- 1Laboratory of Microbiology, Institute of Biology, Federal University of Rio de Janeiro, Rio de Janeiro, Brazil
- 2Instituto de Química de São Carlos, Universidade de São Paulo, São Carlos, Brazil
- 3Center of Technology - CT2, SAGE-COPPE, Federal University of Rio de Janeiro, Rio de Janeiro, Brazil
Sponge holobionts are defined as the host animals and their associated microbiomes. Both host and microbiome produce extracellular products that facilitate interaction within the holobiont. For example, microbes may provide nutrition for the animal host and protection against pathogens. The genomic study of bacterial cultures may shed light on the properties of novel symbiotic bacteria isolated from marine holobionts. In this study, we performed a genome-based analysis of Pseudovibrio brasiliensis Ab134T isolated from the sponge Arenosclera brasiliensis. This novel strain is phylogenetically related to Pseudovibrio denitrificans. In silico DNA-DNA hybridization and calculation of the average amino acid identity between the strain Ab134T and P. denitrificans JCM 12308T showed <70% similarity and <95% identity, respectively. This novel bacterial species possesses genomic features that hint at several possible roles in symbiosis (e.g., production of secondary metabolites, including bromotyrosine-derived alkaloids) in sponge and coral holobionts. We also detected gene clusters encoding type III, type IV, and type VI secretion systems and 26 toxin-like proteins, including probable paralogs. Our results demonstrate the genome versatility of P. brasiliensis Ab134T and the potential to attach to host cells, which may play a role in its symbiotic lifestyle.
Introduction
The genus Pseudovibrio belongs to the order Rhodobacterales of the class Alphaproteobacteria. It comprises six species: Pseudovibrio denitrificans (Shieh et al., 2004), Pseudovibrio ascidiaceicola (Fukunaga et al., 2006), Pseudovibrio japonicus (Hosoya and Yokota, 2007), Pseudovibrio axinellae (O'Halloran et al., 2013), Pseudovibrio hongkongensis (Xu et al., 2015), and Pseudovibrio stylochi (Zhang et al., 2016). Pseudovibrio species have been reported worldwide and are found mainly as members of bacterial communities associated with marine invertebrate holobionts, including tunicates (Sertan-De Guzman et al., 2007), flatworms (Xu et al., 2015), corals (Essack, 2001; Bondarev et al., 2013), sea squirts (Fukunaga et al., 2006), algae (Vizcaino, 2011), and a wide variety of sponges (Breitbart et al., 2003; Muscholl-Silberhorn et al., 2008; O'Halloran et al., 2013; Appolinario et al., 2016). They are also found as free-living bacteria in seawater (Shieh et al., 2004; Hosoya and Yokota, 2007). Pseudovibrio species are heterotrophic, facultative anaerobic, marine bacteria capable of denitrifying and fermenting a range of substrates (Romano et al., 2016).
Pseudovibrio species were reported as dominant in the culturable bacterial fraction of various marine sponges (Webster and Hill, 2001; Muscholl-Silberhorn et al., 2008; Bauvais et al., 2015). A symbiotic relationship appears to exist between Pseudovibrio and sponges (Taylor et al., 2007); however, it remains unclear whether these bacteria are sponge mutualists/commensalists or pathogens/parasites. Pseudovibrio has been isolated only from healthy sponges and other holobionts, except for a single study reporting the association of Pseudovibrio with diseased (bleached) scleractinian corals (Moreira et al., 2014). The abundance of Pseudovibrio strains decreases drastically in diseased sponges (Sweet et al., 2015). Some strains of Pseudovibrio may protect their hosts by inhibiting the growth of the sponge pathogen Bacillus (Webster and Hill, 2001; Esteves et al., 2017). Some strains of Pseudovibrio produce biologically active secondary metabolites with antimicrobial activity (Sertan-De Guzman et al., 2007; Penesyan et al., 2011; Vizcaino, 2011; Nicacio et al., 2017). Genomic analysis of ten Pseudovibrio strains isolated from marine sponges collected on the west coast of Ireland revealed a diverse repertoire of genes involved in prokaryote-eukaryote interactions, including potential toxin-immunity systems and secretion systems (Romano et al., 2016). However, it remains unclear whether these findings apply to other sponge holobionts or other locations.
Rua et al. (2014) analyzed the diversity and antimicrobial potential of culturable heterotrophic bacteria associated with the endemic sponge A. brasiliensis and isolated Pseudovibrio sp. Ab134T. Bromotyrosine-derived alkaloids were recently reported from cultures of this strain (Nicacio et al., 2017). Fistularin-3, one of such metabolites induces apoptosis (Mijares et al., 2013) and exerts antimycobacterial activity against Mycobacterium tuberculosis H37Rv and low cytotoxicity against macrophages (De Oliveira et al., 2006).
In the present study, we compared the genomic attributes of Pseudovibrio sp. Ab134T with those of related Pseudovibrio species to identify genes with biotechnological potential and involved in symbiosis. Our results provide further evidence of Pseudovibrio spp. as members of the stable microbiome of sponge hosts. Furthermore, their usefulness as source of bioactives is highlighted with genomic and experimental evidence, which is advantageous since not all bacterial producers of such chemicals can be easily isolated, cultivated, and yields metabolites in laboratory conditions. We then classified this novel strain Ab134T using a genome-based taxonomic analysis (Thompson et al., 2015), improving the present delineation of this group encompassing metabolically active members yet not identified to species level.
Materials and Methods
Isolation of Pseudovibrio Strain
The Pseudovibrio strain was isolated as previously described (Rua et al., 2014). Pseudovibrio sp. Ab134T has been deposited in the Collection of Environmental and Health (CBAS) at the Oswaldo Cruz Institute (IOC), FIOCRUZ (Rio de Janeiro, Brazil) (http://cbas.fiocruz.br/) and assigned the accession number CBAS 623T. The strain was also deposited in the Collection of Aquatic Microorganisms (CAIM) in Mazátlan, Sinaloa, Mexico (http://www.ciad.mx/caim/CAIM.html) and assigned the accession number CAIM 1924T.
Genome Sequencing, Assembly, and Annotation
Genomic DNA was extracted using a previously described method (Pitcher et al., 1989). DNA libraries were built using the Nextera DNA Sample Preparation Kit (Illumina, San Diego, CA, USA). The DNA was purified using AMPure XP beads and quantified using the fluorometric Qubit dsDNA HS Assay Kit (Life Technologies). Quantification of libraries was performed with the 7500 Real-Time PCR System (Applied Biosystems, Foster City, CA, USA) and the KAPA Library Quantification Kit (Kapa Biosystems, Wilmington, MA, USA). Library size distribution was determined using the Agilent 2100 Bioanalyzer. Genome sequencing was performed using the Illumina MiSeq platform (paired-end sequencing, 2 × 300 base pairs). The sequences obtained were preprocessed using PrinSeq software to remove small reads (<35 bp) and low-score sequences (Phred score <30) (Schmieder and Edwards, 2011). Two programs assembled high-quality reads: A5-miseq (Coil et al., 2015) assembled the sequence data, and then the generated contigs and the singletons were used as input for the CAP3 sequence assembly program (Huang and Madan, 1999). Functional annotation was carried out by the Rapid Annotations using Subsystem Technology (RAST) platform (Aziz et al., 2008).
Phylogenetic Analysis Based on 16S rRNA and recA Genes
The 16S rRNA gene of strain Ab134T was obtained as previously described (Moreira et al., 2014; Rua et al., 2014) and compared to known sequences in the NCBI GenBank database using the Basic Local Alignment Search Tool (BLAST). The closest matches were included in the phylogenetic analysis. The 16S rRNA gene identities were calculated using Jalview V.2 (Waterhouse et al., 2009). Pairwise and multiple alignments were performed using ClustalW (Larkin et al., 2007). Evolutionary history was inferred using the neighbor-joining method (Saitou and Nei, 1987). The bootstrap consensus tree inferred from 1,000 replicates was taken to represent the evolutionary history of the taxa analyzed (Felsenstein, 1985). Evolutionary distances were computed using the p-distance method (Nei and Kumar, 2000), and evolutionary analyses were conducted in MEGA6 (Tamura et al., 2013).
The recA gene sequences for Pseudovibrio genomes available on GenBank (P. denitrificans JCM 12308T, P. sp. FO-BEG1, P. axinellae Ad2T, and P. hongkongensis UST20140214-015BT) were analyzed using the BLASTN algorithm (Altschul et al., 1990). Multiple alignment, phylogenetic reconstruction, and bootstrap consensus were conducted as described above for the 16S rRNA genes (Felsenstein, 1985; Saitou and Nei, 1987; Larkin et al., 2007; Tamura et al., 2013). Accession numbers are listed in Table S1.
Microbial Genomic Taxonomy
In silico DNA-DNA hybridization analysis was carried out by genome-to-genome comparison (Auch et al., 2010). Average nucleotide identity (ANI) was calculated as previously described (Thompson et al., 2013). The intraspecies genomic relatedness ranged from 95 to 100% ANI. Genome distance was calculated using a genome-to-genome distance (GGD) calculator (Meier-kolthoff et al., 2016), with intraspecies genomic similarity ranging from 70 to 100%.
Genome-Based Phenotype
Phenotype analysis was based on genome sequences (Amaral et al., 2014), focusing on eight biochemical characteristics that have been used to identify Pseudovibrio species (Shieh et al., 2004; Hosoya and Yokota, 2007). For each characteristic, we searched for the corresponding genes. If one or more genes involved in a phenotype was present in the genome, the organism was considered positive for this phenotype. Genes encoding proteins involved in those characteristics were detected by using the RAST program (Overbeek et al., 2014). Genes associated with related biochemical pathways were identified with the BLASTP algorithm (Altschul et al., 1990). We used the antiSMASH 2.0 software pipeline (Weber et al., 2015) to identify secondary metabolite biosynthesis clusters in the whole genome sequences of P. brasiliensis Ab134T and P. denitrificans JCM 12308T. The metabolic features of P. denitrificans JCM 12308T and the Ab134T strain were compared using the RAST platform (Overbeek et al., 2014).
Search for Homologous Genes Related to Symbiosis
In order to identify genes related to symbiosis in P. brasiliensis Ab134T, secretion system types III, IV, and VI and toxin-like proteins were predicted using two different approaches. The search for secretion systems was carried out using T346Hunter, a web-based tool for the prediction of type III, type IV, and type VI secretion systems (Martínez-García et al., 2015). Detection of toxin-like proteins was carried out using BLASTP and the Virulence Factors Database (VFDB; Chen et al., 2016). For the BLASTP analysis, amino acid sequences from P. brasiliensis Ab134T were predicted using Prodigal software v2.6.2 (Hyatt et al., 2010) with default parameters and used as query sequences. Results annotated as “toxin” were used in the subsequent analysis. Genes associated with the secretion systems of P. brasiliensis Ab134T and P. denitrificans JCM 12308T were detected out using the same approach.
In Vitro Phenotypic Characterization
Growth of Pseudovibrio sp. Ab134T at different NaCl concentrations (0% and 5.0% [w/v]), temperatures (4, 10, and 13–44°C), and pH values (5–10, adjusted with NaOH or HCl) were tested on marine agar medium. Plates were incubated at 27°C (or the test temperature) for up to 7 days in triplicate. Motility was evaluated on semisolid marine agar and stab inoculation into tubes and confirmed by phase contrast microscopy, which was also used to evaluate Gram staining, shape, and diameter. Features that differed between Ab134T and the reference strains of the three Pseudovibrio species are listed in Table S2.
Antimicrobial Activity
Strains and Growth Conditions
The following three indicator strains were selected for the antimicrobial production assay: Bacillus subtilis CECT 461T (Rua et al., 2014), the coral pathogen Vibrio coralliilyticus P1, and the human pathogen Vibrio parahaemolyticus. V. coralliilyticus P1 produces proteases, including a zinc-containing metalloprotease (Santos Ede et al., 2011). V. parahaemolyticus was PCR-positive for the presence of hemolysin genes tlh and trh (data not shown). The indicator strains were activated in marine/LB agar (1:0.5) at 30°C overnight, and the test strain, P. brasiliensis Ab134T, was activated in marine agar at 30°C overnight.
Antimicrobial Production Assay
P. brasiliensis Ab134T was spotted onto marine agar; the spots were ~1 cm in diameter. After 72 h, the cells were killed by exposure to chloroform vapor for 1–2 h, as follows. A piece of cotton was saturated with 1 mL chloroform and placed on the plate lid. The plate containing agar and cells was then inverted, placed on the lid, and incubated for 1–2 h. Indicator strains were inoculated in marine/LB soft agar, which was poured onto the plates with the dead cells (test strain). After incubation at 30°C for 24 h, clear zones around the spots of dead cells indicated that the test strain had produced an antimicrobial compound. This assay was performed in triplicate.
Results
General Characteristics and Metabolic Features Predicted in the P. brasiliensis Ab134T Genome
A total of 1,967,588 paired-ends reads for the Ab134Tstrain were generated. The genome assembly resulted in 39 contigs, and the coverage was 164-fold. Estimated genome size was 5,975,631 bp, and the number of coding sequences estimated by Prodigal was 5476. Of the 66 RNA sequences, 61 were tRNAs, and 5 were rRNAs. Functional annotation revealed that most of the 2,260 genes identified were assigned to carbohydrates (301), amino acid and derivatives (281), and cofactors, vitamins, prosthetic groups, and pigments (234) (Figure S1).
Our results showed that P. brasiliensis Ab134T is phylogenetically and genetically related to P. denitrificans (Figure S2 and Table S3). A functional comparison between the P. denitrificans JCM 12308T and P. brasiliensis Ab134Tgenomes revealed 51 genes unique to P. denitrificans JCM 12308T, including those related to urea decomposition, acetyl-CoA conversion to butyrate, menaquinone and phylloquinone biosynthesis, curli production, dihydroxyacetone kinases, and the G3E family of P-loop GTPases (metallocenter biosynthesis, urease accessory). The 34 genes unique to the P. brasiliensis Ab134T genome included those related to phages and prophages, maltose and maltodextrin utilization, transport of nickel and cobalt, toxin-antitoxin replicon stabilization systems, and thioredoxin-disulfide reductase (Table S4). Genes shared by P. brasiliensis Ab134Tand P. denitrificans JCM 12308T included those related to nitrogen metabolism (dissimilatory nitrite reductase, nitrate and nitrite ammonification) (Table S5) and fermentation processes (butanol biosynthesis, mixed acid, lactate, acetolactate synthase subunits, and acetyl-CoA fermentation to butyrate) (Table S6), suggesting the potential of P. brasiliensis Ab134T to carry out denitrification and fermentation in the sponge holobiont.
Secondary Metabolite Biosynthesis Clusters Analysis
Using the antiSMASH program we identified clusters related to secondary metabolite biosynthesis (Figures 1,2 and Figures S3,S4) and found differences in gene cluster abundance and diversity between P. denitrificans JCM 12308T and P. brasiliensis Ab134T. The nine clusters identified in P. denitrificans JCM 12308T are involved in the biosynthesis of bacteriocins (2), terpene (1), non-ribosomal peptide synthases (NRPSs) (2), hybrid NRPS-polyketide synthase (PKS)-T1 (1), hybrid PKS T1-bacteriocin (1), homoserine-lactone (1), and T3PKS-T1PKS (1). The five clusters identified in P. brasiliensis Ab134T were assigned to the biosynthesis of terpene (1), bacteriocins (2), PKS (1), and NRPS (1). Some gene clusters showed no similarity to known Pseudovibrio gene clusters.
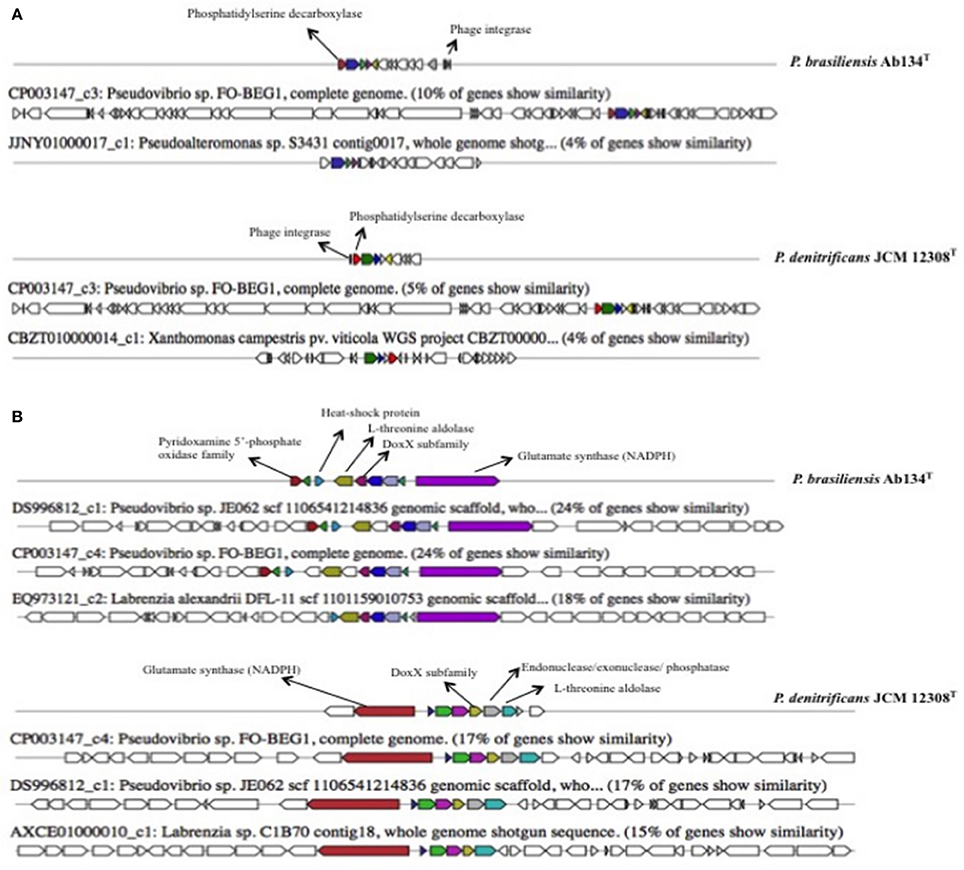
Figure 1. Bacteriocin biosynthesis pathway (cluster 2) (A) and novel bacteriocin pathway (cluster 3) (B) identified in the P. brasiliensis Ab134T genome.
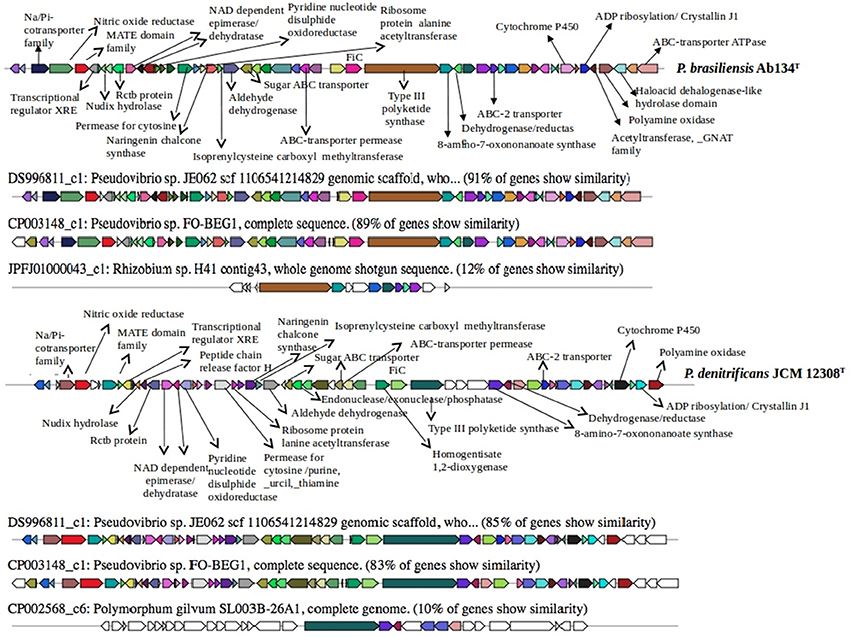
Figure 2. PKS3-PKS1 pathway cluster identified in the P. brasiliensis Ab134T genome and in the P. denitrificans JCM 12308T genome.
Terpene Gene Cluster
Cluster 1 (20.9 kb) contained 20 genes predicted to be involved in terpene production. This cluster showed a 54% sequence similarity to genes in Pseudovibrio sp. FO-BEG1 and 51% sequence similarity to genes in Pseudovibrio sp. JE062 (Figure S3). Both strains (FO-BEG1 and JE062) were genetically characterized as P. denitrificans (Bondarev et al., 2013; Romano et al., 2016).
Bacteriocin Gene Cluster
Clusters 2 and 3 were related to bacteriocin production. Cluster 2 (19.3 kb) shared only 10% sequence similarity to FO-BEG1 genes, and cluster 3 (11 kb) shared 24% sequence similarity to JE062 and FO-BEG1 genes suggesting that they represent two new gene clusters (Figure 1).
Polyketide Synthase and Non-ribosomal Peptide Synthetase Gene Clusters
In the P. brasiliensis Ab134T genome we identified a 7.2-kb cluster containing 41 genes predicted to be involved in NRPS production. This cluster shared only 18% sequence similarity to genes in Pseudomonas sp. GM25 PM124 (Figure S4). The best characterized cluster detected was a hybrid PKS3-PKS1 (Figure 2). Cluster 5 (63.5 kb) contained 120 genes predicted to be involved in the production of this hybrid PKS3-PKS1, and shared 91 and 89% sequence similarity to genes in JE062 and FO-BEG1, respectively (Figure 2). The presence of PKS and NRPS genes is often associated with the production of bioactive secondary metabolites.
Genome analysis of strain Ab134T revealed novel features that allow it to thrive in the sponge holobiont. Using HMMER3 (Eddy, 2009) we identified four types of enzymes that may be related to bromotyrosines' biosynthesis, including bromoperoxidases, S-adenosyl-L-methionine-dependent methyltransferases and ATP-grasp ligases such as glutathione synthetase and friulimicin.
Secretion Systems and Prokaryote-Eukaryote Interaction
We identified four gene clusters encoding non-flagellar type III secretion systems (T3SSs) in the P. brasiliensis Ab134T genome (Figure S5). All essential genes were present in three of these clusters. Genes in the four clusters showed high similarity to those from P. denitrificans JCM12308T (Figure S5). The main difference was the presence of three genes from other secretion systems. T3SS cluster 1 (T3SS-1) contained the gene tfc4 (155 amino acids), which is characteristic of T4SS. T3SS cluster 3 (T3SS-3) and cluster 4 (T3SS-4) contained vasH genes (854 and 421 amino acids, respectively), which are characteristic of T6SS. We also identified one cluster that appears to encode 12 proteins of a T4SS, containing mainly virB genes and trb (Figure S6).
We identified two clusters that encode T6SS genes, both including vgrG and hcp genes (Figure S7A). The distribution of effector protein-coding genes was similar to that of the two T6SS clusters identified in the P. denitrificans JCM 12304T genome (Figure S7B), but the number and arrangement of genes differed. Both Ab134T and P. denitrificans JCM 12304T genomes contained 16 core component genes in the first cluster (T6SS-1). Whereas in the second cluster (T6SS-2), Ab134T and P. denitrificans JCM 12304T genomes showed five and 19 core component genes, respectively (Figures S7A,B). Taken together, these results suggest specific interactions with eukaryotes and the potential ability to target host cell machinery.
Potential Toxin-Like Protein-Coding Genes in P. brasiliensis Ab134T Genome
A wide range of potential toxin-like protein-coding genes was identified in the P. brasiliensis Ab134T genome. Of the 25 hits retrieved from a BLASTP search against the VFDB, 15 genes were unique. The genes argK, frpC, rtxA, and syrE were present in multiple copies, suggesting the presence of paralogs (Table S7).
Phylogenetic and Genomic Delineation of Pseudovibrio brasiliensis sp. nov.
Ab134T clustered tightly with other Pseudovibrio spp. based on the 16S rRNA gene sequences analysis (Figure S2A), showing identity values to P. denitrificans strains of 99.4% (JE062, NW001) and 99.5% (JCM12308T, FO-BEG1, MBIC3368). Strains JE062 and FO-BEG1 shared almost identical 16S rDNA gene sequences (99.9%) with P. denitrificans DN34T. Based on the phylogenetic analysis of recA, Ab134T shared only 93% sequence identity with P. denitrificans JCM 12308T and FO-BEG1 (Figure S2B), suggesting that Ab134T represents a new species of the genus Pseudovibrio. A formal description is provided in the Supplementary Material.
Pairwise genomic comparisons between P. brasiliensis Ab134T and P. denitrificans JCM 12038T (Shieh et al., 2004) showed that they share only 93% ANI and 54.3% (±3) GGD similarity (in silico DNA-DNA hybridization) (Table S3). A bacterial species is defined as a group of strains that share ≥98.7% 16S rRNA gene sequence similarity, >95% ANI and >70% GGD similarity (Thompson et al., 2015). Based on phylogenetic analysis, in silico DNA-DNA hybridization, ANI, and differential phenotypic characteristics, strain Ab134T is proposed as the type strain of a novel species, for which the name Pseudovibrio brasiliensis sp. nov. is proposed.
Discussion
We report the genomic characterization of P. brasiliensis Ab134T previously isolated from the marine sponge A. brasiliensis (Rua et al., 2014). The genome size of 5.9 Mb, with a G + C content of 52.1%, is consistent with previously reported values for this genus of ~3.6–6.2 Mb (Romano et al., 2016; Zhang et al., 2016). The secretion systems detected in P. brasiliensis genome represent a vast repertoire to facilitate interaction with its hosts. They are similar to those of the strains of P. denitricans FO-BEG1 and JE062 (Romano et al., 2016). However, P. brasiliensis possesses a distinct phylogenetic position and unique secondary metabolite and toxin-like genes (e.g., bromotyrosine-related genes). These genes may be useful for host-microbe interactions within the sponge, tunicate, and coral holobionts (Alex and Antunes, 2015).
Secondary Metabolites May Be Important For Holobiont Homeostasis
Secondary metabolites and toxins may complement each other to promote holobiont homeostasis. Secondary metabolites (e.g., bacteriocins, terpenes, and NRPS) are encoded by gene clusters, whereas toxins are encoded by a few genes or a single gene (e.g., argK, frpC, rtxA) (Sertan-De Guzman et al., 2007; Penesyan et al., 2011; Vizcaino, 2011; O'Halloran et al., 2013).
Bacteriocins are ribosomally synthesized antimicrobial peptides that are lethal to closely related bacteria. Bacteriocin producers are protected from the effects of these peptides by a specific immunity protein(s) (Cotter et al., 2005). Bacteriocins have been used extensively as preservatives in the food industry (Deegan et al., 2006) and have been identified as potential alternatives to antibiotics (Piper et al., 2009). Bacteriocins may also serve as anti-competitor toxins, enabling a strain or species to invade an established microbiome (Riley and Gordon, 1999; Lenski and Riley, 2002; Riley and Wertz, 2002). In sponge holobionts, bacteriocins protect the host against pathogenic bacteria, and bacteriocin-producing bacteria may prevent the dissemination of pathogens by occupying the same ecological niche (Desriac et al., 2010).
Kennedy et al. (2008) reported that Pseudovibrio cultures from the marine sponge Haliclona simulans contain both putative PKS and NRPS genes, suggesting a potential for secondary metabolite production. These strains exhibited antimicrobial activity against methicillin-resistant Staphylococcus aureus, Bacillus cereus, Escherichia coli, and B. subtilis. P. brasiliensis Ab134T harbored PKS and NRPS gene cluster and exhibited antimicrobial activity against B. subtilis (Rua et al., 2014) and the pathogens V. coralliilyticus P1 and V. parahaemolyticus (this study).
P. brasiliensis Ab134T also produces bromotyrosine-derived alkaloids (Nicacio et al., 2017). Additional enzymes involved in secondary metabolism were detected in this study. Experiments aiming to elucidate the biosynthetic gene clusters responsible for these alkaloids are in progress and will be reported in due time.
Putative Roles Played by Toxins
Toxin genes detected in multiple copies include argK, frpC, rtxA, and syrE. argK encodes an ornithine carbamoyltransferase that confers self-resistance to phaseolotoxin, responsible for halo blight in beans (Phaseolus vulgaris L.), caused by Pseudomonas syringae pv. phaseolicola, (Mosqueda et al., 1990). This gene is known to be horizontally transferred (Sawada et al., 2002) and the product ArgK protects phaseolotoxin producers (self-resistance) in part by providing an alternative Arginine source (Chen et al., 2015). L-arginine is produced by bacterial fermentation and the main applications are in flavor and pharmaceuticals. Arginine overproducing strains has been a research target for the past decades (Lu, 2006). Another gene that was first described as a virulence determinant of P. syringae pv. syringae B301D was present in two copies. syrE is a phytotoxin gene present within the syringomycin cluster. Syringomycin is a cyclic lipodepsinonapeptide (Guenzi et al., 1998). This protein forms pores in plasma membranes, leading to electrolyte leakage (Bender et al., 1999) and might play a role in protecting the host (Table S7).
Multiple copies of frpC and rtxA were also detected. frpC encodes the iron-repressible repeat-in-toxin (RTX) protein FrpC, and rtxA encodes the structural toxin protein RtxA. Both belong to the RTX family, which consists primarily of cytotoxic pore-forming proteins (Schaller et al., 1999; Linhartová et al., 2010) acting as virulence determinants in many gram-negative pathogens. RTX proteins can also play a role in host protection as bacteriocins or by forming protective bacterial surface layers (S-layers) (Linhartová et al., 2010). RTX proteins exhibit additional biological activities as metalloproteases, lipases, pore-forming toxins, iron-regulated proteins, nodulation-related proteins and are involved both in bacterial adherence/motility and host-receptor interactions (Welch, 2001; de Souza Santos et al., 2015). RTX proteins are secreted by a T1SS via Sec-independent pathway used by gram-negative bacteria to transport proteins from the cytoplasm to the extracellular medium in a single step (Chenal et al., 2015). The presence of multiple copies of frpC and rtxA suggests a possible environmental role, including protection of the host and defense against other microorganisms and pathogens. The extracellular matrix of sponges is rich in proteoglycans, lamnin-like subunits, fibronectin, and other structural proteins (Har-el and Tanzer, 1993; Özbek et al., 2010); thus, the multiple copies of RTX proteins may help to penetrate the sponge mesohyl.
Host-Microbe Interactions Mediated by Secretion Systems
A symbiotic relationship suggested between Pseudovibrio and marine invertebrates (Taylor et al., 2007) may involve interactions with holobiont host cells via secretion systems T3SS, T4SS, and T6SS. Secretion systems were first associated with pathogenic strains but have since been widely detected in symbiotic and free-living bacteria (Dale and Moran, 2006; Persson et al., 2009). Bacteria commonly use these three secretion systems to inject effector proteins in target cells, which facilitate colonization (Costa et al., 2015). For example, the non-flagellar T3SS (injectisome) enables gram-negative bacteria to deliver effector proteins into the cytoplasm of eukaryote hosts (Büttner, 2012). T4SS (VirB system), which was first identified in Agrobacterium tumefaciens, delivers toxins into host cells, as well as DNA that integrates into the host genome (Wallden et al., 2010), contributing to the genome plasticity and virulence of the bacteria (Voth et al., 2012).
Two genes (virB and trb) in the T4SS of P. brasiliensis Ab134T are related to conjugation, suggesting a role in genome plasticity and environmental adaptation. These genes have been identified in other Pseudovibrio strains (Romano et al., 2016). Compared to P. denitrificans JCM 12308T, the number of genes in the T4SS cluster was the same, but the gene annotation and rearrangement differed (Figure S6).
T6SSs are thought to help bacteria conquer an ecological niche (Ma et al., 2014; Russell et al., 2014; Kapitein and Mogk, 2017), and niche-specific distribution of T6SS effectors has recently been described (Egan et al., 2015; Romano et al., 2016). T6SSs appear to be involved in biofilm formation (Aschtgen et al., 2008), quorum sensing (Weber et al., 2009), interbacterial interactions (Hood et al., 2017), and anti-pathogenesis (Chow and Mazmanian, 2010; Jani and Cotter, 2017). The T6SS gene impI was detected only in the P. brasiliensis Ab134T genome, which contained all core components of the T6SS, as described by Boyer et al. (2009).
Conclusion
P. brasiliensis Ab134T displays bioactive secondary metabolite genes which might encode the antimicrobial(s) and bioactives already detected experimentally (this study; Rua et al., 2014; Nicacio et al., 2017). These features might prevent host colonization by pathogens and opportunistic organisms. The metabolic versatility of the species is demonstrated by several transporter systems characterized in its genome. Characterization of the genomic repertoire of P. brasiliensis shed light over putative mechanisms of host-microbe and microbe-microbes interactions within the sponge holobiont.
Data Deposition
This whole-genome shotgun sequencing project has been deposited at DDBJ/ENA/GenBank under the accession number MIEL00000000. The version described in this paper is version MIEL01000000.
Author Contributions
FT and CT: designed and planned the study; AF and TF: performed the bioinformatics analyses; AM: performed 16S rRNA phylogeny and designed the antimicrobial production assay; CS: performed tests. All authors contributed to the acquisition, analysis, and interpretation of the data. All authors contributed to the writing of the manuscript. All authors approved the final version of the manuscript.
Conflict of Interest Statement
The authors declare that the research was conducted in the absence of any commercial or financial relationships that could be construed as a potential conflict of interest.
Acknowledgments
The authors thank FAPERJ, FAPESP (2013/50228-8), CAPES, and CNPq for the financial support.
Supplementary Material
The Supplementary Material for this article can be found online at: https://www.frontiersin.org/articles/10.3389/fmars.2018.00081/full#supplementary-material
Figure S1. General overview of the functional annotation of the P. brasiliensis Ab134T genome.
Figure S2. Neighbor-joining tree showing the phylogenetic position of P. brasiliensis Ab134T based on 16S rRNA gene sequences (A) and recA gene sequences (B). The bootstrap consensus tree inferred from 1,000 replicates is shown. The percentage of replicate trees in which the associated taxa clustered together in the bootstrap test is shown next to the branches (>50%). Bar represents 0.005 nucleotide substitution rate (Knuc-values).
Figure S3. Terpene metabolism gene cluster identified in the P. brasiliensis Ab134T genome.
Figure S4. NRPS pathway cluster identified in the P. brasiliensis Ab134T genome.
Figure S5. Representative genetic organization of type III secretion system (T3SS) in the P. brasiliensis Ab134T genome (A) and P. denitrificans JCM 12308T genome (B).
Figure S6. Representative genetic organization of type IV secretion system (T4SS) of P. brasiliensis Ab134T genome.
Figure S7. Representative gene context of type VI secretion system (T6SS) of the P. brasiliensis Ab134T genome (A) and P. denitrificans JCM 12308T genome (B).
Table S1. Genome and 16S rRNA accession numbers of Pseudovibrio, Stappia stellulata DSM 5886, Pannonibacter indicus HT23, Labrenzia alexandrii DFL-11.
Table S2. Phenotypic characterization of Pseudovibrio species. 1—P. brasiliensis Ab134T; 2—P. denitrificans JCM 12308 T; 3—P. japonicus NCIMB 14279T; 4—P. axinellae Ad2T; +, positive; −, negative.
Table S3. Genomic characterization of P. brasiliensis Ab134T. Identity (%) of the 16S rRNA (1) and recA gene sequences (2), average amino acid identity (AAI) (3) similarity (%) of the whole genome, and (4) in silico DNA-DNA hybridization (GGD) (4) between Pseudovibrio species.
Table S4. Genes unique to P. brasiliensis Ab134T, as assessed by functional comparison with P. denitrificans JCM 12308 T performed by the RAST server.
Table S5. Genes related to nitrogen metabolism in the P. brasiliensis Ab134T genome annotated by the RAST server.
Table S6. Genes related to fermentation present in the P. brasiliensis Ab134T genome based on functional annotation by the RAST server.
Table S7. Probable toxin-like homologous of P. brasiliensis Ab134T were identified by BLASTP search against the virulence factors database. Rows showing redundant functions are highlighted in bold.
References
Alex, A., and Antunes, A. (2015). Whole genome sequencing of the symbiont Pseudovibrio sp. from the intertidal marine sponge polymastia penicillus revealed a gene repertoire for host-switching permissive lifestyle. Genome Biol. Evol. 7, 3022–3032. doi: 10.1093/gbe/evv199
Altschul, S. F., Gish, W., Miller, W., Myers, E. W., and Lipman, D. J. (1990). Basic local alignment search tool. J. Mol. Biol. 215, 403–410. doi: 10.1016/S0022-2836(05)80360-2
Amaral, G. R., Dias, G. M., Wellington-Oguri, M., Chimetto, L., Campeão, M. E., Thompson, F. L., et al. (2014). Genotype to Phenotype: identification of diagnostic vibrio phenotypes using whole genome sequences. Int. J. Syst. Evol. Microbiol. 64(Pt 2), 357–365. doi: 10.1099/ijs.0.057927-0
Appolinario, L. R., Tschoeke, D. A., Rua, C. P. J., Venas, T., Campeão, M. E., Amaral, G. R. S., et al. (2016). Description of Endozoicomonas arenosclerae sp. nov. using a genomic taxonomy approach. Antonie van Leeuwenhoek 109, 431–438. doi: 10.1007/s10482-016-0649-x
Aschtgen, M. S., Bernard, C. S., De Bentzmann, S., Lloubès, R., and Cascales, E. (2008). SciN is an outer membrane lipoprotein required for type VI secretion in enteroaggregative Escherichia coli. J. Bacteriol. 190, 7523–7531. doi: 10.1128/JB.00945-08
Auch, A. F., Jan, M., Von Klenk, H., and Göker, M. (2010). Digital DNA-DNA hybridization for microbial species delineation by means of genome-to-genome sequence comparison. Stand. Genomics Sci. 2, 117–134. doi: 10.4056/sigs.531120
Aziz, R. K., Bartels, D., Best, A. A., Dejongh, M., Disz, T., Edwards, R. A., et al. (2008). The RAST server : rapid annotations using subsystems technology. BMC Genomics 15:75. doi: 10.1186/1471-2164-9-75
Bauvais, C., Zirah, S., Piette, L., Chaspoul, F., Domart-Coulon, I., Chapon, V., et al. (2015). Sponging up metals: bacteria associated with the marine sponge Spongia officinalis. Mar. Environ. Res. 104, 20–30. doi: 10.1016/j.marenvres.2014.12.005
Bender, C. L., Alarcón-Chaidez, F., and Gross, D. C. (1999). Pseudomonas syringae phytotoxins: mode of action, regulation, and biosynthesis by peptide and polyketide synthetases. Microbiol. Mol. Biol. Rev. 63, 266–292.
Bondarev, V., Richter, M., Romano, S., Piel, J., Schwedt, A., and Schulz-Vogt, H. N. (2013). The genus Pseudovibrio contains metabolically versatile bacteria adapted for symbiosis. Environ. Microbiol. 15, 2095–2113. doi: 10.1111/1462-2920.12123
Boyer, F., Fichant, G., Berthod, J., Vandenbrouck, Y., and Attree, I. (2009). Dissecting the bacterial type VI secretion system by a genome wide in silico analysis: what can be learned from available microbial genomic resources? BMC Genomics 10:104. doi: 10.1186/1471-2164-10-104
Breitbart, M., Hewson, I., Felts, B., Mahaffy, J. M., Nulton, J., Salamon, P., et al. (2003). Metagenomic analyses of an uncultured viral community from human feces. J. Bacteriol. 185, 6220–6223. doi: 10.1128/JB.185.20.6220-6223.2003
Büttner, D. (2012). Protein export according to schedule: architecture, assembly, and regulation of type III secretion systems from plant- and animal-pathogenic bacteria. Microbiol. Mol. Biol. Rev. 76, 262–310. doi: 10.1128/MMBR.05017-11
Chen, L., Li, P., Deng, Z., and Zhao, C. (2015). Ornithine transcarbamylase ArgK plays a dual role for the self-defense of phaseolotoxin producing pseudomonas syringae pv. phaseolicola. Sci. Rep. 5:12892. doi: 10.1038/srep12892
Chen, L., Zheng, D., Liu, B., Yang, J., and Jin, Q. (2016). VFDB 2016: hierarchical and refined dataset for big data analysis-−10 years on. Nucleic Acids Res. 44, D694–D697. doi: 10.1093/nar/gkv1239
Chenal, A., Sotomayor-Perez, A. C., and Ladant, D. (2015). 23 - Structure and Function of RTX toxins BT - The Comprehensive Sourcebook of Bacterial Protein Toxins, 4th Edn. Boston, MA: Academic Press.
Chow, J., and Mazmanian, S. K. (2010). A pathobiont of the microbiota balances host colonization and intestinal inflammation. Cell Host Microbe 7, 265–276. doi: 10.1016/j.chom.2010.03.004
Coil, D., Jospin, G., and Darling, A. E. (2015). A5-miseq: an updated pipeline to assemble microbial genomes from Illumina MiSeq data. Bioinformatics 31, 587–589. doi: 10.1093/bioinformatics/btu661
Costa, T. R., Felisberto-Rodrigues, C., Meir, A., Prevost, M. S., Redzej, A., Trokter, M., et al. (2015). Secretion systems in Gram-negative bacteria: structural and mechanistic insights. Nat. Rev. Microbiol. 13:343. doi: 10.1038/nrmicro3456
Cotter, P. D, Hill, C., and Ross, R. P. (2005). Bacteriocins:developing innate immunity for food. Nat. Rev. Microbiol. 3, 777–788. doi: 10.1038/nrmicro1273
Dale, C., and Moran, N. A. (2006). Molecular interactions between bacterial symbionts and their hosts. Cell 126, 453–465. doi: 10.1016/j.cell.2006.07.014
Deegan, L. H., Cotter, P. D., Hill, C., and Ross, P. (2006). Bacteriocins: biological tools for bio-preservation and shelf-life extension. Int. Dairy J. 16, 1058–1071. doi: 10.1016/j.idairyj.2005.10.026
De Oliveira, J. H. H. L., Seleghim, M. H. R., Timm, C., Grube, A., Köck, M., and Nascimento, G. G. F. (2006). Antimicrobial and antimycobacterial activity of cyclostellettamine alkaloids from sponge Pachychalina sp. Mar. Drugs 4, 1–8. doi: 10.1055/5-2005-916239
Desriac, F., Defer, D., Bourgougnon, N., Brillet, B., Le Chevalier, P., and Fleury, Y. (2010). Bacteriocin as weapons in the marine animal-associated bacteria warfare: inventory and potential applications as an aquaculture probiotic. Mar. Drugs 8, 1153–1177. doi: 10.3390/md8041153
de Souza Santos, M., Salomon, D., Li, P., Krachler, A. M., and Orth, K. (2015). “8 - Vibrio parahaemolyticus virulence determinants A2 - Alouf, Joseph,” in, eds. D. Ladant and M. R. B. T.-T. C. S. of B. P. T. Fourth, E. Popoff (Boston: Academic Press), 230–260.
Eddy, S. R. (2009). A new generation of homology search tools based on probabilistic inference. Genome Inform. 23, 205–211. doi: 10.1142/9781848165632_0019
Egan, F., Reen, F. J., and O'Gara, F. (2015). Tle distribution and diversity in metagenomic datasets reveal niche specialization. Environ. Microbiol. Rep. 7, 194–203. doi: 10.1111/1758-2229.12222
Essack, S. Y. (2001). The development of β-lactam antibiotics in response to the evolution of β-lactamases. Pharm. Res. 18, 1391–1399. doi: 10.1023/A:1012272403776
Esteves, A. I., Cullen, A., and Thomas, T. (2017). Competitive interactions between sponge-associated bacteria. FEMS Microbiol. Ecol. 93:fix008. doi: 10.1093/femsec/fix008
Felsenstein, J. (1985). Phylogenies and the comparative method. the american naturalist. Am. Nat. 125, 1–15. doi: 10.1086/284325
Fukunaga, Y., Kurahashi, M., Tanaka, K., Yanagi, K., Yokota, A., and Harayama, S. (2006). Pseudovibrio ascidiaceicola sp. nov., isolated from ascidians (sea squirts). Int. J. Syst. Evol. Microbiol. 56, 343–347. doi: 10.1099/ijs.0.63879-0
Guenzi, E., Galli, G., Grgurina, I., Gross, D. C., and Grandi, G. (1998). Characterization of the syringomycin synthetase gene cluster: a link between prokaryotic and eukaryotic peptide synthetases. J. Biol. Chem. 273, 32857–32863. doi: 10.1074/jbc.273.49.32857
Har-el, R., and Tanzer, M. L. (1993). Extracellular matrix. 3: evolution of the extracellular matrix in invertebrates. FASEB J. 7, 1115–1123.
Hood, R. D., Singh, P., Hsu, F., Güvener, T., Carl, M. A., Trinidad, R. R., et al. (2017). A type VI secretion system of Pseudomonas aeruginosa targets a toxin to bacteria. Cell Host Microbe 7, 25–37. doi: 10.1016/j.chom.2009.12.007
Hosoya, S., and Yokota, A. (2007). Pseudovibrio japonicus sp. nov. isolated from coastal seawater in Japan. Int. J. Syst. Evol. Microbiol. 57, 1952–1955. doi: 10.1099/ijs.0.64922-0
Huang, X., and Madan, A. (1999). CAP 3: a DNA sequence assembly program. Genome Res. 9, 868–877. doi: 10.1101/gr.9.9.868
Hyatt, D., Chen, G. L., LoCascio, P. F., Land, M. L., Larimer, F. W., and Hauser, L. J. (2010). Prodigal: prokaryotic gene recognition and translation initiation site identification. BMC Bioinformatics 11:119. doi: 10.1186/1471-2105-11-119
Jani, A. J., and Cotter, P. A. (2017). Type VI secretion: not just for pathogenesis anymore. Cell Host Microbe 8, 2–6. doi: 10.1016/j.chom.2010.06.012
Kapitein, N., and Mogk, A. (2017). Type VI secretion system helps find a niche. Cell Host Microbe 16, 5–6. doi: 10.1016/j.chom.2014.06.012
Kennedy, J., Marchesi, J. R., and Dobson, A. D. (2008). Marine metagenomics: strategies for the discovery of novel enzymes with biotechnological applications from marine environments. Microb. Cell Fact. 7:27. doi: 10.1186/1475-2859-7-27
Larkin, M. A., Blackshields, G., Brown, N. P., Chenna, R., McGettigan, P. A., McWilliam, H., et al. (2007). Clustal W. and Clustal X version 2.0. Bioinformatics 23, 2947–2948. doi: 10.1093/bioinformatics/btm404
Lenski, R. E., and Riley, M. A. (2002). Chemical warfare from an ecological perspective. Proc. Natl. Acad. Sci. U.S.A. 99, 556–558. doi: 10.1073/pnas.022641999
Linhartová, I., Bumba, L., Mašín, J., Basler, M., Osicka, R., Kamanová, J., et al. (2010). RTX proteins: a highly diverse family secreted by a common mechanism. Fems Microbiol. Rev. 34, 1076–1112. doi: 10.1111/j.1574-6976.2010.00231.x
Lu, C. D. (2006). Pathways and regulation of bacterial arginine metabolism and perspectives for obtaining arginine overproducing strains. Appl. Microbiol. Biotechnol. 70, 261–272. doi: 10.1007/s00253-005-0308-z
Ma, L. S., Hachani, A., Lin, J. S., Filloux, A., and Lai, E. M. (2014). Agrobacterium tumefaciens deploys a superfamily of type VI secretion DNase effectors as weapons for interbacterial competition in planta. Cell Host Microbe 16, 94–104. doi: 10.1016/j.chom.2014.06.002
Martínez-García, P. M., Ramos, C., and Rodríguez-Palenzuela, P. (2015). T346Hunter: a novel web-based tool for the prediction of type, III. Type IV. and Type VI secretion systems in bacterial genomes. PLoS ONE 10:e0119317. doi: 10.1371/journal.pone.0119317
Meier-kolthoff, J. P., Klenk, H., and Go, M. (2016). Taxonomic use of DNA G + C content and DNA – DNA hybridization in the genomic age. 64(Pt 2), 352–356. doi: 10.1099/ijs.0.056994-0
Mijares, M. R., Ochoa, M., Barroeta, A., Martinez, G. P., Suarez, A. I., Compagnone, R. S., et al. (2013). Cytotoxic effects of Fisturalin-3 and 11-Deoxyfisturalin-3 on Jurkat and U937 cell lines. Biomed. Pap. 157, 222–226. doi: 10.5507/bp.2012.089
Moreira, A. P. B., Chimetto Tonon, L. A., Do Valle, P., Pereira, C., Alves, N., Amado-Filho, G. M., Francini-Filho, R. B., et al. (2014). Culturable heterotrophic bacteria associated with healthy and bleached scleractinian madracis decactis and the fireworm hermodice carunculata from the remote St. Peter and St. Paul Archipelago, Brazil. Curr. Microbiol. 68, 38–46. doi: 10.1007/s00284-013-0435-1
Mosqueda, G., Van den Broeck, G., Saucedo, O., Bailey, A. M., Alvarez-Morales, A., and Herrera-Estrella, L. (1990). Isolation and characterization of the gene from Pseudomonas syringae pv.phaseolicola encoding the phaseolotoxin-insensitive ornithine carbamoyltransferase. Mol. Gen. Genet. 222, 461–466. doi: 10.1007/BF00633857
Muscholl-Silberhorn, A., Thiel, V., and Imhoff, J. F. (2008). Abundance and bioactivity of cultured sponge-associated bacteria from the Mediterranean Sea. Microb. Ecol. 55, 94–106. doi: 10.1007/s00248-007-9255-9
Nei, M., and Kumar, S. (2000). Molecular Evolution and Phylogenetics. New York, NY: Oxford University Press.
Nicacio, K. J., Ióca, L. P., Fróes, A. M., Leomil, L., Appolinario, L. R., Thompson, C. C., et al. (2017). Cultures of the marine bacterium Pseudovibrio denitrificans Ab134 produce bromotyrosine-derived alkaloids previously only isolated from marine sponges. J. Nat. Prod. 80, 235–240. doi: 10.1021/acs.jnatprod.6b00838
O'Halloran, J. A., Barbosa, T. M., Morrissey, J. P., Kennedy, J., Dobson, A. D. W., and O'Gara, F. (2013). Pseudovibrio axinellae sp. nov., isolated from an Irish marine sponge. Int. J. Syst. Evol. Microbiol. 63, 141–145. doi: 10.1099/ijs.0.040196-0
Overbeek, R., Olson, R., Pusch, G. D., Olsen, G. J., Davis, J. J., Disz, T., et al. (2014). The SEED and the rapid annotation of microbial genomes using Subsystems Technology (RAST). Nucleic Acids Res. 42, 206–214. doi: 10.1093/nar/gkt1226
Özbek, S., Balasubramanian, P. G., Chiquet-Ehrismann, R., Tucker, R. P., and Adams, J. C. (2010). The evolution of extracellular matrix. Mol. Biol. Cell 21, 4300–4305. doi: 10.1091/mbc.E10-03-0251
Penesyan, A., Tebben, J., Lee, M., Thomas, T., Kjelleberg, S., Harder, T., et al. (2011). Identification of the antibacterial compound produced by the marine epiphytic bacterium Pseudovibrio sp. D323 and related sponge-associated bacteria. Mar. Drugs 9, 1391–1402. doi: 10.3390/md9081391
Persson, O. P., Pinhassi, J., Riemann, L., Marklund, B. I., Rhen, M., Normark, S., et al. (2009). High abundance of virulence gene homologues in marine bacteria. Environ. Microbiol. 11, 1348–1357. doi: 10.1111/j.1462-2920.2008.01861.x
Piper, C., Cotter, P. D., Ross, R. P., and Hill, C. (2009). Discovery of medically significant lantibiotics. Curr. Drug Discov. Technol. 6, 1–18. doi: 10.2174/157016309787581075
Pitcher, D. G., Saunders, N. A., and Owen, R. J. (1989). Rapid extraction of bacterial genomic DNA with guanidium thiocyanate. Lett. Appl. Microbiol. 8, 151–156. doi: 10.1111/j.1472-765X.1989.tb00262.x
Riley, M. A., and Gordon, D. M. (1999). The ecological role of bacteriocins in bacterial competition. Trends Microbiol. 7, 129–133. doi: 10.1016/S0966-842X(99)01459-6
Riley, M. A., and Wertz, J. E. (2002). Bacteriocins: evolution, ecology, and application. Annu. Rev. Microbiol. 56, 117–137. doi: 10.1146/annurev.micro.56.012302.161024
Romano, S., Fernàndez-Guerra, A., Reen, F. J., Glöckner, F. O., Crowley, S. P., O'Sullivan, O., et al. (2016). Comparative genomic analysis reveals a diverse repertoire of genes involved in prokaryote-eukaryote interactions within the Pseudovibrio Genus. Front. Microbiol. 7:387. doi: 10.3389/fmicb.2016.00387
Rua, C. P., Trindade-Silva, A. E., Appolinario, L. R., Venas, T. M., Garcia, G. D., Carvalho, L. S., et al. (2014). Diversity and antimicrobial potential of culturable heterotrophic bacteria associated with the endemic marine sponge Arenosclera brasiliensis. Peer J 2:e419. doi: 10.7717/peerj.419
Russell, A. B., Peterson, S. B., and Mougous, J. D. (2014). Type VI secretion system effectors: poisons with a purpose. Nat. Rev. Microbiol. 12:137. doi: 10.1038/nrmicro3185
Saitou, N., and Nei, M. (1987). The neighbour-joining method: a new method for reconstructing phylogenetic trees. Mol. Biol. Evo. 4, 406–425.
Santos Ede, O., Alves, N. Jr., Dias, G. M., Mazotto, A. M., Vermelho, A., Vora, G. J., et al. (2011). Genomic and proteomic analyses of the coral pathogen Vibrio coralliilyticus reveal a diverse virulence repertoire. ISME J. 5, 1471–1483. doi: 10.1038/ismej.2011.19
Sawada, H., Kanaya, S., Tsuda, M., Suzuki, F., Azegami, K., and Saitou, N. (2002). A phylogenomic study of the OCTase genes in Pseudomonas syringae pathovars: the horizontal transfer of the argK–tox cluster and the evolutionary history of OCTase genes on their genomes. J. Mol. Evol. 54, 437–457. doi: 10.1007/s00239-001-0032-y
Schaller, A., Kuhn, R., Kuhnert, P., Nicolet, J., Anderson, T. J., Maclnnes, J. I., et al. (1999). Characterization of apxlVA, a new RTX determinant of Actinobacillus pleuropneumoniae. Microbiology 145(Pt 8), 2105–2116.
Schmieder, R., and Edwards, R. (2011). Quality control and preprocessing of metagenomic datasets. Bioinformatics 27, 863–864. doi: 10.1093/bioinformatics/btr026
Sertan-De Guzman, A. A., Predicala, R. Z., Bernardo, E. B., Neilan, B. A., Elardo, S. P., Mangalindan, G. C., et al. (2007). Pseudovibrio denitrificans strain Z143-1, a heptylprodigiosin-producing bacterium isolated from a Philippine tunicate. FEMS Microbiol. Lett. 277, 188–196. doi: 10.1111/j.1574-6968.2007.00950.x
Shieh, W. Y., Lin, Y., and Te Jean, W. D. (2004). Pseudovibrio denitrificans gen. nov., sp. nov., a marine, facultatively anaerobic, fermentative bacterium capable of denitrification. Int. J. Syst. Evol. Microbiol. 54(Pt 6), 2307–2312. doi: 10.1099/ijs.0.63107-0
Sweet, M., Bulling, M., and Cerrano, C. (2015). A novel sponge disease caused by a consortium of micro-organisms. Coral Reefs 34, 871–883. doi: 10.1007/s00338-015-1284-0
Tamura, K., Stecher, G., Peterson, D., Filipski, A., and Kumar, S. (2013). MEGA6: molecular evolutionary genetics analysis version 6.0. Mol. Biol. Evol. 30, 2725–2729. doi: 10.1093/molbev/mst197
Taylor, M. W., Radax, R., Steger, D., and Wagner, M. (2007). Sponge-associated microorganisms: evolution, ecology, and biotechnological potential. Microbiol. Mol. Biol. Rev. 71, 295–347. doi: 10.1128/MMBR.00040-06
Thompson, C. C., Amaral, G. R., Campeão, M., Edwards, R. A., Polz, M. F., Dutilh, B. E., et al. (2015). Microbial taxonomy in the post-genomic era: rebuilding from scratch? Arch. Microbiol. 197, 359–370. doi: 10.1007/s00203-014-1071-2
Thompson, C. C., Silva, G. G. Z., Vieira, N. M., Edwards, R., Vicente, A. C. P., and Thompson, F. L. (2013). Genomic taxonomy of the genus Prochlorococcus. Microb. Ecol. 66, 752–762. doi: 10.1007/s00248-013-0270-8
Vizcaino (2011). Identification of the antibacterial compound produced by the marine epiphytic bacterium Pseudovibrio sp. D323 and related sponge-associated bacteria. Mar. Drugs 9, 1391–1402.
Voth, D. E., Broederdorf, L. J., and Graham, J. G. (2012). Bacterial type IV secretion systems: versatile virulence machines. Future Microbiol. 7, 241–257. doi: 10.2217/fmb.11.150
Wallden, K., Rivera-Calzada, A., and Waksman, G. (2010). Type IV secretion systems: versatility and diversity in function. Cell. Microbiol. 12, 1203–1212. doi: 10.1111/j.1462-5822.2010.01499.x
Waterhouse, A. M., Procter, J. B., Martin, D. M., Clamp, M., and Barton, G. J. (2009). Jalview Version 2-A multiple sequence alignment editor and analysis workbench. Bioinformatics 25, 1189–1191. doi: 10.1093/bioinformatics/btp033
Weber, B., Hasic, M., Chen, C., Wai, S. N., and Milton, D. L. (2009). Type VI secretion modulates quorum sensing and stress response in Vibrio anguillarum. Environ. Microbiol. 11, 3018–3028. doi: 10.1111/j.1462-2920.2009.02005.x
Weber, T., Blin, K., Duddela, S., Krug, D., and Kim, H. U. (2015). antiSMASH 3.0 —- a comprehensive resource for the genome mining of biosynthetic gene clusters. 43, W237–W243. doi: 10.1093/nar/gkv437
Webster, N. S., and Hill, R. T. (2001). The culturable microbial community of the Grat Barrier Reef sponge Phopaloeides odorabile is dominated by an α-Proteobacterium. Mar. Biol. 138, 843–851. doi: 10.1007/s002270000503
Welch, R. A. (2001). RTX toxin structure and function: a story of numerous anomalies and few analogies in toxin biology. Curr. Top. Microbiol. Immunol. 257, 85–111.
Xu, Y., Li, Q., Tian, R., Lai, Q., and Zhang, Y. (2015). Pseudovibrio hongkongensis sp. nov. isolated from a marine flatworm. Antonie Van Leeuwenhoek 108, 127–132. doi: 10.1007/s10482-015-0470-y
Keywords: Pseudovibrio, genomic taxonomy, corals, sponges, secondary metabolites, fistularin-3
Citation: Fróes AM, Freitas TC, Vidal L, Appolinario LR, Leomil L, Venas T, Campeão ME, Silva CJF, Moreira APB, Berlinck RGS, Thompson FL and Thompson CC (2018) Genomic Attributes of Novel Symbiont Pseudovibrio brasiliensis sp. nov. Isolated From the Sponge Arenosclera brasiliensis. Front. Mar. Sci. 5:81. doi: 10.3389/fmars.2018.00081
Received: 13 October 2017; Accepted: 22 February 2018;
Published: 12 March 2018.
Edited by:
D. Ipek Kurtboke, University of the Sunshine Coast, AustraliaReviewed by:
Angelina Lo Giudice, Consiglio Nazionale Delle Ricerche (CNR), ItalyPaula Branquinho Andrade, Universidade do Porto, Portugal
Copyright © 2018 Fróes, Freitas, Vidal, Appolinario, Leomil, Venas, Campeão, Silva, Moreira, Berlinck, Thompson and Thompson. This is an open-access article distributed under the terms of the Creative Commons Attribution License (CC BY). The use, distribution or reproduction in other forums is permitted, provided the original author(s) and the copyright owner are credited and that the original publication in this journal is cited, in accordance with accepted academic practice. No use, distribution or reproduction is permitted which does not comply with these terms.
*Correspondence: Fabiano L. Thompson, ZmFiaWFub3Rob21wc29uMUBnbWFpbC5jb20=
Cristiane C. Thompson, dGhvbXBzb25jcmlzdGlhbmVAZ21haWwuY29t