- 1Laboratorio de Ecología de Ecosistemas de Arrecifes Coralinos, Departamento de Recursos del Mar, Centro de Investigación y de Estudios Avanzados del I.P.N. Mérida, Yucatán, Mexico
- 2Departamento de Biología-CURLA, Universidad Nacional Aútonoma de Honduras, La Ceiba, Honduras
- 3Healthy Reefs for Healthy People Initiative, Smithsonian Institution, Florida, FL, United States
This manuscript provides new insights on an unusual morphological plasticity growth form on Acropora spp. in the Caribbean. This abnormal knob-shaped growth is thought to be a progression from the damselfish “chimneys” that are commonly seen in coral-algal farms. However, the diameters of the observed knobs tend to be much larger on Acropora palmata, where they range from 1.37 to 5.44 cm in diameter, and they tend to be slightly smaller on A. prolifera, where they range from 1.1 to 2.72 cm in diameter. These knob-like chimney growths can affect entire colonies. The knobs are mostly covered with live tissue, while some knobs compete with turf algae. We hypothesize that these growths may be linked to stress from multiple predation and environmental conditions. Local stressors could synergistically influence the regeneration of scarred tissue and skeleton that result from predatory lesions, possibly leading to the formation of the knobs. Therefore, we provide preliminary data from a shallow reef site in coastal Honduras located within the Mesoamerican region where we found the knobs. To the best of our knowledge, the conditions that drive the occurrence of these unusual “knob-like chimneys” on Acropora spp. have not been previously assessed. Thus, we propose a series of guidelines to research the coral morphological plasticity that may be linked to this knob-like chimney phenomenon.
Introduction
The assessment of the remaining acroporids in the western Atlantic is of extreme relevance due to their widespread decline over the last 30 years (Aronson and Precht, 2001). Impacts associated with climate change, diseases, hurricanes and anthropogenic disturbances have caused major ecological shifts (Schutte et al., 2010; Williams et al., 2017). According to the International Union for Conservation of Nature (IUCN)1 Red List, these Acropora species are considered as “critically endangered” (Aronson et al., 2008). They are also highly susceptible to at least six diseases and growth anomalies in the Caribbean (Bak, 1983; Weil et al., 2006). Thermal stress and microbes linked to poor water quality increase the vulnerability of these species to other stressors (Sutherland et al., 2011; Zaneveld et al., 2016). However, the understanding of the synergistic impacts of vector-borne diseases, multiple-predator outbreaks, environmental stressors and recovery in the Caribbean is still advancing (Weil, 2004; Shaver et al., 2017). These complex associations may influence the recovery, morphological plasticity and overall coral health of Acropora spp. (Casey et al., 2014; Schopmeyer and Lirman, 2015; Vermeij et al., 2015; Bright et al., 2016).
Scleractinian corals have diverse mechanisms (physiological, morphological and genetic) to respond to biological and environmental stress (Klaus et al., 2007; Todd, 2008; Tambutté et al., 2011, 2015). Phenotypic plasticity can facilitate the better fit of traits in response to an environmental stimulus, leading to a set of phenotypes produced by a genotype (Via et al., 1995). These responses may also differ at the species level due to life history strategies (Henry and Hart, 2005). Predation is a type of biotic stress that causes mechanical damage, and alters tissue regeneration and skeletal growth (Bak, 1983; Peters et al., 1986; Meesters and Bak, 1995; Lirman, 2000a,b; Grober-Dunsmore et al., 2006). Some corallivores feed solely on the live tissue or mucus of coral, while others can induce long-lasting changes in morphology (Wielgus et al., 2002; Todd, 2008). The morphological changes due to predation on corals from damselfish that cause “chimneys” date back to the fossil records from the Pleistocene (125,000 years BP) (Kaufman, 1981; Rotjan and Lewis, 2008). Other corallivores can also cause damage on coral skeleton from the burrowing of polychaetes or scraping bites (Bruckner and Bruckner, 2015). Grazed corals also defend themselves against predation, and some develop an increase in nematocyst density, while others regenerate lesions (Bak, 1983; Gochfeld, 2004). In many cases, excessive predation has led to an increase in mortality and algal competition (Meesters et al., 1996).
Combined biotic and environmental stressors can modify coral morphologies from the smallest of scales at the corallite level to the entire shape of a coral colony (Todd et al., 2004; Erftemeijer et al., 2012). Light and water movement have been the most studied of the parameters that lead to flattened growth forms (Todd, 2008). Light can also prompt changes to corallite direction and growth (Todd et al., 2004). Other factors that influence morphology include food availability, water movement, sedimentation, temperature, salinity (Bruno and Edmunds, 1997) and depth (Klaus et al., 2007). Environmental parameters may affect protein expressions and adjust the factors that drive rates of calcification, thereby changing the skeleton shape (Tambutté et al., 2011). Moreover, in an era of climate change and acidification, pH has been found to be able to cause morphological modifications to coral skeletons (Tambutté et al., 2015).
Only a few acroporid “hope spots” are still alive in the Mesoamerican region, yet they face severe threats (Sutherland et al., 2011; Rodríguez-Martínez et al., 2014; Kramer et al., 2015). These living laboratories currently maintain the patterns of reef zonation that have declined elsewhere in the Caribbean (Álvarez-Filip et al., 2009). We highlight the case of a shallow reef (2–7 m deep) along the coasts of Honduras, where we observed unusual “knob-like chimney” growth forms on entire colonies of Acropora spp. We hypothesize a linkage of these growth forms to predation, which may lead to modified skeletal growth and regeneration that is influenced by local stressors. Furthermore, we provide a general perspective on the observed coral morphological growth and ecological conditions. This paper is not meant to be a causal/mechanistic investigation. Instead, it provides insights to future research needs, regarding plasticity and predator impacts on Acropora spp. in the Caribbean. Despite numerous studies, these “knob-like chimney” growths have not been reported, or studied before.
Coral Morphology and Knobs
Acropora spp. colonies found on the fringing reefs of Cocalito (15°51′50.9″N 87°30′23.8″W), Tela Bay, Honduras have distinctive morphological characteristics. Acropora palmata (Lamark, 1816) colonies can be found growing in laminar/explanate fronds, flattened branches or encrusting forms (Figures 1A,B). The high-density stands of large A. palmata colonies (1 m tall to 2 m wide) create thickets (3–4 m wide). However, colonies this size can be more susceptible to multiple-predator impacts (Grober-Dunsmore et al., 2006). Acropora palmata colonies provide ecosystem services along with structural complexity and habitat for a diverse assemblage of reef organisms (Figure 1C). However, A. palmata and A. prolifera (Lamark, 1816) colonies exhibit abnormal skeletal growths, which we call “knob-like chimney” growth forms, that can cover entire colonies (Figures 1D,E). The assessment of field images revealed that the diameters of the knobs tended to be larger on A. palmata (1.37–5.44 cm, n = 64) and slightly smaller on A. prolifera (1.1–2.72 cm, n = 32) (Figures 2A–C). These protuberances vary and are covered by “algal tufts” or live tissue (Figures 1D,E). Acropora cervicornis colonies were not observed at this site.
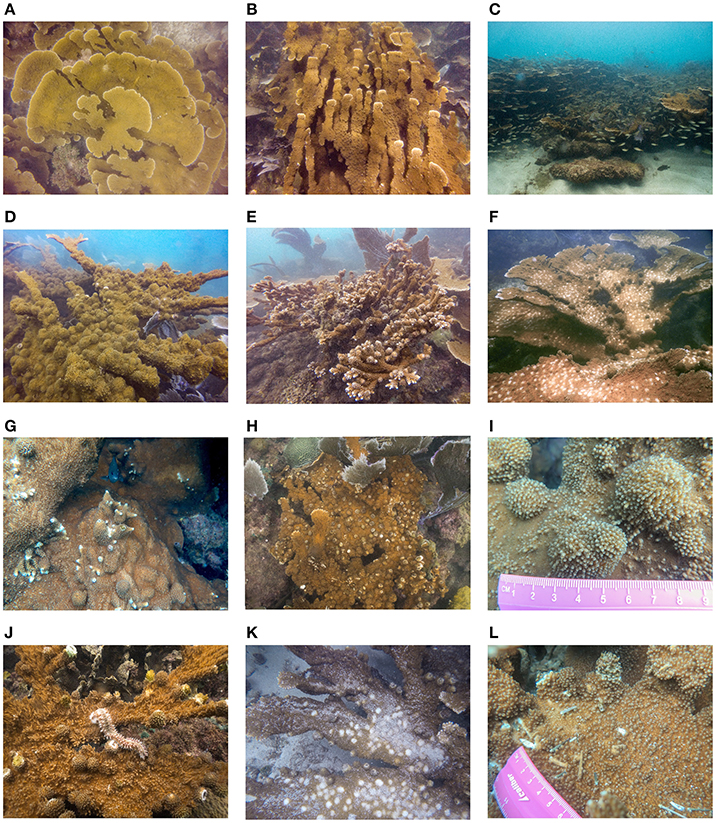
Figure 1. Acropora species and unusual growth morphologies in Cocalito located in Tela, Honduras (A) Plated morphology of Acropora palmata (B) flattened branching morphotype with small, rounded chimneys (C) abundant habitat for juvenile fish (D) entire colony of A. palmata covered with “knob-like chimneys” (E) knobs and algal turf on A. prolifera (F) lesions from fish bites on A. palmata with scattered knobs (G) close up of Stegastes planiforms with chimneys (1 cm) and knobs (2–3 cm) on the encrusted base of A. palmata (H) knobs competing with turf algae on encrusting A. palmata (I) close up of knobs (J) Hermodice carunculata feeding on knobs during the day (K) fish bites covered with sand particles (L) close up on an A. palmata branch showing growth over debris from an urchin spine. All photographs are reproduced with permission from the copyright holder, which belong to Nicole Helgason (Reefdivers.io) with the exceptions of (F,G,I,L), which were provided by Andrea Rivera-Sosa.
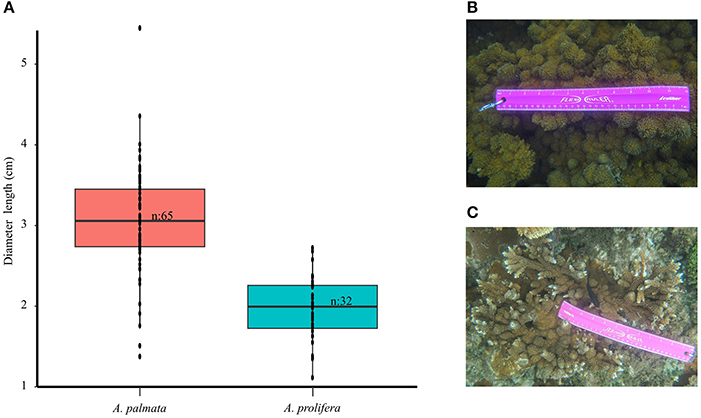
Figure 2. Range of diameters (cm) of knob-like chimney growth forms. (A) Box plot of the diameters of “knob-like chimmey” growth forms on Acropora species obtained by photo analysis and size comparison between: (B) Acropora palmata and (C) Acropora prolifera. Photograph credits belong to Andrea Rivera-Sosa.
We attribute these “knob-like chimney” growths to bites from damselfish that target live Acropora spp. to create algal gardens, and these bites create multifocal and coalescing circular lesions on the upward facing branches [(Kaufman, 1981; Peters, 1984; Work and Aeby, 2006); Figure 1F]. Filamentous algae and cyanobacteria rapidly colonize (usually after 1–2 weeks) the bite-sized lesions (Hernández-Delgado, 2000; Lirman, 2000a). Acropora palmata grows rapidly (5–10 cm year−1) and has extremely fast regeneration rates (Gladfelter et al., 1978; Meesters and Bak, 1995). Studies have shown that the smallest of lesions (2 mm2 to 5 cm2) can heal within 30 days, and this may limit algal cover (Kaufman, 1981; Bak, 1983; Lirman, 2000b). As a consequence, damselfish will continue to allocate energy to produce new bites (Hernández-Delgado, 2000; Bruckner and Bruckner, 2015). According to Bak (1983), a calcifying regeneration lip borders the lesion and grows vertically (and may be hollow) while encapsulating algae or debris. Tissue is further re-sheeted over the wound, and this may lead to the formation of large knobs over time (Figures 1G,H).
Nevertheless, the observed morphology differs from reported chimneys or gall-like growths that are usually 0.25–2.00 cm in diameter (Kaufman, 1981; Bruckner and Bruckner, 2015). Chimneys are a response from localized predation originated by Stegastes planifrons (Cuvier, 1830) and Microspathodon chrysurus (Cuvier, 1830) (Cole et al., 2008; Rotjan and Lewis, 2008). These territorial fish can actively kill coral, increase algal abundance, deter other predators, and lay eggs on algal gardens (Ceccarelli et al., 2001). These upward-thickened knobs are tall and seem to have larger corallites (Figure 1I). Some of the corallites on the branches in scattered colonies seem visually longer and irregular (Tomiak et al., 2016; Figure 1J). However, there are currently no specific data on the morphological aspects of these knobs, and accurate measurements of corallites/calices have not been conducted.
Moreover, the knobs are targeted by the bearded fireworm Hermodice carunculata (Pallas, 1766), and predation is commonly observed during the day (Figure 1J). Fireworms prefer live tissue on rounded branch tips and knobs of milleporids and acroporids, which may cause another cycle of tissue mortality and algal colonization (Witman, 1988; Bruckner et al., 2002; Miller et al., 2014; Bruckner and Bruckner, 2015). Once again, scar tissue is regenerated on the lesion, which may enhance the vertical growth of the knobs (Jordan-Dahlgren, 1992). The presence of H. carunculata is related to the abundance predators (such as lobster), habitat and food availability (Ahrens et al., 2013). H. carunculata is an opportunistic species that can regenerate asexually (Ott and Lewis, 1972). This corallivore has a high adaptability and may be a threat to the already stressed corals (Wolf et al., 2014; Schulze et al., 2017). Nevertheless, it is highly unlikely that fireworm predation occurs across all knobs. For this reason, we conducted an overview of the biotic and environmental conditions.
Stressors Related to the Knob-like Chimney Phenomenon
Environmental and biotic stressors play important roles in coral morphology and recovery from multiple predatory lesions (Sabine et al., 2015). Predation may require resources to be allocated to regeneration at the expense of new colony growth (Meesters and Bak, 1995). Regeneration rates vary with temperature, lesion location, sedimentation and food availability (Lester and Bak, 1985; Meesters et al., 1996; Cróquer et al., 2002). Even though there is a consensus that increased nutrients and sedimentation can be detrimental to coral reefs, there are some benefits to these conditions (Shaver et al., 2017). Anthony (2006), for example, found that corals on coastal and high-turbidity reefs had enhanced energy reserves and lipid levels. Specific adaptation strategies in highly turbid zones may prompt physiological responses and the dependence on coral heterotrophy, which can compensate for reduced photosynthesis (Anthony and Fabricius, 2000).
The corals in Cocalito, Honduras thrive under highly variable and often extreme environmental conditions. These conditions range from chronic turbidity (Supplementary Figure 1), high temperature, excess nutrients and substantial freshwater inputs during the rainy season (in prep). In this wave exposed location, colonies and lesions interact with suspended particles, sedimentation and other debris (Figure 1K). The capacity of A. palmata to overgrow foreign materials was evident when we observed its growth over dead sea urchin spines (Figure 1L). Therefore, it is possible that A. palmata and algae compete for space on the surface of initial chimneys that later become knobs. Damselfish may also affect bioeroding crypto-fauna, which may, in turn, impact skeletal porosity and the recruitment of burrowing polychaetes and sponges (Sammarco et al., 1986). However, a study by Zubia and Peyrot-Clausade (2001) found higher rates of microbioerosion in areas outside damselfish territories. Nevertheless, this growth response requires further assessment.
Damselfish are linked to reef degradation because they promote algal cover, predation and the fragmentation of wild and restored Acropora populations (Hernández-Delgado, 2000; Schopmeyer and Lirman, 2015). In 2016, the benthic cover around Cocalito was dominated by turf algae (40%), live coral (30%), non-aggressive invertebrates (13%) and to a lesser extent fleshy macroalgae (9%) (Supplementary Figure 2). This coral cover is higher than the average in the Caribbean (16.8%) (Jackson et al., 2014). The abundance of damselfish is also increasing on Caribbean reefs (Hernández-Delgado, 2000; Ceccarelli et al., 2001), and this can further stimulate algal growth (Vermeij et al., 2015). This major shift may be due to the low abundance or local extinction of damselfish predators, which include serranids, lutjanids, moray eels, and lizard fishes (Randall, 1967; Robertson, 1996; Hernández-Delgado, 2000; Vermeij et al., 2015). In contrast, others have suggested that damselfish abundances have historically been high (Kaufman, 1981). Moreover, others argue that damselfish densities are related to the availability of microhabitats rather than predator abundance (Precht et al., 2010). In Cocalito, the fish biomass is dominated by grunts (Haemulidae ~100 g/m2), with much lower biomass of snappers (Lutjanidae ~20 g/m2), and a similar biomass of angelfish (Pomacanthidae ~16 g/m2) (Supplementary Figure 3). There is also a low proportion of Pomacentridae biomass (~2 g/m2), which includes herbivorous species such as territorial damselfish. Nonetheless, predator abundance is below the threshold of ~40 g/m2 that Vermeij et al. (2015) suggested may lead to destructive effects on the reef. Although grunts dominate the fish biomass in Cocalito, they are not damselfish predators; their diet is comprised primarily of invertebrates (Bohnsack and Harper, 1988). However, grunts may impact the abundance and distribution of fireworms (Shantz, 2016).
Moreover, this area may also be a “hotspot” of nutrients coming from upstream watersheds, grunts, and damselfish. Grunt aggregations have been found to increase by 7–10 times the rates of organic nutrient delivery to coral colonies (Shantz et al., 2015). It is possible that these combined sources of localized nutrient deliveries may influence faster skeletal growth rates in this area of high nutrients (Bongiorni et al., 2003; Ferrier-Pagès et al., 2003; Shantz et al., 2015). This possible morphological plasticity feedback loop related to nutrients remains to be investigated.
Diseases Linked to Vectors and Abnormal Growths
Damselfish territories have been found to serve as reservoirs of microbes related to coral diseases (Casey et al., 2014). Ironically, this linkage has been poorly studied and has major implications for Acropora spp. as disease outbreaks have caused massive mortality (Aronson and Precht, 2001). However, the remaining Acropora colonies continue to be the preferred microhabitat of damselfish and disease prone vectors (Lirman, 1999; Precht et al., 2010; Bruckner and Bruckner, 2015). On many occasions, damselfish bites and white pox disease have been easily confused, but white pox tends to manifest as irregular lesions, rather than perfectly symmetrical circular lesions (Pollock et al., 2011; Bruckner and Bruckner, 2015). Other pathogens have been associated with sewage (Sutherland et al., 2011). Additionally, the transmission of diseases has been linked to common corallivores. The coral-eating snail Coralliophila abbreviata (Lamarck, 1816) was found to be associated with white band disease (Baums et al., 2003; Williams and Miller, 2005; Gignoux-Wolfsohn et al., 2012), and H. carunculata was found to be associated with the coral-bleaching pathogen Vibrio shiloi (Sussman et al., 2003). Both corallivores target stressed colonies and eat the decaying tissue of diseased corals (Miller and Williams, 2007; Wolf et al., 2014).
Acropora spp. worldwide are susceptible to growth anomalies (GAs) such as tumors, neoplasia (altered calcification patterns) and hyperplasia (number of cells in the tissue) (Bak, 1983; Peters et al., 1986; Work et al., 2008). Worldwide incidences of GAs have been associated with human populations and environmental degradation (Green and Bruckner, 2000; Aeby et al., 2011). In addition, it is important to differentiate GAs from the “knob-like chimneys” caused by damselfish on Acropora spp. (Bak, 1983; Bruckner and Bruckner, 2015). GAs on A. palmata have been found as protuberances and as skeletal growths with discolored tissue that lacks normal corallites (Bak, 1983; Peters et al., 1986; Gladfelter, 2007). Peters (1984) stated that microbial alterations to A. palalmata could occur in epidermal cells and cause hyperplasia in response to chronic physical damage associated with sediment-algae accumulations. In addition, parts of the lesions and dead skeletons could be susceptible to microbioeroders and endolithic bacteria (Tribollet, 2008).
Future Research to Resolve Current Questions
There are extensive opportunities for research related to the formation of “knob-like chimneys” on Acropora spp. Hence, we propose future studies to reduce the current knowledge gaps related to coral morphological plasticity in response to multiple predation and environmental stressors. We suspect that the skeletal formation of these knobs may be irreversible. However, many uncertainties should be explored such as the broader implications of knobs on the growth, energy expenditure and bio-construction of these corals.
Field characterization and mechanistic studies are required to answer many of the remaining questions. First, the population structure and distribution of Acropora spp. (size, cover) should be measured (Grober-Dunsmore et al., 2006), including the hybridization of A. prolifera in Cocalito, since many colonies exhibit unique morphologies (Vollmer and Palumbi, 2002). Further investigations on the spatial patterns of predation by damselfish and polychaetes (fireworms), as well as their abundances, recruitment and relationship to knob growth patterns is warranted. Monitoring present lesions and conducting new coral-wound regeneration experiments in the field under different environmental conditions may reveal the aspects that are key to recovery and algal/coral interactions (Precht et al., 2010; Wolf et al., 2014). These studies could be conducted using field assessments such as permanent transects, colony tagging, and lesion monitoring using photographs and videos. Additionally, non-destructive techniques such as cages placed around coral colonies which would protect against predation could be used to test the causal link between predation and the formation of knobs (Gochfeld, 2004).
Studies that integrate the synergistic impacts of predator lesions and recovery growth rates would be valuable. These impacts could be studied using histological analyses of affected (knob) tissue and adjacent tissues. Further analyses of coral knobs may assist in (1) determining the role of microbial communities, and (2) ruling out diseases, which are currently unknown (Mosses and Hallock, 2015; Shaver et al., 2017). Histological assays may reveal complex interactions of epidermal and gastrodermal tissues layers where microbioeroders and debris such as algae and sand could be present. To view morphological changes at the smallest of scales, techniques using scanning electron microscopy (SEM) can evaluate skeleton conditions including calcification, density, porosity and features such as corallite and calice morphology (Tomiak et al., 2016). Moreover, molecular and microscopic techniques as well as gross dissections can be employed to assess genotypes, endosymbiont density (zooxanthellae), and gonad development in these knobs which may reveal impacts on coral fitness (bleaching and reproduction) (Baums et al., 2014; Miller et al., 2016).
In addition, the temporal variation of predator abundance in relation to long-term environmental parameters such as water quality (nutrients) from biotic and land-based sources should be evaluated. Data on turbidity and nutrients in relation to climatic and oceanographic dynamics are needed as a baseline. We suggest studies on light intensity/turbidity and nutrients in relation photosynthetic activity and diversity of zooxanthellae communities (Klaus et al., 2007). These studies are critical due to the important relationship among the environment, symbionts, genotypes and morphological plasticity for coral adaptation (Tambutté et al., 2011).
In addition, targeted predator removal experiments for adaptive management need to be carried out (Miller, 2001). Synergistic stressors and their implications for the recovery of the Acropora spp. population should be quickly assessed to facilitate the implementation of measures to reduce them (Grober-Dunsmore et al., 2006; Hernández-Delgado et al., 2014). Limits on research funding is a main constraint in the developing world, thus future international collaborations will be crucial for the understanding of this “knob-like chimney” phenomenon on Caribbean coral reefs.
Author Contributions
AR-S: conceived and wrote the perspective article. AM-C: provided data analysis and figures in Supplementary Data. MM: provided additional ecological data. AM-C, JA-G, and MM: commented and revised this piece.
Funding
Funding was provided by Consejo Nacional de Ciencia y Tecnología (CONACYT # 666908) and the project “Strengthening the Doctoral program in Marine Science”-“(FOMIX- #000000000247043), CINVESTAV-Mérida, México”.
Conflict of Interest Statement
The authors declare that the research was conducted in the absence of any commercial or financial relationships that could be construed as a potential conflict of interest.
Acknowledgments
We thank two reviewers for their valuable comments that helped improve our manuscript. We acknowledge Nicole Helgason-Reef Divers.io for providing photographs. Thanks to comments from M. Miller, C. Woodley, and A. Banaszak. Special recognition to the financial support from CONACYT Scholarship #666908, LEEAC-CINVESTAV. Special thanks for field support from Antal Borcsok and Alejandra Thompson-Tela Marine Research Station, Ian Drysdale-Healthy Reefs Initiative, and Jennifer Myton-Coral Reef Alliance.
Supplementary Material
The Supplementary Material for this article can be found online at: https://www.frontiersin.org/articles/10.3389/fmars.2018.00041/full#supplementary-material
Footnotes
1. ^IUCN Red List of Threatened Species. Version 2016-3. www.iucnredlist.org. Downloaded on 5 January 2017.
References
Aeby, G. S., Williams, G. J., Franklin, E. C., Haapkyla, J., Harvell, C. D., Neale, S., et al. (2011). Growth anomalies on the coral genera Acropora and Porites are strongly associated with host density and human population size across the Indo-Pacific. PLoS ONE 6:e16887. doi: 10.1371/journal.pone.0016887
Ahrens, J. B., Borda, E., Barroso, R., Paiva, P. C., Campbell, A. M., Wolf, A., et al. (2013). The curious case of Hermodice carunculata (Annelida: Amphinomidae): evidence for genetic homogeneity throughout the Atlantic Ocean and adjacent basins. Mol. Ecol. 22, 2280–2291. doi: 10.1111/mec.12263
Alvarez-Filip, L., Dulvy, N. K., Gill, J. A., Côté, I. M., and Watkinson, A. R. (2009). Flattening of Caribbean coral reefs: region-wide declines in architectural complexity. Proc. Biol. Sci. 276, 3019–3025. doi: 10.1098/rspb.2009.0339
Anthony, K. R. N. (2006). Enhanced energy status of corals on coastal, high-turbidity reefs. Mar. Ecol. Prog. Ser. 319, 111–116. doi: 10.3354/meps319111
Anthony, K. R., and Fabricius, K. E. (2000). Shifting roles of heterotrophy and autotrophy in coral energetics under varying turbidity. J. Exp. Mar. Biol. Ecol. 252, 221–253. doi: 10.1016/S0022-0981(00)00237-9
Aronson, R., Bruckner, A., Moore, J., Precht, B., and Weil, E. (2008). Acropora palmata. The IUCN Red List of Threatened Species. e.T133006A3536699. doi: 10.2305/IUCN.UK.2008.RLTS.T133006A3536699.en
Aronson, R., and Precht, W. (2001). White-band disease and the changing face of Caribbean coral reefs. Hydrobiologia 460, 25–38. doi: 10.1023/A:1013103928980
Bak, R. P. M. (1983). Neoplasia, regeneration and growth in the reef-building coral Acropora palmata. Mar. Biol. 77, 221–221. doi: 10.1007/BF00395810
Baums, I. B., Devlin-Durante, M. K., and LaJeunesse, T. C. (2014). New insights into the dynamics between reef corals and their associated dinoflagellate endosymbionts from population genetic studies. Mol. Ecol. 23, 4203–4215. doi: 10.1111/mec.12788
Baums, I., Miller, M., and Szmant, A. (2003). Ecology of a corallivorous gastropod, Coralliophila abbreviata, on two scleractinian hosts. II: feeding, respiration and growth. Mar. Bio. 142, 1093–1101. doi: 10.1007/s00227-003-1053-4
Bohnsack, J. A., and Harper, D. E. (1988). Length-Weight Relationships of Selected Marine Reef Fishes from the Southeastern United States and the Caribbean. NOAA Technical Memorandum NMFS-SEFC-215, Southeast Fisheries Center, Miami, FL.
Bongiorni, L., Shafir, S., and Rinkevich, B. (2003). Effects of particulate matter released by a fish farm (Eilat, Red Sea) on survival and growth of Stylophora pistillata coral nubbins. Mar. Poll. Bull. 46, 1120–1124. doi: 10.1016/S0025-326X(03)00240-6
Bright, A. J., Rogers, C. S., Brandt, M. E., Muller, E., and Smith, T. B. (2016). Disease prevalence and snail predation associated with swell-generated damage on the threatened coral, Acropora palmata (Lamarck). Front. Mar. Sci. 3:77. doi: 10.3389/fmars.2016.00077
Bruckner, A., Aronson, R., and Bruckner, R. J. (2002). Endangered acroporid corals of the Caribbean. Coral Reefs 21:41. doi: 10.1007/s00338-001-0209-2
Bruckner, A. W., and Bruckner, R. J. (2015). “Mechanical lesions and corallivory,” in Diseases of Coral, eds C. M. Woodley, C.A. Downs, A.W. Bruckner, J.W. Porter and S.B. Galloway (Hoboken, NJ: John Wiley & Sons Inc.), 242–265.
Bruno, J. F., and Edmunds, P. J. (1997). Clonal variation for phenotypic plasticity in the coral Madracis mirabilis. Ecology 78, 2177–2190. doi: 10.2307/2265954
Casey, J. M., Ainsworth, T. D., Choat, J. H., and Connolly, S. R. (2014). Farming behaviour of reef fishes increases the prevalence of coral disease associated microbes and black band disease. Proc. R. Soc. B. 281:20141032. doi: 10.1098/rspb.2014.1032
Ceccarelli, D. M., Jones, G. P., and McCook, L. J. (2001). Territorial damselfishes as determinants of the structure of benthic communities on coral reefs. Oceanogr. Mar. Biol. Annu. Rev. 39, 355–389. doi: 10.1007/s00338-009-0567-8
Cole, A. J., Pratchett, M. S., and Jones, G. P. (2008). Diversity and functional importance of coral-feeding fishes on tropical coral reefs. Fish Fish. 9, 286–307. doi: 10.1111/j.1467-2979.2008.00290.x
Cróquer, A., Villamizar, E., and Noriega, N. (2002). Environmental factors affecting tissue regeneration of the reef – building coral Montastraea annularis (Faviidae) at Los Roques National Park, Venezuela. Rev. Biol. Trop. 50, 1055–1065.
Erftemeijer, P. L., Riegl, B., Hoeksema, B. W., and Todd, P. A. (2012). Environmental impacts of dredging and other sediment disturbances on corals: a review. Mar. Pollut. Bull. 64, 1737–1765. doi: 10.1016/j.marpolbul.2012.05.008
Ferrier-Pagès, C., Witting, J., Tambutté, É., and Sebens, K. (2003). Effect of natural zooplankton feeding on the tissue and skeletal growth of the scleractinian coral Stylophora pistillata. Coral Reefs 22, 229–240. doi: 10.1007/s00338-003-0312-7
Gignoux-Wolfsohn, S. A., Marks, C. J., and Vollmer, S. V. (2012). White band disease transmission in the threatened coral, Acropora cervicornis. Sci. Rep. 2:804. doi: 10.1038/srep00804
Gladfelter, E. H. (2007). Skeletal development in Acropora palmata (Lamarck 1816): a scanning electron microscope (SEM) comparison demonstrating similar mechanisms of skeletal extension in axial versus encrusting growth. Coral Reefs 26, 883–892. doi: 10.1007/s00338-007-0278-y
Gladfelter, E. H., Monahan, R. K., and Gladfelter, W. B. (1978). Growth rates of five reef-building corals in the northeastern Caribbean. Bull Mar. Sci. 28, 728–734.
Gochfeld, D. J. (2004). Predation-induced morphological and behavioral defenses in a hard coral: implications for foraging behavior of coral-feeding butterflyfishes. Mar. Eco. Prog. Ser. 267, 145–141. doi: 10.3354/meps267145
Green, E. P., and Bruckner, A. W. (2000). The significance of coral disease epizootiology for coral reef conservation. Biol. Cons. 96, 347–361. doi: 10.1016/S0006-3207(00)00073-2
Grober-Dunsmore, R., Bonito, V., and Frazer, T. K. (2006). Potential inhibitors to recovery of Acropora palmata populations in St. John, US Virgin Islands. Mar. Ecol. Prog. Ser. 321, 123–132. doi: 10.3354/meps321123
Henry, L. A., and Hart, M. (2005). Regeneration from injury and resource allocation in sponges and corals—a review. Int. Rev. Hydrobiol. 90, 125–158. doi: 10.1002/iroh.200410759
Hernández-Delgado, E. A. (2000). Effects of Anthropogenic Stress Gradients in the Structure of Coral Reef Fish and Epibenthic Communities. Dissertation, University of Puerto Rico, San Juan.
Hernández-Delgado, E. A., Mercado-Molina, A. E., Alejandro-Camis, P. J., Candelas-Sánchez, F., Fonseca-Miranda, J. S., González-Ramos, C. M., et al. (2014). Community-based coral reef rehabilitation in a changing climate: lessons learned from hurricanes, extreme rainfall, and changing land use impacts. Open J. Ecol. 4, 918–944. doi: 10.4236/oje.2014.414077
Jackson, J. B. C., Donovan, M., Cramer, K., and Lam, V. (eds.). (2014). Status and Trends of Caribbean Coral Reefs 1970–2012. Washington, DC: Global Coral Reef Monitoring Network, International Union for the Conservation of Nature Global Marine and Polar Program. Available online at: http://cmsdata.iucn.org/downloads/executive_summary_caribbean_status_report_eng.pdf
Jordan-Dahlgren, E. (1992). Recolonization patterns of Acropora palmata in a marginal environment. Bull. Mar. Sci. 51, 104–117.
Kaufman, L. (1981). There was biological disturbance on Pleistocene coral reefs. Paleobiology 7, 527–532.
Klaus, J. S., Budd, A. F., Heikoop, J. M., and Fouke, B. W. (2007). Environmental controls on corallite morphology in the reef coral Montastrea annularis. Bull. Mar. Sci. 80, 233–260.
Kramer, P., McField, M., Álvarez-Filip, L., Drysdale, I., Flores, M. R., Giro, A., et al. (2015). Report card for the Mesoamerican Reef. Healthy Reefs Initiative. Available online at: www.healthyreefs.org
Lester, R. T., and Bak, R. P. M. (1985). Effects of environment on regeneration rate of tissue lesions in the reef coral Montastrea annularis (Scleractinia). Mar. Ecol. Prog. Ser. 24, 183–185.
Lirman, D. (1999). Reef fish communities associated with Acropora palmata: relationships to benthic attributes. Bull. Mar. Sci. 65, 235–252.
Lirman, D. (2000a). Lesion regeneration in the branching coral Acropora palmata: effects of colonization, colony size, lesion size, and lesion shape. Mar. Ecol. Prog. Ser. 197, 209–215. doi: 10.3354/meps197209
Lirman, D. (2000b). Fragmentation in the branching coral Acropora palmata (Lamarck): growth, survivorship, and reproduction of colonies and fragments. J. Exp. Mar. Biol. Ecol. 251, 41–57. doi: 10.1016/S0022-0981(00)00205-7
Meesters, E. H., and Bak, R. P. M. (1995). Age-related deterioration of a physiological function in the branching coral Acropora palmata. Mar. Ecol. Prog. Ser. 121, 203–209.
Meesters, E. H., Wesseling, I., and Bak, R. P. M. (1996). Partial mortality in three species o reef-building corals and the relation with colony morphology. Bull. Mar. Sci. 58, 838–852.
Miller, M. W. (2001). Corallivorous snail removal: evaluation of impact on Acropora palmata. Coral Reefs 19, 293–295. doi: 10.1007/PL00006963
Miller, M. W., Marmet, C., Cameron, C., and Williams, D. (2014). Prevalence, consequences, and mitigation of fireworm predation on endangered staghorn coral. Mar. Ecol. Prog. Ser. 516, 187–194. doi: 10.3354/meps10996
Miller, M. W., and Williams, D. E. (2007). Coral disease outbreak at Navassa, a remote Caribbean island. Coral Reefs 26. 97–101. doi: 10.1007/s00338-006-0165-y
Miller, M. W., Williams, D. E., and Fish, J. (2016). Genet-specific spawning patterns in Acropora palmata. Coral Reefs 35, 1393–1398. doi: 10.1007/s00338-016-1472-6
Mosses, E. F., and Hallock, P. (2015). “Coral regeneration assay,” in Diseases of Coral, eds C. M. Woodley, C. A. Downs, A. W. Bruckner, J. W. Porter and S. B. Galloway, (Hoboken, NJ: John Wiley & Sons Inc.), 473–482.
Ott, B., and Lewis, J. B. (1972). Importance of gastropod Coralliophila abbreviata (Lamarck) and polychaete Hermodice carunculata (Pallas) as Coral Reef Predators. Can. J. Zool. 50, 1651–1656.
Peters, E. (1984). A survey of cellular reactions to environmental stress and disease in Caribbean scleractinian corals. Helgolander Meeresunters 37, 113–137.
Peters, E. C., Halas, J. C., and McCarty, H. B. (1986). Calicoblastic neoplasms in Acropora palmata, with a review of reports on anomalies of growth and form in corals. J. Natl. Cancer Inst. 76, 895–912. doi: 10.1093/jnci/76.5.895
Pollock, F. J., Morris, P. J., Willis, B. L., and Bourne, D. G. (2011). The urgent need for robust coral disease diagnostics. PLoS Pathog. 7:e1002183. doi: 10.1371/journal.ppat.1002183
Precht, W. F., Aronson, R. B., Moody, R. M., and Kaufman, L. (2010). Changing patterns of microhabitat utilization by the threespot damselfish, Stegastes planifrons, on Caribbean reefs. PLoS ONE 5:e10835. doi: 10.1371/journal.pone.0010835
Randall, J. E. (1967). Food Habits of Reef Fishes of the West Indies. Dissertation, University of Miami, Miami, FL.
Robertson, D. R. (1996). Interspecific competition controls abundance and habitat use of territorial Caribbean damselfishes. Ecology 77, 885–899. doi: 10.2307/2265509
Rodríguez-Martínez, R. E., Banaszak, A. T., McField, M. D., Beltrán-Torres, A. U., and Álvarez-Filip, L. (2014). Assessment of Acropora palmata in the Mesoamerican Reef System. PLoS ONE 9:4. doi: 10.1371/journal.pone.0096140
Rotjan, R. D., and Lewis, S. M. (2008). Impact of coral predators on tropical reefs. Mar. Ecol. Prog. Ser. 367, 73–91. doi: 10.3354/meps07531
Sabine, A. M., Smith, T. B., Williams, D. E., and Brandt, M. E. (2015). Environmental conditions influence tissue regeneration rates in scleractinian corals. Mar. Pollut. Bull. 95, 253–264. doi: 10.1016/j.marpolbul.2015.04.006
Sammarco, P. W., Carleton, J., and Risk, M. J. (1986). Effects of grazing and damselfish territoriality on bioerosion of dead corals: direct effects. J. Exp. Mar. Biol. Ecol. 98, 1–19.
Schopmeyer, S. A., and Lirman, D. (2015). Occupation dynamics and impacts of damselfish territoriality on recovering populations of the threatened staghorn coral, Acropora cervicornis. PLoS ONE 10:e0141302. doi: 10.1371/journal.pone.0141302
Schulze, A., Grimes, C. J., and Rudek, T. E. (2017). Tough, armed and omnivorous: Hermodice carunculata (Annelida: Amphinomidae) is prepared for ecological challenges. J. Mar. Biol. Assoc. 97, 1075–1080. doi: 10.1017/S0025315417000091
Schutte, V. G. W., Selig, E. R., and Bruno, J. F. (2010). Regional spatio-temporal trends in Caribbean coral reef benthic communities. Mar. Ecol. Prog. Ser. 402, 115–122. doi: 10.3354/meps08438
Shantz, A. A. (2016). Novel enemies – previously unknown predators of the bearded fireworm. Front. Ecol. Environ. 14:6. doi: 10.1002/fee.1305
Shantz, A. A., Ladd, M. C., Shrack, E., and Burkepile, D. E. (2015). Fish-derived nutrient hotspots shape coral reef benthic communities. Ecol. Appl. 25, 2142–2152. doi: 10.1890/14-2209.1
Shaver, E. C., Shantz, A. A., McMinds, R., Burkepile, D. E., Vega-Thurber, R. L., and Silliman, B. R. (2017). Effects of predation and nutrient enrichment on the success and microbiome of a foundational coral. Ecology. 98, 830–839. doi: 10.1002/ecy.1709
Sussman, M., Loya, Y., Fine, M., and Rosenberg, E. (2003). The marine fireworm Hermodice carunculata is a winter reservoir and spring–summer vector for the coral-bleaching pathogen Vibrio shiloi. Environ. Microbiol. 5, 250–255. doi: 10.1046/j.1462-2920.2003.00424.x
Sutherland, K. P., Shaban, S., Joyner, J. L., Porter, J. W., and Lipp, E. K. (2011). Human pathogen shown to cause disease in the threatened eklhorn coral Acropora palmata. PLoS ONE 6:e23468. doi: 10.1371/journal.pone.0023468
Tambutté, E., Venn, A. A., Holcomb, M., Segonds, N., Techer, N., Zoccola, D., et al. (2015). Morphological plasticity of the coral skeleton under CO2-driven seawater acidification. Nat. Commun. 6:7368. doi: 10.1038/ncomms8368
Tambutté, S., Holcomb, M., Ferrier-Pagès, C., Reynaud, S., Tambutté, E., Zoccola, D., et al. (2011). Coral biomineralization: from the gene to the environment. J. Exp. Mar. Biol. Ecol. 408. 58–78. doi: 10.1016/j.jembe.2011.07.026
Todd, P. A. (2008). Morphological plasticity in scleractinian corals. Biol. Rev. Camb. Philos. Soc. 83, 315–337. doi: 10.1111/j.1469-185X.2008.00045.x
Todd, P. A., Sidle, R. C., and Lewin-Koh, N. J. I. (2004). An aquarium experiment for identifying the physical factors inducing morphological change in two massive scleractinian corals. J. Exp. Mar. Biol. Ecol. 299. 97–113. doi: 10.1016/j.jembe.2003.09.005
Tomiak, P. J., Andersen, M. B., Hendy, E., Potter, E.-K., Johnson, K. G., and Penkman, K. E. H. (2016). The role of skeletal micro-architecture in diagenesis and dating of Acropora palmata. Geochim. Cosmochim. Acta 183, 153–175. doi: 10.1016/j.gca.2016.03.030
Tribollet, A. (2008). “The boring microflora in modern coral reef ecosystems: a review of its roles” in Current Developments in Bioerosion, eds M. Wisshak, L. Tapanila (Berlin;Heidelberg: Erlangen Earth Conference Series; Springer-Verlag), 67–94.
Vermeij, M. J. A., DeBey, H., Grimsditch, G., Brown, J., Obura, D., DeLeon, R., et al. (2015). Negative effects of gardening damselfish Stegastes planifrons on coral health depend on predator abundance. Mar. Ecol. Prog. Ser. 528, 289–296. doi: 10.3354/meps11243
Via, S., Gomulkiewicz, R., Jong, G., de Scheiner, S. M., Schlichting, C. D., and van Tienderen, P. H. (1995). Adaptive phenotypic plasticity: consensus and controversy Trends Ecol. Evol. 10, 212–217. doi: 10.1016/S0169-5347(00)89061-8
Vollmer, S. V., and Palumbi, S. R. (2002). Hybridization and the evolution of reef coral diversity. Science 296, 2023–2025. doi: 10.1126/science.1069524
Weil, E. (2004). “Coral reef diseases in the Wider Caribbean,” in Coral Health and Disease, eds E. Rosenbergan and Y. Loya (Berlin; Heidelberg: Springer), 35–68.
Weil, E., Smith, G., and Gil-Agudelo, D. L. (2006). Status and progress in coral reef disease research. Dis. Aquat. Org. 69, 1–7. doi: 10.3354/dao069001
Wielgus, J., Glassom, D., Ben–Shaprut, O., and Chadwick–Furman, N. E. (2002). An aberrant growth form of Red Sea corals caused by polychaete infestations. Coral Reefs 21, 315–316. doi: 10.1007/s00338-002-0246-5
Williams, D. E., and Miller, M. W. (2005). Coral disease outbreak: pattern, prevalence and transmission in Acropora cervicornis. Mar. Ecol. Prog. Ser. 301, 119–128. doi: 10.3354/meps301119
Williams, D. E., Miller, M. W., Bright, A. J., Pausch, R. E., and Valdivia, A. (2017). Thermal stress exposure, bleaching response, and mortality in the threatened coral Acropora palmata. Mar. Poll. Bull. 124, 189–197. doi: 10.1016/j.marpolbul.2017.07.001
Witman, J. D. (1988). Effects of predation by the fireworm Hermodice carunculata on Milleporid hydrocorals. Bull. Mar. Sci. 42. 446–458.
Wolf, A. T., Nugues, M. M., and Wild, C. (2014). Distribution, food preference, and trophic position of the corallivorous fireworm Hermodice carunculata in a Caribbean coral reef. Coral Reefs 33. 1153–1163. doi: 10.1007/s00338-014-1184-8
Work, T. M., and Aeby, G. S. (2006). Systematically describing gross lesions in corals. Dis. Aquat.Org. 70, 155–160. doi: 10.3354/dao070155
Work, T. M., Aeby, G. S., and Coles, S. L. (2008). Distribution and morphology of growth anomalies in Acropora from the Indo-Pacific. Dis. Aquat. Org. 78. 255–264. doi: 10.3354/dao01881
Zaneveld, J. R., Burkepile, D. E., Shantz, A. A., Pritchard, C. E., McMinds, R., Payet, J. P., et al. (2016). Overfishing and nutrient pollution interact with temperature to disrupt coral reefs down to microbial scales. Nat. Commun. 7:11833. doi: 10.1038/ncomms11833
Keywords: Acropora, lesions, damselfish chimneys, knob-like chimney growth, environmental plasticity, Caribbean
Citation: Rivera-Sosa A, Muñiz-Castillo AI, McField M and Arias-González JE (2018) Unusual “Knob-Like Chimney” Growth Forms on Acropora Species in the Caribbean. Front. Mar. Sci. 5:41. doi: 10.3389/fmars.2018.00041
Received: 31 May 2017; Accepted: 29 January 2018;
Published: 13 February 2018.
Edited by:
Thomas K. Frazer, University of Florida, United StatesReviewed by:
Isabelle Domart-Coulon, National Museum of Natural History, FranceDouglas Fenner, NOAA NMFS, United States
Copyright © 2018 Rivera-Sosa, Muñiz-Castillo, McField and Arias-González. This is an open-access article distributed under the terms of the Creative Commons Attribution License (CC BY). The use, distribution or reproduction in other forums is permitted, provided the original author(s) and the copyright owner are credited and that the original publication in this journal is cited, in accordance with accepted academic practice. No use, distribution or reproduction is permitted which does not comply with these terms.
*Correspondence: Andrea Rivera-Sosa, YW5kcmVhLnJpdmVyYUBjaW52ZXN0YXYubXg=
Jesús Ernesto Arias-González, ZWFyaWFzQGNpbnZlc3Rhdi5teA==