- 1Department of Architecture and Design, Università degli Studi di Genova, Genoa, Italy
- 2Institute of Crop Science, Scuola Superiore Sant’Anna Pisa, Pisa, Italy
- 3Department of Agricultural and Environmental Sciences-Production, Landscape, Agroenergy, Università degli Studi di Milano, Milan, Italy
The planning and design of urban and peri-urban green spaces necessitate careful consideration of various factors, encompassing climatic and agronomic parameters, botanical and eco-physiological characteristics of ornamental plants, and interactions between buildings and green elements. These green areas, along with their composition, play a crucial role in providing essential ecosystem services within urban environments. The process of designing and planning green spaces is a complex, multidisciplinary endeavor, involving professionals such as architects, agronomists, botanists, hydrologists, and civil engineers. The initial steps involve evaluating the area and its location and prioritizing environmental and municipal constraints in the analysis. The selection of plants is heavily influenced by soil and climate parameters. In cases where the area is already green, a meticulous assessment of each plant is essential to determine whether preservation or substitution is more appropriate. Upon completion of the site analysis, the design of the future green area must consider its environmental and social functions, potential ecosystem services, its role within the broader context, compositional aspects, and the judicious use of plant species and materials. This paper proposes a working method that is both multidisciplinary and multiscale, aiming to create sustainable, effective green areas that thrive even in challenging conditions linked to human impact and climate change.
1 Introduction
The selection of plants for building a green urban and peri-urban area (UPUA) is performed considering the aesthetic value of plants and their ability to adapt to suboptimal conditions (Ferrante and Ferrini, 2023). Despite the aesthetic and adaptative values, the maintenance requirements must also be carefully considered, especially if maintenance is under the municipality administration, because the funds are not always guaranteed (Molin and van den Bosch, 2014). The group of experts in charge of planning a new green area must consider the selection of plant species and their planting pattern, the composition of the design, the choice of construction materials, and who furnishes the materials. When designing a new green UPUA, it is also important to consider potential ecosystem services and the functions that it performs in relation to the overall context as well (Pulighe et al., 2016). The reduction of green areas around and in cities is usually associated with the increase of pollution and climate mitigation (Francini et al., 2022).
Urban and peri-urban green planning, also known as urban green infrastructure planning (UGI), is a strategic approach to develop interconnected and multifunctional networks of blue and green structures that potentially provide a wide range of environmental, social, and economic benefits and simultaneously enhance the climate resilience of cities (Hansen et al., 2017; Ramyar et al., 2021; Korkou et al., 2023). The sustainable development of cities and the development of urban spaces are of great importance, not only to maintain nature in the city but also as an urgent need to improve the lifestyle of urban dwellers (Langergraber et al., 2020). The quality of life in cities is influenced positively by a range of significant roles that urban green areas play and provide for its inhabitants (Francini et al., 2023; Nguyen and Orange, 2023). Green areas in the urban context contribute to a larger extent to a sustainable city and the improvement of its environment (Liu and Russo, 2021). Urbanization processes can lead to the development of megacities, and green areas are needed to improve the urban environment in terms of air quality and visual appearance. For example, the city of Milan has a vision for 2030 to be a green, livable, and resilient city (Tancredi and Dell’Osso, 2023). This plan should not generate new volumes compared to the previous 2012 but protects 1.7 million m² from possible urbanization through the downsizing of settlement forecasts and the constraint to the agricultural destination of 3 million m², thus reducing soil consumption by 4%. The city aims to give itself a green footprint, zeroing soil consumption, enhancing its green and blue infrastructures, and increasing its resilience to changes that impact the urban ecosystem (Pulighe et al., 2016). The design of green spaces to increase ecosystem services needs to adapt different scientific disciplines at different ecological and urban scales. This includes single plant interactions with the surrounding environmental matrix or the relationship of the vegetation with the municipality (macroscale), neighborhood (mesoscale), and individual buildings (microscale).
Peri-urban green areas are the landscape interface or ecotone between town and countryside, also known as the rural-urban transition zone, where urban and rural uses and functions mix and often clash. They are recognized as “publicly owned and accessible open spaces within UPUAs that are wholly or partly covered by considerable amounts of vegetation” (Semeraro et al., 2021). These areas are important because they may constitute places in which awareness is raised with regard to the need to apply management models that are more respectful of biological diversity, generating a critical social mass that enables the extension of these models to the green belt of UPUAs. To improve UPUAs, state-of-the-art techniques such as water management techniques or power management techniques should be used to assess and analyze the spatial scale of ecosystem service bundles and their relationship with biodiversity. Green infrastructures in UPUAs have the potential to improve the quality of life of local populations by fostering healthy practices, providing beautiful views, and bringing nature closer to the city (Santo-Tomás Muro et al., 2020; Atanasova et al., 2021). They also contribute to the mitigation of emissions (carbon dioxide, particulate matter, etc.) from other urban activities, thus improving urban sustainability. It is therefore more and more necessary to design UPUAs according to interdisciplinary and multiscale methods, capable of providing a multiplicity of ecosystem services (Chiaffarelli and Vagge, 2023; Dal Borgo et al., 2023; Hanna et al., 2023). The project planning team should include experts such as architects, agronomists, civil engineers, landscapers, hydrologists, and data analysts. All this knowledge is required for planning green areas with beneficial effects on citizens, flora, and fauna in urban environments. The green areas need specific knowledge that correctly provides solutions for engineering problems related to buildings, landscape, and nature.
The aim of this paper is to provide a workflow of the activities that need to be considered for green area designing and planning. Pedoclimatic information, economic, soil, social, water, and environmental impact must be associated with the plant species selected and their maintenance. This paper wants to be a reference or checklist of all parameters that should be considered in a project for urban or peri-urban area design and construction. The scope of this work is to avoid the evaluation of key parameters that are missed during project development. Unfortunately, if some parameters are missed or not considered, it can have a negative impact on the areas in a short or long period.
2 Protocol and procedures for urban and peri-urban green area design
2.1 Location and area determination
The location should be correctly reported using geographical information systems (GIS). Physical and digital maps must be obtained. Old and recent maps can be useful for understanding the area under evaluation and allow for the identification of strengths and limitations. These data must allow the representation of vegetation space, buildings, water channels, rivers, lakes, and any other objects in the geographical space of interest (Burrough et al., 2015). The area should be described and designed with information available in a database that can be linked to the project. Aside from the maps, historical documents and studies should be also collected to understand the changes in the area and foresee the future development of the area of interest.
2.2 Environmental and soil parameters
Weather stations related to the project area were identified and 10 or 20 years of data regarding temperature, solar radiation, rain, and air pollution were downloaded (Clark et al., 2015). These data must be analyzed to identify environmental parameter constraints that will affect the new species that may be planted. Soil samples must be randomly collected in the project area as sampling errors can lead to wrong evaluation (Jenkins et al., 1997; Theocharopoulos et al., 2001). The samples should be taken from the topsoil 30 to 40 cm. These samples should provide the overall soil quality and its suitability for ornamental plants that will be used.
2.3 Evaluation of the health status of plants
Existing plants must be monitored and categorized, and their position in the project area can be determined through geographical position satellite (GPS) coordinates. The evaluation of each plant should include the following:
– morphological structure (visible roots, branches, trunk),
– visual appearance or aesthetic value (leaf color, morphology, damage),
– biotic stresses (diseases, insect damage, etc.), and
– abiotic stress effects (salinity-induced necrosis, heavy metal phytotoxicity, etc.).
2.4 Botanical species selection
The meticulous selection of botanical species in urban and peri-urban planning and design is a pivotal aspect, demanding careful consideration of various factors (Leotta et al., 2023). These factors encompass the local climate, soil conditions, maintenance requirements, and the aesthetic allure of the chosen botanical species. In Italy, an exploration of urban street trees uncovered a rich biodiversity of 277 species, with approximately 60% being exotic (Caneva et al., 2020). The prevalent species included Platanus × hispanica Mill. Ex Münchh., Celtis australis L., and Quercus ilex L. It is imperative to employ a judicious selection criterion that incorporates the adaptability of plant species to local climatic conditions, their aesthetic appeal, and their resilience to abiotic and biotic stresses. Another study utilized the analytic hierarchy process (AHP) method and an expert knowledge approach to effectively select tree species for UPUAs (Li et al., 2021). This method, grounded in both objective data and personal subjective idea, offers adaptability for diverse tree planning opportunities in cities.
Nevertheless, emphasis on botanical species selection for UPUAs should extend beyond mere aesthetic considerations to encompass ecological roles and socioeconomic functions (Velasquez-Camacho et al., 2021). Green UPUAs, for instance, significantly contribute to the sustainability of a city and the enhancement of its environment (Caneva et al., 2020; Nguyen and Orange, 2023). They play a pivotal role in shaping street aesthetics and defining the style of the street landscape (Li et al., 2011). Therefore, the process of selecting botanical species for UPUAs must be a meticulously planned undertaking, factoring in a multitude of considerations to ensure the establishment of sustainable and resilient urban environments. For a correct choice of the botanical species, the main criteria are the following:
- Plants with abiotic stress tolerance—Abiotic stress includes drought or water stress, salinity, heavy metals, and shading. Under drought conditions, plants should be able to reduce transpiration or improve water uptake. At the morphological level, plants should have leathery or waxy leaves (Toscano et al., 2021). At the root level, plants should have a greater biomass that allows a larger exploration of the soil volume and improves water use efficiency. Salinity is a common abiotic stress in coastal areas. In these environments, ornamental species should be selected among the halophytes or plants rich in antioxidants, proline, or other amino acids (Toscano et al., 2021). These compounds can be used as indicators or markers for drought and salinity. In polluted UPUAs with heavy metals, plants should be able to exclude the metals or uptake and translocate the heavy metals from their roots to their aerial parts (Francini et al., 2021). Plants that are able to exclude the heavy metals can survive and do not show phytotoxic symptoms. The area will be restored in terms of ornamental quality, but pollutants will not be removed. Plants that uptake and translocate the heavy metals without showing phytotoxic symptoms can be used for removing these pollutants from the environment. Shading represents a stress induced by low light availability. For shaded areas, plants should be selected considering their shading tolerance which can be measured using leaf gas exchange analysis. Based on the light compensation point, the ornamental plant distribution in the planning area should be performed (Francini et al., 2023). The light compensation point represents the balance between respiration and photosynthesis. Plants should be planted in an area where the net photosynthesis (difference between photosynthesis and respiration) is positive in a 24-h period (Atkin and Tjoelker, 2003).
- Coherence with the ecological and environmental characteristics of the place—These indicators (Ellenberg, 1974; Zolotova et al., 2022) should be considered for the evaluation of vegetation and soil properties, macro- and microclimatic characteristics, exposure, brightness, availability of water, structure, texture and pH of the soil, C/N ratio, etc. A species coherent with ecological conditions will be able to adapt better and faster to the place, will grow stronger and more luxuriant, will be less subject to pathologies and failures, will make abundant blooms, and will bear fruits.
- In UPUAs, resistance to pollution and stress, pollutant abatement, and heat island mitigation—In cities, above all, air pollutants represent a major problem for human health and cause stress for plants. Therefore, in UPUAs, it would be appropriate to select botanical species that are able to resist the stress caused by pollutants, often amplified by water stress during summer. In addition, efficient species can be selected for the assimilation and storage of carbon dioxide (CO2) and the reduction of PM-10 and low volatile organic compound (VOC) emissions, which cause an increase in the concentration of ozone in the lower layers of the atmosphere (Bison et al., 2018). The planting layout will be very important to amplify the efficiency of each individual species and to mitigate heat island and noise pollution.
- Ecological connection, biodiversity implementation, and invasive exotic species control—In the selection of botanical species, the connection of the new green area with the existing neighboring ones should be considered. The creation of a network of green areas is fundamental, especially in UPUAs to create a resilient and efficient network for the provision of ecosystem services. A complex ecosystem is more resilient if rich in species and biodiversity. The botanical species chosen for the project and their planting layouts, therefore, will have to guarantee an increase in biodiversity, and so invasive alien species that tend to simplify ecosystems and depress biodiversity will be avoided (Pyšek et al., 2020). The selection of botanical species that attract pollinators, provide food and shelter for wildlife, and contribute to the overall biodiversity of the area is important. Native plant species are often the best choice as they have co-evolved with the local ecosystem and provide habitat for native wildlife.
- Low maintenance—Both in economic and management terms, the correct choice of botanical species that allow for the reduction of water for irrigation, pruning, substitution of plants that died after transplant, and incidence of pathologies as well as the assessment of available resources, such as staff, equipment, and budget, to determine the maintenance capabilities is important. The selection of botanical species that align with the maintenance capabilities will ensure the long-term health and vitality of the green area.
- Ease of supply in nurseries—This will lower project and management costs and facilitate plant replacements.
- Historical, evocative, and aesthetic value—The selection of botanical species should also take into consideration the plants’ historical, evocative, and aesthetic value, but as mentioned above, it will not be the only or principal criterion. The aesthetic appearance, beauty, and pleasantness of the plants can be enhanced through the design of the project. Consideration of color, texture, form, and seasonal interest of the selected botanical species can create a diverse and visually stimulating landscape throughout the year. Incorporating plants with attractive blooms, vibrant foliage, or interesting texture can enhance the overall aesthetic appeal of the green UPUAs.
3 Discussion
Designing and planning green UPUAs can indeed face several critical challenges in cities. The main critical aspects are represented by the urbanization rate. The increase in urban population is in competition with the space that can be used for buildings or for green areas and their utilization (Jim, 2004). The green area development is also associated with financial resources that are necessary for the green area establishment as well as maintenance. The ornamental value of green areas depends on the level of management that can be performed by private companies or by public institutions such as the municipality (Azadi et al., 2011; Södergren and Palm, 2021). In the latter case, the funds are often limited which can compromise the quality of the green area. Recently, a public institution can give the management of green areas to private companies that use the green space. Traditional land-use planning models may prove inadequate to address contemporary issues in sustainable development and protection governance. To overcome these challenges, it is crucial for city planners and policymakers to prioritize the creation and preservation of urban green areas. Innovative approaches, such as integrating ecosystem services provided via green infrastructure into traditional spatial planning models, have been shown to improve environmental performance (Francini et al., 2023). Sustainable urban drainage systems (SUDS) also represent solutions that use natural processes to handle stormwater more sustainably. Another important issue is the quality of plants bought from the nursery. A healthy plant has a higher success rate in terms of growth and development after transplant. Finally, it must be considered that the green areas provide great benefits to inhabitants and the environment.
3.1 Architectural infrastructure and relationships with green areas
In the design of an open space, the composition of all the landscape elements must be considered and should not just focus on the natural ones. Generally, in a newly designed area, the architectural design precedes that of the composition of the greenery, as first of all there is a functional program to be respected with the creation of paths, play areas, meeting points, etc. However, within a project, it is the coherent integration with the living and/or green components, which determines an overall positive result that meets expectations. A strong character imprinted on the space, clearly respecting its functionality, can contribute to a good result; in some cases, there is a prevalence of artificial elements, and in others, natural ones. In the new district of Porta Nuova, among the open spaces, which exceed a surface area of 16 hectares, the BAM (Biblioteca degli Alberi di Milano) is a park with an innovative design, in which paths of different sizes and materials intertwine with the vegetation creating a chessboard of green rooms, irregular fields, circular forests, flowering meadows, small squares, and equipped areas. In this case, even if there is a prevalence of green elements, the architectural design is very clear. When operating in densely built contexts, the benefits of radical green interventions are almost always numerous and of various types: economic, social, and environmental. An emblematic case is the transformation of the neighborhood around the High Line in New York. Another interesting example is the regeneration project of the open spaces of the brutalist “Barbican Centre” complex in London. Nigel Dunnett manages to create a naturalistic environment immersed in a very built context.
A quality open space is characterized by a synergetic design of natural and artificial elements; its success depends on many factors, including a careful design of architectural details. These can characterize an area, enrich it, and emphasize a theme specific to that place. In the design of an open space, especially if it is public, one must comply with basic regulations (overcoming height differences, cycle and pedestrian paths, playgrounds, car parks), those of the current urban plans, and municipal building regulations. One must also investigate whether there are any green plans and/or detailed plans in the context in which one is operating, especially if one is in a historical center. Working on the Italian territory often implies dealing with the stratification of the ancient fabric, which cannot be neglected, even if there is no specific reference legislation. When intervening, it is also important to establish a relationship with the landscape, especially if of quality.
In this regard, the work of Pietro Porcinai (Matteini, 1991) in the last century is characterized by a continuous search for integration between architectural details, vegetation, and the characteristics of the landscape. In the Tuscan context, terracotta or pietra serena pavements with Mediterranean plants are always present. In the churchyard of the church of Sant’Ambrogio in Zoagli (Genoa, Italy), the waves of white and black pebble paving emphasize the traditional Ligurian material and the proximity to the sea. In a garden of a private villa in Portofino (Genoa, Italy), a pool is built with sandstone paving, typical of Liguria, immersed in a pine forest and a cliff covered with Mediterranean scrub, but in total visual connection with the sea.
So, if in a context with historical and/or landscape peculiarities, it is necessary to emphasize its qualities; in other cases, where it is no longer possible to identify the Genuis loci, it is always convenient to give a new character to the open space, using contemporary themes, such as multiculturalism, recycling of materials, and reuse of water. In this regard, the contemporary interventions such as the Soul of Nørrebro (Burlando and Grillo, 2019) for water reuse and IG’s Superkilen for the enhancement of multiculturalism in a former disused railway area in Copenhagen (Burlando and Grillo, 2019) are interesting.
Materials play an important role in a green UPUA project, showing a direct relationship between architectural design and vegetation choices (Orange et al., 2024). Those used in the local tradition are proven by centuries of cultural familiarity and functional verification of use. This is not to say that new materials should not be introduced; clearly, their technical and performance characteristics must be well studied. One aspect that should never be overlooked when choosing architectural details is their compatibility with the place where they are introduced, the same way for the selection of botanical species. For example, one cannot use stones that crack with thermal variations in locations with large temperature ranges. In the choice of materials, inspiration can be drawn not only from traditional elements but also from the project and/or existing and appropriate vegetation, e.g., the use of corten for the edge of a flower bed if there are flowering plants with bark or red leaves.
3.2 Post-planning green UPUA management
Green UPUA management is a multifaceted endeavor encompassing the strategic planning, development, and meticulous maintenance of green spaces within urban settings. These areas, ranging from parks and gardens to tree-lined streets and other natural havens, play a pivotal role in enhancing the overall wellbeing of urban residents and fostering the sustainability of cities (Aronson et al., 2017; Nguyen and Orange, 2023). Agronomic management practices, such as plant irrigation, judicious application of fertilizers, and precise pruning, are integral components ensuring the vitality of these green spaces (Eriksen-Hamel and Danso, 2010). The efficacy of green UPUA management hinges on seamless collaboration among diverse stakeholders, including local government bodies, community organizations, environmental experts, and landscape architects (Orange et al., 2024). This collective effort is crucial for orchestrating a harmonious balance between the urban environment and its green elements. Ongoing assessment and adaptive strategies are imperative to address the dynamic and evolving needs of urban areas, ensuring the continued efficacy of green area management practices. To streamline and standardize these efforts, the development and provision of a comprehensive guideline manual are paramount. This manual should serve as a foundational resource for private companies or municipalities entrusted with the responsibility of green area management, offering clear directives on best practices, sustainable approaches, and strategies tailored to the unique characteristics of the urban landscape (Hughes and Peterson, 2018). By adhering to such a guideline, those overseeing urban green areas can navigate the complexities of management with efficiency and foresight, ultimately contributing to the creation and maintenance of vibrant and resilient urban ecosystems (Conti et al., 2021).
The green area is not a static element of the landscape but is dynamic and changes with time. Therefore, the evolution of the area considering the plant growth and the socioeconomic development should be considered. The maintenance properly performed can ensure that the area keeps the planned ornamental value. A specific post-planning agreement should be signed between the planners and the municipalities or private companies that will oversee the management of the area.
4 Methods, factors, elements, and criteria for the planning of green areas
To design a green area effectively (both in terms of design, choice of botanical species, functions, and ecosystem services), an in-depth multi-scalar analysis of the context is always necessary according to the scheme presented in Figure 1. Local and extra-local scale analyses allow us to highlight the vulnerabilities and resilience of the area, indicating the general planning strategies and highlighting the role and functions of the project area. An example of this approach is presented in the case study of Città Studi, a neighborhood in the city of Milan, presented in the Supplementary Materials (Supplementary Data Sheet 1). In the following paragraphs, the method applied at the scale of a project to design a green area will be presented: the appropriate analysis, choice of species, and design composition.
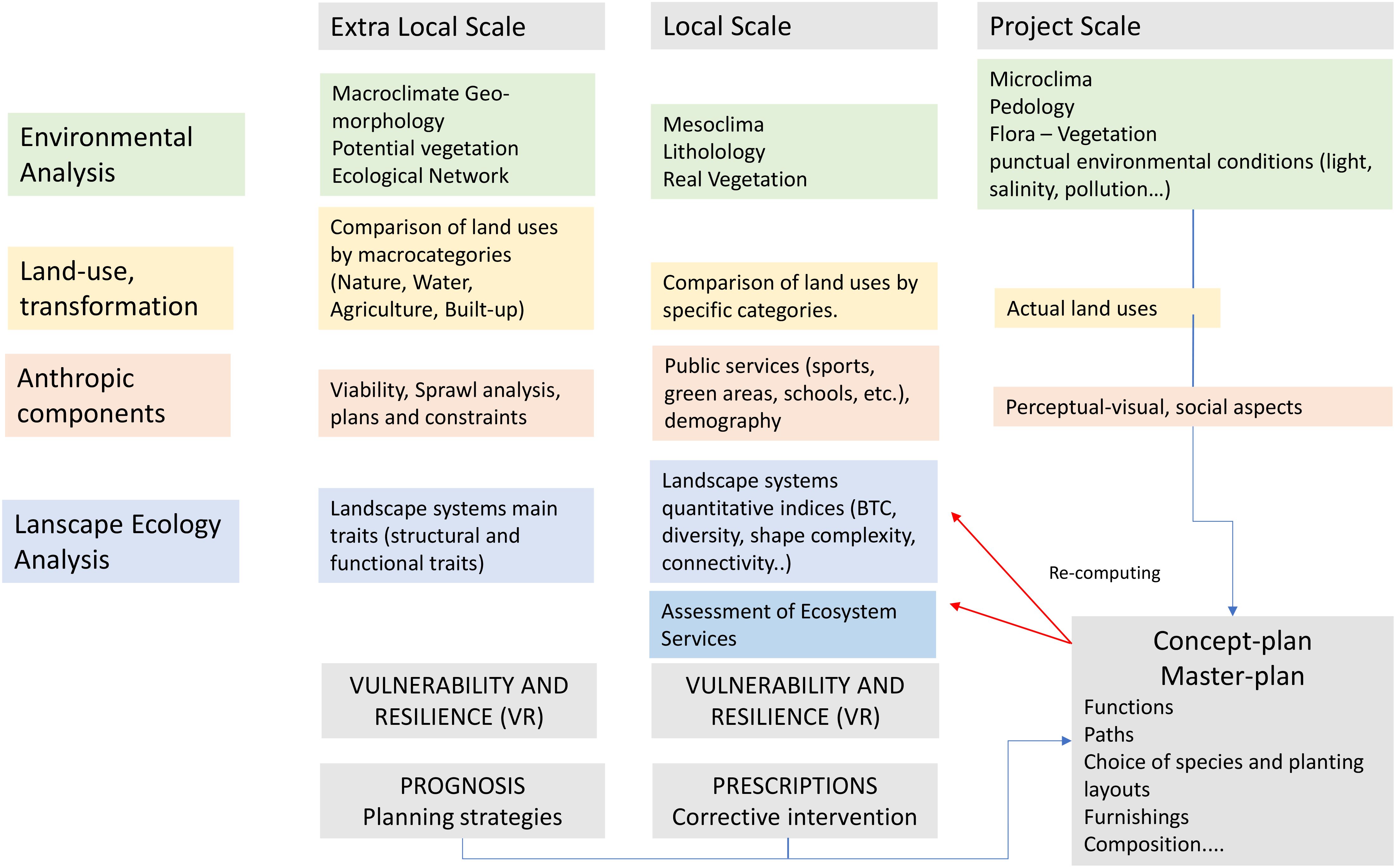
Figure 1. Scheme of the operations that should be considered and followed for urban and landscape design and planning.
4.1 Environmental conditions of the project area
The environmental parameters include temperature, rain intensity and distribution, wind, and snow accumulation. These parameters can be obtained from local weather stations that are closer to the project area. At least 20 years of data should be considered for the project planning and ornamental plant selection.
Temperature: Data should be reported in the project as means together with minimum and maximum values. This information is important for selecting plants that can tolerate suboptimal temperatures in winter and summer. Temperatures are important for plant growth, which will be fast in the optimum temperature range and slower in suboptimal conditions. A historical series evaluation must be performed at the planting site with particular attention to minimum temperatures in colder months and to maximum temperatures in summer months. Based on these intervals, suitable ornamental species can be selected (Tabassum et al., 2023).
Rain intensity and relative humidity of air: Rain intensity and its distribution information can be obtained from weather stations. These data can provide information on wet and dry periods and the potential stresses that ornamental plants can be subjected to. Rain influences the relative humidity of air which regulates the transpiration of plants. High values can increase fungal disease incidence, while low values can cause water stress (Jayamurugan et al., 2013).
Wind: The removal of air from the leaves promotes transpiration, and drought stress is accentuated during water-limited conditions. From a physical point of view, drought stress increases and can encourage the breaking of branches or the uprooting of trees, endangering people and buildings (Francini et al., 2021).
Snow intensity and accumulation: Snow intensity must be considered for its potential danger to cars, pedestrians, and buildings that are under or close to ornamental plants. Snow accumulation on trees depends on their morphological and structural properties.
4.2 Soil chemical and physical properties
Soil fertility and physical properties in the project area must be accurately evaluated. Soil quality affects plant growth and infrastructure stability. Therefore, an appropriate soil analysis must be performed before the planting of ornamental species and the construction of buildings. The most important parameters that must be considered are the pH, salinity, organic matter, soil particle size, structure, and macro- and microelements. The soil’s physical properties refer to the characteristics of the soil related to its physical structure and texture. These properties play a crucial role in determining the suitability of soil for plant adaptability (Toscano et al., 2019).
Soil texture and structure: This parameter refers to particle size, which is classified from largest to smallest as sand, silt, and clay. The relative proportions of these particles can classify the soil as clay, loam, or sand. Sandy soils have larger and coarse particles. Silty soils have medium-sized particles, and finally, clayey soils have smaller and finer particles. Loam soils have a balanced mixture of sand, silt, and clay, providing good fertility and drainage. In an urban environment, the soil is subjected to the activities of different workers such as building contractors, landscape gardeners, etc.; road management activities; and others. The activities of these workers can affect the composition, fertility, and quality of the soil (Huinink, 1998). Soil structure represents the arrangement of soil particles into aggregates or clumps. A good soil structure allows for proper aeration, water drainage, and root penetration. Unfortunately, in urban areas, often, the high traffic or parking close to the plants induces compactness of the soil with a negative effect on plant growth and survival (Mendes et al., 2024).
Understanding and managing these soil physical properties is essential for optimizing agricultural practices, land-use planning, and environmental conservation. Soil testing and analysis are common methods used to assess these properties in the project area.
Soil chemical properties: This information refers to the chemical composition of the soil. These properties play a crucial role in determining the fertility and suitability of the soil for plant growth. Most plants prefer a slightly acidic to neutral pH range for optimal growth. pH regulates nutrient availability, and a lower or higher pH can lead to nutrient deficiency. Soil analysis including pH can help in the determination of fertilizer application. Electrical conductivity (EC) measures the salinity of soils through the electrical conductivity of the soil aqueous extract. High EC levels can indicate salinity issues, which can affect plant growth. Along with soil chemical properties, this information can ensure the growth of plants and the ornamental value of the green area.
Cation exchange capacity (CEC): This measures the ability of the soil to hold and exchange positively charged ions (cations) such as calcium, magnesium, and potassium. Soils with higher CEC generally have better nutrient-holding capacity such as clay soils or soils rich in organic matter. In a specific urban area, the CEC can be increased by the addition of clay or organic matter. The addition of clay has a long-lasting effect, while increasing organic matter is transient unless programmed additions are annually planned.
Soil organic matter: Organic matter in the soil comes from the decomposition of plant and animal residues. It improves soil structure, water retention, nutrient availability, and microbial activity. It is a key component of soil fertility. In an urban environment, the addition of or enrichment with organic matter is quite difficult since soil tillage is not regularly performed.
4.3 Soil and air pollution in the project areas
Pollution can negatively affect plant growth and the ornamental quality of green areas. Therefore, appropriate analysis and monitoring should be taken into consideration before the selection of ornamental plants.
Soil pollution: The most common pollutants of soil in urban or peri-urban areas are represented by heavy metals (mainly Pb, Cu, Zn, Mn, Mo, B, etc.) or organic compounds coming from hydrocarbons (Pierattini et al., 2018).
Air pollution: In UPUAs, pollutants can be particulate matter such as heavy metals, pollens, dust, and ozone (SO2, NO2, PAN, NO, O3).
5 Conclusion
The effectiveness of designing and establishing a green area relies on the integration of numerous environmental elements, encompassing both plants and infrastructure. Plants need to acclimate to diverse soil characteristics, temperature variations, relative humidity levels, and variations in light/shade intensity. Additionally, they must contend with stressors in urban settings, such as pollutants, soil compaction, aridity, floods, and extreme weather events associated with climate change.
Green areas play a vital role in alleviating urban stress and offering a range of ecosystem services. Therefore, an appropriate approach involves a multidisciplinary design and planning framework that places ecology and the environment at its core, incorporating nature-based solutions (NBSs).
Green infrastructure in and around cities has been highlighted as important in the face of climate change effects and plays a major role in providing urban areas with resilience capacity.
The relationship between design and ecology is important, as is the need to include multidisciplinary knowledge and the integration of ecosystem services in planning for the implementation of sustainability in cities.
A successful design and planning of effective UPUAs can be achieved with appropriate educational programs. At the university level, several multidisciplinary courses have been developed to prepare experts with knowledge focused on urban and peri-urban greenery. All disciplines related to agronomy, botany, architecture, hydrology, ecology, and civil engineering are needed, with a focus on planning and the design of green areas and landscape.
Data availability statement
The original contributions presented in the study are included in the article/Supplementary Material. Further inquiries can be directed to the corresponding author.
Author contributions
PB: Writing – original draft. AF: Conceptualization, Writing – original draft. IV: Writing – original draft.
Funding
The author(s) declare that financial support was received for the research and/or publication of this article. This study was funded by the Master’s degree in Green Areas and Landscape Design (https://www.unimi.it/en/education/master-programme/green-areas-and-landscape-design).
Acknowledgments
We would like to thank the Master’s degree students in Green Areas and Landscape Design—Oscar Bassi, Alessandro Cavrioli, Francesco Molteni, Pietro Peretto, Paolo Richelmi, and Matteo Triulzi—for contributing the figures included in the Supplementary Materials. The authors thank the reviewer Dr. Didier Orange, from IRD (Institut de recherche pour le développement) and HysdroSciences Montpellier (HSM) at the University of Montpellier (UM).
Conflict of interest
The authors declare that the research was conducted in the absence of any commercial or financial relationships that could be construed as a potential conflict of interest.
The author(s) declared that they were an editorial board member of Frontiers, at the time of submission. This had no impact on the peer review process and the final decision.
Publisher’s note
All claims expressed in this article are solely those of the authors and do not necessarily represent those of their affiliated organizations, or those of the publisher, the editors and the reviewers. Any product that may be evaluated in this article, or claim that may be made by its manufacturer, is not guaranteed or endorsed by the publisher.
Supplementary material
The Supplementary Material for this article can be found online at: https://www.frontiersin.org/articles/10.3389/fhort.2025.1354764/full#supplementary-material.
References
Aronson M. F., Lepczyk C. A., Evans K. L., Goddard M. A., Lerman S. B., MacIvor J. S., et al. (2017). Biodiversity in the city: key challenges for urban green space management. Front. Ecol. Environ. 15, 189–196. doi: 10.1002/fee.2017.15.issue-4
Atanasova N., Castellar J. A., Pineda-Martos R., Nika C. E., Katsou E., Istenič D., et al. (2021). Nature-based solutions and circularity in cities. Circular Economy Sustainability 1, 319–332. doi: 10.1007/s43615-021-00024-1
Atkin O. K., Tjoelker M. G. (2003). Thermal acclimation and the dynamic response of plant respiration to temperature. Trends Plant Sci. 8, 343–351. doi: 10.1016/S1360-1385(03)00136-5
Azadi H., Ho P., Hafni E., Zarafshani K., Witlox F. (2011). Multi-stakeholder involvement and urban green space performance. J. Environ. Plann. Manage. 54, 785–811. doi: 10.1080/09640568.2010.530513
Bison J. V., Cardoso-Gustavson P., de Moraes R. M., da Silva Pedrosa G., Cruz L. S., Freschi L., et al. (2018). Volatile organic compounds and nitric oxide as responses of a Brazilian tropical species to ozone: the emission profile of young and mature leaves. Environ. Sci. Pollution Res. 25, 3840–3848. doi: 10.1007/s11356-017-0744-1
Burlando P., Grillo S. (2019). “The green protagonist of the contemporary city,” in Di-segnare- ambiente paesaggio città. Ed. Pellegri G.(Genova: Genova University Press), 255–266.
Burrough P. A., McDonnell R. A., Lloyd C. D. (2015). Principles of geographical information systems (USA: Oxford University Press).
Caneva G., Bartoli F., Zappitelli I., Savo V. (2020). Street trees in italian cities: story, biodiversity and integration within the urban environment. Rendiconti Lincei. Sci. Fisiche e Naturali 31, 411–417. doi: 10.1007/s12210-020-00907-9
Chiaffarelli G., Vagge I. (2023). Cities vs countryside: An example of a science-based peri-urban landscape features rehabilitation in Milan (Italy). Urban Forestry urban Greening 86, 128002. doi: 10.1016/j.ufug.2023.128002
Clark L., Majumdar S., Bhattacharjee J., Hanks A. C. (2015). Creating an atmosphere for STEM literacy in the rural south through student-collected weather data. J. Geosci. Educ. 63, 105–115. doi: 10.5408/13-066.1
Conti M. E., Battaglia M., Calabrese M., Simone C. (2021). Fostering sustainable cities through resilience thinking: The role of nature-based solutions (NBSs): Lessons learned from two italian case studies. Sustainability 13, 12875. doi: 10.3390/su132212875
Dal Borgo A. G., Chiaffarelli G., Capocefalo V., Schievano A., Bocchi S., Vagge I. (2023). 2023- Agroforestry as a driver for the provisioning of peri-urban socio-ecological functions: a trans-disciplinary approach. Sustainability 15, 11020. doi: 10.3390/su151411020
Eriksen-Hamel N., Danso G. (2010). Agronomic considerations for urban agriculture in southern cities. Int. J. Agricult. Sustain. 8 (1–2), 86–93. doi: 10.3763/ijas.2009.0452
Ferrante A., Ferrini F. (2023). Floriculture and landscapes: Perspectives and challenges. Front. Horticulture 2, 1123298. doi: 10.3389/fhort.2023.1123298
Francini A., Romano D., Toscano S., Ferrante A. (2022). The contribution of ornamental plants to urban ecosystem services. Earth 3, 1258–1274. doi: 10.3390/earth3040071
Francini A., Toscano S., Ferrante A., Romano D. (2021). Screening and selection of heavy metal tolerant ornamental plants for urban or peri-urban areas. Acta Hortic. 1345, 321–326. doi: 10.17660/ActaHortic.2022.1345.43
Francini A., Toscano S., Ferrante A., Romano D. (2023). Method for selecting ornamental species for different shading intensity in urban green spaces. Front. Plant Sci. 14. doi: 10.3389/fpls.2023.1271341
Hanna E., Bruno D., Comín F. A. (2023). The ecosystem services supplied by urban green infrastructure depend on their naturalness, functionality and imperviousness. Urban Ecosyst. 27, 187–202. doi: 10.1007/s11252-023-01442-9
Hansen R., Rall E., Chapman E., Rolf W., Pauleit S. (Eds.) (2017). Urban green infrastructure planning: A guide for practitioners (GREEN SURGE). Available at: http://greensurge.eu/working-packages/wp5/. (Accessed October 05, 2024).
Hughes S., Peterson J. (2018). Transforming municipal services to transform cities: Understanding the role and influence of the private sector. Sustainability 10 (1), 108. doi: 10.3390/su10010108
Huinink J. T. M. (1998). Soil quality requirements for use in urban environments. Soil tillage Res. 47, 157–162. doi: 10.1016/S0167-1987(98)00087-7
Jayamurugan R., Kumaravel B., Palanivelraja S., Chockalingam M. P. (2013). Influence of temperature, relative humidity and seasonal variability on ambient air quality in a coastal urban area. Int. J. Atmospheric Sci. 264046, 7. doi: 10.1155/2013/264046
Jenkins T. F., Grant C. L., Brar G. S., Thorne P. G., Schumacher P. W., Ranney T. A. (1997). Sampling error associated with collection and analysis of soil samples at TNT-contaminated sites. Field Analytical Chem. Technol. 1, 151–163. doi: 10.1002/(SICI)1520-6521(1997)1:3<151::AID-FACT5>3.0.CO;2-%23
Jim C. Y. (2004). Green-space preservation and allocation for sustainable greening of compact cities. Cities 21, 311–320. doi: 10.1016/j.cities.2004.04.004
Korkou M., Tarigan A. K. M., Hanslin H. M. (2023). The multifunctionality concept in urban green infrastructure planning: A systematic literature review. Urban Forestry Urban Greening 85, 127975. doi: 10.1016/j.ufug.2023.127975
Langergraber G., Pucher B., Simperler L Kisser J., Katsou E., Buehler D., Carmen M., et al. (2020). Implementing nature-based solutions for creating a resourceful circular city. Blue-Green Syst. 2, IWA 173. doi: 10.2166/bgs.2020.933
Leotta L., Toscano S., Ferrante A., Romano D., Francini A. (2023). New strategies to increase the abiotic stress tolerance in woody ornamental plants in mediterranean climate. Plants 12, 2022. doi: 10.3390/plants12102022
Li H., Lin Y., Wang Y., Liu J., Liang S., Guo S., Qiang T. (2021). Multi-criteria analysis of a people-oriented urban pedestrian road system using an integrated fuzzy AHP and DEA approach:a case study in Harbin, China. Symmetry 13 (11), 2214. doi: 10.3390/sym13112214
Li Y. Y., Wang X. R., Huang C. L. (2011). Key street tree species selection in urban areas. Afr. J. Agric. Res. 6, 3539–3550.
Liu O. Y., Russo A. (2021). Assessing the contribution of urban green spaces in green infrastructure strategy planning for urban ecosystem conditions and services. Sustain. Cities Soc. 68, 102772. doi: 10.1016/j.scs.2021.102772
Mendes P., Bourgeois B., Pellerin S., Ziter C. D., Cimon-Morin J., Poulin M. (2024). Linkages between plant functional diversity and soil-based ecosystem services in urban and peri-urban vacant lots. Urban Ecosystems 27, 1011–1026. doi: 10.1007/s11252-023-01470-5
Molin J. F., van den Bosch C. C. K. (2014). Between big ideas and daily realities–the roles and perspectives of Danish municipal green space managers on public involvement in green space maintenance. Urban forestry urban greening 13, 553–561. doi: 10.1016/j.ufug.2014.03.006
Nguyen T. H., Orange D. (2023). Community approach for public flower garden renovation in Hanoi center: perspective for building a green city. Water 15, 2712. doi: 10.3390/w15152712
Orange D., Bousselmi L., Ben Saïd M., Orange M., Nguyen T. H., Gérino M. (2024). “Du rôle de l’architecte dans la promotion de la biodiversité et des SfN pour la Gestion locale des eaux usées en milieu urbain,” in FAAP 2024 - Formation, recherche et projets pratiques en Architecture, Aménagement et Paysage en Asie du Sud-Est: de l’innovation à la structuration(Hanoi (Vietnam). hal-04871090v1.
Pierattini E. C., Francini A., Raffaelli A., Sebastiani L. (2018). Surfactant and heavy metal interaction in poplar: a focus on SDS and Zn uptake. Tree Physiol. 38, 109–118. doi: 10.1093/treephys/tpx155
Pulighe G., Fava F., Lupia F. (2016). Insights and opportunities from mapping ecosystem services of urban green spaces and potentials in planning. Ecosystem Serv. 22, 1–10. doi: 10.1016/j.ecoser.2016.09.004
Pyšek P., Hulme P. E., Simberloff D., Bacher S., Blackburn T. M., Carlton J. T., et al. (2020). Scientists’ warning on invasive alien species. Biol. Rev. 95, 1511–1534.
Ramyar R., Ackerman A., Johnston D. M. (2021). Adapting cities for climate change through urban green infrastructure planning. Cities 117, 103316. doi: 10.1016/j.cities.2021.103316
Santo-Tomás Muro R., Sáenz de Tejada Granados C., Rodríguez Romero E. J. (2020). Green infrastructures in the peri-urban landscape: Exploring local perception of well-being through ‘go-alongs’ and ‘semi-structured interviews’. Sustainability 12, 6836.
Semeraro T., Scarano A., Buccolieri R., Santino A., Aarrevaara E. (2021). Planning of urban green spaces: an ecological perspective on human benefits. Land 10, 105. doi: 10.3390/land10020105
Södergren K., Palm J. (2021). How organization models impact the governing of industrial symbiosis in public wastewater management. An explorative study in Sweden. Water 13, 824. doi: 10.3390/w13060824
Tabassum S., Beaumont L. J., Shabani F., Staas L., Griffiths G., Ossola A., et al. (2023). Which plant where: A plant selection tool for changing urban climates. Arboriculture Urban Forestry (AUF) 49, 190–210. doi: 10.48044/jauf.2023.014
Tancredi G., Dell’Osso R. (2023). Next-Milano. 2015-2030 urban regeneration (Santarcangelo di Romagna (RN: Maggioli Ed.).
Theocharopoulos S. P., Wagner G., Sprengart J., Mohr M. E., Desaules A., Muntau H., et al. (2001). European soil sampling guidelines for soil pollution studies. Sci. total Environ. 264, 51–62. doi: 10.1016/S0048-9697(00)00611-2
Toscano S., Ferrante A., Romano D. (2019). Response of Mediterranean ornamental plants to drought stress. Horticulturae 5, 6. doi: 10.3390/horticulturae5010006
Toscano S., Ferrante A., Romano D., Tribulato A. (2021). Interactive effects of drought and saline aerosol stress on morphological and physiological characteristics of two ornamental shrub species. Horticulturae 7, 517. doi: 10.3390/horticulturae7120517
Velasquez-Camacho L., Cardil A., Mohan M., Etxegarai M., Anzaldi G., de-Miguel S. (2021). Remotely sensed tree characterization in Urban areas: A review. Remote Sens. 13, 4889. doi: 10.3390/rs13234889
Keywords: urban planning, landscape design, plant selection, ecology, landscape architecture, environmental planning
Citation: Burlando P, Ferrante A and Vagge I (2025) Multiscale and multidisciplinary method for plant selection to design green urban and peri-urban areas. Front. Hortic. 4:1354764. doi: 10.3389/fhort.2025.1354764
Received: 12 December 2023; Accepted: 26 March 2025;
Published: 16 April 2025.
Edited by:
Francesco Guarino, University of Salerno, ItalyReviewed by:
José David Flores Félix, University of Salamanca, SpainClaudia Bita-Nicolae, Institute of Biology Bucharest of the Romanian Academy, Romania
Didier Orange, Institut de Recherche Pour le Développement (IRD), France
Copyright © 2025 Burlando, Ferrante and Vagge. This is an open-access article distributed under the terms of the Creative Commons Attribution License (CC BY). The use, distribution or reproduction in other forums is permitted, provided the original author(s) and the copyright owner(s) are credited and that the original publication in this journal is cited, in accordance with accepted academic practice. No use, distribution or reproduction is permitted which does not comply with these terms.
*Correspondence: Antonio Ferrante, YW50b25pby5mZXJyYW50ZUBzYW50YW5uYXBpc2EuaXQ=