan H, Liu S, Wang Y, Huang C. High-performance therapeutic quercetin-doped adhesive for adhesive-dentin interfaces. Sci Rep. (2017) 7:8189. doi: 10.1038/s41598-017-08633-3
95. Neri JR, Yamauti M, Feitosa VP, Pires AP, Araújo RS, Santiago SL. Physicochemical properties of a methacrylate-based dental adhesive incorporated with epigallocatechin-3-gallate. Braz Dent J. (2014) 25:528–31. doi: 10.1590/0103-6440201300096
96. Lee YC, Cho SG, Kim SW, Kim JN. Anticariogenic potential of Korean native plant extracts against Streptococcus mutans. Planta Med. (2019) 85:1242–52. doi: 10.1055/a-1013-1364
97. Dadkan S, Khakbiz M, Ghazanfari L, Chen M, Lee K-B. Evaluation of antibacterial and mechanical features of dental adhesives containing colloidal gold nanoparticles. J Mol Liquids. (2022) 365:119824. .org/doi: 10.1016/j.molliq.2022.119824
98. Keskar M, Sabatini C, Cheng C, Swihart MT. Synthesis and characterization of silver nanoparticle-loaded amorphous calcium phosphate microspheres for dental applications. Nanoscale Adv. (2018) 1:627–35. doi: 10.1039/c8na00281a
99. Garcia IM, Leitune VCB, Visioli F, Samuel SMW, Collares FM. Influence of zinc oxide quantum dots in the antibacterial activity and cytotoxicity of an experimental adhesive resin. J Dent. (2018) 73:57–60. doi: 10.1016/j.jdent.2018.04.003
100. Sodagar A, Akhoundi MSA, Bahador A, Jalali YF, Behzadi Z, Elhaminejad F, et al. Effect of TiO2 nanoparticles incorporation on antibacterial properties and shear bond strength of dental composite used in orthodontics. Dental Press J Orthod. (2017) 22(5):67–74. doi: 10.1590/2177-6709.22.5.067-074.oar
101. Balhaddad AA, Garcia IM, Mokeem L, Alsahafi R, Collares FM, Sampaio de Melo MA. Metal oxide nanoparticles and nanotubes: ultrasmall nanostructures to engineer antibacterial and improved dental adhesives and composites. Bioengineering. (2021) 8:146. doi: 10.3390/bioengineering8100146
102. Aguiar JD, Youssef MN, Medeiros IS. Adhesive systems modified with antimicrobial agents: a literature review. Clin Lab Res Den. (2020) 25:1–17. doi: 10.11606/issn.2357-8041.clrd.2020.164731
103. Zhang K, Cheng L, Imazato S, Antonucci JM, Lin NJ, Lin-Gibson S, et al. Effects of dual antibacterial agents MDPB and nano-silver in primer on microcosm biofilm, cytotoxicity and dentine bond properties. J Dent. (2013) 41:464–74. doi: 10.1016/j.jdent.2013.02.001
104. Zhang K, Li F, Imazato S, Cheng L, Liu H, Arola DD, et al. Dual antibacterial agents of nano-silver and 12-methacryloyloxydodecylpyridinium bromide in dental adhesive to inhibit caries. J Biomed Mater Res B Appl Biomater. (2013) 101:929–38. doi: 10.1002/jbm.b.32898
105. Mei ML, Lo ECM, Chu CH. Arresting dentine caries with silver diamine fluoride: what’s behind it? J Dent Res. (2018) 97:751–58. doi: 10.1177/0022034518774783
106. Marx DE, Barillo DJ. Silver in medicine: the basic science. Burns. (2014) 40(Suppl 1):S9–18. doi: 10.1016/j.burns.2014.09.010
107. Janaki AC, Sailatha E, Gunasekaran S, Sekar T, Gopalakrishnan M. Synthesis, characteristics and antimicrobial activity of Ag nanoparticles. Int J Adv Sci Technol Eng Manag Sci. (2016) 2:10–17. doi: 10.1177/0022034516639271
108. Dibrov P, Dzoiba J, Gosink KK, Häse CC. Chemiosmotic mechanism of antimicrobial activity of Ag(+) in Vibrio cholera. Antimicrob Agents Chemother. (2002) 46:2668–70. doi: 10.1128/AAC.46.8.2668-2670.2002
109. Tian J, Wong KK, Ho CM, Lok CN, Yu WY, Che CM, et al. Topical delivery of silver nanoparticles promotes wound healing. Chem Med Chem. (2007) 2:129–36. doi: 10.1002/cmdc.200600171
110. Lansdown AB, Silver I. Its antibacterial properties and mechanism of action. J Wound Care. (2002) 11:125–30. doi: 10.12968/jowc.2002.11.4.26389
111. Schwendicke F, Frencken JE, Bjorndal L, Maltz M, Manton DJ, Ricketts D, et al. Managing carious lesions: consensus recommendations on carious tissue removal. Adv Dent Res. (2016) 28:58–67. doi: 10.1177/0022034516639271
112. Schwendicke F, Al-Abdi A, Moscardó AP, Cascales AF, Sauro S. Remineralization effects of conventional and experimental ion-releasing materials in chemically or bacterially-induced dentin caries lesion. Dent Mater. (2019) 35:772–9. doi: 10.1016/j.dental.2019.02.021
113. Yoshiyama M, Tay FR, Doi J, Nishitani Y, Yamada T, Itou K, et al. Bonding of self-etch and total-etch adhesives to carious dentin. J Dent Res. (2002) 81:556–60. doi: 10.1177/154405910208100811
114. Yoshiyama M, Doi J, Nishitani Y, Itota T, Tay FR, Carvalho RM, et al. Bonding ability of adhesive resins to caries-affected and caries-infected dentin. J Appl Oral Sci. (2004) 12:171–6. doi: 10.1590/s1678-77572004000300002
115. Sauro S, Osorio R, Watson TF, Toledano M. Therapeutic effects of novel resin bonding systems containing bioactive glasses on mineral-depleted areas within the bonded-dentine interface. J Mater Sci Mater Med. (2012) 23:1521–32. doi: 10.1007/s10856-012-4606-6
116. Sauro S, Osorio R, Watson TF, Toledano M. Influence of phosphoproteins’ biomimetic analogs on remineralization of mineral-depleted resin-dentin interfaces created with ion-releasing resin-based systems. Dent Mater. (2015) 31:759–77. doi: 10.1016/j.dental.2015.03.013
117. Ny S, Fathy SM, Osman MF. Effect of using biominetic analogs on dentin remineralization with bioactive cements. Braz Dent J. (2020) 31:44–51. doi: 10.1590/0103-6440202003083
118. Sauro S, Pashley DH. Strategies to stabilize dentine-bonded interfaces through remineralizing operative approaches—state of the art. Int J Adhes Adhes. (2016) 69:39–57. .org/doi: 10.1016/j.ijadhadh.2016.03.014
119. Hoppe A, Guldal NS, Boccaccini AR. A review of the biological response to ionic dissolution products from bioactive glasses and glass-ceramics. Biomaterials. (2011) 32:2757–74. doi: 10.1016/j.biomaterials.2011.01.004
120. Padovano JD, Ravindran S, Snee PT, Ramachandran A, Bedran-Russo AK, George A. DMP1-derived peptides promote remineralization of human dentin. J Dent Res. (2015) 94:608–14. doi: 10.1177/0022034515572441
121. Osorio R, Yamauti M, Osorio E, Ruiz-Requena ME, Pashley DH, Tay FR, et al. Zinc reduces collagen degradation in demineralized human dentin explants. J Dent. (2011) 39:148–53. doi: 10.1016/j.jdent.2010.11.005
122. Osorio R, Cabello I, Medina-Castillo AL, Osorio E, Toledano M. Zinc-modified nanopolymers improve the quality of resin-dentin bonded interfaces. Clin Oral Investig. (2016) 20:2411–20. doi: 10.1007/s00784-016-1738-y
123. de Souza AP, Gerlach RF, Line SR. Inhibition of human gingival gelatinases (MMP-2 and MMP-9) by metal salts. Dent Mater. (2000) 16:103–8. doi: 10.1016/s0109-5641(99)00084-6
124. Li H, Li T, Li X, Zhang Z, Li P, Li Z. Morphological effects of MMPs inhibitors on the dentin bonding. Int J Clin Exp Med. (2015) 8:10793–803. doi: 10.1021/ja021047i
125. Barbosa-Martins LF, Sousa JP, Alves LA, Davies RPW, Puppin-Rontanti RM. Biomimetic mineralizing agents recover the micro tensile bond strength of demineralized dentin. Materials. (2018) 11:1733. .org/doi: 10.3390/ma11091733
126. Moreira KM, Bertassoni LE, Davies RP, Joia F, Hofling JF, Nascimento FD, et al. Impact of biomoneralization on resin/biomineralized dentin bond longevity in a minimally invasive approach: an “in vitro” 18-month follow-up. Dent Mater. (2021) 37:e276–89. doi: 10.1016/j.dental.2021.01.021
127. Poggio C, Lombardini M, Vigorelli P, Ceci M. Analysis of dentin/enamel remineralization by a CPP-ACP paste: AFM and SEM study. Scanning. (2013) 35:366–74. doi: 10.1002/sca.21077
128. Aggeli A, Bell M, Carrick LM, Fishwick CW, Harding R, Mawer PJ, et al. Ph as a trigger of peptide beta-sheet self-assembly and reversible switching between nematic and isotropic phases. J Am Chem Soc. (2003) 125:9619–28. .org/doi: 10.1021/ja021047i
129. Kirkham J, Firth A, Vernals D, Boden N, Robinson C, Shore RC, et al. Self-assembling peptide scaffolds promote enamel remineralization. J Dent Res. (2007) 86:426–30. doi: 10.1177/154405910708600507
130. Kind L, Stevanovic S, Wuttig S, Wimberger S, Hofer J, MuÃàller B. Biomimetic remineralization of carious lesions by self-assembling peptide. J Dent Res. (2017) 96:790–7. doi: 10.1177/0022034517698419
131. Silvertown JD, Wong BPY, Sivagurunathan KS, Abrams SH, Kirkham J, Amaechi BT. Remineralization of natural early caries lesions in vitro by P11-4 monitored with photothermal radiometry and luminescence. J Investig Clin Dent. (2017) 8:e12257. doi: 10.1111/jicd.12257
132. Bröseler F, Tietmann C, Bommer C, Drechsel T, Heinzel-Gutenbrunner M, Jepsen S. Randomized clinical trial investigating self-assembling peptide P11-4 in the treatment of early caries. Clin Oral Investig. (2020) 24:123–32. doi: 10.1038/s41598-020-60815-8
133. de Sousa JP, Carvalho RG, Barbosa-Martins LF, Torquato RJS, Mugnol KCU, Nascimento FD, et al. The self-assembling peptide P11-4 prevents collagen proteolysis in dentin. J Dent Res. (2019) 98:347–54. .org/doi: 10.1177/0022034518817
134. Kato MT, Bolanho A, Zarella BL, Salo T, Tjäderhane L, Buzalaf MA. Sodium fluoride inhibits MMP-2 and MMP-9. J Dent Res. (2014) 93:74–7. doi: 10.1177/0022034513511820
135. Altinci P, Mutluay M, Seseogullari-Dirihan R, Pashley D, Tja ¨derhane L, Tezvergil-Mutluay A. Naf inhibits matrix-bound cathepsin-mediated dentin matrix degradation. Caries Res. (2016) 50:124–32. doi: 10.1159/000444222
137. Sebold M, Giannini M, André CB, Sahadi BO, Maravic T, Josic U, et al. Bonding interface and dentin enzymatic activity of two universal adhesives applied following different etching approaches. Dent Mater. (2022) 38:907–23. doi: 10.1016/j.dental.2022.03.001
138. Reis A, Chibinski AC, Stanislawczuk R, Wambier DS, Grande RH, Loguercio AD. The role of dentin moisture in the degradation of resin-dentin interfaces under clinical and laboratory conditions. J Am Dent Assoc. (2012) 143:e29–36. doi: 10.14219/jada.archive.2012.0274
139. Pashley DH, Tay FR, Breschi L, Tjäderhane L, Carvalho RM, Carrilho M, et al. State of the art etch-and-rinse adhesives. Dent Mater. (2011) 27:1–16. doi: 10.1016/j.dental.2010.10.016
140. Breschi L, Maravic T, Cunha SR, Comba A, Cadenaro M, Tjäderhane L, et al. Dentin bonding systems: from dentin collagen structure to bond preservation and clinical applications. Dent Mater. (2018) 34:78–96. doi: 10.1016/j.dental.2017.11.005
141. Van Landuyt KL, Snauwaert J, De Munck J, Peumans M, Yoshida Y, Poitevin A, et al. Systematic review of the chemical composition of contemporary dental adhesives. Biomaterials. (2007) 28:3757–85. doi: 10.1016/j.biomaterials.2007.04.044
142. Irmak Ö, Baltacıoğlu İH, Ulusoy N, Bağış YH. Solvent type influences bond strength to air or blot-dried dentin. BMC Oral Health. (2016) 16:77. doi: 10.1186/s12903-016-0247-3
143. Lins R, Sebold M, Magno MB, Maia LC, Martins L, Giannini M. Does the type of solvent in dental adhesives influence the clinical performance of composite restorations placed in non-carious cervical lesions? A systematic review and meta-analysis. Oper Dent. (2020) 45:E237–54. doi: 10.2341/19-124-LIT
144. Pashley DH, Tay FR, Carvalho RM, Rueggeberg FA, Agee KA, Carrilho M, et al. From dry bonding to water-wet bonding to ethanol-wet bonding. A review of the interactions between dentin matrix and solvated resins using a macromodel of the hybrid layer. Am J Dent. (2007) 20:7–20. doi: 10.1016/j.dental.2007.02.009
145. Araújo CTP, Prieto LT, Abuna G, Rocha RL, Coppini EK, Oliveira DCRS, et al. Simplified ethanol wet-bonding technique: an alternative strategy for resin-dentin bonding in root canals. Gen Dent. (2019) 67:e1–e5. doi: 10.1016/j.dental.2018.03.010
146. Souza MY, Jurema ALB, Caneppele TMF, Bresciani E. Six-month performance of restorations produced with the ethanol-wet-bonding technique: a randomized trial. Braz Oral Res. (2019) 33:e052. doi: 10.1590/1807-3107bor-2019.vol33.0052
147. Breschi L, Mazzoni A, Ruggeri A, Cadenaro M, Di Lenarda R, De Stefano Dorigo E. Dental adhesion review: aging and stability of the bonded interface. Dent Mater. (2008) 24:90–101. doi: 10.1016/j.dental.2007.02.009
148. Stape THS, Tjäderhane L, Abuna G, Sinhoreti MAC, Martins LRM, Tezvergil-Mutluay A. Optimization of the etch-and-rinse technique: new perspectives to improve resin-dentin bonding and hybrid layer integrity by reducing residual water using dimethyl sulfoxide pretreatments. Dent Mater. (2018) 34:967–77. doi: 10.1016/j.dental.2018.03.010
149. Loguercio AD, Reis A. Application of a dental adhesive using the self-etch and etch-and-rinse approaches: an 18-month clinical evaluation. J Am Dent Assoc. (2008) 139:53–61. doi: 10.14219/jada.archive.2008.0021
150. Cardoso GC, Nakanishi L, Isolan CP, Jardim PDS, Moraes RR. Bond stability of universal adhesives applied to dentin using etch-and-rinse or self-etch strategies. Braz Dent J. (2019) 30:467–75. doi: 10.1590/0103-6440201902578
151. Soares AD, Ramos JC, Alves LC, Pereira JL, Caramelo F, Costa AL. Evaluation of the efficacy of a 2-step etch-and-rinse, 2-step self-etch and 1-step self-etch adhesive systems, in class II primary molars restorations. An one year prospective, randomized clinical trial. Eur Arch Paediatr Dent. (2022) 23:845–54. doi: 10.1007/s40368-022-00748-0
Keywords: dental materials, dentin adhesion, hybrid layer degradation, dental adhesive bonding formulations, bonding agents
Citation: Porto ICCM, Lôbo TLGF, Rodrigues RF, Lins RBE and da Silva MAB (2023) Insight into the development of versatile dentin bonding agents to increase the durability of the bonding interface. Front. Dent. Med 4: 1127368. doi: 10.3389/fdmed.2023.1127368
Received: 19 December 2022; Accepted: 23 January 2023;
Published: 16 February 2023.
Edited by:
Josette Camilleri, University of Birmingham, United KingdomReviewed by:
Vesna Miletic, The University of Sydney, Australia© 2023 Porto, Lôbo, Rodrigues, Lins and da Silva. This is an open-access article distributed under the terms of the Creative Commons Attribution License (CC BY). The use, distribution or reproduction in other forums is permitted, provided the original author(s) and the copyright owner(s) are credited and that the original publication in this journal is cited, in accordance with accepted academic practice. No use, distribution or reproduction is permitted which does not comply with these terms.
*Correspondence: Isabel Cristina Celerino de Moraes Porto, aXNhYmVsLnBvcnRvQGZvdWZhbC51ZmFsLmJy
Specialty Section: This article was submitted to Dental Materials, a section of the journal Frontiers in Dental Medicine