- 1Unité Biologie des Organismes et Ecosystèmes Aquatiques (BOREA), Muséum National d’Histoire Naturelle, Sorbonne Université, Université de Caen Normandie, Université des Antilles, CNRS, IRD, Eq. Adaptations aux Milieux Extrêmes, Paris, France
- 2InterRidge Office, Institut de Physique du Globe de Paris, Paris, France
- 3UMR CNRS MNHN 7245 Molécules de Communication et Adaptation des Micro-organismes, Muséum National d’Histoire Naturelle et Institut Universitaire de France, Paris, France
In crustaceans, as in other animals, perception of environmental cues is of key importance for a wide range of interactions with the environment and congeners. Chemoreception involves mainly the antennae and antennules, which carry sensilla that detect water-borne chemicals. The functional importance of these as exchange surfaces in the shrimp’s sensory perception requires them to remain free of any microorganism and deposit that could impair the fixation of odorant molecules on sensory neurons. We report here the occurrence of an unexpected dense bacterial colonization on surface of the antennae and antennules of four hydrothermal vent shrimp species. Microscopic observation, qPCR and 16S rRNA barcoding reveal the abundance, diversity and taxonomic composition of these bacterial communities, that are compared with those found on a related coastal shrimp. Bacterial abundances vary among species. Bacteria are almost absent in coastal shrimp, meanwhile they fully cover the antennal flagella in some hydrothermal vent species. Epsilon- and Gammaproteobacteria dominate the hydrothermal shrimp-associated communities, whereas Alphaproteobacteria and Bacteroidetes are dominant in the coastal ones. Bacteria associated with vent shrimp species are most similar to known chemoautotrophic sulfur-oxidizers. Potential roles of these bacteria on the hydrothermal shrimp antennae and antennules and on sensory functions are discussed.
Introduction
Information derived from environmental cues is essential for animals to make behaviorally and ecologically relevant decisions. For mobile fauna inhabiting deep-sea hydrothermal vents, the environment presents several challenges. One of these is to accurately detect active fluid emissions in the absence of light. Hot fluids can indeed be deadly, meanwhile a certain level of exposure is often necessary in order for animals to gain the compounds that their bacterial symbionts use as substrates. Decapod shrimp of the family Alvinocarididae are among the most abundant vagile macrofauna around chimneys at many hydrothermal vents (Desbruyères et al., 2000).
The most studied species, Rimicaris exoculata, lives in the immediate vicinity of fluid exits, forming highly dense and spectacular swarms sheltering thousands of highly motile individuals that constantly swim in and out of the swarm (Segonzac et al., 1993). It relies on chemoautotrophic symbiotic bacteria that use reduced compounds from the fluid and ensure hosts nutrition (Zbinden et al., 2004; Ponsard et al., 2013). Other species including Rimicaris chacei (formerly Chorocaris chacei, reassigned to its initial genus by Vereshchaka et al., 2015), Mirocaris fortunata and Alvinocaris markensis, live at lower densities or as solitary individuals, in areas of weak fluid emissions (Casanova et al., 1993). Shrimp species need to evaluate their environment in order to interact with their congeners, find food, or to find the most suitable habitat. This particular habitat is characterized by steep physicochemical gradients, and deadly risk of exposure to high-temperature fluids (Sarradin et al., 1999; Sarrazin et al., 1999; Le Bris et al., 2006). In crustaceans, sensory perception is mediated by sensilla distributed over almost the entire body (i.e., first and second antennae, mouthparts, legs, cephalothorax, abdomen and telson) (Ache, 1982). Chemoreception is more specifically mediated by two types of chemosensory sensilla: unimodal olfactory sensilla (the aesthetascs) and bimodal sensilla (containing mechano- and chemo-receptor neurons), mainly located on the first and second antennae (Ache, 1982; Derby et al., 2016). The sense of smell is associated with intermittent interaction between the odor environment and these chemosensory organs (Schmitt and Ache, 1979). Aesthetascs sensilla are characterized by a thin cuticle (Kamio and Derby, 2017; Machon et al., 2018), permeable to environment chemicals which will cross the cuticle and bind to the olfactory sensory neurons. Bimodal sensilla, on the other hand, have a thick and non-permeable cuticle, such that environmental chemicals enter them only via a terminal pore, and bind to chemo- and mechano-receptor (Kamio and Derby, 2017).
A recent study describing the general structure of chemosensory organs of vent shrimp (Zbinden et al., 2017) highlighted a previously unsuspected bacterial colonization on the surface of the antennae and antennules, nevertheless without characterization of the microorganisms. If associations with bacteria are widespread in hydrothermal vent ecosystems, including on the surface of arthropod cuticle (Dubilier et al., 2008; Goffredi, 2010), occurrence of bacteria on the chemosensory organs is surprising and was not reported before. Crustaceans living in aquatic environments are under constant exposure to a wide variety of microbial and micro- or macroscopic fouling organisms, and thus spend considerable time and energy in grooming or cleaning their body (Bauer, 1989). Grooming of the antennules, the primary olfactory organs, is the most commonly observed grooming behavior in crustaceans. The antennules are indeed frequently wiped by the setal combs of the third pair of maxillipeds, in order to remove the material and odorants that have accumulated on or between the aesthetascs (Bauer, 1989; Barbato and Daniel, 1997). According to Barbato and Daniel (1997), anything enhancing the level of microbial fouling is detrimental to the structural integrity of the antennules, and presumably to its functional role as a chemoreceptor organ. Experiments preventing shrimp from grooming their antennules indeed lead to extensive structural damage of the aesthetascs, sometimes up to the breakage and loss of all of them within 2 weeks (Bauer, 1977). Identical findings were made on insects, for which preventing antennal grooming lead to the obstruction of sensilla pores, thus impairing olfaction (Böröczky et al., 2013). This result was confirmed by electroantennogram measurements, which show that insect antennae that were prevented from being groomed were significantly less responsive to the pheromones than groomed ones (Böröczky et al., 2013). Other, previously described, associations between R. exoculata and bacteria harbored in the gill chamber (Segonzac et al., 1993; Zbinden et al., 2004) are, on the contrary, beneficial for shrimp and considered as true mutualism: bacteria nourish shrimps through transcuticular transfers of small organic molecules they produce, and/or contribute to the detoxication of the environment (Ponsard et al., 2013).
Observation of bacterial coverage, sometimes very dense, on the chemosensory organs of different species of hydrothermal shrimp raises the question of the potential impact of bacteria on chemoreception and their possible role(s) in shrimp physiology. We propose here a detailed description of the occurrence of bacteria on antennae and antennules of four hydrothermal species (Rimicaris exoculata, Rimicaris chacei, Mirocaris fortunata, and Alvinocaris markensis) and a related species sampled in coastal waters (Palaemon elegans). We assess the coverage, relative abundance and diversity of bacteria associated with antennules and antennae of shrimp using electron microscopy, qPCR, and a high-throughput metabarcoding approach based on the investigation of the bacterial 16S rRNA-encoding gene. This study is the first to investigate the microbial populations associated with sensory organs in deep-sea shrimp, and yields insights on their potential metabolisms and roles for shrimp.
Materials and Methods
Animal Collection, Maintenance, and Conditioning
Specimens of the Alvinocarididae Mirocaris fortunata, Rimicaris exoculata, Rimicaris chacei, and Alvinocaris markensis were collected during the Biobaz, 2013 and Bicose, 2014 cruises, on hydrothermal vent sites located on the Mid-Atlantic Ridge (see Table 1 for cruises and sites). In each sampling event, shrimp were collected using the suction sampler of the ROV ‘Victor 6000’ operating from the RV ‘Pourquoi Pas?’ The ROV then surfaced within a few hours, and living specimens were processed immediately. Specimens were dissected and tissues of interest (see below) were fixed for morphological observations (see below), or frozen in liquid nitrogen for molecular biology experiments. Specimens of the Palaemonidae Palaemon elegans were collected from Saint-Malo region in 2016 (France), using a shrimp hand net. Those specimens were dissected and fixed 1 day after field sampling and used for all molecular biology analyses and some electron microscopy. Other P. elegans specimens used for electron microscopy were collected in 2013, and transferred to aerated aquaria with a 12 h:12 h light:dark cycle, a salinity of 35 g.l-1, and a water temperature of 18°C prior to dissection.
Scanning Electron Microscopy (SEM)
For morphological observations, antennae and antennules (both medial and lateral flagella), as well as branchiostegites (lateral part of the carapace covering the gills), were used. Samples were fixed in a 2.5% glutaraldehyde/seawater solution, rinsed and post-fixed in osmium tetroxide 1%. For SEM, they were then dehydrated through a series of baths of increasing ethanol concentrations, critical-point-dried with an Emitech K850 or a CPD7501 critical point drying apparatus (Quorum Technologies, Laughton, United Kingdom) and platinum-coated in a Scancoat six Edwards sputter-unit or gold-coated with a JEOL JFC-1200 fine coater. Observations were carried out with a scanning electron microscope (Cambridge Stereoscan 260 or Hitachi SU3500), operating at 15 kV.
DNA Extraction and Estimation of Bacterial Densities Using qPCR
From each individual specimen, the antennae, the medial and lateral flagella of the antennules were separated, flash frozen and stored in liquid nitrogen until further processing. Due to small size, the antennae/antennular organs from three to five specimens were pooled (Table 1). DNA was extracted using the QIAGEN Blood and Tissue Kit according to the manufacturer’s instructions (Qiagen, Valencia, CA, United States), and visualized on an agarose gel.
The host gene encoding GAPDH (glyceraldehyde-3-phosphate dehydrogenase) was used as a reference gene, as previously recommended (Cottin et al., 2010). GAPDH is a single copy-gene in vertebrates, and possibly also in crustaceans based on some work (Camacho-Jiméneza et al., 2018), although very few studies have investigated this in detail. It was amplified using two distinct primer sets for the hydrothermal vent shrimp (RimGAPDH-F 5′-CCCCTTCATTGCTCTGGACTAC-3′ and RimGAPDH-R 5′-ACCACCTTGGAAGTGAGCAGA-3′, originally designed for Rimicaris exoculata; Cottin et al., 2010), and for the coastal shrimp Palaemon elegans (PalGAPDH-F 5′-GTGAGGTGAAGGCTGAGGAC-3′ and PalGAPDH-R 5′-CGGATGGAGCAGAGATGATT-3′, this study). The bacterial 16S rRNA encoding gene was amplified using primers Eub-1052F (5′-TGCATGGYTGTCGTCAGCTCG-3′) and Eub-1193R (5′-CGTCRTCCCCRCCTTCC-3′, Wang and Qian, 2009). The specificity of primer sets was tested by gel electrophoresis and the sequencing of PCR products. The qPCR reactions were performed using the LightCycler® 480 SYBR I Master reaction mix on a LightCycler® 480 II Real-Time PCR Detection System (Roche, France) using 45 cycles consisting of denaturation (95°C, 10 s), hybridization (60°C, 15 s) and elongation (72°C, 15 s). Each reaction was run in triplicates for each gene and shrimp specimen, and replicates with CT differing by more than 0.5 were discarded. A dilution series of an equimolar mixture of DNA extracted from all of the samples used in this study was included in each run and used to evaluate the amplification efficiency. The quality of qPCR runs was assessed based on melting curves and measured efficiencies. The bacterial 16S rRNA results were normalized and expressed as “-folds” versus host GAPDH by the ΔCT method. The results for the vent shrimp species were compared with the P. elegans data using the ΔΔCT method.
16S rRNA-Based Metabarcoding of Bacterial Communities Associated With Antennae and Antennules
A control PCR using the standard bacterial 16S rRNA gene-targeting primers 27F and 1492R was performed to test for the presence of bacteria. A ∼500 bp fragment of the 16S rRNA-encoding gene corresponding to the V1–V3 variable regions of Escherichia coli was then amplified using primers 27F (5′-AGAGTTTGATCCTGGCTCAG-3′) and 534R (5′-ATTACCGCGGCTGCTGG-3′, Watanabe et al., 2001). This primer set was used to produce product for sequencing on an Illumina HiSeq platform (2 × 300 bp, paired-end sequencing, GATC Biotech, Germany). Raw reads were deposited into the GENBANK Sequence Read Archive (SRA) database under accession number SRP136751.
Sequence Analysis
Sequence analyses were performed using QIIME2 (Caporaso et al., 2010). Paired-end sequences were imported and demultiplexed. Sequences quality control was performed using the DADA2 plugin (Callaghan et al., 2016). Sequences were trimmed to 280 bp before paired-ends were assembled. The error model was trained using 1,000,000 sequences. Reads displaying “N,” quality score below 2, more than two expected errors were discarded. Assembled sequences below 450 and above 600 bp were discarded. Chimeric sequences were removed based on a consensus approach, and a feature table was built. Identified unique amplicon sequence variants were then used to generate a guide phylogenetic tree that was used to compute UniFrac distances between samples (Lozupone and Knight, 2005). Rarefaction curves and diversity indices were also generated with QIIME2 using a sampling depth of 1,400,000 reads, corresponding to the lowest number of reads obtained for one sample (Alvinocaris markensis A1lat, see Table 1). Variants were classified using a naive Bayesian classifier using the SILVA (release 119-99) database (Quast et al., 2013) and bar-plots were generated. Community composition was compared between samples using alpha diversity indexes (observed variants and the Shannon index). A Venn diagram including all variants except singletons was draw using the web-based tool available at http://bioinformatics.psb.ugent.be/webtools/Venn/. Community composition in the different species was compared using the PERMANOVA test implemented in QIIME2.
Results
Microscopic Observation of Microbial Communities on Antennules and Antennae
Important inter-species variations in the amount of bacteria were observed among the five shrimp species observed. Inter-individual variations were also observed, possibly linked, in part, to the molting stage which was not determined in this study. In the coastal species Palaemon elegans, antennules, antennae and branchiostegites were almost devoid of bacteria (Figures 1A,D), which occurred only as few scattered rods, mainly in intersegment furrows (Figure 1B) and at the base of the sensilla (Figure 1C). The most colonized samples were those from Mirocaris fortunata, where bacteria settled down the whole length of the antennular and antennal flagella (Figures 2A,B). Bacteria occurred either as dense plates of rods which could cover the entire circumference of the flagella (usually toward the base, Figure 2C), or in dense filamentous bacterial mats, which could be as long as the sensilla (toward the apex) (Figure 2D). In between these two extremes, a gradation was observed. Alvinocaris markensis resembled P. elegans in terms of density of bacteria present. Its bacterial cover was globally very modest (Figure 3A), with just a few rods at the base of the sensilla and in the intersegments. Increasing densities were observed in Rimicaris exoculata (Figure 3C), and even more in R. chacei (Figure 3D). On the less colonized samples, bacteria were present as patches of rods on the surface of the flagella, and in the intersegment troughs, with more filamentous bacteria and longer ones when the colonization increased. Aesthetascs were sometimes completely covered by rods, and sometimes by filamentous bacteria (Figures 1D, 3E,F).
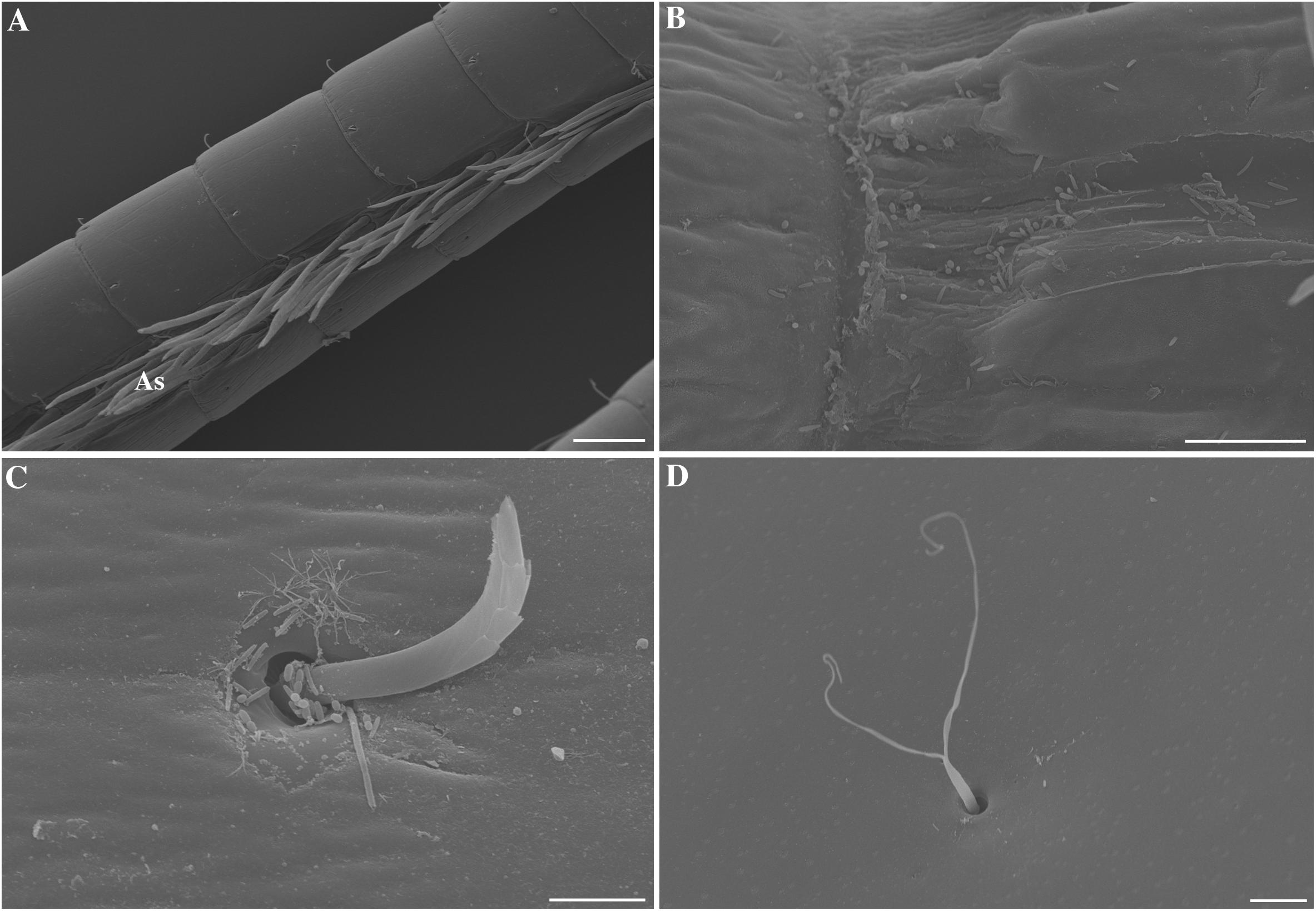
FIGURE 1. Scanning electron micrographs of Palaemon elegans antennules, antennae and branchiostegite. (A) Lateral antennular flagellum bearing the aesthetascs (As). (B) Close-up of a intersegment of the antennae colonized by few rod-type bacteria. (C) Close-up of the base of a non-aesthetasc beaked scaly seta on the antennae. (D) Close-up of a bifid seta on the outer side of the branchiostegite showing no bacterial colonization. Scale bars: (A) = 100 μm; (B), (D) = 10 μm; (C) = 5 μm.
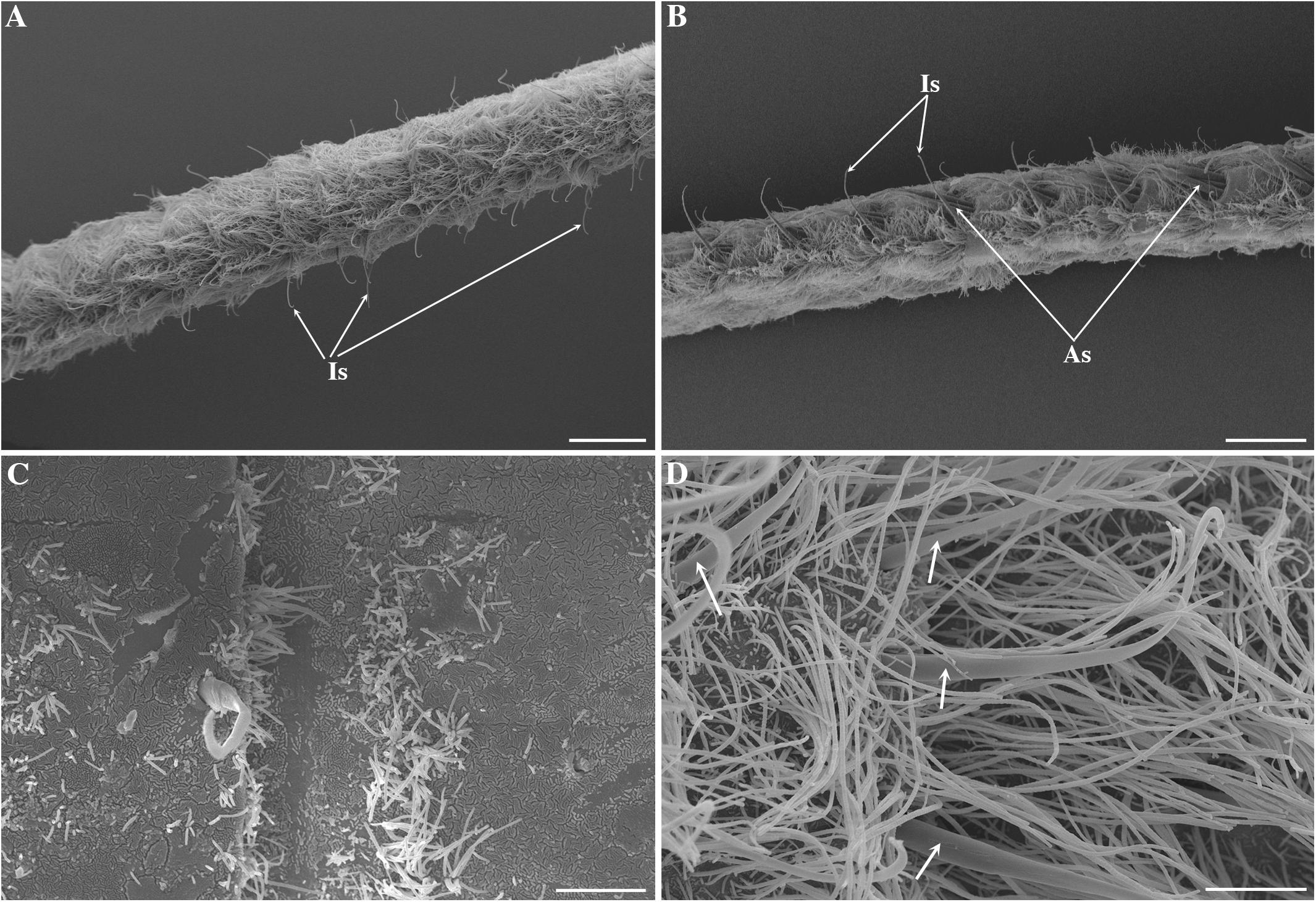
FIGURE 2. Scanning electron micrographs of Mirocaris fortunata antennules. Medial (A) and lateral (B) antennular flagellum completely covered by filamentous bacteria. Intermediate seta (Is) and aesthetasc (As) are visible among the bacterial filaments. (C) Close-up of the base of the antenna showing patches of rod-shaped bacteria and some bacterial filaments. (D) Close-up of the medial flagella of the antennule, showing non-aesthetasc setae (arrows) in the midst of the bacteria. Scale bars: (A) = 200 μm; (B) = 100 μm; (C,D) = 20 μm.
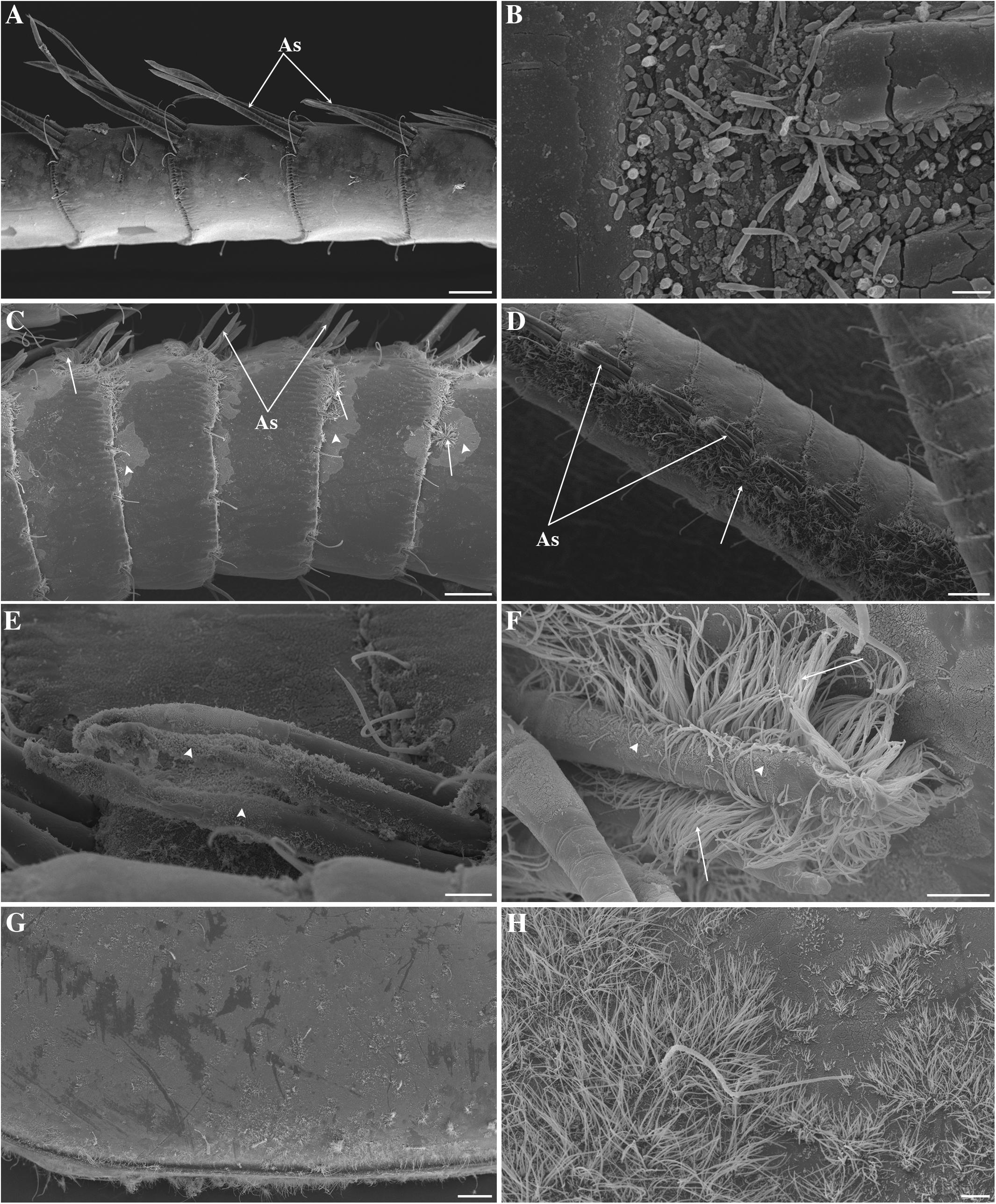
FIGURE 3. Scanning electron micrographs of Alvinocaris markensis, Rimicaris chacei and Rimicaris exoculata antennae and branchiostegite. (A) Alvinocaris markensis lateral antennular flagellum bearing the aesthetascs (As) and almost devoid of bacteria. (B) Close-up on an intersegment of the antennae of A. markensis colonized by rods and rods tapered at their extremity. (C) Rimicaris exoculata lateral antennular flagellum bearing the aesthetascs (As). Patches of rods are visible (arrow heads) along with some short filamentous bacteria (arrows). (D) Rimicaris chacei lateral antennular flagellum bearing the aesthetascs (As), surrounded by patches of filamentous bacteria (arrows). (E,F) Closes-up on the aesthetascs (As) of M. fortunata (E) and R. exoculata (F), covered by rods (arrows heads) and filamentous bacteria (arrows). Scale bars: (A,C,D) = 100 μm; (B) = 2 μm; (E,F,H) = 20 μm; (G) = 200 μm.
The branchiostegites were also observed to test if the bacterial coverage of the antennal flagella was specifically located on these appendages. They revealed bacterial colonization in hydrothermal species, quite in accordance with what was observed on the antennae and antennules (Figures 3G,H): the more the antennae were colonized, the more the branchiostegites were, too.
Morphotypes observed were very similar among the different species, and limited to thin and wide rods and filaments. A. markensis was the only species that displayed a broader diversity, with rods of different sizes, cocci, and a morphotype characteristics of rods tapered at their extremity, found on the antennae, the antennules and branchiostegites (see Figure 3B).
qPCR-Based Comparison of Bacterial Densities Among Species and Appendages
The qPCR-estimated ratio between the bacterial 16S rRNA-encoding and the host GAPDH-encoding gene (used as a host reference gene) was used as a proxy for bacterial density on appendages. We assumed that the higher the ratio of bacterial-to-host DNA, the higher the density of bacteria on the host appendage. All 15 samples were compared. Results were normalized versus the “-fold” value obtained in the coastal species Palaemon elegans in order to compare all appendages and species (Figure 4). Within a host species, values in the three appendages were within an order of magnitude (10-fold). The highest ratios were obtained for Rimicaris chacei and Mirocaris fortunata, with average 16S rRNA values representing 181 and 138 times those found in P. elegans. These values were an order of magnitude above those in Alvinocaris markensis and Rimicaris exoculata (15 and 10, respectively).
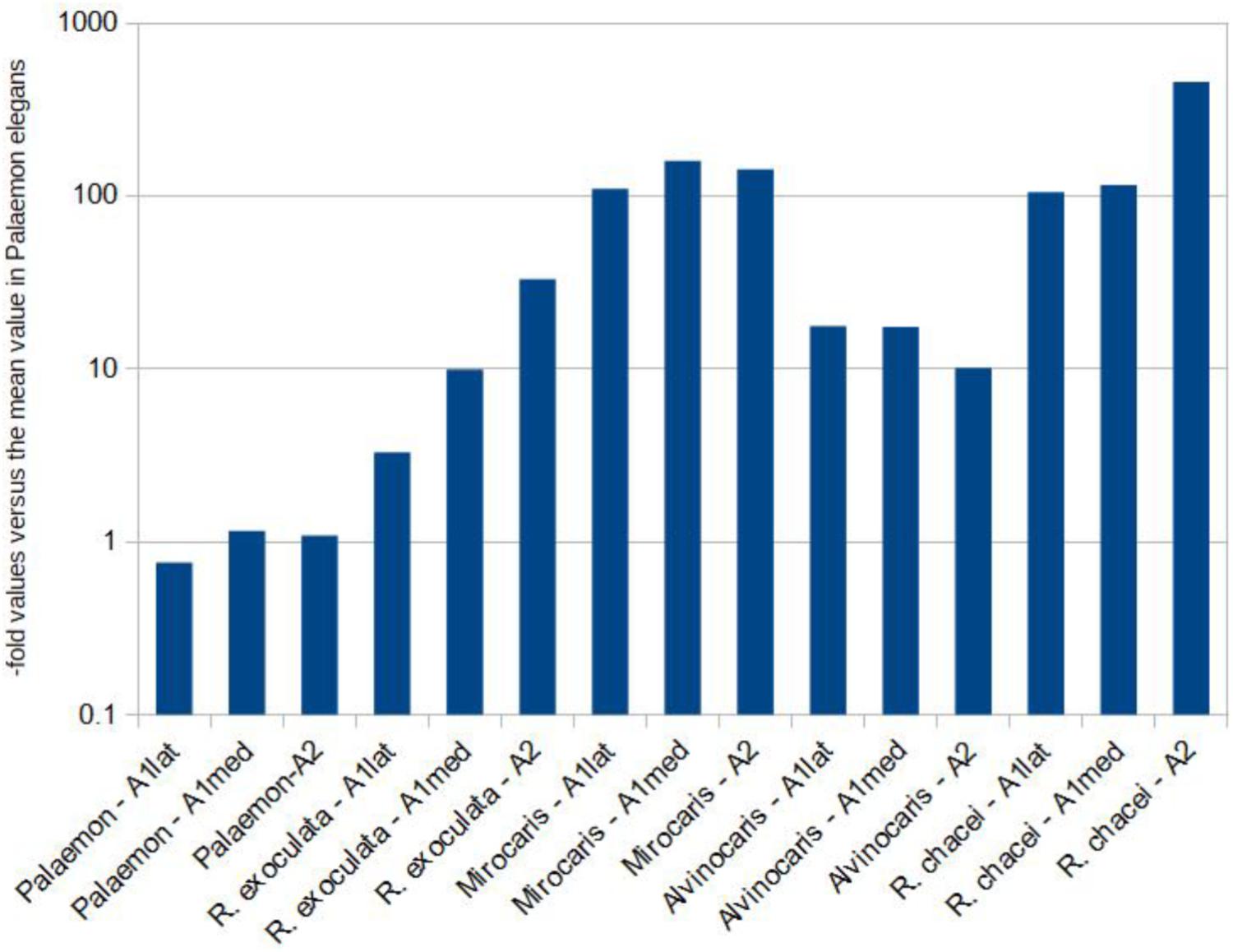
FIGURE 4. Results from the qPCR-based comparison among samples. The bacterial 16S rRNA gene was the target, and host GAPDH was used as a reference gene. Results are expressed as “-folds” versus the average value obtained from the coastal species Palaemon elegans in order to compare the results among samples. Notice the logarithmic y-axis.
Diversity of Bacterial Communities of the Antennules and Antennae
A total of 59,225,649 raw sequences were obtained from the 14 samples comprising the antenna, the medial and the lateral flagella of antennules of five host species. No sequence was obtained from the antenna (A2) of Alvinocaris markensis. After quality filtering, 30,534,420 were retained. They clustered into 4,550 variants averaging 500 bp length. Out of these, 99 variants were considered abundant as they displayed a relative abundance above 1% in at least one of the samples (Supplementary Material S1), and were representing a total of 24,786,444 sequences (81.2%). Ten variants were considered dominant because they represented at least 10% of the reads in at least one sample, overall representing 15,845,985 sequences (63.9%). Between 0.14 and 2.89% of the reads were unassigned.
Rarefaction curves indicate that the coverage was sufficient to properly assess the community composition in all samples (Supplementary Material S2). The highest numbers of observed variants were obtained for Palaemon elegans, and the lowest in Rimicaris exoculata, the two extreme numbers of variants differing by a ratio of 8.3 (Table 1). The highest values of the Shannon Diversity index were also obtained for P. elegans, suggesting more heterogeneous communities, while lowest values obtained for Rimicaris exoculata and Alvinocaris markensis suggest a more homogeneous bacterial community (Table 1). Within a given shrimp species, the diversity levels observed and the indexes (observed variants and Shannon index) of the three samples (medial and lateral flagella of the antennules, and antennae) were overall similar (Table 1). Palaemon elegans displayed notably higher observed variants (1,105–1,711 versus 205–649) and Shannon index (7.75–8.18 versus 3.94–5.53, respectively) compared to vent species altogether.
Comparison of Bacterial Communities Among Appendages and Species
The principal coordinates analysis plot based on unweighted UniFrac distances shows that the bacterial communities associated with the three appendages of a given shrimp species (i.e., their medial flagella, the lateral flagella of the antennule, and the antennae), tend to cluster together, suggesting overall similar compositions (Figure 5). The first and second axes (together representing 56.7% of the variance) clearly separated several groups: P. elegans and A. markensis were well separated from the rest. The three other species displayed more similar communities, with Rimicaris chacei and R. exoculata being the closest. Differences exist between community compositions among species [PERMANOVA test, 14 samples, 5 groups, 999 permutations, pseudo-F = 6.71, p < 0.001 (unweighted UniFrac), pseudo-F = 86.96, p < 0.001 (weighted UniFrac)]. Only six variants were shared among the five species, and additional 11 were shared among the four species from hydrothermal vents (Figure 6). A. markensis and R. exoculata shared the highest number of variants with 126 sequences, out of which 62 were unique to these two species. As a comparison, between 166 and 2,862 variants were unique to a single host shrimp species, indicating a relatively modest level of inter-species overlap at the variants level of resolution.
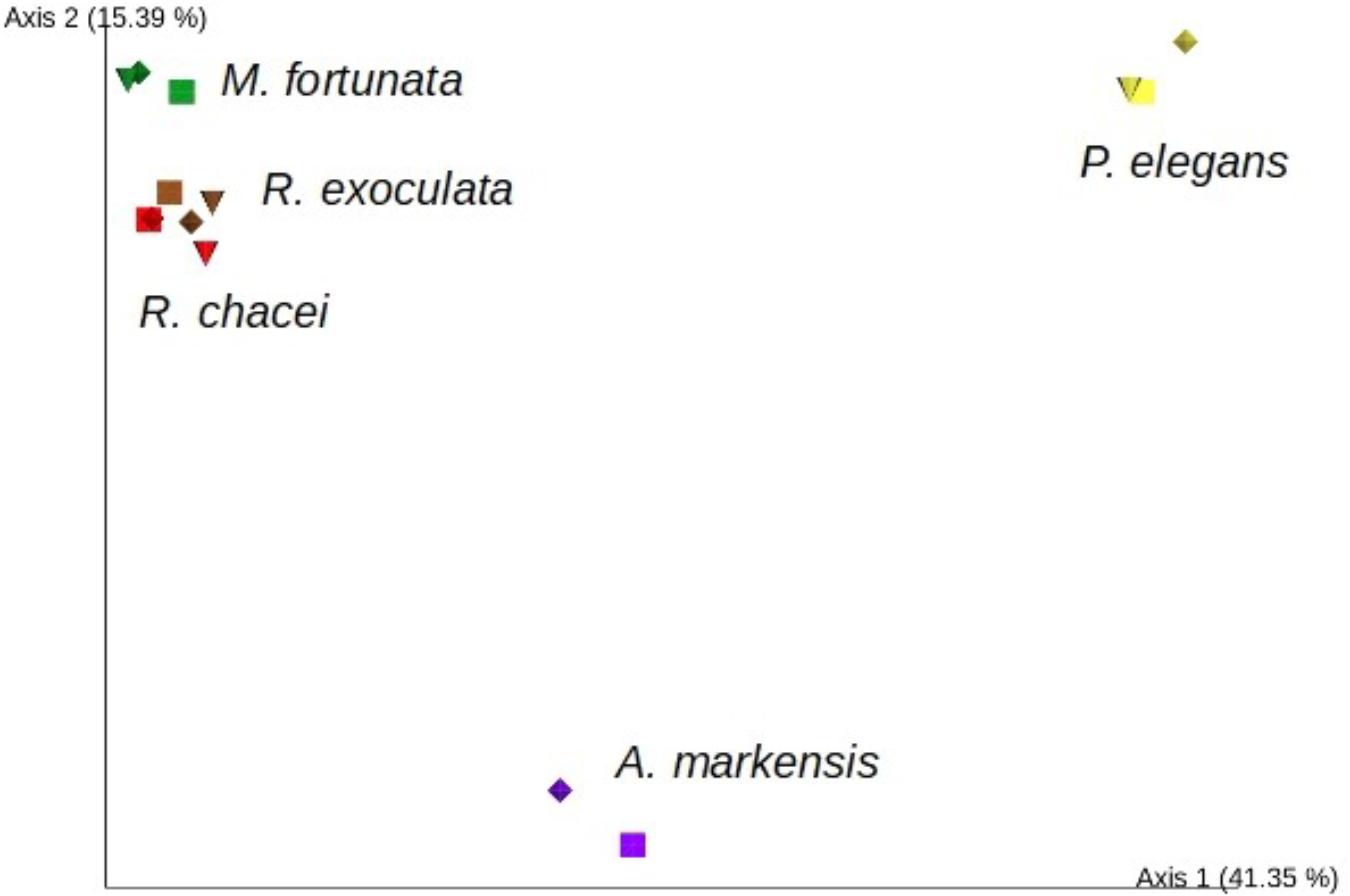
FIGURE 5. Principal coordinates analysis, 2D plot of the distribution of samples from Alvinocaris markensis (purple), Rimicaris chacei (red), Mirocaris fortunata (green), Palaemon elegans (yellow), and Rimicaris exoculata (brown) according to the composition of associated bacterial communities estimated by 16S rRNA metabarcoding. Symbols correspond to the different appendages, namely A1lat (diamonds), A1med (square) and A2 (triangle). Axes 1 and 2 explained 41.35 and 15.39% of the observed variance, respectively.
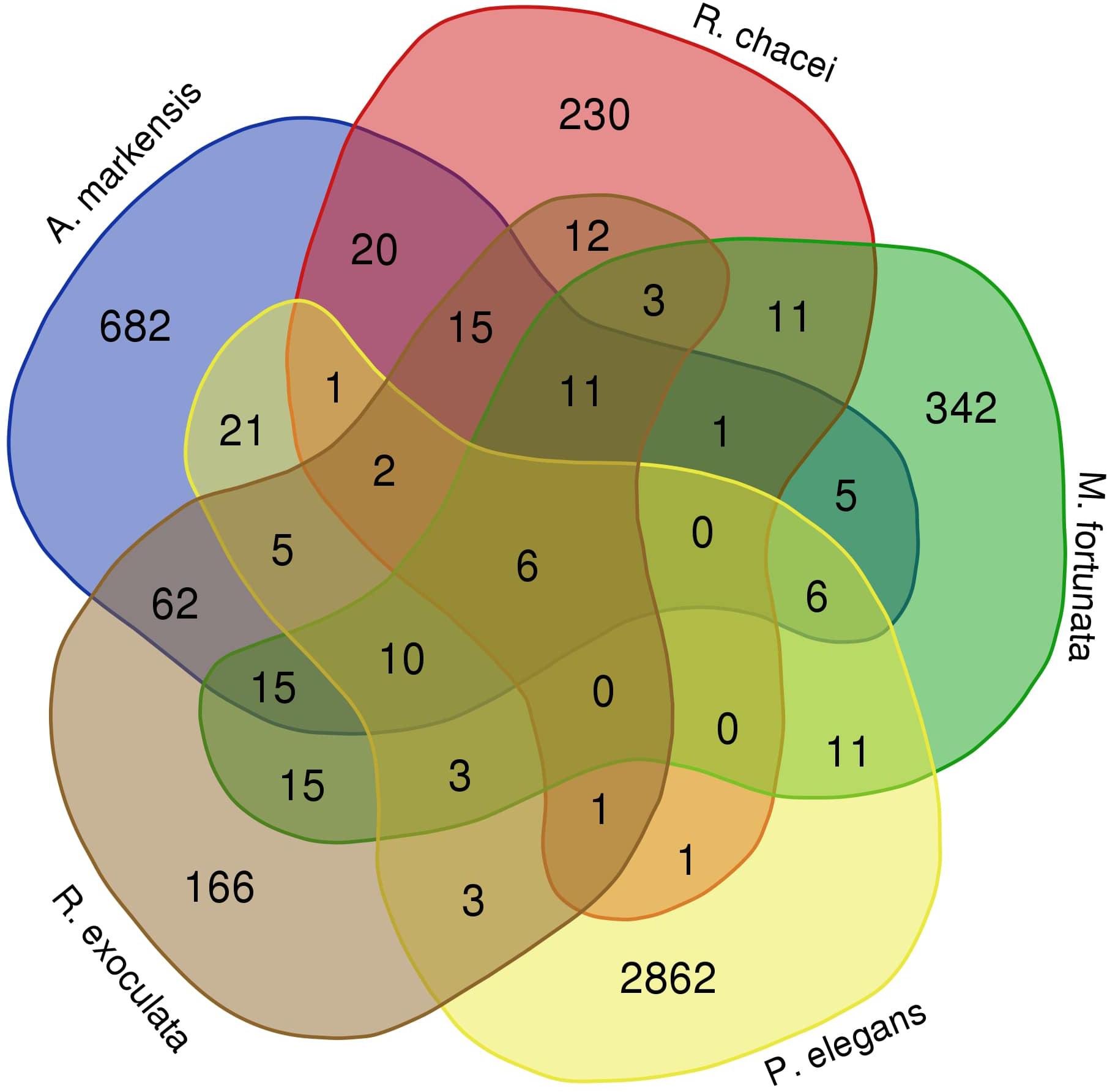
FIGURE 6. Venn diagram displaying the number of variants shared among the five species from this study. This diagram includes all variants with abundances at least equal to two reads.
Taxonomic Composition of Bacterial Communities and Dominant Variants
Datasets of variants obtained for bacterial communities associated with the antennules and antennae of Palaemon elegans were dominated by members of the Alphaproteobacteria (31.0–40.0% depending on appendage, Figure 7) and Bacteroidetes (41.8–47.2%). Epsilonproteobacteria dominated the datasets obtained from the four vent species, representing the majority of the reads in Rimicaris exoculata (84.6–90.5%) and Mirocaris fortunata samples (67.1–80.0%). In R. chacei and Alvinocaris markensis, Epsilon- and Gammaproteobacteria reads were present in similar relative abundances (respectively, 37.3–53.3% and 36.9–47.2%).
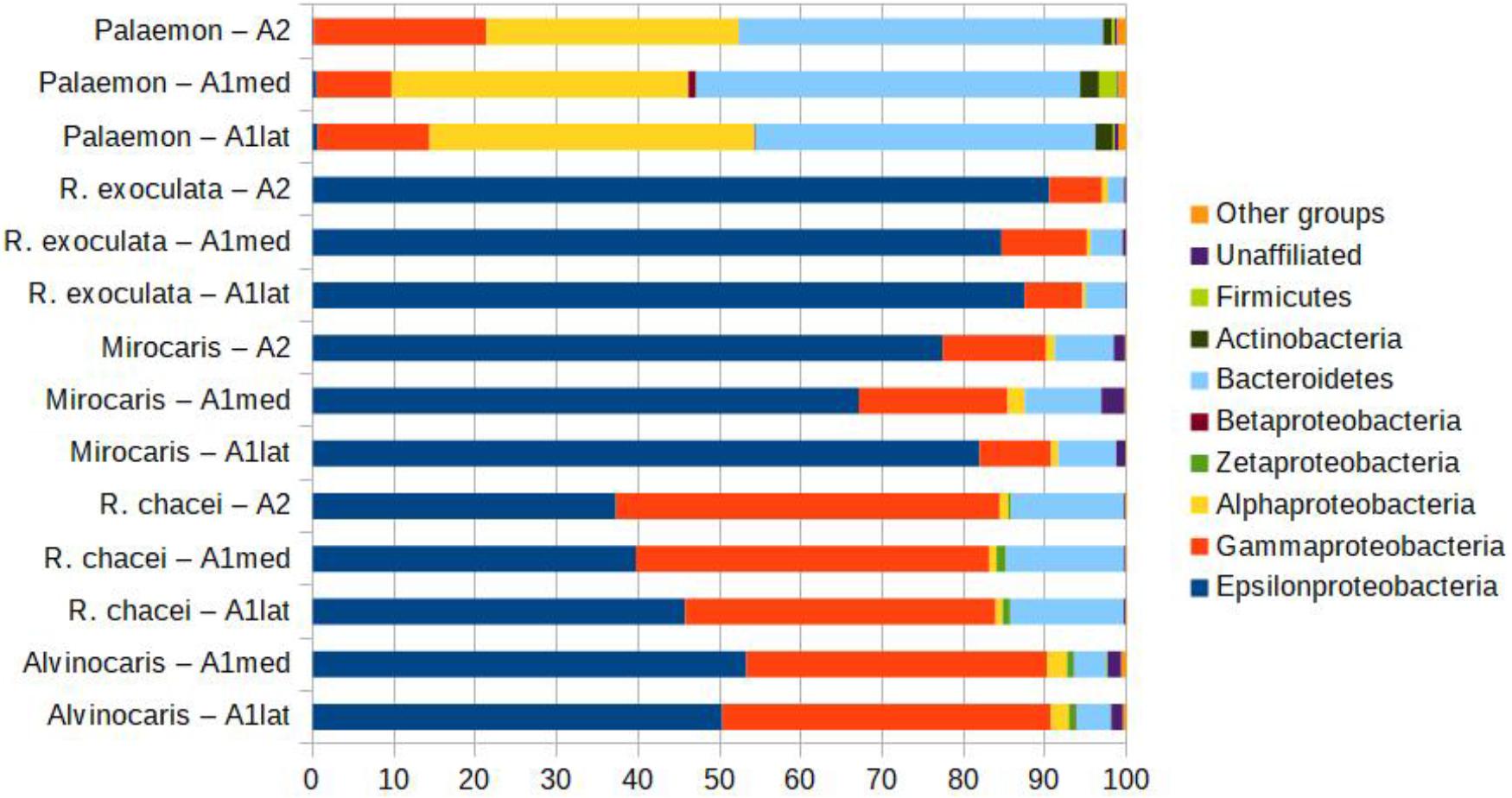
FIGURE 7. Relative abundances of the different bacterial taxa in libraries obtained from the different appendages of the five shrimp species, showing the dominance of a limited number of groups. The category ‘other groups’ correspond to the groups that represent below 1% of the reads in all samples.
Within the whole dataset, 10 variants were considered dominant because they represented at least 10% of the reads in at least one of the 14 samples. They are listed alongside their best BLAST hits in Table 2. None of these was abundant (>1%) in P. elegans. In hydrothermal vent shrimp, the different samples from a given host species shared the same dominant variants in roughly similar abundances, and the dominant variants belong to the Epsilon- and Gammaproteobacteria (8 and 2, respectively). In R. exoculata samples, between 48 and 56.2% of the sequences belonged to one of three variants, namely 1, 2, and 4. According to a phylogenetic reconstruction, these are closely related and they cluster with various epsilonproteobacterial sequences obtained from the gill chamber of R. exoculata (Table 2 and Supplementary Material S3). In M. fortunata, two closely related variants (namely 5 and 8) altogether represented between 21.5 and 25.3% of the reads. They are similar to sequences from vent Epsilonproteobacteria including Rimicaris epibionts (Table 2). With the notable exception of variant 10, which is identical to the sulfur-oxidizing gill endosymbiont of the MAR vent mussels Bathymodiolus azoricus and B. puteoserpentis, all dominant variants are highly similar to sequences of gill chamber epibionts of arthropods (R. exoculata, R. chacei or the galatheid Kiwa sp.), which appear among their best BLAST hits (Table 2). Although eight of the dominant variants occurred (i.e., above 0 reads) in at least another sample, only variant 1 from R. exoculata was abundant (>1%) in another species, namely M. fortunata (Table 2).
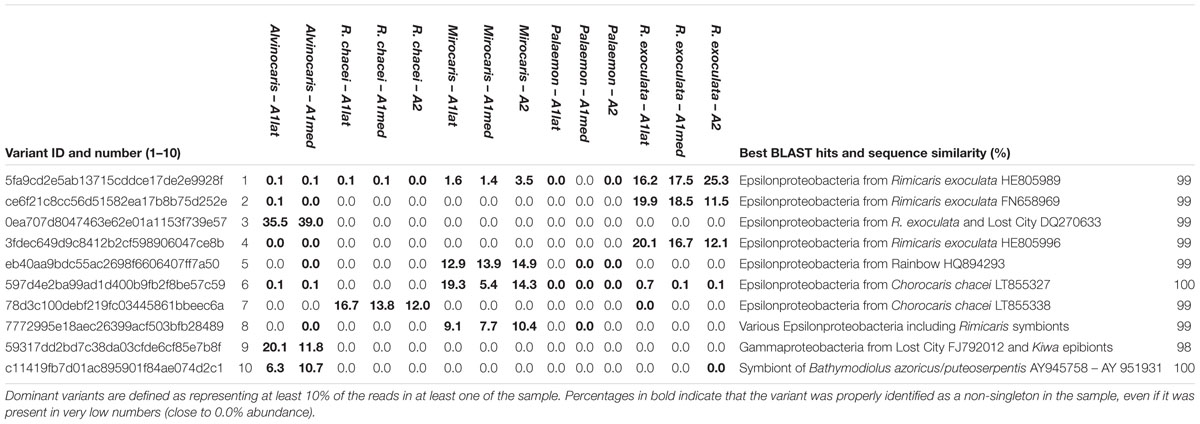
TABLE 2. Percentages of the reads corresponding to the dominant variants identified in this study, best BLAST hits and sequence similarities.
Discussion
Hydrothermal Vent Shrimp Harbor Dense Bacterial Communities on Their Antennules and Antennae
In a previous study describing for the first time the chemosensory organs of hydrothermal shrimp (Zbinden et al., 2017), some of us pointed out that bacteria profusely colonized the antennae and antennules of several hydrothermal vent shrimp (i.e., Rimicaris exoculata, Rimicaris chacei, Mirocaris fortunata, and Alvinocaris markensis). The occurrence of filamentous bacteria had also previously been reported on the antennules and antennae of R. chacei by Casanova et al. (1993). The present study confirms these preliminary observations, highlighting bacterial populations on the antennae and antennules of the four hydrothermal shrimp species. Present in varying quantities depending on the species and the individuals, bacteria can completely cover the antennae or antennules including the whole surface of chemosensory sensilla, and especially the aesthetascs, specialized in olfaction. A stark contrast exists with the coastal species Palaemon elegans for which only anecdotical bacterial coverage was observed. The higher bacterial densities observed under the electron microscope, in particular in M. fortunata and R. chacei, compared to other species, are in agreement with the qPCR results. Indeed, using the bacterial-to-host DNA ratios estimated by qPCR, M. fortunata and R. chacei appendages display values an order of magnitude above those obtained in R. exoculata and A. markensis, and two orders of magnitude above those in P. elegans. This supports that densest bacterial communities occur in M. fortunata and R. chacei, the less dense in P. elegans, whereas R. exoculata and A. markensis are somewhere in between. Different bacterial groups harbor different numbers of copies of their 16S rRNA gene, so qPCR results need to be taken with great caution as they provide only rough estimates of bacterial densities, and by no means true quantification (Větrovský and Baldrian, 2013). However, in this study we used qPCR to compare bacterial densities among shrimp antennae, and the fact that the species displaying the highest ratios, namely M. fortunata and R. chacei, are those for which SEM suggested the densest bacterial cover (and vice versa with P. elegans) supports the hypothesis that the former actually display denser communities. Inter-species and inter-individual differences could be species-specific, possibly related to the molt cycle. A quick molt cycle was estimated for R. exoculata (Corbari et al., 2008), which renews its cuticle twice as fast as a coastal shrimp of the same size (in 10 days for R. exoculata vs. 21 for Penaeus japonicus, Corbari et al., 2008). In many crustaceans, a high molting rate is considered as an antifouling mechanism allowing the regular elimination of epibiotic bacteria (Bauer, 1989), however, the re-colonization process (at least for the ectosymbionts of the cephalothorax) is very fast in R. exoculata and the bacterial community is completely renewed within 2 days after the molt (Corbari et al., 2008). The molt stage would thus be of little influence as an antifouling mechanism and on the inter-species and inter-individual variations herein observed.
Grooming Behavior in Hydrothermal Vent Shrimp
When their exchange surfaces (i.e., gills or olfactory organs) are subjected to fouling by microbial and micro- or macroscopic organisms, crustaceans frequently wipe and groom them with specialized appendices (Bauer, 1989). Indeed important microbial fouling was shown to be potentially detrimental to the structural integrity of the antennules, and presumably to its functional role as a chemoreceptor organ (Barbato and Daniel, 1997). In the four hydrothermal species, we did not observe any structural damage that could be linked to this bacterial coverage, even on the most colonized samples. Moreover, while R. exoculata first pereopods were shown to lack the grooming apparatus necessary to clean its antennae (Komai and Segonzac, 2008), the three other hydrothermal species possess well-developed antennal grooming apparatus on the carpus of the first pereopod. The four species also possess functional pairs of third maxillipeds that should allow the grooming of the antennules (Komai and Segonzac, 2003, 2005, 2008). They should thus be able to efficiently clean these appendages.
Direct in situ observations usually do not allow to observe grooming behavior in hydrothermal shrimp due the high mobility of the animals. We nevertheless performed some observations of isolated individuals of Mirocaris fortunata and Rimicaris exoculata in aquaria (authors observation from incubation experiments performed on other specimens during the same cruise). Grooming behavior was seen in the former, but not in the latter. Grooming behavior thus seem to be present in at least M. fortunata, however, it does not prevent a significant bacterial colonization.
To test whether colonization of the antennae is specific to this part of the body, we observed the surface of the cuticle elsewhere on the shrimp (i.e., the branchiostegites). These body parts also exhibit bacterial colonization. Even if bacterial colonization occurs on other body parts, the very particular role of antennae and antennules in sensory perception raises the question of the presence of these bacteria, of their potential impact on olfaction, and their possible role in shrimp physiology.
Sensory Capacities in Hydrothermal Vent Shrimp
Detection of odorant molecules was tested on Rimicaris exoculata (Renninger et al., 1995) and Mirocaris fortunata (Machon et al., 2018) through electrophysiology approaches. These studies showed that these two species can detect sulfide and organic stimuli (such as bacterial homogenates or dead shrimp extract), but no clear behavioral response could be highlighted (Machon and Ravaux, personal communication). If dense bacterial colonization really impairs the olfactory perception, the occurrence of the bacterial coverage on hydrothermal shrimp antennae and antennules can plead for a secondary role of this sense at adulthood, and rather suggests that the hydrothermal shrimp uses other abilities to orient in their environment. Shrimp might detect the dim light emitted by vents (Pelli and Chamberlain, 1989) through their highly modified eyes; they might detect temperature gradients created by the mixing of the hot hydrothermal fluid and the cold background seawater (2–3°C) (Segonzac et al., 1993; Ravaux et al., 2009); shrimp might detect acoustic vibrations induced by hydrothermal fluid emissions (Segonzac et al., 1993). Crustacean hearing abilities and reaction to acoustic or hydrodynamic vibrations, involving antennal or antennular mechanoreceptors, have previously been demonstrated (Lovell et al., 2005). Crone et al. (2006) recorded the sounds emitted by black smokers and suggest that vent sounds may provide some local organisms with navigational cues. To explain the occurrence of bacteria on the antennae and antennules, we can also raise the hypothesis that the bacteria have a specific role for the shrimp, which could thus cultivate them on purpose.
Bacterial Diversity and Potential Metabolisms
Bacterial communities associated with the three investigated appendages, namely the medial and lateral flagella of the antennules and the antennae, display similar taxonomic composition within a given species. This suggests that these structures are being colonized by the same types of bacteria. None of the tested appendages seems to display a different response to bacterial colonization (for example the exclusion of bacteria via appendage-specific grooming by the shrimp). Communities from the four vent species are on the other hand very different from those found in the non-vent species Palaemon elegans. Only six variants are shared between the two groups, and despite that four of the 10 variants that are dominant in vent shrimp occur in P. elegans, they represent negligible number of reads in the latter. Higher Shannon Diversity index suggests a more even distribution of variant abundances in P. elegans, as does the absence of any dominant variant. Communities from vent shrimp are on the other hand dominated by a limited number of variants belonging to the Epsilon- and Gammaproteobacteria. These are highly similar or identical to sequences already reported as crustacean epibionts in previous studies on Rimicaris exoculata gill chamber, on R. chacei, and on the cuticle of the galatheid Kiwa sp. (Zbinden et al., 2008; Goffredi, 2010; Petersen et al., 2010; Apremont, 2017). Epsilonproteobacteria are among the most efficient colonizers at vents and are very abundant as free-living bacteria as well as epibionts of metazoans (Corre et al., 2001; Lopez-Garcia et al., 2003; Nakagawa et al., 2005). Those associated with the R. exoculata gill chamber were shown to oxidize reduced sulfur and hydrogen and use the energy to fix carbon using the reverse TCA cycle based on direct experiments, as well as metagenomics-based approaches that confirmed the presence of relevant genes (Zbinden et al., 2008; Hügler et al., 2011; Jan et al., 2014). Gammaproteobacteria epibionts of R. exoculata and Kiwa sp. similar to those from the present study are also reported as autotrophic sulfur- and hydrogen-oxidizers that use the Calvin–Benson–Bassham cycle for carbon fixation, while some may instead use methane (Goffredi et al., 2008; Zbinden et al., 2008; Hügler et al., 2011; Jan et al., 2014). A particularly interesting finding is the identification of a variant, dominant in Alvinocaris markensis, which sequence is identical to that of the sulfur-oxidizing gill endosymbiont of the vent mussels Bathymodiolus puteoserpentis and B. azoricus. It may correspond to the free-living form of the symbiont, already suspected to occur on shell-associated biofilms and on an annelid (Petersen et al., 2012), or to a non-symbiotic close relative. Whatever is its status as a potential mussel symbiont, it is likely that it can perform sulfur-oxidation-fuelled autotrophy. Overall, it can be concluded that the most dominant bacteria found on the sensory appendages of vent shrimp are similar to bacteria already documented to engage in associations with metazoans, that have the ability to perform autotrophy and sulfur oxidation. The fact that different host shrimp species display different community compositions dominated by different bacterial OTUs is harder to interpret at this stage, given the limited dataset, but may be linked to their specific sites or microhabitats. The two species that display the most similar communities, namely R. exoculata and R. chacei (see Figure 5), both live in close proximity to the vent fluid emissions and both harbor symbionts in their cephalothorax from which they derive their organic carbon. Mirocaris fortunata and A. markensis live further away from fluids, likely experiencing different locale conditions.
Do Bacteria Have a Specific Role for Shrimp?
Based on their unexpectedly high densities, their localization close to chemosensory organs, and their hypothesized metabolisms, the bacterial communities identified on sensory organs of vent shrimp may play several roles. Some episymbioses are indeed reported to confer protection or defense from predators or the environment itself, while other are nutritional (Goffredi, 2010). At hydrothermal vents, major recognized roles for metazoan-associated bacteria are nutrition through chemosynthesis and detoxication (Haddad et al., 1995; Jan et al., 2014). Sulfide is abundant in the vent fluids, and necessary to the metabolism of symbionts on which Rimicaris exoculata, and partially R. chacei, rely for their nutrition (Gebruk et al., 2000; Ponsard et al., 2013). But it is also toxic to animal cells, in particular by interfering with the cytochrome oxidases in mitochondria (Powell and Somero, 1986). Sulfide removal by the dominant bacteria may thus reduce sulfide levels in the vicinity of host sensory cells and confer a certain protection. Another possible important role could be chemosynthetic primary production. Carbon molecules produced by gill chamber epibionts have been shown to be transferred through the thin (0.5 μm) inner branchiostegite cuticle of R. exoculata, providing trans-tegumental nutrition (Ponsard et al., 2013). The very thin cuticle of the aesthetascs sensilla (0.5–1.5 μm at the apex in M. fortunata), as well as the pore-like structures occurring in the aesthetasc cuticle (Machon et al., 2018) could permit such transfer, allowing host cells to benefit from bacterial primary production. This would be of limited significance to the whole animal, but could be significant for nutrition of sensory cells. In other contexts, bacteria are known to produce volatile odorants that contribute to host odor or other recognition cues, such as mate choice or social cohesion (Archie and Theis, 2011). Other, rarer, bacterial OTUs may thus have major influence on the system thanks to their metabolisms, emitting odorant molecules perceived by the olfactory receptor neurons of the shrimp, but these are much more difficult to demonstrate.
Besides this direct influence, bacterial communities could influence shrimp in other ways. The localization of bacteria places them at the interface between the organism and its immediate environment. Dense bacterial communities coat the surface of appendages. This coating could, to a certain extent, isolate the appendage from the environment, and could be filtering the thermal information (thermal insulation), as well as vibrations (shock absorption). Bacteria could also alter the chemical cues that actually reach the physically close host sensory cells through selective removal or release of chemical substances. This could influence the way environmental cues are perceived by the nervous system, with eventual consequences on host behavior. Microbiote influence on chemical signals is well documented and should not be under-estimated (Archie and Theis, 2011). The physical proximity between bacterial communities and sensory cells provides a potential route for direct molecule transfer from bacteria to the shrimp nervous system. Overall, the possible influence of bacterial epibionts on the sensory apparatus of vent shrimp cannot be ignored and should be considered in further studies dealing with sensory organs.
Author Contributions
MZ conceived the study on sensory perception in hydrothermal shrimp, performed SEM observations, and drafted the paper. AG and JM performed SEM observations. KS performed molecular analyses. JR conceived the study on sensory perception in hydrothermal shrimp. NL performed BM analyses. SD performed molecular analyses and drafted the paper. MZ, AG, KS, JM, JR, and SD participated in data analysis and wrote the manuscript.
Funding
This work was supported by the European Union Seventh Framework Programme (FP7/2007–2013) under the MIDAS project (grant agreement no. 603418), and by the Institut Universitaire de France, project ACSYMB.
Conflict of Interest Statement
The authors declare that the research was conducted in the absence of any commercial or financial relationships that could be construed as a potential conflict of interest.
Acknowledgments
We thank the chief scientist of the Biobaz, 2013 (F. Lallier) and the Bicose, 2014 (MA Cambon-Bonavita) cruises, as well as the captain and crew of the research vessels and the ROV Victor and Nautile teams for their efficiency. We also thank Jozée Sarrazin and Nicolas Rabet for hydrothermal and coastal shrimp sampling, respectively. SEM was performed at the electronic microscopy platform of the Institute of Biology Paris-Seine (IBPS) with the help of Virginie Bazin, and at the ‘Plateau technique de Microscopie Electronique’ du Museum national d’Histoire naturelle (MNHN) with the help of Géraldine Toutirais and Chakib Djediat.
Supplementary Material
The Supplementary Material for this article can be found online at: https://www.frontiersin.org/articles/10.3389/fmars.2018.00357/full#supplementary-material
MATERIAL S1 | Sequences and percentages of the reads corresponding to the abundant variants identified in this study (sheet 1), and most similar sequences based on a RDP SeqMatch search (sheet 2). Dominant variants are defined as representing at least 1% of the reads in at least one of the sample.
MATERIAL S2 | Rarefaction curves displaying the number of variants identified for a given sampling effort for each appendage and each shrimp species, averaged from 10 iterations. The maximum sampling effort (1,400,000) corresponds to the minimum number of sequences that passed the quality filters applied in this study (see Table 1).
MATERIAL S3 | Phylogenetic analysis of the 10 dominant variants (labeled by a blue dot) by Maximum Likelihood method, based on the General Time Reversible model. Gamma distribution was used to model evolutionary rate differences among sites [five categories (+G, parameter = 0.4512)]. The rate variation model allowed for some sites to be invariable [(+I), 31.8% sites]. All positions with less than 95% site coverage were eliminated. A total of 471 positions were kept in the final dataset. Scale bar represents 10% estimated base substitution.
References
Ache, B. (1982). “Chemoreception and thermoreception,” in The Biology of Crustacea, Vol. Vol. 3, ed. D. Bliss (New York, NY: Academic Press), 369–398. doi: 10.1016/B978-0-12-106403-7.50017-3
Apremont, V. (2017). Description de la Diversité Microbienne Associée à Chorocaris Chacei: Une Possible Double Symbiose. Ph.D. thesis, Université de Bretagne Occidentale, Brest.
Archie, E., and Theis, K. (2011). Animal behaviour meets microbial ecology. Anim. Behav. 82, 425–436. doi: 10.1016/j.anbehav.2011.05.029
Barbato, J., and Daniel, P. (1997). Chemosensory activation of an antennular grooming behavior in the spiny lobster, Panulirus argus, is tunned narrowly to L-glutamate. Biol. Bull. 193, 107–115. doi: 10.2307/1542756
Bauer, R. (1977). Antifouling adaptations of marine shrimp (Crustacea: Decapoda: caridea): functional morphology and adaptive significance of antennular preening by third maxillipeds. Mar. Biol. 40, 261–277. doi: 10.1007/BF00390881
Bauer, R. (1989). “Decapod crustaceans grooming: functional morphology, adaptive value, and phylogenetic significance,” in Functional Morphology of Feeding and Grooming in Crustacea, eds B. Felgenhauer, L. Watling, and A. Thistle (Rotterdam: Balkema, AA), 49–73.
Böröczky, K., Wada-Katsumata, A., Batchelor, D., Zhukovskaya, M., and Schal, C. (2013). Insects groom their antennae to enhance olfactory acuity. PNAS 110, 3615–3620. doi: 10.1073/pnas.1212466110
Callaghan, B. J., McMurdie, P., Rosen, M. J., Han, A. W., Johnson, A. J. A., and Holmes, S. P. (2016). DADA2: High-resolution sample inference from Illumina amplicon data. Nat. Methods 13, 581–583. doi: 10.1038/nmeth.3869
Camacho-Jiméneza, L., Peregrino-Uriartea, A. B., Martínez-Quintana, J. A., and Yepiz-Plascenciaa, G. (2018). The glyceraldehyde-3-phosphate dehydrogenase of the shrimp Litopenaeus vannamei: molecular cloning, characterization and expression during hypoxia. Mar. Environ. Res. 138, 65–75. doi: 10.1016/j.marenvres.2018.04.003
Caporaso, G. J., Kuczynski, J., Stombaugh, J., Bittinger, K., Bushman, F. D., Costello, E. K., et al. (2010). QIIME allows abalysis of high-throughput community sequencing data. Nat. Methods 7, 335–336. doi: 10.1038/nmeth.f.303
Casanova, B., Brunet, M., and Segonzac, M. (1993). L’impact d’une épibiose bactérienne sur la morphologie fonctionnelle de crevettes associées à l’hydrothermalisme médio-Atlantique. Cah. Biol. Mar. 34, 573–588.
Corbari, L., Zbinden, M., Cambon-Bonavita, M.-A., Gaill, F., and Compère, P. (2008). Bacterial symbionts and mineral deposits in the branchial chamber of the hydrothermal vent shrimp Rimicaris exoculata: relationship to moult cycle. Aquat. Biol. 1, 225–238. doi: 10.3354/ab00024
Corre, E., Reysenbach, A., and Prieur, D. (2001). ê-Proteobacterial diversity from a deep-sea hydrothermal vent on the Mid-Atlantic Ridge. FEMS Microbiol. Lett. 205, 329–335.
Cottin, D., Shillito, B., Chertemps, T., Thatje, S., Léger, N., and Ravaux, J. (2010). Comparison of heat-shock responses between the hot vent shrimp Rimicaris exoculata and the related coastal shrimp Palaemonetes varians. J. Exp. Mar. Biol. Ecol. 393, 9–16. doi: 10.1016/j.jembe.2010.06.008
Crone, T., Wilcock, W., Barclay, A., and Parsons, J. (2006). The sound generated by mid-ocean ridge black smoker hydrothermal vents. PLoS One 1:e133. doi: 10.1371/journal.pone.0000133
Derby, C., Kozma, M., Senatore, A., and Schmidt, M. (2016). Molecular mechanisms of reception and perireception in custacean chemoreception: a comparative review. Chem. Senses 41, 381–398. doi: 10.1093/chemse/bjw057
Desbruyères, D., Almeida, A., Biscoito, M., Comtet, T., Khripounoff, A., Le Bris, N., et al. (2000). A review of the distribution of hydrothermal vent communities along the northern Mid-Atlantic Ridge: dispersal vs. environmental controls. Hydrobiologia 440, 201–216. doi: 10.1023/A:1004175211848
Dubilier, N., Bergin, C., and Lott, C. (2008). Symbiotic diversity in marine animals : the art of harnessing chemosynthesis. Nat. Rev. Microbiol. 6, 725–740. doi: 10.1038/nrmicro1992
Gebruk, A., Southward, E., Kennedy, H., and Southward, A. (2000). Food sources, behaviour, and distribution of hydrothermal vent shrimp at the Mid-Atlantic Ridge. J. Mar. Biol. Assoc. 80, 485–499. doi: 10.1017/S0025315400002186
Goffredi, S. (2010). Indigenous ectosymbiotic bacteria associated with diverse hydrothermal vent invertebrates. Environ. Microbiol. Rep. 2, 479–488. doi: 10.1111/j.1758-2229.2010.00136.x
Goffredi, S., Jones, W., Ehrlich, H., Springer, A., and Vriejenhoek, C. (2008). Epibiotic bacteria associated with the recently discovered Yeti crab, Kiwa hirsuta. Environ. Microbiol. 10, 2623–2634. doi: 10.1111/j.1462-2920.2008.01684.x
Haddad, A., Camacho, F., Durand, P., and Cary, S. (1995). Phylogenetic characterization of the epibiotic bacteria associated with the hydrothermal vent polychaete Alvinella pompejana. Appl. Environ. Microbiol. 61, 1679–1687.
Hügler, M., Petersen, J., Dubilier, N., Imhoff, J., and Sievert, S. (2011). Pathways of carbon and energy metabolism of the epibiotic community associated with the deep-sea hydrothermal vent shrimp Rimicaris exoculata. PLoS One 6:e16018. doi: 10.1371/journal.pone.0016018
Jan, C., Petersen, J., Werner, J., Huang, S., Teeling, H., Glöckner, F., et al. (2014). The gill chamber epibiosis of deep-sea shrimp Rimicaris exoculata: an in-depth metagenomic investigation and discovery of Zeta Proteobacteria. Environ. Microbiol. 16, 2723–2738. doi: 10.1111/1462-2920.12406
Kamio, M., and Derby, C. (2017). Finding food: how marine invertebrates use chemical cues to track and select food. Nat. Prod. Rep. 34, 514–528. doi: 10.1039/c6np00121a
Komai, T., and Segonzac, M. (2003). Review of the hydrothermal vent shrimp genus Mirocaris, redescription of M. fortunata and reassessment of the taxonomic status of the family Alvinocarididae (Crustacea: Decapoda: Caridea). Cah. Biol. Mar. 44, 199–215.
Komai, T., and Segonzac, M. (2005). A revision of the genus Alvinocaris williams and Chace (Crustacea: Decapoda: Caridea: Alvinocarididae), with descriptions of a new genus and a new species of Alvinocaris. J. Nat. Hist. 39, 1111–1175. doi: 10.1080/00222930400002499
Komai, T., and Segonzac, M. (2008). Taxonomic review of the hydrothermal vent shrimp genera Rimicaris Williams and Rona and Chorocaris Martin and Hessler (Crustacea: Decapoda; Caridea: Alvinocarididae). J. Self. Res. 27, 21–41.
Le Bris, N., Govenar, B., Le Gall, C., and Fisher, C. (2006). Variability of physico-chemical conditions in 9 degrees 50′ NEPR diffuse flow vent habitats. Mar. Chem. 98, 167–182. doi: 10.1016/j.marchem.2005.08.008
Lopez-Garcia, P., Duperron, S., Phillipot, P., Foriel, J., Susini, J., and Moreira, D. (2003). Bacterial diversity in hydrothermal sediment and epsilon proteobacterial dominance in experimental microcolonizers at the Mid-Atlantic Ridge. Environ. Microbiol. 5, 961–976. doi: 10.1046/j.1462-2920.2003.00495.x
Lovell, J., Findlay, M., Moate, R., and Yan, H. (2005). The hearing abilities of the prawn Palaemon serratus. Comp. Biochem. Physiol. A 140, 89–100. doi: 10.1016/j.cbpb.2004.11.003
Lozupone, C., and Knight, R. (2005). UniFrac: a new phylogenetic method for comparing microbial communities. Appl. Environ. Microbiol. 71, 8228–8235. doi: 10.1128/AEM.71.12.8228-8235.2005
Machon, J., Lucas, P., Ravaux, J., and Zbinden, M. (2018). Comparison of chemoreceptive abilities of the hydrothermal shrimp Mirocaris fortunata and the coastal shrimp Palaemon elegans. Chem. Senses 43, 489–501. doi: 10.1093/chemse/bjy041
Nakagawa, S., Takai, K., Inagaki, F., Hirayama, H., Nunoura, T., Horikoshi, K., et al. (2005). Distribution, phylogenetic diversity and physiological characteristics of epsilon-Proteobacteria in a deep-sea hydrothermal field. Environ. Microbiol. 7, 1619–1632. doi: 10.1111/j.1462-2920.2005.00856.x
Pelli, D., and Chamberlain, S. (1989). The visibility of 350°C black-body radiation by the shrimp Rimicaris exoculata and man. Nature 337, 460–461. doi: 10.1038/337460a0
Petersen, J., Ramette, A., Lott, C., Cambon-Bonavita, M.-A., Zbinden, M., and Dubilier, N. (2010). Dual symbiosis of the vent shrimp Rimicaris exoculata with filamentous gamma- and epsilon proteobacteria at four Mid-Atlantic Ridge hydrothermal vent fields. Environ. Microbiol. 12, 2204–2218. doi: 10.1111/j.1462-2920.2009.02129.x
Petersen, J. M., Wentrup, C., Verna, C., Knittel, K., and Dubilier, N. (2012). Origins and evolutionary flexibility of chemosynthetic symbionts from deep-sea animals. Biol. Bull. 223, 123–137. doi: 10.1086/BBLv223n1p123
Ponsard, J., Cambon-Bonavita, M.-A., Zbinden, M., Lepoint, G., Joassin, A., Corbari, L., et al. (2013). Inorganic carbon fixation by chemosynthetic ectosymbionts and nutritional transfers to the hydrothermal vent host-shrimp, Rimicaris exoculata. ISME J. 7, 96–109. doi: 10.1038/ismej.2012.87
Powell, M., and Somero, G. (1986). Adaptations to sulfide by hydrothermal vent animals: sites and mechanisms of detoxification and metabolism. Biol. Bull. 171, 274–290. doi: 10.2307/1541923
Quast, C., Pruesse, E., Yilmaz, P., Gerken, J., Schweer, T., Yarza, P., et al. (2013). The SILVA ribosomal RNA gene database project: improved data processing and web-based tools. Nucl. Acids Res. 41, D590–D596. doi: 10.1093/nar/gks1219
Ravaux, J., Cottin, D., Chertemps, T., Hamel, G., and Shillito, B. (2009). Hydrothermal vent shrimp display low expression of heat-inducible hsp70 gene in nature. Mar. Ecol. Prog. Ser. 396, 153–156. doi: 10.3354/meps08293
Renninger, G., Kass, L., Gleeson, R., Van Dover, C., Battelle, B., Jinks, R., et al. (1995). Sulfide as a chemical stimulus for deep-sea hydrothermal vent shrimp. Biol. Bull. 189, 69–76. doi: 10.2307/1542456
Sarradin, P., Caprais, J., Riso, R., and Kerouel, R. (1999). Chemical environment of the hydrothermal mussel communities in the lucky strike and menez gwen vent fields, Mid Atlantic ridge. Cah. Biol. Mar. 40, 93–104. doi: 10.1016/j.marenvres.2008.02.015
Sarrazin, J., Juniper, K., Massoth, G., and Legendre, P. (1999). Physical and chemical factors influencing species distributions on hydrothermal sulfide edifices of the Juan de Fuca Ridge,Northeast Pacific. Mar. Ecol. Prog. Ser. 190, 89–112. doi: 10.3354/meps190089
Schmitt, B., and Ache, B. (1979). Olfaction: responses of a decapod crustacean are enhanced by flicking. Science 205, 204–206. doi: 10.1126/science.205.4402.204
Segonzac, M., de Saint-Laurent, M., and Casanova, B. (1993). L’énigme du comportement trophique des crevettes Alvinocarididae des sites hydrothermaux de la dorsale médio-atlantique. Cah. Biol. Mar. 34, 535–571.
Vereshchaka, A. L., Kulagin, D. N., and Lunina, A. A. (2015). Phylogeny and new classification of hydrothermal vent and seep shrimps of the family Alvinocarididae (Decapoda). PLoS One 10:e0129975. doi: 10.1371/journal.pone.0129975
Větrovský, T., and Baldrian, P. (2013). The variability of the 16S rRNA gene in bacterial genomes and its consequences for bacterial community analyses. PLoS One 8:e57923. doi: 10.1371/journal.pone.0057923
Wang, Y., and Qian, P. Y. (2009). Conservative fragments in bacterial 16SrRNA genes and primer design for 16S ribosomal DNA amplicons in metagenomic studies. PLoS One 4:e7401. doi: 10.1371/journal.pone.0007401
Watanabe, K., Kodama, Y., and Harayama, S. (2001). Design and evaluation of PCR primers to amplify bacterial 16S ribosomal DNA fragments used for community fingerprinting. J. Microbiol. Methods 44, 253–262. doi: 10.1016/S0167-7012(01)00220-2
Zbinden, M., Berthod, C., Montagné, N., Machon, J., Léger, N., Chertemps, T., et al. (2017). Comparative study of chemosensory organs of shrimp from hydrothermal vent and coastal environments. Chem. Senses 42, 319–331. doi: 10.1093/chemse/bjx007
Zbinden, M., Le Bris, N., Gaill, F., and Compère, P. (2004). Distribution of bacteria and associated minerals in the gill chamber of the vent shrimp Rimicaris exoculata and related biogeochemical processes. Mar. Ecol. Prog. Ser. 284, 237–251. doi: 10.3354/meps284237
Zbinden, M., Shillito, B., Le Bris, N., De Vilardi de Montlaur, C., Roussel, E., Guyot, F., et al. (2008). New insights on the metabolic diversity among the epibiotic microbial community of the hydrothermal shrimp Rimicaris exoculata. J. Exp. Biol. Ecol. 159, 131–140. doi: 10.1016/j.jembe.2008.03.009
Keywords: hydrothermal, shrimp, Alvinocarididae, chemosensory perception, antennules, bacteria, grooming
Citation: Zbinden M, Gallet A, Szafranski KM, Machon J, Ravaux J, Léger N and Duperron S (2018) Blow Your Nose, Shrimp! Unexpectedly Dense Bacterial Communities Occur on the Antennae and Antennules of Hydrothermal Vent Shrimp. Front. Mar. Sci. 5:357. doi: 10.3389/fmars.2018.00357
Received: 27 June 2018; Accepted: 18 September 2018;
Published: 08 October 2018.
Edited by:
David William Waite, University of Auckland, New ZealandReviewed by:
Adrian Ochoa-Leyva, Universidad Nacional Autónoma de México, MexicoPatrick Joseph Kearns, Tufts University, United States
Copyright © 2018 Zbinden, Gallet, Szafranski, Machon, Ravaux, Léger and Duperron. This is an open-access article distributed under the terms of the Creative Commons Attribution License (CC BY). The use, distribution or reproduction in other forums is permitted, provided the original author(s) and the copyright owner(s) are credited and that the original publication in this journal is cited, in accordance with accepted academic practice. No use, distribution or reproduction is permitted which does not comply with these terms.
*Correspondence: Sébastien Duperron, c2ViYXN0aWVuLmR1cGVycm9uQG1uaG4uZnI=