- 1Division of Microbiology, Department of Pathology and Microbiology, Nihon University School of Medicine, Tokyo, Japan
- 2Division of Immune Homeostasis, Department of Pathology and Microbiology, Nihon University School of Medicine, Tokyo, Japan
- 3Gabrichevsky Research Institute of Epidemiology and Microbiology, Moscow, Russia
- 4Microbiology and Immunology, Nihon University, School of Dentistry at Matsudo, Chiba, Japan
- 5Department of Food Science, Jumonji University, Saitama, Japan
The female reproductive tract (FRT) and remote/versatile organs in the body share bidirectional communication. In this review, we discuss the framework of the “FRT-organ axes.” Each axis, namely, the vagina-gut axis, uterus-gut axis, ovary-gut axis, vagina-bladder axis, vagina-oral axis, uterus-oral axis, vagina-brain axis, uterus-brain axis, and vagina-joint axis, is comprehensively discussed separately. Each axis could be involved in the pathogenesis of not only gynecological diseases but also diseases occurring apart from the FRT. Although the microbiota is clearly a key player in the FRT-organ axes, more quantitative insight into the homeostasis of the microbiota could be provided by host function measurements rather than current microbe-centric approaches. Therefore, investigation of the FRT-organ axes would provide us with a multicentric approach, including immune, neural, endocrine, and metabolic aspects, for understanding the homeostatic mechanism of women’s bodies. The framework of the FRT-organ axes could also provide insights into finding new therapeutic approaches to maintain women’s health.
Introduction
The gastrointestinal (GI) tract and remote organs in the body share bidirectional communication via immune, neural, endocrine, or metabolic links called the “gut-organ axis” (1). Organs such as the brain, skin, bone, liver, heart, and kidney have been known to communicate multidirectionally with the GI tract, and the microbiota appears to be the key player within these axes. Metabolites produced by the microbiota contribute to the metabolic phenotype of the host and alter the risk of disease (2–4).
The female reproductive tract (FRT) is composed of the lower FRT (vulva, vagina, and ectocervix) and the upper FRT (endocervix, uterus, fallopian tubes, and ovaries). Accumulating evidence has suggested the existence of a vagina-organ axis, such as the vagina-gut axis and vagina-bladder axis (5, 6). Since the vagina is exposed to various pathogens, the physiological condition and microbiota of the lower reproductive tract are crucial for women’s health (7–11). In addition, knowledge of the vagina-organ axis may promote further understanding of not only vaginal health but also the health of other organs apart from the vagina. For example, a nationwide study of American women revealed that dysbiotic conditions in the vagina were significantly associated with periodontitis (12). In addition to the vagina-organ axis, the uterus-organ axis could also be involved in a variety of physiological roles. Recent evidence suggests that the endometrial microbiota appears to be involved in fertility and uterine-related diseases (13, 14). Therefore, modulation of the endometrial microbiota via the uterus-gut axis may play a critical role in fertility (15). In addition, a study showed that short-chain fatty acids (SCFAs) produced by the maternal gut microbiota influenced the metabolic programming of offspring during pregnancy (16), indicating the importance of the uterus-gut axis not only in fertility but also in the development of offspring. Furthermore, recent studies have indicated an interaction between the uterus and brain (17). Overall, the physiological condition of the FRT could be important for the systemic health of women.
In this review, we discuss the framework of the “FRT-organ axes”, i.e., bidirectional communication between the FRT and remote/versatile organs. We provide an overview of each axis, namely, the vagina-gut axis, uterus-gut axis, ovary-gut axis, vagina-bladder axis, vagina-oral axis, uterus-oral axis, vagina-brain axis, uterus-brain axis, and vagina-joint axis. Innate and adaptive immunity regulate the composition of the microbiota and vice versa (18–22). The production of antimicrobial peptides and proteins (AMPs) and mucus is directly triggered via the activation of pattern-recognition receptors, such as Toll-like receptors and NOD-like receptors, by the microbiota, while AMPs and mucus can affect the composition of the microbiota. Immunization with microbes also induces immunoglobulins (Igs), which regulate the composition of the microbiota. In addition to such an immunological route, the neuronal route, the endocrine route, and the metabolic route connect the FRT and distal organs (17). We also discuss potential therapeutic opportunities to restore the microbiota, as a variety of treatments have been tried as approaches to women’s health, and vaginal probiotics seem somewhat promising (17, 18). Finally, future directions in this field are discussed.
Microbiota in the FRT
Although the surface area of the vaginal mucosa is much smaller than that of the gastrointestinal and respiratory mucosa, the presence of a very large number of bacteria, dominated by a single or a few species of bacteria, is characteristic. Investigations using culture-independent techniques have revealed that the human vaginal bacterial community is dominated by Lactobacillus. The vaginal microflora is classified into five community state types: L. crispatus dominant (CST I), L. gasseri dominant (CST II), L. iners dominant (CST III), L. jensenii dominant (CST V), and CST IV, which contains anaerobic bacteria, such as Gardnerella vaginalis and Prevotella bivia (bacterial vaginosis (BV)-associated bacteria), and a relatively low population of Lactobacillus (23, 24). Many factors, such as age, ethnicity, menstrual cycle, lifestyle, immune system, infection, and probiotics, can affect the composition of the vaginal microbiota [for review, see (25–27)].
In contrast to the vaginal microbiota, the composition of the normal endometrial microbiota is still under debate (28, 29). In particular, whether Lactobacillus dominates in the endometrium needs to be clarified (14, 30, 31). A recent study revealed that the directionality of bacterial translocation was from the vagina to the uterus (32). Compared to that in the vagina, the total amount of bacteria decreases by more than three orders of magnitude in the endometrium (median fold change in Cq value was 1.75), which was previously thought to be sterile (31, 33). Therefore, possible contamination of vaginal microbiota during sampling may disturb the results of the investigation of endometrial microbiota, especially in the detection of dominant species. Although the uterine endometrium is even smaller and contains very few bacteria in absolute terms, it appears to play an important biological role in nurturing fetuses. For example, L. crispatus significantly promoted invasion of the trophoblast cell line (34). Low amounts of Fusobacterium nucleatum also promoted the invasion of trophoblast cells and induced the secretion of mediators for pregnancy establishment (35). Further studies in this field are strongly needed to establish adequate reproductive medicine. Although the placenta has been reported to harbor a microbiota similar to that of the oral cavity (36), some studies have questioned the existence of the placental microbiota, especially in pregnant women at term (37). A recent systematic review using structured quality bias assessment concluded that the existence of a low biomass placental microbiota in healthy pregnancies cannot be disproved by current evidence (38). Compared with the vagina, the fallopian tube has microbiota with higher diversity (Acinetobacter, Burkholderia, Comamonas, Coprococcus, Corynebacterium, Enterococcus, Lactobacillus, Prevotella, Propionibacterium, Pseudomonas, Staphylococcus) (33, 39, 40). However, little is known about the roles of the microbiota in the fallopian tube.
In addition to the bacteriome, the virome, mycobiome, archaeome and candidate phyla radiation also consist of the microbiome of FRT (30, 41–43). Trichomonas vaginalis, the causative agent of trichomoniasis, is also a well-known protist parasite in the vagina (44). The virome in FRT, especially bacteriophages, appears to be a potential player in the establishment and maintenance of vaginal dysbiosis (45). However, little is known about the role of these biomes in FRT-organ axes.
Anatomical overview of the FRT-organ axes
Anatomically proximal and distal interactions comprise the FRT-organ axes (5) (Figure 1). The openings of the urethra and vagina are in close proximity to each other. Therefore, some microbial species can be transient members of the microbiota between the bladder and the vagina (vagina-bladder axis). Although the vagina-gut axis is characterized by distal interactions (5), the rectum is relatively close to the vagina. Bacteria can also directly migrate from the anus to the vagina and urethra (5, 46). In addition to bacteria, fungi such as Candida albicans may migrate between the anus and the vagina (47). Furthermore, recent studies indicate that the interaction between the microbiota and immunity in the small intestine is different from that in the colon (48, 49), suggesting that we may separate the vagina-gut axis into the vagina-small intestine axis, the vagina-colon axis, and the vagina-rectum axis. In this review, however, we still use the phrase “vagina-gut axis” because few studies have analyzed the microbiota and its effects on immunity in the small intestine. Some studies have also reported distal interactions of the FRT with the ovary, oral cavity, brain, and joints. For example, bacterial translocation could occur from the oral cavity or gut via the bloodstream to the uterus (5, 28, 50). Microbial translocation also occurs during sexual intercourse, and some urogenital microorganisms are shared with sexual partners, which is beyond the scope of this review [for review, see (5, 51)]. Below, we describe each axis in detail.
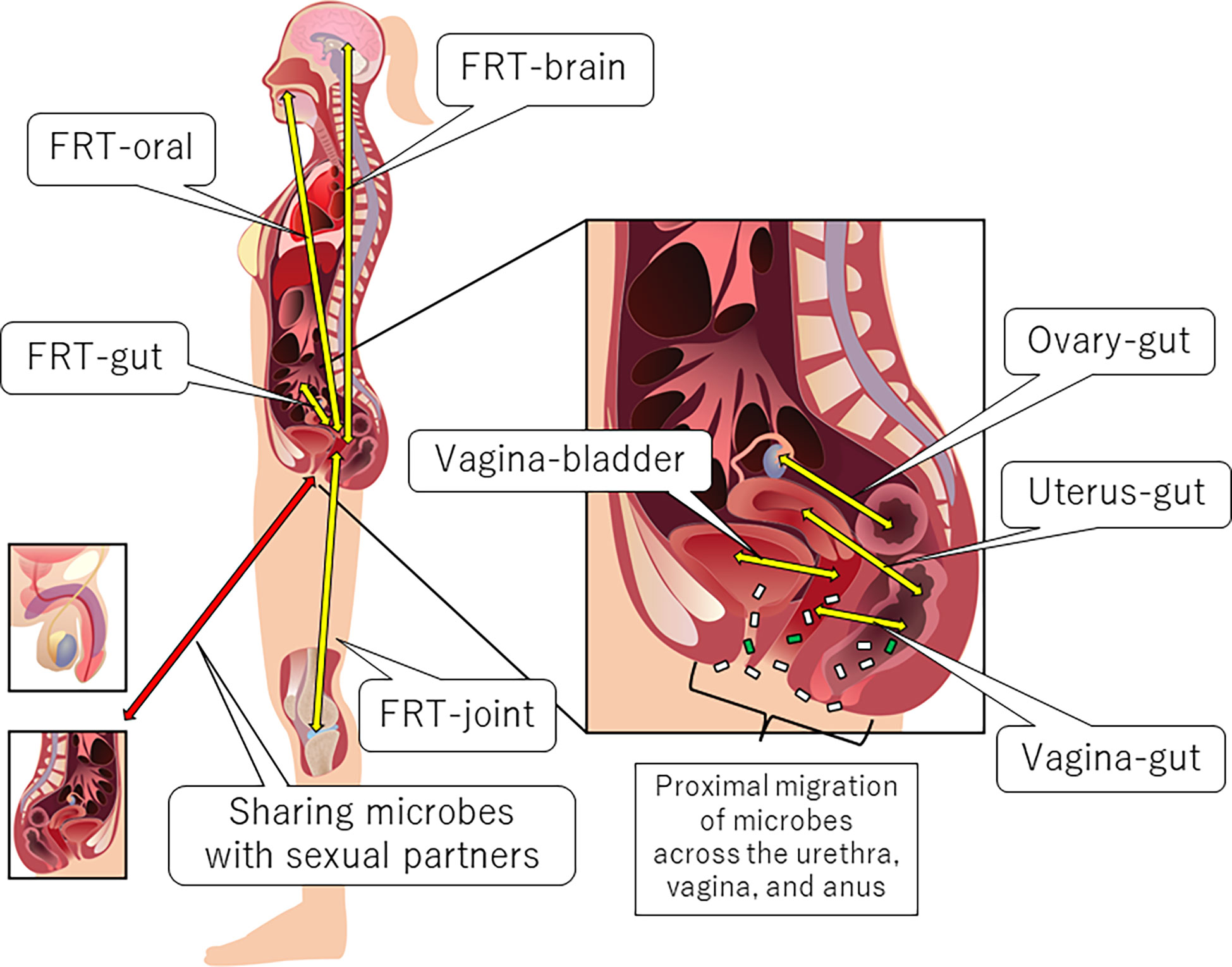
Figure 1 Anatomical overview of the FRT-organ axes. Each axis can be characterized by proximal or distal interactions. Trafficking of bacterial species is a unique aspect of proximal axes compared to other distal axes. However, distal bacterial translocation might occur systemically via the bloodstream, especially in pathological conditions accompanied by the dysregulation of epithelial barrier function.
Vagina-gut axis
Among the FRT-organ axes, the vagina-gut axis is the most studied and discussed. Reducing the risk of BV (dysbiotic condition of the vaginal microbiota) by a healthy diet (52, 53) has enhanced the importance of the interaction between the GI tract and the vagina. One of the oldest descriptions of the term “gut-vagina axis” was referred to by Ravel and Brotman in 2016 (54). Trafficking of bacterial species across the vagina and gut, as mentioned above, is a unique aspect of proximal axes compared to other distal axes. Some Lactobacillus strains are found in both the rectum and the vagina (55). In a previous study, 63 bacterial species were identified in the vagina or rectum, 44% of which were found in both organs, and the genotypes of 68% of the species were identical (56). A recent study showed that BV-associated bacteria in the vagina and rectum in pregnant women were individually detected by species-level Spearman correlation coefficient analysis (57). Furthermore, orally administered probiotic strains were also detected in the vagina (58–61). These observations suggest that the rectum could preserve vaginal bacteria (15, 57) and, possibly, vice versa. Importantly, a genomic study of L. crispatus and L. rhamnosus from a variety of sources, including the human gut and vagina, suggested body site-specific adaptation (62, 63). L. crispatus and L. gasseri from the reproductive tract and GI tract exhibited source-dependent gene expression and phenotypic characteristics, such as growth rates, stress resistance, adhesion properties, and fermentation profiles, in addition to strain-dependent differences (64). Hence, the preference of Lactobacillus for each organ should be considered when discussing the sharing of Lactobacillus by both the rectum and the vagina, although further research is needed.
In addition to direct bacterial translocation, indirect interaction between the vagina and gut has also been implicated. Metabolites produced by the microbiota, such as SCFAs, could be considered indirect players of the vagina-gut axis. The roles of SCFAs are prominently different between the vagina and gut [for review, see (15)]. SCFAs in the gut exhibit beneficial functions, such as maintenance of barrier functions, while those in the vagina are associated with dysbiotic and proinflammatory conditions (65). SCFAs can also influence immune cell subsets and their functions (66). As SCFAs produced by the gut microbiota can be transferred to other organs via systemic circulation (67), SCFAs may be involved in the vagina-gut axis. SCFAs produced by vaginal microbes are thought to contribute to the development of a dysbiotic environment (65). An in vitro study showed that excessive SCFAs can be a potential source of cervicovaginal inflammation (68). Therefore, the circulation of SCFAs from the gut to the vagina might cause vaginal dysbiosis.
In addition to metabolites, sex hormones contribute to the vagina-gut axis. The vaginal microbial community can be influenced by an estrobolome-mediated mechanism [for review, see (5, 69, 70)]. Briefly, the estrobolome is defined as the collection of microorganisms that can metabolize estrogens. For example, Bifidobacterium, Clostridium, and Lactobacillus are involved in the deconjugation/conjugation of estrogens (71, 72). The estrobolome can deconjugate hepatically conjugated estrogens in the GI tract. Deconjugated estrogen is then reabsorbed to the systemic circulation. Circulating estrogen reaches the distal epithelium of the vagina and alters the physiological characteristics of vaginal epithelial cells, such as glycogen and mucus production. Increased glycogen supports Lactobacillus dominance in the vagina, as glycogen can serve as an important energy source for lactobacilli (73, 74). Thus, the amount of estrogen-metabolizing bacteria in the gut microbiota can affect the amount of Lactobacillus in the vaginal microbiota. The proposed mechanisms of vaginal microbiota shaping via the vagina-gut axis are shown in Figure 2.
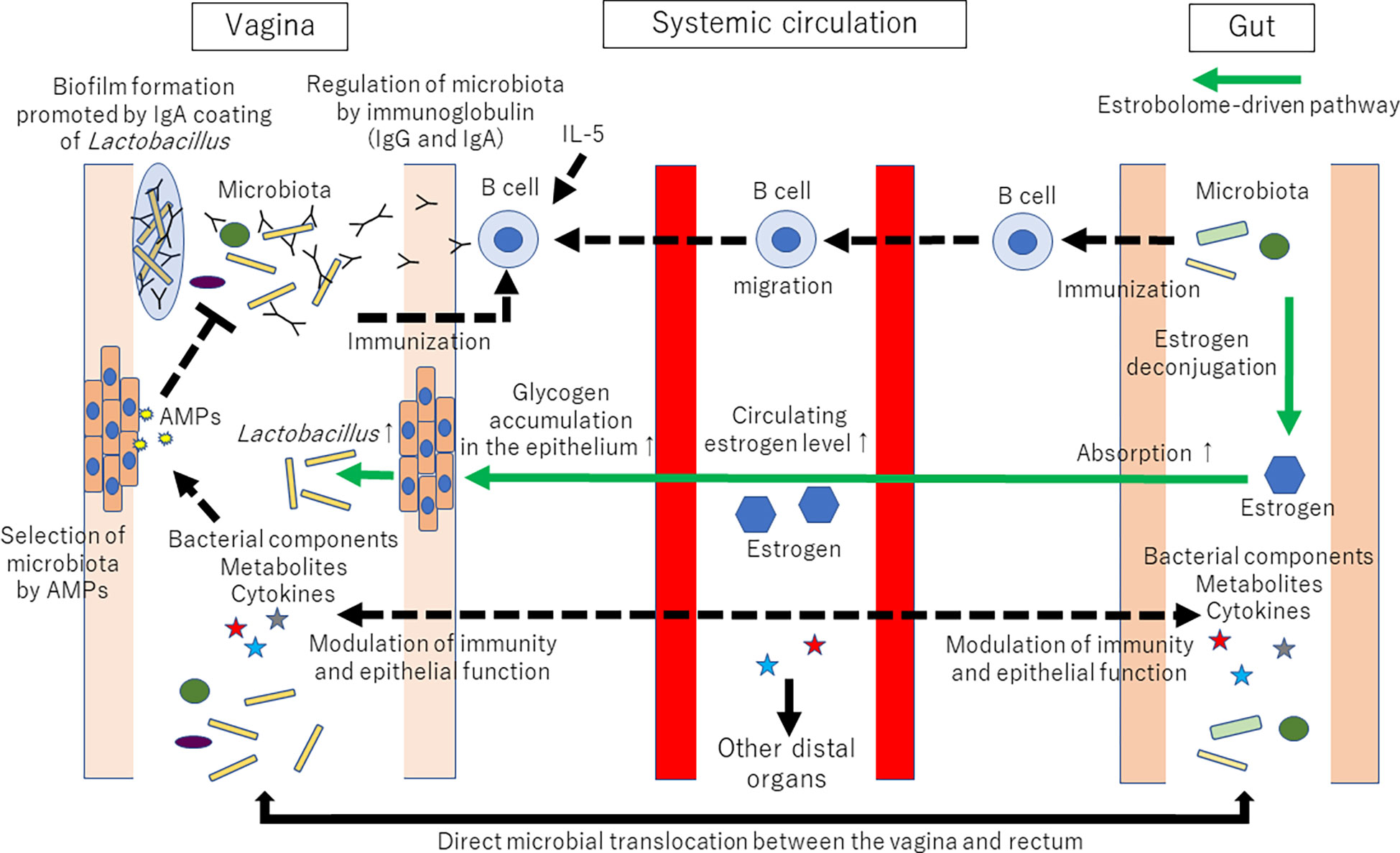
Figure 2 Proposed mechanisms of vaginal microbiota shaping via the vagina-gut axis. Many factors, such as age, ethnicity, menstrual cycle, lifestyle, immune system, infection, and probiotics, are known to affect the composition of the vaginal microbiota. However, why the human vaginal microbiota is often dominated by a single or a few strains of Lactobacillus is still unknown. Tolerance and promotion of Lactobacillus dominance in the vagina might be regulated via the vagina-gut axis. Immunization with Lactobacillus might occur both in the vagina and the gut. The collection of microorganisms that can metabolize estrogens (coined the estrobolome) can deconjugate hepatically conjugated estrogens in the gastrointestinal tract. Deconjugated estrogen is then reabsorbed to the systemic circulation. Circulating estrogen will affect the distal epithelia of the vagina, resulting in glycogen and mucus production. Then, increased glycogen supports Lactobacillus dominance in the vagina because glycogen can serve as an energy source for lactobacilli. AMPs: antimicrobial peptides and proteins. The established pathways are represented by solid lines. The proposed mechanisms are represented by dotted lines. The estrobolome-driven pathway is represented by green lines.
One of the most important immunological roles of the vagina-gut axis is to shape the components of the vaginal microbiota. Some factors, such as AMPs secreted from epithelial or immune cells from the mucosal site of hosts, regulate the microbiota and vice versa (21, 26). IgA also regulates the composition of the gut microbiota (18–20, 22). IgA is the principal antibody class secreted in mucosal fluid (75, 76). The origin of most IgA in the gut is as a B-cell response to commensal bacteria (77). However, unlike other mucosal tissues, the IgA level is lower than the IgG level (78, 79), and IgG instead dominates antigen-specific antibodies in the vagina (80). This difference can indicate a different defensive function between the vagina and other mucosal tissues (81). IgA and IgG appear to be stably associated with cervical mucus, while only IgG appears to be associated with cervicovaginal mucus (82). IgA-Seq (bacterial flow cytometry with 16S rRNA gene sequencing) revealed that lactobacilli were coated with IgA in vivo (83). In contrast, most bacteria in the Firmicutes phylum were not coated with IgA (83). Of note, Breedveld and colleagues recently reported that higher levels of bacterial IgA coating were observed when the vaginal microbiota was dominated by L. crispatus compared with non-L. crispatus-dominated microbiota (84). The bacterial IgA coating might modulate biogeography, metabolic processes, proliferation, and survival in mucosal tissue by modifying bacterial gene expression (85, 86). IgA coating is proposed to promote adhesion to the mucus layer and facilitate the formation of bacterial biofilms on the mucosal surface, which is important for bacterial colonization in the gut (87). Therefore, similar to its regulatory function in the GI tract, IgA could play a role in maintaining the L. crispatus-dominated microbiota (84). A previous study showed that genetic variants of IL-5 were associated with the abundance of Prevotella spp. in the vaginal microbiota (88). As IL-5 is implicated in IgA responses (89), host genetics could affect the vaginal microbiota via immunological regulation. Furthermore, competition among Lactobacillus species of the vaginal microbiota appears to be less likely to occur (90, 91). These studies might support the importance of host factors, rather than bacterial competition of lactobacilli, in shaping the Lactobacillus-dominant vaginal microbiota (91).
Furthermore, questions arise about the immunization of vaginal Lactobacillus, specifically when and where immunoglobulin binding to Lactobacillus could be induced. The components of the vaginal microbiota dramatically change throughout a woman’s life (15, 69). Similar to a mother’s vaginal microbiota, the meconium of newborns delivered vaginally contains microbes such as Lactobacillus and Prevotella (92), suggesting that one of the sources of the infant’s gut microbiota would be the mother’s vaginal microbiota. Interestingly, L. crispatus from the mother’s vagina might play an important role in establishing the mucosal barrier function of the infant gut (93). In contrast, after birth and during childhood, the vaginal microbiota does not resemble that of the mother and contains streptococci, enterococci, and anaerobes with high microbial diversity, and Lactobacillus is deficient (94). Therefore, the immunization of Lactobacillus, which will be the dominant vaginal species, is less likely to occur in the vagina before puberty. Instead, as the rectum could preserve vaginal bacteria (15), immunization of vaginal Lactobacillus after puberty might occur in the gut before puberty. After puberty, with an increase in circulating estrogen levels and accumulation of glycogen in the thick vaginal epithelium as an energy source for Lactobacillus (73, 74), the vaginal microbiota is dominated by one or a few Lactobacillus species. After menopause, the estrogen level decreases, resulting in a reduction in Lactobacillus abundance.
After puberty, immunization with Lactobacillus might also occur in the vagina, in addition to the gut. Although the importance of the local immune structures in the vagina is still under investigation (95), the vaginal microbiota can stimulate the mucosa continuously. Therefore, B cells that respond to vaginal commensal organisms might be recruited to the vaginal mucosa, and IgG and IgA secreted from these B cells might have a role in shaping the microbiota in the vagina. Furthermore, L. crispatus might have immunomodulatory capabilities mediated by the S-layer protein, which comprises the outer surface layer of the bacteria (96). In addition, S-layer protein was reported to be a mediator of Lactobacillus adhesion to epithelial cells (93, 96, 97).
Although the mechanism of immunizing with Lactobacillus as a component of the vaginal microbiota is uncertain, investigations of genital mucosal antibodies after vaginal challenge with pathogens have provided some insights. In mice, after vaginal infection immunization with herpes simplex virus 2 (HSV-2), circulating antibodies rarely entered the vaginal lumen, and this primary infection did not recruit plasma cells in the vaginal mucosa (98). However, after secondary vaginal challenge with HSV-2, induction of CXCL9 and CXCL10 by IFN-γ from tissue-resident memory T cells in the vagina recruited circulating memory B cells to the vaginal mucosa in a CXCR-3-dependent manner, and the B cells secreted virus-specific IgG and IgA into the vaginal lumen (98). In macaques, two vaginal challenges and three oral Simian immunodeficiency virus challenges induced virus-specific IgA and IgG antibodies in the vaginal fluid and serum (99). This vaginal IgA had a J chain and a secretory component, indicating that it was of secretory origin (99). In humans, vaginal vaccination with the cholera toxin B subunit increased the concentrations of IgG and IgA in cervical secretions, while nasal vaccination showed less induction of IgG and IgA (100). In contrast, more induction in vaginal IgA secretions was observed when the antigen was administered nasally (100). To date, however, a mechanism for establishing a vaginal microbiota has not been developed.
The vagina-gut axis appears to be involved in disease development. The presence of G. vaginalis in the gut microbiota significantly increased the odds of irritable bowel syndrome (IBS) (101). Gardnerella was significantly more enriched in the gut microbiota of patients with ulcerative colitis than in patients with Crohn’s disease (102). These results may represent translocation or contamination of bacteria from the vagina before or during sampling. However, a significant link between inflammatory bowel disease (IBD) and the urogenital biofilm of G. vaginalis in urine specimens suggested dysfunction of the vaginal epithelial barrier (103). Recent studies have also indicated the association of vaginal dysbiosis and IBD in pregnant women. Pregnant women with IBD had a risk for BV and Candida colonization (104). Although the causal relationship was unknown, the prevalence of Mycoplasma and/or Ureaplasma was also significantly higher in pregnant women with IBD than in pregnant women without IBD (105).
The link between BV and IBD might also be explained by lipopolysaccharide (LPS), a component of the membrane of gram-negative bacteria. As a consequence of the downregulation of intestinal barrier integrity induced by gut dysbiosis accompanied by IBD, LPS can translocate into the systemic circulation (106, 107), causing acute or chronic inflammation at distal sites (108). Although there is no evidence showing that vaginal dysbiosis could increase circulating LPS, LPS from the gut might cause/worsen inflammation at the vagina.
Furthermore, infection of G. vaginalis in murine vaginas increased the concentration of TNF-α and myeloperoxidase activity and decreased the concentration of IL-10 in colon tissue (109). This infection also decreased the Bacteroidetes composition and increased the Proteobacteria composition in the gut microbiota (109). Overall, vaginal dysbiosis might be a trigger/aggravating factor of these bowel diseases and perhaps vice versa.
An association between the gut microbiota and cervical cancer has also been suggested. Altered diversity and composition of the gut microbiota of patients with cervical cancer have been reported (110). SCFAs inhibited the proliferation of HeLa cells (human cervical cancer cell line) via the downregulation of free fatty acid receptor 2 expression (111). Therefore, such metabolites produced by the gut microbiota could indirectly affect the progression of cervical cancer (72). Oral lactic acid bacteria (LAB)-based mucosal human papillomavirus (HPV) vaccines may influence the course of cervical cancer (persistent infection with HPV is associated with oncogenesis of cervical cancer) through the flow of immune signals from the gut to the vagina (112). In addition, a meta-analysis showed a causal link between cervical cancer and vaginal dysbiosis (113). Therefore, another indirect link between cervical cancer and the gut microbiota can be postulated if vaginal dysbiosis is induced via the vagina-gut axis.
To consider the mechanism of the vagina-gut axis, one suggestive example is Helicobacter infection in the stomach. H. pylori is highly virulent in the stomach (114), and some studies have shown an inverse correlation between H. pylori infection and IBD onset, indicating that H. pylori could induce systematic immune tolerance and the suppression of the inflammatory response (115). In contrast, recurrent BV can be cured after antibiotic therapy for H. pylori (116). Although antibiotic therapy for H. pylori can directly change the vaginal flora, H. pylori-induced systematic immune tolerance might promote colonization of pathogens in the vagina. Therefore, these studies might represent a double-edged sword of gastric H. pylori infection to modulate systemic mucosal immune modulation, including the vagina-gut axis.
Uterus-gut axis
The uterus has unique immune regulation (117, 118). However, how uterine immunity and its microbiota interact with each other requires further investigation. The hematogenous spread of bacteria from the gut to the uterus has been reported (28). Recently, an association between the gut microbiota and preterm birth has been highlighted. Reductions in Bifidobacterium, Clostridium, and Bacteroides have been observed in the gut microbiota of patients with preterm birth (119, 120). As these bacteria have many anti-inflammatory properties, such as inhibition of LPS-induced NF-κB activation, IL-8 and COX-2 production, and induction and activation of IL-10-secreting regulatory T cells, a reduction in these bacteria could lead to increased susceptibility to inflammation-induced preterm birth (46).
The estrobolome is involved in not only the vagina-gut axis but also the uterus-gut axis. Endometrial hyperplasia and cancer are estrogen-dependent (29). Therefore, the estrobolome in the gut can influence the development of these estrogen-dependent diseases (5, 17, 70, 72).
Active IBD can impair fertility, probably via multifactorial mechanisms, including pelvic inflammation of the ovaries and fallopian tube (121). The change in endometrial microbiota accompanied by IBD remains unknown, although predominant taxa in the endometrial microbiota for different gynecological disorders have gradually become clear (14). LPS from the gut might impair fertility and preterm birth by inducing apoptosis of embryonic cells, delaying stromal cell proliferation, and modulating hormones (122).
Interestingly, SCFAs from the maternal gut microbiota affected the metabolic phenotype of offspring in mice during pregnancy (16). Therefore, the uterus-gut axis can play roles not only in fertility but also in the development of offspring.
Ovary-gut axis
Ovarian functions, including ovulation and lutein body formation, are precisely controlled by pituitary hormones but are also affected by intestinal functions, including the microbiota. For example, IBD can be a risk factor for ovarian dysfunction, including premature ovarian failure (123). Furthermore, patients with polycystic ovary syndrome (PCOS), which is often accompanied by hyperandrogenism, showed reduced diversity and different phylogenetic compositions of the gut microbiota (124, 125). Bacteroidaceae, Bacteroides, Coprococcus, Escherichia/Shigella, Faecalibacterium prausnitzii, Lactobacillus, Parabacteroides, and Prevotella were identified as the most common bacterial alterations in PCOS patients (126). Interestingly, SCFAs restored ovarian function in a PCOS rat model (127). Insulin resistance with abnormal SCFA metabolism by the gut microbiota might be associated with PCOS (128). Therefore, regulation of SCFA by the gastrointestinal/vaginal microbiota could be involved in the pathogenesis of PCOS. In addition, as IL-22 produced by intestinal group 3 innate lymphoid cells (ILC3) reversed insulin resistance (129), interactions among the gut microbiota, bile acid, and IL-22 appear to have roles in the pathophysiology of PCOS (130). A chronic inflammatory state and disrupted gut mucosal integrity caused by gut dysbiosis are other inducible candidates for PCOS (131). A previous study also showed that an increased prevalence of BV was found in patients with PCOS (132). Hence, the vagina-gut axis in addition to the ovary-gut axis might be important in PCOS patients (131).
In addition to PCOS, the gut microbiota can modulate ovarian cancer. The presence of sex hormone receptors in many putative tissues of origin for ovarian cancer suggests potential roles for sex hormones in the origin and promotion of ovarian cancer (133). Therefore, the estrobolome in the gut might have an important role in the induction of estrogen-dependent ovarian cancer (72).
In terms of reproduction, LPS from the gut might affect not only the uterus but also the ovary. LPS can activate macrophages in the ovaries, which produce cytotoxic proinflammatory cytokines (134, 135). These cytokines could exert autocrine/paracrine effects causing regression of the ovary (122).
Vagina-bladder axis
Common genera of the urinary microbiota are Citrobacter, Escherichia, Enterococcus, Prevotella, and Streptococcus (136, 137). Corynebacterium and Streptococcus are reported to be more abundant in men, and Lactobacillus is more abundant in women (137). The urinary microbiota shares 62.5% of species with the gut microbiota and 32% with the vaginal microbiota (137). As urine samples showed substantial concordance to paired mid-vaginal samples regarding bacterial composition, the genitourinary microbiota could be a good indicator for the overall composition of the vaginal microbiota (138).
A typical starting point of a urinary tract infection (UTI) is periurethral contamination by a uropathogen residing in the gut (139). Then, the uropathogen colonizes the urethra and subsequently migrates to the bladder. Therefore, dysbiotic states with pathogenic microbes in the gut would increase the risk of UTI (140). Similar to this gut-bladder axis, vaginal dysbiosis may prompt a UTI via the vagina-bladder axis as a reservoir for uropathogens such as E. coli (6). Although G. vaginalis is a rare cause of symptomatic UTI, an in vitro study showed that G. vaginalis altered bladder gene expression and increased susceptibility to subsequent UTI caused by uropathogenic E. coli (141). Compared to women without BV, those with BV have a significantly increased risk of UTI, with an odds ratio of 13.75 (142), and a clinical trial showed that the efficacy of intravaginally administered L. crispatus probiotics reduced the recurrence of UTI (143). Furthermore, as G. vaginalis is often detected in the urinary microbiota (141), the urine microbiota may prompt BV via the vagina-bladder axis as a reservoir for G. vaginalis.
FRT-oral axes
Following the GI microbiota, the oral cavity harbors the second most diverse and largely populated microbiota in the body (144). Different sites and conditions in the oral cavity have different microbial compositions (145–147). Marginal gingival biofilms contain Corynebacterium, Streptococcus, Neisseria, Fusobacterium, Leptotrichia, Porphyromonas and Haemophilus, while dorsal tongue biofilms contain Rothia, Veillonella, Actinomyces, Streptococcus, and Neisseria (148). Cooperation of the oral microbiota and the host reflects the information and status of immunity and metabolism via a two-way axis along the oral cavity and systemic organs (149). Below, we discuss the vagina-oral axis and the uterus-oral axis.
Vagina-oral axis
Evidence indicates the existence of the vagina-oral axis. There is a significant association between periodontitis and BV (150). Women with BV have a higher risk of gingivitis (151). An association with a significant diversification of salivary microbiota and higher counts of Prevotella intermedia in the subgingival gingival microbiota was reported in women with BV compared with women without BV (152). Sexual risk behaviors such as oral sex could translocate pathogens between the oral cavity and the vagina and would be one mechanism of the vagina-oral axis (150). Furthermore, a recent study showed an association between periodontitis and higher systemic markers of inflammation in women with BV, indicating systemic inflammation as the underlying mechanism (12). The coexistence of BV and periodontitis in smokers (12) is also good evidence of the vagina-oral axis. The association was previously thought to be due to women who smoke being more sexually active than nonsmokers, but more recently, it has been suggested that several chemical factors in cigarettes, together with common abnormalities in mucosal immunity (153), might kill oral and vaginal commensal bacteria directly or via bacteriophages (154, 155).
Uterus-oral axis
The relationship between labor onset and the oral cavity raises interesting questions. In general, dental caries and periodontal disease are associated with preterm labor (156). Although the existence of a normal placental microbiota is under debate, as mentioned above (37, 38), some studies have suggested that inflammatory conditions of the oral cavity could cause bacterial translocation from the oral cavity via the bloodstream to the uterus (157–159). DNA of periodontal disease-related pathogens, such as Porphyromonas gingivalis, Fusobacterium nucleatum, Prevotella intermedia, and Treponema denticola, is often detected in the placenta of patients with periodontal disease (160). Such pathogens can cause placental dysfunction (161). Furthermore, the presence of P. gingivalis in the umbilical cord is associated with preeclampsia. The genus Aggregatibacter, which includes some periodontal disease-related bacteria, is significantly enriched in the oral microbiota of women with threatened preterm labor (57). Overall, oral bacteria may reach the uterus in the bloodstream and cause inappropriate labor, either directly or via inflammatory cytokines. Therefore, an association between periodontal disease and adverse pregnancy outcomes is suggested (162), although further mechanisms of the uterus-oral axis are still under investigation.
FRT-brain axes
The gut-brain axis consists of multiple connections, such as bacterial metabolites and products, the vagus nerve, and the immune system [for review, see (163, 164)]. Therefore, similar physiology could be postulated in the FRT-brain axis. Microbial-derived metabolites appear to be modulators of the FRT-brain axis. For example, SCFAs produced by the GI microbiota are speculated to have a key role in the gut-brain axis (67), indicating that the production of SCFAs in the vagina might affect brain function similarly. Although it is still unknown whether an efferent vagal influence on pelvic organs exists, vagal afferent supply of the uterus has been indicated by retrograde and anterograde tracing studies in vivo (165). Normal immune function could be impaired or dysregulated by exposure to chronic stress through the hypothalamic−pituitary−adrenal pathway and the sympathetic-adrenal-medullary pathway with associated hormones (166). Some studies have indicated the existence of the vagina-brain and uterus-brain axes, as discussed below. However, the FRT-brain axis needs further investigation.
Vagina-brain axis
Stress might affect the vaginal microbiota (27). Stress-induced cortisol could inhibit vaginal glycogen deposition, resulting in lower Lactobacillus dominance, elevated vaginal pH, and an increase in the proinflammatory response (167). Increased psychosocial stress is associated with greater BV prevalence (168), although few associations between stress and BV have been observed in pregnant women (169). Another study reported no significant associations between the vaginal microbiota and mood, although the sample size was limited (170). SCFAs produced by the gut microbiota may regulate brain function directly or indirectly (67). Therefore, similar functions can be assumed in SCFAs from the vaginal microbiota.
Uterus-brain axis
Accumulating evidence is providing a clearer understanding of the brain-uterus axis (17). Gonadotropin releasing hormone (GnRH) signals the pituitary gland to secrete luteinizing hormone (LH) and follicle-stimulating hormone (FSH), which consequently increase the production of estrogens from the ovary (171, 172). Adenomyosis and endometriosis are estrogen-dependent gynecological disorders that cause pelvic pain, abnormal uterine bleeding, and infertility (173, 174). GnRH agonists or antagonists are administered to treat these diseases, and improvements in chronic pelvic pain have been reported (175). It is hypothesized that pain sensitivity might increase the secretion of GnRH (17). Therefore, further studies of pelvic pain and GnRH secretion from the pituitary gland would promote our understanding of the uterus-brain axis.
Vagina-joint axis
Some studies have reported the involvement of the vagina-joint axis in joint diseases. Reactive arthritis and rheumatoid arthritis have been revealed to be associated with BV and Gardnerella in the gut microbiota (176–178). Although the mechanisms of interactions between the microbial antigen and the host remain unknown, the cause of reactive arthritis is considered an overstimulated autoimmune response or bacterial antigens that deposit in the joints (179). The antigens that can trigger reactive arthritis are gram-negative aerobic bacteria, and these bacteria invade gastrointestinal/urogenital mucosal sites (179). Therefore, reactive arthritis could be caused by vaginal G. vaginalis (gram-negative) infection (180), which would be considered the vagina-joint axis. In contrast, the mechanism by which vaginal dysbiosis may cause rheumatoid arthritis remains a mystery.
Therapeutic opportunities
The FRT-organ axes appear to be involved in a variety of diseases (Figure 3). Therefore, restoration of dysbiotic conditions is a potential treatment. Therapeutic opportunities for pharmabiotics, including prebiotics, probiotics, synbiotics, and fecal microbiota transplantation, are well acknowledged. Recently, a clinical trial of vaginal microbiota transplantation for BV was conducted (181). However, although L. crispatus seems preferable for vaginal application, indigenous vaginal lactobacilli often surpass colonization of the probiotic L. crispatus strain from the vaginal source (CTV-05) (182). After 24 weeks, approximately 50% of patients who received this probiotic had not retained the strain (183).
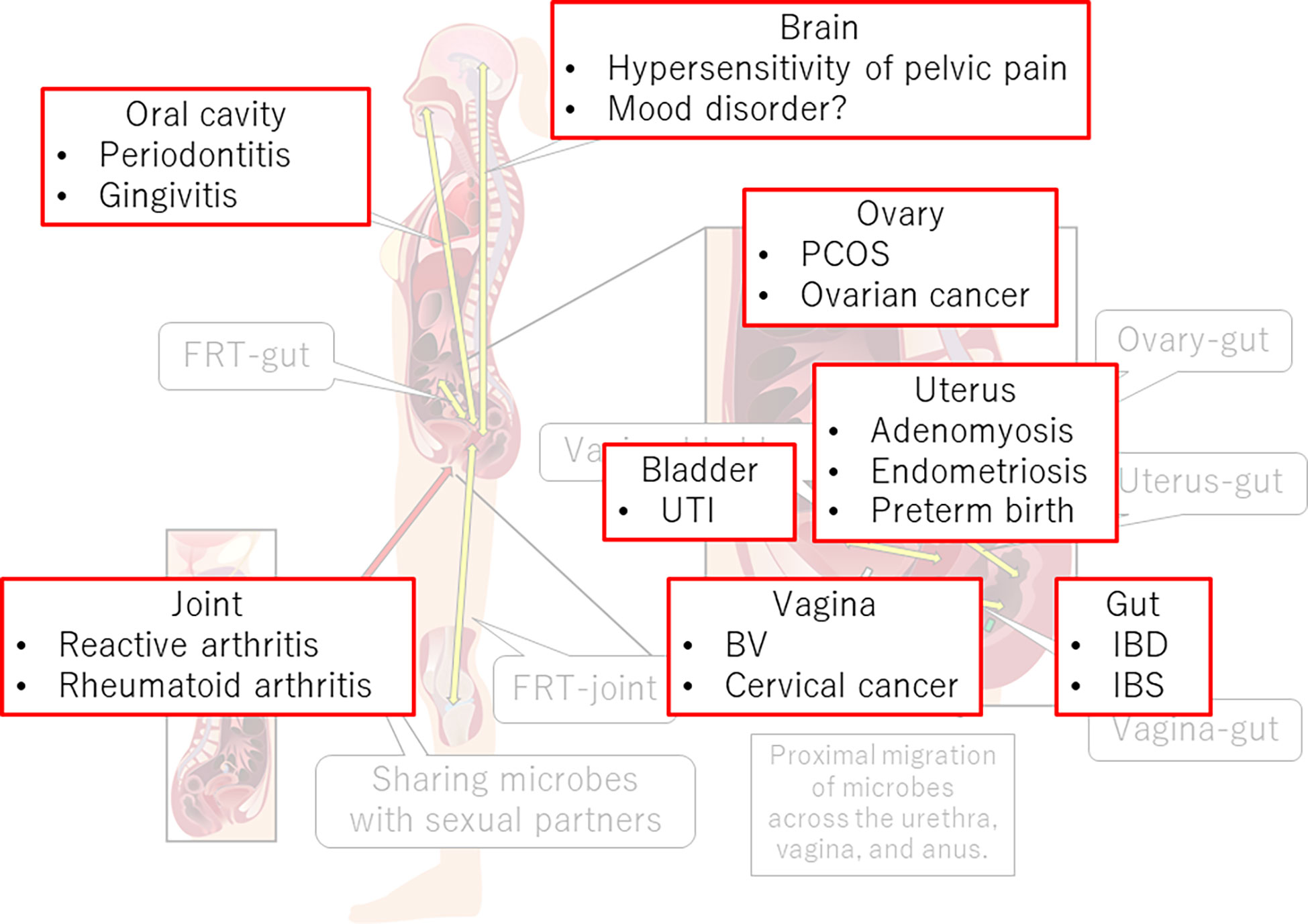
Figure 3 FRT-organ axes and diseases. Dysregulation of immune, neural, endocrine, or metabolic functions in the FRT-organ axes implicates its involvement in the pathogenesis of not only gynecological diseases but also diseases occurring apart from the FRT. PCOS, polycystic ovary syndrome; UTI, urinary tract infection; BV, bacterial vaginosis; IBD, inflammatory bowel disease; IBS, irritable bowel syndrome.
Mutual regulation of mucosal factors and the resident microbiota (76) may explain the insufficient clinical efficacy of pharmabiotics, which are “alien” to the body’s own microbiota and local immunity. The colonization resistance of the mucous membranes prevents exogenous bacteria from colonizing the body. In some cases, the use of commercial probiotics even has a negative effect on the process of microbiota recovery after antibiotic therapy (184). An alternative to commercial probiotics is an approach based on the restoration of the microbiota in the event of dysbiosis by using bacterial strains from a person’s own microbiota. This approach, known as autoprobiotic technology or personalized symbiont therapy (PERST), involves the isolation of individual representatives of the resident beneficial microbiota in pure culture, checking for the absence of genes associated with pathogenicity, accumulating these bacteria by cultivation, and returning them back to the host (185, 186). These autoprobiotic strains easily colonize mucous membranes and do not induce a rejection response from the host’s resident microbiota and immune system. Autoprobiotic treatment is accompanied by recovery of the immune status and distinctive anti-inflammatory effects (185). A prospective randomized study showed that autoprobiotic treatment with lactobacilli was more effective than that with allogeneic strains in restoring the level of lactic acid bacteria in the vagina and reducing the risk of recurrence of BV (187). Thus, instead of the classic application of probiotics, PERST technology based on autologous lactobacilli can be used to cure vaginal dysbiosis-related disorders.
The stability of the microflora partially depends on intra- and interspecies bacterial antagonism (8, 188). Although interspecies antagonism might be less likely to occur among vaginal Lactobacillus, as discussed above (90, 91), whole-genome sequencing revealed that the species L. crispatus is heterogeneous in the vagina (189). Therefore, to understand this advantage of autoprobiotic strains, in-depth research on intraspecies bacterial antagonism also seems to be needed. Furthermore, regarding FRT organ axes, simultaneous administration of autoprobiotics at different sites might synergistically restore dysbiosis.
Future direction and conclusion
Dysbiosis can be characterized by an underlying disruption of host functions that regulate the microbiota and microbial metabolism (190). Hence, more quantitative insights into the homeostasis of the microbiota could be provided by host function measurements rather than current microbe-centric approaches (190), although the microbiota is clearly a key player in the FRT-organ axes.
Among the FRT-organ axes, the underlying mechanism shared by the “mucus-mucus” axes (such as the vagina-gut axis and the vagina-oral axis) can be discussed; dysfunction at one mucosal tissue may cause dysfunction at other mucosal tissues. Bacterial direct translocation is important for considering these “mucus-mucus” axes. However, indirect interaction should be noted. It is also well established that mucosal dysregulation and dysbiosis can cause chronic inflammation in distal tissues via systemic circulation of inflammatory cytokines (191, 192). As discussed above, systemic inflammation could be the underlying mechanism of the oral-gut axis (12). Dysregulated intercellular junction proteins and barrier properties allow bacterial components (e.g., LPS), bacterial metabolites, and cytokines to enter the systemic circulation, resulting in the dysregulation of other tissues. Overall, systemic modulation of the immune system can be the underlying mechanism of the interactions of mucosal tissues in the FRT-organ axes.
Controversial roles of SCFAs in the development of cervical cancer also remain to be elucidated. As discussed above, SCFAs can cause vaginal dysbiotic and inflammatory conditions (65, 68). A meta-analysis supports a causal link between cervical cancer and vaginal dysbiosis (113). However, SCFAs also have antitumor effects (72, 111). Butyrate can trigger cascades of responses that not only lead to malignancy but also inhibit it (193). Therefore, determining the role of SCFAs in cervical cancer development or prevention is a future need.
In addition, although each axis is discussed separately for a comprehensive presentation in this review, the axes can interact with each other, such as the vagina-uterus-gut axes. Therefore, integration of each axis is required for further understanding of the FRT-organ axes. Investigations of not only the bacteriome but also the virome, mycobiome, archaeome and candidate phyla radiation in FRT are still needed to consider the FRT organ axes.
Furthermore, in the current era of diversity, we should also consider the preferable composition of the microbiota for transgender people (7). For transgender women, the microbiota of the penile skin-lined neovagina is substantially similar to that in women with BV with a very limited number of lactobacilli (194). For transgender men prescribed testosterone, the vaginal microbiota is less likely to have Lactobacillus (195). Long-term administration of high-dose testosterone disrupts the normal architecture of the vaginal epithelium and reduces glycogen deposition (196), which could result in a reduction in vaginal Lactobacillus. Regarding vaginal microbiota modulation, the neovaginal microflora of transgender women was significantly enriched with lactobacilli following an orally administered mixture of lactobacilli (197). A positive association was also found between intravaginal estrogen administration and the presence of Lactobacillus in transgender men (195). However, several key gaps in this field remain in the literature, such as the systemic effect of local estrogen therapy in transgender men (198). Furthermore, the role of the FRT-organ axes in transgender people is completely unknown.
In addition to the investigation of the FRT-organ axis, a more effective approach to cure the dysbiotic condition in FRT is needed. One promising approach in the future may be IgA-coated probiotics. IgA facilitates Bacteroides fragilis colonization of the murine gut (18). IgA-coated L. jensenii, not IgA-free lactic acid bacterial strains, from the fecal microbiota of a healthy woman significantly inhibits dyslipidemia and gut barrier damage in high-fat diet-fed mice (199). As mentioned above, IgA-coated bacteria are observed more frequently in vaginal microbiota with L. crispatus dominance compared with that with non-L. crispatus-dominant microbiota (84). Therefore, IgA-coated L. crispatus as a probiotic might colonize the vagina longer than current probiotic strains. However, to establish this approach, further age-range studies to investigate IgA-coated bacteria in the vagina are needed.
In conclusion, investigation of the FRT-organ axes would provide a multicentric approach including immune, neural, endocrine, and metabolic aspects for understanding the homeostatic mechanism of women’s bodies. The framework of the FRT-organ axes could also provide a cue to find new therapeutic approaches to maintain women’s health.
Author contributions
The manuscript was conceptualized by KT and VM. KT, VM and SH wrote the manuscript. RK, SK-A, NT and SH supervised the project. All authors contributed to the article and approved the submitted version.
Funding
This study was supported by a Grant-in-Aid for Young Scientists from the Ministry of Education, Culture, Sports, Science and Technology, Japan, with contract grant number 20K18177 (to KT) and Nihon University Research Grant for 2022 (to SH).
Acknowledgments
We thank American Journal Experts for their editorial assistance.
Conflict of interest
The authors declare that the research was conducted in the absence of any commercial or financial relationships that could be construed as a potential conflict of interest.
Publisher’s note
All claims expressed in this article are solely those of the authors and do not necessarily represent those of their affiliated organizations, or those of the publisher, the editors and the reviewers. Any product that may be evaluated in this article, or claim that may be made by its manufacturer, is not guaranteed or endorsed by the publisher.
References
1. Ahlawat S, Asha, Sharma KK. Gut-organ axis: a microbial outreach and networking. Lett Appl Microbiol (2021) 72(6):636–68. doi: 10.1111/lam.13333
2. Clemente JC, Ursell LK, Parfrey LW, Knight R. The impact of the gut microbiota on human health: an integrative view. Cell (2012) 148(6):1258–70. doi: 10.1016/j.cell.2012.01.035
3. Fan Y, Pedersen O. Gut microbiota in human metabolic health and disease. Nat Rev Microbiol (2021) 19(1):55–71. doi: 10.1038/s41579-020-0433-9
4. Krautkramer KA, Fan J, Bäckhed F. Gut microbial metabolites as multi-kingdom intermediates. Nat Rev Microbiol (2021) 19(2):77–94. doi: 10.1038/s41579-020-0438-4
5. Łaniewski P, Ilhan ZE, Herbst-Kralovetz MM. The microbiome and gynaecological cancer development, prevention and therapy. Nat Rev Urol (2020) 17(4):232–50. doi: 10.1038/s41585-020-0286-z
6. Meštrović T, Matijašić M, Perić M, Čipčić Paljetak H, Barešić A, Verbanac D. The role of gut, vaginal, and urinary microbiome in urinary tract infections: From bench to bedside. Diagnostics (Basel) (2020) 11(1):7. doi: 10.3390/diagnostics11010007
7. Takada K, Komine-Aizawa S, Tsuji N, Hayakawa S. Chapter 20. interactions between the epithelial barrier and the microbiota in the reproductive tract. In: Reproductive immunology: Basic concepts. 50 Hampshire Street, 5th Floor, Cambridge, MA 02139, United States: Academic Press (2021). p. 387–414.
8. Petrova MI, Lievens E, Malik S, Imholz N, Lebeer S. Lactobacillus species as biomarkers and agents that can promote various aspects of vaginal health. Front Physiol (2015) 6:81. doi: 10.3389/fphys.2015.00081
9. Anahtar MN, Gootenberg DB, Mitchell CM, Kwon DS. Cervicovaginal microbiota and reproductive health: The virtue of simplicity. Cell Host Microbe (2018) 23(2):159–68. doi: 10.1016/j.chom.2018.01.013
10. France M, Alizadeh M, Brown S, Ma B, Ravel J. Towards a deeper understanding of the vaginal microbiota. Nat Microbiol (2022) 7(3):367–78. doi: 10.1038/s41564-022-01083-2
11. Plesniarski A, Siddik AB, Su RC. The microbiome as a key regulator of female genital tract barrier function. Front Cell Infect Microbiol (2021) 11:790627. doi: 10.3389/fcimb.2021.790627
12. Escalda C, Botelho J, Mendes JJ, Machado V. Association of bacterial vaginosis with periodontitis in a cross-sectional American nationwide survey. Sci Rep (2021) 11(1):630. doi: 10.1038/s41598-020-79496-4
13. Medina-Bastidas D, Camacho-Arroyo I, García-Gómez E. Current findings in endometrial microbiome: impact on uterine diseases. Reproduction (2022) 163(5):R81–r96. doi: 10.1530/rep-21-0120
14. Molina NM, Sola-Leyva A, Saez-Lara MJ, Plaza-Diaz J, Tubić-Pavlović A, Romero B, et al. New opportunities for endometrial health by modifying uterine microbial composition: Present or future? Biomolecules (2020) 10(4):593. doi: 10.3390/biom10040593
15. Amabebe E, Anumba DOC. Female gut and genital tract microbiota-induced crosstalk and differential effects of short-chain fatty acids on immune sequelae. Front Immunol (2020) 11:2184. doi: 10.3389/fimmu.2020.02184
16. Kimura I, Miyamoto J, Ohue-Kitano R, Watanabe K, Yamada T, Onuki M, et al. Maternal gut microbiota in pregnancy influences offspring metabolic phenotype in mice. Science (2020) 367(6481):eaaw8429. doi: 10.1126/science.aaw8429
17. Salliss ME, Farland LV, Mahnert ND, Herbst-Kralovetz MM. The role of gut and genital microbiota and the estrobolome in endometriosis, infertility and chronic pelvic pain. Hum Reprod Update (2021) 28(1):92–131. doi: 10.1093/humupd/dmab035
18. Donaldson GP, Ladinsky MS, Yu KB, Sanders JG, Yoo BB, Chou WC, et al. Gut microbiota utilize immunoglobulin a for mucosal colonization. Science (2018) 360(6390):795–800. doi: 10.1126/science.aaq0926
19. Nakajima A, Vogelzang A, Maruya M, Miyajima M, Murata M, Son A, et al. IgA regulates the composition and metabolic function of gut microbiota by promoting symbiosis between bacteria. J Exp Med (2018) 215(8):2019–34. doi: 10.1084/jem.20180427
20. Bunker JJ, Bendelac A. IgA responses to microbiota. Immunity (2018) 49(2):211–24. doi: 10.1016/j.immuni.2018.08.011
21. Thaiss CA, Zmora N, Levy M, Elinav E. The microbiome and innate immunity. Nature (2016) 535(7610):65–74. doi: 10.1038/nature18847
22. Breedveld A, van Egmond M. IgA and FcαRI: Pathological roles and therapeutic opportunities. Front Immunol (2019) 10:553. doi: 10.3389/fimmu.2019.00553
23. Ravel J, Gajer P, Abdo Z, Schneider GM, Koenig SS, McCulle SL, et al. Vaginal microbiome of reproductive-age women. Proc Natl Acad Sci (2011) 108(Supplement 1):4680–7. doi: 10.1073/pnas.1002611107
24. Gajer P, Brotman RM, Bai G, Sakamoto J, Schutte UM, Zhong X, et al. Temporal dynamics of the human vaginal microbiota. Sci Transl Med (2012) 4(132):132ra52. doi: 10.1126/scitranslmed.3003605
25. Smith SB, Ravel J. The vaginal microbiota, host defence and reproductive physiology. J Physiol (2017) 595(2):451–63. doi: 10.1113/jp271694
26. Villa P, Cipolla C, D'Ippolito S, Amar ID, Shachor M, Ingravalle F, et al. The interplay between immune system and microbiota in gynecological diseases: a narrative review. Eur Rev Med Pharmacol Sci (2020) 24(10):5676–90. doi: 10.26355/eurrev_202005_21359
27. Lehtoranta L, Ala-Jaakkola R, Laitila A, Maukonen J. Healthy vaginal microbiota and influence of probiotics across the female life span. Front Microbiol (2022) 13:819958. doi: 10.3389/fmicb.2022.819958
28. Baker JM, Chase DM, Herbst-Kralovetz MM. Uterine microbiota: Residents, tourists, or invaders? Front Immunol (2018) 9:208. doi: 10.3389/fimmu.2018.00208
29. Boutriq S, González-González A, Plaza-Andrades I, Laborda-Illanes A, Sánchez-Alcoholado L, Peralta-Linero J, et al. Gut and endometrial microbiome dysbiosis: A new emergent risk factor for endometrial cancer. J Pers Med (2021) 11(7):659. doi: 10.3390/jpm11070659
30. Sola-Leyva A, Andrés-León E, Molina NM, Terron-Camero LC, Plaza-Díaz J, Sáez-Lara MJ, et al. Mapping the entire functionally active endometrial microbiota. Hum Reprod (2021) 36(4):1021–31. doi: 10.1093/humrep/deaa372
31. Winters AD, Romero R, Gervasi MT, Gomez-Lopez N, Tran MR, Garcia-Flores V, et al. Does the endometrial cavity have a molecular microbial signature? Sci Rep (2019) 9(1):9905. doi: 10.1038/s41598-019-46173-0
32. Wang J, Li Z, Ma X, Du L, Jia Z, Cui X, et al. Translocation of vaginal microbiota is involved in impairment and protection of uterine health. Nat Commun (2021) 12(1):4191. doi: 10.1038/s41467-021-24516-8
33. Chen C, Song X, Wei W, Zhong H, Dai J, Lan Z, et al. The microbiota continuum along the female reproductive tract and its relation to uterine-related diseases. Nat Commun (2017) 8(1):875. doi: 10.1038/s41467-017-00901-0
34. Yoshida T, Takada K, Komine-Aizawa S, Kamei Y, Ishihara O, Hayakawa S. Lactobacillus crispatus promotes invasion of the HTR-8/SVneo trophoblast cell line. Placenta (2021) 111:76–81. doi: 10.1016/j.placenta.2021.06.006
35. Heusler M, Einenkel R, Ehrhardt J, Muzzio DO, Zygmunt M. Low abundance fusobacterium nucleatum supports early pregnancy development - an In vitro study. Front Immunol (2021) 12:698045. doi: 10.3389/fimmu.2021.698045
36. Aagaard K, Ma J, Antony KM, Ganu R, Petrosino J, Versalovic J. The placenta harbors a unique microbiome. Sci Transl Med (2014) 6(237):237ra65. doi: 10.1126/scitranslmed.3008599
37. Dudley DJ. The placental microbiome: yea, nay or maybe? Bjog (2020) 127(2):170. doi: 10.1111/1471-0528.15994
38. Zakis DR, Paulissen E, Kornete L, Kaan AMM, Nicu EA, Zaura E. The evidence for placental microbiome and its composition in healthy pregnancies: A systematic review. J Reprod Immunol (2022) 149:103455. doi: 10.1016/j.jri.2021.103455
39. Pelzer ES, Willner D, Buttini M, Hafner LM, Theodoropoulos C, Huygens F. The fallopian tube microbiome: implications for reproductive health. Oncotarget (2018) 9(30):21541–51. doi: 10.18632/oncotarget.25059
40. Miles SM, Hardy BL, Merrell DS. Investigation of the microbiota of the reproductive tract in women undergoing a total hysterectomy and bilateral salpingo-oopherectomy. Fertil Steril (2017) 107(3):813–20.e1. doi: 10.1016/j.fertnstert.2016.11.028
41. Abou Chacra L, Fenollar F. Exploring the global vaginal microbiome and its impact on human health. Microb Pathog (2021) 160:105172. doi: 10.1016/j.micpath.2021.105172
42. Bradford LL, Ravel J. The vaginal mycobiome: A contemporary perspective on fungi in women's health and diseases. Virulence (2017) 8(3):342–51. doi: 10.1080/21505594.2016.1237332
43. Happel AU, Varsani A, Balle C, Passmore JA, Jaspan H. The vaginal virome-balancing female genital tract bacteriome, mucosal immunity, and sexual and reproductive health outcomes? Viruses (2020) 12(8):832. doi: 10.3390/v12080832
44. Leitsch D. Recent advances in the molecular biology of the protist parasite trichomonas vaginalis. Fac Rev (2021) 10:26. doi: 10.12703/r/10-26
45. Madere FS, Sohn M, Winbush AK, Barr B, Grier A, Palumbo C, et al. Transkingdom analysis of the female reproductive tract reveals bacteriophages form communities. Viruses (2022) 14(2):430. doi: 10.3390/v14020430
46. Bayar E, Bennett PR, Chan D, Sykes L, MacIntyre DA. The pregnancy microbiome and preterm birth. Semin Immunopathol (2020) 42(4):487–99. doi: 10.1007/s00281-020-00817-w
47. Fong IW. The rectal carriage of yeast in patients with vaginal candidiasis. Clin Invest Med (1994) 17(5):426–31.
48. Kastl AJ Jr., Terry NA, Wu GD, Albenberg LG. The structure and function of the human small intestinal microbiota: Current understanding and future directions. Cell Mol Gastroenterol Hepatol (2020) 9(1):33–45. doi: 10.1016/j.jcmgh.2019.07.006
49. Million M, Tomas J, Wagner C, Lelouard H, Raoult D, Gorvel J-P. New insights in gut microbiota and mucosal immunity of the small intestine. Hum Microbiome J (2018) 7:23–32. doi: 10.1016/j.humic.2018.01.004
50. Pelzer E, Gomez-Arango LF, Barrett HL, Nitert MD. Review: Maternal health and the placental microbiome. Placenta (2017) 54:30–7. doi: 10.1016/j.placenta.2016.12.003
51. Altmäe S, Franasiak JM, Mändar R. The seminal microbiome in health and disease. Nat Rev Urol (2019) 16(12):703–21. doi: 10.1038/s41585-019-0250-y
52. Neggers YH, Nansel TR, Andrews WW, Schwebke JR, Yu KF, Goldenberg RL, et al. Dietary intake of selected nutrients affects bacterial vaginosis in women. J Nutr (2007) 137(9):2128–33. doi: 10.1093/jn/137.9.2128
53. Thoma ME, Klebanoff MA, Rovner AJ, Nansel TR, Neggers Y, Andrews WW, et al. Bacterial vaginosis is associated with variation in dietary indices. J Nutr (2011) 141(9):1698–704. doi: 10.3945/jn.111.140541
54. Ravel J, Brotman RM. Translating the vaginal microbiome: gaps and challenges. Genome Med (2016) 8(1):35. doi: 10.1186/s13073-016-0291-2
55. Antonio MA, Rabe LK, Hillier SL. Colonization of the rectum by lactobacillus species and decreased risk of bacterial vaginosis. J Infect Dis (2005) 192(3):394–8. doi: 10.1086/430926
56. El Aila NA, Tency I, Claeys G, Verstraelen H, Saerens B, Santiago GL, et al. Identification and genotyping of bacteria from paired vaginal and rectal samples from pregnant women indicates similarity between vaginal and rectal microflora. BMC Infect Dis (2009) 9:167. doi: 10.1186/1471-2334-9-167
57. Fudaba M, Kamiya T, Tachibana D, Koyama M, Ohtani N. Bioinformatics analysis of oral, vaginal, and rectal microbial profiles during pregnancy: A pilot study on the bacterial Co-residence in pregnant women. Microorganisms (2021) 9(5):1027. doi: 10.3390/microorganisms9051027
58. Reid G, Bruce AW, Fraser N, Heinemann C, Owen J, Henning B. Oral probiotics can resolve urogenital infections. FEMS Immunol Med Microbiol (2001) 30(1):49–52. doi: 10.1111/j.1574-695X.2001.tb01549.x
59. Morelli L, Zonenenschain D, Del Piano M, Cognein P. Utilization of the intestinal tract as a delivery system for urogenital probiotics. J Clin Gastroenterol (2004) 38(6 Suppl):S107–10. doi: 10.1097/01.mcg.0000128938.32835.98
60. Strus M, Chmielarczyk A, Kochan P, Adamski P, Chelmicki Z, Chelmicki A, et al. Studies on the effects of probiotic lactobacillus mixture given orally on vaginal and rectal colonization and on parameters of vaginal health in women with intermediate vaginal flora. Eur J Obstet Gynecol Reprod Biol (2012) 163(2):210–5. doi: 10.1016/j.ejogrb.2012.05.001
61. Russo R, Edu A, De Seta F. Study on the effects of an oral lactobacilli and lactoferrin complex in women with intermediate vaginal microbiota. Arch Gynecol Obstet (2018) 298(1):139–45. doi: 10.1007/s00404-018-4771-z
62. Pan M, Hidalgo-Cantabrana C, Barrangou R. Host and body site-specific adaptation of lactobacillus crispatus genomes. NAR Genomics Bioinf (2020) 2(1):lqaa001. doi: 10.1093/nargab/lqaa001
63. Nissila E, Douillard FP, Ritari J, Paulin L, Jarvinen HM, Rasinkangas P, et al. Correction: Genotypic and phenotypic diversity of lactobacillus rhamnosus clinical isolates, their comparison with strain GG and their recognition by complement system. PloS One (2017) 12(7):e0181292. doi: 10.1371/journal.pone.0181292
64. Pan M, Hidalgo-Cantabrana C, Goh YJ, Sanozky-Dawes R, Barrangou R. Comparative analysis of lactobacillus gasseri and lactobacillus crispatus isolated from human urogenital and gastrointestinal tracts. Front Microbiol (2020) 10:3146(3146). doi: 10.3389/fmicb.2019.03146
65. Aldunate M, Srbinovski D, Hearps AC, Latham CF, Ramsland PA, Gugasyan R, et al. Antimicrobial and immune modulatory effects of lactic acid and short chain fatty acids produced by vaginal microbiota associated with eubiosis and bacterial vaginosis. Front Physiol (2015) 6:164. doi: 10.3389/fphys.2015.00164
66. Rooks MG, Garrett WS. Gut microbiota, metabolites and host immunity. Nat Rev Immunol (2016) 16(6):341–52. doi: 10.1038/nri.2016.42
67. Dalile B, Van Oudenhove L, Vervliet B, Verbeke K. The role of short-chain fatty acids in microbiota-gut-brain communication. Nat Rev Gastroenterol Hepatol (2019) 16(8):461–78. doi: 10.1038/s41575-019-0157-3
68. Delgado-Diaz DJ, Tyssen D, Hayward JA, Gugasyan R, Hearps AC, Tachedjian G. Distinct immune responses elicited from cervicovaginal epithelial cells by lactic acid and short chain fatty acids associated with optimal and non-optimal vaginal microbiota. Front Cell Infect Microbiol (2019) 9:446. doi: 10.3389/fcimb.2019.00446
69. Łaniewski P, Herbst-Kralovetz MM. Connecting microbiome and menopause for healthy ageing. Nat Microbiol (2022) 7(3):354–8. doi: 10.1038/s41564-022-01071-6
70. Baker JM, Al-Nakkash L, Herbst-Kralovetz MM. Estrogen–gut microbiome axis: physiological and clinical implications. Maturitas (2017) 103:45–53. doi: 10.1016/j.maturitas.2017.06.025
71. Ervin SM, Li H, Lim L, Roberts LR, Liang X, Mani S, et al. Gut microbial β-glucuronidases reactivate estrogens as components of the estrobolome that reactivate estrogens. J Biol Chem (2019) 294(49):18586–99. doi: 10.1074/jbc.RA119.010950
72. Han M, Wang N, Han W, Ban M, Sun T, Xu J. Gut microbes in gynecologic cancers: Causes or biomarkers and therapeutic potential. Front Oncol (2022) 12:902695. doi: 10.3389/fonc.2022.902695
73. Witkin SS. Lactic acid alleviates stress: good for female genital tract homeostasis, bad for protection against malignancy. Cell Stress Chaperones (2018) 23(3):297–302. doi: 10.1007/s12192-017-0852-3
74. Linhares IM, Sisti G, Minis E, de Freitas GB, Moron AF, Witkin SS. Contribution of epithelial cells to defense mechanisms in the human vagina. Curr Infect Dis Rep (2019) 21(9):30. doi: 10.1007/s11908-019-0686-5
75. Woof JM, Kerr MA. The function of immunoglobulin a in immunity. J Pathol (2006) 208(2):270–82. doi: 10.1002/path.1877
76. Strugnell RA, Wijburg OL. The role of secretory antibodies in infection immunity. Nat Rev Microbiol (2010) 8(9):656–67. doi: 10.1038/nrmicro2384
77. Chen K, Magri G, Grasset EK, Cerutti A. Rethinking mucosal antibody responses: IgM, IgG and IgD join IgA. Nat Rev Immunol (2020) 20(7):427–41. doi: 10.1038/s41577-019-0261-1
78. Lehner T, Panagiotidi C, Bergmeier LA, Tao L, Brookes R, Gearing A, et al. Genital-associated lymphoid tissue in female non-human primates. Adv Exp Med Biol (1995) 371a:357–65. doi: 10.1007/978-1-4615-1941-6_75
79. Mestecky J, Russell MW. Induction of mucosal immune responses in the human genital tract. FEMS Immunol Med Microbiol (2000) 27(4):351–5. doi: 10.1111/j.1574-695X.2000.tb01449.x
80. Iwasaki A. Antiviral immune responses in the genital tract: clues for vaccines. Nat Rev Immunol (2010) 10(10):699–711. doi: 10.1038/nri2836
81. Zhou JZ, Way SS, Chen K. Immunology of the uterine and vaginal mucosae. Trends Immunol (2018) 39(4):302–14. doi: 10.1016/j.it.2018.01.007
82. Fahrbach KM, Malykhina O, Stieh DJ, Hope TJ. Differential binding of IgG and IgA to mucus of the female reproductive tract. PloS One (2013) 8(10):e76176. doi: 10.1371/journal.pone.0076176
83. Bunker JJ, Flynn TM, Koval JC, Shaw DG, Meisel M, McDonald BD, et al. Innate and adaptive humoral responses coat distinct commensal bacteria with immunoglobulin a. Immunity (2015) 43(3):541–53. doi: 10.1016/j.immuni.2015.08.007
84. Breedveld AC, Schuster HJ, van Houdt R, Painter RC, Mebius RE, van der Veer C, et al. Enhanced IgA coating of bacteria in women with lactobacillus crispatus-dominated vaginal microbiota. Microbiome (2022) 10(1):15. doi: 10.1186/s40168-021-01198-4
85. Peterson DA, McNulty NP, Guruge JL, Gordon JI. IgA response to symbiotic bacteria as a mediator of gut homeostasis. Cell Host Microbe (2007) 2(5):328–39. doi: 10.1016/j.chom.2007.09.013
86. Kawamoto S, Maruya M, Kato Lucia M, Suda W, Atarashi K, Doi Y, et al. Foxp3+ T cells regulate immunoglobulin a selection and facilitate diversification of bacterial species responsible for immune homeostasis. Immunity (2014) 41(1):152–65. doi: 10.1016/j.immuni.2014.05.016
87. León ED, Francino MP. Roles of secretory immunoglobulin a in host-microbiota interactions in the gut ecosystem. Front Microbiol (2022) 13:880484. doi: 10.3389/fmicb.2022.880484
88. Si J, You HJ, Yu J, Sung J, Ko G. Prevotella as a hub for vaginal microbiota under the influence of host genetics and their association with obesity. Cell Host Microbe (2017) 21(1):97–105. doi: 10.1016/j.chom.2016.11.010
89. Mora JR, Iwata M, Eksteen B, Song S-Y, Junt T, Senman B, et al. Generation of gut-homing IgA-secreting b cells by intestinal dendritic cells. Science (2006) 314(5802):1157–60. doi: 10.1126/science.1132742
90. Nilsen T, Swedek I, Lagenaur LA, Parks TP. Novel selective inhibition of lactobacillus iners by lactobacillus-derived bacteriocins. Appl Environ Microbiol (2020) 86(20):e01594–20. doi: 10.1128/aem.01594-20
91. Hayashida S, Takada K, Melnikov VG, Komine-Aizawa S, Tsuji NM, Hayakawa S. How were lactobacillus species selected as single dominant species in the human vaginal microbiota? coevolution of humans and lactobacillus. Med Hypotheses (2022) 163:110858. doi: 10.1016/j.mehy.2022.110858
92. Dominguez-Bello MG, Costello EK, Contreras M, Magris M, Hidalgo G, Fierer N, et al. Delivery mode shapes the acquisition and structure of the initial microbiota across multiple body habitats in newborns. Proc Natl Acad Sci U S A (2010) 107(26):11971–5. doi: 10.1073/pnas.1002601107
93. Abramov VM, Kosarev IV, Priputnevich TV, Machulin AV, Abashina TN, Chikileva IO, et al. S-layer protein 2 of vaginal lactobacillus crispatus 2029 enhances growth, differentiation, VEGF production and barrier functions in intestinal epithelial cell line caco-2. Int J Biol Macromol (2021) 189:410–9. doi: 10.1016/j.ijbiomac.2021.08.150
94. Xiaoming W, Jing L, Yuchen P, Huili L, Miao Z, Jing S. Characteristics of the vaginal microbiomes in prepubertal girls with and without vulvovaginitis. Eur J Clin Microbiol Infect Dis (2021) 40(6):1253–61. doi: 10.1007/s10096-021-04152-2
95. Labuda JC, Pham OH, Depew CE, Fong KD, Lee BS, Rixon JA, et al. Circulating immunity protects the female reproductive tract from chlamydia infection. Proc Natl Acad Sci U S A (2021) 118(21):e2104407118. doi: 10.1073/pnas.2104407118
96. Abramov V, Khlebnikov V, Kosarev I, Bairamova G, Vasilenko R, Suzina N, et al. Probiotic properties of lactobacillus crispatus 2,029: Homeostatic interaction with cervicovaginal epithelial cells and antagonistic activity to genitourinary pathogens. Probiotics Antimicrob Proteins (2014) 6(3-4):165–76. doi: 10.1007/s12602-014-9164-4
97. Toba T, Virkola R, Westerlund B, Bjorkman Y, Sillanpaa J, Vartio T, et al. A collagen-binding s-layer protein in lactobacillus crispatus. Appl Environ Microbiol (1995) 61(7):2467–71. doi: 10.1128/aem.61.7.2467-2471.1995
98. Oh JE, Iijima N, Song E, Lu P, Klein J, Jiang R, et al. Migrant memory b cells secrete luminal antibody in the vagina. Nature (2019) 571(7763):122–6. doi: 10.1038/s41586-019-1285-1
99. Bergmeier LA, Tao L, Gearing AJ, Adams S, Lehner T. Induction of IgA and IgG antibodies in vaginal fluid, serum and saliva following immunization of genital and gut associated lymphoid tissue. Adv Exp Med Biol (1995) 371b:1567–73.
100. Johansson EL, Wassén L, Holmgren J, Jertborn M, Rudin A. Nasal and vaginal vaccinations have differential effects on antibody responses in vaginal and cervical secretions in humans. Infect Immun (2001) 69(12):7481–6. doi: 10.1128/iai.69.12.7481-7486.2001
101. Agnello M, Carroll LN, Imam N, Pino R, Palmer C, Varas I, et al. Gut microbiome composition and risk factors in a large cross-sectional IBS cohort. BMJ Open Gastroenterol (2020) 7(1):e000345. doi: 10.1136/bmjgast-2019-000345
102. Zhou Y, He Y, Liu L, Zhou W, Wang P, Hu H, et al. Alterations in gut microbial communities across anatomical locations in inflammatory bowel diseases. Front Nutr (2021) 8:615064. doi: 10.3389/fnut.2021.615064
103. Schilling J, Loening-Baucke V, Dörffel Y. Increased gardnerella vaginalis urogenital biofilm in inflammatory bowel disease. J Crohns Colitis (2014) 8(6):543–9. doi: 10.1016/j.crohns.2013.11.015
104. Rosta K, Mazzucato-Puchner A, Kiss H, Malik V, Mandl P, Petricevic L, et al. Vaginal microbiota in pregnant women with inflammatory rheumatic and inflammatory bowel disease: A matched case-control study. Mycoses (2021) 64(8):909–17. doi: 10.1111/myc.13288
105. Hill JE, Peña-Sánchez JN, Fernando C, Freitas AC, Withana Gamage N, Fowler S. Composition and stability of the vaginal microbiota of pregnant women with inflammatory bowel disease. Inflammation Bowel Dis (2022) 28(6):905–11. doi: 10.1093/ibd/izab314
106. Violi F, Cammisotto V, Bartimoccia S, Pignatelli P, Carnevale R, Nocella C. Gut-derived low-grade endotoxaemia, atherothrombosis and cardiovascular disease. Nat Rev Cardiol (2023) 20(1):24–37. doi: 10.1038/s41569-022-00737-2
107. Stephens M, von der Weid PY. Lipopolysaccharides modulate intestinal epithelial permeability and inflammation in a species-specific manner. Gut Microbes (2020) 11(3):421–32. doi: 10.1080/19490976.2019.1629235
108. Candelli M, Franza L, Pignataro G, Ojetti V, Covino M, Piccioni A, et al. Interaction between lipopolysaccharide and gut microbiota in inflammatory bowel diseases. Int J Mol Sci (2021) 22(12):6242. doi: 10.3390/ijms22126242
109. Kim DE, Kim JK, Han SK, Jang SE, Han MJ, Kim DH. Lactobacillus plantarum NK3 and bifidobacterium longum NK49 alleviate bacterial vaginosis and osteoporosis in mice by suppressing NF-κB-Linked TNF-α expression. J Med Food (2019) 22(10):1022–31. doi: 10.1089/jmf.2019.4419
110. Wang Z, Wang Q, Zhao J, Gong L, Zhang Y, Wang X, et al. Altered diversity and composition of the gut microbiome in patients with cervical cancer. AMB Express (2019) 9(1):40. doi: 10.1186/s13568-019-0763-z
111. Matsuya-Ogawa M, Shibata T, Itoh H, Murakami H, Yaguchi C, Sugihara K, et al. Oncoprotective effects of short-chain fatty acids on uterine cervical neoplasia. Nutr Cancer (2019) 71(2):312–9. doi: 10.1080/01635581.2019.1578388
112. Taghinezhad SS, Keyvani H, Bermúdez-Humarán LG, Donders GGG, Fu X, Mohseni AH. Twenty years of research on HPV vaccines based on genetically modified lactic acid bacteria: an overview on the gut-vagina axis. Cell Mol Life Sci (2021) 78(4):1191–206. doi: 10.1007/s00018-020-03652-2
113. Brusselaers N, Shrestha S, van de Wijgert J, Verstraelen H. Vaginal dysbiosis and the risk of human papillomavirus and cervical cancer: systematic review and meta-analysis. Am J Obstet Gynecol (2019) 221(1):9–18.e8. doi: 10.1016/j.ajog.2018.12.011
114. Yamaoka Y. Mechanisms of disease: Helicobacter pylori virulence factors. Nat Rev Gastroenterol Hepatol (2010) 7(11):629–41. doi: 10.1038/nrgastro.2010.154
115. Yu Y, Zhu S, Li P, Min L, Zhang S. Helicobacter pylori infection and inflammatory bowel disease: a crosstalk between upper and lower digestive tract. Cell Death Dis (2018) 9(10):961. doi: 10.1038/s41419-018-0982-2
116. Bleicher J, Stockdale CK. Association between recurrent bacterial vaginosis and helicobacter pylori infection: a case report. Proc Obstetrics Gynecology (2015) 5(2):7. doi: 10.17077/2154-4751.1289
117. Schumacher A, Costa SD, Zenclussen AC. Endocrine factors modulating immune responses in pregnancy. Front Immunol (2014) 5:196. doi: 10.3389/fimmu.2014.00196
118. Agostinis C, Mangogna A, Bossi F, Ricci G, Kishore U, Bulla R. Uterine immunity and microbiota: A shifting paradigm. Front Immunol (2019) 10:2387. doi: 10.3389/fimmu.2019.02387
119. Dahl C, Stanislawski M, Iszatt N, Mandal S, Lozupone C, Clemente JC, et al. Gut microbiome of mothers delivering prematurely shows reduced diversity and lower relative abundance of bifidobacterium and streptococcus. PLoS One (2017) 12(10):e0184336. doi: 10.1371/journal.pone.0184336
120. Shiozaki A, Yoneda S, Yoneda N, Yonezawa R, Matsubayashi T, Seo G, et al. Intestinal microbiota is different in women with preterm birth: results from terminal restriction fragment length polymorphism analysis. PloS One (2014) 9(11):e111374. doi: 10.1371/journal.pone.0111374
121. Van Assche G, Dignass A, Reinisch W, van der Woude CJ, Sturm A, De Vos M, et al. The second European evidence-based consensus on the diagnosis and management of crohn's disease: Special situations. J Crohns Colitis (2010) 4(1):63–101. doi: 10.1016/j.crohns.2009.09.009
122. Deb K, Chaturvedi MM, Jaiswal YK. Comprehending the role of LPS in gram-negative bacterial vaginosis: ogling into the causes of unfulfilled child-wish. Arch Gynecol Obstet (2004) 270(3):133–46. doi: 10.1007/s00404-004-0623-0
123. Moktan VP, Daoud ND, Tremaine WJ, Loftus EV Jr., Kane SV, Hochwald AP, et al. A cohort study of the age at menopause in female patients with and without inflammatory bowel disease. Crohns Colitis 360 (2022) 4(3):otac027. doi: 10.1093/crocol/otac027
124. Lindheim L, Bashir M, Münzker J, Trummer C, Zachhuber V, Leber B, et al. Alterations in gut microbiome composition and barrier function are associated with reproductive and metabolic defects in women with polycystic ovary syndrome (PCOS): A pilot study. PLoS One (2017) 12(1):e0168390. doi: 10.1371/journal.pone.0168390
125. Torres PJ, Siakowska M, Banaszewska B, Pawelczyk L, Duleba AJ, Kelley ST, et al. Gut microbial diversity in women with polycystic ovary syndrome correlates with hyperandrogenism. J Clin Endocrinol Metab (2018) 103(4):1502–11. doi: 10.1210/jc.2017-02153
126. Guo J, Shao J, Yang Y, Niu X, Liao J, Zhao Q, et al. Gut microbiota in patients with polycystic ovary syndrome: a systematic review. Reprod Sci (2022) 29(1):69–83. doi: 10.1007/s43032-020-00430-0
127. Olaniyi KS, Bashir AM, Areloegbe SE, Sabinari IW, Akintayo CO, Oniyide AA, et al. Short chain fatty acid, acetate restores ovarian function in experimentally induced PCOS rat model. PLoS One (2022) 17(7):e0272124. doi: 10.1371/journal.pone.0272124
128. Zhao X, Jiang Y, Xi H, Chen L, Feng X. Exploration of the relationship between gut microbiota and polycystic ovary syndrome (PCOS): a review. Geburtshilfe Frauenheilkd (2020) 80(2):161–71. doi: 10.1055/a-1081-2036
129. Qi X, Yun C, Liao B, Qiao J, Pang Y. The therapeutic effect of interleukin-22 in high androgen-induced polycystic ovary syndrome. J Endocrinol (2020) 245(2):281–9. doi: 10.1530/joe-19-0589
130. Qi X, Yun C, Sun L, Xia J, Wu Q, Wang Y, et al. Publisher correction: Gut microbiota-bile acid-interleukin-22 axis orchestrates polycystic ovary syndrome. Nat Med (2019) 25(9):1459. doi: 10.1038/s41591-019-0562-8
131. Gu Y, Zhou G, Zhou F, Li Y, Wu Q, He H, et al. Gut and vaginal microbiomes in PCOS: Implications for women's health. Front Endocrinol (Lausanne) (2022) 13:808508. doi: 10.3389/fendo.2022.808508
132. Salah RM, Allam AM, Magdy AM, Mohamed A. Bacterial vaginosis and infertility: cause or association? Eur J Obstet Gynecol Reprod Biol (2013) 167(1):59–63. doi: 10.1016/j.ejogrb.2012.10.031
133. Modugno F, Laskey R, Smith AL, Andersen CL, Haluska P, Oesterreich S. Hormone response in ovarian cancer: time to reconsider as a clinical target? Endocr Relat Cancer (2012) 19(6):R255–79. doi: 10.1530/erc-12-0175
134. Taylor CC, Terranova PF. Lipopolysaccharide inhibits in vitro luteinizing hormone-stimulated rat ovarian granulosa cell estradiol but not progesterone secretion. Biol Reprod (1996) 54(6):1390–6. doi: 10.1095/biolreprod54.6.1390
135. Gorospe WC, Hughes FM Jr., Spangelo BL. Interleukin-6: effects on and production by rat granulosa cells. vitro. Endocrinol (1992) 130(3):1750–2. doi: 10.1210/endo.130.3.1537322
136. Roth RS, Liden M, Huttner A. The urobiome in men and women: a clinical review. Clin Microbiol Infection (2022). Online ahead of print. doi: 10.1016/j.cmi.2022.08.010
137. Perez-Carrasco V, Soriano-Lerma A, Soriano M, Gutiérrez-Fernández J, Garcia-Salcedo JA. Urinary microbiome: Yin and yang of the urinary tract. Front Cell Infect Microbiol (2021) 11:617002. doi: 10.3389/fcimb.2021.617002
138. Brown SE, Robinson CK, Shardell MD, Holm JB, Ravel J, Ghanem KG, et al. Assessing the concordance between urogenital and vaginal microbiota: Can urine specimens be used as a proxy for vaginal samples? Front Cell Infect Microbiol (2021) 11:671413. doi: 10.3389/fcimb.2021.671413
139. Flores-Mireles AL, Walker JN, Caparon M, Hultgren SJ. Urinary tract infections: epidemiology, mechanisms of infection and treatment options. Nat Rev Microbiol (2015) 13(5):269–84. doi: 10.1038/nrmicro3432
140. Magruder M, Sholi AN, Gong C, Zhang L, Edusei E, Huang J, et al. Gut uropathogen abundance is a risk factor for development of bacteriuria and urinary tract infection. Nat Commun (2019) 10(1):5521. doi: 10.1038/s41467-019-13467-w
141. Gilbert NM, O'Brien VP, Waller C, Batourina E, Mendelsohn CL, Lewis AL. Gardnerella exposures alter bladder gene expression and augment uropathogenic escherichia coli urinary tract infection in mice. Front Cell Infect Microbiol (2022) 12:909799. doi: 10.3389/fcimb.2022.909799
142. Sumati AH, Saritha NK. Association of urinary tract infection in women with bacterial vaginosis. J Glob Infect Dis (2009) 1(2):151–2. doi: 10.4103/0974-777x.56254
143. Stapleton AE, Au-Yeung M, Hooton TM, Fredricks DN, Roberts PL, Czaja CA, et al. Randomized, placebo-controlled phase 2 trial of a lactobacillus crispatus probiotic given intravaginally for prevention of recurrent urinary tract infection. Clin Infect Dis (2011) 52(10):1212–7. doi: 10.1093/cid/cir183
144. Suárez LJ, Arboleda S, Angelov N, Arce RM. Oral versus gastrointestinal mucosal immune niches in homeostasis and allostasis. Front Immunol (2021) 12:705206. doi: 10.3389/fimmu.2021.705206
145. Lamont RJ, Koo H, Hajishengallis G. The oral microbiota: dynamic communities and host interactions. Nat Rev Microbiol (2018) 16(12):745–59. doi: 10.1038/s41579-018-0089-x
146. Mark Welch JL, Rossetti BJ, Rieken CW, Dewhirst FE, Borisy GG. Biogeography of a human oral microbiome at the micron scale. Proc Natl Acad Sci U S A (2016) 113(6):E791–800. doi: 10.1073/pnas.1522149113
147. Wilbert SA, Mark Welch JL, Borisy GG. Spatial ecology of the human tongue dorsum microbiome. Cell Rep (2020) 30(12):4003–15.e3. doi: 10.1016/j.celrep.2020.02.097
148. Tuganbaev T, Yoshida K, Honda K. The effects of oral microbiota on health. Science (2022) 376(6596):934–6. doi: 10.1126/science.abn1890
149. Peng X, Cheng L, You Y, Tang C, Ren B, Li Y, et al. Oral microbiota in human systematic diseases. Int J Oral Sci (2022) 14(1):14. doi: 10.1038/s41368-022-00163-7
150. Zabor EC, Klebanoff M, Yu K, Zhang J, Nansel T, Andrews W, et al. Association between periodontal disease, bacterial vaginosis, and sexual risk behaviours. J Clin Periodontol (2010) 37(10):888–93. doi: 10.1111/j.1600-051X.2010.01593.x
151. Persson R, Hitti J, Verhelst R, Vaneechoutte M, Persson R, Hirschi R, et al. The vaginal microflora in relation to gingivitis. BMC Infect Dis (2009) 9(1):1–8. doi: 10.1186/1471-2334-9-6
152. Balle C, Esra R, Havyarimana E, Jaumdally SZ, Lennard K, Konstantinus IN, et al. Relationship between the oral and vaginal microbiota of south African adolescents with high prevalence of bacterial vaginosis. Microorganisms (2020) 8(7):1004. doi: 10.3390/microorganisms8071004
153. Murphy K, Mitchell CM. The interplay of host immunity, environment and the risk of bacterial vaginosis and associated reproductive health outcomes. J Infect Dis (2016) 214 Suppl 1(Suppl 1):S29–35. doi: 10.1093/infdis/jiw140
154. Pavlova SI, Tao L. Induction of vaginal lactobacillus phages by the cigarette smoke chemical benzo[a]pyrene diol epoxide. Mutat Res (2000) 466(1):57–62. doi: 10.1016/s1383-5718(00)00003-6
155. Ali A, Jørgensen JS, Lamont RF. The contribution of bacteriophages to the aetiology and treatment of the bacterial vaginosis syndrome. Fac Rev (2022) 11:8. doi: 10.12703/r/11-8
156. Terzic M, Aimagambetova G, Terzic S, Radunovic M, Bapayeva G, Laganà AS. Periodontal pathogens and preterm birth: Current knowledge and further interventions. Pathogens (2021) 10(6):730. doi: 10.3390/pathogens10060730
157. Fardini Y, Chung P, Dumm R, Joshi N, Han YW. Transmission of diverse oral bacteria to murine placenta: evidence for the oral microbiome as a potential source of intrauterine infection. Infect Immun (2010) 78(4):1789–96. doi: 10.1128/iai.01395-09
158. Barak S, Oettinger-Barak O, Machtei EE, Sprecher H, Ohel G. Evidence of periopathogenic microorganisms in placentas of women with preeclampsia. J Periodontol (2007) 78(4):670–6. doi: 10.1902/jop.2007.060362
159. Zaura E, Nicu EA, Krom BP, Keijser BJ. Acquiring and maintaining a normal oral microbiome: current perspective. Front Cell infection Microbiol (2014) 4:85. doi: 10.3389/fcimb.2014.00085
160. Swati P, Ambika Devi K, Thomas B, Vahab SA, Kapaettu S, Kushtagi P. Simultaneous detection of periodontal pathogens in subgingival plaque and placenta of women with hypertension in pregnancy. Arch Gynecol Obstet (2012) 285(3):613–9. doi: 10.1007/s00404-011-2012-9
161. Katz J, Chegini N, Shiverick KT, Lamont RJ. Localization of p. gingivalis preterm delivery placenta. J Dent Res (2009) 88(6):575–8. doi: 10.1177/0022034509338032
162. Komine-Aizawa S, Aizawa S, Hayakawa S. Periodontal diseases and adverse pregnancy outcomes. J Obstet Gynaecol Res (2019) 45(1):5–12. doi: 10.1111/jog.13782
163. Rutsch A, Kantsjö JB, Ronchi F. The gut-brain axis: How microbiota and host inflammasome influence brain physiology and pathology. Front Immunol (2020) 11:604179. doi: 10.3389/fimmu.2020.604179
164. Cryan JF, O'Riordan KJ, Cowan CSM, Sandhu KV, Bastiaanssen TFS, Boehme M, et al. The microbiota-Gut-Brain axis. Physiol Rev (2019) 99(4):1877–2013. doi: 10.1152/physrev.00018.2018
165. Neuhuber WL, Berthoud HR. Functional anatomy of the vagus system - emphasis on the somato-visceral interface. Auton Neurosci (2021) 236:102887. doi: 10.1016/j.autneu.2021.102887
166. Padgett DA, Glaser R. How stress influences the immune response. Trends Immunol (2003) 24(8):444–8. doi: 10.1016/s1471-4906(03)00173-x
167. Amabebe E, Anumba DOC. Psychosocial stress, cortisol levels, and maintenance of vaginal health. Front Endocrinol (Lausanne) (2018) 9:568. doi: 10.3389/fendo.2018.00568
168. Nansel TR, Riggs MA, Yu KF, Andrews WW, Schwebke JR, Klebanoff MA. The association of psychosocial stress and bacterial vaginosis in a longitudinal cohort. Am J Obstet Gynecol (2006) 194(2):381–6. doi: 10.1016/j.ajog.2005.07.047
169. Harville EW, Savitz DA, Dole N, Thorp JM Jr., Herring AH. Psychological and biological markers of stress and bacterial vaginosis in pregnant women. Bjog (2007) 114(2):216–23. doi: 10.1111/j.1471-0528.2006.01209.x
170. Song SD, Acharya KD, Zhu JE, Deveney CM, Walther-Antonio MRS, Tetel MJ, et al. Daily vaginal microbiota fluctuations associated with natural hormonal cycle, contraceptives, diet, and exercise. mSphere (2020) 5(4):e00593–20. doi: 10.1128/mSphere.00593-20
171. Cheng CK, Leung PC. Molecular biology of gonadotropin-releasing hormone (GnRH)-I, GnRH-II, and their receptors in humans. Endocr Rev (2005) 26(2):283–306. doi: 10.1210/er.2003-0039
172. Howles CM. Role of LH and FSH in ovarian function. Mol Cell Endocrinol (2000) 161(1-2):25–30. doi: 10.1016/s0303-7207(99)00219-1
173. García-Solares J, Donnez J, Donnez O, Dolmans MM. Pathogenesis of uterine adenomyosis: invagination or metaplasia? Fertil Steril (2018) 109(3):371–9. doi: 10.1016/j.fertnstert.2017.12.030
174. Lebovic DI, Mueller MD, Taylor RN. Immunobiology of endometriosis. Fertility sterility (2001) 75(1):1–10. doi: 10.1016/s0015-0282(00)01630-7
175. Morelli M, Rocca ML, Venturella R, Mocciaro R, Zullo F. Improvement in chronic pelvic pain after gonadotropin releasing hormone analogue (GnRH-a) administration in premenopausal women suffering from adenomyosis or endometriosis: a retrospective study. Gynecol Endocrinol (2013) 29(4):305–8. doi: 10.3109/09513590.2012.743017
176. Aminzadeh Z, Fadaeian A. Reactive arthritis induced by bacterial vaginosis: prevention with an effective treatment. Int J Prev Med (2013) 4(7):841–4.
177. Kishikawa T, Maeda Y, Nii T, Motooka D, Matsumoto Y, Matsushita M, et al. Metagenome-wide association study of gut microbiome revealed novel aetiology of rheumatoid arthritis in the Japanese population. Ann Rheum Dis (2020) 79(1):103–11. doi: 10.1136/annrheumdis-2019-215743
178. Kishikawa T, Maeda Y, Nii T, Okada Y. Response to: 'Can sexual dimorphism in rheumatoid arthritis be attributed to the different abundance of gardnerella?' by liu et al. Ann Rheum Dis (2022) 81(3):e37. doi: 10.1136/annrheumdis-2020-217264
179. Selmi C, Gershwin ME. Diagnosis and classification of reactive arthritis. Autoimmun Rev (2014) 13(4-5):546–9. doi: 10.1016/j.autrev.2014.01.005
180. Schapira D, Braun-Moscovici Y, Nahir AM. Reactive arthritis induced by gardnerella vaginalis. Clin Exp Rheumatol (2002) 20(5):732–3.
181. Lev-Sagie A, Goldman-Wohl D, Cohen Y, Dori-Bachash M, Leshem A, Mor U, et al. Vaginal microbiome transplantation in women with intractable bacterial vaginosis. Nat Med (2019) 25(10):1500–4. doi: 10.1038/s41591-019-0600-6
182. Antonio MA, Meyn LA, Murray PJ, Busse B, Hillier SL. Vaginal colonization by probiotic lactobacillus crispatus CTV-05 is decreased by sexual activity and endogenous lactobacilli. J Infect Dis (2009) 199(10):1506–13. doi: 10.1086/598686
183. Cohen CR, Wierzbicki MR, French AL, Morris S, Newmann S, Reno H, et al. Randomized trial of lactin-V to prevent recurrence of bacterial vaginosis. N Engl J Med (2020) 382(20):1906–15. doi: 10.1056/NEJMoa1915254
184. Suez J, Zmora N, Zilberman-Schapira G, Mor U, Dori-Bachash M, Bashiardes S, et al. Post-antibiotic gut mucosal microbiome reconstitution is impaired by probiotics and improved by autologous FMT. Cell (2018) 174(6):1406–23.e16. doi: 10.1016/j.cell.2018.08.047
185. Suvorov A, Karaseva A, Kotyleva M, Kondratenko Y, Lavrenova N, Korobeynikov A, et al. Autoprobiotics as an approach for restoration of personalised microbiota. Front Microbiol (2018) 9:1869. doi: 10.3389/fmicb.2018.01869
186. Gromova LV, Ermolenko EI, Sepp AL, Dmitrieva YV, Alekseeva AS, Lavrenova NS, et al. Gut digestive function and microbiome after correction of experimental dysbiosis in rats by indigenous bifidobacteria. Microorganisms (2021) 9(3):522. doi: 10.3390/microorganisms9030522
187. Mel'nikov V, Stulova S, Tyumina O, Denisova N, Shchukin VY. Autotransplantation of lactobacilli in the vagina biocenosis restoration of individual women. Fundamental'nye issledovaniya= Fundam Res (2012) 1:64–7.
188. Garcia-Bayona L, Comstock LE. Bacterial antagonism in host-associated microbial communities. Science (2018) 361(6408):eaat2456. doi: 10.1126/science.aat2456
189. Callahan BJ, McMurdie PJ, Rosen MJ, Han AW, Johnson AJ, Holmes SP. DADA2: High-resolution sample inference from illumina amplicon data. Nat Methods (2016) 13(7):581–3. doi: 10.1038/nmeth.3869
190. Lee JY, Tsolis RM, Bäumler AJ. The microbiome and gut homeostasis. Science (2022) 377(6601):eabp9960. doi: 10.1126/science.abp9960
191. Duan Y, Zeng L, Zheng C, Song B, Li F, Kong X, et al. Inflammatory links between high fat diets and diseases. Front Immunol (2018) 9:2649. doi: 10.3389/fimmu.2018.02649
192. Dabke K, Hendrick G, Devkota S. The gut microbiome and metabolic syndrome. J Clin Invest (2019) 129(10):4050–7. doi: 10.1172/jci129194
193. Mirzaei R, Afaghi A, Babakhani S, Sohrabi MR, Hosseini-Fard SR, Babolhavaeji K, et al. Role of microbiota-derived short-chain fatty acids in cancer development and prevention. BioMed Pharmacother (2021) 139:111619. doi: 10.1016/j.biopha.2021.111619
194. Weyers S, Verstraelen H, Gerris J, Monstrey S, Santiago Gdos S, Saerens B, et al. Microflora of the penile skin-lined neovagina of transsexual women. BMC Microbiol (2009) 9:102. doi: 10.1186/1471-2180-9-102
195. Winston McPherson G, Long T, Salipante SJ, Rongitsch JA, Hoffman NG, Stephens K, et al. The vaginal microbiome of transgender men. Clin Chem (2019) 65(1):199–207. doi: 10.1373/clinchem.2018.293654
196. Baldassarre M, Giannone FA, Foschini MP, Battaglia C, Busacchi P, Venturoli S, et al. Effects of long-term high dose testosterone administration on vaginal epithelium structure and estrogen receptor-α and -β expression of young women. Int J Impot Res (2013) 25(5):172–7. doi: 10.1038/ijir.2013.9
197. Kaufmann U, Domig KJ, Lippitsch CI, Kraler M, Marschalek J, Kneifel W, et al. Ability of an orally administered lactobacilli preparation to improve the quality of the neovaginal microflora in male to female transsexual women. Eur J Obstet Gynecol Reprod Biol (2014) 172:102–5. doi: 10.1016/j.ejogrb.2013.10.019
198. Krakowsky Y, Potter E, Hallarn J, Monari B, Wilcox H, Bauer G, et al. The effect of gender-affirming medical care on the vaginal and neovaginal microbiomes of transgender and gender-diverse people. Front Cell Infect Microbiol (2021) 11:769950. doi: 10.3389/fcimb.2021.769950
Keywords: female reproductive tract, microbiota, vagina, uterus, ovary, gut, brain, oral cavity
Citation: Takada K, Melnikov VG, Kobayashi R, Komine-Aizawa S, Tsuji NM and Hayakawa S (2023) Female reproductive tract-organ axes. Front. Immunol. 14:1110001. doi: 10.3389/fimmu.2023.1110001
Received: 28 November 2022; Accepted: 16 January 2023;
Published: 31 January 2023.
Edited by:
Gerard Kaiko, The University of Newcastle, AustraliaReviewed by:
Emily C. Hoedt, The University of Newcastle, AustraliaZili Lei, Guangdong Pharmaceutical University, China
Copyright © 2023 Takada, Melnikov, Kobayashi, Komine-Aizawa, Tsuji and Hayakawa. This is an open-access article distributed under the terms of the Creative Commons Attribution License (CC BY). The use, distribution or reproduction in other forums is permitted, provided the original author(s) and the copyright owner(s) are credited and that the original publication in this journal is cited, in accordance with accepted academic practice. No use, distribution or reproduction is permitted which does not comply with these terms.
*Correspondence: Kazuhide Takada, takada.kazuhide@nihon-u.ac.jp; Satoshi Hayakawa, hayakawa.satoshi@nihon-u.ac.jp
†These authors have contributed equally to this work