- 1Ecological Genetics Research Unit, Organismal and Evolutionary Biology Research Program, University of Helsinki, Helsinki, Finland
- 2Unité Mixte de Service Patrimoine Naturel – Centre D'expertise et de Données (UMS 2006 AFB – Centre National de la Recherche Scientifique – MNHN), Muséum National d'Histoire Naturelle, Paris, France
- 3Muséum National d'Histoire Naturelle, UMR BOREA – Muséum National d'Histoire Naturelle – Centre National de la Recherche Scientifique 7208 – IRD 207 – Sorbonne Université – UCBN, Paris, France
- 4Tvärminne Zoological Station, University of Helsinki, Hanko, Finland
The European flounder Platichthys flesus (Linnaeus, 1758) displays two contrasting reproductive behaviors in the Baltic Sea: offshore spawning of pelagic eggs and coastal spawning of demersal eggs, a behavior observed exclusively in the Baltic Sea. Previous studies showed marked differences in behavioral, physiological, and life-history traits of flounders with pelagic and demersal eggs. Furthermore, a recent study demonstrated that flounders with pelagic and demersal eggs represent two reproductively isolated, parapatric species arising from two distinct colonization events from the same ancestral population. Using morphological data we first established that the syntypes on which the original description of P. flesus was based belong to the pelagic-spawning lineage. We then used a combination of morphological and physiological characters as well as genome-wide genetic data to describe flounders with demersal eggs as a new species: Platichthys solemdali sp. nov. The new species can be clearly distinguished from P. flesus based on egg morphology, egg and sperm physiology as well as via population genetic and phylogenetic analyses. While the two species do show some minor morphological differences in the number of anal and dorsal fin rays, no external morphological feature can be used to unambiguously identify individuals to species. Therefore, we developed a simple molecular diagnostic test able to unambiguously distinguish P. solemdali from P. flesus with a single PCR reaction, a tool that should be useful to fishery scientists and managers, as well as to ecologists studying these species.
Introduction
The European flounder Platichthys flesus (Linnaeus, 1758) is a flatfish from the family Pleuronectidae (Rafinesque, 1815) with a broad distribution in coastal and brackish European waters, from the Black Sea to the White Sea (Whitehead et al., 1986). As migratory fish, European flounders are commonly found in shallow coastal waters and in estuaries (Summers, 1979); they can enter freshwater systems for extended periods of time, though they do not successfully reproduce in freshwater (Nissling et al., 2002). The European flounder is one of the few marine species that thrive in the low salinities of the Baltic Sea, a large body of brackish water that became connected to the North Sea at the end of the last glaciation, ~8.5 kya (Gustafsson and Westman, 2002). The transition zone between the North Sea and the Baltic Sea (Figure 1A) presents a steep gradient in salinity, which rapidly decreases from the typical values of the marine environment (>30 psu) to values as little as 5–7 psu encountered in the shallow coastal waters of the Baltic Proper (Figure 1A). To spawn pelagic propagules, the most common reproductive strategy in marine fishes, is no trivial affair in the low salinity waters of the Baltic Sea. As salinity decreases, so does the specific gravity of seawater, rendering the production of neutrally buoyant eggs a challenging task. The relatively few marine fish species that thrive in the Baltic Sea exhibit adaptations to the low salinity and temperature they have encountered (Nissling et al., 2002, 2006; Hemmer-Hansen et al., 2007; Florin and Höglund, 2008; Nissling and Dahlman, 2010; Guo et al., 2015). Some of them show ecological adaptations allowing the spawning of pelagic eggs that can maintain neutral or positive buoyancy in deep basins, where salinity is higher than in coastal waters (Nissling et al., 1994, 2002; Vallin and Nissling, 2000; Nissling and Dahlman, 2010). Other species have changed their life-history strategies by switching to coastal spawning of demersal eggs (Nissling et al., 2006). European flounders in the Baltic Sea display both strategies. Numerous studies have investigated these two types of flounders, hereafter referred to as “demersal flounders” and “pelagic flounders.”
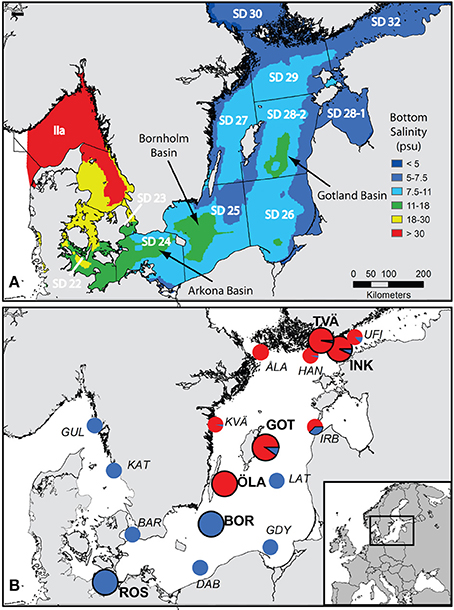
Figure 1. Map of the Baltic Sea showing (A) modeled mean bottom salinity in the Baltic Sea from Bendtsen et al. (2007) and the borders and names of ICES subdivisions; deep-water basins where pelagic flounders can reproduce have a salinity above 11 psu (green color) and are indicated by arrows, and (B) the sampling locations from this study (large pie charts with black rings and bold text labels) and from Momigliano et al. (2017a) (small pie charts and italics text labels), with an inset showing the position of the study area within Europe. Pie charts represent the proportion of demersal flounders (P. solemdali sp. nov., in red) and pelagic flounders (P. flesus, in blue) identified by genetic tests in both studies, and thus give a contemporary snapshot of each of the two species distribution in the Baltic Sea and their relative abundance. To be noticed that P. flesus were present in almost every location (with the exception of Öland and Åland), while P. solemdali sp. nov. distribution seems not to extend to the southern Baltic Sea. Abbreviations are as follows: UFI = Helsinki, Gulf of Finland (Momigliano et al., 2017a); INK = Inkoo, Gulf of Finland (this study); TVÄ = Tvärminne, Gulf of Finland (this study); HAN = Hanko, Gulf of Finland (Momigliano et al., 2017a); ÅLA = Åland Archipelago (Momigliano et al., 2017a); IRB = Irbe Strait, Estonia (Momigliano et al., 2017a); GOT = Lausvik, Gotland (this study); KVÄ = Kvädofjärden, Sweden (Momigliano et al., 2017a); LAT = Latvian Sea, Latvia (Momigliano et al., 2017a); ÖLA = Öland, Sweden (this study; Momigliano et al., 2017a); BOR = Bornholm Basin (this study; Momigliano et al., 2017a); GDY = Gdynia, Poland (Momigliano et al., 2017a); DAB = Dabki, Poland (Momigliano et al., 2017a); ROS = Rostock, Germany (this study), BAR = Barsebäck, Sweden (Momigliano et al., 2017a); KAT = Kattegat, Sweden (Momigliano et al., 2017a); GUL = Gullmaren, Sweden (Momigliano et al., 2017a). For further details on sampling locations from this study and from Momigliano et al. (2017a) see Table 1 and Table S1.
Per Solemdal was the first to notice that reproductive traits such as egg size and buoyancy in Baltic Sea flounders are population-specific (Solemdal, 1970, 1971, 1973; Lønning and Solemdal, 1974). He found that flounders produce larger eggs with lower specific gravity along the decreasing salinity gradient from the Atlantic toward the northern Baltic Sea, and that this feature is an innate population-specific characteristic, suggestive of genetic adaptation rather than phenotypic plasticity (Solemdal, 1967, 1970, 1973). Eggshell (chorion) thickness was shown to co-vary with egg size and specific gravity, so that the chorion is thinner in flounder eggs from less saline waters (Lønning and Solemdal, 1974). As the chorion is the densest of the egg components, its thickness explains the differences in the egg specific gravity and consequently the salinity required for obtaining neutral buoyancy (Solemdal, 1973). Solemdal discovered that flounder eggs with the lowest specific gravity are found in waters of 10–12 psu salinity, and the limit for successful egg development in pelagic flounders is ~11 psu (Solemdal, 1967, 1971, 1973). Nevertheless, reproductive flounder populations occur in Baltic coastal areas with salinities as low as 6 psu (Sandman, 1906; Bonsdorff and Norkko, 1994). These flounders produce sinking eggs that are smaller, denser, and have thicker chorions than the other flounders in the Baltic (Solemdal, 1967, 1971, 1973; Lønning and Solemdal, 1974). Artificial fertilization of these demersal eggs was also possible at salinities as low as 6.5 psu (Solemdal, 1967, 1973). Long-term acclimatization experiments did not alter these characteristics, suggesting these features to be a product of genetic adaptations rather than phenotypic plastic responses (Solemdal, 1973).
The main findings of different reproductive characteristics were later confirmed by the experiments and measurements by Nissling et al. (2002, 2017). These studies revealed adaptations not only in egg size and buoyancy, but also in sperm physiology: the activation of sperm from pelagic flounders requires salinities of above 10 psu, while sperm from demersal flounders can be activated at salinities as low as 3 psu and maintains high motility at salinities encountered in the northern Baltic Sea (Nissling et al., 2002). In addition to spawning behavior and reproductive traits, also migration patterns (Aro, 1989), growth differences (Nissling and Dahlman, 2010), maturation time (Erlandsson et al., 2017), fecundity (Nissling and Dahlman, 2010), fecundity regulation (Nissling et al., 2015), and morphology (Jonsson, 2014; Stålberg, 2015) for different flounder populations across the Baltic have been described and compared between the two ecotypes. For instance, several tagging studies—reviewed by Aro (1989, 2002) and Florin (2005)—have shown that the pelagic spawning flounders generally feed in shallow waters and migrate to spawn in deep waters, while demersal flounders usually reside in the archipelago and coastal areas throughout their lives (Bagge, 1981; Aro and Sjöblom, 1983). Also, while the growth of flounders varies geographically, decreasing from southwest to northeast (Drevs et al., 1999), there are also differences in growth between the two flounder types; pelagic flounders grow faster than the demersal flounders (Nissling and Dahlman, 2010). Furthermore, the demersal flounders commence spawning also somewhat earlier and have higher fecundity than the pelagic ones (Nissling and Dahlman, 2010).
Genetic studies carried out over the past decade demonstrated that these morphological, physiological, and life-history differences are linked to stable and distinct genetic populations. Hemmer-Hansen et al. (2007) and Florin and Höglund (2008) investigated P. flesus population genetics using microsatellite markers, and discovered significant (albeit very weak, FST 0.01–0.03) genetic differentiation associated with spawning behavior. Using a combination of genome-wide SNP genotyping and approximate Bayesian computation modeling, a recent study demonstrated that the two flounder types originated via two distinct colonizations of the Baltic Sea from the same ancestral population (Momigliano et al., 2017a). The demersal flounders invaded the Baltic Sea at the beginning of the Litorina Sea stage, a period of marine transgression, which started ~8,000 years BP transforming the freshwater Ancylus Lake into the large body of brackish water that is the modern Baltic Sea (Björck, 1995; Gustafsson and Westman, 2002); at the very start of the Litorina Sea stage, salinity in the newly formed Baltic Sea was extremely low (Gustafsson and Westman, 2002) and pelagic spawning would not have been possible. The pelagic flounders invaded the Baltic Sea later on, possibly during peak salinity (6,500–5,000 years BP), when salinity averaged at 10–15 psu and pelagic spawning would have been possible in extended areas of the Baltic Sea (Gustafsson and Westman, 2002). However, the spawning grounds of pelagic flounders have likely been contracting since due to the steady decrease in salinity that the Baltic Sea has experienced in the past 5,000 years (Gustafsson and Westman, 2002). Today salinity in the Baltic Sea averages at 7–8 psu, and pelagic flounders encounter environmental conditions suitable for reproduction only in the deep waters of the Arkona, the Bornholm, and the Gotland Basins (Figure 1; Nissling et al., 2002). The two groups have a parapatric distribution with extensive overlap in the central Baltic where they co-occur in the same feeding and wintering grounds, yet they exhibit very strong reproductive isolation: even in locations where they co-occur, there is no evidence of hybrids (Momigliano et al., 2017a). Pelagic and demersal flounders show strongly bimodal genotypic clustering, no evidence of contemporary migration, and they represent clearly distinct phylogenetic clades (Momigliano et al., 2017a). Furthermore, their genomes revealed strong signatures of selection associated with salinity and reproductive traits such as sperm motility (Momigliano et al., 2017a). Genetic differentiation across most of the genome is still weak (FST ~ 0.05); this, however, is a reflection of the fact that the two flounder types have not yet reached mutation-drift equilibrium, rather than evidence of ongoing gene flow (Momigliano et al., 2017a). Momigliano et al. (2017a) argued these differences in sperm motility, egg buoyancy, and reproductive behavior (all of which are expected to be under strong ecological selection) act as reproductive barriers, suggesting the two lineages diverged via a rapid process of ecological speciation (Nosil, 2012).
Thus, according to the body of research on Baltic Sea flounders summarized above, the demersal and pelagic flounders can be considered as distinct species under several species concepts (see De Queiroz, 2007). The two lineages are distinguishable by diagnosable morphological (e.g., egg size and structure), physiological (sperm motility), and molecular (genotypes) characters as required by the phylogenetic species concept sensu Cracraft (1983). The absence of specimens with intermediate genotypes, even in locations where both taxa co-occur in similar proportions, shows these lineages are genotypic clusters (sensu Mallet, 1995) and there is strong reproductive isolation suggesting they are distinct species according to the biological species concept as well (Mayr, 1942; Dobzhansky and Dobzhansky, 1970). These lineages occupy distinct ecological niches as they exhibit morphological, physiological, and life history adaptations to spawning grounds that differ in terms of salinity, oxygen concentration, temperature regime, wave abrasion, spawning substrate, and food availability, and hence, they also fulfill the criteria dictated by the ecological species concept (Van Valen, 1976; Andersson, 1990). Finally, according to the evolutionary species concept (sensu Wiley and Mayden, 2000), by which species can be considered as “individuals” (sensu Mayden, 2002) which are born, develop, and generate new species before dying, the demersal lineage can be viewed as a “newborn” species which can be described and diagnosed (Mayden, 2002).
The aim of this article is to bring forth a description of the new species of flounder occurring in the Baltic Sea using a combination of molecular, physiological, and morphological data, and to develop a molecular tool for the unambiguous identification of pelagic and demersal flounders. This description is not only of academic interest for a number reasons. First, the European flounder Platichthys flesus (Linnaeus, 1758) is a species of economic importance in the Atlantic coast of Europe as well as in the Baltic Sea, where 16–24 thousand tons of this flatfish are caught per year (ICES, 2017). As the sustainable management of fish stocks requires understanding what constitutes a biologically meaningful demographic unit (Reiss et al., 2009), recognition of cryptic species within P. flesus can aid sustainable fisheries (Utter and Ryman, 1993; Begg et al., 1999; Sterner, 2007). As to the latter, we note that the pelagic and demersal flounders can co-occur, at times in similar proportions, in various locations of the central and northern Baltic Sea (Momigliano et al., 2017a; see also Figure 1B), and therefore, constitute a mixed stock fishery (Begg et al., 1999). This unobserved diversity could lead to overexploitation of the most vulnerable stock component (Sterner, 2007), and it is therefore important to provide means for distinguishing between the two species. In the absence of clear morphological distinctions, developing genetic diagnostic tools is invaluable to unambiguously identify each species, enabling fishery scientists to carry out mixed stock fishery analyses (Utter and Ryman, 1993). Current management assumes that in some of the International Council for the Exploration of the Sea subdivisions of the Baltic Sea (SD 27, and SD29–32, Figure 1A), only demersal flounders occur (ICES, 2017), though recent results from Momigliano et al. (2017a) and Hinrichsen et al. (2016) suggest that both SD 32 and SD 29 are likely to receive migrant pelagic flounders from southern spawning grounds. Secondly, in some regions of the Baltic Sea (particularly in the Finnish coast, SD 32) flounders have declined, possibly as a result of reduced larval supply and changes in environmental conditions in nursery areas (Jokinen et al., 2015, 2016). To understand the drivers of the decline and to make future predictions, knowledge of species identities and stock composition is imperative. Thirdly, as noted by Momigliano et al. (2017a), eutrophication and climate change are likely to further contract the already geographically limited spawning grounds of pelagic flounders in the Baltic Sea. In order to study and monitor this process, we need to have means to distinguish between the two co-occurring species. Finally, the European flounder is also of interest to taxonomists because of the previous description of another species in the same area (Pleuronectes flesoides Pontoppidan 1765), as well as a description of varieties (viz. Pleuronectes flesus var. trachurus Duncker, 1892; Pleuronectes flesus race baltica Suvorov, 1925; Pleuronectes flesus trachurus natio baltica Suvorov, 1927) based on presence or absence of bony plates (Kottelat, 1997; Evseenko, 2003). However, only one species is currently recognized on the basis of morphological characters (Kottelat, 1997; Voronina, 1999).
Materials and Methods
Sampling
The specimens used in this study were sampled from four different locations in the Baltic Sea: (1) Rostock (Germany), southern Baltic Sea, (2) Gotland (Sweden), central Baltic Sea, (3) Tvärminne (Finland), Gulf of Finland, and (4) Inkoo (Finland), Gulf of Finland (see Table 1 and Figure 1). The majority of specimens of the pelagic population, or P. flesus sensu stricto, were collected from Germany in December 2016 (three individuals) and July 2017 (twelve individuals), but a few individuals were also collected from Gotland (two individuals), Tvärminne (one individual), and Inkoo (one individual). Most samples from Inkoo (15 individuals), Tvärminne (19 individuals), and Gotland (18 individuals) belong to the demersal lineage, which is the new species described here. The fish from Germany and Sweden were sourced through local fishermen, frozen in −20°C, and shipped to Finland, where the specimens were thawed, measured, and photographed. A tissue sample was then excised from the muscle above the spine on the blind side of the animal, and the specimens were then fixed in 10% buffered formalin for 30 days and preserved in 70% EtOH thereafter. The specimens from Inkoo were euthanized with an overdose of MS-222 after which they were measured and fixed exactly as described above. Samples from Tvärminne were collected, measured, and euthanized with an overdose of MS-222 and fixed as above. The 69 specimens used in this study were deposited in the collection of the Finnish Museum of Natural History (MZF), Helsinki, Finland. Furthermore, we also obtained fin clips for genetic analyses from an additional 12 specimens from Tvärminne (Table 1). These specimens where collected as described above; a small fin clip was then excised using sterile 2 mm Rapid-Core® Biopsy punches and the animals were then released.
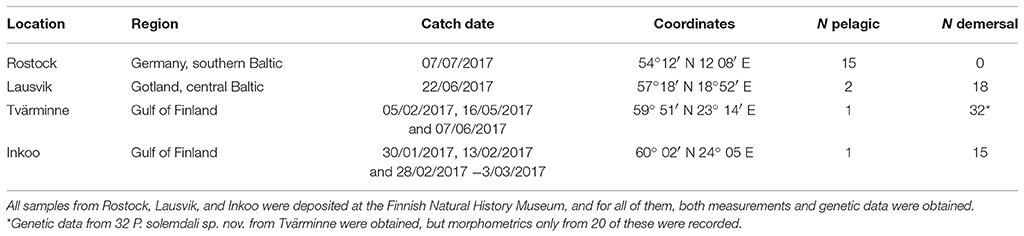
Table 1. Summary of the samples of P. flesus (N pelagic) and P. solemdali sp. nov. (N demersal) collected across the Baltic Sea and analyzed in this study.
Genetic Analyses
Two approaches were used and compared to assign individuals to either putative species. Firstly, we used a genome-wide single nucleotide polymorphism (SNP) approach for a subsample of the individuals (see 2b-RAD genotyping section below) as well as a number of reference P. flesus and demersal flounders from Momigliano et al. (2017a) and Florin and Höglund (2008). Secondly, we developed a multiplex PCR assay to genotype six of the outlier loci identified by Momigliano et al. (2017a) as being under divergent selection between the two species, and assessed their utility as a genetic diagnostic tool to assign individuals to each species.
2b-RAD Genotyping
We used the 2b-RAD method described by Wang et al. (2012) to genotype thousands of genome-wide SNPs from nine reference P. flesus individuals, 11 reference demersal flounders, and 21 of the samples collected and analyzed in this study. The nine reference P. flesus individuals were collected at the time of spawning from the Bornholm basin (55° 25′ N 16° 06′ E) in March 2003 and the 11 reference samples of the demersal flounders were collected at the time of spawning in the coastal waters of Öland (57° 08′ N 17° 03′ E) between April and June 2004. These reference samples represent a subset of the individuals analyzed both in Florin and Höglund (2008) and Momigliano et al. (2017a).
DNA was extracted using a DNeasy Blood and Tissue Kit (Qiagen) following manufacturer's instruction. DNA quality and purity were checked via gel electrophoresis and by spectrophotometry using a Nanodrop 2000 (Thermo Fisher Scientific Inc.). DNA extracts were quantified using a QuBit DNA quantification system (Invitrogen). For each sample, ~200 ng of DNA were digested in a 6 μl reaction containing two units of BcgI enzyme (New England Biolabs, Inc.), 15 μM S-adenosyl-methionine, and 1× NEB Buffer 3.1. Reactions were overlaid with a drop of mineral oil, incubated at 37°C for 2 h and the enzyme inactivated at 65°C for 20 min. Adaptors, one of which included degenerate sequences for the identification of PCR duplicates (Tin et al., 2015), were ligated in a 25 μl reaction at 16°C for 12 h, and T4 ligase was heat-inactivated at 65°C for 20 min. The libraries were amplified using platform-specific and individually barcoded oligos. PCR reactions were prepared as follows: 10 μl ligation, 0.2 μM IC1-P5 primer (5′-ATGATACGGCGACCACCGA-3′), 0.2 μM IC2-P7 (5′-CAAGCAGAAGACGGCATACGA-3′) primer, 0.15 μM Mpx primer (5′-AATGATACGGCGACCACCGAGATCTACACTCTTTCCCTACACGACGCTCTTCCGAT-3′) and, 0.15 μM of indexed primer (5′ P7-8bpIndex-R2primingSite 3′), one unit of Phusion High Fidelity DNA Polymerase (NEB), and 1× Phusion PCR buffer in a total reaction volume of 50 μl. PCR reactions underwent an initial denaturation step of 30 s at 98°C, followed by 12 cycles of 5 s at 98°C, 3 min at 65°C, and 30 s at 72°C, and a final extension of 2 min at 72°C. Libraries were pooled in equimolar concentration; only the target fragment (~170 bp in length) was retained by size-selecting the pooled library using a BluePippin size selector (Sage Science). The library was sequenced on an Illumina NextSeq 500 machine, aiming at obtaining ~4 million reads per sample. For six individuals which were used as technical replicates, we prepared libraries and sequenced them twice.
SNP Calling
SNPs were called de novo using a combination of perl-scripts developed for 2b-RAD data (available from: https://github.com/z0on/2bRAD_denovo). Firstly, we removed PCR duplicates from raw reads, filtered reads retaining only those with 100% matching restriction site, and trimmed all sequences retaining only the 2b-fragments using the script trim2bRAD_2barcodes_dedup.pl. Reads were then quality-filtered using the fastq_quality_filter command in fastx toolkit, retaining only bases with a PHRED quality score ≥20. A table of reference 2b-RAD fragments was produced using the scripts uniquerOne.pl and mergeUniq.pl, and reads counted at least five times were assembled into clusters using cd-hit, allowing up to three mismatches. A locus-cluster annotated table was assembled using the script uniq2loci.pl, filtering by read depth (>5×) as well as strand bias (≥5, calculated as the ratio of the fragments with the least common and more common orientations × 100). Individual genotypes were called using the script haplocall_denovo.pl, filtering for read depth (10×), allele bias (≥10%, calculated as the average fraction of minor allele counts in a heterozygote), strand bias (≥10), and maximum heterozygosity of <0.5. We used six technical replicates to generate a composite quality score (based on read depth, strand bias, allele bias, and SNP position) for genotype quality using the script recalibrateSNPs.pl, and then filtered the SNPs using vcftools retaining only one bi-allelic SNP per locus with a composite quality score of at least 20 and genotyped for at least 80% of the individuals.
Phylogeny
A Maximum Likelihood phylogeny was constructed from the matrix of 5861 concatenated SNPs. The tree was constructed using the software PhyML v. 3.1 (Guindon et al., 2010). A Neighbor Joining starting tree, tree improvement by Nearest Neighbor Interchange and the GTR+G model were used, with gamma estimated from the data and four gamma rate categories, as per Momigliano et al. (2017a). Branch support was estimated by analyzing 100 bootstrapped datasets.
SNaPshot Assay
The species identity of all specimens (with the exception of one individual from Inkoo, KS.3026) was verified using diagnostic SNPs, which can confidently assign the specimens to respective species (Momigliano et al., 2017a). We selected six loci (Table 2) which were identified as under selection in the two flounder spawning types by Momigliano et al. (2017a), and which show very different allele frequencies in the two putative species. We used the dataset from Momigliano et al. (2017a) to determine whether a smaller subset of these loci was sufficient to unambiguously assign unknown individuals to the correct species.
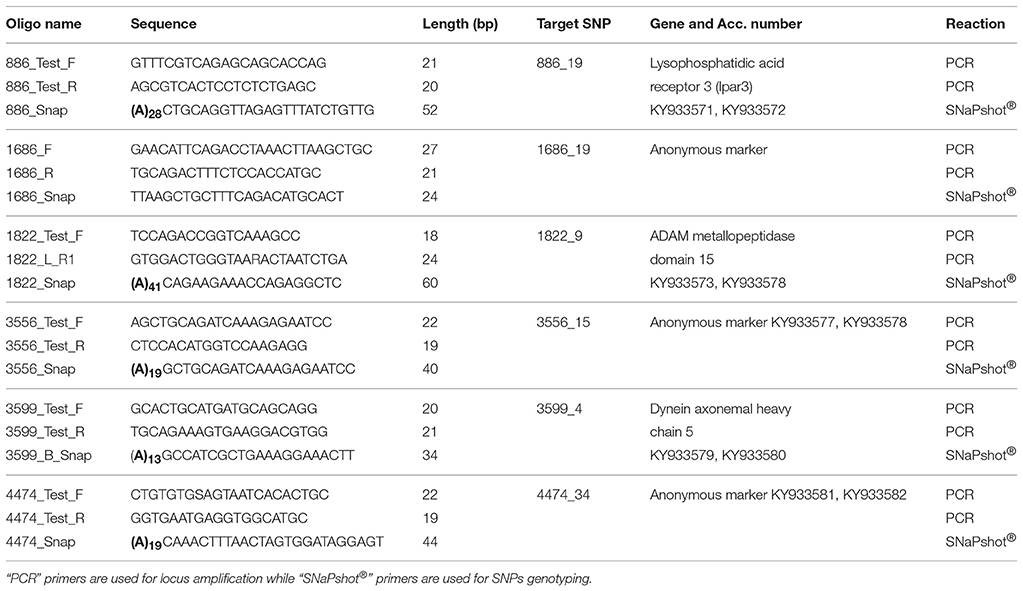
Table 2. List of oligonucleotides used in the multiplex SNaPshot® assay, as well as targeted SNPs as per Momigliano et al. (2017a) and their corresponding target genes and GenBank accession (Acc.) numbers for both alleles.
For all the samples included in this study, the six loci were amplified via polymerase chain reaction (PCR), using the primers listed in Table 2, which were designed based on the GenBank sequences of the genomic regions flanking the loci deposited by Momigliano et al. (2017a), accession nos. KY933571–KY933582. All loci were amplified simultaneously in a multiplex PCR, including 1 μl of diluted purified DNA (~20 ng), 2 μM each primer, 1× Phusion taq MasterMix in a total 10 μl reaction. PCR conditions were as follows: an initial denaturation at 98°C (30 s), followed by 35 cycles of 98°C for 5 s, 59°C for 10 s, 72°C extension for 10 s, and a final extension of 72°C for 2 min. The SNPs were then genotyped using a SNaPshot Assay, with primers listed in Table 2, following manufacturers' instructions. Alleles were scored using the software GeneMapper® (Applied Biosystems).
Assignment Tests
The individuals were assigned to putative species of origin using a Discriminant Analyses of Principal Components (DAPC; Jombart et al., 2010) performed using the R package adegenet (Jombart, 2008) on both the 2b-RAD dataset and the dataset composed of the six outlier loci. For the 2b-RAD dataset, we used the 11 demersal flounders from Öland and the nine demersal flounders from Bornholm as reference samples, while for the SNaPshot dataset we used the genotypes from Momigliano et al. (2017a) as reference samples (Momigliano et al., 2017b); the individuals from this study where then assigned to population of origin using the function predict.sup. We carried out the same analyses using the 2b-RAD data (5861 loci), the six outlier loci, and a subset of three loci (3599, 1822, and 886), and two loci (3599 and 886) to determine the minimum number of SNPs necessary to unambiguously identify individuals to species level. Reference data as well as all genotypes from this study are deposited in the Dryad digital repository (doi: 10.5061/dryad.8vr1001).
Egg Buoyancy and Sperm Motility Trials
For individuals that were ready to spawn at the time of collection, we carried out egg buoyancy as well as sperm motility tests at different salinities. Seawater of different salinities (6–28 psu) was prepared by adding Instant Ocean® Sea Salt (Aquarium Systems, Sarrebourg—France) to sterile filtered seawater, which at the site of research (Tvärminne) has a salinity of ~6 psu. To prepare water at salinity of 2 and 4 psu, sterile filtered seawater was diluted with deionized water.
Eggs were stripped from the only mature female (KS.3052), and small amounts of eggs were placed in 5 ml vials containing sterile seawater at salinities ranging from 8 to 28 psu. After 10 min, we recorded whether eggs had positive or negative buoyancy, and fixed them in 5% Acidic Lugol's solution. Fixed eggs were viewed using a stereo microscope (Nikon SM745T), photographed using a Nikon DS-fi1 imaging system, and their diameter measured by taking two perpendicular measurements for each egg. A drop of sperm (50 μl) was collected from each male with running milt (N = 8) and added to 2 ml of filtered sterile seawater at salinities ranging from 2 to 12 psu, at 2 psu intervals. The sperm was immediately visualized at 200× magnification under an inverted microscope in a climate controlled room at 8°C. Sperm motility was calculated as the time until which >90% of the spermatozoa became immotile or vibrating.
Morphological Analyses
Counts and measurements (see below) were taken from the eye side. All measurements were made point to point, never by projection, using an electronic caliper and are expressed to the nearest tenth of a millimeter. Maximal body depth was measured. Vertebrae as well as fin rays counts from each specimen were obtained from X-ray pictures taken with an Indico 100® X-ray machine (Medical Imaging Systems, Inc.) at the University of Helsinki's Veterinary Hospital. Bony plates on the eye side were observed under black light (Figure 2), and each individual was assigned to one of the five morphotypes identified by Voronina (1999): (1) body fully covered, (2) dorsal and anal parts less covered, (3) only along the lateral line and on the head, (4) only along the lateral line, (5) bony plates on lateral line not reaching the pectoral fin (Figure 2). Sex was only recorded for individuals which were ready to spawn at the time of sampling. A total of 28 morphomeristic characters were used.
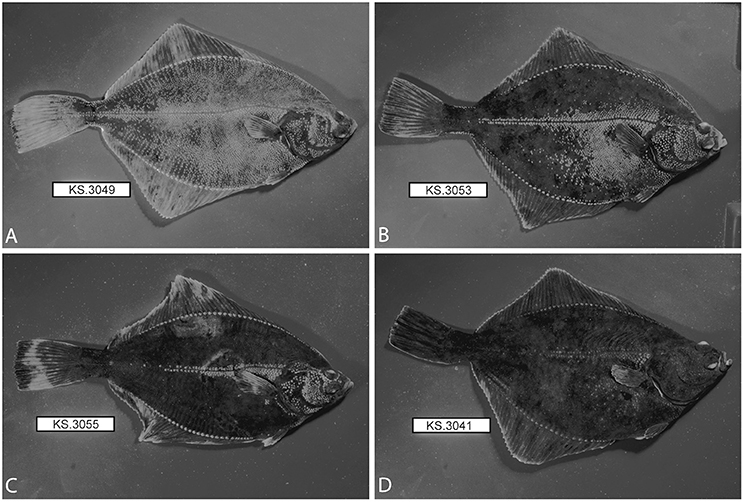
Figure 2. UV photographs showing variation in bony scales, representing phenotypes 1–4 sensu Voronina (1999). Body fully plated (A, phenotype 1): P. flesus, KS.3049, 228.2 mm SL, western Gulf of Finland, Tvärminne, Rävskär-Brännskär, 59° 51′ N 23° 16′ E, 7th June 2017, coll. P. Momigliano and H. Jokinen; less plates on the dorsal and anal parts (B, phenotype 2): P. solemdali sp. nov., KS.3053, 266.9 mm SL, western Gulf of Finland, Tvärminne, Furuskär (island), northern side, 59° 50′ N 23° 16′ E, 7th June 2017, coll. P. Momigliano and H. Jokinen; bony plates along the lateral line and on the head (C, phenotype 3): P. solemdali sp. nov., KS.3055, 193.6 mm SL, western Gulf of Finland, Tvärminne, Sundholmen (island), southern side, 59° 50′ N 23° 15′ E, 7th June 2017, coll. P. Momigliano and H. Jokinen; and few plates present along the lateral line and spine (D, phenotype 4): P. solemdali sp. nov., paratype, KS.3041, 230.8 mm SL, western Gulf of Finland, Tvärminne, Furuskär (island), northern side, 59° 50′ N 23° 15′ E, 16th May 2017, coll. P. Momigliano and H. Jokinen.
Comparative Material
Platichthys flesus (22 specimens): syntype BMNH 1853.11.12.132, 143 mm, right form specimen, ocular side; syntype BMNH 1853.11.12.133, 139 mm, left form specimen, ocular side; syntype BMNH 1853.11.12.134, 152 mm, right form specimen, blind side; KS.3020, 242.9 mm SL, right form specimen, south-western Baltic, Rostock, 54° 11′ N 12° 01′ E, December 2016, coll. S. Hille; KS.3021, 279 mm SL, right form specimen, south western Baltic, Rostock, 54° 11′ N 12° 01′ E, December 2016, coll. S. Hille; KS.3022, 266 mm SL, right form specimen, south-western Baltic, Rostock, Germany, 54° 11′ N 12° 01′ E, December 2016, coll. S. Hille; KS.3038, 197.4 mm SL, right form specimen, western Gulf of Finland, Inkoo, 60° 02′ N 24° 05′ E, 28th February–3rd March 2017, coll. U. Wasström; KS.3049, 228.2 mm SL, right form specimen, western Gulf of Finland, Tvärminne, Rävskär-Brännskär, 59° 51′ N 23° 16′ E, 7th June 2017, coll. P. Momigliano and H. Jokinen; KS.3061, 240.0 mm SL, right form specimen, central Baltic, Lausvik, Gotland, 57° 18′ N 18° 42′ E, 22nd June 2017, coll. O. Nordahl; KS.3067, 272.9 mm SL, right form specimen, central Baltic, Lausvik, Gotland, 57° 18′ N 18° 42′ E, 22nd June 2017, coll. O. Nordahl; KS.3076, 236.9 mm SL, right form specimen, south-western Baltic, Rostock, 54° 11′ N 12° 01′ E, 7th July 2017, coll. S. Hille; KS.3077, 245.7 mm SL, right form specimen, south-western Baltic, Rostock, 54° 11′ N 12° 01′ E, 7th July 2017, coll. S. Hille; KS.3078, 264.3 mm SL, right form specimen, south-western Baltic, Rostock, 54° 11′ N 12° 01′ E, 7th July 2017, coll. S. Hille; KS.3079, 248.2 mm SL, right form specimen, south-western Baltic, Rostock, 54° 11′ N 12° 01′ E, 7th July 2017, coll. S. Hille; KS.3080, 244.7 mm SL, left form specimen, south-western Baltic, Rostock, 54° 11′ N 12° 01′ E, 7th July 2017, coll. S. Hille; KS.3081, 273.6 mm SL, right form specimen, south-western Baltic, Rostock, 54° 11′ N 12° 01′ E, 7th July 2017, coll. S. Hille; KS.3082, 272.7 mm SL, right form specimen, south-western Baltic, Rostock, 54° 11′ N 12° 01′ E, 7th July 2017, coll. S. Hille; KS.3083, 253.6 mm SL, right form specimen, south-western Baltic, Rostock, 54° 11′ N 12° 01′ E, 7th July 2017, coll. S. Hille; KS.3084, 237.3 mm SL, left form specimen, south-western Baltic, Rostock, 54° 11′ N 12° 01′ E, 7th July 2017, coll. S. Hille; KS.3085, 273.7mm SL, right form specimen, south-western Baltic, Rostock, 54° 11′ N 12° 01′ E, 7th July 2017, coll. S. Hille; KS.3086, 244.4 mm SL, left form specimen, south-western Baltic, Rostock, 54° 11′ N 12° 01′ E, 7th July 2017, coll. S. Hille; KS.3087, 242.2 mm SL, left form specimen, south-western Baltic, Rostock, 54° 11′ N 12° 01′ E, 7th July 2017, coll. S. Hille.
Morphological data of genotyped specimens were compared with the diagnosis of the syntype specimens of Pleuronectes flesus Linnaeus 1758 (BMNH 1853.11.12.132–134) (Wheeler, 1958) in order to affiliate the latter ones either to the demersal or the pelagic lineage. Data from North Sea, Skagerrak Strait, and the Atlantic Ocean specimens from Voronina (1999) as well as data from Britain, the English channel, and the Danish Belt Sea from Galleguillos and Ward (1982) were used too for comparison. The specimens NRM 20 and ZIU 191 designated as a potential syntype by Kottelat (1997) were not integrated because of their doubtful type status (Fernholm and Wheeler, 1983; Wheeler, 1991). We also used the description of Platichthys stellatus (Pallas 1787) from Morrow (1980) in order to compare it with our dataset. In order to morphologically discriminate among pelagic and demersal flounders, as well as to compare them to type material meristic traits and measurements (see above), we conducted Principal Component Analyses (PCA) in the R statistical environment (R Core Team, 2017).
Results
Genetic Analyses
A total of 46 libraries were sequenced: 40 individuals as well as six technical replicates. An average of 4.24 million reads per library and a total of ~195 million reads were obtained. After removing PCR duplicates and quality filtering, these figures were 2.1 and 96 million reads, respectively. The pipeline identified 125 238 loci, with an average coverage across samples of 17×. After quality filtering, a total of 5861 bi-allelic SNPs with less than 20% missing data were retained.
A maximum likelihood tree generated from the SNP matrix identified two well-supported clades (Figure 3), with results nearly identical to Momigliano et al. (2017a). A DAPC analysis based on the 2b-RAD data was able to assign individuals to the two clades with very high certainty (i.e., the lowest assignment probability = 99.9999%), as expected from a large genomic dataset. However, genotyping as few as three outlier loci was sufficient to assign every individual to species with accuracy and precision (the lowest assignment probability = 99.998%), and even screening just two outlier loci was sufficient to unambiguously assign all individuals but one to the correct species (Figure 4).
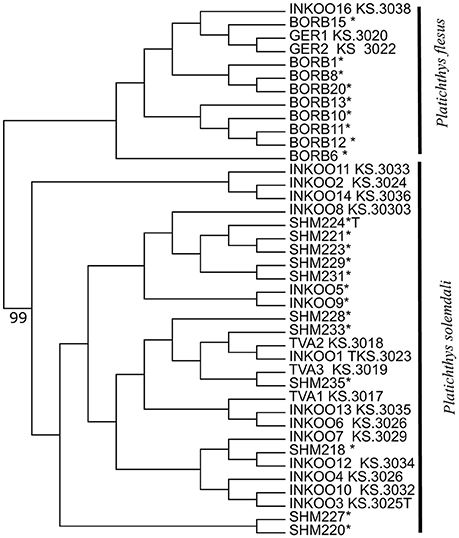
Figure 3. Mid-point rooted Maximum Likelihood phylogenetic tree based on the alignment of 5861 SNPs obtained from 2b-RAD libraries. Reference samples from both species from Momigliano et al. (2017a) are marked with asterisks. Node label indicates bootstrap support (out of 100 replicates), and is reported for the only node (the one separating the two species) that is supported by over 75% of bootstrap replicates.
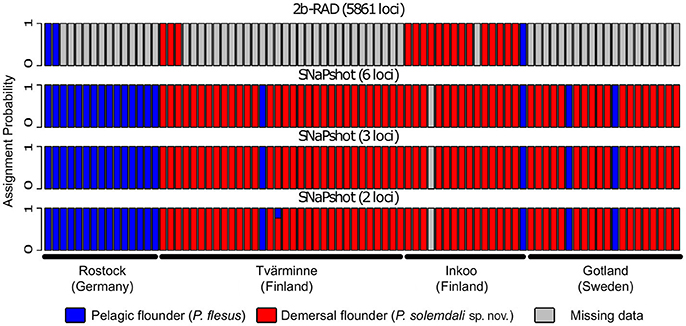
Figure 4. Results from assignment tests performed on the specimens deposited in the Finnish Natural History Museum. The individual specimens were assigned to species with a Discriminant Analyses of Principal Components (DAPC) using individuals of the two known species from Momigliano et al. (2017a) as reference samples. The analyses were carried out using genome wide SNPs obtained from 2b-RAD libraries, as well as with six, three, and two loci under divergent selection in the two species. Each bar represents an individual, and the y-axis represents assignment probabilities to P. flesus (blue) or P. solemdali sp. nov. (red). Missing data are shown in gray. As little as three outlier loci were sufficient to unambiguously assign each individual to correct species.
Morphological Analyses
Sixty-nine new specimens were included in the analyses. All of the newly collected samples used in morphological analyses were identified to species level (19 pelagic and 50 demersal flounders), either using 2b-RAD data (20 individuals genotyped) or the set of SNPs screened. Meristic characters were counted for all 69 new specimens and were available also for the syntypes, and for 67 specimens all morphometric measurements were recorded (two, KS.3041 and KS.3042, had been fixed in a position that prevented appropriate measurement of standard length). Syntypes of P. flesus were integrated only in the meristic analysis. PCA analyses using all the individuals obtained in this study and all the morphometric data documented show some slight differences (Figure S1) between demersal and pelagic flounders, yet do not separate distinctly both lineages due to overlap in all of the included characters. A multivariate analysis on meristic characters shows slight differences between pelagic and demersal lineages (Figure 5A). The two principal characters highlighting differences are the numbers of dorsal and anal fin rays (see inset in Figure 5A). The demersal form is distinct from the pelagic one by tending to have less dorsal and anal fin rays (Table 3; Figure 5B). The syntype specimens of P. flesus (BMNH 1853.11.12.132–134) have, respectively, 54, 62, and 61 dorsal fin rays, and 41, 43, and 42 anal fin rays (Figures 5B,C). Hence, they are more similar to the pelagic than to the demersal group, except for one specimen (BMNH 1853.11.12.132) which cannot be unambiguously assigned to either group.
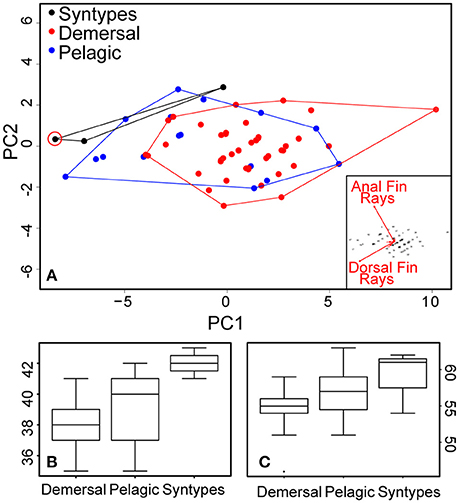
Figure 5. Results from the meristic analyses. (A) PCA based on fin rays count data; the biplot in the inset shows that the variables with the highest loadings are anal fin rays and dorsal fin rays counts. The newly designated lectotype of P. flesus is circled in red. (B) boxplot of anal fin ray count data for demersal and pelagic flounders as well as P. flesus syntypes. (C) boxplot of dorsal fin rays count data for demersal and pelagic flounders as well as P. flesus syntypes.
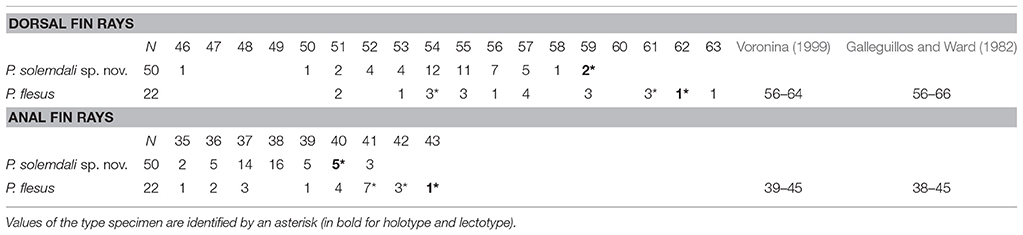
Table 3. Dorsal and anal fin rays counts of 50 specimens of Platichthys solemdali sp. nov., 19 specimens of Platichthys flesus from this study as well as the lectotype BMNH 1853.11.12.133 and paralectotypes BMNH 1853.11.12.132–134, the results of Voronina (1999) on the specimens from North Sea, Skagerrak Strait and Atlantic Ocean off Portugal, and the results from Galleguillos and Ward (1982) from Britain, the English channel and the Danish Belt Sea.
Systematic Account
The present article in portable document (.pdf) format is a published work in the sense of the International Code of Zoological Nomenclature (International Commission on Zoological Nomenclature 1999) and the new names contained herein are effectively published under the Code. The Zoobank numbers for the article and for the new taxon herein described are DC659778-90A7-4E2C-AEDE-04D5D82488E9 and 05645402-62B0-4E96-97B5-8B9D4FD566F7, respectively.
Family Pleuronectidae Rafinesque 1815
Genus Platichthys Girard 1854
Platichthys flesus (Linnaeus, 1758)
Common name: European flounder
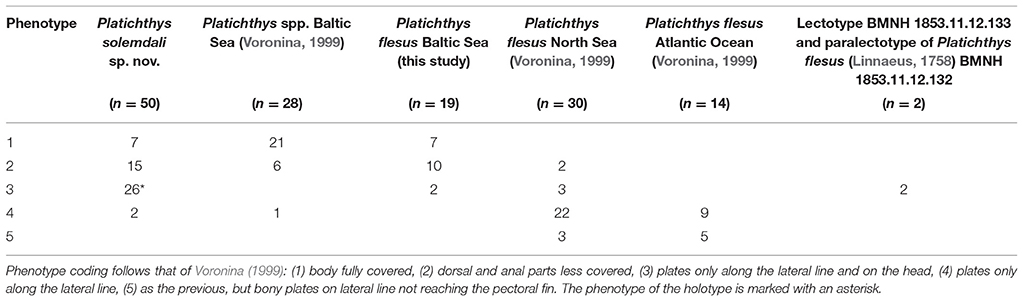
Table 5. Bony plate phenotypes observed on Platichthys solemdali sp. nov., the lectotype BMNH 1853.11.12.133, and paralectotype of Platichthys flesus (Linnaeus, 1758) BMNH 1853.11.12.132 and the results of Voronina (1999) on the specimens from North Sea, Atlantic Ocean off Portugal, and Baltic Sea.
Pleuronectes flesus Linnaeus, 1758 (type locality: European seas); Pleuronectes passer Linnaeus, 1758 (type locality: European ocean); Pleuronectes flesoides Pontoppidan, 1766 (type locality: Denmark); Pleuronectes roseus Shaw, 1796 (type locality: river Thames, United Kingdom); Platessa carnaria Brown, 1830 (type locality: Prestonpans, Scotland, United-Kingdom); Pleuronectes italicus Günther, 1862 (type locality: Dalmatia, Croatia); Flesus vulgaris Moreau, 1881 (type locality: French Atlantic and Channel coasts); Pleuronectes flesus var. trachurus Duncker, 1892 (type locality: Baltic Sea at the mouth of river Trave, Germany); Pleuronectes flesus var. leiurus Duncker, 1892 (type locality: Elbe river at Hamburg, Germany); Pleuronectes Bogdanovii Sandeberg, 1878 (type locality: White Sea); Pleuronectes flesus race septentrionalis Suvorov, 1925 (type locality: Kola Bay, Russia); Pleuronectes flesus caninensis Suvorov, 1929 (type locality: rivers discharging to Chosha Bay, Arctic Ocean, Russia); Platichthys flesus trachurus natio baltica infranatio pelagicus Mikelsaar, 1984 (type locality: from Gotland to Hiiumaa, Baltic Sea).
Lectotype Designation
The original description of the European flounder was done by Linnaeus (1758: 270) based on five earlier descriptions (Artedi, 1738: 17, 59; Linnaeus, 1746: 112; Linnaeus, 1751: 326; Gronovius, 1754: 15). In this description, Linnaeus (1758) gives the diagnoses of four specimens, and three of them are known because they belonged to Gronovius' collections and are now deposited in the Natural History Museum in London, United-Kingdom (BMNH 1853.11.12.132–134) (Wheeler, 1958). The fourth specimen belonging to the type series and diagnosed “D.55, P.11, V.6, A.40, C.14” is still unknown. Kottelat (1997) mentioned two potential syntypes but their type status is doubtful. The first one is NRM 20 (deposited in the Naturhistoriska Riksmuseet in Stockholm, Sweden), whose diagnosis (D.62, P.10, V.6, A.46, C-) does not correspond to Linnaeus' description (Fernholm and Wheeler, 1983). The second one, ZIU 191 (deposited in the Uppsala University, Zoological Museum at Uppsala, Sweden), is not certain to have been consulted by Linnaeus and there is no comment concerning its type status (Wheeler, 1991).
The meristic analysis of genotyped specimens found slight differences between demersal and pelagic lineages on the number of dorsal [respectively, 50–59 vs. 51–63; Figure 5C], and anal fin rays (35–41 vs. 35–42; Figure 5B). The three syntype specimens with respectively 54, 62, and 61 dorsal fin rays, and 41, 43, and 42 anal fin rays (Figure 5A) are more similar to the individuals from the pelagic lineage and distinct from the individuals of the demersal group, except for one specimen (BMNH 1853.11.12.132), which cannot be assigned to either group. Considering this result, a lectotype designation is needed. No illustration was given in the description of Linnaeus (1758) nor in the earlier ones (Artedi, 1738; Linnaeus, 1746, 1751; Gronovius, 1754). Thus, we designate the specimen BMNH 1853.11.12.133 (143 mm, left form specimen, ocular side) as the lectotype of Platichthys flesus (Linnaeus, 1758). The two other specimen BMNH 1853.11.12.132 (139 mm, right form specimen, ocular side) and BMNH 1853.11.12.134 (152 mm, right form specimen, blind side) are the paralectotypes of this species.
Systematic notes
The lectotype specimen of P. flesus, with 62 dorsal fin rays and 43 anal fin rays, is more similar to individuals from the pelagic lineage and distinct from individuals from the demersal lineage. Therefore, we conclude that the pelagic lineage belongs to Platichthys flesus sensu stricto. Available data suggest that P. flesus is more common in the southern Baltic Sea (Figure 1B; Momigliano et al., 2017a; this study) and occurs alone in the rest of its distribution range outside of the Baltic Sea. The demersal lineage is only found within the Baltic Sea and is more common in the northern Baltic Proper and the Gulf of Finland (Figure 1B). Both lineages can occur in the central Baltic Sea, and P. flesus is also encountered in the northern Baltic Sea and the Gulf of Finland, where demersal flounders dominate the local assemblage (Figure 1B, Momigliano et al., 2017a; this study).
Some flatfish taxa have already been described in the Baltic Sea: Pleuronectes flesoides Pontoppidan, 1766 and Pleuronectes flesus var. trachurus Duncker, 1892, whose type localities are, respectively, Denmark and the mouth of river Trave in Germany—both in the southern Baltic Sea. Mikelsaar (1984) additionally named the pelagic flounders of the Baltic Sea as Platichthys flesus trachurus natio baltica infranatio pelagicus. All these names are junior synonyms of Platichthys flesus (Linnaeus, 1758). The demersal flounders have been earlier assigned to Pleuronectes flesus race baltica Suvorov, 1925, Pleuronectes flesus trachurus natio baltica Suvorov, 1927 or Platichthys flesus trachurus natio baltica infranatio sublitoralis Mikelsaar, 1984, but all these names are infrasubspecific and therefore not valid.
Platichthys solemdali sp. nov.
2. Suggested common name: Baltic flounder
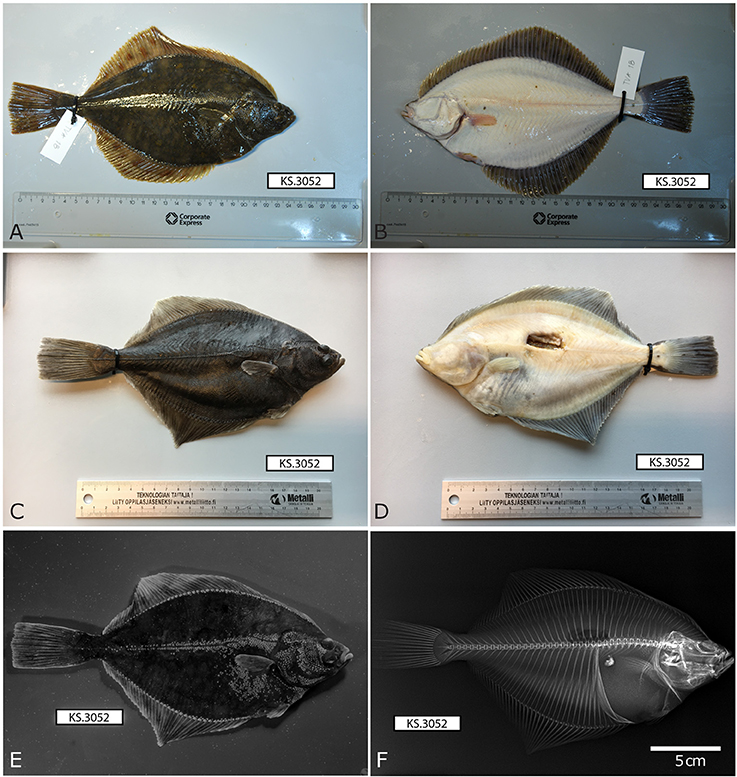
Figure 6. Photographic images of the holotype of Platichthys solemdali sp. nov. KS.3052, adult female, 213.8 mm SL, right form specimen, western Gulf of Finland, Tvärminne, Furuskär (island), northern side, 59° 50′ N 23° 15′ E, 7th June 2017, coll. P. Momigliano and H. Jokinen. (A) Dorsal view before fixation, (B) ventral view before fixation, (C) dorsal view after fixation, (D) ventral view after fixation, (E) photograph under UV light showing bony plates, and (F) X-ray image.
Pleuronectes flesus race baltica Suvorov, 1925 (infrasubspecific, name not available, type locality: Baltic Sea); Pleuronectes flesus trachurus natio baltica Suvorov, 1927 (infrasubspecific, name not available, type locality: Baltic Sea); Platichthys flesus trachurus natio baltica infranatio sublitoralis Mikelsaar, 1984 (infrasubspecific, name not available, type locality: Gulf of Finland, Baltic Sea).
Holotype
KS.3052 deposited in the Finnish Museum of Natural History (MZF, Helsinki, Finland, 11/04/2018), adult female, 213.8 mm SL, right form specimen, western Gulf of Finland, Tvärminne, Furuskär (island), northern side, 59° 50′ N 23° 15′ E, 7th June 2017, coll. Paolo Momigliano and Henri Jokinen.
Paratypes
(11 specimens): KS.3040, adult male, 250.5 mm SL, right form specimen, western Gulf of Finland, Tvärminne, Furuskär (island), northern side, 59° 50′ N 23° 15′ E, 16th May 2017, coll. P. Momigliano and H. Jokinen; KS.3041, adult female, 230.8 mm SL, right form specimen, western Gulf of Finland, Tvärminne, Furuskär (island), northern side, 59° 50′ N 23° 15′ E, 16th May 2017, coll. P. Momigliano and H. Jokinen; KS.3043, adult male, 184.4 mm SL, left form specimen, western Gulf of Finland, Tvärminne, Storfjärden (coastal inlet), 59° 51′ N 23° 15′ E, 7th June 2017, coll. P. Momigliano and H. Jokinen; KS.3044, adult male, 203.2 mm SL, left form specimen, western Gulf of Finland, Tvärminne, Rävskär (island), northwestern side, 59° 51′ N 23° 16′ E, 7th June 2017, coll. P. Momigliano and H. Jokinen; KS.3045, adult male, 169.2 mm SL, right form specimen, western Gulf of Finland, Tvärminne, Rävskär-Brännskär (strait), 59° 51′ N 23° 16′ E, 7th June 2017, coll. P. Momigliano and H. Jokinen; KS.3046, adult male, 180.2 mm SL, right form specimen, western Gulf of Finland, Tvärminne, Rävskär-Brännskär (strait), 59° 51′ N 23° 16′ E, 7th June 2017, coll. P. Momigliano, and H. Jokinen; KS.3047, adult male, 200.7 mm SL, right form specimen, western Gulf of Finland, Tvärminne, Rävskär-Brännskär (strait), 59° 51′ N 23° 16′ E, 7th June 2017, coll. P. Momigliano and H. Jokinen; KS.3051, adult male, 206 mm SL, right form specimen, western Gulf of Finland, Tvärminne, Furuskär (island), northern side, 59° 50′ N 23° 15′ E, 7th June 2017, coll. P. Momigliano and H. Jokinen; KS.3053, adult male, 266.9 mm SL, right from specimen, western Gulf of Finland, Tvärminne, Furuskär (island), northern side, 59° 50′ N 23° 16′ E, 7th June 2017, coll. P. Momigliano and H. Jokinen; KS.3054, adult male, 220.9 mm SL, right form specimen, western Gulf of Finland, Tvärminne, Sundholmen (island), southern side, 59° 50′ N 23° 15′ E, 7th June 2017, coll. P. Momigliano and H. Jokinen; KS.3055, adult male, 193.6 mm SL, right form specimen, western Gulf of Finland, Tvärminne, Sundholmen (island) southern side, 59° 50′ N 23° 15′ E, 7th June 2017, coll. P. Momigliano and H. Jokinen.
Non-type material
(38 specimens): KS.3017, 207.2 mm SL, right form specimen, western Gulf of Finland, Tvärminne, 59° 51′ N 23° 14′ E, 5th February 2017, coll. T. Sjölund; KS.3018, 195 mm SL, right form specimen, western Gulf of Finland, Tvärminne, 59° 51′ N 23° 14′ E, 5th February 2017, coll. T. Sjölund; KS.3019, 210.4 mm SL, left form specimen, western Gulf of Finland, Raseborg, 59° 46′ N 23° 23′ E, 4th February 2017, coll. T. Sjölund; KS.3023, 190.7 mm SL, right form specimen, western Gulf of Finland, Inkoo, 60° 02′ N 24° 05′ E, 30th January−13th February 2017, coll. U. Wasström; KS.3024, 199.2 mm SL, right form specimen, western Gulf of Finland, Inkoo, 60° 02′ N 24° 05′ E, 30th January−13th February 2017, coll. U. Wasström; KS.3025, 211.8 mm SL, right form specimen, western Gulf of Finland, Inkoo, 60° 02′ N 24° 05′ E, 30th January−13th February 2017, coll. U. Wasström; KS.3026, 231.7 mm SL, right form specimen, western Gulf of Finland, Inkoo, 60° 02′ N 24° 05′ E, 30th January−13th February 2017, coll. U. Wasström; KS.3027, 238.8 mm SD, right form specimen, western Gulf of Finland, Inkoo, 60° 02′ N 24° 05′ E, 30th January−13th February 2017, coll. U. Wasström; KS.3028, 236.3 mm SL, right form specimen, western Gulf of Finland, Inkoo, 60° 02′ N 24° 05′ E, 30th January−13th February 2017, coll. U. Wasström; KS.3029, 185.5 mm SL, right form specimen, western Gulf of Finland, Inkoo, 60° 02′ N 24° 05′ E, 30th January−13th February 2017, coll. U. Wasström; KS.3030, 186.6 mm SL, right form specimen, western Gulf of Finland, Inkoo, 60° 02′ N 24° 05′ E, 30th January−13th February 2017, coll. U. Wasström; KS.3031, 196.3 mm SL, left form specimen, western Gulf of Finland, Inkoo, 60° 02′ N 24° 05′ E, 28th February−3rd March 2017, coll. U. Wasström; KS.3032, 274.4 mm SL, right form specimen, western Gulf of Finland, Inkoo, 60° 02′ N 24° 05′ E, 28th February−3rd March 2017, coll. U. Wasström; KS.3033, 234.3 mm SL, right form specimen, western Gulf of Finland, Inkoo, 60° 02′ N 24° 05′ E, 28th February−3rd March 2017, coll. U. Wasström; KS.3034, 204.9 mm SL, right form specimen, western Gulf of Finland, Inkoo, 60° 02′ N 24° 05′ E, 28th February−3rd March 2017, coll. U. Wasström; KS.3035, 279.8 mm SL, right form specimen, western Gulf of Finland, Inkoo, 60° 02′ N 24° 05′ E, 28th February−3rd March 2017, coll. U. Wasström; KS.3036, 253.0 mm SL, right form specimen, western Gulf of Finland, Inkoo, 60° 02′ N 24° 05′ E, 28th February−3rd March 2017, coll. U. Wasström; KS.3037, 262.3 mm SL, right form specimen, western Gulf of Finland, Inkoo, 60° 02′ N 24° 05′ E, 28th February−3rd March 2017, coll. U. Wasström; KS.3039, male, no SL available, right form specimen, western Gulf of Finland, Tvärminne, 59° 50′ N 23° 16′ E, 16th May 2017, coll. P. Momigliano and H. Jokinen; KS.3042, no SL available, right form specimen, western Gulf of Finland, Tvärminne, 59° 50′ N 23° 16′ E, 16th May 2017, coll. P. Momigliano and H. Jokinen; KS.3056, 225.0 mm SL, right form specimen, central Baltic, Gotland, Lausvik, 57° 18′ N 18° 42′ E, 22nd June 2017, coll. O. Nordahl; KS.3057, 253.4 mm SL, right form specimen, central Baltic, Gotland, Lausvik, 57° 18′ N 18° 42′ E, 22nd June 2017, coll. O. Nordahl; KS.3058, 233.3 mm SL, right form specimen, central Baltic, Gotland, Lausvik, 57° 18′ N 18° 42′ E, 22nd June 2017, coll. O. Nordahl; KS.3059, 228.7 mm SL, right form specimen, central Baltic, Gotland, Lausvik, 57° 18′ N 18° 42′ E, 22nd June 2017, coll. O. Nordahl; KS.3060, 215.7 mm SL, left form specimen, central Baltic, Gotland, Lausvik, 57° 18′ N 18° 42′ E, 22nd June 2017, coll. O. Nordahl; KS.3062, 258.0 mm SL, right form specimen, central Baltic, Gotland, Lausvik, 57° 18′ N 18° 42′ E, 22nd June 2017, coll. O. Nordahl; KS.3063, 236.6 mm SL, left form specimen, central Baltic, Gotland, Lausvik, 57° 18′ N 18° 42′ E, 22nd June 2017, coll. O. coll. Nordahl; KS.3064, 235.0 mm, right form specimen, central Baltic, Gotland, Lausvik, 57° 18′ N 18° 42′ E, 22nd June 2017, coll. O. Nordahl; KS.3065, 219.4 mm, right form specimen, central Baltic, Gotland, Lausvik, 57° 18′ N 18° 42′ E, 22nd June 2017, coll. O. Nordahl; KS.3066, 208.5 mm SL, right form specimen, central Baltic, Gotland, Lausvik, 57° 18′ N 18° 42′ E, 22nd June 2017, coll. O. Nordahl; KS.3068, 252.8 mm SL, right form specimen, central Baltic, Gotland, Lausvik, 57° 18′ N 18° 42′ E, 22nd June 2017, coll. O. Nordahl; KS.3069, 261.4 mm SL, right form specimen, central Baltic, Gotland, Lausvik, 57° 18′ N 18° 42′ E, 22nd June 2017, coll. O. Nordahl; KS.3070, 245.9 mm SL, right form specimen, central Baltic, Gotland, Lausvik, 57° 18′ N 18° 42′ E, 22nd June 2017, coll. O. Nordahl; KS.3071, 241.8 mm SL, right form specimen, central Baltic, Gotland, Lausvik, 57° 18′ N 18° 42′ E, 22nd June 2017, coll. O. Nordahl; KS.3072, 248.2 mm SL, right form specimen, central Baltic, Gotland, Lausvik, 57° 18′ N 18° 42′ E, 22nd June 2017, coll. O. Nordahl; KS.3073, 237.6 mm SL, right form specimen, central Baltic, Gotland, Lausvik, 57° 18′ N 18° 42′ E, 22nd June 2017, coll. O. Nordahl; KS.3074, 259.5 mm SL, right form specimen, central Baltic, Gotland, Lausvik, 57° 18′ N 18° 42′ E, 22nd June 2017, coll. O. Nordahl; KS.3075, 204.7 mm SL, left form specimen, central Baltic, Gotland, Lausvik, 57° 18′ N 18° 42′ E, 22nd June 2017, coll. O. Nordahl.
Diagnosis
Platichthys solemdali sp. nov. is diagnosable from P. stellatus by the absence of stripes on the dorsal and anal fin rays [Figures 6A, 2B; vs. presence of stripes for P. stellatus (Morrow, 1980)]. It can be distinguished with more than 99.999% certainty from P. flesus using genotypes of at least three of the outlier loci which were genotyped in this study (Loci 886, 3599, and 1822) by comparison with publically available reference data deposited in the Dryad digital repository (Momigliano et al., 2017a). P. solemdali sp. nov. (N = 50) has 46–59 dorsal fin rays vs. 51–66 for P. flesus recorded in this study, in Voronina (1999) and in Galleguillos and Ward (1982), and 35–41 anal fin rays vs. 35–45 in P. flesus from this study, Voronina (1999) and Galleguillos and Ward (1982). Hence, none of these meristic characters provide unambiguous species diagnosis. However, reproductive traits (viz. egg morphology and buoyancy, as well as sperm physiology) are unambiguous diagnostic characters. Eggs of P. solemdali sp. nov. become neutrally buoyant at salinities between 16 and 21.5 psu and are 0.99 ± 0.05 mm in diameter (Table 6; Figure 7), whereas the eggs of P. flesus in the Baltic Sea are larger (1.3–1.5 mm) and reach neutral buoyancy between 11 and 18 psu (Table 6; Nissling et al., 2002). Spermatozoa of P. solemdali sp. nov. activate at minimum salinities between 2 and 4 psu, in contrast to a required salinity above 10 psu for P. flesus (Table 7).

Table 6. Mean (±SD) Salinity of Neutral Buoyancy (SNB) from P. flesus and P. solemdali sp. nov. from different locations of the Baltic Sea (identified by the International Council for the Exploration of the Sea Subdivisions-SD), data from Nissling et al. (2002), along with SNB of eggs from P. solemdali holotype.
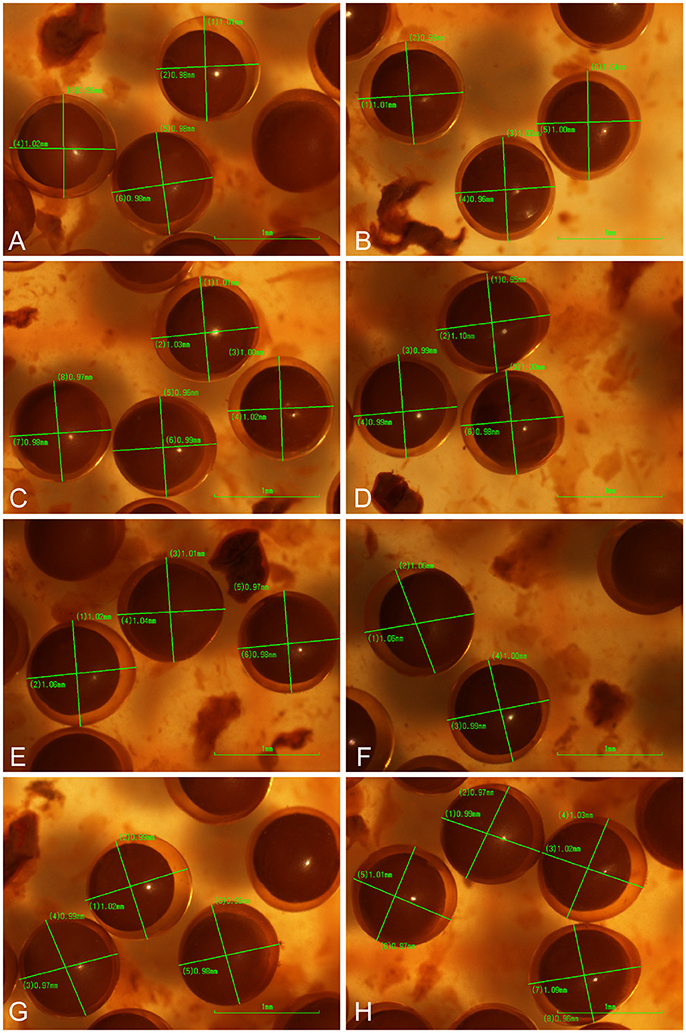
Figure 7. Photographs (A–H) of eggs collected from the holotype of Platichthys solemdali sp. nov. KS.3052 and fixed in 5% Acidic Lugol's solution. Fixed eggs were viewed using a stereo microscope (Nikon SM745T), photographed using a Nikon DS-fi1 imaging system and their diameter measured by taking two perpendicular measurements for each egg.
Description
Counts and measurements of meristic and morphometric traits are summarized in Tables 3–5.
Proportion of right- and left-eyed specimens
The right-eyed side form was predominant. Of the 50 specimens analyzed, four (8%) were a left-eyed form. Nevertheless, the proportion of left-eyed specimens may be variable in space and time. In the waters off Prangli Island (Estonia), which resides within the core distribution of the P. solemdali sp. nov., left-eyed flounders may constitute up to 50% of the individuals (Mikelsaar, 1984; Ojaveer and Drevs, 2003).
External morphology
(Tables 3, 4). The specimens belonging to P. solemdali sp. nov. (N = 50) have 46–59 dorsal fin rays (holotype 59), 35–41 anal fin rays (holotype 40), 8–12 pectoral fin rays (holotype 11) on the eye-side and 6 to 11 on the blind side (holotype 10), 5–6 pelvic fin rays (holotype 6) and 16–19 caudal fin rays (holotype 17). Concerning the morphometric data, the body depth measures 43.8–53.5% of the standard length SL (holotype 47.1% SL), the head length 25.7–31.9% SL (holotype 27.6% SL), the dorsal fin base length 76.8–85.6% SL (holotype 80.9% SL), the anal fin base length 50.9–59.4% SL (holotype 55.9% SL), the eye-side pectoral fin length 12.1–15.9% SL (holotype 14.0% SL), the blind side pectoral fin length 10.0–15.5% SL (holotype 12.0% SL), the pelvic fin length 7.7%−11.8% (holotype 9.3% SL), the caudal peduncle length 8.9–13.1% SL (holotype 11.1% SL), the caudal peduncle depth 8.1–11.0% SL (holotype 8.9% SL), the pre-dorsal fin length 5.9–11.4% SL (holotype 7.0% SL), the pre-anal fin length 30.7–45.1% SL (holotype 33.6% SL), the pre-pectoral fin length 24.2–32.7% SL (holotype 28.1% SL), the pre-pelvic fin length 20.0 to 30.0 % SL (holotype 21.0% SL), the snout length 15.8–27.6% of the head length HL (holotype 17.5% HL), the eye diameter 13.8–17.7% HL (holotype 16.1% HL), the interorbital width 0.0–7.4% HL (holotype 6.6% HL) and the post-orbital length 59.2–70.1% HL (holotype 67.6% HL).
Bony plates
(Figure 2 and Table 5). Of the 50 specimens belonging to P. solemdali sp. nov., seven had the body fully plated (phenotype 1), 15 had less plates on the dorsal and anal parts (phenotype 2), 26 (including the holotype) had bony plates along the lateral line and on the head only (phenotype 3), and two had only plates along the lateral line (phenotype 4). No specimen was observed with bony plates not reaching the pectoral fin (phenotype 5), whereas the P. flesus in the North Sea and Atlantic Ocean come with the phenotypes 2–5 (lectotype and paralectotype 3, but mainly 4; Voronina, 1999). Nevertheless, in the Baltic Sea where both species are sympatric, phenotype 1 dominates according to Voronina (1999). Thus, the extent of bony plating may associate with the environment. In our data, of the 19 P. flesus specimens collected from Germany (Rostock, N = 15), the central Baltic (Gotland, N = 2), and the Gulf of Finland (Tvärminne and Inkoo, N = 2), seven were fully plated (phenotype 1), 10 had less plates on the dorsal and anal parts (phenotype 2), and only two had bony plates just along the lateral line and on the head (phenotype 3). No P. flesus specimens exhibiting phenotype 4 and 5 sensu Voronina (1999) were observed. One specimen of P. solemdali sp. nov. (KS.3071) from Gotland had its blind side completely covered in bony plates, a phenotype never reported before.
Coloration in life
(Figures 6A,B). The eye side is dull to dark brown, gray and olive, with scattered reddish spots. Coloration is highly variable among individuals and the species can change its coloration according to the substratum providing effective camouflage, as is the case for P. flesus and some other flatfish species (Sumner, 1911; Ramachandran et al., 1996; Akkaynak et al., 2017). The blind side is usually white, with or without scattered brown patches of varying size.
Coloration after fixation
(Figures 6C,D). Eye side dark brown/gray, scattered spots not visible. Blind side white/pinkish.
Vertebral counts
10–12 pre-caudal vertebrae; 22–24 caudal vertebrae (holotype 11 and 23, Figure 6F); 33–35 vertebrae in total (holotype 34, Figure 6F).
Egg diameter and buoyancy
(Figure 7, Table 7). Egg diameter and buoyancy were measured using eggs stripped from the holotype (KS.3052). Mean egg diameter was 1.003 mm (SD = 0.024 mm). Neutral buoyancy was reached at 20–22 psu (negative buoyancy at 20, positive at 22). Both egg size and buoyancy from P. solemdali sp. nov. match the values reported by Nissling et al. (2002) for demersal flounders and are clearly different from the values reported by Nissling et al. (2002) for pelagic P. flesus in the Baltic Sea (Table 6).
Spermatozoa activation and motility, and fertilization success
(Table 7). Sperm activation and sperm motility trials were run for eight mature males. In two out of eight some limited sperm motility (less than 30 s) was already observed at 2 psu, while for the rest of the individuals sperm activation started at 4 psu. Sperm motility was very low at 4 psu (30 ± 15 s), but increased quickly when salinity was raised to 6 psu (153 ± 26 s), 8 psu (205 ± 73 s), 10 psu (244 ± 57 s), and was highest at 12 psu (293 ± 85 s). Previous studies also suggest that sperm from P. solemdali sp. nov. can activate in a salinity as low as 3–4 psu and achieve high motility already in a salinity of 6–7 psu (Nissling et al., 2002). Fertilization and successful development of egg require a salinity of ~6 psu (Nissling et al., 2002).
Geographic distribution
(Figure 1). P. solemdali sp. nov. is endemic to the Baltic Sea, where it has a wide distribution in coastal and bank areas across the region up to the Gulf of Finland and the southern Bothnian Sea. Confirmed individuals of P. solemdali sp. nov. have been sampled as far south as Öland (SD 27) (species identity confirmed via genetic analyses, Figure 1) and Hanö Bay (SD 25) (based on egg morphology, see Wallin, 2016; Nissling et al., 2017). In a recent paper Orio et al. (2017) suggested that environmental conditions in the entire southern Baltic Sea are suitable for demersal spawning flounders, and already Mielck and Künne (1935) reported ripe female flounders with small eggs from shallow low-saline (6–7‰) areas in the southern Baltic Sea (Oder Bank, SD 24). However, the current occurrence of P. solemdali sp. nov. in the southern regions is poorly known and, hence, it is still unclear whether the species is found throughout the coastal Baltic Sea area.
Habitat
P. solemdali sp. nov. lives in brackish water of varying salinities in the coastal zone at 0.5–50 m depth on soft and hard bottoms.
Biology and ecology
P. solemdali sp. nov. is a demersal fish adapted to the brackish conditions of the central and northern Baltic Sea. Adults reside in the archipelago and coastal areas throughout their lives undertaking only limited migrations between deeper wintering grounds and shallower spawning and feeding areas (e.g., Aro and Sjöblom, 1983). The Baltic flounder is a benthivore, feeding on various mussels and other benthic invertebrates from its post-settled juvenile stage onwards (Aarnio et al., 1996; Borg et al., 2014). Spawning occurs in April–June in nearshore areas on a 5 to 20 m depth with a preferable water temperature of around 8°C (Sandman, 1906; Bonsdorff and Norkko, 1994; Nissling and Dahlman, 2010). Mature females lay up to 2 million relatively small eggs that after fertilization develop on the bottom (Nissling et al., 2002; Nissling and Dahlman, 2010) above stony, sandy, or vegetated substrates (Sandman, 1906; Bonsdorff and Norkko, 1994). Little is known about the larval phase of P. solemdali. In terms of early development and juvenile habitat use, the life-history generally resembles the common features of flatfishes (Gibson et al., 2015). There are no studies reporting specifically age and length at maturity for demersal Baltic flounder. However, around Gotland in the central Baltic Sea (SD 28) where both P. flesus and P. solemdali sp. nov. co-occur, female flounders recruit to the adult population and attain maturity around the age of 3 years (range: 2–5) at a length of 20–25 cm (Erlandsson et al., 2017), with males often maturing earlier (2–3 years) than females (3–4 years).
Genotype
P. solemdali sp. nov. can be identified by diagnostic nucleotides at three loci (Loci 886, 3599, and 1822), which are nearly fixed for different alleles than in P. flesus. The allele frequencies provided here are based on a combined dataset of 191 P. solemdali sp. nov. and 126 P. flesus from the Baltic Sea from this study and Momigliano et al. (2017a). At Locus 886 (GenBank Acc. no. KY933571-2), P. solemdali is characterized by adenine (A) at position 236 (allele frequency is 92% in P. solemdali sp. nov. vs. 7% in P. flesus), while guanidine (G) is more common in P. flesus (allele frequency: 93% in P. flesus vs. 8% in P. solemdali sp. nov.). At locus 1822 (GenBank Acc. no. KY933573-4), P. solemdali sp. nov. is characterized by cytosine (C) at position 363 (allele frequency: 87% in P. solemdali sp. nov. vs. 11% in P. flesus), while P. flesus is characterized by higher frequencies of A (allele frequency: 89% in P. flesus vs. 13% in P. solemdali sp. nov.). At locus 3599 (GenBank Acc. no. KY933578-9), P. solemdali is characterized by thymine (T) at position 237 (allele frequency: 92% in P. solemdali sp. nov. vs. 2% in P. flesus), while P. flesus is characterized by cytosine (C; allele frequency: 98% in P. flesus vs. 8% in P. solemdali sp. nov.). Other loci identified by Momigliano et al. (2017a) as under divergent selection in P. flesus and P. solemdali sp. nov. [Locus 2178 (GenBank Acc. no. KY933575-6), Locus 3556 (GenBank Acc. no. KY933577-8) and Locus 4474 (GenBank Acc. no. KY933581-2)], also shows very different frequencies in the P. flesus and P. solemdali sp. nov. though differences are not as marked as in the three diagnostic loci mentioned above (Momigliano et al., 2017a).
Etymology
This species is dedicated to Per Solemdal (1941–2016) who was the first researcher to study the Baltic Sea flounder's eggs and sperm in connection to salinity and discovered that “the specific gravity of the eggs is a fixed population characteristic which is almost unchangeable” (Solemdal, 1973) laying the foundations on which many subsequent studies on local adaptation and speciation of Baltic Sea marine fishes were built.
Discussion
The phylogenomic analysis identified two clearly distinct and well-supported clades, which correspond to the demersal and pelagic flounder genetic clusters identified by Momigliano et al. (2017a) and Florin and Höglund (2008). These two lineages were found to co-occur in multiple locations of the central and northern Baltic Sea, where no intermediate genotypes have yet been found (Figures 1, 4; Momigliano et al., 2017a), confirming previous suggestions that spawning behavior and reproductive traits are likely acting as strong reproductive barriers (Momigliano et al., 2017b).
According to our morphological description of adult specimens, there are no diagnostic meristic or external morphometric traits allowing unambiguous identification of the two flounder species occurring in the Baltic Sea. If anything, and in contrary to Mikelsaar (1958), the demersal Baltic flounder might differ from the European flounders from North Sea, Skagerrak Strait and Atlantic Ocean off Portugal (Voronina, 1999) by a fewer dorsal and anal fin rays (see Ojaveer and Drevs, 2003). However, a more thorough morphometric analysis, based on a larger sample of specimens of the pelagic lineage, including individuals from the North Sea and the Atlantic Ocean, should be conducted in order to determine whether fin-ray counts can be used as diagnostic characters.
The flounders' bony plates are modified scales with ctenial spines that retain a thin layer of sediment over the eye side of the fish, helping in camouflage (Spinner et al., 2016). It has also been speculated that bony plates may provide a defense against predation (Spinner et al., 2016), as in three-spined Gasterosteus aculeatus (Barrett, 2010) and nine-spined Pungitius pungitius (Merilä, 2013) sticklebacks. Baltic flounders show high variability in terms of numbers of bony plates, exhibiting phenotypes 1–4 described by Voronina (1999). The majority of them exhibit phenotype 3, with bony plates present mainly along the lateral lines, the spine and the head. At the first sight, this appears to be in contrast with data from sympatric P. flesus, which according to Voronina (1999) is fully plated (note that the variety P. flesus var. trachurus was described by Duncker (1892) due to this feature) in the Baltic Sea. However, the plate phenotypes of the P. flesus individuals sampled in this study ranged from 1 to 3, with phenotype 2 being the most common, and only two specimens (just over 10% of the individuals) exhibited phenotype 3. Hence, the bony plate coverage in flounders seems to vary both spatially and temporarily. While it is possible that the variation in the number of bony plates in flounders is largely genetically determined, similarly to lateral plate number variation in the three-spined stickleback Gasterosteus aculeatus (Colosimo et al., 2005), it is also possible that plate coverage is a partially or entirely phenotypically plastic trait determined by local environmental conditions. Unfortunately, distinguishing between these alternatives is not straightforward as this would require long-term common garden studies or mapping of genetic variants underlying plate variability (e.g., Merilä and Hendry, 2014). Nevertheless, additional sampling of both P. solemdali sp. nov. and P. flesus individuals from several allopatric and sympatric Baltic Sea localities would help in establishing whether bony plate numbers associate with environmental gradients in the Baltic Sea, as well as to get better idea of the possible spatial variability in platedness. At any rate, it seems safe to conclude that variation in bony plate numbers does not seem to be a helpful diagnostic trait in distinguishing between the two flounder species that occur in the Baltic Sea.
While external morphological characters cannot discriminate between the European and Baltic flounders, the two taxa can be clearly distinguished based on gamete physiology and morphology. Baltic populations of P. flesus spawn pelagic eggs which are far larger and lighter than both North Sea P. flesus and the Baltic Sea P. solemdali sp. nov., (Solemdal, 1971, 1973; this study; Nissling et al., 2002). This adaptation enables the spawning of pelagic propagules in deep water basins of the Baltic Sea (Solemdal, 1970) and has been observed also in cod (Nissling and Westin, 1997). The Baltic flounder evolved an opposite solution to the challenges of reproduction in hypohaline waters: they produce small and though eggs that sink to the bottom and spawn in shallower coastal waters (Solemdal, 1971, 1973; this study; Nissling et al., 2002). Sperm physiology also provides a means to clearly differentiate between the two species, as spermatozoa from Baltic flounders activate at salinities as low as 3–4 psu (successful fertilization, however, requires a salinity of at least 6 psu), while in European flounders from the Baltic Sea sperm activation occurs when salinity exceeds 10 psu (Nissling et al., 2002; this study). While both egg morphology and sperm physiology are important diagnostic traits, their utility in identifying individuals from each species in the wild is limited, as these traits can only be observed in adult individuals around the time of spawning.
The Baltic flounder is unlikely to opportunistically spawn in deep water basins, as demersal eggs will sink into the hypoxic waters that layer the bottom of the deep basins in the Baltic Sea, where they cannot survive. Similarly, P. flesus are unlikely to opportunistically fertilize demersal eggs, as their sperm only activates at salinities that are never encountered in the coastal waters of the Baltic proper (Nissling et al., 2002). While theoretically Baltic flounders could opportunistically fertilize P. flesus eggs in deep water basins, given the genetic results from Momigliano et al. (2017a) and this study, it seems certain that if such hybridization ever occurs it is either rare or geographically restricted. The evolution of opposite reproductive strategies might be a reflection of the different environmental conditions at the time the ancestors of the two contemporary species invaded the Baltic Sea. Momigliano et al. (2017a) estimated that the ancestors of the Baltic flounders invaded the Baltic Sea during the early stages of the Litorina sea, when salinity throughout the Baltic Sea was lower than today (Gustafsson and Westman, 2002). At that time, spawning of demersal eggs would have been the only viable reproductive strategy. The second invasion, from which the contemporary Baltic Sea populations of P. flesus derive, most likely happened at peak salinity some 6.5–4.5 kya (Momigliano et al., 2017a), when pelagic spawning would have been a viable option throughout a large portion of the Baltic Sea. Rather than collapsing in a hybrid swarm following secondary contact, the two lineages have persisted for thousands of years, suggesting that divergent selection on gamete morphology and physiology as well as spawning behavior may be acting as “magic traits”: that is, traits which are under ecological selection and directly cause reproductive isolation as a byproduct (Servedio et al., 2011; Nosil, 2012). The Baltic and European flounders also differ in potential fecundity (Nissling and Dahlman, 2010) and in feeding behavior and fecundity-regulation prior to spawning (Nissling et al., 2015). The Baltic flounder have higher fecundity than the European flounder, suggesting that the coastal spawning species allocate relatively more resources into reproductive growth than the pelagic flounder (Nissling and Dahlman, 2010). European flounders cease feeding and oocyte down-regulation well before spawning, resulting in a decrease in energy reserves (Nissling et al., 2015), characteristics typical of capital breeders (Jönsson, 1997). The Baltic flounder, in contrast, displays continuous oocyte down-regulation and continues feeding prior and during spawning (Nissling et al., 2015). It is however uncertain whether these differences in life history traits are plastic responses (for example, to food availability in pelagic and coastal spawning habitats) or evolutionary adaptations.
The results of this study support the results of Momigliano et al. (2017a), suggesting P. flesus and P. solemdali sp. nov. represent two clearly distinct lineages and have largely overlapping distributions within the Baltic Sea. Sperm motility and egg buoyancy trials confirmed that one of the two clades represent the demersal-spawning flounder, which shows clear differences in egg morphology (size and buoyancy), sperm physiology (activation and motility), spawning behavior, life history, and genetics from the pelagic-spawning flounder. This example of ecological speciation is comparable to that of lacustrine whitefishes Coregonus sp. in Europe and North America. In each lake where these taxa occur, species-pairs have evolved along depth gradients so that the two forms are distinguishable by molecular, morphological and physiological data (see Gagnaire et al., 2013; Hudson et al., 2017). In Europe some of these species-pairs show clear differences in reproductive ecology and are now considered as distinct species (Hudson et al., 2017).
The development of a simple genetic assay to clearly identify individuals to species should be very useful to both fishery managers and researchers interested in studying the ecology of the two species. As the results from this study and from Momigliano et al. (2017a) clearly show, the two flounder species co-occur in multiple locations in the central and northern Baltic Sea, as well as in the Gulf of Finland. Therefore, local populations should be managed within the framework of a mixed stock fishery. Failure to take into account this unobserved diversity may lead to unsustainable fishing practices, as declines in the most vulnerable stock components (which may vary from a location to another) may go undetected (Sterner, 2007). Furthermore, both flounder species in the Baltic Sea live close to their physiological limits, and salinity and oxygen content, the most important environmental factors driving the distribution of both species, are not stable in time. Short term fluctuations are largely due to the occurrence of large inflows or oxygen rich, high salinity waters from the North Sea, and due to stagnation periods in which salinity and oxygen concentrations decrease (Neumann et al., 2017). In the long term, eutrophication is deoxygenating the Baltic Sea (Carstensen et al., 2014) and climate change is decreasing salinity via increased freshwater runoff (Vuorinen et al., 2015). The latter will likely result in range contractions for many marine species in the Baltic Sea (MacKenzie et al., 2007; Vuorinen et al., 2015). Therefore, the contemporary distribution of the two flounder species in the Baltic Sea is likely to be non-stationary, subject to short- and long-term environmental changes driven by natural and anthropogenic factors. In this view, the genetic tools developed in this study will enable monitoring the responses of the two flounder species to these factors, and therefore to access essential information for the management and conservation of this economically and culturally important species pair.
In conclusion, the Baltic flounder P. solemdali sp. nov. is a new species according to the biological (Mayr, 1942; Dobzhansky and Dobzhansky, 1970), phylogenetic (Cracraft, 1983), ecological (Van Valen, 1976; Andersson, 1990), and genotypic clusters (Mallet, 1995) species concepts as well as considering the evolutionary species concept sensu Wiley and Mayden (2000), and Mayden's (2002) interpretation in particular. This species is very young (~7,200 years; Momigliano et al., 2017a), and little if any morphometric or meristic differentiation from P. flesus has occurred. In fact, P. solemdali sp. nov. is the only known fish species endemic to the Baltic Sea, and one of the two species endemic to this area, the other being the phytobenthic Fucus radicans (Ojaveer et al., 2010).
Compliance with Ethical Standards
Ethical Approval
All applicable international, national, and institutional guidelines for the care and use of animals were followed by the authors.
Field Study Permits
Sampling around Tvärminne (the only samples not obtained from commercial fisheries) was conducted in a nature reserve owned by the University of Helsinki, and permission for research was granted by Tvärminne Zoological Station (University of Helsinki). No permit numbers are issued for this.
Data Availability
The short sequence reads used in this study have been deposited in the GenBank Short Read Archive (Bioproject PRJNA382467, Short Read Archive SRP103564, Accessions SRX3862514-SRX3862559). Genotype data and the alignment used for population genomics and phylogenomics analyses, genotypes for diagnostic outlier loci, as well as data on relative proportions of the two species in sampling locations from this study and Momigliano et al. (2017a) are accessible via the Dryad digital repository (doi: 10.5061/dryad.8vr1001).
Author Contributions
PM, HJ, and JM designed the research. PM and HJ collected the data. PM, GD, and HJ analyzed the data. PM, GD, JM, and HJ wrote the paper.
Funding
Our research was supported by Maj & Tor Nessling Foundation (grant to JM), the Academy of Finland (project 292737), and the Finnish Cultural Foundation (grant to PM).
Conflict of Interest Statement
The authors declare that the research was conducted in the absence of any commercial or financial relationships that could be construed as a potential conflict of interest.
Acknowledgments
We thank Sven Hille, Oscar Nordahl, Torsten Sjölund, Ulf Wasström, and Petter Tibblin for helping with sample collection and Miinastiina Issakainen for her help in the laboratory. We are also grateful toward James Maclaine, curator of the British Museum of Natural History (BMNH), for getting access to the syntypes pictures of Pleuronectes flesus Linnaeus 1758.
Supplementary Material
The Supplementary Material for this article can be found online at: https://www.frontiersin.org/articles/10.3389/fmars.2018.00225/full#supplementary-material
Figure S1. PCA based on morphometric data (N = 64) of the samples of Baltic (demersal) and European (pelagic) flounders collected in this study.
Table S1. Summary of the samples collected and analyzed in this study and in Momigliano et al. (2017a). N = sample size; % demersal = proportion of the sampled individuals that were identified as demersal flounders using genotypic data. Population codes are as per Figure 1. Samples from locations indicated by an asterisk (Öland and Bornholm) were originally part of the study published by Momigliano et al. (2017a), but are used in this study as reference demersal and pelagic populations, (respectively), for 2b-RAD analyses.
Abbreviations
TL, total length; SL, standard length; BD, body depth; HL, head length; DL, dorsal fin base length; AL, anal fin base length; PL1, eye-side pectoral fin length; PL2, blind side pectoral fin length; VL, pelvic fin length; CPL, caudal peduncle length; CPD, caudal peduncle depth; PreDL, pre-dorsal fin length; PreAL, pre-anal fin length; PrePL, pre-pectoral fin length; PreVL, pre-pelvic fin length; SnL, snout length; ED, eye diameter; IW, interorbital width; PostOL, postorbital length; S, sidedness; PreCV, number of pre-caudal vertebrae; CV, number of caudal vertebrae; D, number of dorsal fin rays; A, number of anal fin rays; P, number of pectoral fin rays; V, number of pelvic fin rays; C, number of caudal fin rays; BP, bony plates phenotype.
References
Aarnio, K., Bonsdorff, E., and Rosenback, N. (1996). Food and feeding habits of juvenile flounder Platichthys flesus (L.), abd turbot Scophthalmus maximus L. in the Åland archipelago, northern Baltic Sea. J. Sea Res. 36, 311–320. doi: 10.1016/S1385-1101(96)90798-4
Akkaynak, D., Siemann, L. A., Barbosa, A., and Mäthger, L. M. (2017). Changeable camouflage: how well can flounder resemble the colour and spatial scale of substrates in their natural habitats? R. Soc. Open Sci. 4:160824. doi: 10.1098/rsos.160824.
Andersson, L. (1990). The driving force: species concepts and ecology. Taxon 39, 375–382. doi: 10.2307/1223084
Aro, E. (1989). A review of fish migration patterns in the Baltic. Rap. Proc. Verb. Ré. Cons. Int. Explor. Mer 190, 72–96.
Aro, E. (2002). Fish migration studies in the Baltic Sea—a historical review. ICES Marine Science Symposia. 215, 361–370.
Aro, E., and Sjöblom, V. (1983). The Migration of Flounder in the Northern Baltic Sea. Helsinki: International Council for the Exploration of the Sea, Baltic Fish Committee.
Artedi, P. (1738). Ichtyologia sive opera omnia de piscibus scilicet: Bibliotheca ichthyologica. Philosophia ichthyologica. Genera piscium. Synonymia specierum. Descriptiones specierum. Omnia in hoc genere perfectora quam antea ulla posthuma vindicavit, recognovit, coaptavit et edidit Carolus Linnaeus. Lugduni Batavorum: Wishoff.
Bagge, O. (1981). “Demersal fishes,” in The Baltic Sea, ed A. Voipio. (Amsterdam: Elsevier Scientific Company), 311–333.
Barrett, R. (2010). Adaptive evolution of lateral plates in three-spined stickleback Gasterosteus aculeatus: a case study in functional analysis of natural variation. J. Fish Biol. 77, 311–328. doi: 10.1111/j.1095-8649.2010.02640.x
Begg, G. A., Friedland, K. D., and Pearce, J. B. (1999). Stock identification and its role in stock assessment and fisheries management: an overview. Fish. Res. 43, 1–8. doi: 10.1016/S0165-7836(99)00062-4
Bendtsen, J., Söderkvist, J., Dahl, K., Hansen, J. L., and Reker, J. (2007). Model Simulations of Blue Corridors in the Baltic Sea. BALANCE interim Report 9:26.
Björck, S. (1995). A review of the history of the Baltic Sea, 13.0-8.0 ka BP. Q. Int. 27, 19–40. doi: 10.1016/1040-6182(94)00057-C.
Bonsdorff, E., and Norkko, A. (1994). Flounder (Platichthys flesus) spawning in Finnish archipelago waters. Memoranda Soc. Fauna Flora Fennica 70, 30–31.
Borg, J. P., Westerbom, M., and Lehtonen, H. (2014). Sex-specific distribution and diet of Platichthys flesus at the end of spawning in the northern Baltic Sea. J. Fish Biol. 84, 937–951. doi: 10.1111/jfb.12326
Carstensen, J., Andersen, J. H., Gustafsson, B. G., and Conley, D. J. (2014). Deoxygenation of the Baltic Sea during the last century. Proc. Natl. Acad. Sci. U.S.A. 111, 5628–5633. doi: 10.1073/pnas.1323156111.
Colosimo, P. F., Hosemann, K. E., Balabhadra, S., Villarreal, G., Dickson, M., Grimwood, J., et al. (2005). Widespread parallel evolution in sticklebacks by repeated fixation of ectodysplasin alleles. Science 307, 1928–1933. doi: 10.1126/science.1107239
Cracraft, J. (1983). Species concepts and speciation analysis. Curr. Ornithol. 1, 159–187. doi: 10.1007/978-1-4615-6781-3_6
De Queiroz, K. (2007). Species concepts and species delimitation. Syst. Biol. 56, 879–886. doi: 10.1080/10635150701701083
Dobzhansky, T., and Dobzhansky, T. G. (1970). Genetics of the Evolutionary Process. New York, NY: Columbia University Press.
Drevs, T., Kadakas, V., Lang, T., and Mellergaard, S. (1999). Geographical variation in the age/length relationship in Baltic flounder (Platichthys flesus). ICES J. Mar. Sci. 56, 134–137. doi: 10.1006/jmsc.1999.0460
Duncker, G. (1892). Der Elbbutt, eine Varietät der Flunder. (Pleuronectes flesus L. var. leiurus). Schrif. Nat. Vereins Schleswig Holstein 9, 275–291.
Erlandsson, J., Östman, Ö., Florin, A.-B., and Pekcan-Hekim, Z. (2017). Spatial structure of body size of European flounder (Platichthys flesus L.) in the Baltic Sea. Fish. Res. 189, 1–9. doi: 10.1016/j.fishres.2017.01.001
Evseenko, S. (2003). An annotated catalogue of pleuronectiform fishes (order Pleuronectiformes) of the seas of Russia and adjacent countries. J. Ichthyol. 43, S57–S74.
Fernholm, B., and Wheeler, A. (1983). Linnaean fish specimens in the Swedish Museum of Natural History, Stockholm. Zool. J. Linn. Soc. 78, 199–286. doi: 10.1111/j.1096-3642.1983.tb00867.x
Florin, A. B. (2005). Flatfishes in the Baltic Sea — a Review of Biology and Fishery With a Focus on Swedish Conditions. (Finfo 2005:14).
Florin, A., and Höglund, J. (2008). Population structure of flounder (Platichthys flesus) in the Baltic Sea: differences among demersal and pelagic spawners. Heredity 101, 27–38. doi: 10.1038/hdy.2008.22
Gagnaire, P. A., Pavey, S. A., Normandeau, E., and Bernatchez, L. (2013). The genetic architecture of reproductive isolation during speciation-with-gene-flow in lake whitefish species pairs assessed by rad sequencing. Evolution 67, 2483–2497. doi: 10.1111/evo.12075
Galleguillos, R., and Ward, R. (1982). Genetic and morphological divergence between populations of the flatfish Platichthys flesus (L.)(Pleuronectidae). Biol. J. Linnean Soc. 17, 395–408. doi: 10.1111/j.1095-8312.1982.tb02029.x
Gibson, R. N., Nash, R. D., Geffen, A. J., and Van der Veer, H. W. (2015). Flatfishes: Biology and Exploitation. John Wiley & Sons.
Gronovius, L. T. (1754). Museum ichthyologicum, sistens piscium indigenorum e quorundam exoticorum, qui in museuo Laurentii Theodori Gronovii, adservantur, descriptiones, ordine systematico; Accedunt Nonnullorum Exoticorum Piscium Icones, Aeri Incisae. Vol. 1, Lugduni Batavorum, Leiden: Haak.
Guindon, S., Dufayard, J.-F., Lefort, V., Anisimova, M., Hordijk, W., and Gascuel, O. (2010). New algorithms and methods to estimate maximum-likelihood phylogenies: assessing the performance of PhyML 3.0. Syst. Biol. 59, 307–321. doi: 10.1093/sysbio/syq010
Guo, B., DeFaveri, J., Sotelo, G., Nair, A., and Meril,ä, J. (2015). Population genomic evidence for adaptive differentiation in Baltic Sea three-spined sticklebacks. BMC Biol. 13:8. doi: 10.1186/s12915-015-0130-8.
Gustafsson, B. G., and Westman, P. (2002). On the causes for salinity variations in the Baltic Sea during the last 8500 years. Paleoceanography 17, 12-1–12-14. doi: 10.1029/2000PA000572
Hemmer-Hansen, J., Nielsen, E. E., Grønkjær, P., and Loeschcke, V. (2007). Evolutionary mechanisms shaping the genetic population structure of marine fishes; lessons from the European flounder (Platichthys flesus L.). Mol. Ecol. 16, 3104–3118. doi: 10.1111/j.1365-294X.2007.03367.x
Hinrichsen, H.-H., Petereit, C., Nissling, A., Wallin, I., Ustups, D., Florin, A.-B., et al. (2016). Survival and dispersal variability of pelagic eggs and yolk-sac larvae of central and eastern baltic flounder (Platichthys flesus): application of biophysical models. ICES J. Mar. Sci. 74, 41–55. doi: 10.1093/icesjms/fsw163
Hudson, A. G., Lundsgaard-Hansen, B., Lucek, K., Vonlanthen, P., and Seehausen, O. (2017). Managing cryptic biodiversity: fine-scale intralacustrine speciation along a benthic gradient in Alpine whitefish (Coregonus spp.). Evol. Appl. 10, 251–266. doi: 10.1111/eva.12446
ICES (2017). ICES WGBFAS REPORT 2016: Report of the Baltic Fisheries Assessment Working Group (WGBFAS). ICES HQ, Copenhagen, Denmark.
Jokinen, H., Wennhage, H., Lappalainen, A., Ådjers, K., Rask, M., and Norkko, A. (2015). Decline of flounder (Platichthys flesus (L.)) at the margin of the species' distribution range. J. Sea Res. 105, 1–9. doi: 10.1016/j.seares.2015.08.001
Jokinen, H., Wennhage, H., Ollus, V., Aro, E., and Norkko, A. (2016). Juvenile flatfish in the northern Baltic Sea—long-term decline and potential links to habitat characteristics. J. Sea Res. 107, 67–75. doi: 10.1016/j.seares.2015.06.002
Jombart, T. (2008). adegenet: a R package for the multivariate analysis of genetic markers. Bioinformatics 24, 1403–1405. doi: 10.1093/bioinformatics/btn129
Jombart, T., Devillard, S., and Balloux, F. (2010). Discriminant analysis of principal components: a new method for the analysis of genetically structured populations. BMC Genet. 11:94. doi: 10.1186/1471-2156-11-94
Jonsson, A. L. (2014). Morfometrisk och Konditionsmässig Variation hos Platichthys Flesus i Östersjön. Gothenburg: Göteborgs Universitet. Institutionen för Biologi och Miljövetenskap.
Jönsson, K. I. (1997). Capital and income breeding as alternative tactics of resource use in reproduction. Oikos 78, 57–66. doi: 10.2307/3545800
Kottelat, M. (1997). European freshwater fishes. An heuristic checklist of the freshwater fishes of europe (exclusive of former USSR), with an introduction for nonrsystematists and comments on nomenclature and conservation. Biologia Bratislava. 52(Suppl. 5), 1–271.
Linnaeus, C. (1751). Skånska Resa på Höga Öfwerhetens Befallning Förrättad år 1749. Salviani, Stockholm.
Linnaeus, C. (1758). Systema Naturae per Regna Tria Naturae, Secundum Classes, Ordines, Genera, Species, Cum Characteribus, Differentiis, Synonymis, Locis. Tomus I. Edn decima, reformata. Salvius: Holmiae.
Lønning, S., and Solemdal, P. (1974). The Relation Between Thickness of Chorion and Specific Gravity of Eggs From Norwegian and Baltic Flatfish Populations. FiskDir. Skr. Ser.HavUnders. 16, 77–88.
MacKenzie, B. R., Gislason, H., Möllmann, C., and Köster, F. W. (2007). Impact of 21st century climate change on the Baltic Sea fish community and fisheries. Glob. Chang. Biol. 13, 1348–1367. doi: 10.1111/j.1365-2486.2007.01369.x
Mallet, J. (1995). A species definition for the modern synthesis. Trends Ecol. Evol. 10, 294–299. doi: 10.1016/0169-5347(95)90031-4
Mayden, R. L. (2002). On biological species, species concepts and individuation in the natural world. Fish Fish. 3, 171–196. doi: 10.1046/j.1467-2979.2002.00086.x
Mayr, E. (1942). Systematics and the Origin of Species, From the Viewpoint of a Zoologist. New York, NY: Harvard University Press.
Merilä, J. (2013). Nine-spined stickleback (Pungitius pungitius): an emerging model for evolutionary biology research. Ann. N. Y. Acad. Sci. 1289, 18–35. doi: 10.1111/nyas.12089
Merilä, J., and Hendry, A. P. (2014). Climate change, adaptation, and phenotypic plasticity: the problem and the evidence. Evol. Appl. 7, 1–14. doi: 10.1111/eva.12137
Mielck, W. W., and Künne, C. (1935). Fischbrut-und Plankton-Untersuchungen auf dem Reichsforschungsdampfer'Poseidon'in der Ostsee, Mai-Juni 1931. Lipsius & Tischer.
Mikelsaar, N. (1958). “Über die Anwendung der Methode der ausgeglischenen Skalen bei der Untersuchung der artinneren Gruppierungen der Flunder,” in Hüdrobioloogilised Uurimused, eds H. Haberman, H. Simm, and N. Mikelsaar (Tartu: Eesti NSV Teaduste Akadeemia Zoologia ja Botaanika Instituut), 287–314.
Momigliano, P., Jokinen, H., Fraimout, A., Florin, A.-B., Norkko, A., and Merilä, J. (2017a). Extraordinarily rapid speciation in a marine fish. Proc. Natl Acad. Sci. U.S.A. 114, 6074–6079. doi: 10.1073/pnas.1615109114
Momigliano, P., Jokinen, H., Fraimout, A., Florin, A., Norkko, A., and Merilä, J. (2017b). Data from: extraordinarily rapid speciation in a Marine fish. Dryad Data Repository. doi: 10.5061/dryad.f6154. [Epub ahead of print].
Morrow, J. E. (1980). The Freshwater Fishes of Alaska. Anchorage, AK: Alaska Northwest Publishing Company.
Neumann, T., Radtke, H., and Seifert, T. (2017). On the importance of Major Baltic Inflows for oxygenation of the central Baltic Sea. J. Geophys. Res. Oceans 122, 1090–1101. doi: 10.1002/2016JC012525
Nissling, A., and Dahlman, G. (2010). Fecundity of flounder, Pleuronectes flesus, in the Baltic Sea—reproductive strategies in two sympatric populations. J. Sea Res. 64, 190–198. doi: 10.1016/j.seares.2010.02.001
Nissling, A., Johansson, U., and Jacobsson, M. (2006). Effects of salinity and temperature conditions on the reproductive success of turbot (Scophthalmus maximus) in the Baltic Sea. Fish. Res. 80, 230–238. doi: 10.1016/j.fishres.2006.04.005
Nissling, A., Kryvi, H., and Vallin, L. (1994). Variation in egg buoyancy of Baltic cod Gadus morhua and its implications for egg survival in prevailing conditions in the Baltic Sea. Mar. Ecol. Prog. Ser. 110, 67–74. doi: 10.3354/meps110067
Nissling, A., Nyberg, S., and Petereit, C. (2017). Egg buoyancy of flounder, Platichthys flesus, in the Baltic Sea—adaptation to salinity and implications for egg survival. Fish. Res. 191, 179–189. doi: 10.1016/j.fishres.2017.02.020
Nissling, A., Thorsen, A., and da Silva, F. F. (2015). Fecundity regulation in relation to habitat utilisation of two sympatric flounder (Platichtys flesus) populations in the brackish water Baltic Sea. J. Sea Res. 95, 188–195. doi: 10.1016/j.seares.2014.06.003
Nissling, A., and Westin, L. (1997). Salinity requirements for successful spawning of Baltic and Belt Sea cod and the potential for cod stock interactions in the Baltic Sea. Mar. Ecol. Prog. Ser. 152, 261–271. doi: 10.3354/meps152261
Nissling, A., Westin, L., and Hjerne, O. (2002). Reproductive success in relation to salinity for three flatfish species, dab (Limanda limanda), plaice (Pleuronectes platessa), and flounder (Pleuronectes flesus), in the brackish water Baltic Sea. ICES J. Mar. Sci. 59, 93–108. doi: 10.1006/jmsc.2001.1134
Ojaveer, E., and Drevs, T. (2003). “Flounder, Platichthys flesus trachurus (Duncker),” in Fishes of Estonia, eds E. Ojaveer, E. Pihu, and T. Saat (Tallin: Estonian Academy Publisher), 362–370.
Ojaveer, H., Jaanus, A., MacKenzie, B. R., Martin, G., Olenin, S., Radziejewska, T., et al. (2010). Status of biodiversity in the Baltic Sea. PLoS ONE 5:e12467. doi: 10.1371/journal.pone.0012467
Orio, A., Bergström, U., Casini, M., Erlandsson, M., Eschbaum, R., Hüssy, K., et al. (2017). Characterizing and predicting the distribution of Baltic Sea flounder (Platichthys flesus) during the spawning season. J. Sea Res. 126, 46–55. doi: 10.1016/j.seares.2017.07.002
Rafinesque, C. S. (1815). Analyse de la Nature, ou Tableau de l'univers et des Corps Organisés. Palermo: l'Imprimerie de Jean Barravecchia.
Ramachandran, V. S., Tyler, C. W., Gregory, R. L., Rogers-Ramachandran, D., Duensing, S., Pillsbury, C., et al. (1996). Rapid adaptive camouflage in tropical flounders. Nature 379, 815–818. doi: 10.1038/379815a0
Reiss, H., Hoarau, G., Dickey-Collas, M., and Wolff, W. J. (2009). Genetic population structure of marine fish: mismatch between biological and fisheries management units. Fish Fish. 10, 361–395. doi: 10.1111/j.1467-2979.2008.00324.x
Sandman, J. (1906). Kurzer bericht über in Finnland ausgeführte untersuchungen über den Flunder, den Steinbutt und den Kabeljau. Rapp Pv Réun Cons int Explor Mer 5, 37–44.
Servedio, M. R., Van Doorn, G. S., Kopp, M., Frame, A. M., and Nosil, P. (2011). Magic traits in speciation:‘magic’but not rare? Trends Ecol. Evol. 26, 389–397. doi: 10.1016/j.tree.2011.04.005
Solemdal, P. (1967). The effect of salinity on buoyancy, size and development of flounder eggs. Sarsia 29, 431–442. doi: 10.1080/00364827.1967.10411098
Solemdal, P. (1970). The Reproductive Adaption of Marine Teleosts to Water of Low Salinity. ICES:1970/F:30.
Solemdal, P. (1971). Prespawning flounders transferred to different salinities and the effects on their eggs. Vie Milieu Suppl. 22, 409–423.
Solemdal, P. (1973). Transfer of Baltic flatfish to a marine environment and the long termeffects on reproduction. Oikos 22, 268–276.
Spinner, M., Kortmann, M., Traini, C., and Gorb, S. N. (2016). Key role of scale morphology in flatfishes (Pleuronectiformes) in the ability to keep sand. Sci. Rep. 6:26308. doi: 10.1038/srep26308
Stålberg, L. (2015). Morfologisk Variation Hos Skrubbskädda (Platichthys flesus): Finns det en Koppling till Ekologi? Uppsala: Bachelor Degree, Swedish University of Agricultural Sciences.
Sterner, T. (2007). Unobserved diversity, depletion and irreversibility The importance of subpopulations for management of cod stocks. Ecol. Econ. 61, 566–574. doi: 10.1016/j.ecolecon.2006.05.015
Summers, R. (1979). Life cycle and population ecology of the flounder Platichthys flesus (L.) in the Ythan estuary, Scotland. J. Nat. Hist. 13, 703–723. doi: 10.1080/00222937900770531
Sumner, F. B. (1911). The adjustment of flatfishes to various backgrounds: a study of adaptive color change. J. Exp. Zool. 10, 409–506. doi: 10.1002/jez.1400100405
Tin, M. M. Y., Rheindt, F. E., Cros, E., and Mikheyev, A. S. (2015). Degenerate adaptor sequences for detecting PCR duplicates in reduced representation sequencing data improve genotype calling accuracy. Mol. Ecol. Resour. 15, 329–336. doi: 10.1111/1755-0998.12314
Vallin, L., and Nissling, A. (2000). Maternal effects on egg size and egg buoyancy of Baltic cod, Gadus morhua: implications for stock structure effects on recruitment. Fish. Res. 49, 21–37. doi: 10.1016/S0165-7836(00)00194-6
Van Valen, L. (1976). Ecological species, multispecies, and oaks. Taxon 25, 233–239. doi: 10.2307/1219444
Voronina, E. (1999). Morphology and systematics of river flounders of the genus Platichthys. J. Ichthyol. 39, 588–599.
Vuorinen, I., Hänninen, J., Rajasilta, M., Laine, P., Eklund, J., Montesino-Pouzols, F., et al. (2015). Scenario simulations of future salinity and ecological consequences in the Baltic Sea and adjacent North Sea areas–implications for environmental monitoring. Ecol. Indic. 50, 196–205. doi: 10.1016/j.ecolind.2014.10.019
Wallin, I. (2016). Opportunities for Hybridization Between Two Sympatric Flounder (Platichthys flesus) Ecotypes in the Baltic Sea. Uppsala: Master Uppsala Universet.
Wang, S., Meyer, E., McKay, J. K., and Matz, M. V. (2012). 2b-RAD: a simple and flexible method for genome-wide genotyping. Nat. Methods 9, 808–810. doi: 10.1038/nmeth.2023
Wheeler, A. (1991). The Linnaean fish collection in the Zoological Museum of the University of Uppsala. Zool. J. Linn. Soc. 103, 145–195. doi: 10.1111/j.1096-3642.1991.tb00901.x
Wheeler, A. C. (1958). The Gronovius Fish Collection: A Catalogue and Historical Account (London: British Museum of Natural History), 187–249.
Keywords: ecological speciation, Baltic Sea, Platichthys solemdali, Platichthys flesus, Pleuronectidae, mixed-stock fishery
Citation: Momigliano P, Denys GPJ, Jokinen H and Merilä J (2018) Platichthys solemdali sp. nov. (Actinopterygii, Pleuronectiformes): A New Flounder Species From the Baltic Sea. Front. Mar. Sci. 5:225. doi: 10.3389/fmars.2018.00225
Received: 24 January 2018; Accepted: 11 June 2018;
Published: 11 July 2018.
Edited by:
Wei-Jen Chen, National Taiwan University, TaiwanReviewed by:
Matthew Campbell, Hokkaido University, JapanPhilippe Borsa, Institut de Recherche Pour le Développement (IRD), France
Copyright © 2018 Momigliano, Denys, Jokinen and Merilä. This is an open-access article distributed under the terms of the Creative Commons Attribution License (CC BY). The use, distribution or reproduction in other forums is permitted, provided the original author(s) and the copyright owner(s) are credited and that the original publication in this journal is cited, in accordance with accepted academic practice. No use, distribution or reproduction is permitted which does not comply with these terms.
*Correspondence: Paolo Momigliano, cGFvbG8ubW9taWdsaWFub0BoZWxzaW5raS5maQ==