- 1Sydney Brenner Institute for Molecular Bioscience (SBIMB), Faculty of Health Sciences, University of the Witwatersrand, Johannesburg, South Africa
- 2Division of Human Genetics, School of Pathology, National Health Laboratory Service and Faculty of Health Sciences, University of the Witwatersrand, Johannesburg, South Africa
- 3MRC/Wits Rural Public Health and Health Transitions Research Unit (Agincourt), School of Public Health, University of the Witwatersrand, Johannesburg, South Africa
Background: Despite hypertension being highly prevalent in individuals with African-ancestry, they are under-represented in large genome-wide association studies. Inclusion of African participants is essential to better understand genetic associations with blood pressure-related traits in Africans. This systematic review critically evaluates existing studies with African-ancestry participants and identifies knowledge gaps.
Methods: We followed the PRISMA protocol, HuGE Review handbook to identify literature on original research, in English, on genetic association studies for blood pressure-related traits (systolic and diastolic blood pressure, pulse and mean-arterial pressure, and hypertension) in populations with African-ancestry (January 2007 to April 2020). A narrative synthesis of the evidence was conducted.
Results: Twelve studies with African-ancestry participants met the eligibility criteria, within which 10 studies met the additional genetic association data criteria (i.e., reporting only on African-ancestry participants). Across the five blood pressure-related traits, 26 genome-wide significantly associated SNPs were identified, with six SNPs linked to more than one trait, illustrating pleiotropic effects. Among the SNP associations, 12 had not previously been described in non-African studies.
Discussion: The limited number of relevant studies highlights the dearth of genomic association studies on participants with African-ancestry, especially those located within Africa. Variations in study methodology, participant inclusion, adjustment for covariates (e.g., antihypertensive medication) and relatively small sample sizes make comparisons challenging, and have resulted in fewer significant associations, compared to large European studies. Regional variation in the prevalence and associated risk factors of hypertension across Africa makes a compelling argument to develop African cohorts to facilitate large genomic studies, using African-centric arrays. Data harmonisation and comparable study designs, such as described in the H3Africa CHAIR initiative, provide a good example toward achieving this goal.
Other relevant information: SS and J-TB were funded by the South African National Research Foundation. MR is a South African Research Chair in Genomics and Bioinformatics of African populations hosted by the University of the Witwatersrand, funded by the Department of Science and Innovation, and administered by the NRF. This review was registered at PROSPERO (registration number: CRD42020179221) and OSF (registration DOI: 10.17605/OSF.IO/QT2HA).
Introduction
Hypertension (HTN) or high blood pressure is a risk factor for premature deaths and disability worldwide (Forouzanfar et al., 2016; Juma et al., 2016), accounting for 17.9 million deaths in 2018. It is present in approximately 22% of the global population (affecting approximately 1 in 4 men and 1 in 5 women), with the highest prevalence observed in Africa (27%) and lowest in America (average of 18%, where North America < 20% and South America 20–24.9%) (WHO, 2016; 2018).
Over the past decade, the genome-wide association study (GWAS) approach has become the preferred approach to identify genetic factors associated with complex diseases and traits, such as HTN and blood pressure (BP) (Tam et al., 2019). GWAS aims to identify genetic variants and genes associated with the regulation and control of BP. The first case-control studies for HTN and quantitative BP phenotypes were conducted in 2007 as part of the Wellcome Trust Case Control Consortium (Burton et al., 2007) and the Framingham Heart Study (Levy et al., 2007). The GWAS Catalog currently includes 5,152 genetic associations with BP based on 164 studies and 589 associations with HTN based on 76 studies (https://www.ebi.ac.uk/gwas, SNP (single nucleotide polymorphisms) trait p-value < 1E-5, accessed April 17, 2020).
The importance of increasing the representation of diverse populations in genomic research, including Africans, has been addressed in many studies (Ramsay et al., 2016; Martin et al., 2018; Popejoy et al., 2018; Bentley et al., 2020). Most GWAS studies have focused on the European ancestry populations (Azam and Azizan, 2018), and studies based on African-ancestry participants comprise mainly African Americans (AAs) (Manolio et al., 2009; Franceschini et al., 2013; Franceschini et al., 2016; Taylor et al., 2016; Liang et al., 2017). The first GWAS for HTN in AAs was conducted by Adeyemo et al. (2009). Larger studies are needed to understand the role of genetic variants and gene-environment interactions on BP and related phenotypes in African populations (Hendry et al., 2018).
This review critically evaluates the literature on GWASs for BP and HTN in participants with African-ancestry, using PubMed, PubMed Central (PMC), Scopus, Web of Science (WoS) and the GWAS Catalog databases.
Methods
Protocol and Registration
The systematic review was conducted using: 1) The Preferred Reporting Items for Systematic Reviews and Meta-Analysis (PRISMA) framework (Moher et al., 2009), 2) HuGENET™ (HuGE) Review handbook (Little et al., 2006), and 3) Systematic reviews of genetic association studies (Sagoo et al., 2009). The PRISMA checklist can be found in Supplementary Table S1.
To prevent any duplication of systematic reviews based on the topics addressed in this paper, the Cochrane Library (https://www.cochranelibrary.com), PROSPERO (the international prospective register of systematic review, https://www.crd.york.ac.uk/prospero), OSF (Open Science Framework, https://osf.io) and the Research Registry databases (https://www.researchregistry.com) were used to check for similar reviews. This systematic review was registered at PROSPERO database (registration number: CRD42020179221. Available at: https://www.crd.york.ac.uk/prospero/display_record.php?ID=CRD42020179221). The systematic review was also registered with the OSF as recommended by PROSPERO (registration DOI: 10.17605/OSF.IO/QT2HA, available at: https://osf.io/wg2ty), due to the large turnover time for PROSPERO (∼ 4 months).
Eligibility Criteria
The eligibility criteria were based on study and report characteristics, outlined in Table S 2 (inclusion of articles were based on GWAS with BP traits and/or HTN with African-ancestries). Studies were excluded if they were: 1) not based on the inclusion criteria (Irrelevant articles, i.e., did not include GWAS, African, Human, BP or HTN); 2) published prior to 2007; 3) not based on whole genome arrays (Targeted or Non-genome-wide arrays); 4) not based on conducting a discovery GWAS within the study or focused on environmental interactions (Replication Study Only, Method Only, GxE Only); 4) focused on the effect of antihypertensive medication treatment (Clinical Study); or 5) not an experimental study (Reviews, Non-Journal Articles).
Additional eligibility criteria were included for genetic association data: 1) Meta-analyses across multiple studies (based on multi-ancestries) were included for only African-ancestry data (studies excluded if they were not separated by ethnicity). 2) Secondary studies (based on the same consortium) were included if the trait was different from that described in the primary study (studies excluded if the trait was previously reported by primary study).
Database Search Strategy
PubMed, Scopus and WoS were searched for relevant papers and additional papers were found using GWAS Catalog and PMC, published between January 2007 and April 2020 (search date: April 8, 2020). Publication references, from papers that met the eligibility criteria, were further searched to improve the search strategy.
Pubmed, PMC, Scopus and WoS: Search criteria are shown in Supplementary Table S3: Database search strategy. The query terms consisted of words related to BP and/or HTN, GWAS, genetic association and African-ancestry. MeSH (Medical Subject Headings) terms were searched and included for each keyword. Boolean operators such as AND/OR/NOT were used to string terms together. The initial PubMed search was without filter restrictions. Whereas the Scopus, WoS and PMC searches were further filtered by inclusion of keywords in the abstract, to get papers that were more relevant (Supplementary Table S3).
GWAS Catalog: Papers based on the regular expression “*blood pressure*” and “hypertens*” (trait codes: EFO_0004325, EFO_0000537) were searched in the GWAS database, in the “MAPPED_TRAIT” column. These traits included anything related to BP such as HTN, systolic BP (SBP), diastolic BP (DBP), pulse pressure (PP) and mean-arterial pressure (MAP). The study database was further filtered in “BROAD.ANCESTRAL.CATEGORY” column, using “African*” regular expression, to include only studies with African-ancestry participants. The inclusion criteria for papers within the GWAS catalog can be found at https://www.ebi.ac.uk/gwas/docs/methods/criteria.
Data Collection
Published studies were identified through database searching, against the selection criteria and screened by titles and abstracts. Full articles were obtained for studies that met the inclusion criteria and where inclusion was uncertain. SS recorded reasons for exclusion (full details in Supplementary Table S4: Inclusion/exclusion criteria of the screened records). Screening of the full-text articles, and selected studies in accordance with the inclusion criteria was conducted by SS and checked by RM, BJ, and CA, with final inclusion by consensus.
Data Extraction and Evaluation
Data was extracted from the included studies in the form of summary tables. All authors screened the extracted data and ambiguities were resolved through discussion. Data was evaluated by following the guidelines defined by Sagoo et al. (2009) i.e., assessment by: risk of bias in individual studies and across studies case/trait adjustment definition (such as HTN, anti-hypertensive medication (AHM) and covariant adjustment), population stratification, reporting of methods used (sample size of a study population, genotyping method and its reliability/accuracy, validation of results, statistical analyses). This reduced publication bias within this systematic review (avoiding risk of bias caused by selective reporting).
Summary Measures and Synthesis of Results
The summary of population characteristics and phenotype measures for BP traits for the eligible studies were derived from total population calculations for n and percentage average calculations for mean and SD (Supplementary Table S5). Population characteristics were reported only for African-ancestry. Phenotype measures were reported for BP traits i.e., SBP, DBP, PP and MAP (the effect of continuous variables was measured by beta values) and HTN (the effect of binary variables was measured by odds ratio). BP multi-trait analysis, for the SHet and SHom methods were also reported. The summary of genetic associations was reported for only genome-wide associations (p < 5E-8) (Suggestive associations as per study definition were included in supplementary tables, Supplementary Table S6).
Additional Analyses
The genetic associations were compared with findings in non-African-ancestry studies reported in the GWAS Catalog (under build 37) and further compared with Evangelou et al. (2018) summary statistics data (currently the largest published study with 757,601 European ancestry individuals), to determine which signals were uniquely identified in studies with African-ancestry populations.
Results
Few studies were identified on genetic association of BP and HTN in African-ancestry populations, using the GWAS approach. Therefore, a narrative synthesis of the results was conducted, instead of a meta-analysis.
Selection of Publications for Inclusion
A total of 1,361 papers were identified (PubMed = 330, Scopus = 342, WoS = 534, PMC = 125 and GWAS Catalog = 30, publication references = 0). After removing duplicates, 724 papers remained eligible for screening. After screening by title, abstract and full-text, in cases where it was not clear if inclusion criteria had been met, only 12 papers were considered eligible and retained for the systematic review (Figure 1).
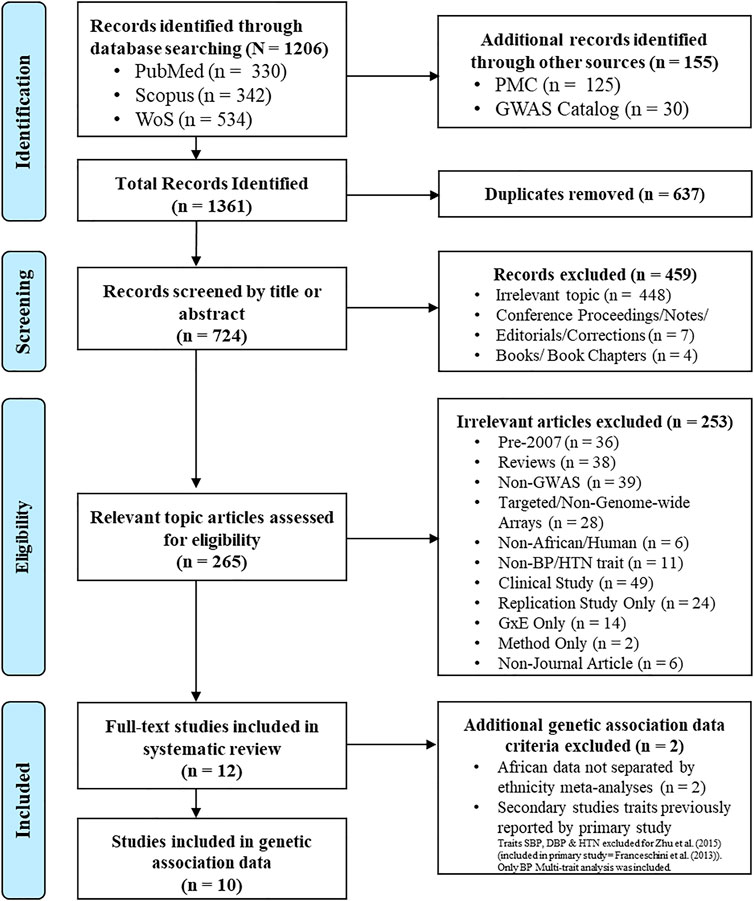
FIGURE 1. Study schema. Study inclusion/exclusion criteria process, based on PRISMA 2009 flow diagram (Moher et al., 2009). Databases: PubMed (https://pubmed.ncbi.nlm.nih.gov), Scopus (http://www.scopus.com), WoS (http://thomsonreuters.com/thomson-reuters-web-of-science), PMC (https://www.ncbi.nlm.nih.gov/pmc) and GWAS Catalog (https://www.ebi.ac.uk/gwas).
The first GWAS with BP and HTN in an African-ancestry population was conducted in 2009 (Adeyemo et al., 2009), with the most recent being conducted in 2020 lines (Willems et al., 2020). Over the years, the number of publications increased (Supplementary Figure S1).
Cohort Characteristics
The African-ancestry population size ranged between 815 (Lule et al., 2019) to 31968 (Liang et al., 2017) individuals (Table 1). Most studies were conducted on AAs (study setting = United States for nine studies) (Table 1). Four studies were from participants living in Africa i.e., Nigeria (Franceschini et al., 2013; Zhu et al., 2015) and Uganda (Gurdasani et al., 2019; Lule et al., 2019). All studies, where age was reported, included only adults (> 18 years), with the exception of Lule et al. (2019), which included 10–11 year old Ugandan adolescents. Meta-analyses were conducted for most studies (Supplementary Table S5), with exception of two (Adeyemo et al., 2009; Lule et al., 2019). Most studies included more women (Supplementary Table S5), with exception of Giri et al. (2019) and Lule et al. (2019) which had 8.5 and 48.7% women respectively.
Eight studies were based on only participants of African-ancestry (Table 1). Of the 4 studies with other ethnicities (Table 1), Wojcik et al. (2019) and Hoffmann et al. (2017) reported ethnicity based GWASs and therefore only the African-ancestry data was reported for this systematic review (34 and 14% African-ancestry, respectively). Giri et al. (2019) and Willems et al. (2020) reported low percentages of African-ancestry within the conducted GWASs i.e., 14% and 18% respectively and the GWAS was based on multiple ethnicities, therefore, these studies were excluded when reporting genetic associations. There were two secondary studies. The Lettre et al. (2011) publication was the secondary study to that reported in Fox et al. (2011), based on the Candidate Gene Association Resource (CARe) consortium. The Zhu et al. (2015) publication was the secondary study of the Franceschini et al. (2013) study and was based on the Continental Origins and Genetic Epidemiology Network (COGENT) consortium.
Phenotype Measurement
From the studies that reported phenotype characteristics (Table 2), three used the automated Omron device and one used a sphygmomanometer to measure BP. From the reported readings, five studies used the average of the second and third recorded readings as the final reading, with the measurements taken with the patient in a seated position. All BP traits were derived from standard calculation, as reported by respective studies. For five of the studies, if a patient was taking AHM, then SBP and DBP was adjusted by adding 15 andand 10 mmHg (three studies) or adding 10 andand 5 mmHg (two studies), respectively. All publications examined SBP and DBP, with the exception of Lettre et al. (2011) who only looked at HTN as a trait (Supplementary Figure S1). SBP and DBP average measures were reported by most studies (Table 2).
Genotyping and Genome-Wide Association Study Parameters
DNA was extracted from blood samples (Giri et al., 2019; Gurdasani et al., 2019), red cell pellets (Lule et al., 2019) and Epstein-Barr virus (EBV) transformed blood lymphocyte cell lines (Willems et al., 2020) (Table 2).
Genotyping were performed using Illumina arrays (four studies), and Affymetrix arrays (eight studies) (Table 3). While the three initial studies were based on 600 K SNP arrays, studies that are more recent used the 1 M (Affymetrix array studies) and 2.5 M (Illumina studies) arrays. All the studies used standard GWAS quality control (QC) parameters. SNP imputation was done for all studies, with the exception of Adeyemo et al. (2009). A range of reference panels was used, with the most common being the 1,000 Genomes Project reference panel (six studies). Merged reference panels were used for six studies. For example, the African Genome Variation Project (AGVP) reference panel was used by both Ugandan studies (Gurdasani et al., 2019; Lule et al., 2019). The final number of SNPs ranged between 808,465 and 30,072,738 SNPs. Population structure was adjusted for in most studies.
For genome-wide association the following tests were performed; 1) Linear regression models (LRMs)/linear mixed models (LMMs) regression, under an additive model, to measure continuous BP traits (i.e., SBP, DBP and PP) and 2) logistic regression models (LoRMs), under an additive model, to measure binary traits (i.e., HTN). All studies adjusted for covariates or confounding variables, with the exception of Zhu et al. (2015). The most common covariates adjusted for in the association testing were age, age2, sex, body mass index (BMI), and first 10 principal components (PCs) of the genotypes. The distributions of association were displayed by means of Manhattan and Q–Q plots for all studies, with most studies (seven) using locus/regional plots. Almost all studies used the standard cutoff for genome-wide significance (p < 5E-8), with exception to Gurdasani et al. (2019) who used p < 5E-9 and Wojcik et al. (2019) who used MAF (minor allele frequency) specific p-value thresholds (p < 5E-8 for MAF >5%; p < 3E-9 for MAF <5%). No suggestive significance was reported in these two studies. Replication of the genome-wide associations was conducted for most studies, with three exceptions (Zhu et al., 2015; Gurdasani et al., 2019; Willems et al., 2020).
Summary of Genetic Associations Detected
The analysis of genetic associations was based on the 10 studies with only African-ancestry participants (Table 4). Liang et al. (2017), based on 31968 African-ancestry individuals (largest), reported the most associations across all BP-related traits (SBP, DBP, HTN, PP and BP multi-trait analysis). The distribution of genome-wide signals across the 22 chromosomes, per trait, is shown in Figure 2. The 26 genome-wide significant SNPs found across BP-related traits, are summarized in Table 5 (suggestive associations are shown in Supplementary Table S6). Six SNPs were associated with more than one BP phenotype from the same study (Figure 3), but none of associations were found in more than one study.
1) Genetic associations detected in each study
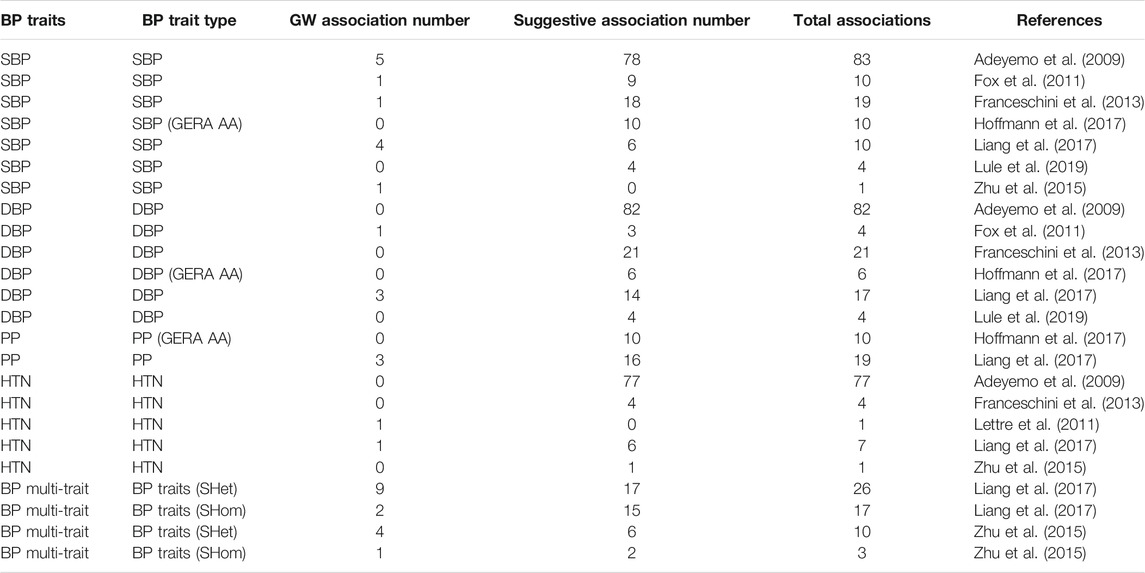
TABLE 4. GWAS associated SNPs detected for BP-related traits in the 10 studies, with 100% African-ancestry participants.
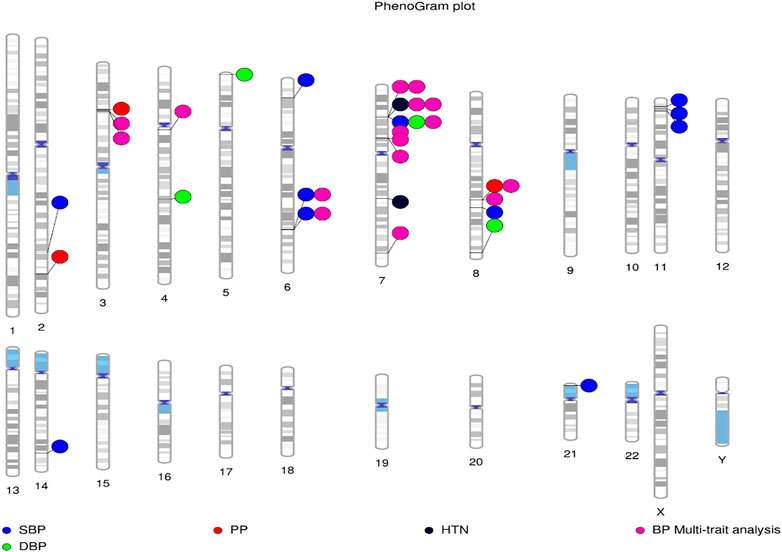
FIGURE 2. Distribution of loci identified by GWAS for BP-associated traits in African-ancestry populations. Lines are plotted on the chromosomes corresponding to the base-pair location of each SNP, and the coloured circles represent the associated phenotype(s). Constructed using PhenoGram (Wolfe et al., 2013).
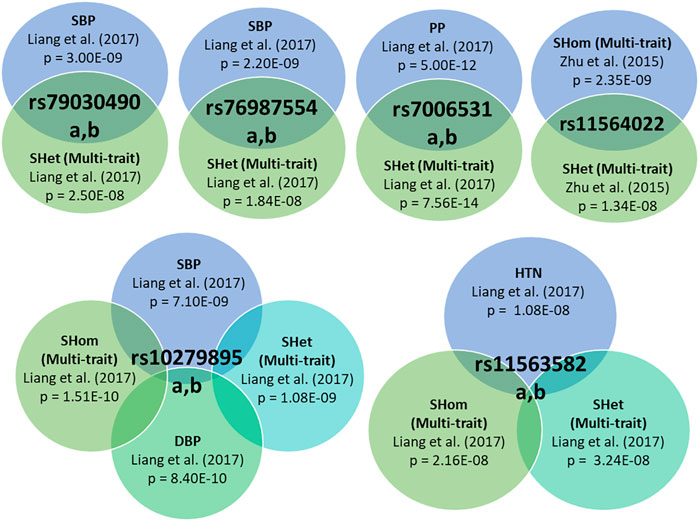
FIGURE 3. Individual variants with associations to multiple BP-related traits, exhibiting pleiotropic effects.
Genome-wide associations were reported for six studies, briefly summarised below.
Adeyemo et al. (2009) reported genome-wide significant associations with SBP for one intergenic SNP (rs16877320 in AL365265.23), and four intronic SNPs (rs5743185 in PMS1, rs17365948 in YWHAZ, rs12279202 in IP O 7 and rs111600591 in SLC24A4). Replication was found in each gene for at least one SNP with low p-values for the Diabetes Genetics Initiative study (Saxena et al., 2007). A non-synonymous-coding SNP in CACNA1H was also discussed in study, however this SNP just missed the significance threshold (p = 6.71E-08) (Supplementary Table S6). Two of these genes (CACNA1H and SLC24A4), have annotations that suggest a role in BP regulation (Adeyemo et al., 2009).
Fox et al. (2011) identified two novel loci i.e., rs2258119 (genotyped SNP in C21orf91) and rs10474346 (imputed in GPR98/ARRDC3) for SBP and DBP respectively. These SNPs did not replicate in the AA population. The SNP rs10474346 is within the region of arrestin C, which is a peroxisome proliferator-activated receptor gamma that may play a role in HTN through modification of inflammation and the innate immunity system in vascular cells. A genotyped SNP (rs1990151) in IP O 7, which showed suggestive significance with p = 7.39E-07 (Table S 6), is within the same importin beta protein gene (IP O 7) and may be a potential link between early-onset glucocorticoid exposure and HTN, through changes in gene expression and kidney function (Fox et al., 2011).
Franceschini et al. (2013) found only one genome-wide associated SNP for SBP i.e., rs11041530 which is 10 kb downstream of the cytochrome b5 reductase 2 gene (CYB5R2 [MIM608342]). In addition, an intergenic SNP rs17428471 (in HOXA3), which showed suggestive significance (p = 4E-07) (Supplementary Table S6), replicated for SBP in additional AA samples (another associated SNP, rs11564022, is also intergenic in HOXA3), and was found to be a trans-ethic SNP since it was found significant for both SBP and DBP in trans-ethnic meta analyses (Franceschini et al., 2013).
Lettre et al. (2011) found one novel genome-wide associated SNP for HTN (rs7801190, in SLC12A9; p = 2.5E-08). SLC12A9 is a potassium/chloride transporter gene. However, this SNP was identified using imputed genotypes (imputation quality r2_hat = 0.70) and replication (also obtained by imputation) was not significant (p = 0.29), suggesting that this association may be due to chance (Lettre et al., 2011).
Liang et al. (2017) is to date the largest African-ancestry GWAS conducted (n = 31968). This study reported 14 independent genome-wide associated SNPs (Table 5). These were spread in 11 independent SNPs at 11 loci, including three novel loci (TARID/TCF21, FRMD3, and LLPH/TMBIM4). This study also pointed out that the African-specific SNPs were not well tagged by HAPMAP2 data (https://medicagohapmap2.org/) which could have limited the association detection in their previous study i.e., Franceschini et al. (2013). The SNPs identified in this study were found in immune, kidney, heart, and vascular system pathways and suggests that several associated genes may be involved in the kidneys renin-angiotensin pathways kidney during HTN (Liang et al., 2017).
Zhu et al. (2015) detected five genome-wide significant loci for the BP multi-trait analyses i.e., SHom detected the HOXA-EVX1 locus, whereas SHet detected the loci CHIC2, HOXA-EVX1, IGFBP1/IGFBP3, and CDH17 (Table 5). Three of the SHet associated loci (CHIC2, HOXA-EVX1, and IGFBP1/IGFBP3) were confirmed to be associated with HTN-related traits. It was suggested that SHet was more powerful compared to combined p-values when accounting for heterogeneity (Zhu et al., 2015).
2) Comparison of genetic associations detected
Six SNPs were pleiotropic (associated with more than one trait) (Figure 3). Liang et al. (2017) identified significant associations with five SNPs of the same polymorphisms for different traits, with most occurring with at least one BP Multi-trait analysis method. Zhu et al. (2015) reported that rs11564022 (HOXA-EVX1) was significant for both BP multi-trait analysis methods (SHet and SHom). This SNP was also found to be suggestively significant in Franceschini et al. (2013) for SBP (p = 1.83E-06), DBP (p = 7.66E-08) and HTN (p = 6.78E-08) (Supplementary Table S6).
The lookup of the 26 genome-wide associated lead SNPs detected in African populations (Table 5), in summary statistics of the largest GWAS to date for BP (Evangelou et al., 2018), found only 14 of 26 SNPs to be present in the study (for SBP, DBP and PP). Of these, 4 SNPs show at least a suggestive p-value (rs7651190b, rs11725861, rs11563582a,b, rs11977526b) in this European ancestry based GWAS (Supplementarty Table S7) and the other ten had p-values ranging from 1.5E-04 to 9.74E-01.
Furthermore, the look up of these 26 SNPs in other BP related GWASs (based on the GWAS Catalog, with a cut-off value p < 5E-6), detected three SNPs (also found in Evangelou et al. (2018), with p < 5E-6) that were associated with BP in four non-African-ancestry based GWASs. All three SNPs were associated in the African-ancestry population with multiple BP traits using the SHet method: rs7651190b (Liang et al., 2017), was found to be associated with PP in Japanese ancestry based GWAS (Takeuchi et al., 2018). rs11725861 (Zhu et al., 2015), was found to be associated with PP in European ancestry based GWAS (Hoffmann et al., 2017). rs11977526 (Zhu et al., 2015; Liang et al., 2017), was found to be associated with SBP in European ancestry based GWAS (Surendran et al., 2016), DBP in another European ancestry based GWAS (Hoffmann et al., 2017) and PP in both European (Hoffmann et al., 2017) and Japanese (Takeuchi et al., 2018) ancestry based GWAS.
After comparing the 26 genome-wide associated SNPs (Table 5) to the GWAS catalog (with a cut-off value p < 5E-6) and Evangelou et al. (2018) summary statistics, only 12 of the SNPs were observed in studies with African-ancestry participants (Table 6).
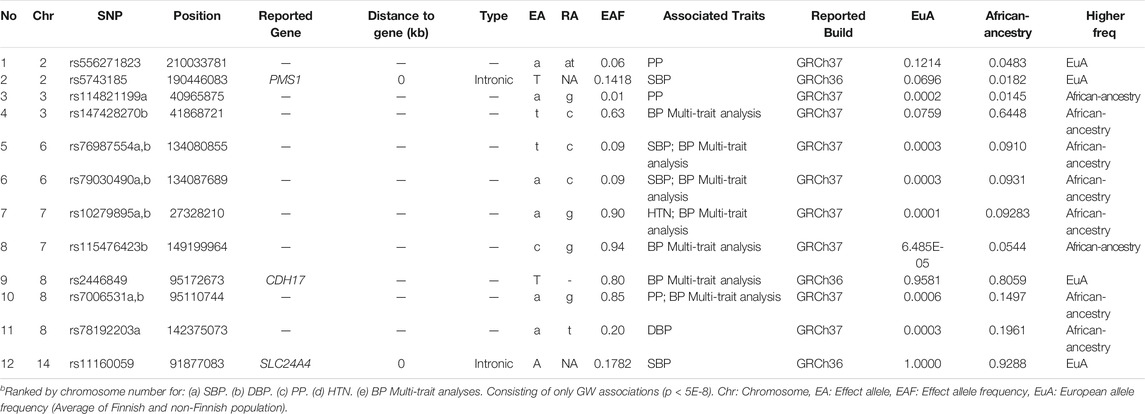
TABLE 6. Potential unique African-ancestry GW associations signals for 10 studies, based on 100% African-ancestry.
Discussion
This systematic review of genetic association studies for BP, HTN and related phenotypes in African-ancestry populations, has highlighted the need for further studies from Africa, where HTN is most prevalent.
Despite the first GWAS with BP and HTN being conducted in 2007 and there being over 160 published studies, there are just a handful of studies on African-ancestry participants and even fewer on continental African populations. It is however encouraging that the number of studies is increasing and that sample sizes are also becoming larger in individual studies, although many remain small (e.g., Willems et al. (2020) had only 281 African-ancestry participants). Large sample sizes, especially in continental African populations are necessary to enhance our understanding of the genetic architecture of BP traits.
Meta-analysis of studies from diverse populations can lead to higher power for detecting associations across multiple populations and can begin to address the transferability of associations across populations (Willems et al., 2020).
Summary of Studies and Associations
The variability between associations found across studies may be partly attributable to differences in study protocols between studies, but could also be due to major differences in sample size and sampling geography. Regional/geographical variability may also play a major role in the differences observed in genetic architecture. Most studies reported mainly on AA participants (Table 1), with African studies limited to Nigeria (Franceschini et al., 2013; Zhu et al., 2015) and Uganda (Gurdasani et al., 2019; Lule et al., 2019), and the origin of eight specified “African-ancestry” studies were not mentioned. African American populations are admixed and according to several studies and the recorded history of the trans-Atlantic slave trade, predominately represent a West African-ancestry, and is limited to specific countries or regions (Tishkoff et al., 2009; Patin et al., 2017). Therefore, genetic associations may differ within groups of AAs due to regional variability in the origins of their African ancestors, with a potential genetic stratification bias which would also be affected by the proportion of European ancestry in different participants and groups (Zakharia et al., 2009). Studies focusing on a selection of other countries within Africa are necessary to capture the ethnic diversity across sub-Saharan Africa and the role of genetic variation in BP related traits (Ramsay et al., 2016; Choudhury et al., 2020).
For studies that provided details on how phenotype data was collected, there was variability in the instrument used and how readings were taken to measure SBP and DBP, and the guidelines used to determine HTN and adjustments made for AHM (Table 2). Variability of BP-related phenotype traits in different populations could influence genetic associations, and may be partly attributed to environmental factors and comorbidities, as well as different age ranges in the participants.
The use of AHM was reported by four studies (Fox et al., 2011; Franceschini et al., 2013; Liang et al., 2017; Giri et al., 2019), however, the methods used for adjustments were not always mentioned. This may introduce bias when making comparisons across studies, as studies that make adjustments would not be comparable to studies that did not make adjustments. Two studies conducted BP multi-trait analyses (Zhu et al., 2015; Liang et al., 2017), using the SHet and SHom approaches. It was reported that these methods gave greater power to detect associations and the SHet method resulted in more associations compared to SHom for both studies. SHet was able to capture evidence for association, even when there was noise caused by heterogeneity among traits and cohorts (Zhu et al., 2015).
Data and statistical models were different in terms of final array sizes (depending on the genotyping array, imputation strategy and reference panel used), GWAS method (depending on adjustments for population structure/PCA (principal components analysis), association model, covariates adjusted for) and thresholds used for determining suggestive significance (Table 3). Adjusting for age, age2, sex and the first 10 PCs, is common practice for most GWASs, however, the reason BMI is used as an adjustment variable for most studies is not mentioned in the studies. This may have to do with the strong associations found between BMI and high BP/HTN (Landi et al., 2018).
Lack of Power to Detect Small Effects
The lack of significant associations found for BP traits from several of the African-ancestry studies is largely due to the low power to detect small effects of common SNPs, and the fact that genotyping arrays are usually Eurocentric and enriched for common European SNPs. Given that sample sizes tend to be smaller in African-ancestry studies, they only have power to detect large effect associations. Therefore, many true associations with small effects will not be significant and many suggestive associations may be spurious. The use of multiple cohorts, to maximize sample size and increase power, could bias findings as some associations may be due to heterogeneity in BP measurements across the different cohorts (Fox et al., 2011).
Many genetic associations found in European studies may not replicate because of the low power of the small studies to detect small effect variants. It is well recognised that BP traits are influenced by many genetic variants, with each conferring a small change in BP, and modulated by environmental factors, behaviour and other physiological markers (Lule et al., 2019). This leads to failure to replicate most associated variants, since many GWAS are only powered to identify common variants of moderate to large effects (Adeyemo et al., 2009; Lettre et al., 2011). Since the African-ancestry samples are from different geographical regions and data is so limited, population admixture may result in different linkage disequilibrium (LD) patterns across African populations, thus limiting replication across different African populations (Fox et al., 2011). Failure to replicate SNPs in Lule et al. (2019) could be due to differences in MAF across the different populations or differences in LD patterns, as well the lack of knowledge of causative variants or due to spurious initial findings.
The Importance of Africa-Centric Arrays, Panels and Africa-Ancestry Studies
GWAS arrays are designed to capture common variation and rely on LD with causative variants to detect association. Most SNP arrays have generally been enriched for common European variants, suggesting that there may be many common African-ancestry SNPs that have not yet been identified as being associated with specific traits (Hoffmann et al., 2017). Interestingly, 14 of the 26 genome-wide SNPs associated in African-ancestry studies were also found in Evangelou et al. (2018) and 12 were potentially unique to African-ancestry populations (Table 6). This could, in part, be due to differences in linkage disequilibrium, genomic coverage of SNPs and allele frequencies that could lead to differences in associations between African and non-African-ancestry groups. African populations have over half a million more SNPs sites per genome in comparison to Europeans, therefore, GWASs performed on this population require more SNPs with a wide genomic coverage to ensure that most LD blocks are covered in the analyses (Ramsay, 2012; Choudhury et al., 2020). The development of Africa-centric arrays are important to detect signals that are unique to African-ancestries. One example of such an array is the H3Africa SNP array, consisting of ∼2.3 million SNPs, including a large proportion of common African variants that effectively tag haplotype blocks in relevant populations and also included disease relevant variants from databases (such as the GWAS Catalog, ClinVar, and Cosmic).
African specific imputation panels are also important to better capture African specific variants. The AGVP reference panel, which includes 320 whole-genome sequences from 320 individuals across sub-Saharan Africa, has an efficient genotype array design capturing common genetic variation in Africa (Gurdasani et al., 2015) and hence this panel was used by both studies Ugandan studies. Moreover, the availability of larger panels, such as the TopMed with substantial African-ancestry representation, is expected to enhance the quality of imputation and thereby increasing the number of variants that could be included in future association studies (Bentley et al., 2020). This might also benefit the meta-analysis with some of the existing GWAS data.
This review emphasizes the value of including African-ancestry studies, as there are BP and HTN signals unique to African populations. Therefore, further studies in this under-represented region are needed to identify more associations and to validate signals that appear to be unique to African-ancestry populations. Since most GWAS that include African-ancestry participants are AA, the results reported here are unlikely to be widely transferable to populations in Africa and emphasize the need for future studies based in continental Africa.
Limitations
There is a limited body of literature on GWAS in populations of African-ancestry, mainly represented by AA. This is well recognized in summaries of GWAS findings and the universal call for more representation of Africans and minority populations in such studies. Variations between population characteristics, phenotypic measures and genotypic analyses could cause bias when comparing different studies. It is therefore important for all studies to report more detail in the protocols to enable better replication and minimise bias between studies. Recent studies suggest that the genetic architecture of BP is different between longitudinal trajectories and cross sectional studies and therefore cohort analyses would be important in African settings. The relatively low overlap between signals detected in African and non-African-ancestry GWASs could be due to LD differences and the same locus being represented by different lead SNPs in the two groups. Although, a regional replication was not performed in the current review, which might have enhanced the overlap, we anticipate that many of the African-specific signals could indeed be continent-specific. Due to these limitations, the results are essentially a list of sentinel findings. A narrative synthesis of the results was conducted (limited to reporting lead SNPs), as a meta-analysis was not possible and additional analyses, such as regional mining or looking at credible sets, were challenging.
Future Directions
Genetic diversity is high within African populations, because of a deep evolutionary history, population admixture and genetic drift. It is therefore vital for future studies to capture contributions from African studies and to understand the functional and biological relevance of associated SNPs. Improved methods need to be developed to understand and compare heritability across populations and studying participants from other parts of the African continent, such as South and Central Africa are essential.
For enhanced discovery in GWAS in Africans, future studies should aim for increased sample size, multiple BP-related traits (including SBP, DBP, HTN, PP, MAP, BP multi-trait analysis and longitudinal data) to enhance discoverability across phenotypes, the use of Africa-centric genotyping arrays and larger imputation reference panels, which adequately represent African-genetic diversity (Hoffmann et al., 2017).
Conclusions
There is much need for more genetic-association studies based on BP and HTN in large-scale African-ancestry research, specifically in participants from the African continent. Further studies should also investigate rare variants, adjust appropriately for the use of anti-hypertensive medication, follow standardised procedures and provide justification for the different approaches used.
Data Availability Statement
The original contribution presented in the study is included in the article/Supplementary Material, further inquiries can be directed to the corresponding authors.
Author Contributions
Screening of the full-text articles, and selected studies in accordance to the inclusion criteria was conducted by SS and checked by MR, J-TB, and AC. All authors screened the extracted data and disagreements were resolved through a discussion. SS drafted, formatted and edited the paper following feedback and edits by MR, J-TB and AC. Critical review and domain knowledge were added by FXG-O. All authors read and approved the submitted version.
Funding
The authors would like to acknowledge the South African Research Chairs Initiative (SARChI), NIH Africa Wits-INDEPTH Partnership for the Genomic Research (AWI-Gen) (Grant number U54HG006938) and NRF for financial support. SS and J-TB were supported by the South African National Research Foundation (NRF) through MR’s South African Research Chair in Genomics and Bioinformatics of African populations hosted by the University of the Witwatersrand, funded by the Department of Science and Technology, and administered by the NRF. AC was supported by NIH grant U54HG006938. The funders played no role in the construction of this systematic review paper.
Conflict of Interest
The authors declare that the research was conducted in the absence of any commercial or financial relationships that could be construed as a potential conflict of interest.
Publisher’s Note
All claims expressed in this article are solely those of the authors and do not necessarily represent those of their affiliated organizations, or those of the publisher, the editors, and the reviewers. Any product that may be evaluated in this article, or claim that may be made by its manufacturer, is not guaranteed or endorsed by the publisher.
Supplementary Material
The Supplementary Material for this article can be found online at: https://www.frontiersin.org/articles/10.3389/fgene.2021.699445/full#supplementary-material
Abbreviations
AA, African-American; AHM, antihypertensive medication; AWI-Gen Africa Wits-INDEPTH Partnership for Genomic Research; BMI, body mass index; BP, blood pressure; CARe, candidate gene association resource; COGENT, continental origins and genetic epidemiology network; DBP, diastolic blood pressure; pressure in the arteries when the heart rests between beats, measured as a continuous trait; GW, genome-wide; GWAS, genome-wide association study; HTN, hypertension; JNC7, guidelines were used i.e., HTN if SBP ≥140 mmHg and/or DBP>= 90 mmHg and/or treatment with AHM, measured as a binary trait; LD, linkage disequilibrium; LMM, linear mixed model; LoRM, logistic regression model; LRM, linear regression model; MAF, minor allele frequency; MAP, mean arterial pressure; sum of DBP and a third of the PP (i.e., DBP +1/3 PP), measured as a continuous trait; PC, principle component; PCA, principle components analysis; PP, pulse pressure; difference between the SBP and DBP (i.e., SBP-DBP), measured as a continuous trait; SBP, systolic blood pressure; pressure in the arteries when the heart beats, measured as continuous trait; SHet extension of SHom and it can increase the statistical power over SHom when a variant affects only a subset of traits; The distribution of SHet under the null hypothesis is obtained through an estimated beta distribution; SHom, Similar to fixed effect meta-analysis method but accounts for the correlation of summary statistics of the multi-traits and for overlapping or related samples among the cohorts; Uses the trait sample size as the weight, so that it is possible to combine traits with different measurement scales; SNP, single nucleotide polymorphism.
References
Adeyemo, A., Gerry, N., Chen, G., Herbert, A., Doumatey, A., Huang, H., et al. (2009). A Genome-wide Association Study of Hypertension and Blood Pressure in African Americans. Plos Genet. 5 (7), e1000564. doi:10.1371/journal.pgen.1000564
Azam, A. B., and Azizan, E. A. B. (2018). Brief Overview of a Decade of Genome-wide Association Studies on Primary Hypertension. Int. J. Endocrinol. 2018. doi:10.1155/2018/7259704
Bentley, A. R., Callier, S. L., and Rotimi, C. N. (2020). Evaluating the Promise of Inclusion of African Ancestry Populations in Genomics. NPJ Genom. Med. 5 (1), 5–9. doi:10.1038/s41525-019-0111-x
Burton, P. R., Clayton, D. G., Cardon, L. R., Craddock, N. J., Deloukas, P., Duncanson, A., et al. (2007). Genome-wide Association Study of 14,000 Cases of Seven Common Diseases and 3,000 Shared Controls. Nature 447 (7145), 661–678. doi:10.1038/nature05911
Choudhury, A., Aron, S., Botigué, L. R., Sengupta, D., Botha, G., Bensellak, T., et al. (2020). High-depth African Genomes Inform Human Migration and Health. Nature 586 (7831), 741–748. doi:10.1038/s41586-020-2859-7
Evangelou, E., Warren, H. R., Mosen-Ansorena, D., Mifsud, B., Pazoki, R., Gao, H. H., et al. (2018). Genetic Analysis of over 1 Million People Identifies 535 New Loci Associated with Blood Pressure Traits. Nat. Genet. 50 (10), 1412–1425. doi:10.1038/s41588-018-0205-x
Forouzanfar, M. H., Afshin, A., Alexander, L. T., Anderson, H. R., Bhutta, Z. A., Biryukov, S., et al. (2016). Global, Regional, and National Comparative Risk Assessment of 79 Behavioural, Environmental and Occupational, and Metabolic Risks or Clusters of Risks, 1990–2015: a Systematic Analysis for the Global Burden of Disease Study 2015. The Lancet 388 (10053), 1659–1724. doi:10.1016/S0140-6736(16)31679-8
Fox, E. R., Young, J. H., Li, Y., Dreisbach, A. W., Keating, B. J., Musani, S. K., et al. (2011). Association of Genetic Variation with Systolic and Diastolic Blood Pressure Among African Americans: the Candidate Gene Association Resource Study. Hum. Mol. Genet. 20 (11), 2273–2284. doi:10.1093/hmg/ddr092
Franceschini, N., Fox, E., Zhang, Z., Edwards, T. L., Nalls, M. A., Sung, Y. J., et al. (2013). Genome-wide Association Analysis of Blood-Pressure Traits in African-Ancestry Individuals Reveals Common Associated Genes in African and Non-african Populations. Am. J. Hum. Genet. 93 (3), 545–554. doi:10.1016/j.ajhg.2013.07.010
Franceschini, N., Carty, C. L., Lu, Y., Tao, R., Sung, Y. J., Manichaikul, A., et al. (2016). Variant Discovery and fine Mapping of Genetic Loci Associated with Blood Pressure Traits in Hispanics and African Americans. PloS one 11 (10), e0164132. doi:10.1371/journal.pone.0164132
Giri, A., Hellwege, J. N., Keaton, J. M., Park, J., Qiu, C., Warren, H. R., et al. (2019). Trans-ethnic Association Study of Blood Pressure Determinants in over 750,000 Individuals. Nat. Genet. 51 (1), 51–62. doi:10.1038/s41588-018-0303-9
Gurdasani, D., Carstensen, T., Fatumo, S., Chen, G., Franklin, C. S., Prado-Martinez, J., et al. (2019). Uganda Genome Resource Enables Insights into Population History and Genomic Discovery in Africa. Cell 179 (4), 984–1002. doi:10.1016/j.cell.2019.10.004
Gurdasani, D., Carstensen, T., Tekola-Ayele, F., Pagani, L., Tachmazidou, I., Hatzikotoulas, K., et al. (2015). The African Genome Variation Project Shapes Medical Genetics in Africa. Nature 517 (7534), 327–332. doi:10.1038/nature13997
Hendry, L. M., Sahibdeen, V., Sahibdeen, V., Choudhury, A., Norris, S. A., Ramsay, M., et al. (2018). Insights into the Genetics of Blood Pressure in Black South African Individuals: the Birth to Twenty Cohort. BMC Med. Genomics 11 (1), 2. doi:10.1186/s12920-018-0321-6
Hoffmann, T. J., Ehret, G. B., Nandakumar, P., Ranatunga, D., Schaefer, C., Kwok, P.-Y., et al. (2017). Genome-wide Association Analyses Using Electronic Health Records Identify New Loci Influencing Blood Pressure Variation. Nat. Genet. 49 (1), 54–64. doi:10.1038/ng.3715
Juma, P. A., Mohamed, S. F., Wisdom, J., Kyobutungi, C., and Oti, S. (2016). Analysis of Non-communicable Disease Prevention Policies in Five Sub-saharan African Countries: Study Protocol. Arch. Public Health 74 (1), 25. doi:10.1186/s13690-016-0137-9
Landi, F., Calvani, R., Picca, A., Tosato, M., Martone, A. M., Ortolani, E., et al. (2018). Body Mass index Is Strongly Associated with Hypertension: Results from the Longevity Check-Up 7+ Study. Nutrients 10 (12), 1976. doi:10.3390/nu10121976
Lettre, G., Palmer, C. D., Young, T., Ejebe, K. G., Allayee, H., Benjamin, E. J., et al. (2011). Genome-wide Association Study of Coronary Heart Disease and its Risk Factors in 8,090 African Americans: the NHLBI CARe Project. Plos Genet. 7 (2), e1001300. doi:10.1371/journal.pgen.1001300
Levy, D., Larson, M. G., Benjamin, E. J., Newton-Cheh, C., Wang, T. J., Hwang, S. J., et al. (2007). Framingham Heart Study 100K Project: Genome-wide Associations for Blood Pressure and Arterial Stiffness. BMC Med. Genet. 1(1), S3.doi:10.1186/1471-2350-8-S1-S3
Liang, J., Le, T. H., Edwards, D. R. V., Tayo, B. O., Gaulton, K. J., Smith, J. A., et al. (2017). Single-trait and Multi-Trait Genome-wide Association Analyses Identify Novel Loci for Blood Pressure in African-Ancestry Populations. Plos Genet. 13 (5), e1006728. doi:10.1371/journal.pgen.1006728
Little, J., Higgins, J., Bray, M., Ioannidis, J., Khoury, M., Manolio, T., et al. (2006). The HuGENet™ HuGE Review Handbook, Ottawa, Ontario, Canada: HuGENet Canada Coordinating Centre.
Lule, S. A., Mentzer, A. J., Namara, B., Muwenzi, A. G., Nassanga, B., Kizito, D., et al. (2019). A Genome‐wide Association and Replication Study of Blood Pressure in Ugandan Early Adolescents. Mol. Genet. Genomic Med. 7 (10), e00950. doi:10.1002/mgg3.950
Manolio, T. A., Collins, F. S., Cox, N. J., Goldstein, D. B., Hindorff, L. A., Hunter, D. J., et al. (2009). Finding the Missing Heritability of Complex Diseases. Nature 461 (7265), 747–753. doi:10.1038/nature08494
Martin, A. R., Teferra, S., Möller, M., Hoal, E. G., and Daly, M. J. (2018). The Critical Needs and Challenges for Genetic Architecture Studies in Africa. Curr. Opin. Genet. Develop. 53, 113–120. doi:10.1016/j.gde.2018.08.005
Moher, D., Liberati, A., Tetzlaff, J., and Altman, D. G. (2009). Preferred Reporting Items for Systematic Reviews and Meta-Analyses: The PRISMA Statement. J. Clin. Epidemiol. 62, 1006–1012. doi:10.1016/j.jclinepi.2009.06.005
Patin, E., Lopez, M., Grollemund, R., Verdu, P., Harmant, C., Quach, H., et al. (2017). Dispersals and Genetic Adaptation of Bantu-speaking Populations in Africa and North America. Science 356 (6337), 543–546. doi:10.1126/science.aal1988
Popejoy, A. B., Ritter, D. I., Crooks, K., Currey, E., Fullerton, S. M., Hindorff, L. A., et al. (2018). The Clinical Imperative for Inclusivity: Race, Ethnicity, and Ancestry (REA) in Genomics. Hum. Mutat. 39 (11), 1713–1720. doi:10.1002/humu.23644
Ramsay, M. (2012). Africa: Continent of Genome Contrasts with Implications for Biomedical Research and Health. FEBS Lett. 586 (586), 2813–2819. doi:10.1016/j.febslet.2012.07.061
Ramsay, M., Crowther, N., Tambo, E., Agongo, G., Baloyi, V., Dikotope, S., et al. (2016). H3Africa AWI-Gen Collaborative Centre: a Resource to Study the Interplay between Genomic and Environmental Risk Factors for Cardiometabolic Diseases in Four Sub-saharan African Countries. Glob. Health Epidemiol. Genom. 1, e20. doi:10.1017/gheg.2016.17
Sagoo, G. S., Little, J., and Higgins, J. P. (2009). Systematic Reviews of Genetic Association Studies. Human Genome Epidemiology Network. Plos Med. 6 (3), e28. doi:10.1371/journal.pmed.1000028
Saxena, R., Saxena, R., Voight, B. F., Lyssenko, V., Burtt, N. P., de Bakker, P. I. W., et al. (2007). Genome-wide Association Analysis Identifies Loci for Type 2 Diabetes and Triglyceride Levels. Science 316 (5829), 1331–1336. doi:10.1126/science.1142358
Surendran, P., Drenos, F., Young, R., Warren, H., Cook, J. P., Manning, A. K., et al. (2016). Trans-ancestry Meta-Analyses Identify Rare and Common Variants Associated with Blood Pressure and Hypertension. Nat. Genet. 48 (10), 1151–1161. doi:10.1038/ng.3654
Takeuchi, F., Akiyama, M., Matoba, N., Katsuya, T., Nakatochi, M., Tabara, Y., et al. (2018). Interethnic Analyses of Blood Pressure Loci in Populations of East Asian and European Descent. Nat. Commun. 9 (1), 5052–5116. doi:10.1038/s41467-018-07345-0
Tam, V., Patel, N., Turcotte, M., Bossé, Y., Paré, G., and Meyre, D. (2019). Benefits and Limitations of Genome-wide Association Studies. Nat. Rev. Genet. 20, 467–484. doi:10.1038/s41576-019-0127-1
Taylor, J. Y., Schwander, K., Kardia, S. L. R., Arnett, D., Liang, J., Hunt, S. C., et al. (2016). A Genome-wide Study of Blood Pressure in African Americans Accounting for Gene-Smoking Interaction. Sci. Rep. 6, 18812. doi:10.1038/srep18812
Tishkoff, S. A., Reed, F. A., Friedlaender, F. R., Ehret, C., Ranciaro, A., Froment, A., et al. (2009). The Genetic Structure and History of Africans and African Americans. Science 324 (5930), 1035–1044. doi:10.1126/science.1172257
Who (2016). Global NCD Target: Reduce High Blood pressureNCD Management-Screening, Diagnosis and Treatment. Geneva, World Health Organization.
Willems, E. L., Wan, J. Y., Norden‐Krichmar, T. M., Edwards, K. L., and Santorico, S. A. (2020). Transethnic Meta‐analysis of Metabolic Syndrome in a Multiethnic Study. Genet. Epidemiol. 44 (1), 16–25. doi:10.1002/gepi.22267
Wojcik, G. L., Graff, M., Nishimura, K. K., Tao, R., Haessler, J., Gignoux, C. R., et al. (2019). Genetic Analyses of Diverse Populations Improves Discovery for Complex Traits. Nature 570 (7762), 514–518. doi:10.1038/s41586-019-1310-4
Wolfe, D., Dudek, S., Ritchie, M. D., and Pendergrass, S. A. (2013). Visualizing Genomic Information across Chromosomes with PhenoGram. BioData Min. 6 (1), 18–12. doi:10.1186/1756-0381-6-18
Zakharia, F., Basu, A., Absher, D., Assimes, T. L., Go, A. S., Hlatky, M. A., et al. (2009). Characterizing the Admixed African Ancestry of African Americans. Genome Biol. 10 (12), R141. doi:10.1186/gb-2009-10-12-r141
Keywords: African-ancestry, blood pressure, genetic association, genome-wide association study, hypertension, systematic review
Citation: Singh S, Brandenburg J-T, Choudhury A, Gómez-Olivé F and Ramsay M (2021) Systematic Review of Genomic Associations with Blood Pressure and Hypertension in Populations with African-Ancestry. Front. Genet. 12:699445. doi: 10.3389/fgene.2021.699445
Received: 23 April 2021; Accepted: 10 September 2021;
Published: 20 October 2021.
Edited by:
Karoline Kuchenbaecker, University College London, United KingdomReviewed by:
Todd L Edwards, Vanderbilt University Medical Center, United StatesTaobo Hu, Peking University People’s Hospital, China
Copyright © 2021 Singh, Brandenburg, Choudhury, Gómez-Olivé and Ramsay. This is an open-access article distributed under the terms of the Creative Commons Attribution License (CC BY). The use, distribution or reproduction in other forums is permitted, provided the original author(s) and the copyright owner(s) are credited and that the original publication in this journal is cited, in accordance with accepted academic practice. No use, distribution or reproduction is permitted which does not comply with these terms.
*Correspondence: S. Singh, c3VyaW5zaW5naEBob3RtYWlsLmNvLnph; M. Ramsay, TWljaGVsZS5SYW1zYXlAd2l0cy5hYy56YQ==