- 1Faculty of Dentistry, University of Toronto, Toronto, ON, Canada
- 2Stomatological Hospital of Chongqing, Key Laboratory of Oral Diseases and Biomaterial Sciences, Chongqing Municipal Key Laboratory of Oral Biomedical Engineering of Higher Education, Chongqing, China
- 3Department of Chemistry, University of Toronto, Toronto, ON, Canada
- 4Department of Materials Science and Engineering, University of Toronto, Toronto, ON, Canada
- 5Department of Laboratory Medicine and Pathobiology, University of Toronto, Toronto, ON, Canada
- 6Department of Dentistry, Mt. Sinai Hospital, Toronto, ON, Canada
Periodontitis is a multifactorial disease that involves the destruction of hard and soft tissues surrounding the tooth. Routine periodontal treatment includes mechanical debridement (surgical and non-surgical) and the systemic administration of antibiotics. In contrast, severe and chronic periodontitis involves aggressive tissue destruction and bone resorption, and the damage is usually irreversible. In these severe cases, bone grafts, the delivery of growth hormones, and guided tissue regeneration can all be used to stimulate periodontal regeneration. However, these approaches do not result in consistent and predictable treatment outcomes. As a result, advanced biomaterials have evolved as an adjunctive approach to improve clinical performance. These novel biomaterials are designed to either prolong the release of antibacterial agents or osteogenic molecules, or to act as immunomodulators to promote healing. The first half of this review briefly summarizes the key immune cells and their underlying cellular pathways implicated in periodontitis. Advanced biomaterials designed to promote periodontal regeneration will be highlighted in the second half. Finally, the limitations of the current experimental design and the challenges of translational science will be discussed.
1. Introduction
Periodontitis is a multifactorial inflammatory disease that affects nearly 20%–50% of the global population (1). Its clinical signs include the inflammation and destruction of the periodontium, the supporting structure of the tooth composed of gingiva, periodontal ligament, cementum, and alveolar bone (2). Disruption in the equilibrium of bacterial biofilms can result in microbial dysbiosis, which can lead to oral diseases. Periodontal inflammation is associated with an increase in the prevalence of specific bacterial species, which triggers the recruitment of inflammatory immune cells to eradicate the pathogenic bacterial population (3–5). However, bacteria are difficult to eliminate when they are found in a dental biofilm, as they are protected by a 3D matrix of extracellular polymeric substances, which restricts the penetration of antibiotics and immune cells (6). As a result, the continuous interaction between microbial dysbiosis and the host immune response prolongs the inflammatory response. Moreover, immune cells and their associated pro-inflammatory cytokines not only target the bacteria but also activate the receptor activator of the NF-kB ligand (RANKL) pathway, which is responsible for osteoclastogenesis (the breakdown of bone tissue) (7) (Figure 1). Thus, both the host inflammatory response and bacterial collagenases contribute to tissue destruction and alveolar bone resorption (8, 9).
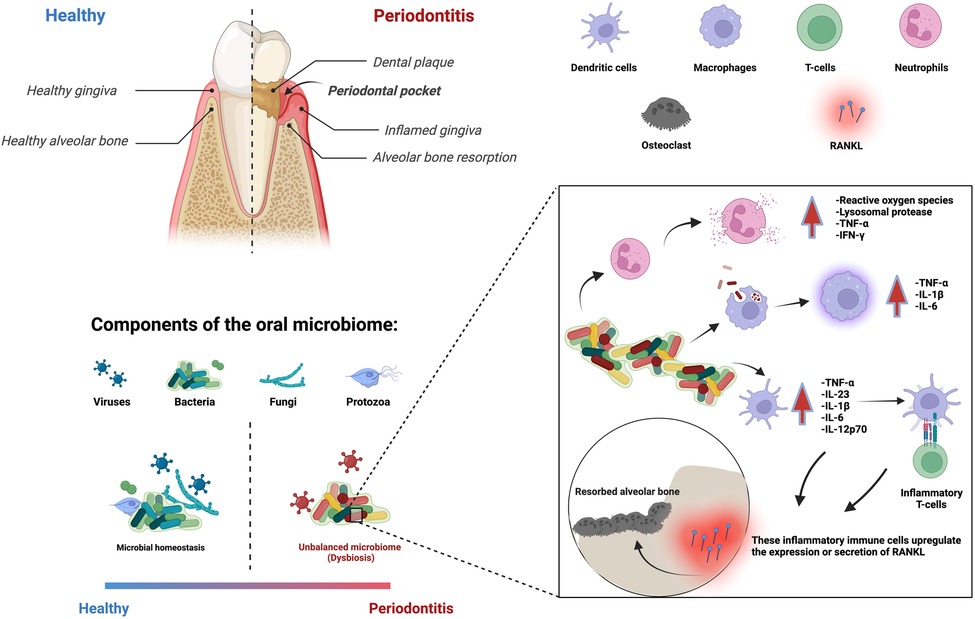
Figure 1. Periodontitis is an immune-inflammatory disease in response to bacterial biofilms that results in the loss of cementum, periodontal ligament, and bone resorption. Multiple immune cells are involved in this process.
Furthermore, the effect of periodontitis extends beyond the oral cavity; numerous studies have associated periodontitis with systemic diseases, such as diabetes mellitus (10), cardiovascular disease (11), respiratory disease (12), and Alzheimer's disease (13). For instance, diabetes mellitus is considered a major risk factor for periodontitis, with an increased susceptibility of approximately threefold (14). The prevalence of periodontitis in diabetic patients was found to be close to 90% in a recent cross-sectional study (15). Although the exact mechanism between periodontitis and diabetes remains unclear, immune cells are known to be affected, resulting in worsened disease progression and delayed healing (16). Hyperglycemia, a hallmark of diabetes, is known to affect immune cells, resulting in chronic systemic inflammation (17, 18). Subsequently, this inflammatory state can negatively affect pancreatic cells and further reduce insulin production, which in turn negatively affects glycemic control, making it a vicious cycle (19). Moreover, activated inflammatory immune cells also contribute to inflammation-induced alveolar bone loss (20), leading to aggravated bone resorption in diabetic patients (10). Some studies have suggested that non-surgical interventions, such as scaling and root planing, can improve the clinical outcomes of diabetic periodontitis. A single-masked randomized clinical trial based on 90 patients has shown that scaling and root planing significantly improved periodontal parameters (pocket depth, gingival index, and plaque index) and metabolic parameters (serum glycated hemoglobin) compared to the group that did not receive scaling and root planing (21). These results are in good agreement with other clinical studies (22, 23). In addition, these data demonstrate that the counts of periodontal pathogenic bacteria correlate poorly with the clinical progression of diabetic patients, indicating that there are other factors at play other than bacterial-induced inflammation. Alternatively, insulin has been the gold standard to treat diabetes mellitus. In a recent study, it was suggested that insulin not only controlled blood glucose concentration, but it also suppressed inflammatory cytokines and ameliorated periodontitis without local periodontitis treatment in diabetic rats (24). These results further corroborate that the association between diabetes mellitus and periodontitis is indeed a two-way relationship.
Current periodontitis treatments range from non-surgical debridement (e.g., tooth scaling and root planing) to surgical debridement (flap procedure), combined with local or systemic antibiotic delivery to further inhibit bacterial activities (25). An alternate method to eliminate periodontal bacteria is known as photodynamic therapy (PDT). It is a non-invasive treatment that involves the use of a photosensitizer, which can be activated by a low-energy laser light to generate singlet oxygen and reactive oxygen species to eliminate microorganisms (26). Although in vitro antibacterial studies demonstrated PDT to be an effective approach against periodontal pathogens, such as Porphyromonas gingivalis (P. gingivalis) and Aggregatibacter actinomycetemcomitans (A. actinomycetemcomitans) (27, 28), systematic reviews of the utilization of PDT in clinical studies concluded that the efficacy of PDT as an adjunctive treatment is limited by the inadequate number of studies and the heterogeneity of study designs (29, 30). In most cases, scaling and root planing have been shown to be effective in attenuating periodontitis (31, 32). However, treatment outcomes vary based on factors such as the severity of periodontitis at baseline, the type of tooth affected, behaviors such as smoking and compliance, and other risk factors, such as diabetes mellitus, which leads to dysregulation of the immune system (33–36). However, while these treatments attenuate inflammation and preserve tissue loss, they do not actively promote the regeneration of periodontium.
In severe cases where there is substantial connective tissue and alveolar bone loss, surgical treatments such as bone grafts, delivery of growth hormones, and guided tissue/bone regeneration are recommended. These methods have shown some promise in promoting the regrowth of three-walled defects and narrow dehiscence defects (37). However, they are relatively ineffective at regenerating horizontal patterns of alveolar bone loss (38, 39). More recently, instead of delivering growth hormones, autologous platelet concentrates have been used. They are platelets isolated from patients’ own blood that are rich in growth factors and cytokines, such as platelet-derived growth factor (PDGF), transforming growth factor beta (TGFβ), vascular endothelial growth factor (VEGF), epithelial growth factor (EGF), insulin-like growth factor-1 (IGF-1), and basic fibroblast growth factor (bFGF), together with proteins such as fibrin, fibronectin, and vitronectin (40). These mediators regulate cell proliferation, migration, and differentiation, which are crucial in tissue regeneration and healing. Platelet-rich fibrin (PRF) is a well-studied second-generation derivative; its fibrin network functions as a three-dimensional scaffold that allows cell migration and the sustained release of growth factors. In one study, PRF was used as an adjunctive treatment combined with open flap debridement in a canine periodontitis model. The addition of PRF reduced the inflammation and improved soft tissue healing, but it did not affect alveolar bone gain (41). Another systemic review also reported that there is insufficient evidence that the addition of PRF significantly improved periodontal parameters such as probing pocket depth, clinical attachment level, and bone defect filling (40).
Given the intrinsic complexity of periodontitis and the inconsistent regenerative capability of current treatments, alternative or adjunctive treatments are constantly being explored. Regenerative strategies, such as the utilization of advanced biomaterials to promote periodontal regeneration, have emerged as viable alternatives. This review paper will first focus on the key immune cells involved in periodontitis, followed by the discussion of some of the latest biomaterial-based developments in treatment strategies to promote periodontal regeneration. The limitations of the current experimental designs, as well as the roadblocks to clinical translatability, are also discussed to provide some insight into the future development of innovative biomaterial-based periodontal therapies.
2. Immune cells and cellular pathways involved in periodontitis
Immune cells play an important role in alveolar bone homeostasis in chronic periodontitis (42). Neutrophils, mast cells, macrophages, dendritic cells (DCs), T-cells, and B-cells are among the innate and adaptive immune cells involved in this process. Under chronic microbial dysbiosis, the prolonged inflammatory states of these immune cells lead to periodontal disease progression and delayed healing (43).
2.1. Neutrophils
Polymorphonuclear leukocytes, also known as neutrophils, are one of the first responders to infections and play an important role in the innate immune system. They eliminate bacteria through a variety of key mechanisms, including phagocytosis (the ingestion and elimination of microbes), degranulation (the extracellular release of granules such as antimicrobial peptides), the production of reactive oxygen species (cytotoxic to both microbes and host cells), and neutrophil extracellular traps (trapping and eliminating pathogens while minimizing damage to host cells) (44).
Neutrophil homeostasis is critical for maintaining periodontal health; the absence or excess of neutrophils has been linked to aggravated periodontal inflammation (45, 46). Even in the absence of inflammation, neutrophils constantly infiltrate the periodontium in small quantities. These infiltrated neutrophils are also referred to as oral neutrophils, are frequently found in saliva and gingival crevicular fluid. Their number and activity are affected by periodontitis. For example, the presence of pathogenic dental plaque-derived chemoattractants and pro-inflammatory cytokines attracts more circulating neutrophils into the gingival tissues (47). A clinical study found an association between neutrophil cell counts and the severity of periodontitis (48). Patients with chronic periodontitis showed a 2.5-fold higher recruitment of neutrophils to their oral cavity when compared to healthy participants (49). Oral neutrophils are also functionally altered in periodontitis (50), characterized by dysfunctional chemotaxis with reduced speed, velocity, and chemotactic accuracy. In addition, neutrophils displayed increased levels of activation and degranulation markers such as CD10, CD63, CD64, and CD66a (47), as well as an altered transcriptome with a tendency to favor pro-survival genes, resulting in longer-lived neutrophils in periodontitis (51). The abundance and longevity of these neutrophils may impact the severity and length of inflammation-induced periodontal tissue destruction. These results indicate that neutrophils are chemotactically compromised, hyperactivated, and present in higher quantities in the presence of periodontitis.
Aside from their ability to fight microbial infections, neutrophils also exhibit plasticity. They can suppress the immune response via the suppression of T-cell activation (52). In one study, periodontal treatment reduced the number of suppressive neutrophils while increasing the number of normal neutrophils (53), implying that suppressive neutrophils may play a role in the etiology of periodontitis. However, due to the limited publications on the role of suppressive neutrophils in periodontitis, their exact contribution requires further investigation.
2.2. Mast cells
Mast cells are yet another important component of the innate immune response. They can be activated by several mechanisms, such as complement proteins, damage-associated molecular patterns (DAMPs), and pathogen-associated molecular patterns (PAMPs). Mast cells degranulate upon activation to induce inflammation in the local microenvironment. They secrete a variety of mediating cytokines, growth factors, and granules, such as tumor necrosis factor alpha (TNF-α), interleukin 1-beta (IL-1β), histamine, and monocyte chemoattractant protein (MCP-1), which all aid in the recruitment of other inflammatory cells and fibrocyte migration (54, 55).
The depletion of mast cells significantly reduced the production of inflammatory cytokines and periodontitis-induced alveolar bone loss in a rat experimental periodontitis model (56). A similar trend was reported in human gingival tissues (57), where the density of mast cells in periodontitis patients was 1.53-fold higher than in healthy patients. Another study compared healthy gingival tissues to groups with moderate and advanced periodontitis (58). The findings revealed an association between mast cell density, extent of degranulation, and severity of periodontitis. Furthermore, the expression of Toll-like receptor 4 (TLR4) on mast cells has been demonstrated to be positively correlated with the severity of chronic periodontitis (59). TLR4 is a sensing receptor for gram-negative bacteria that, when activated, induces a pro-inflammatory response (60).
2.3. Macrophages
Macrophages serve a vital role in both acute and chronic inflammation. Depending on their polarization, they can be either pro-inflammatory (M1) or anti-inflammatory (M2) (61). It is widely accepted that macrophages can adopt a continuum of phenotypes between these two distinct polarizations. Such intermediate macrophages could exhibit both M1 and M2 characteristics to different extents (62).
Resident oral mucosal macrophages adopt various phenotypes in the defense against periodontal pathogens. They also orchestrate the recruitment of monocytes (63, 64), which can differentiate into macrophages and dendritic cells. Environmental cues, such as the presence of periodontal pathogens, can influence macrophage polarization and PAMPs from bacteria can stimulate M1 macrophage differentiation, which establishes a pro-inflammatory environment and secretes pro-inflammatory cytokines such as IL-1β, IL-6, IL-23, and TNF-α (65, 66). In contrast, immunomodulatory cytokines such as IL-4, IL-13, and IL-10 are necessary to polarize macrophages toward the M2 phenotype (67, 68). Because M1 macrophages are associated with diseased tissues and M2 macrophages with healthy gingival tissues (69), an elevated M1/M2 ratio observed in periodontitis signifies an imbalance between inflammation and healing. Restoring this balance has been shown to be a potential treatment strategy for periodontitis. Recent research using bioactive molecules capable of inhibiting M1 differentiation effectively attenuated periodontal bone resorption in a murine periodontitis model (70). Similarly, another study found that injecting M2 macrophages into local periodontal tissue significantly alleviated the inflammation of periodontitis in mice (71).
Despite the findings that have associated M2 macrophages with the healing phase, many studies have reported contradictory results. In one study, it was observed that gingivitis tissues had the highest total number of macrophages as well as the highest M1 and M2 polarization than periodontitis and healthy samples (72); there was also no difference in the relative amounts of M1 and M2 macrophages between periodontitis and healthy tissues. Some of these discrepancies could be attributed to patient variability, differences in the analytical methods used to characterize and numerate the macrophage population, the timing of determining macrophage polarization, and the selection of surface markers, as some intermediate macrophages may exhibit characteristics of both M1 and M2.
2.4. Dendritic cells
Dendritic cells (DCs) are professional antigen-presenting cells that bridge the innate and adaptive immune responses in chronic periodontal disease (73, 74). They can elicit either immunogenic or tolerogenic immune responses depending on their activation state (75, 76). Immunogenic DCs activate inflammatory T-cells such as CD8+ T-cells (cytotoxic T-cells) and CD4+ T-cells (helper T-cells). Tolerogenic DCs, in contrast, have a limited capability to activate CD8+ T-cells and CD4+ T-cells. Rather, they stimulate regulatory T-cells (77), a subgroup of T-cells that suppress the immune system to maintain tolerance to self-antigens and prevent autoimmune disorders. In the case of periodontitis, inflammatory DCs can differentiate T-cells into a pro-inflammatory state. These activated CD4+ and CD8+ T-cells increase the expression and secretion of RANKL (78–80), which may facilitate osteoclastogenesis and contribute to inflammation-induced alveolar bone resorption.
Like macrophages, DCs can also recognize PAMPs presented by periodontal pathogens. Unstimulated DCs derived from the peripheral blood monocytes of patients with chronic periodontitis exhibited an immature phenotype, but their cytokine profiles indicated a proinflammatory response (81). This finding corroborates previous studies that have correlated chronic periodontitis with elevated systemic inflammation (82, 83). In another study, gingival samples from patients with chronic periodontitis showed higher levels of pro-inflammatory cytokines, such as IL-2, TNF-α, interferon gamma (IFN-γ), and IL-17A, when compared to healthy gingival tissues (84). Although the anti-inflammatory cytokine IL-10 was also elevated in the chronic periodontitis group, it did not alleviate periodontal inflammation. Moreover, clinical periodontal parameters (clinical attachment level >3) were correlated with a higher density of inflammatory DCs and negatively correlated with the density of immature DCs. Like other immune cells, inflammatory DCs can also be targeted to treat periodontitis. In one study, systemically administered antibiotics metronidazole and amoxicillin were given to patients with periodontitis; the treatment effectively reduced inflammatory DCs and improved the clinical measures of periodontitis (85). Aside from immunogenic DCs, tolerogenic DCs also serve a crucial role in immune regulation. They have been extensively investigated for their potential to maintain immunologic tolerance in clinical transplantation, autoimmunity, and inflammatory diseases (86–88). However, they have been underexplored as a possible therapeutic target for treating periodontitis.
2.5. T-cells
Oral mucosa constitutes resident T-cells, primarily memory CD4+ and CD8+ T-cells (89). Naïve T-cells from the lymph nodes can also be activated by antigen-presenting cells, such as DCs. When DCs encounter periodontal pathogens, they migrate to the lymph nodes, where naïve T-cells can be differentiated into various effector T-cells (74). The exact differentiation pathway depends on the type of DCs and the presence of immunomodulatory cytokines.
In periodontitis, two T-cell subsets have received much attention: regulatory T-cells and TH17 cells. Regulatory T-cells promote bone regeneration by downregulating the levels of TNF-α and IFN-γ. Moreover, they also secrete IL-4, a chemoattractant for osteoblasts (90). The adoptive transfer of CD8+ regulatory T-cells reduced alveolar bone destruction and osteoclast formation in experimental periodontitis (91, 92). In contrast, TH17 cells are associated with periodontitis (93). A murine experimental periodontitis model demonstrated that TH17 cells and the accumulation of neutrophils were necessary for inflammatory tissue destruction (94). In the same study, a patient population with a genetic defect in TH17 cell differentiation was associated with reduced periodontal inflammation and bone loss, despite an increased prevalence of recurrent oral fungal infections. These results emphasize the significance of TH17 cells in periodontitis.
The ratio of TH17 to regulatory T-cells has been linked to the health of periodontal tissues. Age was found to influence this ratio in blood samples from patients with chronic periodontitis (95). When compared to older patients, the TH17/regulatory T-cells ratio was much higher in younger individuals. Furthermore, DCs were also overactivated (upregulation of the CD40 marker) in younger patients. Cell culture experiments demonstrated that DCs with a high expression of CD40 elevated the Th17/regulatory T-cells ratio. In addition, hyperactivated DCs secreted cytokines that inhibited the induction of regulatory T-cells (96). Although these cells were not isolated from the gingival tissues, other studies have linked periodontitis-induced local inflammation to systemic inflammation (82, 97).
Similar to the M1/M2 ratio, the TH17/regulatory T-cells ratio can also be altered to attenuate periodontitis. An experimental periodontitis model established in rats revealed that the amount of CD8+ regulatory T-cells from the gingival tissues did not differ significantly from that of healthy animals (92). However, the population of TH17 cells was significantly elevated in the periodontitis group, while the amount of CD4+ regulatory T-cells decreased significantly when compared to healthy animals. Adoptive transfer of CD8+ regulatory T-cells reduced alveolar bone resorption and osteoclast formation. These findings suggest that periodontitis alters the proportion of regulatory T-cells and TH17 cells. Restoring this ratio to a healthy range by inducing new regulatory T-cells can be an effective approach for promoting periodontal regeneration. It is crucial to point out that in the presence of periodontitis, regulatory T-cells could become functionally unstable and lose their anti-osteoclastogenic properties. In a murine experimental periodontitis model, ex vivo suppression capacity on osteoclastic differentiation was dramatically reduced in regulatory T-cells obtained from periodontally diseased animals compared to healthy controls (98). Like macrophages, T-cells can undergo phenotypic transition. In periodontitis, regulatory T-cells have been shown to be converted to TH17 cells (99, 100). Thus, it may be necessary to induce new populations of regulatory T-cells directly from naïve T-cells or to introduce regulatory T-cells by adoptive transfer. Alternatively, the conversion of regulatory T-cells to TH17 could be modulated.
2.6. B-cells/plasma cells
B-cells are another set of APCs that are activated by helper T-cells after encountering a foreign antigen. Memory B-cells or plasma cells are two specialized forms. Plasma cells provide immediate protection via the secretion of specific antibodies, whereas memory B-cells generate a faster and enhanced response upon subsequent encounters with the same antigen (101).
Like other immune cells, B-cells have important implications in periodontitis as well (102). Most of the B-cells found in healthy gingiva and gingivitis tissues were memory B-cells. In contrast, the major B-cell population in periodontitis was plasma cells, which were more prominent at the base of the periodontal pocket and scattered throughout the gingiva. Plasma cells residing in periodontitis tissue secrete IgG antibodies that are specific to periodontal pathogens, such as P. gingivalis and A. actinomycetemcomitans. In addition, these plasma cells also express RANKL, which is involved in osteoclastogenesis. Following scaling and root planing, the B-cell population in periodontitis tissues shifted from primarily plasma cells to memory B-cells. This result implies that the plaque biofilm was responsible for the differentiation of B-cells into plasma cells in periodontitis tissues. Circulating B-cells are also altered in periodontitis patients, as these cells have higher levels of RANKL expression (103).
Plasma cells are also heterogeneous. IgG plasma cells can differentiate into IL-37 producing plasma cells and IL-35 and IL-37 co-producing plasma cells (104). IL-35 and IL-37 are both anti-inflammatory cytokines that have been demonstrated to inhibit osteoclast formation in vitro in a dose-dependent manner. Thus, it is reasonable to hypothesize that these two subsets of plasma cells could attenuate periodontitis-induced alveolar bone resorption via the inhibition of osteoclast formation. These findings suggest that B-cells are essential to maintain alveolar bone homeostasis.
In summary, periodontitis is a multifactorial disease caused by interactions between bacteria and their by-products, immunomodulatory cytokines, and different immune cell populations (Figure 2). Furthermore, immune cells also communicate with one another via cytokines or cell–cell interaction (105). Elevated levels of neutrophils, plasma cells, and naïve B-cells were found in periodontitis-affected tissues. In terms of T-cells, periodontitis-affected tissues had a larger proportion of activated CD4 memory T-cells, whereas healthy tissues had a lower number of regulatory T-cells.
3. Biomaterials for modulating periodontal bone regeneration
Biomaterial scaffolds are three-dimensional constructs designed to support the proliferation and differentiation of cells for the regeneration of defective tissues (106). The optimal scaffold would be the extracellular matrix (ECM) of the native tissue. However, it is difficult to recreate the exact native ECM due to its intricacy and composition, as there are many types of ECM found in various human tissues. A biomaterial scaffold should serve several key functions similar to those of native ECM. First, the biomaterial scaffold should exhibit a highly interconnected porous structure to enable the transportation of nutrients and metabolites, hence facilitating the attachment or passage of cells and supporting their cellular activities. A porous structure also provides the necessary volume for vascularization and the development of new tissues. Second, the biomaterial scaffold should also be biocompatible and biodegradable. Biocompatibility refers to the absence of any serious adverse effects elicited by the biomaterial scaffolds. Biodegradability is another crucial factor since non-biodegradable biomaterials could face the challenge of foreign body reaction leading to the formation of a fibrous capsule, which may compromise the stability and functionality of implanted biomaterial scaffolds (107). Another advantage of degradable biomaterials is that they allow the newly formed tissues to eventually replace the degraded biomaterial scaffolds. Third, the scaffolds should possess similar mechanical properties to those of the native tissues in order to provide the proper mechanical cues to local cells. Previous studies have shown that scaffold surface morphology and stiffness can affect how cells differentiate (108, 109). Lastly, scaffolds may serve as a delivery vehicle for the sustained release of bioactive molecules, such as growth factors or therapeutic agents, to promote regeneration (110).
There are many ways to characterize biomaterials, one method is to classify them based on their structural and compositional complexity, mainly monophasic versus multiphasic scaffolds. Monophasic scaffolds have been extensively studied as potential delivery vehicles for biomedical applications. Although they are easy to fabricate and their interconnected porous structure promotes cell adhesion, they do not possess layers with distinct properties. In contrast, multiphasic scaffolds offer the advantage of having different layers with different characteristics such as material composition, architecture, and functionalization that allow them to target different tissues at once. For example, a triphasic collagen scaffold was developed by combining collagen self-assembly and diffusion gradients in mineralization (111). The resulting tri-layered scaffold resembled the architecture of the periodontium, with successive layers that mimicked the bone, periodontal ligament, and cementum, respectively. Each individual compartment can provide the necessary mechanical cues to help facilitate the regeneration of the corresponding tissue. Another study constructed a multiphasic scaffold composed of three different layers, each with its own specific microstructure and delivering a distinct bioactive cue that targets the regeneration of the cementum, periodontal ligament, and alveolar bone, respectively (112). Implantation of such a multiphasic scaffold into the dorsum of immunodeficient mice for 6 weeks resulted in the formation of a periodontium-like multi-tissue matrix. However, the main drawback of multiphasic scaffolds is that, in order to enable precise control of the microarchitecture of each layer, the scaffolds need to be prefabricated and then surgically implanted. This complicates the fabrication process, as different compartments need to be constructed differently. In addition, the geometry of the scaffold needs to be personalized based on the defect size.
Most often, for regenerative applications in the oral cavity, injectable biomaterials are preferred over their implantable counterparts. Injectable biomaterials are viscous formulations that are administered in a flowable state, either liquid or gel-like. This type of biomaterial includes but is not limited to hydrogels (113–115), cryogels (116, 117), supramolecular hydrogels (118), microspheres (119–121), cement (122–126), and so on. Their most distinctive advantage is that they can be readily administered through a needle, a simple and much less invasive procedure that minimizes collateral damage surrounding the injury site. Another advantage of injectable biomaterials arises from their viscoelastic property. Upon injection, the fluidic biomaterials can fill the irregularly shaped pockets associated with periodontal complications. However, to ensure injectability, the choice and design are often limited to biomaterials that are cross-linked physically via weak attractions, such as hydrogen bonding, electrostatic interactions, pie-pie interactions, van der Waals forces, and so on. Utilizing a dual-syringe system is one approach to circumvent this limitation; separation of the polymeric materials and cross-linkers maintains their respective stability; and, upon injection, the two components immediately mix and cross-link, resulting in a hydrogel with significantly stronger mechanical properties (127). Photopolymerizable biomaterials constitute an additional viable solution (128).
3.1. Biomaterials as delivery vehicles
The use of antibiotics is a common treatment option for chronic and aggressive periodontitis. Systemic administration of antibiotics has been proven to be effective at attenuating periodontitis by eradicating periodontal pathogens from deep periodontal pockets (129). However, systemic administration has several drawbacks. First, it is highly non-specific; administered antibiotics will circulate throughout the body, increasing the likelihood of adverse events occurring elsewhere. Second, overuse of antibiotics leads to bacterial resistance, which is becoming a global health crisis. Lastly, it is challenging to continuously maintain a high local concentration of antibiotics over time when they are administered systemically.
After systemic administration of metronidazole in rabbits with experimental periodontitis (130), the suspension was quickly detected in the blood after 6 h and the minimum inhibitory concentration was maintained for 24 h around the periodontal pocket. As opposed to systemic dosing, the sustained local release of antibiotics would be desirable. Biomaterials in the form of hydrogels, cements, micro-/nanoparticles, and microspheres are commonly utilized to deliver a variety of bioactive molecules and release them sustainably over an extended period of time (131) (Table 1). To enhance metronidazole distribution, a solution–gel-based inverse lyotropic liquid crystalline (LLC) system was developed. It gelled quickly, and its crystalline nanostructures improved adhesion to the periodontal pocket. Furthermore, the LLC sustainably released metronidazole, and its concentration was maintained above the minimum inhibition concentration for over 10 days without detectable concentration in the blood. Metronidazole was also delivered locally using an injectable adhesive chitosan and polyvinyl alcohol hydrogel in a comparable study (132). The hydrogel sustainably released the encapsulated metronidazole. In addition, it displayed excellent adhesion and prevented water seepage in a model tooth for 30 min. This feature can be exploited to seal the periodontal pocket and protect the periodontal tissues from further infection. In an in vivo rat periodontitis model, the mean probing depth of the periodontal pocket was significantly reduced in the hydrogel-treated group.
In addition to antibiotics, other therapeutic agents, such as statins and natural substances, have also been investigated for their efficacy to ameliorate local inflammation. Simvastatin is a statin commonly used to treat hyperlipidemia by decreasing elevated lipid levels. It also exhibits antioxidant properties, which may help reduce inflammation and support bone growth (139). However, its poor water solubility restricted its use in the oral cavity to treat periodontitis. To address this limitation, an injectable hydrogel was synthesized to load simvastatin and release it locally into the periodontal pocket (140). The loaded hydrogel resolved inflammation and preserved periodontal bone in a ligature-induced periodontitis rat model. Naringin is a flavonoid compound naturally found in citrus fruits, such as oranges, grapefruits, and bergamots, that has antioxidant and anti-inflammatory properties (141). Like simvastatin, naringin also has poor water solubility. One study synthesized an injectable thermosensitive hydrogel from amphipathic carboxymethyl-hexanoyl chitosan, β-glycerol phosphate, and glycerol to improve the oral delivery of naringin (133). The resultant hydrogel selectively released naringin between pH 5.5 and 6.5; it also significantly reduced alveolar bone loss and inflammatory infiltration relative to the untreated periodontitis group in a murine periodontitis model.
However, the main limitation of these studies is that they only targeted microbial dysbiosis-induced inflammation. As a result, this only reduced the extent of bone resorption but did not actively promote periodontium regrowth.
One approach to promoting tissue regeneration is by delivering regenerative molecules to directly facilitate site-specific regeneration. Injectable formulations of macroporous calcium phosphate cement loaded with bone morphogenetic protein-2 (BMP-2) or fibroblast growth factor-2 (FGF-2) showed periodontal regenerative potential. In a rat periodontitis model, the cement/FGF-2 group resulted in superior periodontal ligament and bone healing compared to the free cement and cement/BMP-2 groups (134). Injectable paste-like poly(lactic-co-glycolic acid) (PLGA) carriers have also been used to encapsulate recombinant human growth/differentiation factor-5 (rhGDF-5), a growth factor known to induce cartilage and bone formation (135). Following injection into the periodontal pockets in a canine periodontitis model, PLGA/rhGDF-5 was largely resorbed by the end of the second week. By week 4, there was a significant increase in the regeneration of cementum, periodontal ligament, and alveolar bone compared to the sham surgery control.
Multiple bioactive molecules can be delivered simultaneously to achieve a synergistic effect to promote alveolar bone regeneration. A supramolecular hydrogel was utilized to improve the stability of stromal cell derived factor-1 (SDF-1) and BMP-2 (118). The delivery of the hydrogel loaded with these two bioactive molecules synergistically improved the bone regeneration outcome in a maxillary critical-sized periodontal bone defect rat model. Some studies have also focused on combination therapy to co-deliver both antibacterial agents and regenerative molecules to achieve a dual outcome. An injectable and self-healing hydrogel was constructed via a dynamically cross-linked network of dynamic Schiff base bonds and dynamic coordination bonds. The hydrogel was loaded with ginsenoside Rg1, a class of steroid glycosides that exhibits antioxidant properties, which also increases the proliferation activity and alkaline phosphatase activity of periodontal ligament cells (136), as well as amelogenin, a protein that promotes periodontal regeneration. The fully loaded hydrogel improved the quality of regenerated alveolar bone in terms of bone mineral density, bone volume/tissue volume, and trabecular thickness (Figure 3) (136). Similarly, a thermosensitive hydrogel loaded with aspirin (anti-inflammatory effect) and erythropoietin (promoting angiogenesis and bone regeneration) (137) suppressed inflammation and stimulated alveolar bone growth in a rat periodontitis model.
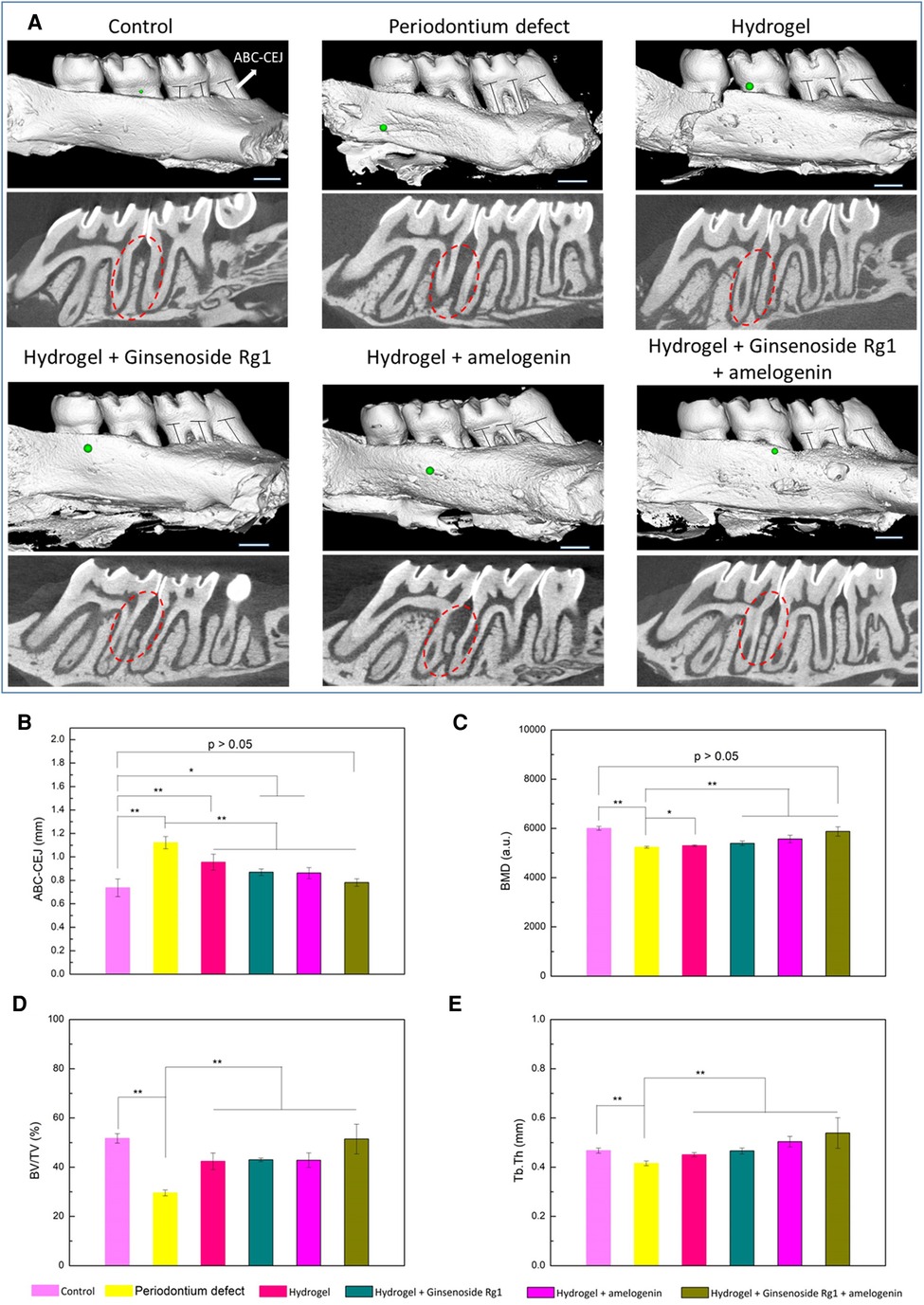
Figure 3. Evaluation of alveolar bone regeneration by micro-CT. (A) Three-dimensional and sectioned micro-CT images. Quantitative analysis of (B) the distance between ABC and CEJ, (C) BMD, (D) BV/TV, and (E) Tb.Th measured by micro-CT images. *p < 0.05, **p < 0.01. Scale bar is 1 mm. Reprinted with permission from (136). Copyright (2021) American Chemical Society.
So far, it has been the case that most of the discussed injectable biomaterials release their encapsulated bioactive molecules by passive diffusion. While sustained release is achieved, the release kinetics are largely independent of environmental cues. Thus, it would be ideal to design a smart delivery system that only releases the encapsulated therapeutic agents in response to environmental cues to prolong the therapeutic window and reduce unnecessary release. In one study (138), a smart on-demand delivery system was engineered to only release the antimicrobial peptide in response to gingipain produced by P. gingivalis. The smart hydrogel was also loaded with SDF-1 to induce tissue regeneration. In vitro results demonstrated that the smart hydrogel had a sensitive response to gingipain stimulation and released the antimicrobial peptide in a controlled manner, which strongly inhibited the growth of P. gingivalis. In addition, the smart hydrogel effectively controlled inflammation, recruited stem cells, and facilitated bone regeneration in a rat periodontitis model.
These studies on biomaterials showed promising results. However, inconsistencies have been reported between different in vitro and in vivo studies. Because conventional regenerative strategies mainly promote osteoblastic cell differentiation, the treatment outcome depends on the elimination of pathogenic microorganisms, the stimulation of bone cells, and the host immune response.
3.2. Biomaterials for immunomodulation
Recently, it has been recognized that immune cells play a critical role in bone homeostasis, and there are complex interactions between the immune system and the skeletal system. This emerging field is known as osteoimmunology (142). Instead of focusing solely on osteogenic cells, this new approach has fostered advancement in developing biomaterials that target immune cells to indirectly promote bone regeneration, termed osteoimmunomodulatory biomaterials.
Compared to conventional biomaterial delivery systems, osteoimmunomodulatory biomaterials offer several advantages. First, immunomodulation of the local immune response could establish an anti-inflammatory microenvironment that is favorable to bone regeneration. Growth factors such as BMP-2 and platelet-derived growth factor-BB (PDGF-BB) have shown regenerative potential (143, 144). However, pro-inflammatory signals can hinder their regenerative activity, and an anti-inflammatory microenvironment could enhance their efficacy to induce superior regeneration (145). In addition, an immune system-initiated response is much more robust, as the modulated immune cells can secrete numerous immunomodulatory cytokines that can initiate a cascade of downstream cellular processes. It can be highly beneficial for immunocompromised patients when such biomaterials can provide a targeted approach. For instance, in diabetic patients with periodontitis, the pro-inflammatory microenvironment and the lack of regulatory immune cells following periodontal treatment can impair healing and bone regeneration. Therefore, apart from antimicrobial treatments, targeted site-specific immunomodulation can aid in diminishing the contribution of systemic inflammation associated with diabetes to the oral cavity.
Immunomodulatory molecules have been investigated to treat periodontitis. Pro-resolving lipid mediators are endogenous cell-signaling molecules released during inflammation to promote resolution of inflammation, aimed at restoring tissue integrity and function (146). They have shown to regulate many immune cells, such as neutrophils (147), macrophages (148), dendritic cells (149), T-cells (150), and memory b-cells (151). Lipoxin A4 is one of these mediators and its analog is associated with dampened neutrophils-mediated tissue degradation and bone loss in periodontitis (152). In one study, doxycycline and lipoxin A4 were loaded into a thermosensitive polyisocyanopeptide hydrogel (153). The hydrogel treatment significantly improved gingival clinical attachment than mechanical debridement alone in a canine periodontitis model. However, the immunological aspect was not investigated in this study.
More specifically, many published studies have mainly focused on the regenerative potential of M2 macrophages and regulatory T-cells (Table 2). They have been shown to create an anti-inflammatory environment that is favorable to bone regrowth.
Various immunomodulatory cytokines have been encapsulated to induce M2 macrophages in situ. The C-C motif chemokine ligand 2 (CCL2), also known as MCP-1, was locally delivered by controlled-release microparticles to induce M2 macrophages (154). A decrease in the M1/M2 phenotype ratio and an anti-inflammatory microenvironment were established, which prevented alveolar bone loss in a murine periodontitis model. Similarly, liposomal systems loaded with resveratrol polarized macrophages from the M1 to the M2 phenotype, resulting in decreased levels of pro-inflammatory cytokines, such as IL-1β, IL-6, and TNF-α. In an in vivo murine periodontitis model, resveratrol-loaded liposomes reduced inflammation in the gingival tissues and ameliorated alveolar bone resorption, accompanied by an increased expression of M2 markers and a decreased expression of M1 markers (155).
Another commonly targeted immune cell population is the regulatory T-cells. PLGA microparticles were used to load and release a known chemoattractant for regulatory T-cells, CCL22 (156). In a murine periodontitis model, these PLGA microparticles containing CCL22 lowered pro-inflammatory cytokines and substantially reduced alveolar bone resorption. Furthermore, this formulation also reduced clinical measures of inflammation and lessened alveolar bone loss under severe inflammatory conditions in beagle dogs.
However, regulatory T-cells may become unstable and lose their anti-osteoclastogenic properties under prolonged periodontal inflammation (98). Therefore, the additional recruitment of existing regulatory T-cells to the oral cavity may not be an effective approach, as the systemic inflammation associated with periodontitis may have already compromised existing regulatory T-cells. Alternatively, regulatory T-cells can be induced from naïve T-cells in situ. In one study (157), a multibiologic delivery vehicle was fabricated by integrating nanofibrous spongy microspheres with functionalized mesoporous silica nanoparticles to release IL-2, TGFβ, and miR-10a to locally recruit T-cells and induce their differentiation into regulatory T-cells. In a murine periodontitis model, this injectable formulation successfully enriched and expanded regulatory T-cells locally, which mediated against periodontal bone loss (Figure 4). In a similar study, multiple bioactive molecules, including TGFβ, rapamycin, and IL2 were loaded into microspheres to induce regulatory T-cells locally (158). In a ligature-induced murine periodontitis model, the microsphere formulation decreased alveolar bone loss by increasing the local ratio of regulatory T-cells to effector T-cells. In a separate study (159), an injectable hydrogel was formulated to deliver the prolyl hydroxylase inhibitor, 1,4-dihydrophenonthrolin-4-one-3-carboxylic acid to promote regeneration of alveolar bone in a murine periodontitis model. Injection of the hydrogel induced an increase in bone regeneration, accompanied by an elevated expression of osteogenic genes, decreased expression of pro-inflammatory cytokine genes, and an increase in the number of FOXP3+ regulatory T-cells in the periodontal tissue.
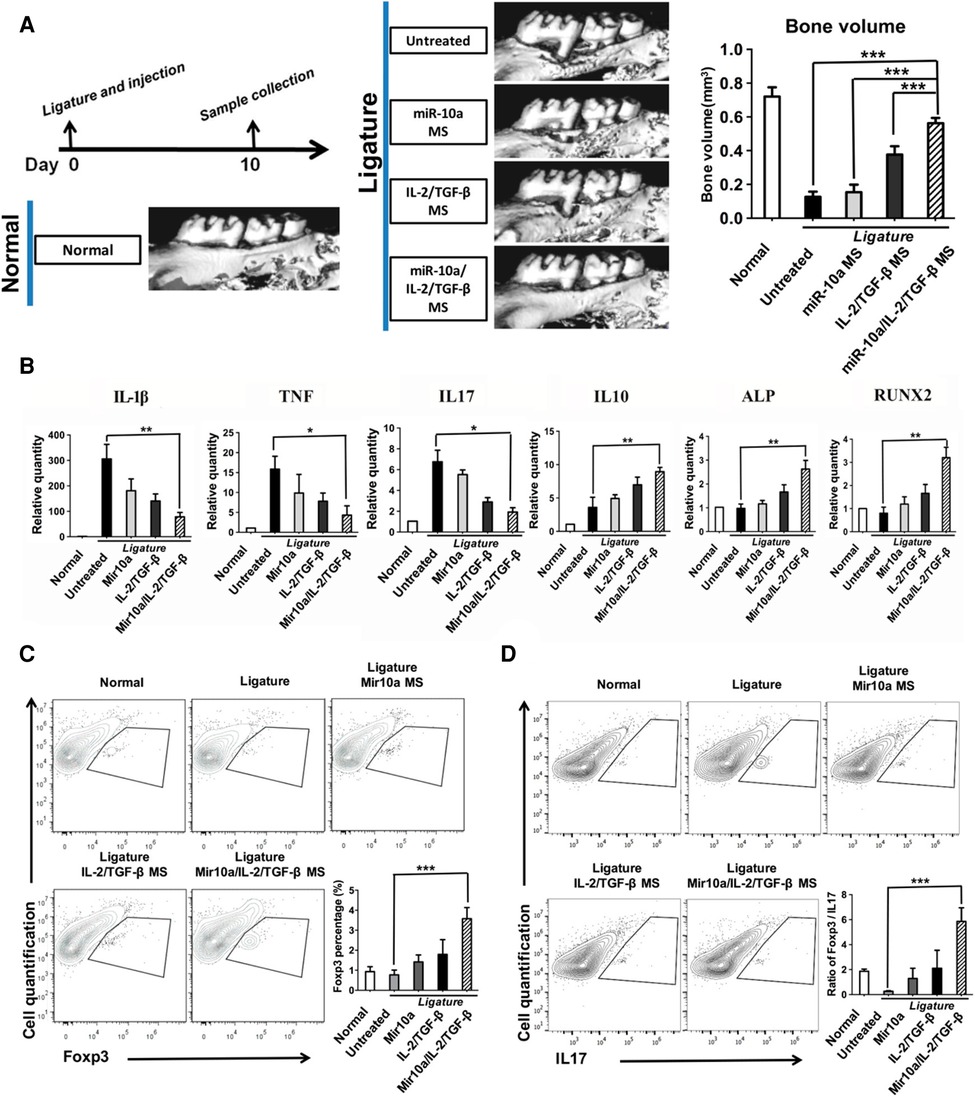
Figure 4. Multifunctionalized PLLA NF-SMS rescued bone resorption in a mouse periodontal disease model. (A) MicroCT results show bone loss between the first and the second molars in the periodontitis model and the bone volume changes in various treatment groups. (B) The gene expression of gingival tissues was quantified using real-time PCR, showing the effector T cell cytokines IL-1β, TNF, IL17, anti-inflammatory cytokine IL-10, and osteogenic markers ALP and RUNX2. (C) The flow cytometry analysis of the isolated gingival tissues shows the percentage of Foxp3+ cells (gated on CD4-expressing cells) in different treatment groups. (D) The IL17+ cells (gated on CD4-expressing cells) and the ratios of Treg/Th17 cells of the isolated gingival tissues are shown. Reprinted with permission from (157). Copyright 2018 American Chemical Society.
Another approach is to target the major cytokines involved in periodontitis rather than the cells. The cytokine IL-17A plays an important role in periodontitis-induced bone resorption, and it has been identified as a possible treatment target. In one study (160), anti-IL-17A antibodies were incorporated into PLGA microparticles to neutralize IL-17A-mediated periodontal bone resorption. The delivery system sustainably delivered anti-IL-17A antibodies into the periodontium of mice with ligature-induced periodontitis, the released antibodies inhibited alveolar bone loss and suppressed osteoclastic activity (the anti-IL-17A formulation also decreased expression of IL-6, an IL17A target gene known to induce bone resorption in periodontal tissues).
However, the delivery of therapeutic agents faces other limitations, such as bioavailability and toxicity issues. Thus, it would be ideal to modulate immune cells using only the biomaterial scaffold's physicochemical properties. In one study (161), gold nanoparticles were synthesized with various diameters, including 5, 13, and 45 nm. In vitro cell culture results showed the 45 nm nanoparticles significantly increased the expression of Arg-1, IL-10, and TGFβ (markers of M2 macrophages). In a rat periodontal model, the 45 nm nanoparticles greatly enhanced bone formation and attachment while reducing tissue destruction. This could be attributed to the immunomodulatory effect of the nanoparticles, which promoted a macrophage phenotype switch from M1 to M2. In another study (162), autoclaved chitosan powder was dissolved and mixed with beta-glycerophosphate to formulate a thermosensitive hydrogel. In a canine periodontitis model, the hydrogel promoted a significant regeneration of periodontal ligament formation of new bone and cementum without encapsulating any therapeutic agents. However, the hydrogel-host immune response was not thoroughly explored. But based on the treatment outcomes, it is reasonable to postulate that the chitosan-based thermosensitive hydrogel exhibited an immunomodulatory effect that is favorable for regeneration.
4. Future considerations
Despite numerous publications on the development of novel biomaterial-based treatments for periodontitis, conventional treatments, such as scaling and root planing, and guided tissue regeneration remain the gold standard in the clinical setting. To better translate these scientific findings to the clinical setting, future research needs to take the following key factors into consideration.
4.1. Experimental periodontitis design and evaluation
The first hurdle that limits the translatability of periodontal research comes from the design of the experimental periodontitis model, as it is crucial to standardize the periodontitis model to allow better comparisons between different studies. The three most common experimental periodontitis models found in the literature are ligature-induced periodontitis, bacterial solution instillation-induced periodontitis, and ligature pre-soaked in bacterial solution-induced periodontitis. From the perspective of pathophysiology, it is necessary to include bacterial infection to study the interaction between dysbiotic microbiota and host immunity. Ligature-induced periodontitis, on the other hand, can rapidly establish the disease model by inducing bone loss (163). Thus, the most appropriate experimental periodontitis model would be the combination of bacterial instillation and ligature-induced periodontitis.
Equally important is the standardization of experimental parameters for evaluating treatment outcomes; currently, the primary method of assessing alveolar bone regrowth is via microcomputed tomography (micro-CT). It provides quantitative information about the extent of distribution of the bone mineralization at the macromolecular level of the bone structure, enabling the assessment of trabecular thickness and mineral density. In addition, a histological analysis, such as hematoxylin and eosin stain, is utilized to help visualize the local inflammatory response and the formation of other connective tissues, such as cementum and periodontal ligament. However, many published studies only focused on the healing and regeneration of the alveolar bone and neglected to investigate the regeneration of other connective tissues of the periodontium. Furthermore, immunohistochemistry can help characterize cell phenotypes based on surface markers and intracellular markers, and cells can also be isolated from the surrounding gingival tissues for gene expression analysis. In addition, other analyses, such as chemical analysis (byproducts released from the biomaterials) and mechanical testing (structural integrity of biomaterials post-implantation/injection), can also be performed to evaluate the biomaterial-based scaffolds, aside from treatment outcomes (164), to provide a complete assessment of the biomaterial scaffolds.
4.2. Animal model selection
The selection of an animal model is another important factor, with the challenge being that each animal model has its own set of advantages and limitations. Many animal models have been proposed to study periodontitis-induced bone loss (165). Non-human primates have similar tooth structures to those of humans and can naturally develop dental plaque, calculus, oral microbial infections, and periodontal disease on their own (166). However, they are expensive and have special ethical constraints, which have limited their use in periodontitis models. In contrast, although rats are inherently more resistant to periodontitis and their microbiota differ from that of humans, they are often used as an experimental periodontitis model because their periodontal anatomy in the molar region is comparable to that of humans. Furthermore, similar to humans, the bacterial instillation of P. gingivalis with ligature placement can successfully induce periodontitis in rats and result in alveolar bone loss (138, 161). More recently, immunodeficient mice that have been engrafted with human cells or tissues, commonly known as humanized mouse models, have been used to study periodontitis (167). In one study, subgingival tissues from periodontitis patients were engrafted into immunodeficient mice, and subsequently, the human periodontitis-associated bacteria efficiently colonized and further aggravated bone resorption (168). This new periodontitis model offers the opportunity to better understand the effect of human periodontal-associated microbiota in periodontitis and to develop innovative biomaterial-based treatments.
4.3. Treatment modality
Aside from standardizing the periodontitis design, evaluation, and model selection, it is also necessary to differentiate between the different treatment modalities, mainly prevention and treatment. Only studies that started the biomaterial treatment after the periodontitis model was successfully established should be considered as treatment. While studies that commenced the biomaterial treatment and ligature placement simultaneously before the periodontitis model was established should only be classified as prevention. For instance, in one study (140), a thermoresponsive hydrogel was administered concurrently with ligature placement in a rat periodontitis model. Despite the conclusion that the hydrogel formulation reduced inflammation and preserved periodontal bone, it should only be considered a successful preventive measure rather than an effective treatment since the periodontitis model was not fully developed when the intervention started.
5. Conclusion
In conclusion, the regeneration of periodontium is a complex process as it involves the regeneration of cementum, periodontal ligament, and alveolar bone. Currently, many studies have solely focused on the regeneration of alveolar bone alone by utilizing biomaterial-based therapy. Although some successes have been reported, it is important to point out that a newly formed alveolar bone could happen without the formation of a new cementum and periodontal ligament; instead, bone growth could be accompanied by the down growth of epithelial tissues into the periodontal defect (169, 170). Thus, regeneration of the alveolar bone alone does not necessarily constitute regeneration of the entire periodontium. The challenge lies in the complexity of the periodontium, as it is composed of both soft and hard tissues with differences in architecture, mechanical property, and composition. Therefore, fundamental studies should be conducted to investigate the lineages of progenitor cells that are involved in the regeneration of each individual tissue, along with the crosstalk of cells between these tissues. Currently, the majority of the injectable biomaterials being developed are monophasic; although multiple components may be incorporated, the final mixture is often homogeneous and lacks a gradient of properties. The future design of scaffolds should also take the multi-tissue interface into consideration, as cell differentiation is heavily regulated by local environmental cues. Hence, it may be helpful to design a scaffold with a gradient of properties that allows the regeneration of both soft and hard tissues.
Author contributions
YZ conducted all of the literature searches and drafted the first version of the manuscript. CT conceptualized and discussed the original content of the manuscript. CG and AS conceptualized, revised, and polished the manuscript for publication. All authors contributed to the article and approved the submitted version.
Funding
This study was supported by funding from Colgate CARE Program and Research Grant from the Foundation for Endodontics, American Association of Endodontists (#510174). Colgate was not involved in the study design, collection, analysis, interpretation of data, the writing of this article or the decision to submit it for publication.
Acknowledgments
All figures were created with BioRender.com.
Conflict of interest
The author AS declared that they were an editorial board member of Frontiers, at the time of submission. This had no impact on the peer review process and the final decision. The remaining authors declare that the research was conducted in the absence of any commercial or financial relationships that could be construed as a potential conflict of interest.
Publisher's note
All claims expressed in this article are solely those of the authors and do not necessarily represent those of their affiliated organizations, or those of the publisher, the editors and the reviewers. Any product that may be evaluated in this article, or claim that may be made by its manufacturer, is not guaranteed or endorsed by the publisher.
References
1. Nazir MA. Prevalence of periodontal disease, its association with systemic diseases and prevention. Int J Health Sci. (2017) 11(2):72–80. PMID: 28539867
2. Könönen E, Gursoy M, Gursoy UK. Periodontitis: a multifaceted disease of tooth-supporting tissues. J Clin Med. (2019) 8(8):1135. doi: 10.3390/jcm8081135
3. Herbert BA, Novince CM, Kirkwood KL. Aggregatibacter actinomycetemcomitans, a potent immunoregulator of the periodontal host defense system and alveolar bone homeostasis. Mol Oral Microbiol. (2016) 31(3):207–27. doi: 10.1111/omi.12119
4. Mysak J, Podzimek S, Sommerova P, Lyuya-Mi Y, Bartova J, Janatova T, et al. Porphyromonas gingivalis: major periodontopathic pathogen overview. J Immunol Res. (2014) 2014:476068. doi: 10.1155/2014/476068
5. Socransky SS, Haffajee AD, Cugini MA, Smith C, Kent RL. Microbial complexes in subgingival plaque. J Clin Periodontol. (1998) 25(2):134–44. doi: 10.1111/j.1600-051X.1998.tb02419.x
6. Di Martino P. Extracellular polymeric substances, a key element in understanding biofilm phenotype. AIMS Microbiol. (2018) 4(2):274–88. doi: 10.3934/microbiol.2018.2.274
7. Yu F, Huo F, Li F, Zuo Y, Wang C, Ye L. Aberrant NF-κB activation in odontoblasts orchestrates inflammatory matrix degradation and mineral resorption. Int J Oral Sci. (2022) 14(1):1–11. doi: 10.1038/s41368-021-00151-3
8. Deng ZL, Szafrański SP, Jarek M, Bhuju S, Wagner-Döbler I. Dysbiosis in chronic periodontitis: key microbial players and interactions with the human host. Sci Rep. (2017) 7(1):3703. doi: 10.1038/s41598-017-03804-8
9. Houle MA, Grenier D, Plamondon P, Nakayama K. The collagenase activity of Porphyromonas gingivalis is due to arg-gingipain. FEMS Microbiol Lett. (2003) 221(2):181–5. doi: 10.1016/S0378-1097(03)00178-2
10. Lalla E, Papapanou PN. Diabetes mellitus and periodontitis: a tale of two common interrelated diseases. Nat Rev Endocrinol. (2011) 7(12):738–48. doi: 10.1038/nrendo.2011.106
11. Stewart R, West M. Increasing evidence for an association between periodontitis and cardiovascular disease. Circulation. (2016) 133(6):549–51. doi: 10.1161/CIRCULATIONAHA.115.020869
12. Gomes-Filho IS, Cruz SSD, Trindade SC, Passos-Soares JS, Carvalho-Filho PC, Figueiredo ACMG, et al. Periodontitis and respiratory diseases: a systematic review with meta-analysis. Oral Dis. (2020) 26(2):439–46. doi: 10.1111/odi.13228
13. Ide M, Harris M, Stevens A, Sussams R, Hopkins V, Culliford D, et al. Periodontitis and cognitive decline in Alzheimer’s disease. PLoS One. (2016) 11(3):e0151081. doi: 10.1371/journal.pone.0151081
14. Mealey BL, Oates TW, American Academy of Periodontology. Diabetes mellitus and periodontal diseases. J Periodontol. (2006) 77(8):1289–303. doi: 10.1902/jop.2006.050459
15. Singh M, Bains VK, Jhingran R, Srivastava R, Madan R, Maurya SC, et al. Prevalence of periodontal disease in type 2 diabetes mellitus patients: a cross-sectional study. Contemp Clin Dent. (2019) 10(2):349–57. doi: 10.4103/ccd.ccd_648_18
16. Preshaw PM, Taylor JJ. How has research into cytokine interactions and their role in driving immune responses impacted our understanding of periodontitis? J Clin Periodontol. (2011) 38(Suppl 11):60–84. doi: 10.1111/j.1600-051X.2010.01671.x
17. Lisco G, Giagulli VA, De Pergola G, Guastamacchia E, Jirillo E, Triggiani V. Hyperglycemia-Induced immune system disorders in diabetes Mellitus and the concept of hyperglycemic memory of innate immune cells: a perspective. Endocr Metab Immune Disord Drug Targets. (2022) 22(4):367–70. doi: 10.2174/1871530321666210924124336
18. Berbudi A, Rahmadika N, Tjahjadi AI, Ruslami R. Type 2 diabetes and its impact on the immune system. Curr Diabetes Rev. (2020) 16(5):442–9. doi: 10.2174/1573399815666191024085838
19. Preshaw PM, Alba AL, Herrera D, Jepsen S, Konstantinidis A, Makrilakis K, et al. Periodontitis and diabetes: a two-way relationship. Diabetologia. (2012) 55(1):21–31. doi: 10.1007/s00125-011-2342-y
20. Daryabor G, Atashzar MR, Kabelitz D, Meri S, Kalantar K. The effects of type 2 diabetes mellitus on organ metabolism and the immune system. Front Immunol. (2020) 11:1582. doi: 10.3389/fimmu.2020.01582
21. Mauri-Obradors E, Merlos A, Estrugo-Devesa A, Jané-Salas E, López-López J, Viñas M. Benefits of non-surgical periodontal treatment in patients with type 2 diabetes mellitus and chronic periodontitis: a randomized controlled trial. J Clin Periodontol. (2018) 45(3):345–53. doi: 10.1111/jcpe.12858
22. Chen L, Luo G, Xuan D, Wei B, Liu F, Li J, et al. Effects of non-surgical periodontal treatment on clinical response, serum inflammatory parameters, and metabolic control in patients with type 2 diabetes: a randomized study. J Periodontol. (2012) 83(4):435–43. doi: 10.1902/jop.2011.110327
23. Koromantzos PA, Makrilakis K, Dereka X, Katsilambros N, Vrotsos IA, Madianos PN. A randomized, controlled trial on the effect of non-surgical periodontal therapy in patients with type 2 diabetes. Part I: effect on periodontal status and glycaemic control. J Clin Periodontol. (2011) 38(2):142–7. doi: 10.1111/j.1600-051X.2010.01652.x
24. Nishikawa T, Suzuki Y, Sawada N, Kobayashi Y, Nakamura N, Miyabe M, et al. Therapeutic potential for insulin on type 1 diabetes-associated periodontitis: analysis of experimental periodontitis in streptozotocin-induced diabetic rats. J Diabetes Investig. (2020) 11(6):1482–9. doi: 10.1111/jdi.13276
25. Graziani F, Karapetsa D, Alonso B, Herrera D. Nonsurgical and surgical treatment of periodontitis: how many options for one disease? Periodontol 2000. (2017) 75(1):152–88. doi: 10.1111/prd.12201
26. Takasaki AA, Aoki A, Mizutani K, Schwarz F, Sculean A, Wang CY, et al. Application of antimicrobial photodynamic therapy in periodontal and peri-implant diseases. Periodontol 2000. (2009) 51:109–40. doi: 10.1111/j.1600-0757.2009.00302.x
27. Valle LA, Lopes MMR, Zangrando MSR, Sant’Ana ACP, Greghi SLA, de Rezende MLR, et al. Blue photosensitizers for aPDT eliminate Aggregatibacter actinomycetemcomitans in the absence of light: an in vitro study. J Photochem Photobiol, B. (2019) 194:56–60. doi: 10.1016/j.jphotobiol.2019.03.005
28. Yoshida A, Sasaki H, Toyama T, Araki M, Fujioka J, Tsukiyama K, et al. Antimicrobial effect of blue light using Porphyromonas gingivalis pigment. Sci Rep. (2017) 7(1):5225. doi: 10.1038/s41598-017-05706-1
29. Salvi GE, Stähli A, Schmidt JC, Ramseier CA, Sculean A, Walter C. Adjunctive laser or antimicrobial photodynamic therapy to non-surgical mechanical instrumentation in patients with untreated periodontitis: a systematic review and meta-analysis. J Clin Periodontol. (2020) 47(Suppl 22):176–98. doi: 10.1111/jcpe.13236
30. Zhao Y, Pu R, Qian Y, Shi J, Si M. Antimicrobial photodynamic therapy versus antibiotics as an adjunct in the treatment of periodontitis and peri-implantitis: a systematic review and meta-analysis. Photodiagnosis Photodyn Ther. (2021) 34:102231. doi: 10.1016/j.pdpdt.2021.102231
31. Cobb CM. Clinical significance of non-surgical periodontal therapy: an evidence-based perspective of scaling and root planing. J Clin Periodontol. (2002) 29:6–16. PMID: 12010523
32. Cobb CM, Sottosanti JS. A re-evaluation of scaling and root planing. J Periodontol. (2021) 92(10):1370–8. doi: 10.1002/JPER.20-0839
33. Tolo K. Periodontal disease mechanisms in immunocompromised patients. J Clin Periodontol. (1991) 18(6):431–5. doi: 10.1111/j.1600-051X.1991.tb02312.x
34. Genco RJ, Borgnakke WS. Diabetes as a potential risk for periodontitis: association studies. Periodontol 2000. (2020) 83(1):40–5. doi: 10.1111/prd.12270
35. Arweiler NB, Auschill TM, Sculean A. Patient self-care of periodontal pocket infections. Periodontol 2000. (2018) 76(1):164–79. doi: 10.1111/prd.12152
36. Van der Weijden GAF, Dekkers GJ, Slot DE. Success of non-surgical periodontal therapy in adult periodontitis patients: a retrospective analysis. Int J Dent Hyg. (2019) 17(4):309–17. doi: 10.1111/idh.12399
37. Liang Y, Luan X, Liu X. Recent advances in periodontal regeneration: a biomaterial perspective. Bioact Mater. (2020) 5(2):297–308. doi: 10.1016/j.bioactmat.2020.02.012
38. Liu J, Ruan J, Weir MD, Ren K, Schneider A, Wang P, et al. Periodontal bone-ligament-cementum regeneration via scaffolds and stem cells. Cells. (2019) 8(6):E537. doi: 10.3390/cells8060537
39. Reynolds MA, Aichelmann-Reidy ME, Branch-Mays GL. Regeneration of periodontal tissue: bone replacement grafts. Dent Clin North Am. (2010) 54(1):55–71. doi: 10.1016/j.cden.2009.09.003
40. Del Fabbro M, Karanxha L, Panda S, Bucchi C, Nadathur Doraiswamy J, Sankari M, et al. Autologous platelet concentrates for treating periodontal infrabony defects. Cochrane Database Syst Rev. (2018) 2018(11):CD011423. doi: 10.1002/14651858.cd011423.pub2
41. Kornsuthisopon C, Pirarat N, Osathanon T, Kalpravidh C. Autologous platelet-rich fibrin stimulates canine periodontal regeneration. Sci Rep. (2020) 10:1850. doi: 10.1038/s41598-020-58732-x
42. Hathaway-Schrader JD, Novince CM. Maintaining homeostatic control of periodontal bone tissue. Periodontol 2000. (2021) 86(1):157–87. doi: 10.1111/prd.12368
43. Taubman MA, Valverde P, Han X, Kawai T. Immune response: the key to bone resorption in periodontal disease. J Periodontol. (2005) 76(11 Suppl):2033–41. doi: 10.1902/jop.2005.76.11-S.2033
44. Wang J, Zhou Y, Ren B, Zou L, He B, Li M. The role of neutrophil extracellular traps in periodontitis. Front Cell Infect Microbiol. (2021) 11:639144. doi: 10.3389/fcimb.2021.639144
45. Silva LM, Brenchley L, Moutsopoulos NM. Primary immunodeficiencies reveal the essential role of tissue neutrophils in periodontitis. Immunol Rev. (2019) 287(1):226–35. doi: 10.1111/imr.12724
46. Cortés-Vieyra R, Rosales C, Uribe-Querol E. Neutrophil functions in periodontal homeostasis. J Immunol Res. (2016) 2016:1396106. doi: 10.1155/2016/1396106
47. Hirschfeld J. Neutrophil subsets in periodontal health and disease: a mini review. Front Immunol. (2020) 10:3001. doi: 10.3389/fimmu.2019.03001
48. Landzberg M, Doering H, Aboodi GM, Tenenbaum HC, Glogauer M. Quantifying oral inflammatory load: oral neutrophil counts in periodontal health and disease. J Periodontal Res. (2015) 50(3):330–6. doi: 10.1111/jre.12211
49. Lakschevitz FS, Aboodi GM, Glogauer M. Oral neutrophil transcriptome changes result in a pro-survival phenotype in periodontal diseases. PLoS One. (2013) 8(7):e68983. doi: 10.1371/journal.pone.0068983
50. Roberts HM, Ling MR, Insall R, Kalna G, Spengler J, Grant MM, et al. Impaired neutrophil directional chemotactic accuracy in chronic periodontitis patients. J Clin Periodontol. (2015) 42(1):1–11. doi: 10.1111/jcpe.12326
51. Nicu EA, Rijkschroeff P, Wartewig E, Nazmi K, Loos BG. Characterization of oral polymorphonuclear neutrophils in periodontitis patients: a case-control study. BMC Oral Health. (2018) 18:149. doi: 10.1186/s12903-018-0615-2
52. Pillay J, Tak T, Kamp VM, Koenderman L. Immune suppression by neutrophils and granulocytic myeloid-derived suppressor cells: similarities and differences. Cell Mol Life Sci. (2013) 70(20):3813. doi: 10.1007/s00018-013-1286-4
53. Medara N, Lenzo JC, Walsh KA, Reynolds EC, O’Brien-Simpson NM, Darby IB. Peripheral neutrophil phenotypes during management of periodontitis. J Periodontal Res. (2021) 56(1):58–68. doi: 10.1111/jre.12793
54. Thevenot PT, Baker DW, Weng H, Sun MW, Tang L. The pivotal role of fibrocytes and mast cells in mediating fibrotic reactions to biomaterials. Biomaterials. (2011) 32(33):8394–403. doi: 10.1016/j.biomaterials.2011.07.084
55. Mukai K, Tsai M, Saito H, Galli SJ. Mast cells as sources of cytokines, chemokines and growth factors. Immunol Rev. (2018) 282(1):121–50. doi: 10.1111/imr.12634
56. Brito VGB, Patrocinio MS, Sousa MCL, Barreto AEA, Frasnelli SCT, Lara VS, et al. Mast cells contribute to alveolar bone loss in spontaneously hypertensive rats with periodontal disease regulating cytokines production. PLoS One. (2021) 16(3):e0247372. doi: 10.1371/journal.pone.0247372
57. E Ribeiro LSF, dos Santos JN, Rocha CAG, Cury PR. Association between mast cells and collagen maturation in chronic periodontitis in humans. J Histochem Cytochem. (2018) 66(6):467–75. doi: 10.1369/0022155418765131
58. Huang S, Lu F, Chen Y, Huang B, Liu M. Mast cell degranulation in human periodontitis. J Periodontol. (2013) 84(2):248–55. doi: 10.1902/jop.2012.120066
59. Huang B, Dai Q, Huang SG. Expression of toll-like receptor 4 on mast cells in gingival tissues of human chronic periodontitis. Mol Med Rep. (2018) 17(5):6731–5. doi: 10.3892/mmr.2018.8648
60. Molteni M, Gemma S, Rossetti C. The role of toll-like receptor 4 in infectious and noninfectious inflammation. Mediators Inflamm. (2016) 2016:6978936. doi: 10.1155/2016/6978936
61. Ley K. M1 means kill; M2 means heal. J Immunol. (2017) 199(7):2191–3. doi: 10.4049/jimmunol.1701135
62. Palma A, Jarrah AS, Tieri P, Cesareni G, Castiglione F. Gene regulatory network modeling of macrophage differentiation corroborates the continuum hypothesis of polarization states. Front Physiol. (2018) 9:1659. doi: 10.3389/fphys.2018.01659
63. Bajpai G, Bredemeyer A, Li W, Zaitsev K, Koenig AL, Lokshina I, et al. Tissue resident CCR2- and CCR2+ cardiac macrophages differentially orchestrate monocyte recruitment and fate specification following myocardial injury. Circ Res. (2019) 124(2):263–78. doi: 10.1161/CIRCRESAHA.118.314028
64. Vega-Pérez A, Villarrubia LH, Godio C, Gutiérrez-González A, Feo-Lucas L, Ferriz M, et al. Resident macrophage-dependent immune cell scaffolds drive anti-bacterial defense in the peritoneal cavity. Immunity. (2021) 54(11):2578–2594.e5. doi: 10.1016/j.immuni.2021.10.007
65. Arango Duque G, Descoteaux A. Macrophage cytokines: involvement in immunity and infectious diseases. Front Immunol. (2014) 5:491. doi: 10.3389/fimmu.2014.00491
66. Scull CM, Hays WD, Fischer TH. Macrophage pro-inflammatory cytokine secretion is enhanced following interaction with autologous platelets. J Inflamm. (2010) 7(1):53. doi: 10.1186/1476-9255-7-53
67. Makita N, Hizukuri Y, Yamashiro K, Murakawa M, Hayashi Y. IL-10 enhances the phenotype of M2 macrophages induced by IL-4 and confers the ability to increase eosinophil migration. Int Immunol. (2015) 27(3):131–41. doi: 10.1093/intimm/dxu090
68. Van Dyken SJ, Locksley RM. Interleukin-4- and interleukin-13-mediated alternatively activated macrophages: roles in homeostasis and disease. Annu Rev Immunol. (2013) 31:317–43. doi: 10.1146/annurev-immunol-032712-095906
69. Almubarak A, Tanagala KKK, Papapanou PN, Lalla E, Momen-Heravi F. Disruption of monocyte and macrophage homeostasis in periodontitis. Front Immunol. (2020) 11:330. doi: 10.3389/fimmu.2020.00330
70. Chen MH, Wang YH, Sun BJ, Yu LM, Chen QQ, Han XX, et al. HIF-1α activator DMOG inhibits alveolar bone resorption in murine periodontitis by regulating macrophage polarization. Int Immunopharmacol. (2021) 99:107901. doi: 10.1016/j.intimp.2021.107901
71. Miao Y, He L, Qi X, Lin X. Injecting immunosuppressive M2 macrophages alleviates the symptoms of periodontitis in mice. Front Mol Biosci. (2020) 7:603817. doi: 10.3389/fmolb.2020.603817
72. Garaicoa-Pazmino C, Fretwurst T, Squarize CH, Berglundh T, Giannobile WV, Larsson L, et al. Characterization of macrophage polarization in periodontal disease. J Clin Periodontol. (2019) 46(8):830–9. doi: 10.1111/jcpe.13156
73. Zhu FJ, Tong YL, Sheng ZY, Yao YM. Role of dendritic cells in the host response to biomaterials and their signaling pathways. Acta Biomater. (2019) 94:132–44. doi: 10.1016/j.actbio.2019.05.038
74. Song L, Dong G, Guo L, Graves DT. The function of dendritic cells in modulating the host response. Mol Oral Microbiol. (2018) 33(1):13–21. doi: 10.1111/omi.12195
75. Li H, Shi B. Tolerogenic dendritic cells and their applications in transplantation. Cell Mol Immunol. (2015) 12(1):24–30. doi: 10.1038/cmi.2014.52
76. Xie ZX, Zhang HL, Wu XJ, Zhu J, Ma DH, Jin T. Role of the immunogenic and tolerogenic subsets of dendritic cells in multiple sclerosis. Mediat Inflamm. (2015) 2015:e513295. doi: 10.1155/2015/513295
77. Zhu Y, Winer D, Goh C, Shrestha A. Injectable thermosensitive hydrogel to modulate tolerogenic dendritic cells under hyperglycemic condition. Biomater Sci. (2023) 11(6):2091–102. doi: 10.1039/D2BM01881K
78. Kanamaru F, Iwai H, Ikeda T, Nakajima A, Ishikawa I, Azuma M. Expression of membrane-bound and soluble receptor activator of NF-kappaB ligand (RANKL) in human T cells. Immunol Lett. (2004) 94(3):239–46. doi: 10.1016/j.imlet.2004.05.010
79. Kim AR, Kim JH, Choi YH, Jeon YE, Cha JH, Bak EJ, et al. The presence of neutrophils causes RANKL expression in periodontal tissue, giving rise to osteoclast formation. J Periodontal Res. (2020) 55(6):868–76. doi: 10.1111/jre.12779
80. Teng YT, Nguyen H, Gao X, Kong YY, Gorczynski RM, Singh B, et al. Functional human T-cell immunity and osteoprotegerin ligand control alveolar bone destruction in periodontal infection. J Clin Invest. (2000) 106(6):R59–67. doi: 10.1172/JCI10763
81. Cury PR, Carmo JP, Horewicz VV, Santos JN, Barbuto JA. Altered phenotype and function of dendritic cells in individuals with chronic periodontitis. Arch Oral Biol. (2013) 58(9):1208–16. doi: 10.1016/j.archoralbio.2013.03.013
82. Hajishengallis G, Chavakis T. Local and systemic mechanisms linking periodontal disease and inflammatory comorbidities. Nat Rev Immunol. (2021) 21(7):426–40. doi: 10.1038/s41577-020-00488-6
83. D’Aiuto F, Parkar M, Andreou G, Suvan J, Brett PM, Ready D, et al. Periodontitis and systemic inflammation: control of the local infection is associated with a reduction in serum inflammatory markers. J Dent Res. (2004) 83(2):156–60. doi: 10.1177/154405910408300214
84. Souto GR, Queiroz-Junior CM, de Abreu MHNG, Costa FO, Mesquita RA. Pro-inflammatory, Th1, Th2, Th17 cytokines and dendritic cells: a cross-sectional study in chronic periodontitis. PLoS One. (2014) 9(3):e91636. doi: 10.1371/journal.pone.0091636
85. Rajendran M, Looney S, Singh N, Elashiry M, Meghil MM, El-Awady AR, et al. Systemic antibiotic therapy reduces circulating inflammatory dendritic cells and treg-Th17 plasticity in periodontitis. J Immunol. (2019) 202(9):2690–9. doi: 10.4049/jimmunol.1900046
86. Bell GM, Anderson AE, Diboll J, Reece R, Eltherington O, Harry RA, et al. Autologous tolerogenic dendritic cells for rheumatoid and inflammatory arthritis. Ann Rheum Dis. (2017) 76(1):227–34. doi: 10.1136/annrheumdis-2015-208456
87. Qiao H, Mei J, Yuan K, Zhang K, Zhou F, Tang T, et al. Immune-regulating strategy against rheumatoid arthritis by inducing tolerogenic dendritic cells with modified zinc peroxide nanoparticles. J Nanobiotechnology. (2022) 20(1):323. doi: 10.1186/s12951-022-01536-0
88. Yuan S, Chen Y, Zhang M, Wang Z, Hu Z, Ruan Y, et al. Overexpression of miR-223 promotes tolerogenic properties of dendritic cells involved in heart transplantation tolerance by targeting Irak1. Front Immunol. (2021) 12:676337. doi: 10.3389/fimmu.2021.676337
89. Lange J, Rivera-Ballesteros O, Buggert M. Human mucosal tissue-resident memory T cells in health and disease. Mucosal Immunol. (2022) 15(3):389–97. doi: 10.1038/s41385-021-00467-7
90. Kolar P, Schmidt-Bleek K, Schell H, Gaber T, Toben D, Schmidmaier G, et al. The early fracture hematoma and its potential role in fracture healing. Tissue Eng Part B Rev. (2010) 16(4):427–34. doi: 10.1089/ten.teb.2009.0687
91. Buchwald ZS, Kiesel JR, Yang C, DiPaolo R, Novack DV, Aurora R. Osteoclast-induced Foxp3+ CD8 T-cells limit bone loss in mice. Bone. (2013) 56(1):163–73. doi: 10.1016/j.bone.2013.05.024
92. Han YK, Jin Y, Miao YB, Shi T, Lin XP. CD8+ Foxp3+ T cells affect alveolar bone homeostasis via modulating Tregs/Th17 during induced periodontitis: an adoptive transfer experiment. Inflammation. (2018) 41(5):1791–803. doi: 10.1007/s10753-018-0822-7
93. Deng J, Lu C, Zhao Q, Chen K, Ma S, Li Z. The TH17/Treg cell balance: crosstalk among the immune system, bone and microbes in periodontitis. J Periodontal Res. (2022) 57(2):246–55. doi: 10.1111/jre.12958
94. Dutzan N, Kajikawa T, Abusleme L, Greenwell-Wild T, Zuazo CE, Ikeuchi T, et al. A dysbiotic microbiome triggers TH17 cells to mediate oral mucosal immunopathology in mice and humans. Sci Transl Med. (2018) 10(463):eaat0797. doi: 10.1126/scitranslmed.aat0797
95. Su X, Zhang J, Qin X. CD40 up-regulation on dendritic cells correlates with Th17/treg imbalance in chronic periodontitis in young population. Innate Immun. (2020) 26(6):482–9. doi: 10.1177/1753425920917731
96. Okeke EB, Uzonna JE. The pivotal role of regulatory T cells in the regulation of innate immune cells. Front Immunol. (2019) 10:680. doi: 10.3389/fimmu.2019.00680
97. Bui FQ, Almeida-da-Silva CLC, Huynh B, Trinh A, Liu J, Woodward J, et al. Association between periodontal pathogens and systemic disease. Biomed J. (2019) 42(1):27–35. doi: 10.1016/j.bj.2018.12.001
98. Alvarez C, Suliman S, Almarhoumi R, Vega ME, Rojas C, Monasterio G, et al. Regulatory T cell phenotype and anti-osteoclastogenic function in experimental periodontitis. Sci Rep. (2020) 10(1):19018. doi: 10.1038/s41598-020-76038-w
99. Okui T, Aoki Y, Ito H, Honda T, Yamazaki K. The presence of IL-17+/FOXP3+ double-positive cells in periodontitis. J Dent Res. (2012) 91(6):574–9. doi: 10.1177/0022034512446341
100. Kou Y, Jiang Y, Liu S, Yang P, Lu Y, Liu H, et al. Regulatory T cells showed characteristics of T helper-17(Th17) cells in mice periodontitis model. Oral Dis. (2023) 29(3):1149–62. doi: 10.1111/odi.14072
101. Lanzavecchia A, Sallusto F. Human B cell memory. Curr Opin Immunol. (2009) 21(3):298–304. doi: 10.1016/j.coi.2009.05.019
102. Mahanonda R, Champaiboon C, Subbalekha K, Sa-Ard-Iam N, Rattanathammatada W, Thawanaphong S, et al. Human memory B cells in healthy gingiva, gingivitis, and periodontitis. J Immunol. (2016) 197(3):715–25. doi: 10.4049/jimmunol.1600540
103. Demoersman J, Pochard P, Framery C, Simon Q, Boisramé S, Soueidan A, et al. B cell subset distribution is altered in patients with severe periodontitis. PLoS One. (2018) 13(2):e0192986. doi: 10.1371/journal.pone.0192986
104. Jing L, Kim S, Sun L, Wang L, Mildner E, Divaris K, et al. IL-37- and IL-35/IL-37-producing plasma cells in chronic periodontitis. J Dent Res. (2019) 98(7):813–21. doi: 10.1177/0022034519847443
105. Li W, Zhang Z, Wang ZM. Differential immune cell infiltrations between healthy periodontal and chronic periodontitis tissues. BMC Oral Health. (2020) 20(1):293. doi: 10.1186/s12903-020-01287-0
106. Kim TG, Shin H, Lim DW. Biomimetic scaffolds for tissue engineering. Adv Funct Mater. (2012) 22(12):2446–68. doi: 10.1002/adfm.201103083
107. Amer LD, Saleh LS, Walker C, Thomas S, Janssen WJ, Alper S, et al. Inflammation via myeloid differentiation primary response gene 88 signaling mediates the fibrotic response to implantable synthetic poly(ethylene glycol) hydrogels. Acta Biomater. (2019) 100:105–17. doi: 10.1016/j.actbio.2019.09.043
108. Cai L, Liu W, Cui Y, Liu Y, Du W, Zheng L, et al. Biomaterial stiffness guides cross-talk between chondrocytes: implications for a novel cellular response in cartilage tissue engineering. ACS Biomater Sci Eng. (2020) 6(8):4476–89. doi: 10.1021/acsbiomaterials.0c00367
109. Zhu Y, Goh C, Shrestha A. Biomaterial properties modulating bone regeneration. Macromol Biosci. (2021) 21(4):2000365. doi: 10.1002/mabi.202000365
110. Borselli C, Cezar CA, Shvartsman D, Vandenburgh HH, Mooney DJ. The role of multifunctional delivery scaffold in the ability of cultured myoblasts to promote muscle regeneration. Biomaterials. (2011) 32(34):8905–14. doi: 10.1016/j.biomaterials.2011.08.019
111. Lausch AJ, Chong LC, Uludag H, Sone ED. Multiphasic collagen scaffolds for engineered tissue interfaces. Adv Funct Mater. (2018) 28(48):1804730. doi: 10.1002/adfm.201804730
112. Lee CH, Hajibandeh J, Suzuki T, Fan A, Shang P, Mao JJ. Three-dimensional printed multiphase scaffolds for regeneration of periodontium complex. Tissue Eng Part A. (2014) 20(7–8):1342–51. doi: 10.1089/ten.tea.2013.0386
113. Cheng W, Ding Z, Zheng X, Lu Q, Kong X, Zhou X, et al. Injectable hydrogel systems with multiple biophysical and biochemical cues for bone regeneration. Biomater Sci. (2020) 8(9):2537–48. doi: 10.1039/D0BM00104J
114. Cui ZK, Kim S, Baljon JJ, Wu BM, Aghaloo T, Lee M. Microporous methacrylated glycol chitosan-montmorillonite nanocomposite hydrogel for bone tissue engineering. Nat Commun. (2019) 10(1):3523. doi: 10.1038/s41467-019-11511-3
115. Hasani-Sadrabadi MM, Sarrion P, Pouraghaei S, Chau Y, Ansari S, Li S, et al. An engineered cell-laden adhesive hydrogel promotes craniofacial bone tissue regeneration in rats. Sci Transl Med. (2020) 12(534):eaay6853. doi: 10.1126/scitranslmed.aay6853
116. Wang B, Liu W, Xing D, Li Y, Li R, Du Y, et al. Injectable gelatin-chitosan-hydroxyapatitemicro-cryogels enabling stem cell osteogenic differentiation and accelerating theregeneration of subchondral bone cyst. Osteoarthr Cartil. (2017) 25:S333. doi: 10.1016/j.joca.2017.02.558
117. Yuan Z, Yuan X, Zhao Y, Cai Q, Wang Y, Luo R, et al. Injectable GelMA cryogel microspheres for modularized cell delivery and potential vascularized bone regeneration. Small. (2021) 17(11):2006596. doi: 10.1002/smll.202006596
118. Tan J, Zhang M, Hai Z, Wu C, Lin J, Kuang W, et al. Sustained release of two bioactive factors from supramolecular hydrogel promotes periodontal bone regeneration. ACS Nano. (2019) 13(5):5616–22. doi: 10.1021/acsnano.9b00788
119. Wu J, Li G, Ye T, Lu G, Li R, Deng L, et al. Stem cell-laden injectable hydrogel microspheres for cancellous bone regeneration. Chem Eng J. (2020) 393:124715. doi: 10.1016/j.cej.2020.124715
120. Matamoros-Veloza A, Hossain KMZ, Scammell BE, Ahmed I, Hall R, Kapur N. Formulating injectable pastes of porous calcium phosphate glass microspheres for bone regeneration applications. J Mech Behav Biomed Mater. (2020) 102:103489. doi: 10.1016/j.jmbbm.2019.103489
121. Yuan S, Shen Y, Li Z. Injectable cell- and growth factor-free poly(4-hydroxybutyrate) (P4HB) microspheres with open porous structures and great efficiency of promoting bone regeneration. ACS Appl Bio Mater. (2021) 4(5):4432–40. doi: 10.1021/acsabm.1c00188
122. Bai M, Yin H, Zhao J, Li Y, Yang Y, Wu Y. Application of PMMA bone cement composited with bone-mineralized collagen in percutaneous kyphoplasty. Regen Biomater. (2017) 4(4):251–5. doi: 10.1093/rb/rbx019
123. Tan S, Wang Y, Du Y, Xiao Y, Zhang S. Injectable bone cement with magnesium-containing microspheres enhances osteogenesis via anti-inflammatory immunoregulation. Bioact Mater. (2021) 6(10):3411–23. doi: 10.1016/j.bioactmat.2021.03.006
124. Liao H, Walboomers XF, Habraken WJEM, Zhang Z, Li Y, Grijpma DW, et al. Injectable calcium phosphate cement with PLGA, gelatin and PTMC microspheres in a rabbit femoral defect. Acta Biomater. (2011) 7(4):1752–9. doi: 10.1016/j.actbio.2010.12.020
125. Babo PS, Carvalho PP, Santo VE, Faria S, Gomes ME, Reis RL. Assessment of bone healing ability of calcium phosphate cements loaded with platelet lysate in rat calvarial defects. J Biomater Appl. (2016) 31(5):637–49. doi: 10.1177/0885328216669474
126. Shirakata Y, Setoguchi T, Machigashira M, Matsuyama T, Furuichi Y, Hasegawa K, et al. Comparison of injectable calcium phosphate bone cement grafting and open flap debridement in periodontal intrabony defects: a randomized clinical trial. J Periodontol. (2008) 79(1):25–32. doi: 10.1902/jop.2008.070141
127. Gao X, Zhou Y, Ma G, Shi S, Yang D, Lu F, et al. A water-soluble photocrosslinkable chitosan derivative prepared by Michael-addition reaction as a precursor for injectable hydrogel. Carbohydr Polym. (2010) 79(3):507–12. doi: 10.1016/j.carbpol.2009.08.033
128. Liu Y, Li T, Sun M, Cheng Z, Jia W, Jiao K, et al. ZIF-8 modified multifunctional injectable photopolymerizable GelMA hydrogel for the treatment of periodontitis. Acta Biomater. (2022) 146:37–48. doi: 10.1016/j.actbio.2022.03.046
129. Rabelo CC, Feres M, Gonçalves C, Figueiredo LC, Faveri M, Tu YK, et al. Systemic antibiotics in the treatment of aggressive periodontitis. A systematic review and a Bayesian network meta-analysis. J Clin Periodontol. (2015) 42(7):647–57. doi: 10.1111/jcpe.12427
130. Mei L, Huang X, Xie Y, Chen J, Huang Y, Wang B, et al. An injectable in situ gel with cubic and hexagonal nanostructures for local treatment of chronic periodontitis. Drug Deliv. (2017) 24(1):1148–58. doi: 10.1080/10717544.2017.1359703
131. Zhu Y, Marin LM, Xiao Y, Gillies ER, Siqueira WL. pH-sensitive chitosan nanoparticles for salivary protein delivery. Nanomaterials. (2021) 11(4):1028. doi: 10.3390/nano11041028
132. Dong Z, Sun Y, Chen Y, Liu Y, Tang C, Qu X. Injectable adhesive hydrogel through a microcapsule cross-link for periodontitis treatment. ACS Appl Bio Mater. (2019) 2(12):5985–94. doi: 10.1021/acsabm.9b00912
133. Chang PC, Chao YC, Hsiao MH, Chou HS, Jheng YH, Yu XH, et al. Inhibition of periodontitis induction using a stimuli-responsive hydrogel carrying naringin. J Periodontol. (2017) 88(2):190–6. doi: 10.1902/jop.2016.160189
134. Oortgiesen DAW, Walboomers XF, Bronckers ALJJ, Meijer GJ, Jansen JA. Periodontal regeneration using an injectable bone cement combined with BMP-2 or FGF-2. J Tissue Eng Regen Med. (2014) 8(3):202–9. doi: 10.1002/term.1514
135. Kwon DH, Bennett W, Herberg S, Bastone P, Pippig S, Rodriguez NA, et al. Evaluation of an injectable rhGDF-5/PLGA construct for minimally invasive periodontal regenerative procedures: a histological study in the dog. J Clin Periodontol. (2010) 37(4):390–7. doi: 10.1111/j.1600-051X.2010.01546.x
136. Guo H, Huang S, Yang X, Wu J, Kirk TB, Xu J, et al. Injectable and self-healing hydrogels with double-dynamic bond tunable mechanical, gel–sol transition and drug delivery properties for promoting periodontium regeneration in periodontitis. ACS Appl Mater Interfaces. (2021) 13(51):61638–52. doi: 10.1021/acsami.1c18701
137. Xu X, Gu Z, Chen X, Shi C, Liu C, Liu M, et al. An injectable and thermosensitive hydrogel: promoting periodontal regeneration by controlled-release of aspirin and erythropoietin. Acta Biomater. (2019) 01(86):235–46. doi: 10.1016/j.actbio.2019.01.001
138. Liu S, Wang YN, Ma B, Shao J, Liu H, Ge S. Gingipain-responsive thermosensitive hydrogel loaded with SDF-1 facilitates in situ periodontal tissue regeneration. ACS Appl Mater Interfaces. (2021) 13(31):36880–93. doi: 10.1021/acsami.1c08855
139. Aljudaibi S, Duane B. Do adjunctive statins improve periodontal treatment outcomes in patients with chronic periodontitis? Evid Based Dent. (2019) 20(1):18–9. doi: 10.1038/s41432-019-0009-6
140. Chen N, Ren R, Wei X, Mukundan R, Li G, Xu X, et al. Thermoresponsive hydrogel-based local delivery of simvastatin for the treatment of periodontitis. Mol Pharm. (2021) 18(5):1992–2003. doi: 10.1021/acs.molpharmaceut.0c01196
141. Yang Y, Trevethan M, Wang S, Zhao L. Beneficial effects of citrus flavanones naringin and naringenin and their food sources on lipid metabolism: an update on bioavailability, pharmacokinetics, and mechanisms. J Nutr Biochem. (2022) 104:108967. doi: 10.1016/j.jnutbio.2022.108967
142. Qiu P, Li M, Chen K, Fang B, Chen P, Tang Z, et al. Periosteal matrix-derived hydrogel promotes bone repair through an early immune regulation coupled with enhanced angio- and osteogenesis. Biomaterials. (2020) 227:119552. doi: 10.1016/j.biomaterials.2019.119552
143. Alkindi M, Ramalingam S, Alghamdi O, Alomran OM, Binsalah MA, Badwelan M. Guided bone regeneration with osteoconductive grafts and PDGF: a tissue engineering option for segmental bone defect reconstruction. J Appl Biomater Funct Mater. (2021) 19:2280800020987405. doi: 10.1177/2280800020987405
144. Kim SE, Yun YP, Lee JY, Shim JS, Park K, Huh JB. Co-delivery of platelet-derived growth factor (PDGF-BB) and bone morphogenic protein (BMP-2) coated onto heparinized titanium for improving osteoblast function and osteointegration. J Tissue Eng Regen Med. (2015) 9(12):E219–228. doi: 10.1002/term.1668
145. Julier Z, Karami R, Nayer B, Lu YZ, Park AJ, Maruyama K, et al. Enhancing the regenerative effectiveness of growth factors by local inhibition of interleukin-1 receptor signaling. Sci Adv. (2020) 6(24):eaba7602. doi: 10.1126/sciadv.aba7602
146. Ali M, Yang F, Plachokova AS, Jansen JA, Walboomers XF. Application of specialized pro-resolving mediators in periodontitis and peri-implantitis: a review. Eur J Oral Sci. (2021) 129(1):e12759. doi: 10.1111/eos.12759
147. Wu B, Walker J, Spur B, Rodriguez A, Yin K. Effects of lipoxin A4 on antimicrobial actions of neutrophils in sepsis. Prostaglandins Leukot Essent Fatty Acids. (2015) 94:55–64. doi: 10.1016/j.plefa.2014.11.005
148. Yuan J, Lin F, Chen L, Chen W, Pan X, Bai Y, et al. Lipoxin A4 regulates M1/M2 macrophage polarization via FPR2-IRF pathway. Inflammopharmacology. (2022) 30(2):487–98. doi: 10.1007/s10787-022-00942-y
149. Vassiliou EK, Kesler OM, Tadros JH, Ganea D. Bone marrow-derived dendritic cells generated in the presence of resolvin E1 induce apoptosis of activated CD4+ T cells. J Immunol. (2008) 181(7):4534–44. doi: 10.4049/jimmunol.181.7.4534
150. Machado FS, Johndrow JE, Esper L, Dias A, Bafica A, Serhan CN, et al. Anti-inflammatory actions of lipoxin A4 and aspirin-triggered lipoxin are SOCS-2 dependent. Nat Med. (2006) 12(3):330–4. doi: 10.1038/nm1355
151. Ramon S, Bancos S, Serhan CN, Phipps RP. Lipoxin A₄ modulates adaptive immunity by decreasing memory B-cell responses via an ALX/FPR2-dependent mechanism. Eur J Immunol. (2014) 44(2):357–69. doi: 10.1002/eji.201343316
152. Serhan CN, Jain A, Marleau S, Clish C, Kantarci A, Behbehani B, et al. Reduced inflammation and tissue damage in transgenic rabbits overexpressing 15-lipoxygenase and endogenous anti-inflammatory lipid mediators. J Immunol. (2003) 171(12):6856–65. doi: 10.4049/jimmunol.171.12.6856
153. Wang B, Booij-Vrieling HE, Bronkhorst EM, Shao J, Kouwer PHJ, Jansen JA, et al. Antimicrobial and anti-inflammatory thermo-reversible hydrogel for periodontal delivery. Acta Biomater. (2020) 116:259–67. doi: 10.1016/j.actbio.2020.09.018
154. Zhuang Z, Yoshizawa-Smith S, Glowacki A, Maltos K, Pacheco C, Shehabeldin M, et al. Induction of M2 macrophages prevents bone loss in murine periodontitis models. J Dent Res. (2019) 98(2):200–8. doi: 10.1177/0022034518805984
155. Shi J, Zhang Y, Zhang X, Chen R, Wei J, Hou J, et al. Remodeling immune microenvironment in periodontitis using resveratrol liposomes as an antibiotic-free therapeutic strategy. J Nanobiotechnol. (2021) 19(1):429. doi: 10.1186/s12951-021-01175-x
156. Glowacki AJ, Yoshizawa S, Jhunjhunwala S, Vieira AE, Garlet GP, Sfeir C, et al. Prevention of inflammation-mediated bone loss in murine and canine periodontal disease via recruitment of regulatory lymphocytes. Proc Natl Acad Sci U S A. (2013) 110(46):18525–30. doi: 10.1073/pnas.1302829110
157. Liu Z, Chen X, Zhang Z, Zhang X, Saunders L, Zhou Y, et al. Nanofibrous spongy microspheres to distinctly release miRNA and growth factors to enrich regulatory T cells and rescue periodontal bone loss. ACS Nano. (2018) 12(10):9785–99. doi: 10.1021/acsnano.7b08976
158. Greene AC, Shehabeldin M, Gao J, Balmert SC, Ratay M, Sfeir C, et al. Local induction of regulatory T cells prevents inflammatory bone loss in ligature-induced experimental periodontitis in mice. Sci Rep. (2022) 12(1):5032. doi: 10.1038/s41598-022-09150-8
159. Nagai K, Ideguchi H, Kajikawa T, Li X, Chavakis T, Cheng J, et al. An injectable hydrogel-formulated inhibitor of prolyl-4-hydroxylase promotes T regulatory cell recruitment and enhances alveolar bone regeneration during resolution of experimental periodontitis. FASEB J. (2020) 34(10):13726–40. doi: 10.1096/fj.202001248R
160. Pacheco CMF, Maltos KLM, Shehabeldin MS, Thomas LL, Zhuang Z, Yoshizawa S, et al. Local sustained delivery of anti-IL-17A antibodies limits inflammatory bone loss in murine experimental periodontitis. J Immunol. (2021) 206(10):2386–92. doi: 10.4049/jimmunol.2001432
161. Ni C, Zhou J, Kong N, Bian T, Zhang Y, Huang X, et al. Gold nanoparticles modulate the crosstalk between macrophages and periodontal ligament cells for periodontitis treatment. Biomaterials. (2019) 206:115–32. doi: 10.1016/j.biomaterials.2019.03.039
162. Zang S, Dong G, Peng B, Xu J, Ma Z, Wang X, et al. A comparison of physicochemical properties of sterilized chitosan hydrogel and its applicability in a canine model of periodontal regeneration. Carbohydr Polym. (2014) 113:240–8. doi: 10.1016/j.carbpol.2014.07.018
163. Marchesan J, Girnary MS, Jing L, Miao MZ, Zhang S, Sun L, et al. An experimental murine model to study periodontitis. Nat Protoc. (2018) 13(10):2247–67. doi: 10.1038/s41596-018-0035-4
164. Muschler GF, Raut VP, Patterson TE, Wenke JC, Hollinger JO. The design and use of animal models for translational research in bone tissue engineering and regenerative medicine. Tissue Eng Part B Rev. (2010) 16(1):123–45. doi: 10.1089/ten.teb.2009.0658
165. Oz HS, Puleo DA. Animal models for periodontal disease. J Biomed Biotechnol. (2011) 2011:754857. doi: 10.1155/2011/754857
166. Schou S, Holmstrup P, Kornman KS. Non-human primates used in studies of periodontal disease pathogenesis: a review of the literature. J Periodontol. (1993) 64(6):497–508. doi: 10.1902/jop.1993.64.6.497
167. Rojas C, García MP, Polanco AF, González-Osuna L, Sierra-Cristancho A, Melgar-Rodríguez S, et al. Humanized mouse models for the study of periodontitis: an opportunity to elucidate unresolved aspects of its immunopathogenesis and analyze new immunotherapeutic strategies. Front Immunol. (2021) 12:663328. doi: 10.3389/fimmu.2021.663328
168. Bai L, Chen BY, Liu Y, Zhang WC, Duan SZ. A mouse periodontitis model with humanized oral bacterial community. Front Cell Infect Microbiol. (2022) 12:842845. doi: 10.3389/fcimb.2022.842845
169. Caton J, Nyman S, Zander H. Histometric evaluation of periodontal surgery. II. Connective tissue attachment levels after four regenerative procedures. J Clin Periodontol. (1980) 7(3):224–31. doi: 10.1111/j.1600-051x.1980.tb01965.x
Keywords: periodontitis, injectable hydrogel, biomaterials, immune modulation, bone regeneration
Citation: Zhu Y, Tao C, Goh C and Shrestha A (2023) Innovative biomaterials for the treatment of periodontal disease. Front. Dent. Med 4:1163562. doi: 10.3389/fdmed.2023.1163562
Received: 10 February 2023; Accepted: 3 May 2023;
Published: 30 May 2023.
Edited by:
Amr S. Fawzy, University of Western Australia, AustraliaReviewed by:
Nandini Suresh, Meenakshi Academy of Higher Education and Research, IndiaEraldo Luiz Batista, Dalhousie University, Canada
© 2023 Zhu, Tao, Goh and Shrestha. This is an open-access article distributed under the terms of the Creative Commons Attribution License (CC BY). The use, distribution or reproduction in other forums is permitted, provided the original author(s) and the copyright owner(s) are credited and that the original publication in this journal is cited, in accordance with accepted academic practice. No use, distribution or reproduction is permitted which does not comply with these terms.
*Correspondence: Annie Shrestha, YW5uaWUuc2hyZXN0aGFAZGVudGlzdHJ5LnV0b3JvbnRvLmNh