- 1Leibniz Centre for Tropical Marine Research, Bremen, Germany
- 2Faculty of Biology and Chemistry, University of Bremen, Bremen, Germany
- 3Research and Development Centre for Marine, Coastal, and Small Islands, Hasanuddin University, Makassar, Indonesia
- 4Department of Marine Science, Faculty of Marine Science and Fisheries, Hasanuddin University, Makassar, Indonesia
- 5Laboratory of Water Quality Management, Pangkep State Polytechnic of Agriculture, Pangkep, Indonesia
Pollution, fishing, and outbreaks of predators can heavily impact coastal coral reef ecosystems, leading to decreased water quality and benthic community shifts. To determine the main environmental drivers of coral reef status in the Spermonde Archipelago, Indonesia, we monitored environmental variables and coral reef benthic community structure along an on-to-offshore gradient annually from 2012 to 2014. Findings revealed that concentrations of phosphate, chlorophyll a-like fluorescence, suspended particulate matter, and light attenuation significantly decreased from on-to-offshore, while concentrations of dissolved O2 and values of water pH significantly increased on-to-offshore. Nitrogen stable isotope signatures of sediment and an exemplary common brown alga were significantly enriched nearshore, identifying wastewater input from the city of Makassar as primary N source. In contrast to the high temporal variability in water quality, coral reef benthic community cover did not show strong temporal, but rather, spatial patterns. Turf algae was the dominant group next to live coral, and was negatively correlated to live coral, crustose coralline algae (CCA), rubble and hard substrate. Variation in benthic cover along the gradient was explained by water quality variables linked to trophic status and physico-chemical variables. As an integrated measure of reef status and structural complexity, the benthic index, based on the ratio of relative cover of live coral and CCA to other coral reef organisms, and reef rugosity were determined. The benthic index was consistently low nearshore and increased offshore, with high variability in the midshelf sites across years. Reef rugosity was also lowest nearshore and increased further offshore. Both indices dropped in 2013, increasing again in 2014, indicating a period of acute disturbance and recovery within the study and suggesting that the mid-shelf reefs are more resilient to disturbance than nearshore reefs. We thus recommend using these two indices with a selected number of environmental variables as an integral part of future reef monitoring.
Introduction
Coastal coral reef systems close to populated urban areas are often highly impacted by increased nutrient inputs, sedimentation rates, and fishing pressure resulting from land based activities (Burke et al., 2011). Terrestrial effluents can increase near-shore nutrient levels, promoting primary production (Jessen et al., 2015), and deforestation can increase sedimentation rates, leading to increased suspended particulate matter (SPM) in the water-column and accelerating light attenuation (Fabricius, 2005; Cooper et al., 2007; Smith et al., 2008). The effects of terrestrial-derived inputs in coastal waters across coral reefs have led to noticeable spatial gradients in water quality in many parts of the world (Edinger et al., 1998; Mallela et al., 2004; Fabricius et al., 2005; Lirman and Fong, 2007). They generally lead to reduced scleractinian coral fitness and greater competition for benthic space between corals and other organisms (Fabricius, 2005).
Coral reefs closer to shore are generally characterized by lower hard coral vs. higher fleshy algae abundances, and by a decrease in coral and fish species diversity or even complete shifts in the sets of species (Fabricius et al., 2005). Initial work on benthic community changes in coral reefs, in particular from the Caribbean, suggested the existence of discrete alternative states dominated either by stony corals or macroalgae (e.g., Hughes, 1994); however, this view has been challenged based on the observation that many reefs do not display strict dichotomous benthic communities (Bruno et al., 2009). Rather, a more nuanced view that understands benthic community configuration as changing gradually along a continuum, notwithstanding the existence of thresholds and tipping points, is gaining prominence, necessitating a more holistic approach that considers benthic parameters beyond stony corals and macroalgae alone (Jouffray et al., 2014; Smith et al., 2016).
Spatial gradients of water quality do not affect reefs in a discrete fashion, but rather expose reef organisms to gradually changing abiotic conditions. The decrease of water quality may increase stress, thereby acting as a filter on benthic communities, where only organisms with the physiological capacity to adapt would be left within the community, resulting in a taxonomic change (Smith et al., 1981; Edinger et al., 1998; Fabricius, 2005). This in turn could negatively impact the fish species that rely on live coral cover (Alvarez-Filip et al., 2011), while other species could benefit from the new habitat composition (Öhman et al., 1997). However, the relationship between water quality and benthic composition can be even more complex due to seasonal and inter-annual variation in weather conditions that alters the composition of land-based effluents and their resultant water quality gradients (Alvarez-Filip et al., 2011; Baum et al., 2015). Mitigating the impacts that affect coral reefs can only be accomplished once the source of the impact has been identified. Thus, discerning the effects on coral reefs from land-derived water quality changes, locally-based marine resource use, or from outbreaks of coral predators, may help in the management of these perturbations.
Monitoring of coral reefs has led to measurement of a number of different environmental, biological, and chemical variables which can vary spatially on the local, regional, and global scale, and temporally over seasons, years, and decades (Fabricius, 2005). Because of this high variation, it is often difficult to identify trends and drivers of coral reef status or health and to communicate to managers and politicians the needs for action (Jameson et al., 2001). There is also often a discrepancy among response times of water quality and benthic variables, which makes monitoring, analysis and interpretation of the data more difficult (Cooper et al., 2009). Additionally, long-term monitoring programs are costly and work intensive. Identifying a few integrated multimetric indices as indicators of change in coral reef status at the regional scale and using developed frameworks would allow for simplification of monitoring efforts and provide an opportunity for more effective coral reef management (Jameson et al., 2001; Flower et al., 2017).
A number of useful benthic indicators exist and have been tested and used in many monitoring programs (Hill and Wilkinson, 2004). Among them, a benthic index calculated as the ratio of the sum of benthic cover of important reef calcifiers necessary for reef accretion (i.e., “promotors,” or live scleractinian corals and CCA) to the sum of benthic groups often associated with altered coral reef ecosystems (i.e., “detractors,” or turf algae, macroalgae, sponges, cyanobacteria, and other invertebrates) has been used by the AGRRA coral reef monitoring program (Kramer, 2003; http://www.agrra.org/coral-reef-monitoring/benthos-indicator/), and is a method that integrates relative benthic cover data. This index allows for measuring relative changes in status of coral reef benthic communities over time or differences within space and provides a health score of the reef. Another index which often has been used to measure complexity of reef structure is reef rugosity. The diversity and ecosystem functioning of coral reefs is closely associated to structural complexity because higher complexity provides more niches to sustain the biotic community. Rugosity is often used to provide an indication of the benthic substrate quality for reef fishes (Alvarez-Filip et al., 2015), but it can also nicely show changes in reef community composition over time, natural accretion processes, as well as structural damage or disturbance (Alvarez-Filip et al., 2009).
Some areas of the Southeast Asian region are densely populated and this has resulted in low coastal water quality (Burke et al., 2012). The Spermonde Archipelago of Southwest Sulawesi, Indonesia lies off the coast from the city of Makassar, a highly urbanized area with a population of 1.7 million people. Effluents originating from the city have been shown to affect the archipelago to varying degrees. Nearshore islands (up to 7 km offshore) are exposed to regular sediments, aquaculture outflow and wastewater from fluvial discharge of the nearby rivers, while midshelf reefs (7–27 km offshore) are impacted by effluents during the onset of the rainy season during the north-western monsoon (Moll, 1983; Renema and Troelstra, 2001; Lukman et al., 2014; Nasir et al., 2015, 2016). Finally, offshore islands (>27 km) are rarely exposed to high levels of terrestrial effluents from the mainland. Benthic communities, sponges, and corals have shown strong on-to-offshore patterns in diversity (Edinger et al., 2000a; Cleary et al., 2005; Becking et al., 2006; de Voogd et al., 2006). Local island human populations rely heavily on nearby coral reefs (Ferse et al., 2014). Additionally, islands with small population sizes, ranging from a few to 1000s of inhabitants, generally have minimal waste management facilities resulting in vast dumping of waste materials into surrounding waters (Sur et al., 2018). This has impacted local water nutrient concentrations and bacterial communities among inhabited islands (Kegler et al., 2017).
Although previous studies have identified spatial variation in benthic communities in relation to water quality (Cleary et al., 2005, 2008; Polonia et al., 2015), no study has explored the temporal component in this relationship and if specific water quality drivers are consistent across time. Moreover, while other non-water quality based impacts, such as outbreaks of coral predators, resource extraction and localized waste production, may also contribute to habitat degradation (Edinger et al., 2000a,b), previous studies have frequently overlooked their potential impact on benthic patterns.
In the current study, we use the Spermonde Archipelago to explore variations in coral reef benthic habitats in relation to environmental stressors. We aimed to identify primary environmental drivers that spatially structure coral reef benthic communities across the reef shelf of the Spermonde Archipelago. This spatial relationship is explored annually across 3 years (2012–2014). We investigated the following questions: (1) Which water quality and environmental variables drive benthic coral reef community structure across the Spermonde Archipelago, (2) is there temporal variability in these relationships, (3) which indices can be used to detect spatial and temporal change in coral reef status and health for future monitoring, and (4) can these indices help identify the contribution of localized disturbances? To answer these questions, we designed an annual survey on water quality, benthic reef cover, and fish communities along an on-to-offshore gradient in Spermonde Archipelago. In this first paper we examine spatio-temporal relationships within and among environmental variables and benthic reef groups. In the second paper (Plass-Johnson et al., 2018), we examine changes in fish communities in relation to water quality and benthic cover. Through these analyses, we aimed to develop a better understanding of the processes driving coral reef community structure and recommendations for a feasible monitoring plan for future assessment of coral reef status and health of the Spermonde Archipelago.
Materials and Methods
Study Sites
This study was conducted across three consecutive years during the same annual dry season of the Spermonde Archipelago, Indonesia. The first sampling campaign occurred between the 20th of September and the 11th of October, 2012. The second sampling was between the September 22nd October 1st, 2013, and the third sampling was between the 16th and 29th of November, 2014. Eight islands with increasing distance from the city of Makassar were chosen (Figure 1). The islands, with distance in brackets, comprised of Laelae (LL; 05°08S, 119°23E, 1 km), Samalona (SA; 05°07S, 119°20E, 7 km), Barrang Lompo (BL; 05°02S, 119°19E, 11 km), Bonetambung (BO; 05°01S, 119°16E, 14 km), Badi (BA; 04°57S, 119°16E, 19 km), Lumulumu (LU; 04°58S, 119°12E, 22 km), Karang Kassi (KS; 04°53S, 119°09E, 27 km) and Kapoposang (KP; 04°41S, 118°57E, 55 km distance) (Figure 1).
All islands are located on the continental shelf and, apart from KS and KP, experience similar oceanographic conditions with respect to wave exposure and currents. Bathymetric data show uniform shallow carbonate platforms around the islands (Kench and Mann, 2017). KS is a submerged reef plateau and KP is located on the edge of the continental shelf, exposing it to deep oceanic waters, and stronger waves and currents. The northwest corner of each site (except KP) was used to standardize the sampling sites among reefs. The western coast of the islands generally features a well-developed, carbonate fore-reef and a sandy back-reef and flat. The reef crest is shallow (~4–5 m) and the slope reaches down to 15 m. The last study site, KP, was located on the top of the outer continental shelf wall (Figure 1). Although KP differs from the other seven sites in habitat structure and wave exposure, it shares many biotic aspects with the other sites (Allen and Erdmann, 2012) and given its distance from the mainland, it receives no land-based effluents and minimal fishing pressure. Thus, the benthic and fish communities there represent a relatively healthy state of reef habitat allowing for comparative analyses.
At each site, three 50 m permanent transects were installed where all subsequent water and benthic data were collected. Therefore transects were surveyed along identical reef areas for each reef over time. Transects were placed at 6–7 m depth, always standardized at 2 m below the reef crest at low tide. Transects were separated by 10 m, and the beginning and end of each transect was marked with steel rebar to provide attachment points for the transect tapes. All work, including water sampling and benthic cover surveys, was carried out during 1 day at each site. Work was started at a standardized time each day (08:00 h); weather was always sunny and dry with minimal wave energy.
Environmental Variables
Water quality variables including nutrient concentrations of nitrate + nitrite (NOX), phosphorus (), and silicate (Si), in situ Chlorophyll a-like fluorescence (Chl a), dissolved organic carbon (DOC), suspended particulate matter (SPM), dissolved oxygen (DO), temperature, pH, salinity, and light attenuation (Kd) were collected during the sampling campaigns (see section Study Sites). Due to logistic limitations with sampling, some variables were not measured in certain years, including DOC and SPM in 2013 and Kd in 2014. Additionally, some water samples for nutrient analysis were missing from specific sites in year 2013. At each site seawater samples (n = 6) were collected along the permanent transects at 2–5 m water depth using a Niskin bottle. The water was transferred into six acid-cleaned canisters, and from each canister subsamples for inorganic nutrient and DOC were collected immediately. For DOC, 50 ml subsamples were collected, filtered through pre-combusted (450°C, 5 h) GF/F filters (VWR: 25 mm, 0.7 μm pore size) into acid-washed 30 ml HDPE sample bottles and acidified with 80 μl of 18.5% HCl. In addition, 50 ml samples for the determination of NOX, Si and were also filtered through pre-combusted GF/F filters into 50 ml Falcon-tubes and directly pre-served with HgCl. Back in the lab all samples were stored refrigerated in the dark until analysis, and the remaining water (3 L) of each canister was filtered through pre-combusted GF/F filters for SPM analysis. All filters were dried in the oven (48 h at 40°C) and stored in the dark until analysis.
All samples were brought back to the Leibniz Centre for Tropical Marine Research (ZMT), Bremen, Germany for analysis. DOC samples were analyzed by high-temperature catalytic oxidation on a Shimadzu TOC-VCPH total organic carbon analyzer. The instrument was calibrated with serial dilutions from a potassium hydrogen phthalate certified stock solution (1,000 ppm Standard Fluka 76067-500ML-F). Consensus reference material was provided by DA Hansell and W Chan of the University of Miami (batch 13, lot no. 08-13, 41–45 mmol C l21). Each sample was measured with five replicate injections, and analytical precision was within <3% of the certified value. Inorganic nutrient analyses were performed on a flow through auto-analyzer (FlowSys Alliance Instruments) using standardized photometric techniques (Murphy and Riley, 1962; Strickland and Parsons, 1972).
Temperature, pH, salinity, and in situ Chl a-like fluorescence were obtained from a Eureka Manta logger (GEO Scientific Ltd.) recording at 2 min intervals at each sampling site. Chl a-like fluorescence was measured with an optical sensor with a light emitting diode with an excitation wavelength of 460 nm and emission wavelength of 685 nm (resolution of 0.01 μgL−1 and accuracy of ± 3%). To match sample sizes obtained from the manta logger with physical water samples, data were averaged every 10 min between the hours of 10:00 and 11:00, creating a sample size of six per site. Kd, the light attenuation coefficient, was calculated from underwater light profiles taken with a light meter (planar sensor, LiCor Li-192SA, Lincoln, USA), where:
Ed(z2) and Ed(z1) are measurements at 0.05 m (z1) and 4 m (z2) below the surface (Kirk, 2011). To complement these measurements, data on mean annual temperatures and total rainfall were acquired from NOAA Coastwatch program, (https://coastwatch.pfeg.noaa.gov/infog/MP_sstd_las.html).
Benthic Community Assessment
Benthic communities were quantified with 50 photographic quadrats per 50 m transect. Photographs were taken at 1 m (standardized with a measuring pole) above the substratum. Every 2 m along the transect, a photograph was taken on both sides of the transect tape, with a section of the tape within the picture, to identify total area of the picture. Analysis of the pictures was conducted with Coral Point Count with Excel extensions (CPCE; Kohler and Gill, 2006). Pictures were analyzed with fifty randomized points (based on results from power analysis) per photograph for the following biotic groups: sponges, crustose coralline algae (CCA), other invertebrates (including bivalves, crustaceans, feather stars, gorgonians, hydroids, starfish, tubeworms, urchins, zooanthids, ascidians, and soft coral), cyanobacteria, macroalgae, turf algae, and live hard coral. The selection of benthic groups was based on English et al. (1997). Biotic groups were classified including any epiphytic growth, irrespective of the underlying surface structure type. To complement this, surface structures that provide available substrate were also quantified and recorded. These substrate types included sand, rubble, and hard substrate (i.e., primarily dead coral, pavement). Live coral could also be seen as a hard surface structure in addition to a biotic group, but is unavailable for recruitment.
N Isotope Signatures
To identify variation in δ15N of reef sediment and the commonly found brown calcifying algae Padina sp., sediment and algae were collected from each site in sampling year 2014. Collection depth was always 5 m below low tide to standardize light-associated change in plant isotopic values. Padina and sediment samples were collected in replicates of five. Sediment was taken from the top 2 mm of the sediment using 2.0 ml Eppendorf tubes. All algal samples were cleaned of detritus and epiphytes, rinsed with distilled water, and then dried at 60°C for 48 h. Samples were ground to powder with mortar and pestle and analyzed for δ 15N using a Thermo Finnigan Delta Plus mass spectrometer coupled with a Flash EA 112 elemental analyzer. Isotopic results are expressed in standard δ unit notation as:
where X is 15N, and R is the ratio of 15N/14N for nitrogen. The variation in analytical precision of the measurement was <0.06‰. All δ15N values were reported as ‰ vs. air, respectively, and normalized to atmospheric nitrogen (nitrogen) and calibrated to the International Atomic Energy reference materials of IAEN N1 and N2.
Data Analyses
Spatial and temporal patterns in environmental variables and benthic data were log or square root transformed after testing for normality, and then analyzed using permutational analysis of variance (PERMANOVA; PRIMER 7, version 7.0.10) to see whether significant differences were detected among years, with distance as a covariate. If significant differences were detected across years, a pairwise comparison test was performed to see which years were significantly different from each other. Years that were not significantly different were then pooled. Regression analyses were carried out on environmental and benthic variables using distance from shore as the independent variable (STATISTICA 12). Correlation analysis among benthic groups and substrate type were performed, based on Pearson r (STATISTICA 12).
To better visualize and integrate the different patterns in environmental variables and benthic cover over space and time, we ran separate Principal Component Analyses (PCA) on selected environmental variables and benthic cover (PRIMER 7, version 7.0.10). To determine whether any of the environmental variables could be driving factors of change in benthic assemblages, the first two principal components (PC1 and PC2) from the PCA on environmental variables were then used in multivariate generalized linear models (manyglm) from the mvabund package in R (Wang et al., 2012). Manyglm fits a separate, univariate, generalized linear model to the recorded cover of each benthic group and relates each cover to a common set of explanatory variables to create a multivariate analysis across taxa. The function uses resampling-based hypothesis testing to make community-level and group-specific inferences, returning a multivariate analysis testing where environmental variables are associated with the multivariate abundances (i.e., with the community of benthic groups as a whole). Manyglm was applied to each year with a negative binomial distribution to determine the environmental variables that were most related to benthic cover. The fits of the models were assessed by examining residual plots. The relationships of individual benthic groups with the environmental PCs were also examined to see which benthic groups are contributing to patterns at the community level.
Benthic Indices of Coral Reef Health
To evaluate trends in coral reef status over space and time, we chose to use the AGGRA benthic index (Kramer, 2003; Lang et al., 2010). This index was calculated as the ratio of the sum of benthic cover of important reef calcifiers necessary for reef accretion and preferred for coral recruitment, as well as for enhancement of coral reef biodiversity (i.e., live scleractinian corals and CCA; Adey, 1998) to the sum of benthic groups often associated with altered coral reef ecosystems (i.e., turf algae, macroalgae, sponges, cyanobacteria, and other invertebrates). The second index was reef rugosity, measured using the linear distance-fitted chain method (Risk, 1972). The chain length used was 20 m, and measurement was conducted once per transect, starting at the first 10 m point. These two indices were chosen as possible indicators of coral reef health based on the assumption that relatively higher abundance of benthic sessile calcifiers compared to other reef organisms indicates better reef health, and that coral dominance will result in higher structural complexity.
Results
Spatio-temporal Patterns in Environmental Variables
Environmental variables significantly differed across years (with the exception of Kd) and varied in their patterns across the on-to-offshore gradient (Figure 2, Table 1), with the exception of NOx and NOx:P (Figure 2, Table S1). The clearest spatial patterns were seen in , Chl a-like fluorescence, SPM, and Kd, which were generally higher nearshore and decreased offshore, while DO and pH were generally lower nearshore and increased offshore (Figure 2, Table 1). Yet, differences across time were recorded. was generally < 0.2 μM, with no variation across sites in 2012. In 2013 and 2014, values decreased across the on-to-offshore gradient, with values starting at 0.2 μM in 2013, and <0.1 μM in 2014. NOx concentrations ranged from 0 to 1.2 μM and showed no significant relationship across distance (Figure 2). NOx:P was quite variable over time and space from 0 to 12, but seemed to be higher in the midshelf region in year 2014 (Figure 2). Si showed significant contrasting positive and negative trends on-to-offshore in 2012 and 2014, respectively, with concentrations in 2013 ranging between approximately 2–4 μM. In 2012, Chl a-like fluorescence was approximately twice as high as other years, up to 4 μgl−1in the nearshore site, corresponding with a DO of 4.6 mgl−1, and DOC up to 150 μgl−1. In 2013 and 2014, Chl a-like fluorescence also decreased with distance, reaching up to 2.5 μgl−1, and corresponded to DO in nearshore sites around 6 mgl−1. DOC was not measured in 2013, but values in 2014 varied across the shelf between 50 and 90 μgl−1. In contrast to Chl a-like fluorescence, SPM was highest in 2014, up to 33 mgl−1, and decreased offshore. pH increased with distance from 8.1 to 8.3, with 2014 being higher than 2012 and 2013 (Figure 2, Table 1).
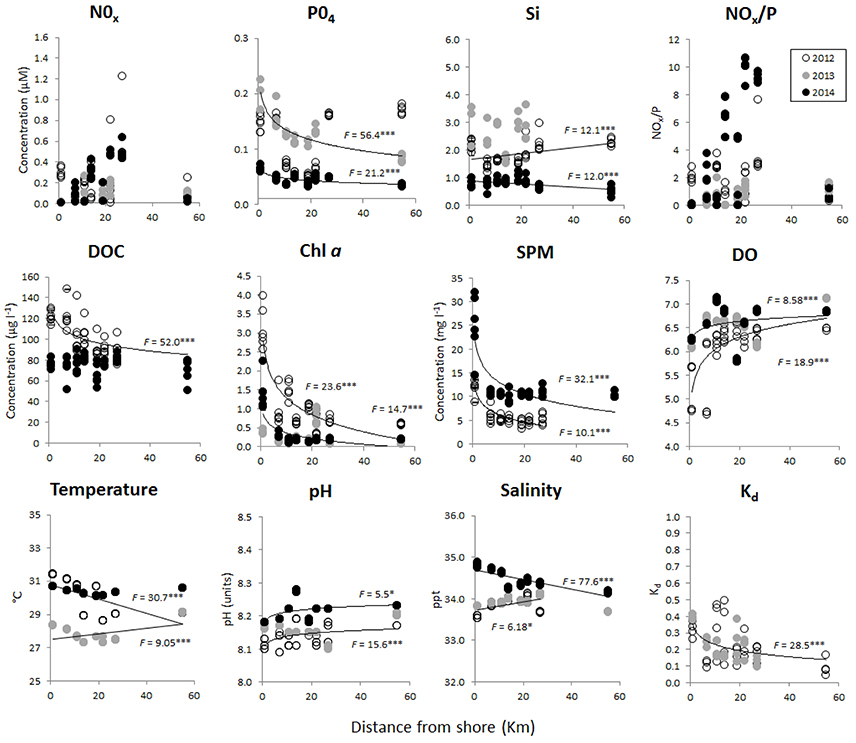
Figure 2. Environmental variables measured at each site in 2012 (white), 2013 (gray), and 2014 (black). Sites are represented by distance from shore (km) on the x-axis. Regressions are indicated for variables that showed a significant increase or decrease with distance, after testing first for differences across years and pooling years that were not significantly different from each other (PERMANOVA, results from Table S1). Full results from regression analyses equations and R2 values are shown in Table 1. Asterisks indicate level of significance (*** < 0.001 and * < 0.5).
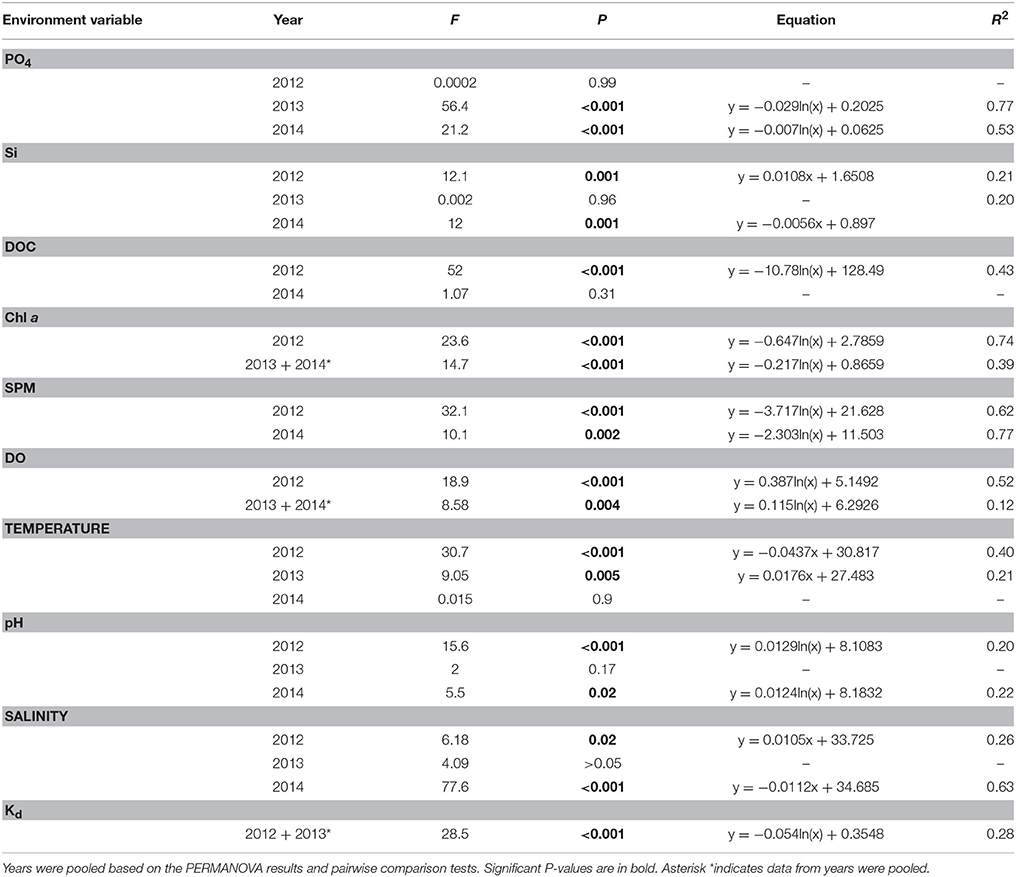
Table 1. Results of regression analyses of environmental variables with distance from shore for all environmental variables that showed a significant difference with distance or an interaction effect in the PERMANOVA analysis (Table S1).
Other environmental variables, including temperature and salinity, showed contrasting increasing and decreasing trends with distance in different years, with temperature being 2°C lower in 2013, from 27 to 29°C, compared to 29 to 31°C in 2012 and 2014 (Figure 2, Table 1). Salinity was slightly higher nearshore in 2014 than in 2012 and 2013, at 35 ppt compared to 34 ppt (Figure 2, Table 1). Mean annual SST from the Spermonde Archipelago (latitude:−5.16 to −4.66 S; longitude:118.83 to 119.5 E) and recorded total annual rainfall for Makassar were 29.3, 29.5, and 29.5°C and 282, 131, and 94 mm for years 2012, 2013, and 2014, respectively. For the PCA of the environmental variables, only 5 variables in which full datasets were available from 2012 to 2014 were used for the spatial-temporal analysis. This included Chl a-like fluorescence, DO, pH, temperature, and salinity. PC1 explained 45% of the variation, and was strongly, positively driven by pH (loading: 0.565) and DO (loading: 0.583) and negatively by Chl a (loading: −0.428) (Figure 3, Table 2). PC2 explained 32.5% of the variation and was positively driven by temperature (loading: 0.730), Chl-a (loading: 0.445), and salinity (loading: 0.406) (Figure 3, Table 2). Environmental data from 2013 clearly separated from 2012 and 2014 along lower values of PC2, and from 2012 along higher values of PC1, while data from 2012 and 2014 separated along PC1, but were similar along PC2 (Figure 3).
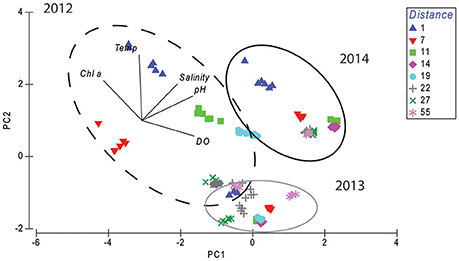
Figure 3. Principal component analysis of environmental data from water quality variables Chl a, DO, temperature (Temp), salinity, and pH, indicated for each site as distance from shore in years 2012, 2013, and 2014. Principal components 1 and 2 are used in subsequent multivariate GLMs of benthic communities (scores listed in Table S4).
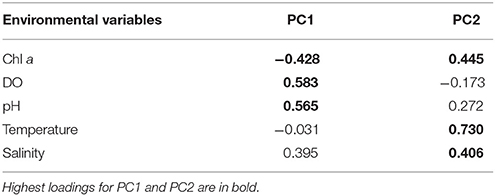
Table 2. Loadings on PC1 and PC2 of Principal Component Analysis of environmental variables across sites and time.
To better identify the suite of variables that may be useful for further monitoring, we carried out an additional PCA using mean values calculated with available data over all 3 years with the complete set of environmental variables. The first four sites separated along PC1, in relation to Chl a, DO, pH, temperature, salinity, SPM, Kd, and DOC, and the last 4 sites separated along PC2 in relation to silicate, NOx:P, temperature, and pH (Figure S1, Table S5).
Although dissolved inorganic nutrients, including NOx, were generally low across all sites, δ15N of sediment and the brown calcifying macroalga Padina sp. were heavier nearshore and significantly decreased further offshore (Figure 4). Sediment δ15N values were heavier than those of macroalgae, ranging from 3–7‰ compared to 0.5–4.5‰, respectively, and showed a better overall fit with distance than macroalgae (Figure 4).
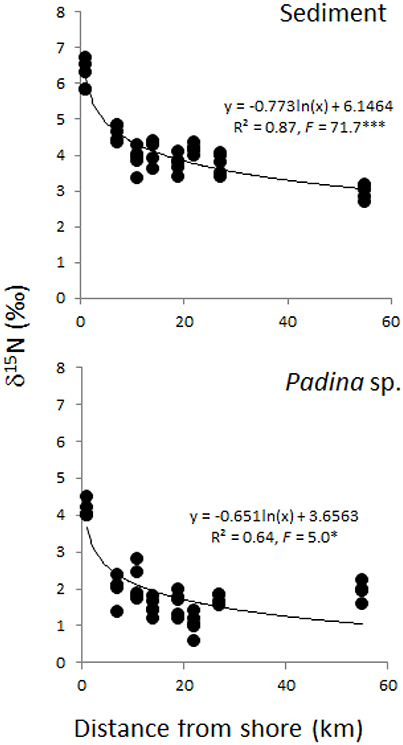
Figure 4. N stable isotope values (δ15N) of sediment samples and of the macroalga Padina sp. collected in 2014 at each site plotted as distance from shore (km). Asterisks indicate level of significance (*** < 0.001 and * < 0.5).
Spatio-temporal Patterns in Benthic Coral Reef Composition and Substrate Type
Coral reef benthic community cover varied with distance from shore but showed few differences across years, with the exception of CCA and macroalgae (Figure 5, Table S2). Overall, live coral and turf algae were dominant in terms of reef cover (Figure 5). Live coral cover increased from less than 10% in the nearshore site to as high as 40–50% in the middle and outer reef sites (Figure 5, Table 3). In contrast, turf algal cover was up to 90% in some transects in nearshore sites, and decreased further offshore (Figure 5, Table 3). CCA cover was 0 % at nearshore sites and increased with distance up to 20% offshore in 2012 and 2013. In 2014, CCA cover was slightly higher, with one midshelf site reaching up to 30–40% (Figure 5, Table 3). Macroalgae cover did not significantly change with distance, but ranged between 0 and 20% at nearshore sites compared to 5–7% offshore. Cyanobacteria and sponge cover were also not significantly different across sites; however, high variation in cover was observed in midshelf sites, from 5 to 40% among transects (Figure 5, Table 3). Other invertebrates were 10–25% nearshore, decreasing offshore to less than 5% (Figure 5, Table 3).
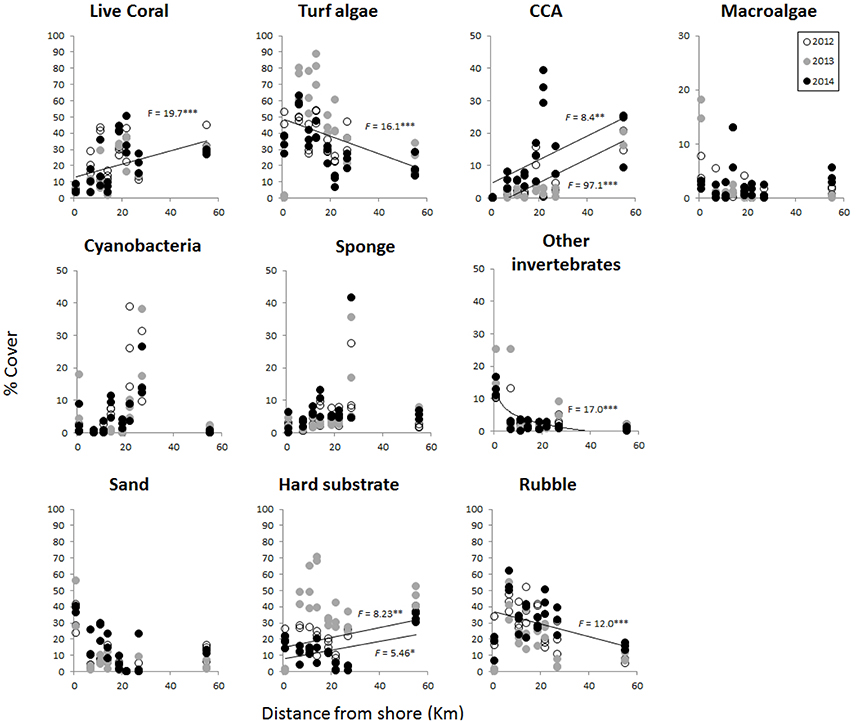
Figure 5. Percentage of benthic cover of dominant groups of coral reef organisms and substrate type measured at each site in 2012 (white), 2013 (gray), and 2014 (black). Sites are represented by distance from shore (km) on the x-axis. Regressions are indicated for variables that showed a significant increase or decrease with distance, after testing first for differences across years and pooling years that were not significantly different from each other (PERMANOVA, results from Table S2). Full results from regression analyses, equations and R2 values are shown in Table 2. Asterisks indicate level of significance (***Indicates < 0.001, **Indicates > 0.001 and < 0.1, *Indicates > 0.01 and < 0.5).
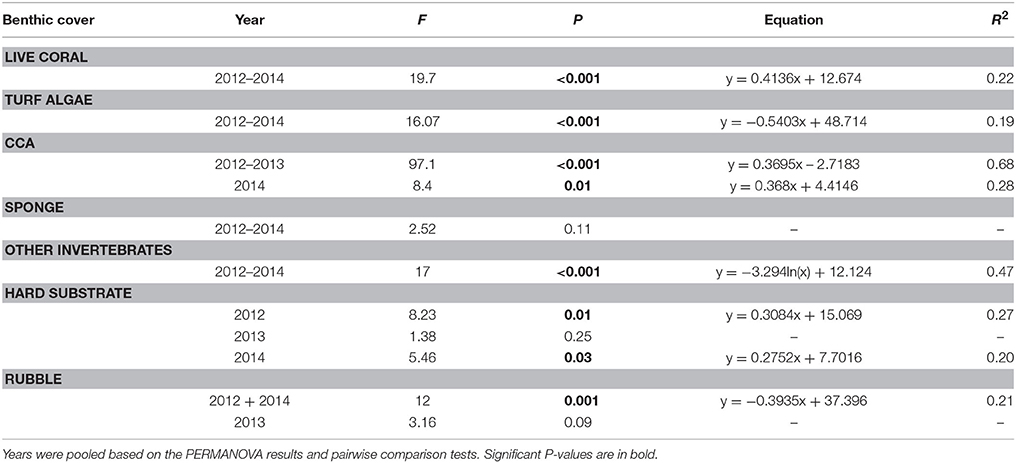
Table 3. Results of regression analyses of benthic cover (%) and substrate type with distance from shore for all benthic variables that showed a significant difference with distance in the PERMANOVA analysis (Table S2).
Along with live coral, other surfaces included sand, hard substrate (i.e., primarily dead coral pavement), and rubble. Sand contribution to benthic cover was not significantly different across distance and years, at less than 30% at all sites, except the nearshore site (20–60%; Figure 5, Table S2). Hard substrate was significantly different with distance and across years (Figure 5, Table S2), following an increasing trend with distance in 2012 and 2014, from 0 to 30% (Figure 5, Table 3). In 2013, hard substrate coverage was highest across most sites (30–70%) and did not decrease with distance (Figure 5, Table 3). With the exception of the site closest to shore where sand, hard substrate, and rubble were all present, rubble had the highest cover nearshore up to 60% and significantly decreased with distance from shore throughout each year (Figure 5, Table 3, Table S2).
As the benthic biotic cover showed little differentiation across years, all years were pooled together for the PCA analysis (Figure 6). Additionally, substrate type was added to see whether there were any particular relationships with substrate and biotic groups apart from live coral. Together, PC1, PC2, and PC3 explained 65% of the variation in benthic cover across sites. PC1 was most driven by macroalgae (loading: −0.434), sand (loading: −0.491), other invertebrates (loading: −0.496), and live coral cover (loading: 0.455), representing the large-scale gradient on-to-offshore (Figure 6). PC2 was primarily driven by hard substrate (loading: −0.406) and turf algae (loading: −0.632), while PC3 was most affected by sponge (loading: 0.496), cyanobacteria (loading: 0.468), and rubble (loading: −0.450). General groupings of the data show that the nearshore site was dominated by macroalgae, other invertebrates, and sandy substrate (PC1), while midshelf sites from 7 to 14 km were dominated by turf, rubble, and hard substrate (PC2, PC3). Sites further offshore from 19 to 55 km were primarily associated with CCA and live coral (PC1), as well as sponge and cyanobacteria at 22 and 27 km from shore (PC3; Figure 6).
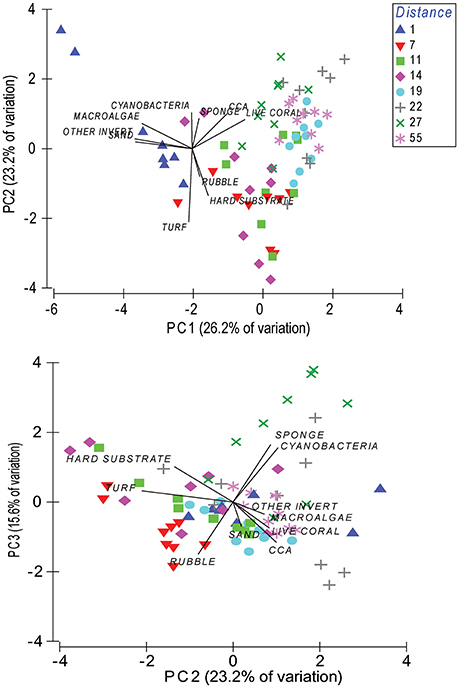
Figure 6. Principal component analysis of benthic groups and substrate type for all sites indicated by distance from shore for 2012, 2013, and 2014 combined. PC1, PC2, and PC3 together account for 65% of the variation.
Correlations among and between benthic groups and substrate types revealed a number of significant relationships (Table S3). Here we focus primarily on the two groups with highest cover, live coral and turf algae, which were found across all sites. Live coral was positively correlated to CCA, and negatively correlated to macroalgae, other invertebrates, and turf algae (Table S3, Figure 7). Turf algae also showed a strong negative correlation with CCA and were positively correlated to rubble and hard substrate (Figure 7). In 2012 and 2014, turf algae were correlated primarily with rubble, but in 2013, high turf algae cover was related primarily to an increase in hard substrate (Figure 7).
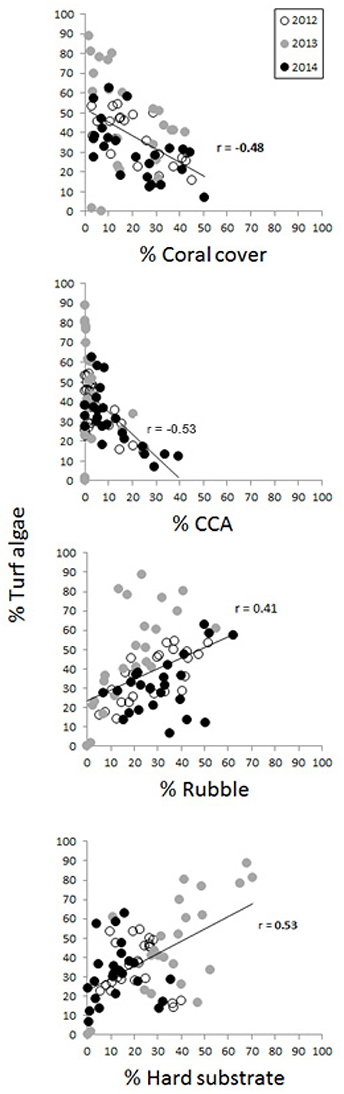
Figure 7. Correlations between turf algae and live coral cover, CCA, rubble and hard substrate. Years are shown in separately by color but pooled for the correlation analyses. All correlation coefficients are significant (P < 0.001).
Relationship among Environmental Variables and Benthic Communities
Multivariate GLM tests showed that benthic community composition was significantly related to environmental PC1 in 2012 and to both PC1 and PC2 in 2013 (Table 4). In contrast to 2012, benthic communities were significantly related to environmental PC2 in 2014 (Table 4). PC1 of the environmental variables was characterized primarily by Chl a, DO, and pH, while PC2 was characterized primarily by temperature, salinity, and Chl a (Table 2). Univariate analyses indicated that other invertebrate cover was significantly affected by all environmental variables, as shown by a significant relationship to both environmental PC1 and PC2 in all years (Table 5). Relationships between cover of all other groups -including live corals, turf, macroalgae, CCA, sponge, and cyanobacteria- and PC1 and PC2 varied across years (Table 5). Of the most dominant groups, live coral cover was only significantly related to PC1 in 2012 and PC2 in 2014. Turf algae was significantly related to PC1 in 2012 and PC2 in 2013, but not related to PC1 or PC2 in 2014. CCA was related to PC1 in years 2012 and 2013, but to PC2 in 2014. Sponge was related to PC1 in 2012 and PC2 in 2014, while cyanobacteria were significantly related to PC1 and PC2 in 2012 and PC1 in 2013 (Table 5).
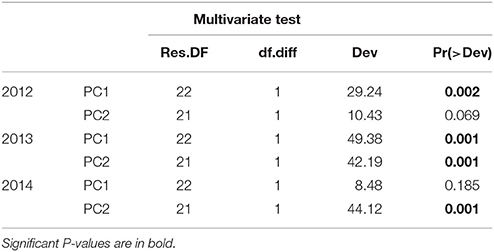
Table 4. Results of multivariate GLMs between PC1 and PC2 of environmental water data showing the relationship between water quality and benthic community composition for 2012, 2013, and 2014.
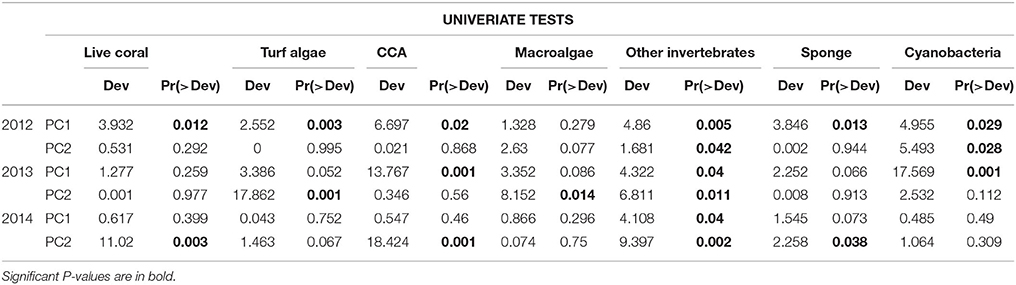
Table 5. Results of univariate GLMs between PC1 and PC2 of environmental water data showing the relationship between water quality and benthic community composition for 2012, 2013, and 2014.
Benthic Indices of Coral Reef Health
The benthic index showed a general increasing trend on-to-offshore, but with variation among years (Figure 8, top). Midshelf and offshore sites greater than 20 km from shore had values ≥ 1, indicating an equal or higher percent of live coral and CCA cover compared to other benthic biotic groups. Nearshore and midshore sites up to 20 km from shore had mean values below 1 reaching as low as 0.05 (Figure 8, top). The benthic index at the site closest to shore was approximately 0.10, while the site furthest away from shore had the highest index value around 3, with little changes noticeable across time in both of these sites. All other sites in between showed some variation among years, with an interesting sequential pattern emerging. From 2012 to 2013, there was a noticeable decline in the benthic index in a number of these sites. Interestingly, all of these sites experienced a recovery, or increase in the benthic index in 2014 with some sites actually exceeding the values of 2012 (Figure 8, top).
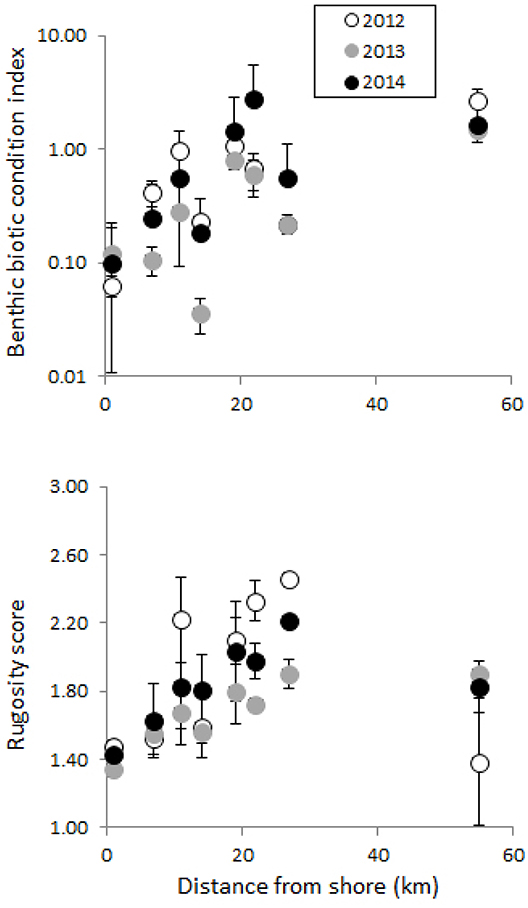
Figure 8. Benthic biotic condition index and reef rugosity score for each year at each site with distance from shore (km). Benthic biotic index is calculated as a ratio between live coral + CCA cover and all other biotic groups (turf algae + macroalgae + sponge + cyanobacteria + other invertebrates).
Reef rugosity followed a similar pattern as the benthic index, generally increasing on-to-offshore (Figure 8, bottom). The site closest to shore had the lowest reef rugosity score and little variation over time. However, the site furthest offshore was considerably lower than some of the midshelf sites, and did not match the pattern seen in the benthic index. Other sites showed a similar decrease in reef rugosity in 2013 with a subsequent increase in 2014, in line with the drop in benthic index. However, no sites showed a complete recovery of the reef rugosity index back to 2012 levels (Figure 8, bottom).
Discussion
The coral reef system of the Spermonde Archipelago has been the focus of extensive studies in the past two decades, serving as a model system to examine spatial differences in biodiversity, ecological and geological processes, and anthropogenic influences along a cross-shelf gradient within the Coral Triangle (Edinger et al., 1998; Renema and Troelstra, 2001; Cleary et al., 2005; Becking et al., 2006; Sawall et al., 2011, 2013; Hoeksema, 2012; Plass-Johnson et al., 2015a, 2016a,b; Polonia et al., 2015). In this study, we go beyond previous research by identifying several important indicators of water quality and the main environmental drivers of coral reef community composition in the Spermonde Archipelago, including an assessment of temporal trends. Through comprehensive reef monitoring of environmental variables, benthic community structure, and substrate type across an on-to-offshore gradient over three consecutive years, we show that both chronic and acute local disturbances acted together as drivers of coral reef community composition. Chronic disturbances in the form of poor water quality impacted nearshore reefs, while acute disturbances played a role in shaping reefs in the mid-shelf, where water quality was better, but disturbances such as blast fishing and Acanthaster planci outbreaks (Plass-Johnson et al., 2015b) were likely affecting live corals and substrate type and leading to changes in relative cover of benthic groups. Acute disturbances in the mid-shelf reefs also led to higher variability in the community structure over time, revealing both the potential for decline and recovery within the study period.
Evidence of a Cross-Shelf Gradient of Water Quality in Spermonde Archipelago
Environmental variables associated with water quality, including in situ Chl a-fluorescence, DO, pH, SPM, and light attenuation, Kd (Fabricius et al., 2012; Jessen et al., 2015), showed consistent increasing or decreasing trends spatially with distance from shore. They serve as strong indicators of eutrophication and of high inputs of particulate matter, especially in the nearshore site. Despite the consistency in this spatial trend, some variability in the magnitude was observed among years. The high Chl a concentration in 2012 likely drove higher DOC and lower DO concentrations in that year as a result of increased microbial activity, a clear sign of poorer water quality driven by symptoms of eutrophication. SPM was higher in 2014, which may have directly limited phytoplankton production due to light reduction. However, SPM can also be influenced directly by runoff of particulate matter from land. A recent study in the same system showed the close correlation of Chl a, SPM, and DOC (Kegler et al., 2017), however, values in our study for 2012 were much higher than the reported values in that study (Kegler et al., 2017). The variability among these environmental parameters within our sampling may be due to the timing of the phytoplankton bloom, or to ENSO related climate conditions that influence riverine nutrient inputs. Rainfall was highest in 2012, which may have increased riverine inputs and stimulated the high phytoplankton production and other eutrophic symptoms. The combination of high SPM and phytoplankton concentrations caused a reduction of light penetration nearshore, as shown by the higher Kd values. Satellite-derived observations have confirmed large scale spatial gradients in chl a fluorescence in the region, and have been useful in other studies (Polonia et al., 2015; https://oceancolor.gsfc.nasa.gov/cgi/l3/A20021852015304.L3m_CU_CHL_chlor_a_4km.nc.png?sub=img), but may be less reliable on the smaller spatial-scale. They, however, may be useful for predicting onset of blooms in strong La Niña years, as data are readily available with high temporal resolution.
Despite signs of eutrophication and high inputs of SPM, dissolved inorganic nutrients remained low near the detection limits in our study sites. This pattern has been shown in other coral reef systems, and is likely due to the rapid uptake of nutrients from the water-column by abundant phytoplankton and other benthic marine plants (Smith et al., 1981; Furnas et al., 2005). In the Great Barrier Reef, dissolved inorganic nitrogen concentrations in the water-column have been shown to be relatively depleted, <0.1 μM, despite high riverine inputs (Furnas et al., 2005). This has been linked to rapid uptake by phytoplankton, which then stimulates higher production of dissolved organic nutrients in the coral reef system available for benthic organisms. Although dissolved inorganic nutrient concentrations were relatively low in Spermonde Archipelago, a range of <1 to 10 of NOx:P indicates that this system is N-limited nearshore and gradually moves toward P-limitation further away, with the exception of KP, the site furthest off-shore. In carbonate-rich coral reef systems, P is usually the limiting nutrient, due to inorganic P being readily absorbed by carbonate sediments (Lapointe, 1997); however, some evidence of N-limitation has been found in other reef systems (Larned, 1998) and is well supported for coastal areas worldwide (Howarth and Marino, 2006). Our findings of low N to P ratios in the water-column indicate that N inputs to the nearshore area are driving increases in primary production. High inputs of N, primarily in the form of , and SPM, which carries a high proportion of particulate organic matter (POM), is discharged from the mouth of the Tallo River into the nearshore coastal area of Spermonde Archipelago (Nasir et al., 2015, 2016). Chl a concentrations at the river mouth have been found to be as high as 2–7 mg m−3 (Lukman et al., 2014; Nasir et al., 2015), which supports the relatively high concentrations we observed in 2012 at 1 km from the coast. The change in magnitude of Chl a concentrations over seasons and across years serves as a good indicator of current nutrient availability status, despite the low levels of inorganic nutrients found in the water-column.
Impacts of anthropogenic nutrient inputs were further confirmed by the enriched δ15N signatures found in the sediment and macroalgae in the nearshore sites (Lindau et al., 1989). Wastewater is generally enriched in 15N, from 10 to 20‰, compared to other N sources (Heaton, 1986). Macroalgae take up primarily inorganic nutrients in the form of NOx and directly from the water column, with little to no isotopic fractionation, being lighter than or equal to the N isotopic signature of their source (Heikoop et al., 2000; Lapointe et al., 2005; Umezawa et al., 2007; Dailer et al., 2012). Enriched δ15N values of macroalgae ranging from approximately 6–13‰ have been shown to be correlated to high N inputs from wastewater to coastal areas (Costanzo et al., 2001; Cole et al., 2004; Savage and Elmgren, 2004; Lapointe et al., 2005; Deutsch and Voss, 2006). Isotopic N signatures of sediment have also been used as an indicator of wastewater sewage effluent (Savage et al., 2004) and may directly reflect the source of settled organic particulate matter, as well as various fractionation processes during microbial degradation which could lead to further enrichment. In our study, sediment δ15N signatures were higher than that of Padina sp., and may more accurately reflect the direct source of N, as in subtropical waters of Okinawa, Japan, the macroalga Padina australis was shown to isotopically discriminate during N assimilation (Umezawa et al., 2007). Nonetheless, both sediment and macroalgal δ15N signatures were significantly higher nearshore, signaling a change in N source along the on-to-offshore gradient. These data further support the need for management of N inputs, particularly from wastewater, from the city of Makassar, which should be prioritized to reduce eutrophication symptoms in the nearshore coral reefs.
Environmental variables related to climate, such as temperature and salinity, showed some spatial and temporal variability during our study periods, but were less predictable along the cross-shelf gradient, and did not correlate strongly with inter-annual differences in temperature and rainfall driven by climatic conditions of ENSO. Annual SST and rainfall data available from the NOAA Coastwatch program revealed that rainfall was much higher in 2012 than in 2013 and 2014, and annual SST in the region was actually 0.3°C higher on average in 2013 and 2014 compared to 2012 (https://coastwatch.pfeg.noaa.gov/infog/MP_sstd_las.html). Salinity differences among years in our study periods may partly be explained by climate-driven changes in rainfall among the years, as 2014 had the least rainfall and was highest in salinity. However 2012, a La Niña year, had the highest rainfall but did not show any noticeable effect on salinity at the end of the dry season when we sampled. Temperature did not reflect the inter-annual trends in SST, as 2013 was reported to be approximately 2°C lower than 2012 and 2014 during our sampling period. Temperature differences may have been driven by varying local conditions influenced by small-scale differences in tides and movement of water masses among shallow water depths. These temperature conditions, however, may play a role in influencing short-term responses in primary productivity and other biological and biogeochemical processes within the study period.
Evidence of a Cross-Shelf Gradient in Coral Reef Benthic Community Structure and Potential Environmental Drivers
Spatial differences in coral reef benthic communities and substrate type across the shelf-gradient of Spermonde Archipelago highlighted the relative role of water quality along the on-to-offshore gradient. While macroalgae and other invertebrates were relatively low in % cover compared to live coral and turf algae throughout the system, their increased presence and the low live coral cover at <10% in the nearshore sites was linked to poor water quality in this area. Although macroalgae can become abundant where nutrient inputs are high, a recent study (Plass-Johnson et al., 2015a) found nearly no top-down control at the site nearest shore suggesting that water conditions can also be detrimental to macroalgal proliferation. This includes light limitation as well as high DOC, which both have led to negative impacts on corals and marine algae, causing mortality and reduced net photosynthesis (Kline et al., 2006; Meyer et al., 2015). Alternatively, invertebrate filter feeders can benefit from high particulate organic matter concentrations (Fabricius, 2005), which at LL was shown to result in increased barnacle recruitment (Plass-Johnson et al., 2016a). The increase in live coral and CCA cover further offshore demonstrates the influence of increasing water quality on community structure with distance from the coast. This pattern along a cross-shelf gradient is evident in other reef systems within Indonesia (Baum et al., 2015; Breckwoldt et al., 2016; Cleary et al., 2016) and around the world (Grigg, 1995; Mallela et al., 2004; Fabricius et al., 2005; Lirman and Fong, 2007) that are directly influenced by heavily populated cities on the coastline. Coastal areas along the Great Barrier Reef, Australia similarly showed community shifts from phototrophic invertebrates to macroalgae and heterotrophic invertebrates under high nutrient and sediment loads (Fabricius et al., 2012).
The coral reefs of Spermonde Archipelago, however, seem to follow a trend of increasing dominance of turf algae, which has also recently been reported in other areas within the Indo-Pacific (McCook, 2001; Bruno et al., 2009; Smith et al., 2016). These trends of increasing turf algae are in contrast to the classical model of phase-shifts from coral to macroalgae often observed in the Caribbean (Hughes, 1994; Roff and Mumby, 2012) and the Great Barrier reef (Hughes, 1994; Bellwood et al., 2006). Turf algal cover, which was found to be negatively correlated with coral and CCA cover in Spermonde Archipelago, reached >50% cover in many sites over the 3 year study period, and was highly correlated to percent rubble and hard substrate, consisting of dead coral pavement. In 2013, in particular, an increase in turf algae was observed and was correlated to an increase in hard substrate. In a parallel study in Spermonde Archipelago, turf algae were also found to play a major role in the early succession of coral reefs, particularly in the midshelf sites, and were not found to be influenced by herbivores (Plass-Johnson et al., 2016a). These findings further support the dominance of turf algae in the Spermonde Archipelago, particularly as acute disturbances, such as destructive blast-fishing (Pet-Soede and Erdmann, 1998), storms and Ancanthaster-crown of thorns-outbreak (Plass-Johnson et al., 2015b), drive increases in relative cover of rubble and dead coral which then become available as substrate. In order to understand whether phases of turf algal dominance represent a transitional reef state or a resilient stable state due to the presence of an unbreakable negative feedback loop (Nyström et al., 2012; Glaser et al., 2018), further knowledge is needed on the ecological role of turf algae in reef succession and recovery, and the relative controls acting upon them.
An additional observation in the coral reef benthic community structure that did not follow an on-to-offshore gradient, but was nonetheless important, was the increase in the relative cover of sponge and cyanobacteria cover that occurred in the mid-offshore islands. Sponge and cyanobacteria were positively correlated to each other as well as to rubble and hard substrate, and therefore, may also be linked to acute physical disturbances. Within the sampling periods, high sponge cover was observed to increase dramatically over parts of the coral reef in highly physically disturbed areas exposed to blast fishing (Plass-Johnson, 2015). Another interesting association with the increase in cyanobacteria was the higher inorganic nitrogen concentrations in the offshore reefs, which may be in part driven by cyanobacteria that are capable of N2 fixation (Biegala and Raimbault, 2008; Charpy et al., 2012a,b; Benavides et al., 2017). As cyanobacteria can quickly overgrow bare substrate or dead coral skeletons, increased N2 fixation rates have been observed on coral reefs after A. planci-crown of thorns-outbreak or bleaching mortality (Larkum, 1988; Davey et al., 2008). High sponge cover could also be responsible for the increased DIN content in the water at KS due to associations of the sponges with nitrifying and N2 fixing bacteria (Diaz and Ward, 1997).
Although significant patterns in environmental variables and coral reef benthic community structure were found along the cross-shelf-gradient, discerning the individual environmental variables driving community structure was more difficult. This is primarily due to the discrepancy between indicators with different time scale integration. Even though comparisons were conducted in the same season each year, there remains a major source of variability and error due primarily to variation in responses of hydrological variables across time as well as the magnitude and variation of these differences, which was not similarly observed in the benthic community as a whole. Despite these drawbacks, several important trends emerged. First, the multivariate analyses showed that the benthic community responded to PC1, driven by differences in Chl a, DO, and pH, in 2012, the year in which these variables combined showed signs of eutrophic conditions. Second, in 2013, when eutrophic conditions and temperature were lower, the benthic community responded both to PC1 and PC2, in which PC2 was driven by differences primarily in temperature. Some individual groups responded more to PC1 and others more to PC2. Turf algae, macroalgae, and other invertebrates, which all were slightly higher in percent cover in 2013, were among the groups significantly related to PC2. Thirdly, in 2014, when salinity was generally found to be higher, benthic community structure, primarily live coral and CCA, responded to PC2, driven also in part by differences in salinity.
As previously discussed, the degree of variability among salinity and temperature measurements over varying time-scales needs to be considered when interpreting long-term changes in community composition. This high variability in coral reef community spatial and temporal responses to environmental variables points out the complexity of interpreting the multiple environmental drivers of community structure. It also highlights the need for monitoring at a higher temporal resolution that may help to better predict relationships between water parameters and benthic biota and structure, helping to better understand drivers of coral reef status. Cooper et al. (2009) reviewed coral reef bioindicators and identified a number that were useful as time-integrated measures of water quality, responding from minutes to months to years. Knowledge of ecological and physiological response times of organisms and communities helps to interpret relationships to different water quality parameters.
Recommended Biotic Indices of Coral Reef Status and Health for Future Monitoring
Among the environmental variables analyzed, several are recommended to include in future monitoring of Spermonde Archipelago. The PCA of the full dataset of environmental variables confirmed that Chl a-like fluorescence, DO, pH, temperature, and salinity were among the most important driving variables along the on-to-offshore gradient, and that Chl a-like fluorescence correlated quite well with SPM, Kd, and DOC, and can therefore serve as a proxy indicator for eutrophication symptoms when monitoring is limited.
Chl a, DO, and pH are easily obtained measurements that were found to significantly impact coral reef benthic composition. The change in magnitude of these variables across years can also be used as an indication of changes in nutrient availability over time. Small changes in temperature and salinity were also found to explain some of the variation in coral reef community composition, but while useful for understanding potential climate responses, would need to be monitored on a more frequent basis to differentiate short term variability from long term, climate change community responses. Other environmental variables, such as inorganic nutrient concentrations, were less reliable indicators of coral reef condition, showing contrasting spatial patterns with distance over time; however, NOx:P ratios showed some importance in comparing changes in nutrient limitation among nearshore, midshelf, and offshore reefs.
The benthic index which measures relative dominance of coral reef benthic organisms effectively revealed the influence of chronic cross-shelf water quality on the coral reef benthic community. The Atlantic Gulf Rapid Reef Assessment program (AGRRA) has used the same benthic index to measure the relative cover of reef “promotors” and “detractors” (http://www.agrra.org/coral-reef-monitoring/benthos-indicator/), in order to have an integrated metric for assessing reef health. A recent analysis of a large reef data set of over 50 central Pacific reefs across five archipelagos used a similar comparative approach of cover of reef builders (corals and CCA) to fleshy algae (macroalgae, turf algae), and revealed a shift in dominance from reef builders to fleshy algae across the region, particularly around inhabited reefs (Smith et al., 2016). Reef rugosity also effectively demonstrated acute impacts of other local physical disturbances to midshelf sites, such as destructive fishing methods and an Ancanthaster planci-crown of thorns-outbreak (Plass-Johnson et al., 2015b), providing a clear picture of rapid reef decline and recovery among the 3 years. However, reef rugosity did not fit the general cross-shelf gradient at the furthest offshore site, KP. This may be due to its location on the edge of a shelf. Horizontal extension of the reef is limited by the shelf wall while the hard carbonate substratum limits erosion due to wave energy. This differs to the other sites where coral growth facilitated horizontal growth and wave energy contributed to the modification of this structure. This has resulted in the reef being located on a slope allowing for increased horizontal and vertical complexity. Except for KP, the ability of these two indices to detect changes over time, and decline and recovery processes, make them very useful and suitable for long term monitoring in Spermonde Archipelago as well as potentially other similar reef systems.
The spatio-temporal analysis of water quality and benthic community structure of Spermonde Archipelago revealed several important considerations for future monitoring of reef status and health. First, water quality variables showed a higher degree of variability than benthic community structure over time, and therefore, should be monitored across different time intervals. While monthly monitoring is recommended to better capture changes in water quality, seasonal to annual monitoring of benthic community cover, as well as the benthic index and rugosity, is adequate to capture shifts in dominance of benthic groups as well as periods of reef decline and recovery. To more accurately determine causal relationships among environmental variables and relative benthic cover, more frequent monitoring of both environmental variables and benthic cover is recommended over monthly to bimonthly intervals. This sampling would have the advantage of observing strong acute changes (e.g., live coral loss/increases in fleshy algae due to A. planci outbreak or storms) and their cause, but this regime may be impractical given the financial and human resources needed. At a minimum, monitoring over an annual interval would allow managers and resource users to understand the long-term trends in reef status. Finally, the use of permanent transects would decrease the variability in the dataset and better show local trends. A moving window approach for data analysis of longer time series (when available) may smooth out or emphasize general trends.
Author Contributions
MT, CW, and SF conceived the project idea. The data collection was organized by MT, CW, JP-J, HR, SF, and ML. All contributing authors participated in collection of data. MT and JP-J led data analysis. MT wrote the manuscript with improvements from all contributing authors.
Funding
This study was funded by the German Federal Ministry of Education and Research (BMBF; Grant No. 03F0643A) under the bilateral German Indonesian project, Science for the Protection of Indonesian Coastal Ecosystems (SPICE) III. SF acknowledges additional funding from the German Federal Ministry of Education and Research, BMBF (REPICORE project, grant number 01LN1303A).
Conflict of Interest Statement
The authors declare that the research was conducted in the absence of any commercial or financial relationships that could be construed as a potential conflict of interest.
Acknowledgments
We want to thank the University of Hasanuddin for access to the Barrang Lompo research station, Matthias Birkicht and Dorothea Dasbach for technical help with nutrient analyses of water, sediment, and plant tissue, and Agustín Moreira Saporiti for sample preparation and analysis. The Indonesian Ministry for Research and Technology (RISTEK) kindly provided permission to carry out this research (permits 353/SIP/FRP/SM/IX/2012 and 3544/FRP/SM/X/2014).
Supplementary Material
The Supplementary Material for this article can be found online at: https://www.frontiersin.org/articles/10.3389/fmars.2018.00033/full#supplementary-material
References
Adey, W. H. (1998). Coral Reefs: algal structured and mediated ecosystems in shallow, turbulent, alkaline waters. J. Phycol. 34, 393–406. doi: 10.1046/j.1529-8817.1998.340393.x
Alvarez-Filip, L., Dulvy, N. K., Gill, J. A., Cote, I. M., and Watkinson, A. R. (2009) Flattening of Caribbean coral reefs: region-wide declines in architectural complexity. Proc. R. Soc. B 276:3019–3025. doi: 10.1098/rspb.2009.0339
Alvarez-Filip, L., Gill, J. A., Dulvy, N. K., Perry, A. L., Watkinson, A. R., and Côté, I. M. (2011). Drivers of region-wide declines in architectural complexity on Caribbean reefs. Coral Reefs 30, 1051–1060. doi: 10.1007/s00338-011-0795-6
Alvarez-Filip, L., Paddack, M. J., Collen, B., Robertson, D. R., and Côté, I. M. (2015). Simplification of Caribbean reef-fish assemblages over decades of coral reef degradation. PLoS ONE 10:e0126004. doi: 10.1371/journal.pone.0126004
Baum, G., Januar, H. I., Ferse, S. C. A., and Kunzmann, A. (2015). Local and regional impacts of pollution on coral reefs along the thousand islands north of the megacity Jakarta, Indonesia. PLoS ONE 10:e0138271. doi: 10.1371/journal.pone.0138271
Becking, L., Cleary, D., De Voogd, N., Renema, W., De Beer, M., Van Soest, R. W. M., et al. (2006). Beta diversity of tropical marine benthic assemblages in the Spermonde Archipelago, Indonesia. Mar. Ecol. 27, 67–88. doi: 10.1111/j.1439-0485.2005.00051.x
Bellwood, D. R., Hoey, A. S., Ackerman, J. L., and Depczynski, M. (2006). Coral bleaching, reef fish community phase shifts and the resilience of coral reefs. Glob. Chang. Biol. 12, 1587–1594. doi: 10.1111/j.1365-2486.2006.01204.x
Benavides, M., Bednarz, V. N., and Ferrier-Pagès, C. (2017). Diazotrophs: overlooked key players within the coral symbiosis and tropical reef ecosystems? Front. Mar. Sci. 4:10. doi: 10.3389/fmars.2017.00010
Biegala, I. C., and Raimbault, P. (2008). High abundance of diazotrophic picocyanobacteria (<3 Ã,Âμm) in a Southwest Pacific coral lagoon. Aquatic Microbial Ecol. 51, 45–53. doi: 10.3354/ame01185
Breckwoldt, A., Dsikowitzky, L., Baum, G., Ferse, S. C. A., van der Wulp, S., Kusumanti, I., et al. (2016). A review of stressors, uses and management perspectives for the larger Jakarta Bay Area, Indonesia. Mar. Pollut. Bull. 110, 790–794. doi: 10.1016/j.marpolbul.2016.08.040
Bruno, J. F., Sweatman, H., Precht, W. F., Selig, E. R., and Schutte, V. G. (2009). Assessing evidence of phase shifts from coral to macroalgal dominance on coral reefs. Ecology 90, 1478–1484. doi: 10.1890/08-1781.1
Burke, L., Reytar, K., Spalding, M., and Perry, A. (2012). Reefs at Risk Revisited in the Coral Triangle. Washington, DC: World Resource Institute.
Burke, L., Reytar, K., Spalding, M., and Perry, A. (2011). Reefs at risk: Revisited. Defenders 74, 116.
Charpy, L., Casareto, B. E., Langlade, M. J., and Suzuki, Y. (2012a). Cyanobacteria in coral reef ecosystems: a review. J. Mar. Biol. 2012, 9. doi: 10.1155/2012/259571
Charpy, L., Palinska, K. A., Abed, R. M. M., Langlade, M. J., and Golubic, S. (2012b). Factors influencing microbial mat composition, distribution and dinitrogen fixation in three western Indian Ocean coral reefs. Eur. J. Phycol. 47, 51–66. doi: 10.1080/09670262.2011.653652
Cleary, D. F. R., Becking, L. E., De Voogd, N. J., Renema, W., De Beer, M., Van Soest, R. W. M., et al. (2005). Variation in the diversity and composition of benthic taxa as a function of distance offshore, depth and exposure in the Spermonde Archipelago, Indonesia. Estuar. Coast. Shelf Sci. 65, 557–570. doi: 10.1016/j.ecss.2005.06.025
Cleary, D. F. R., Polónia, A. R. M., Renema, W., Hoeksema, B. W., Rachello-Dolmen, P. G., Moolenbeek, R. G., et al. (2016). Variation in the composition of corals, fishes, sponges, echinoderms, ascidians, molluscs, foraminifera and macroalgae across a pronounced in-to-offshore environmental gradient in the Jakarta Bay–Thousand Islands coral reef complex. Mar. Pollut. Bull. 110, 701–717. doi: 10.1016/j.marpolbul.2016.04.042
Cleary, D. F. R., Vantier, L., Vail, L., Manto, P., Voogd, N. J., Rachello-Dolmen, P. G., et al. (2008). Relating variation in species composition to environmental variables: a multi-taxon study in an Indonesian coral reef complex. Aquat. Sci. 70, 419–431. doi: 10.1007/s00027-008-8077-2
Cole, M. L., Valiela, I., Kroeger, K. D., Tomasky, G. L., Cebrian, J., Wigand, C., et al. (2004). Assessment of a δ15N isotopic method to indicate anthropogenic eutrophication in Aquatic ecosystems. J. Environ. Qual. 33, 124–132. doi: 10.2134/jeq2004.1240
Cooper, T. F., Gilmour, J. P., and Fabricius, K. E. (2009). Bioindicators of changes in water quality on coral reef: review and recommendations for monitoring programmes. Coral Reefs 28, 589–606 doi: 10.1007/s00338-009-0512-x
Cooper, T. F., Uthicke, S., Humphrey, C., and Fabricius, K. E. (2007). Gradients in water column nutrients, sediment parameters, irradiance and coral reef development in the whitsunday region, central great barrier reef. Estuar. Coast. Shelf Sci. 74, 458–470. doi: 10.1016/j.ecss.2007.05.020
Costanzo, S. D., O'donohue, M. J., Dennison, W. C., Loneragan, N. R., and Thomas, M. (2001). A new approach for detecting and mapping sewage impacts. Mar. Pollut. Bull. 42, 149–156. doi: 10.1016/S0025-326X(00)00125-9
Dailer, M. L., Ramey, H. L., Saephan, S., and Smith, C. M. (2012). Algal δ15N values detect a wastewater effluent plume in nearshore and offshore surface waters and three-dimensionally model the plume across a coral reef on Maui, Hawai'i, USA. Mar. Pollut. Bull. 64, 207–213. doi: 10.1016/j.marpolbul.2011.12.004
Davey, M., Holmes, G., and Johnstone, R. (2008). High rates of nitrogen fixation (acetylene reduction) on coral skeletons following bleaching mortality. Coral Reefs 27, 227–236. doi: 10.1007/s00338-007-0316-9
de Voogd, N., Cleary, D., Hoeksema, B., Noor, A., and Van Soest, R. (2006). Sponge beta diversity in the Spermonde Archipelago, SW Sulawesi, Indonesia. Mar. Ecol. Prog. Ser. 309, 131–142. doi: 10.3354/meps309131
Deutsch, B., and Voss, M. (2006). Anthropogenic nitrogen input traced by means of δ15N values in macroalgae: results from in-situ incubation experiments. Sci. Total Environ. 366, 799–808. doi: 10.1016/j.scitotenv.2005.10.013
Diaz, M. C., and Ward, B. B. (1997). Sponge-mediated nitrification in tropical benthic communities. Mar. Ecol. Prog. Ser. 156, 97–107. doi: 10.3354/meps156097
Edinger, E. N., Jompa, J., Limmon, G. V., Widjatmoko, W., and Risk, M. J. (1998). Reef degradation and coral biodiversity in Indonesia: effects of land-based pollution, destructive fishing practices and changes over time. Mar. Pollut. Bull. 36, 617–630. doi: 10.1016/S0025-326X(98)00047-2
Edinger, E. N., Kolasa, J., and Risk, M. J. (2000a). Biogeographic variation in coral species diversity on coral reefs in three regions of indonesia. Div. Distrib. 6, 113–127. doi: 10.1046/j.1472-4642.2000.00076.x
Edinger, E. N., Limmon, G. V., Jompa, J., Widjatmoko, W., Heikoop, J. M., and Risk, M. J. (2000b). Normal coral growth rates on dying reefs: are coral growth rates good indicators of reef health? Mar. Pollut. Bull. 40, 404–425. doi: 10.1016/S0025-326X(99)00237-4
English, S. A., Baker, V. J., and Wilkinson, C. R. (1997). Survey Manual for Tropical Marine Resources. Townsville, QLD: Australian Institute of Marine Science.
Fabricius, K., De'ath, G., McCook, L., Turak, E., and Williams, D. M. (2005). Changes in algal, coral and fish assemblages along water quality gradients on the inshore great Barrier Reef. Mar. Pollut. Bull. 51, 384–398. doi: 10.1016/j.marpolbul.2004.10.041
Fabricius, K. E. (2005). Effects of terrestrial runoff on the ecology of corals and coral reefs: review and synthesis. Mar. Pollut. Bull. 50, 125–146. doi: 10.1016/j.marpolbul.2004.11.028
Fabricius, K. E., Cooper, T. F., Humphrey, C., Uthicke, S., De'ath, G., Davidson, J., et al. (2012). A bioindicator system for water quality on inshore coral reefs of the great barrier reef. Mar. Pollut. Bull. 65, 320–332. doi: 10.1016/j.marpolbul.2011.09.004
Ferse, S. C. A., Glaser, M., Neil, M., and Schwerdtner Máñez, K. (2014). To cope or to sustain? Eroding long-term sustainability in an Indonesian coral reef fishery. Reg. Environ. Change 14, 2053–2065. doi: 10.1007/s10113-012-0342-1
Flower, J., Ortiz, J. C., Chollett, I., Abdullah, S., Castro-Sanguino, C., Hock, K., et al. (2017). Interpreting coral reef monitoring data: a guide for improved management decisions. Ecol. Indic. 72, 848–869. doi: 10.1016/j.ecolind.2016.09.003
Furnas, M., Mitchell, A., Skuza, M., and Brodie, J. (2005). In the other 90%: phytoplankton responses to enhanced nutrient availability in the great barrier reef Lagoon. Mar. Pollut. Bull. 51, 253–265. doi: 10.1016/j.marpolbul.2004.11.010
Glaser, M., Plass-Johnson, J. G., Ferse, S. C. A., Neil, M., Satari, D. Y., Teichberg, M., et al. (2018). Breaking resilience for a sustainable future: thoughts for the anthropocene. Front. Mar. Sci. 5:34. doi: 10.3389/fmars.2018.00034
Grigg, R. W. (1995). Coral reefs in an urban embayment in Hawaii: a complex case history controlled by natural and anthropogenic stress. Coral Reefs 14, 253–266. doi: 10.1007/BF00334349
Heaton, T. H. E. (1986). Isotopic studies of nitrogen pollution in the hydrosphere and atmosphere: a review. Chem. Geol. 59, 87–102. doi: 10.1016/0168-9622(86)90059-X
Heikoop, J. M., Risk, M. J., Lazier, A. V., Edinger, E. N., Jompa, J., Limmon, G. V., et al. (2000). Nitrogen-15 signals of anthropogenic nutrient loading in reef corals. Mar. Pollut. Bull. 40, 628–636. doi: 10.1016/S0025-326X(00)00006-0
Hill, J. and Wilkinson, C. (2004). Methods for Ecological Monitoring of Coral Reefs. Townsville, QLD: Australian Institute of Marine Science, 123.
Hoeksema, B. (2012). Distribution patterns of mushroom corals (Scleractinia: Fungiidae) across the spermonde shelf, South Sulawesi. Raffles Bull. Zool. 60, 183–212.
Howarth, R. W., and Marino, R. (2006). Nitrogen as the limiting nutrient for eutrophication in coastal marine ecosystems: evolving views over three decades. Limnol. Oceanogr. 51, 364–376. doi: 10.4319/lo.2006.51.1_part_2.0364
Hughes, T. (1994). Catastrophes, phase shifts, and large-scale degradation of a Caribbean coral reef. Science 265, 1547–1551. doi: 10.1126/science.265.5178.1547
Jameson, S. C., Erdmann, M. V., Karr, J. R., and Potts, K. W. (2001). Charting a course toward diagnostic monitoring: a continuing review of coral reef attributes and a research strategy for creating coral reef indexes of biotic integrity. Bull. Mar. Sci. 69, 701–744.
Jessen, C., Bednarz, V. N., Rix, L., Teichberg, M., and Wild, C. (2015). “Marine eutrophication,” in Environmental Indicators, eds R. H. Armon and O. Hänninen (Dordrecht: Springer Science+Besiness Media), 177–203.
Jouffray, J.-B., Nyström, M., Norstrom, A. V., Williams, I. D., Wedding, L. M., Kittinger, J. N., et al. (2014). Identifying multiple coral reef regimes and their drivers across the Hawaiian archipelago. Philos. Trans. R. Soc. B 370:20130268. doi: 10.1098/rstb.2013.0268
Kegler, H. F., Lukman, M., Teichberg, M., Plass-Johnson, J., Hassenrück, C., Wild, C., et al. (2017). Bacterial community composition and potential driving factors in different reef habitats of the Spermonde Archipelago, Indonesia. Front. Microbiol. 8:662. doi: 10.3389/fmicb.2017.00662
Kench, P. S., and Mann, T. (2017). Reef island evolution and dynamics:Insights from the Indian and Pacific oceans and perspectives for the Spermonde Archipelago. Front. Mar. Sci. 4:145. doi: 10.3389/fmars.2017.00145
Kirk, J. T. O. (2011). Light and Photosynthesis in Aquatic Ecosystems. Cambridge: Cambridge University Press.
Kline, D. I., Kuntz, N. M., Breitbart, M., Knowlton, N., and Rohwer, F. (2006). Role of elevated organic carbon levels and microbial activity in coral mortality. Mar. Ecol. Prog. Ser. 314, 119–125. doi: 10.3354/meps314119
Kohler, K. E., and Gill, S. M. (2006). Coral point count with excel extensions (CPCe): a visual basic program for the determination of coral and substrate coverage using random point count methodology. Comput. Geosci. 32, 1259–1269. doi: 10.1016/j.cageo.2005.11.009
Kramer, P. A. (2003). Synthesis of coral reef health indicators for the Western Atlantic: results of the AGRRA Program (1997-2000). Atoll Res. Bull. 496, 1–55. doi: 10.5479/si.00775630.496-3.1
Lang, J. C., Marks, K. W., Kramer, P. A., Richards Kramer, P., and Gingsburg, R. N. (2010). AGRRA Protocols Version 5.4. Atlantic and Gulf Rapid Reef Assessment Program, 31.
Lapointe, B. E. (1997). Nutrient thresholds for bottom-up control of macroalgal blooms on coral reefs in Jamaica and southeast Florida. Limnol. Oceanogr. 42, 1119–1131. doi: 10.4319/lo.1997.42.5_part_2.1119
Lapointe, B. E., Barile, P. J., Littler, M. M., and Littler, D. S. (2005). Macroalgal blooms on southeast Florida coral reefs: II. Cross-shelf discrimination of nitrogen sources indicates widespread assimilation of sewage nitrogen. Harmful Algae 4, 1106–1122. doi: 10.1016/j.hal.2005.06.002
Larkum, A. W. D. (1988). High rates of nitrogen fixation on coral skeletons after predation by the crown of thorns starfish Acanthaster planci. Mar. Biol. 97, 503–506. doi: 10.1007/BF00391046
Larned, S. T. (1998). Nitrogen- versus phosphorus-limited growth and sources of nutrients for coral reef macroalgae. Mar. Biol. 132, 409–421. doi: 10.1007/s002270050407
Lindau, C. W., Delaune, R. D., Patrick, W. H., and Lambremont, E. N. (1989). Assessment of stable nitrogen isotopes in fingerprinting surface water inorganic nitrogen sources. Water Air Soil Pollut. 48, 489–496. doi: 10.1007/BF00283346
Lirman, D., and Fong, P. (2007). Is proximity to land-based sources of coral stressors an appropriate measure of risk to coral reefs? An example from the florida reef tract. Mar. Pollut. Bull. 54, 779–791. doi: 10.1016/j.marpolbul.2006.12.014
Lukman, M., Nasir, A., Amri, K., Tambaru, R., Hatta, M., Nurfadilah, N., et al. (2014). Dissolved silicate in coastal water of South Sulawesi. Jurnal Ilmu dan Teknologi Kelautan Tropis 6, 461–478.
Mallela, J., Perry, C. T., and Haley, M. P. (2004). Reef morphology and community structure along a fluvial gradient, Rio Bueno, Jamaica. Caribbean J. Sci. 40, 299–311.
McCook, L. (2001). Competition between corals and algal turfs along a gradient of terrestrial influence in the nearshore central great barrier reef. Coral Reefs, 19, 419–425. doi: 10.1007/s003380000119
Meyer, F. W., Vogel, N., Teichberg, M., Uthicke, S., and Wild, C. (2015). The physiological response of two green calcifying algae from the great barrier reef towards high dissolved inorganic and organic carbon (DIC and DOC) Availability. PLoS ONE 10:e0133596. doi: 10.1371/journal.pone.0133596
Murphy, J., and Riley, J. P. (1962). A modified single solution method for the determination of phosphate in natural waters. Anal. Chim. Acta 27, 31–36. doi: 10.1016/S0003-2670(00)88444-5
Nasir, A., Lukman, M., Tuwo, A., Hatta, M., Tambaru, R., and Nurfadilah (2016). The use of C/N ratio in assessing the influence of land-based material in coastal water of South Sulawesi and Spermonde Archipelago, Indonesia. Front. Mar. Sci. 3:266. doi: 10.3389/fmars.2016.00266
Nasir, A., Tuwo, A., Lukman, M., and Usman, H. (2015). Impact of increased nutrient on the variability of chlorophyll-a in the west coast of South Sulawesi, Indonesia. Int. J. Sci. Eng. Res. 6, 821–826.
Nyström, M., Norström, A. V., Blenckner, T., De La Torre-Castro, M., Eklöf, J. S., Folke, C., et al. (2012). Confronting feedbacks of degraded marine ecosystems. Ecosystems 15, 695–710. doi: 10.1007/s10021-012-9530-6
Öhman, M. C., Rajasuriya, A., and Ólafsson, E. (1997). Reef fish assemblages in north-western Sri Lanka: distribution patterns and influeces of fishing practises. Environ. Biol. Fish. 49, 45–61. doi: 10.1023/A:1007309230416
Pet-Soede, L., and Erdmann, M. (1998). An overview and comparison of destructive fishing practices in Indonesia. SPC Live Reef Fish Inf. Bull. 4, 28–36.
Plass-Johnson, J. G. (2015). The Functioning of Coral Reef Communities Along Environmental Gradients. Bremen: University of Bremen.
Plass-Johnson, J. G., Teichberg, M., Bednarz, V. N., Gärdes, A., Heiden, J. P., Lukman, M., et al. (2018). Spatio-temporal patterns in the coral reef communities of the spermonde archipelago, 2012–2014, II: fish assemblages display structured variation related to benthic condition. Front. Mar. Sci. 5:36. doi: 10.3389/fmars.2018.00036
Plass-Johnson, J. G., Ferse, S. C. A., Jompa, J., Wild, C., and Teichberg, M. (2015a). Fish herbivory as key ecological function in a heavily degraded coral reef system. Limnol. Oceanogr. 60, 1382–1391. doi: 10.1002/lno.10105
Plass-Johnson, J. G., Heiden, J. P., Abu, N., Lukman, M., and Teichberg, M. (2016a). Experimental analysis of the effects of consumer exclusion on recruitment and succession of a coral reef system along a water quality gradient in the Spermonde Archipelago, Indonesia. Coral Reefs 35, 229–243. doi: 10.1007/s00338-015-1369-9
Plass-Johnson, J. G., Schwieder, H., Heiden, J., Weiand, L., Wild, C., Jompa, J., et al. (2015b). A recent outbreak of crown-of-thorns starfish (Acanthaster planci) in the Spermonde Archipelago, Indonesia. Reg. Environ. Change 15, 1157–1162. doi: 10.1007/s10113-015-0821-2
Plass-Johnson, J. G., Taylor, M. H., Husain, A. A. A., Teichberg, M. C., and Ferse, S. C. A. (2016b). Non-random variability in functional composition of coral reef fish communities along an environmental gradient. PLoS ONE 11:e0154014. doi: 10.1371/journal.pone.0154014
Polonia, A. R., Cleary, D. F., de Voogd, N. J., Renema, W., Hoeksema, B. W., Martins, A., et al. (2015). Habitat and water quality variables as predictors of community composition in an Indonesian coral reef: a multi-taxon study in the Spermonde Archipelago. Sci. Total Environ. 537, 139–151. doi: 10.1016/j.scitotenv.2015.07.102
Renema, W., and Troelstra, S. R. (2001). Larger foraminifera distribution on a mesotrophic carbonate shelf in SW Sulawesi (Indonesia). Palaeogeogr. Palaeoclimatol. Palaeoecol. 175, 125–146. doi: 10.1016/S0031-0182(01)00389-3
Risk, M. J. (1972). Fish diversity on a coral reef in the Virgin Island. Atoll Res. Bull. 153, 1–6. doi: 10.5479/si.00775630.153.1
Roff, G., and Mumby, P. J. (2012). Global disparity in the resilience of coral reefs. Trends Ecol. Evol. 27, 404–413. doi: 10.1016/j.tree.2012.04.007
Savage, C., and Elmgren, R. (2004). Macroalgal (Fucus vesiculosus) δ15N values trace decrease in sewage influence. Ecol. Applic. 14, 517–526. doi: 10.1890/02-5396
Savage, C., Leavitt, P. R., and Elmgren, R. (2004). Distribution and retention of effluent nitrogen in surface sediments of a coastal bay. Limnol. Oceanogr. 49, 1503–1511. doi: 10.4319/lo.2004.49.5.1503
Sawall, Y., Jompa, J., Litaay, M., Maddusila, A., and Richter, C. (2013). Coral recruitment and potential recovery of eutrophied and blast fishing impacted reefs in Spermonde Archipelago, Indonesia. Mar. Pollut. Bull. 74, 374–382. doi: 10.1016/j.marpolbul.2013.06.022
Sawall, Y., Teichberg, M. C., Seemann, J., Litaay, M., Jompa, J., and Richter, C. (2011). Nutritional status and metabolism of the coral Stylophora subseriata along a eutrophication gradient in Spermonde Archipelago (Indonesia). Coral Reefs 30, 841–853. doi: 10.1007/s00338-011-0764-0
Smith, J. E., Brainard, R., Carter, A., Grillo, S., Edwards, C., Harris, J., et al. (2016). Re-evaluating the health of coral reef communities: baselines and evidence for human impacts across the central Pacific. Proc. R. Soc. B 283:20151985. doi: 10.1098/rspb.2015.1985
Smith, S. V., Kimmerer, W. J., Laws, E. A., Brock, R. E., and Walsh, T. W. (1981). Kaneohe Bay Sewage diversion experiment - perspectives on ecosystem responses to nutritional perturbation. Pacific. Sci. 35, 279–402.
Smith, T. B., Nemeth, R. S., Blondeau, J., Calnan, J. M., Kadison, E., and Herzlieb, S. (2008). Assessing coral reef health across onshore to offshore stress gradients in the US Virgin Islands. Mar. Pollut. Bull. 56, 1983–1991. doi: 10.1016/j.marpolbul.2008.08.015
Strickland, J. D. H., and Parsons, T. R. (1972). A Practical Handbook of Seawater Analysis. Ottawa: Fisheries Research Board of Canada.
Sur, C., Abbott, J. M., Ambo-Rappe, R., Asriani, N., Hameed, S. O., Jellison, B. M., et al. (2018). Marine debris on small islands: Insights from an educational outreach program in the Spermonde Archipelago, Indonesia. Front. Mar. Sci. 5:35. doi: 10.3389/fmars.2018.00035
Umezawa, Y., Miyajima, T., Tanaka, Y., Koike, I., and Hayashibara, T. (2007). Variation in internal δ15N and δ13C distributions and their bulk values in the brown macroalga Padina australis growing in subtropical oligotrophic waters. J. Phycol. 43, 437–448. doi: 10.1111/j.1529-8817.2007.00347.x
Keywords: eutrophication, water quality, turf algae, resilience, benthic index, rugosity, Indonesia
Citation: Teichberg M, Wild C, Bednarz VN, Kegler HF, Lukman M, Gärdes AA, Heiden JP, Weiand L, Abu N, Nasir A, Miñarro S, Ferse SCA, Reuter H and Plass-Johnson JG (2018) Spatio-Temporal Patterns in Coral Reef Communities of the Spermonde Archipelago, 2012–2014, I: Comprehensive Reef Monitoring of Water and Benthic Indicators Reflect Changes in Reef Health. Front. Mar. Sci. 5:33. doi: 10.3389/fmars.2018.00033
Received: 14 February 2017; Accepted: 24 January 2018;
Published: 16 February 2018.
Edited by:
Christian Grenz, UMR7294 Institut Méditerranéen d'Océanographie (MIO), FranceReviewed by:
Dionysios E. Raitsos, Plymouth Marine Laboratory, United KingdomRenaud Fichez, IRD, France
Copyright © 2018 Teichberg, Wild, Bednarz, Kegler, Lukman, Gärdes, Heiden, Weiand, Abu, Nasir, Miñarro, Ferse, Reuter and Plass-Johnson. This is an open-access article distributed under the terms of the Creative Commons Attribution License (CC BY). The use, distribution or reproduction in other forums is permitted, provided the original author(s) and the copyright owner are credited and that the original publication in this journal is cited, in accordance with accepted academic practice. No use, distribution or reproduction is permitted which does not comply with these terms.
*Correspondence: Mirta Teichberg, bWlydGEudGVpY2hiZXJnQGxlaWJuaXotem10LmRl
†Present Address: Vanessa N. Bednarz, Marine Department, Centre Scientifique de Monaco, Monaco, Monaco;
Jasmin P. Heiden, Section Biogeoscience, Department EcoTrace, Alfred Wegener Institute for Polar and Marine Research, Bremerhaven, Germany;
Sara Miñarro, Institute of Environmental Science and Technology (ICTA), Universitat Autònoma de Barcelona, Bellaterra, Spain;
Jeremiah G. Plass-Johnson, Centre for Ocean Life, National Institute of Aquatic Resources (DTU-Aqua), Technical University of Denmark, Lyngby, Denmark