- 1TAXPO—Laboratório de Taxonomia de Porifera, Departamento de Invertebrados, Museu Nacional, Universidade Federal do Rio de Janeiro, Rio de Janeiro, Brazil
- 2Laboratório de Microbiologia, Departamento de Biologia Marinha, Instituto de Biologia, Universidade Federal do Rio de Janeiro, Rio de Janeiro, Brazil
- 3Instituto de Pesquisas Jardim Botânico do Rio de Janeiro, Rio de Janeiro, Brazil
Two new Arenosclera are described here on the basis of materials obtained from Amazon reefs in 2014, A. amazonensis sp. nov. and A. klausi sp. nov. Both are clearly distinct from all other Arenosclera by their erect, solid funnel to lamellate habit, larger oxeas, and ectosomal architecture bearing occasional multispicular tracts. An integrative approach to find the best classification for both new species failed to group them and A. heroni, the genus' type species. Nearly complete 28S rRNA sequences obtained from these species' metagenomes suggested instead a better placement for the new species and A. brasiliensis in clade C (sensu Redmond et al., 2013), while A. heroni fits best in clade A. We propose to name three clades according to the rules of the PhyloCode: Arenospiculap, Dactyclonap, and Dactyspiculap, respectively for the clade originating with the most recent common ancestor of the three Brazilian Arenosclera spp.; the most inclusive clade containing Dactylia varia (Gray, 1843) and Haliclona curacaoensis (van Soest, 1980); and the least inclusive clade containing Arenospiculap and Dactyclonap. A Karlin dinucleotide dissimilarity analysis of metagenomes carried out on cryopreserved samples recognized A. amazonensis sp. nov. as the most dissimilar species, thus suggesting a more particular microbiota is present in this Amazon species, an open avenue for extended applied study of this holobiont.
Introduction
“Integrative taxonomy” is defined as the science that aims to delimit the units of life's diversity from multiple and complementary perspectives (phylogeography, comparative morphology, population genetics, ecology, development, behavior; Dayrat, 2005). Although, traditional procedures remain useful in many cases, taxonomy has to be pluralistic and integrate new approaches for species delimitation, which appears to be the most reliable way to evaluate the specific status of specimens (Padial et al., 2010), thus permitting arrival at sounder diagnoses (Schlick-Steiner et al., 2010). It has been said that morphological data are frequently insufficient to resolve taxonomic questions in Porifera, as a consequence of their great simplicity coupled to great intraspecific variability. Molecular, cytological, chemical, biogeographic, and ecologic characters are increasingly used in an integrative manner to help solve taxonomic dilemmas (Boury-Esnault et al., 2013). Today, this approach is generally accepted as the best way to answer the challenges of sponge systematics (Bergquist, 1994; Cárdenas et al., 2012).
The Haplosclerida Topsent, 1928 is a case in point. This is currently the third largest order in Porifera, with nearly 1,100 described species (Morrow and Cárdenas, 2015; van Soest, 2015). A growing body of evidence (McCormack et al., 2002; Raleigh et al., 2007; Redmond et al., 2007, 2011, 2013), highlights a staggering mismatch between the order's currently accepted morphology-based classification (van Soest, 2015) and its phylogenetic framework, as retrieved from 18S and 28S rRNA, and the COX I and NAD I gene sequences. The order is subdivided in five extant families, namely Callyspongiidae de Laubenfels, 1936; Chalinidae Gray, 1867; Niphatidae van Soest, 1980; Petrosiidae van Soest, 1980; and Phloeodictyidae Carter, 1882; and coincidently, in five main clades, viz. A–E, alas bearing no correspondence to the Linnean classification. The identification of haplosclerid sponges, thus carries a presently unavoidable ambiguity, where Linnean names are needed for practical purposes, and rank-free phylogenetic names (following the PhyloCode, www.ohio.edu/phylocode/preface.html) for narrowing the gap between names and clades.
In dealing with the haplosclerid sponges dredged off the mouth of the Amazon, we needed workable identifications, as well as an understanding of the phylogenetic affinities of these species. Among these, two Arenosclera spp. caught our attention, because the genus' type species, A. heroni, already integrates a molecular phylogeny based on nearly complete 28S rRNA, thus indicating a good marker to search for the affinities of the Amazon reef species. Furthermore, the detection of a particular class of tetracyclic alkylpiperidine alkaloids (the Arenosclerines and Haliclonaciclamines) in the Australian Haliclona sp. (Charana et al., 1996), and the Brazilian A. brasiliensis and Pachychalina alcaloidifera Pinheiro et al., 2005 (Torres et al., 2000; Oliveira et al., 2007), suggests that a deepened study of the taxonomy, phylogeny, metagenomics and metabolomics of Arenosclera spp. from the Amazon mouth may yield rewarding results, both in the natural products chemistry field, as well as possibly in chemosystematics. The distribution of these alkaloids cuts through three separate families, respectively Chalinidae, Callyspongiidae, and Niphatidae.
The objective of this paper is to identify and assess the phylogenetic relationships for the Arenosclera spp. from the reef systems off the mouth of the Amazon, using complete or nearly complete 28S sequences retrieved from their metagenomes, and to provide full descriptions of these sponges.
Materials and Methods
Study Area and Sampling
The Amazon River mouth drains an enormous sedimentary basin carrying a massive load of siliciclastic sediment of Andean origin, suspended in 20% of the freshwater input in the oceans (Lentz and Limeburner, 1995). A large, low salinity plume (up to 2 × 106 km2) moves northwest influencing the insular environment in the Southeast Caribbean (Lumpkin and Garzoli, 2005). The seabed in this area presents a regionalization. The central and southern areas are predominantly carbonate, while the northern area suffers more direct influence of the Amazon plume and its terrigenous sedimentation (Collette and Rützler, 1977; Moura et al., 2016). The confluence between the Amazon River and the Atlantic Ocean generates high fisheries production in this relatively shallow and wide shelf, including important demersal resources (fishes and crustaceans), associated to reefal environments (Moura et al., 2016).
An oceanographic expedition was carried in the Amazon mouth with the Brazilian Navy ship NHo “Cruzeiro do Sul” in 24–29 September 2014. A total of 90 specimens were collected by bottom trawls and dredges. The specimens were sorted, photographed and labeled on ship before fixation in 92% ethanol. After that, they were deposited in the Porifera Collection of Museu Nacional—UFRJ (MNRJ). A fragment of each species sorted on board was preserved in liquid N2, but one of the Arenosclera spp. later recognized as new (namely A. klausi sp. nov. described below), was not cryopreserved because both were thought conspecific.
Taxonomy and Morphological Study
Samples were identified based on microscopic preparations of dissociated spicules, and thick anatomical sections obtained from fragments. These procedures are described in detail in Hajdu et al. (2011). For each specimen 100 oxeas were randomly selected and measured, unless stated otherwise. Dissociated spicules were also analyzed in a JEOL JSM6390LV Scanning Electron Microscope (SEM). Spicule dimensions are presented in micrometers as minimum–mean–maximum (±standard deviation). New species were compared with the specimens listed in Table 1.
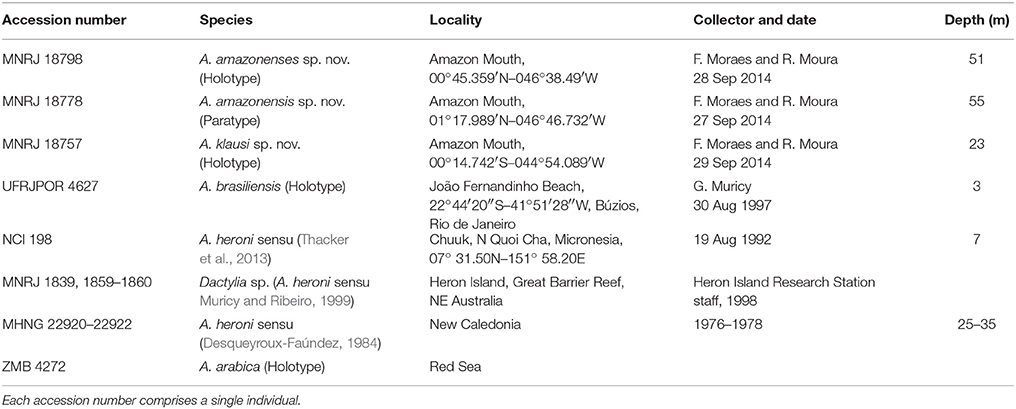
Table 1. List of the studied specimens and comparative materials used to assess the phylogenetic relationships of Amazon Arenosclera spp.
Nomenclatural Acts
The new species described in this paper are registered online at ZooBank (http://zoobank.org/) as requested by the International Code of Zoological Nomenclature. Each species received a Life Science Identifier (LSID) discriminated above.
DNA Extraction, Sequencing, and Quality Control of the Metagenomes
Fragments of specimens were washed in filtered sea water to remove all microorganisms attached to the sponge's outer surface. Afterwards, samples were macerated and embedded in Solution A [CTBA—2% + 100 mM EDTA + 1.4 M NaCl + 100 mM Tris-HCL (pH 8.0) + 2.0 μL Beta-mercaptanol + 0.5 μL proteinase K a 20 mg/mL]. Three heat-shock cycles were made (65°C by 3 min and −80°C by 30 min). After that, 1 ml of phenol:chloroform:isoamilic Alcohol (25:24:1) was added, and centrifuged in 13,400 rpm for 10 min at 4°C. The supernatant was then transferred to a new tube, and the spin filters from PowerSoil DNA Isolation Kit (MoBio, USA) were used to further purify the solution containing nucleic acids (Garcia et al., 2013).
Next generation sequences were obtained through HiSeq (Illumina, USA) by the staff of Laboratório de Microbiologia (at Departamento de Biologia Marinha/UFRJ). DNA libraries were prepared using Nextera XT DNA Sample Preparation Kit (Illumina, USA), that uses a tagmentation reaction for transposon cleaving and tagging of the double-stranded DNA with a universal adapter, followed by a limited cycle PCR to add primer sequences and indices. The size distribution of reads in each library was evaluated with a 2100 Bioanalyzer and its High Sensitivity DNA Kit (Agilent, USA). The accurate quantification of the libraries was accomplished using the 7500 Real Time PCR (Applied Biosystems, USA) and the KAPA Library Quantification Kit (Kapa Biosystems, USA). Paired-end sequencing (2 × 150 bp) was performed using the kits TruSeq® Rapid SBS Kit–HS (50 cycles and 200 cycles) and TruSeq® Rapid PE Cluster Kit–HS. Quality control of the metagenomes was undertaken with the PRINSEQ software (Standalone Lite Version 0.20.4; Schmieder and Edwards, 2011) by removal of sequences presenting Phred score lower than 30 (Q < 30), and with duplicate reads. Paired-end reads were merged using PEAR (Zhang et al., 2014) with a base Phred quality score of 20.
Besides Brazilian Arenosclera, we used the metagenomes of Amphimedon compressa Duchassaing and Michelotti, 1864 and Callyspongia vaginalis (Lamarck, 1814) collected in the same expedition, as controls to verify if there is influence of the environmental microbiome in this analysis. In addition, these species are important for the recovery of the phylogenetic affinities of Arenosclera as shown below. The biological materials available for each of these species are illustrated as a Figure S1. Sequences of the 28S rRNA from Arenosclera spp. nov., Arenosclera brasiliensis, A. compressa, and C. vaginalis were submitted to Genbank (Table 2). Arenosclera brasiliensis materials used here came from the Cabo Frio region (Rio de Janeiro, Brazil), and had been reported upon by Trindade-Silva et al. (2012). This metagenome originated from SANGER sequencing which generates larger reads (>500 bp). All other metagenomes were generated by HiSeq (Illumina, USA), and are thus composed of smaller reads.
Karlin's Signatures
Karlin's signatures compare the relative abundances of dinucleotides in different sequences (Karlin and Burge, 1995), and were used in the comparison among Amazon reef sponges' metagenomes and those obtained by Trindade-Silva et al. (2012) for A. brasiliensis. Frequency tabulation of the sequence data was performed using homemade Perl scripts according to Willner et al. (2009).
Phylogeny
We chose as genetic marker the 28S because it is a well-established gene for evolutionary studies with sponges, and this is the only sequence available from the type species of Arenosclera. The 28S sequences used were recovered from metagenomes compared to a referential database compiling all Genbank sequences showing over 80% similarity to the available nearly complete 28S sequence of Arenosclera heroni (2,488 bp; Thacker et al., 2013). Sequences compiled for this database were KC869461, KC869526, KC869599, KC869567, KC869609, KC869497, KC869562, KC869607, KC869455, AB511881, KC869527, KC869473, AY561893, KC869622, KC869553, KC869626, KC869626, KC869620, KC869460 from Genbank. Contigs were aligned against the 28S referential database created using BLASTN algorithm with at least 70% query coverage and an E-value cut-off of 10−5 (Altschul et al., 1990). We compared the metagenomes of A. brasiliensis, C. vaginalis, A. compressa, and the two new Arenosclera sponges from Amazon reefs. SPAdes software (v. 3.5; Bankevich et al., 2012) was used as a first approach to assemble the high quality short reads (<300 bp), followed by a second approach using CAP3 software (Huang and Madan, 1999).
Additional sequences reported in Thacker et al. (2013) were obtained from GenBank to settle our comparative nearly complete 28S rRNA dataset. We refrained from using further 28S GenBank sequences for the Haplosclerida because in general these were much smaller than the sequences we were working with, and spurious preliminary results suggested that extensive discussion of phylogenetic affinities within this order might be needed, going way beyond the scope of this contribution. A global alignment was performed using MAFFT software (version 7; Katoh and Standley, 2013) with FFT-NS-2 parameter. Gaps were only eliminated when occurring on 20% or more of analyzed sequences. Maximum Likelihood analysis was run in RAxML (Stamatakis, 2014) in CIPRES platform (Miller et al., 2010) using the substitutions model GTR+GAMMA+I selected by Model Generator (Keane et al., 2006) with 1,000 bootstrap.
Results
We have found two new species of Haplosclerida, morphologically similar to known species of Arenosclera (see diagnosis below), and perfectly matching the genus definition proposed in the latest revised classification of the Callyspongiidae (Desqueyroux-Faúndez and Valentine, 2002). According to the 28S phylogeny obtained from our own and previously published sequences (Figure 1), the Amazon reefs' A. amazonensis sp. nov. and A. klausi sp. nov. (described below) cluster with A. brasiliensis with 100% bootstrap support. This clade of Brazilian Arenosclera sponges paired with Dactylia varia and Haliclona curacaoensis with 70% bootstrap support. A more inclusive clade with 69% bootstrap support comprises the latter, Dasychalina melior (Niphatidae), and the sister pair Xestospongia sp. and Petrosia lignosa. Amphimedon compressa (Niphatidae) comes next as sister to this large previous clade, with 100% bootstrap support.
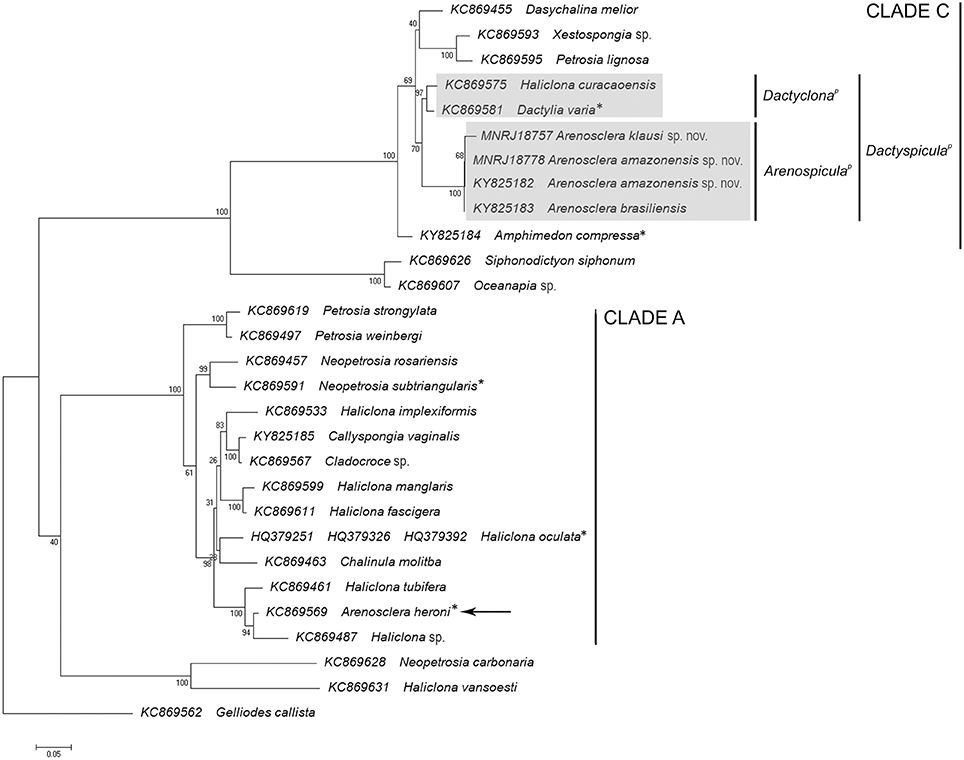
Figure 1. ML phylogeny based on 28S rRNA including nearly complete sequences blasted from the metagenomes of Arenosclera amazonensis sp. nov., Arenosclera klausi sp. nov., Amphimedon compressa, Callyspongia vaginalis, all species belonging to Haplosclerida in Thacker et al. (2013), and Haliclona oculata from Morrow et al. (2012), for which complete or nearly complete 28S sequences are available in Genbank. *Type species of the genus.
Arenosclera heroni, the genus' type species, was retrieved in the same relationships obtained by Thacker et al. (2013), in a highly supported clade comprising several species of Haliclona. This clade integrated a more inclusive one, also highly supported, with additional Haliclona spp., Chalinula molitba, and C. vaginalis. This later clade appears only distantly related to the clade including Brazilian Arenosclera sponges, a fact we take as best coped with at the moment, through the parallel erection of a new group, Arenospiculap, not yet recognizable on the basis of morphology alone, for which reason we refrain to erect a new higher taxon in the Linnean classification. Instead, we followed the PhyloCode rules and recommendations delineated in Cantino and de Queiroz (2010), which determine that the proposition of a branch-based definition, as done below for the clade containing the Brazilian Arenosclera spp., should be accompanied by proposition of another branch-based definition for the sister clade of the former (Recommendation 11E), and a node-based definition for the clade comprising both branch-based definitions (Recommendation 11F).
Table 3 lists the next-generation HiSeq (Illumina, USA) results. Karlin's signature of dinucleotides recognized a greater similarity in all six metagenomes of A. brasiliensis when compared to those of the other species analyzed. In addition, we clearly detected a divergence between A. amazonensis sp. nov. and A. brasiliensis (Figure 2). The former exhibited 20% dissimilarity toward all other species considered. The species pair A. compressa and C. vaginalis formed a separated group as well. Since A. klausi sp. nov. and the paratype of A. amazonensis sp. nov. were available only from ethanol preserved vouchers, it appeared to us inappropriate to compare their metagenomes to those of specimens which had gone through cryopreservation and permitted generation of considerably more data.
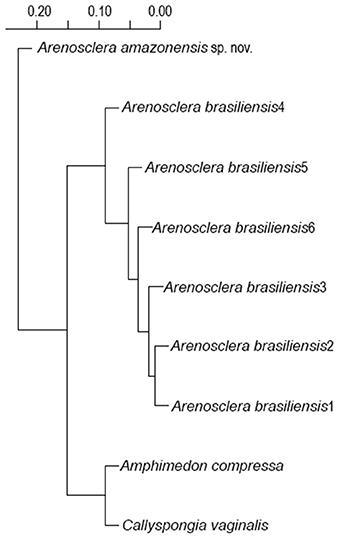
Figure 2. Hierarchical clustering of metagenomes from dinucleotide relative abundances. Metagenomes are labeled according to their sponge source.
Systematics
Class Demospongiae Sollas, 1885
Sub-class Heteroscleromorpha Cárdenas, Pérez and Boury-Esnault, 2012
Order Haplosclerida Topsent, 1928
Family Callyspongiidae de Laubenfels, 1936
Genus Arenosclera Pulitzer-Finali, 1982
Diagnosis: Callyspongiidae with ectosomal skeleton of sand and foreign debris cemented by scarce spongin, rounded meshes. Choanosomal fibers irregular, discontinuous, with foreign debris, proper spicules or both (Desqueyroux-Faúndez and Valentine, 2002).
Arenosclera amazonensis sp. nov. Leal, Moraes, Thompson, and Hajdu (Figure 3, Table 4)
LSID: urn:lsid:zoobank.org:act:28C5BD00-0AA4-4903-82ACBC1AAD666EA5
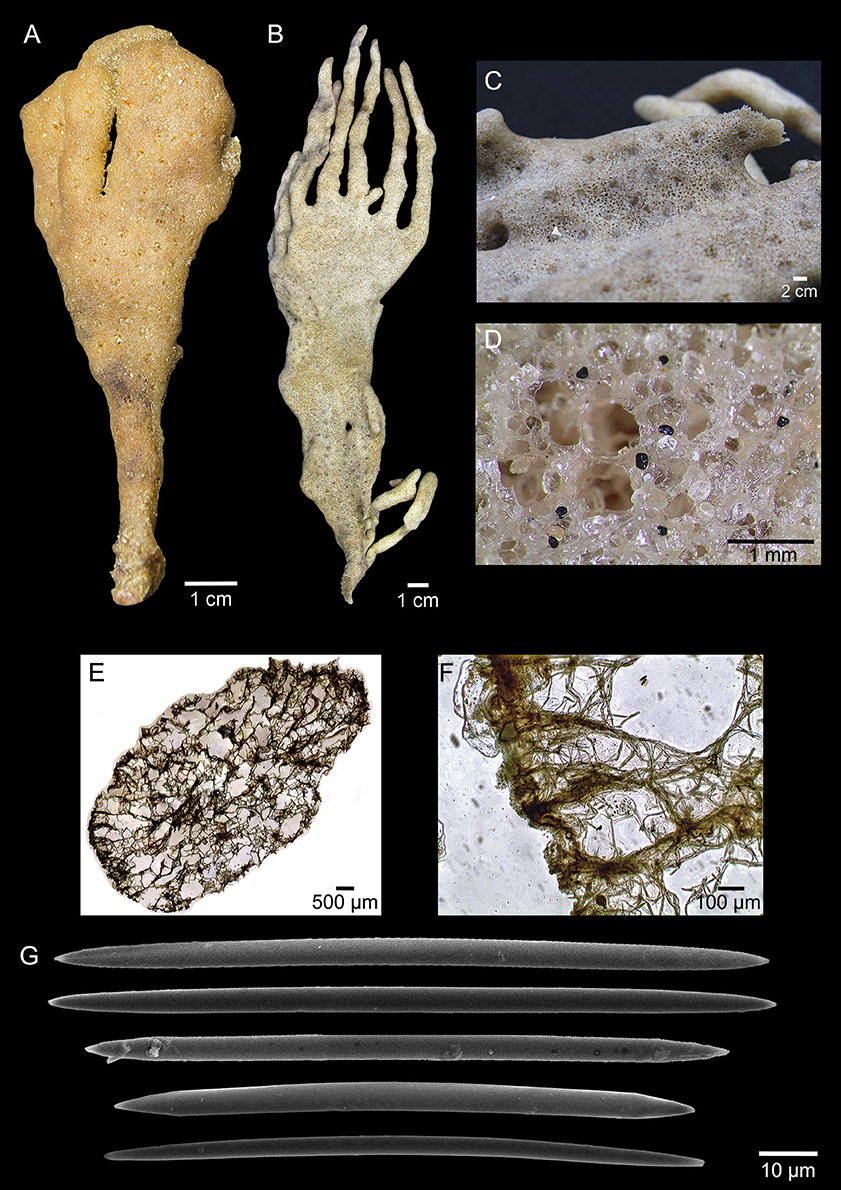
Figure 3. Arenosclera amazonensis sp. nov. (holotype, A, MNRJ 18778; paratype, B–F, MNRJ 18798) morphological and anatomical traits. (A–B) Fixed specimens. (C) Sponge surface with several oscules. (D) Detail of surface and oscule rim showing rounded siliceous sand grains incorporated to spongin fibers. (E) ectosome and choanosome in transverse section showing reticulated spongin fibers. (F) Detail of ectosome and choanosome in transverse section showing spicule tracts inside spongin fibers. (G) Oxeas (both specimens).
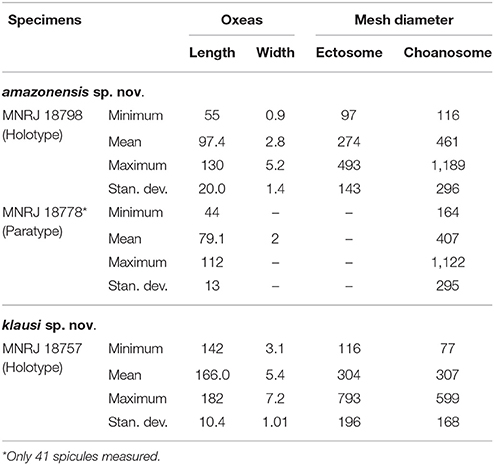
Table 4. Spicule micrometries and mesh diameter (μm) for the new species of Arenosclera reported here.
Type Material
Holotype
MNRJ 18798, Station #8, off Marajó Bay, Amazon River mouth, Pará State, Brazil (00°45.359′N–046°38.49′W), 51 m depth, coll. F. Moraes and R. Moura/NHo Cruzeiro do Sul, 28 September 2014.
Paratype
MNRJ 18778, Station #6, off Marajó Bay, Amazon River mouth, Pará State, Brazil (01°17.989′N–046°46.732′W), 55 m depth, coll. F. Moraes and R. Moura/ NHo Cruzeiro do Sul, 27 September 2014.
Diagnosis
Erect, solid funnel to lamellate, stalked habit. Oscula clustered in slightly concave circular regions, which are randomly arranged. Ectosome with spongin fibers cored by uni- to multispicular tracts (9.6–46 μm wide), forming irregular to rounded meshes (diam. 97–493 μm). Choanosome with spongin fibers forming irregular to rounded meshes (diam. 116–1189 μm). Abundant sand grains, among and in the fibers, more so in the ectosome. Oxeas reach 130 μm in the holotype.
Description
External Morphology
Erect, solid funnel to lamellate habit, slightly flattened, pedunculate sponge, measuring 25 × 8 × 1 cm (larger specimen) with digitiform projections (9–12 cm long) showing wider base than top. Color light brown to beige in vivo and after fixation. Consistency soft and elastic. Oscula are grouped in slightly concave, round areas (diam. 1–2 mm), which are dispersed over the sponge surface, or aligned on the edges of the projections. Surface regular, slightly rough to the touch, heavily cored by siliceous sand grains.
Skeleton
Ectosome formed by irregular to rounded meshes [diameter 97–274–493 (±143) μm] of spongin fibers cored by uni- to multi-spicular tracts [9.6–21.2–46 (±10) μm wide] and abundant sand grains, among and in the fibers. No clear distinction between primary and secondary fibers. Choanosome formed by irregular to rounded meshes [diameter 116–961–1189 (±296) μm] of spongin fibers cored by uni- to multispicular tracts [15–17.5–28 (±4.7) μm wide]. Sand and foreign debris, including exogenous spicules, dispersed among fibers in smaller quantity than the ectosome. Smallest oxeas dispersed in the free space in between meshes. No distinction between primary and secondary fibers.
Spicules
Oxeas straight to slightly curved (Holotype: 55–97.4–130 × 1–3–5 μm; Paratype: 44–79.1–112 × 2 μm).
Ecology
Specimens were rare and associated to rhodolith beds at 51–55 m depth. No organisms were recorded associated to this species.
Distribution
Known only from its type locality, the northern Brazilian continental shelf at the central sector off the Amazon River mouth (Pará, Brazil).
Etymology
In reference to the type locality, the Amazon River mouth.
Arenosclera klausi sp. nov. Leal, Moraes, Thompson, and Hajdu (Figure 4, Table 4)
LSID: urn:lsid:zoobank.org:act:16CBBD72-49FF-41A0-82E9-A2FA9D6C6930
Type Material
Holotype
MNRJ 18757, Station #10, off Mutuoca Bay, Maranhão State, Brazil (00°14.742′S-044°54.089′W), 23 m depth, coll. F. Moraes and R. Moura/NHo Cruzeiro do Sul, 29 September 2014.
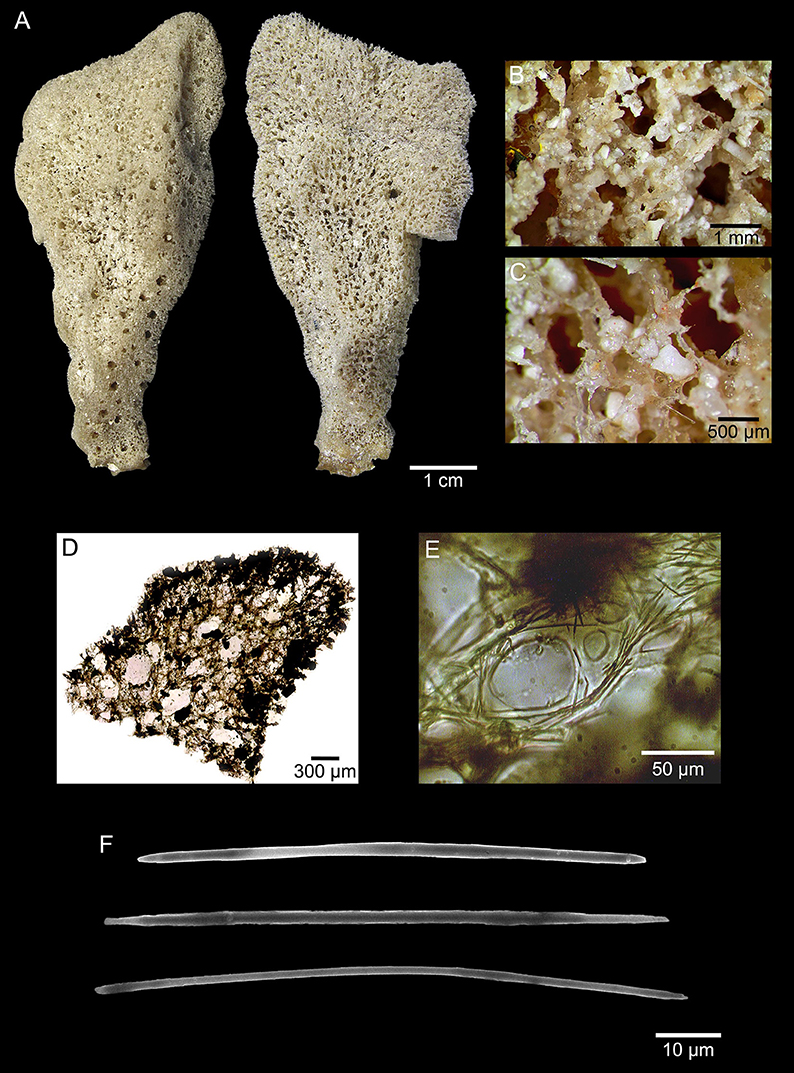
Figure 4. Arenosclera klausi sp. nov. (holotype, MNRJ 18757) morphological and anatomical traits. (A) Both sides of the fixed specimen. (B) Detail of sponge surface. (C) Detail of oscule rim and calcareous debris incorporated to spongin fibers. (D) Ectosome and choanosome transverse section. (E) Detail of choanosome spongin fibers cored by oxeas. (F) Oxeas.
Diagnosis
Erect, somewhat lamellate with a cylindrical stalk. Oscula flat on the surface, and concentrated on one edge of the sponge. Ectosome with spongin fibers cored by pauci- to multispicular tracts (7–22 μm wide), forming irregular to rounded meshes (diam. 116–793 μm). Choanosome with spongin fibers cored by pauci- to multispicular tracts (8–28 μm wide), forming irregular meshes (diam. 77–599 μm), becoming aspicular in the deeper parts of the sponge. Carbonate sand and foreign debris dispersed in large quantities among fibers all over the sponge. Only Arenosclera with larger oxeas attaining over 150 μm in length.
Description
Erect, somewhat lamellate sponge (7 × 3 × 1 cm), presenting a cylindrical stalk of 1 cm in diameter. Consistency soft and elastic, with softness increasing away from stalk. Oscula rounded (diameter 1–3 mm), flat on the surface, and concentrated on one edge of the sponge. Color beige in vivo (on deck) and after fixation. Surface irregular, slightly conulose, rough to the touch, heavily filled by foreign debris (mainly carbonate grains).
Skeleton
Ectosome formed by irregular to rounded meshes [diameter 116–304–793 (±196) μm] of spongin fibers cored by pauci- to multispicular tracts [7–13.7–22 (±4.2) μm wide]. Carbonate sand and foreign debris dispersed in large quantities among fibers. Primary and secondary fibers undistinguished. Choanosome formed by irregular meshes [diameter 77–307–599 (±168) μm] of spongin fibers cored by pauci- to multispicular tracts [8–14.9–28 (±6.8) μm wide] close to the ectosome, becoming aspicular in the deeper parts of the sponge. Sand and foreign debris, including exogenous spicules, are dispersed in large quantities among fibers. Primary and secondary fibers undistinguished.
Spicules
Oxeas thin, slightly curved, with irregular ends (142–166.0–182 × 3.1–5.4–7.2 μm).
Ecology
Rare species, with only one specimen collected, associated to coral-algal hard bottom at 23 m depth. No organisms were recorded associated to this species.
Distribution
Known only from its type locality, the northern Brazilian continental shelf at the south sector off the Amazon River mouth (Maranhão, Brazil).
Etymology
The species is named in honor of Dr. Klaus Rützler, a pioneer in the taxonomic study of sponges from off the Amazon River mouth.
PhyloCode
Arenospiculap (nomen cladi novum, stem-based)
The most inclusive clade containing A. brasiliensis, A. amazonensis sp. nov., and Arenosclera klausi sp. nov. Etymology: from the included Arenosclera spp. (Areno, = sand in Latin; spicula, = diminutive of point, spear in Latin).
Dactyclonap (nomen cladi novum, stem-based)
The most inclusive clade containing D. varia (Gray, 1843) and H. curacaoensis (van Soest, 1980). Etymology: from the included species D. varia (Dacty) and H. curacaoensis (clona).
Dactyspiculap (nomen cladi novum, node-based)
The least inclusive clade containing Arenospiculap and Dactyclonap. Etymology: from the included groups Dactyclonap (Dacty) and Arenospiculap (spicula).
Discussion
Identification of Arenosclera spp. nov
The state of uncertainty as regards higher taxa diagnoses in the Haplosclerida renders the assignment of new species to currently accepted generic names a taxonomic roulette. On the basis of the 18S marker, Redmond et al. (2013) retrieved not only polyphyletic families and genera, but also species such as Callyspongia fallax, C. vaginalis, H. curacaoensis, and H. tubifera. How can higher taxa boundaries in the Haplosclerida be discussed if species-limits remain ambiguous? Currently, one has to resort to morphology to recognize a new species' genus, regardless of the certainty of forthcoming major changes in higher taxa classification in the order; and to molecules for its clade A–E (Redmond et al., 2011, 2013), alas frequently not containing the genus' type species.
Accordingly, we observed the single available 28S sequence of A. heroni to cluster with two species of Haliclona, as originally retrieved by Thacker et al. (2013), instead of with the other Arenosclera spp. dealt with here. We had the opportunity to revise the voucher of Thacker et al.'s (Op. cit.) A. heroni (NCI 198), and found it to bear a clearly callyspongiid architecture, with a neat reticulation of spicule- and debris-cored fibers with abundant cementing spongin. However, our included C. vaginalis is inserted nowhere close to A. heroni. Rather, it groups with Cladocroce sp. with high support, and then, with moderate support to Haliclona implexiformis. Several clade A species reported in Redmond et al. (2013), namely C. molitba, Haliclona fascigera, H. manglaris, H. oculata, and H. tubifera integrate a larger clade including A. heroni with 87% bootstrap support. Still, the same two species of Neopetrosia (N. rosariensis and N. subtriangularis) and two species of Petrosia (P. strongylata and P. weinbergi), present in Redmond et al.'s (Op. cit.) clade A, are here placed in this same clade, and highly supported. Unfortunately, these authors were unsuccessful in retrieving an 18S sequence for A. heroni, which prevents further discussion on this genus' best phylogenetic assignment.
As pointed out above, all three Brazilian Arenosclera spp. clustered elsewhere, in a clade containing the type species of the niphatid genus Amphimedon. The same clade includes H. curacaoensis, a sponge of uncertain phylogenetic affinity (see above), and D, varia, allegedly a callyspongiid. The latter, given its lack of spicules, is at best taken as a possible callyspongiid. However, it is interesting to note that Redmond et al.'s (2013) clade C, including both niphatid species clustering with Brazilian Arenosclera spp., also includes Niphates erecta, the type species of Niphates. Thus, albeit indirectly so, reinforcing the niphatid affinity of these Arenosclera spp. Obviously, Arenosclera needs a thorough revision, considering not only the species above, but also other additional little-known species from the Indo-Pacific.
Comparison with Additional Arenosclera spp.
Table 1 lists the comparative haplosclerid materials used here. Specimens from Heron Island referred to, as A. heroni, by Muricy and Ribeiro (1999) were found to be better assigned to Dactylia, as not a single spicule could be found in them. Thus, aside from Pulitzer-Finali's (1982) original description, our comprehension of A. heroni's morphospace derives from hands-on study of Desqueyroux-Faúndez (1984) and Thacker et al.'s (2013) materials, alas, not topotypical. Both new species proposed appear quite distinct from this West Pacific species (Table 5) in overall habit (erect, somewhat lamellate), ectosomal architecture (commonly bearing uni- or pauci- to multispicular tracts), and usually considerably larger spicule dimensions (up to 112, 130, or 180 μm in the new species, against a maximum of 90 μm in A. heroni).
As simple as it is, these larger oxeas in the Amazon reefs' species set them apart from every other species previously assigned to Arenosclera (Table 5), where the maximum reported length for the oxeas was 108 μm in A. brasiliensis. The remaining Arenosclera spp. all possess oxeas smaller than 90 μm. Nevertheless, the paratype of A. amazonensis sp. nov. has comparatively smaller oxeas, thus coming quite close to the spectrum observable in A. brasiliensis. We are confident that both species are distinct as not a single (among hundreds observed by CVL and EH) A. brasiliensis possessed a lamellate-pedunculate body. Moreover, extensive surveys conducted along the Brazilian coast and its oceanic islands in the last couple decades, failed to spot any A. brasiliensis further north than 20°S, thus suggesting this species has a geographic range determined by the influence of SE Brazilian upwelling. This is in marked contrast to the Amazon environment were both new Arenosclera spp. were retrieved. While both new species proposed have flat, erect, somewhat lamellate morphologies, all other species considered bear massive to tubular habit (Table 5). In this way, we do not see risk of mistaken identifications, and the new species appear easy to recognize among other congeners.
Given the insertions of A. heroni, and the Brazilian Arenosclera spp. in our 28S phylogeny, monophyly of the remaining Arenosclera spp. is unlikely. This is an obvious assumption, consequence of the findings by McCormack et al. (2002), Raleigh et al. (2007), and Redmond et al. (2007, 2011, 2013), who have shown rampant polyphyletism of haplosclerid genera classified in every family. For instance, distribution of alkaloids such as arenosclerines and haliclonaciclamines cuts right through current genera and families. These compounds are present in A. brasiliensis (currently in the Callyspongiidae; Torres et al., 2000), Haliclona (Chalinidae; Charana et al., 1996) and Pachychalina (Niphatidae; Oliveira et al., 2007). It is thus clear that a major taxonomic revolution is necessary, unlikely to spare any such tool as detailed morphology, multi locus sponge genetics, metagenomics and metabolomics.
Preliminary Metagenome Data
The use of metagenomes as a data source for phylogenetic analyses in place of the traditional approach of isolation, amplification and sequencing of individual markers, proved a good strategy. Despite its currently larger cost, the advantages of producing a database for the genes of a given species, to be assessed at any time, without the need for further primers, isolation, amplification and sequencing, far outweighs any drawbacks. The characterization aimed at for the biome off the mouth of the Amazon river included metagenome analyses of the water column, sediments and biota (Moura et al., 2016), so that several sponge metagenomes had already been generated independently of any foreseen taxonomic necessity.
A curious outcome of our metagenome dinucleotide dissimilarity analyses is the observed 10% dissimilarity in the six available metagenomes of A. brasiliensis (Trindade-Silva et al., 2012), which is of the same magnitude as the value retrieved for A. compressa and C. vaginalis, species currently assigned to distinct families. These three species exhibit 15% dissimilarity, while A. amazonensis sp. nov. appears as most dissimilar. Given that all species, aside A. brasiliensis, were collected in the Amazon reefs area, it appears that neither a phylogenetic, nor an ecological signal were determinants for the observed dissimilarity.
One way to look at dissimilarity focuses on its translation into the uniqueness of the associated microbiota. As such, the higher the dissimilarity, the greater the potential for the finding of novelties, which suggests good avenues for the investigation of the chemo-pharmacological applicability of the associated microbiota in A. amazonensis sp. nov.
On the other hand, it is remarkable that despite originating from the same short stretch of SE Brazilian rocky coast, the six specimens of A. brasiliensis yielded 10% dissimilar metagenomes. This reminds of the importance to consider multiple samples when characterizing metagenomes, which has unfortunately not been possible here for many species considered, due to the limited number of duplicate and triplicate specimens obtained in the Amazon cruises.
Author Contributions
FT designed and coordinated the fieldwork. FM, AM, and AS took part in fieldwork. CL, FM, AF, AS, Ld, AM, FT, and EH contributed with data and data analyses. CL, FM, FT, and EH wrote and revised the paper.
Funding
Conselho Nacional de Desenvolvimento Científico e Tecnológico (CNPq), Coordenadoria de Aperfeiçoamento de Pessoal de Nível Superior (CAPES), Fundação Carlos Chagas Filho de Amparo à Pesquisa do Estado do Rio de Janeiro (FAPERJ), and ANP/Brasoil provided essential funding. MCTI and the Brazilian Navy provided support with the NHo Cruzeiro do Sul in 2014. Authors are thankful to the Gordon and Betty Moore Foundation for payment of processing fees.
Conflict of Interest Statement
The authors declare that the research was conducted in the absence of any commercial or financial relationships that could be construed as a potential conflict of interest.
The handling Editor declared a shared affiliation, though no other collaboration, with several of the authors, and the handling Editor states that the process met the standards of a fair and objective review.
Acknowledgments
We thank Gisele Lôbo-Hajdu and Thiago S. de Paula (UERJ), and Cristiano Lazoski and Cristiane C. Thompson (UFRJ), for guidance with the molecular analyses undertaken. Allen Collins (Smithsonian Institution, Washington, D.C.) and Shirley Pomponi (Harbor Branch Oceanographic Institute, Florida) are thanked for the provision of, respectively, access to the NCI fragment of A. heroni sequenced in Thacker et al. (2013), and granting of lab space for the study of this fragment.
Supplementary Material
The Supplementary Material for this article can be found online at: https://www.frontiersin.org/articles/10.3389/fmars.2017.00291/full#supplementary-material
Figure S1. (A) Amphimedon compressa Duchasssaing and Michelotti, 1864 (MNRJ 18771) and (B) Callyspongia vaginalis Lamarck, 1814 (MNRJ 18812) used to obtain HiSeq (Illumina, USA) metagenomes.
References
Altschul, S. F., Gish, W., Miller, W., Myers, E. W., and Lipman, D. J. (1990). Basic local alignment search tool. J. Mol. Biol. 215, 403–410. doi: 10.1016/S0022-2836(05)80360-2
Bankevich, A., Nurk, S., Antipov, D., Gurevich, A. A., Dvorkin, M., Kulikov, A. S., et al. (2012). SPAdes: a new genome assembly algorithm and its applications to single-cell sequencing. J. Comput. Biol. 19, 455–477. doi: 10.1089/cmb.2012.0021
Bergquist, P. R. (1994). “Onwards and upwards with sponges,” in Sponges in Time and Space, eds R. W. M. Van Soest, T. M. G. Van Kempen, and J. C. Braekman (Rotterdam: Balkema), XIII–XVIII.
Boury-Esnault, N., Lavrov, D. V., Ruiz, C. A., and Pérez, T. (2013). The integrative taxonomic approach applied to Porifera: a case study of the Homoscleromorpha. Integr. Comp. Biol. 53, 416–427. doi: 10.1093/icb/ict042
Cantino, P. D., and de Queiroz, K. (2010). International Code of Phylogenetic Nomenclature. Version 4c. Available online at: http://www.ohio.edu/phylocode/
Cárdenas, P., Pérez, T., and Boury-Esnault, N. (2012). Sponge systematics facing new challenges. Adv. Mar. Biol. 61, 79–209. doi: 10.1016/B978-0-12-387787-1.00010-6
Carter, H. J. (1882). Some sponges from the West Indies and Acapulco in the Liverpool free museum described, with general and classificatory remarks. Ann. Mag. Nat. Hist. 9, 266–368. doi: 10.1080/00222938209459039
Charana, R. D., Garsona, M. J., Breretonb, I. M., Willisc, A. C., and Hooper, J. N. A. (1996). Haliclonacyclamines A and B, cytotoxic alkaloids from the tropical marine sponge Haliclona sp. Tetrahedron 52, 9111–9120. doi: 10.1016/0040-4020(96)00436-X
Collette, B. B., and Rützler, K. (1977). “Reef fishes over sponge bottoms off the mouth of the Amazon river,” in Proceedings 3rd International Coral Reef Symposium, ed D. L. Taylor (University of Miami, Miami), 305–310.
Dayrat, B. (2005). Towards integrative taxonomy. Biol. J. Linn. Soc. 85, 407–415. doi: 10.1111/j.1095-8312.2005.00503.x
Desqueyroux-Faúndez, R. (1984). Description de la Faune des Haplosclerida (Porifera) de la Nouvelle-Calédonie. I. Niphatidae-Callyspongiidae. Rev. Suisse Zool. 91, 765–827. doi: 10.5962/bhl.part.81580
Desqueyroux-Faúndez, R., and Valentine, C. (2002). “Family Callyspongiidae,” in Systema Porifera: A Guide to the Classification of Sponges, eds J. N. A. Hooper and R. W. M. van Soest (New York, NY: Kluwer Academic/Plenum Publishers), 835–851.
Garcia, G. D., Gregoracci, G. B., Santos, E. O., Meirelles, P. M., Silva, G. G., Edwards, R., et al. (2013). Metagenomic analysis of healthy and white plague-affected Mussismilia braziliensis corals. Microb. Ecol. 65, 1076–1086. doi: 10.1007/s00248-012-0161-4
Gray, J. E. (1843). “Additional radiated animals and annelids,” in Travels in New Zealand; With Contributions to the Geography, Geology, Botany, and Natural History of that Country, ed E. Dieffenbach (London: Murray), 292–295.
Hajdu, E., Peixinho, S., and Fernandez, J. C. C. (2011). Esponjas Marinhas da Bahia. Guia de Campo e Laboratório. Rio de Janeiro: Museu Nacional.
Huang, X., and Madan, A. (1999). CAP3: a DNA sequence assembly program. Genome Res. 9, 868–877. doi: 10.1101/gr.9.9.868
Karlin, S., and Burge, C. (1995). Dinucleotide relative abundance extremes: a genomic signature. Trends Genet. 11, 283–290. doi: 10.1016/S0168-9525(00)89076-9
Katoh, K., and Standley, D. M. (2013). MAFFT multiple sequence alignment software version 7: improvements in performance and usability (outlines version 7). Mol. Biol. Evol. 30, 772–780. doi: 10.1093/molbev/mst010
Keane, T. M., Creevey, C. J., Pentony, M. M., Naughton, T. J., and McInerney, J. O. (2006). Assessment of methods for amino acid matrix selection and their use on empirical data shows that ad hoc assumptions for choice of matrix are not justified. BMC Evol. Biol. 6:29. doi: 10.1186/1471-2148-6-29
Lamarck, J. B. P. (1814). Sur les polypiers empâtés. Annales du Museum National d'Histoire Naturelle. 20, 432–458.
Lentz, S. J., and Limeburner, R. (1995). The Amazon river plume during AMASSEDS: spatial characteristics and salinity variability. J. Geophys. Res. 100, 2355–2375. doi: 10.1029/94JC01411
Lumpkin, R., and Garzoli, S. L. (2005). Near-surface circulation in the Tropical Atlantic Ocean. Deep Sea Res. 52, 495–518. doi: 10.1016/j.dsr.2004.09.001
McCormack, G. P., Erpenbeck, D., and van Soest, R. W. M. (2002). Major discrepancy between phylogenetic hypotheses based on molecular and morphological criteria within the order Haplosclerida (phylum Porifera: class Demospongiae). J. Zool. Syst. Evol. Res. 40, 237–240. doi: 10.1046/j.1439-0469.2002.00204.x
Miller, M. A., Pfeiffer, W., and Schwartz, T. (2010). “Creating the CIPRES science gateway for inference of large phylogenetic trees” in Proceedings of the Gateway Computing Environments Workshop (New Orleans, LA: GCE), 1–8.
Morrow, C., and Cárdenas, P. (2015). Proposal for a revised classification of the Demospongiae (Porifera). Front. Zool. 12:7. doi: 10.1186/s12983-015-0099-8
Morrow, C., Picton, B., Erpenbeck, D., Boury-Esnault, N., Maggs, C., and Allcock, A. (2012). Congruence between nuclear and mitochondrial genes in Demospongiae: a new hypothesis for relationships within the G4 clade (Porifera: Demospongiae). Mol. Phylogenet. Evol. 62, 174–190. doi: 10.1016/j.ympev.2011.09.016
Moura, R. L., Amado-Filho, G. M., Moraes, F. C., Brasileiro, P. S., Salomon, P. S., Mahiques, M. M., et al. (2016). An extensive reef system at the Amazon river mouth. Sci. Adv. 2:e1501252. doi: 10.1126/sciadv.1501252
Muricy, G., and Ribeiro, S. M. (1999). Shallow-water Haplosclerida (Porifera, Demospongiae) from Rio de Janeiro State, Brazil (Southwestern Atlantic). Beaufortia 49, 83–108.
Oliveira, J. H., Nascimento, A. M., Kossuga, M. H., Cavalcanti, B. C., Pessoa, C. O., Moraes, M. O., et al. (2007). Cytotoxic alkylpiperidine alkaloids from the Brazilian marine sponge Pachychalina alcaloidifera. J. Nat. Prod. 70, 538–543. doi: 10.1021/np060450q
Padial, J. M., Miralles, A., De la Riva, I., and Vences, M. (2010). The integrative future of taxonomy. Front. Zool. 7:16. doi: 10.1186/1742-9994-7-16
Pulitzer-Finali, G. (1982). Some new or little-known sponges from the great barrier reef of Australia. Boll. Mus. Ist. Biol. Univ. Genova. 48–49, 87–141.
Raleigh, J., Redmond, N. E., Delehan, E., Torpey, S., van Soest, R. W. M., Kelly, M., et al. (2007). Mitochondrial phylogeny Supports alternative taxonomic scheme for the marine Haplosclerida. J. Mar. Biol. Assoc. U.K. 87, 1577–1584. doi: 10.1017/S0025315407058341
Redmond, N. E., Morrow, C. C., Thacker, R. W., Diaz, M. C., Boury-Esnault, N., Cárdenas, P., et al. (2013). Phylogeny and systematics of Demospongiae in light of new small-subunit ribosomal DNA (18S) sequences. Integr. Comp. Biol. 53, 388–415. doi: 10.1093/icb/ict078
Redmond, N. E., Raleigh, J., van Soest, R. W. M., Kelly, M., Travers, S. A. A., Bradshaw, B., et al. (2011). Phylogenetic relationships of the marine Haplosclerida (phylum Porifera) employing ribosomal (28S rRNA) and mitochondrial (cox1, nad1) gene sequence data. PLoS ONE 6:e24334. doi: 10.1371/journal.pone.0024344
Redmond, N. E., van Soest, R. W. M., Kelly, M., Raleigh, J., and Travers, S. A. A. (2007). Reassessment of the classification of the order Haplosclerida (class Demospongiae, phylum Porifera) using 18S rRNA gene sequence data. Mol. Phylogenet. Evol. 43, 344–352. doi: 10.1016/j.ympev.2006.10.021
Schlick-Steiner, B. C., Steiner, F. M., Seifert, B., Stauffer, C., Christian, E., and Crozier, R. H. (2010). Integrative taxonomy: a multisource approach to exploring biodiversity. Annu. Rev. Entomol. 55, 421–438. doi: 10.1146/annurev-ento-112408-085432
Schmieder, R., and Edwards, R. (2011). Quality control and preprocessing of metagenomic datasets. Bioinformatics 27, 863–864. doi: 10.1093/bioinformatics/btr026
Stamatakis, A. (2014). RAxML version 8: a tool for phylogenetic analysis and post-analysis of large phylogenies. Bioinformatics 30, 1312–1313. doi: 10.1093/bioinformatics/btu033
Thacker, R. W., Hill, A. L., Hill, M. S., Redmond, N. E., Collins, A. G., Morrow, C. C., et al. (2013). Nearly complete 28S rRNA gene sequences confirm new hypotheses of sponge evolution. Integr. Comp. Biol. 53, 373–387. doi: 10.1093/icb/ict071
Torres, Y. R., Berlinck, R. G., Magalhães, A., Schefer, A. B., Ferreira, A. G., Hajdu, E., et al. (2000). Arenosclerins A–C and haliclonacyclamine E, new tetracyclic alkaloids from a Brazilian endemic Haplosclerid sponge Arenosclera brasiliensis. J. Nat. Prod. 63, 1098–1105. doi: 10.1021/np9905618
Trindade-Silva, A. E., Rua, C., Silva, G. G. Z., Dutilh, B. E., Moreira, A. P. B., Edwards, R. A., et al. (2012). Taxonomic and functional microbial signatures of the endemic marine sponge Arenosclera brasiliensis. PLoS ONE 7:e39905. doi: 10.1371/journal.pone.0039905
van Soest, R. W. M. (2015). “Haplosclerida,” in World Porifera Database, eds R. W. M. van Soest, N. Boury-Esnault, J. N. A. Hooper, K. Rützler, N. J. de Voogd, B. Alvarez de Glasby, E. Hajdu, A. B. Pisera, R. Manconi, C. Schoenberg, M. Klautau, B. Picton, M. Kelly, J. Vacelet, M. Dohrmann, M.-C. Díaz, P. Cárdenas, J. L. Carballo, P. Rios Lopez. Available online at: http://www.marinespecies.org/porifera/porifera.php?p=taxdetails&id=131598 on 2017-06-16
van Soest, R. W. M. (1980). “Marine sponges from curaçao and other caribbean localities. Part II. Haplosclerida,” in Studies on the Fauna of Curaçao and other Caribbean Islands, eds P. W. Hummelinck and L. J. van der Steen (Netherlands: Utrecht), 1–173.
Willner, D., Thurber, R. V., and Rohwer, F. (2009). Metagenomic signatures of 86 microbial and viral metagenomes. Environ. Microbiol. 11, 1752–1766. doi: 10.1111/j.1462-2920.2009.01901.x
Arenosclera amazonensis sp. nov. LSID: urn:lsid:zoobank.org:act:28C5BD00-0AA4-4903-82AC-BC1AAD666EA5
Arenosclera klausi sp. nov. LSID: urn:lsid:zoobank.org:act:16CBBD72-49FF-41A0-82E9-A2FA9D6C6930
Keywords: integrative taxonomy, sponges, biodiversity, Brazil, Atlantic Ocean, 28S phylgeny, Haplosclerida, Karlin's Signatures
Citation: Leal CV, Moraes FC, Fróes AM, Soares AC, de Oliveira LS, Moreira APB, Thompson FL and Hajdu E (2017) Integrative Taxonomy of Amazon Reefs' Arenosclera spp.: A New Clade in the Haplosclerida (Demospongiae). Front. Mar. Sci. 4:291. doi: 10.3389/fmars.2017.00291
Received: 27 March 2017; Accepted: 25 August 2017;
Published: 06 October 2017.
Edited by:
Raquel Peixoto, Federal University of Rio de Janeiro, BrazilReviewed by:
Grace Patricia McCormack, NUI Galway, IrelandPaco Cardenas, Uppsala University, Sweden
Copyright © 2017 Leal, Moraes, Fróes, Soares, de Oliveira, Moreira, Thompson and Hajdu. This is an open-access article distributed under the terms of the Creative Commons Attribution License (CC BY). The use, distribution or reproduction in other forums is permitted, provided the original author(s) or licensor are credited and that the original publication in this journal is cited, in accordance with accepted academic practice. No use, distribution or reproduction is permitted which does not comply with these terms.
*Correspondence: Camille V. Leal, Y2FtaWxsZS52aWN0b3JpYUBnbWFpbC5jb20=