- 1Laboratório de Biologia Pesqueira e Manejo dos Recursos Aquáticos, Universidade Federal do Pará, Belém, Brazil
- 2Laboratório de Ictiologia, Departamento de Oceanografia e Ecologia, Universidade Federal do Espírito Santo, Vitória, Brazil
- 3Instituto de Biologia, Universidade Federal da Bahia, Salvador, Brazil
- 4Laboratório de Ecologia Marinha, Universidade Vila Velha, Vila Velha, Brazil
Intertidal zones shelter a wealth of species and natural resources, provide important ecological services, and sustain several economic activities in coastal communities. However, the tidepool fish species that inhabit the intertidal zone are subject to a wide array of impacts due to the human presence and their accessibility, creating a challenge for the mitigation of habitat loss, in particular in tropical regions where the ecology and distribution of species are poorly known. In this study, we investigated tidepool fish species distribution patterns systematically across ca. 4,900 km of tropical Brazilian coastline (00–21∘ latitude) in order to verify the latitudinal trends and environmental variables influencing tidepool communities. A total of 5,113 fish specimens belonging to 67 taxa were collected at the 19 sites, revealing four distinct biogeographic subprovinces: Amazon Estuary (AE), Northern Mangrove (NM), Northeastern Semiarid (NS), and Tropical Warm (TW). Distance-based linear modeling evidenced in sequence water salinity, tidal range, shape of rocky shore formation, algae cover, distance to subtidal zone, latitude and rainfall as the most important environmental variables to shape biogeographic subprovinces. Fish species such as Bathygobius soporator, Bathygobius geminatus, Labrisomus nuchipinnis, and Scartella cristata presented wide distribution, occupying more than one subprovince. The trophic structure of the tidepool fishes also varied among subprovinces, with carnivores being associated with the AE subprovince, omnivores with the NM, and herbivores dominating the NS and TW. These findings reinforce the determining role of local and regional factors in the geographic distribution of fish and, in particular, highlight a new arrangement for the intertidal subprovinces of the tropical Brazilian shoreline, which may provide a valuable tool for the more effective management and conservation of this vulnerable ecosystem at the land-ocean interface.
Introduction
The latitudinal distribution patterns of aquatic and terrestrial organisms have been discussed by ecologists worldwide in an attempt to understand large-scale patterns and predict human-made impacts on wildlife (Mittelbach et al., 2007; Schemske et al., 2009; Wiens, 2015; Chaudhary et al., 2016; Cutter and Gray, 2016; Pecl et al., 2017). The ecological dynamics shaping natural populations are also dependent on processes at a local scale (Vilar et al., 2013; Heffernan et al., 2014; Elahi et al., 2015). This scale is especially important in the case of the intertidal zone, which comprises a harsh, but biologically productive habitat where exposure to sunlight, wave, and tidal processes create a highly dynamic habitat in which conditions fluctuate substantially over time (Raffaelli and Hawkins, 1996; Zander et al., 1999). In this context, biogeographic provinces and subprovinces can be proposed in accordance with the variation in these environmental features and related differences in species composition (see Briggs and Bowen, 2012; Pinheiro et al., 2018). In the beginning of this century some important global studies have addressed the challenge in proposing biogeographic arrangements and successfully contributed on this issue in terrestrial (Olson et al., 2001), freshwater (Abell et al., 2008) and marine realms (Spalding et al., 2007).
Historically, tidepool fishes studies have focused primarily on inventories and small-scale spatial patterns (Bennett and Griffiths, 1984; Nieder, 1993; Mahon and Mahon, 1994; Gibson and Yoshiyama, 1999; Castellanos-Galindo et al., 2005; Griffiths et al., 2006; Macieira and Joyeux, 2011), given that large-scale, biogeographic questions are more difficult to assess due to the variation among studies in sampling procedures, and the absence of a standardized sampling protocol (Prochazka et al., 1999). Since the publication of ‘Intertidal fishes’ by Horn et al. (1999), latitudinal (biogeographic) patterns of intertidal fishes have only been investigated, on a major spatial scale, by Griffiths (2003) and Harasti et al. (2016), in Australia, and Arakaki et al. (2014), in Japan. Intertidal fish communities may vary in response to latitude (see Harasti et al., 2016), however, local factors (e.g., substrate composition, water temperature, tidepool morphology, and height above sea level) may also have a strong influence on the distribution of fish species (Mahon and Mahon, 1994; Arakaki and Tokeshi, 2011; Macieira and Joyeux, 2011; White et al., 2015), even in a large-scale setting (Arakaki et al., 2014). Even so, the paucity of the data available for this vulnerable environment, which is at risk from both local and global impacts, is a major drawback (Helmuth et al., 2002, 2006; Thompson et al., 2002; Halpern et al., 2008; Vinagre et al., 2016; Andrades et al., 2017, 2018). In general, the large-scale distribution of species can be explained by dispersal capacity and geographic patchiness (Macieira et al., 2015), while community patterns at a local scale are driven strongly by local environmental characteristics, such as substrate type, the morphology of tidepools, and oceanographic features (Gibson and Yoshiyama, 1999; Cox et al., 2011).
As local habitat features can influence communities to the degree that they affect regional arrangements, it is imperative understand the underlying processes that determine these patterns (Ricklefs, 1987; Rivadeneira et al., 2002; Arakaki et al., 2014; Navarrete et al., 2014). The processes include biological interactions, such as herbivory, predation, and competition, as well as the effects of the physical environment (Horn and Ojeda, 1999; Arakaki and Tokeshi, 2011; Arakaki et al., 2014; Ahmadia et al., 2018). Given this, we will also attempt to understand how these regulatory mechanisms influence the organization of communities, in particular in tropical regions, where there is a scarcity of primary data. In the tropical Brazilian seascape, recent studies have investigated large-scale patterns in estuarine and reef fishes distributions, as well as the influence of local and regional factors (Vilar et al., 2013; Pinheiro et al., 2018). These studies have identified a number of local factors that structure fish assemblages on the Brazilian coast, but only Pinheiro et al. (2018) has discussed in detail the role of ecological and evolutionary processes in the fish distribution and proposed a set of biogeographic subprovinces based on the existing configuration of the Brazilian Province (sensu Briggs and Bowen, 2012). As intertidal habitats on the tropical Brazilian coastline are used by subtidal marine and estuarine fish species, also as nursery habitats or during their whole life-cycle (Macieira and Joyeux, 2011; Andrades et al., 2018), it is reasonable to expect that the intertidal fish fauna is highly influenced by local factors, resulting in more heterogeneous and complex biogeographic patterns than previously observed in reef fishes (Pinheiro et al., 2018).
We conducted our study in Brazilian tropical coast to verify the spatial shifts in the structure of tidepool fish assemblages in response to intertidal biological, physical, and physicochemical variables at a large-scale, while also considering local-scale variables. In addition, we predicted four subprovinces for tropical Brazilian intertidal coastline that have confirmed under both environmental and biological variables from 19 sites (0°–21° latitude).
Materials and Methods
Study Area
The Brazilian coast extends over ca. 7,490 km, from 4° N to 34° S (Figure 1), with an enormous diversity of climatic, geomorphologic, oceanographic, and ecologic features. As the ecological dynamics of classic rocky shores and flat intertidal reefs are distinct (Mahon and Mahon, 1994; Macieira and Joyeux, 2011; Andrades et al., 2018), the present study surveyed sites between 0°S and 21°S, which encompasses the transition of intertidal flat reefs to rocky shore (slope is present).
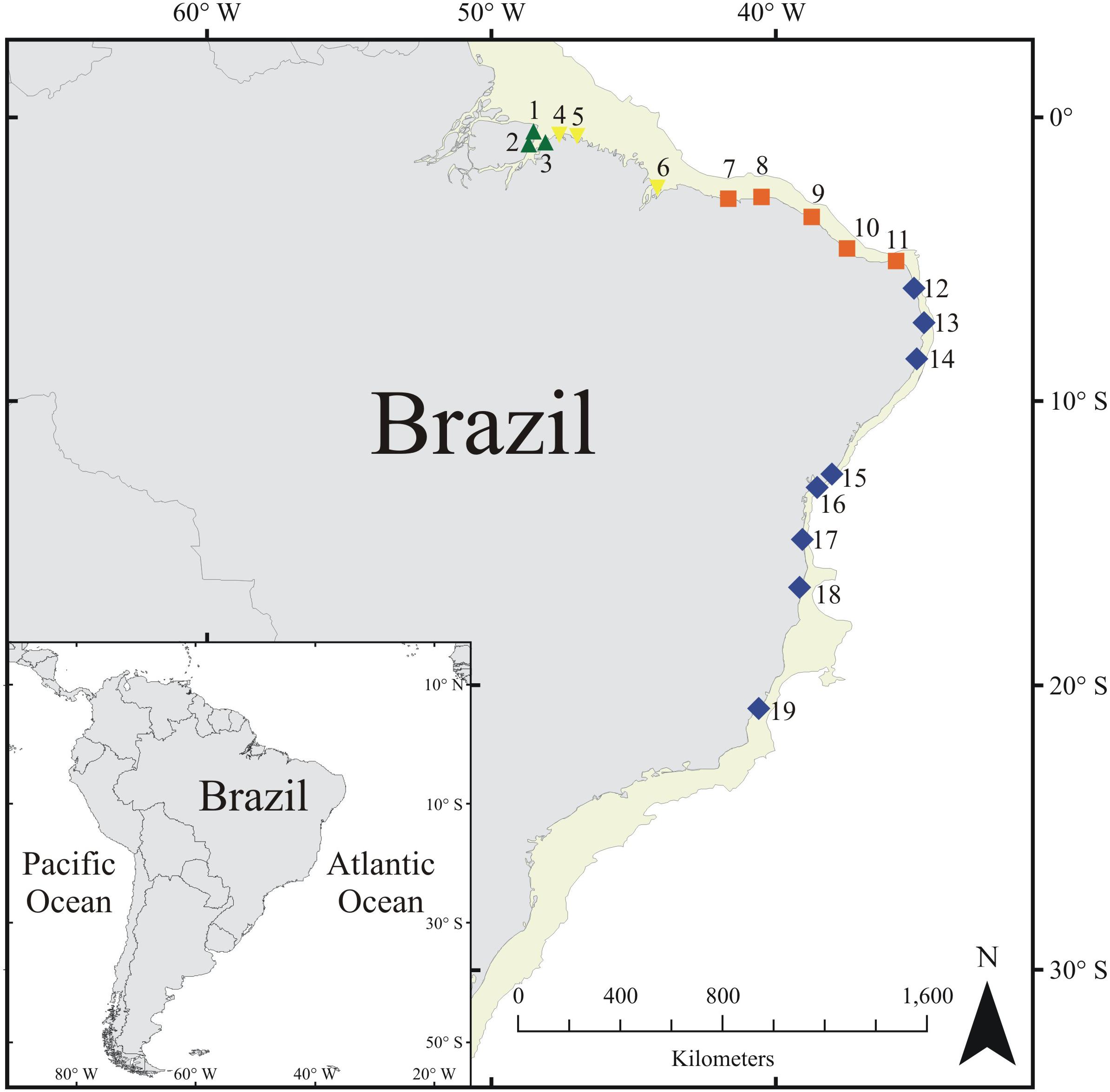
FIGURE 1. The 19 sites sampled in our study. Each symbol and color denotes one of the four Brazilian intertidal subprovinces identified here; Amazon estuary (AE) = green triangles, Northern mangrove (NM) = yellow inverted triangles, Northeastern semiarid (NS) = orange squares, and Tropical warm (TW) = blue diamonds.
The northern coast has a humid tropical climate (Köppen Am type), while the northeast region is divided in two main climates, a tropical coast recording dry summer (semi-arid) (Köppen As type) and another section recording humid tropical type (Köppen Af and Aw types), and subtropical climate dominating southern coast (Köppen Cfa and Cfb types) (Bernardes et al., 2012; Alvares et al., 2013). The Brazilian coast can be divided into four major geological sectors (Bernardes et al., 2012), the Northern Quaternary or Amazon plain (4° N to 3° S), Eastern Tertiary (3° to 20° S), Southeastern Granitic (20° to 29° S), and Southern Quaternary (29° to 34° S). The Brazilian coast is dominated by semi-diurnal tides with the greatest tidal range in the North region (>4 m), declining southward to less than 1 m (Dominguez, 2009). Three principal surface currents flow across the Brazilian Continental Shelf: the oligotrophic South Equatorial Current off the northeast coast (12–15° S), the North Brazil Current, which meets the Amazon plume off the northern coast, and the southward meandering Brazil Current off the eastern, southeastern, and southern coasts as far as its confluence with the Malvinas current (Peterson and Stramma, 1991; Campos et al., 1996). Based on this set of features, we divided the tropical intertidal Brazilian coast (0–21° S) into four major subprovinces (Table 1 and Figure 1):

TABLE 1. Summary of environmental characteristics found in the four subprovinces of the Brazilian coast.
(i) The Amazon estuary (AE) subprovince (0–1° S) is characterized by a strong riverine influence, with a run-off of ca. 180,000 m3s-1 representing 30% of the freshwater discharge into the Atlantic Ocean (Perry et al., 1996; Wisser et al., 2010). Turbid waters with mean annual salinity below 10 and a tidal range of up to 3–4 m. The intertidal areas are dominated by tropical freshwater swamp-forest, mud flats, mangrove forest, saltmarsh, and rock formations (see Machado et al., 2017). Mean water temperature is 26°C throughout the year, and mean annual rainfall is 2,800 mm.
(ii) The Northern mangrove (NM) subprovince (0–2° S) encompasses 23 estuaries and 30 freshwater basins, draining a total area of 330,000 km2 along a 480-km coastline (Kjerfve et al., 2002). Salinity varies considerably throughout the year, ranging from 15 to 35. Semidiurnal tides range up to 4–6 m, flooding an area of ca. 7,600 km2 of continuous mangrove forests (Souza-Filho, 2005). Mean annual temperature is 27°C and mean annual rainfall is 2,500 mm. This subprovince extends from eastern tip of the mouth of the southern channel of the Amazon estuary to Parnaíba coast (Delta do Parnaíba; 2°43′S), state of Piauí.
(iii) The Northeastern semiarid (NS) subprovince (2–5° S) comprises a coastline of 720 km dominated by mobile dune fields propelled by south-easterly and easterly trade winds, which reach speeds of over 15 m/s (Freire et al., 2008). Low riverine input and clear and salty waters (annual salinity range 32–42) are characteristics of this region, in addition to relatively reduced mangrove forest cover along the coastline in comparison with the AE and NM subprovinces. Small coral reefs and rock formations are common. Mean annual temperature is 27°C and mean annual rainfall is 989 mm. This subprovince extends to Rio Grande do Norte state (5°22′ S).
(iv) The Tropical warm (TW) subprovince (5–21° S) is a sector of coastline influenced by the Brazil Current, with a moderate continental run-off (Ekau and Knoppers, 1999; Leão and Dominguez, 2000). In this subprovince, tidal range decreases southward to 1–2 m, the coast is fringed by a mosaic-rich landscape, including coral, carbonate and rocky reefs (see Rosa et al., 1997; Macieira and Joyeux, 2011), as well as mangrove-reef ecotones, Atlantic rainforest, seagrass meadows, estuarine mud flats, and sandy beaches can be observed (Leão and Dominguez, 2000; Kjerfve et al., 2002). The mean annual temperature is 24°C and mean annual rainfall is 1,400 mm.
Sampling Design
A total of 19 sites (Figure 1), intertidal flat reefs, were sampled along ca. 4,900 km of the Brazilian coast. The distance between each site ranged from 10 to 200 km. Field sampling was conducted in a single season (March–June/2012) in order to minimize the effect of seasonal shifts in environmental data among sites. Eight tidepools (N = 152) were randomly chosen at each site to describe its fish assemblage. Sampling was always carried out during diurnal low tides in isolated tidepools (i.e., without connection to either the sea or other tidepools).
Environmental Variables
Water temperature (±0.1°C; mercury thermometer), salinity (±0.1; optical refractometer – Model: Q767), and pH (±1; ColorpHast tape) were recorded during the low tide in each tidepool immediately prior to the sampling procedures. The topography and bathymetry of the tidepools were surveyed using a 20 cm × 20 cm grid for the measurement of the depth, area, and volume of each tidepool. The area and volume were calculated by the kriging method. The type of substrate in each pool was assigned to one of four categories: (i) mud (silty substrate), (ii) sand (grain size < 1 mm), (iii) gravel (grain size ≤ 50 mm), and (iv) rock (solid aggregated substrate > 50mm). The substrate type and algal cover within each pool were estimated using a 50 cm × 50 cm measuring grid, subdivided internally into a 5 cm × 5 cm grid, which was used to estimate relative coverage. The rugosity index was calculated by the ratio of surface to planar area. The distances between the tidepool and the coastal plateau and subtidal areas were calculated with a tape measure, and height was measured as the vertical distance between water surface of the tidepool and sea level. The tidal range at each site was calculated as the difference between the maximum and minimum height of the water, recorded on the sampling day from Brazilian National Oceanographic Database (DHN, 2012). Rainfall data were based on the mean annual rainfall of the preceding 10 years (INMET, 2012) and the latitude was determined by GPS. The area (m2) and perimeter (m) of the rock formation of each intertidal landscape were calculated using satellite images. Rocky shore was assessed based on the method described by Burrough (1981) and Milne (1988), defined by the Fractal Dimension, or Frac (McGarigal and Marks, 1995): Frac = 2 ∗ ln [(P/4) / ln (A)], where P is the patch perimeter and A is the patch area. Lower Frac values indicate square or more structured shapes, while higher values indicate more irregular shapes. To assess the orientation of the rocky shore formation, the lengths of two axes (orthogonal – D1, and parallel – D2 to the coastline) were measured from image satellites in ArcGis software to determine the L1/L2 ratio. High ratio values indicate that the rocky intertidal shore is more orthogonal to the coast. The intertidal shores sampled in the present study were identified based on the lithological classification, as carbonate, ferruginous sandstone or sandstone. All 25 environmental variables determined in this study are described in Supplementary Table S1.
Tidepool Fish Fauna
The tidepool fish fauna was sampled using anesthetic clove oil (see Griffiths, 2000) and the specimens were fixed in 10% formalin solution, after being preserved in 70% alcohol. Density was calculated by the number of individuals per pool volume (m3). The fish taxa were assigned to one of three major feeding categories: Carn = carnivore; Omni = omnivore; Herb = herbivore, and the degree of residency was classified according to Macieira and Joyeux (2011), Oliveira et al. (2016), complemented by personal observations from the authors.
Data Analysis
Multivariate analyses were performed in PRIMER-E (following Clarke and Gorley, 2006). Firstly, the full dataset containing all 25 environmental variables (Supplementary Table S1) was tested for collinearity using a Draftsman plot and Spearman correlation matrix. Where pairs of variables had a correlation coefficient of 0.9 or more, one of the correlated variables was excluded from the analysis (Clarke and Ainsworth, 1993). Two pairs of variables (tidepool area vs. planar area, and perimeter of the rock formation vs. Frac) were highly correlated (r2 > 0.9), so one of each pair (tidepool area and perimeter of the rock formation) was omitted from the analysis, to avoid collinearity. Eight variables required transformation (e.g., log- or fourth root-transformation) to compensate for skew.
All the selected variables were standardized and a Principal Components Analysis (PCA) was used to evaluate their variation among sites and subprovinces. A permutational multivariate analysis was also used to test for differences in tidepool fish composition and environmental characteristics in the different “subprovinces” (4 fixed levels: Amazon estuary, Northern mangrove, Northeastern semiarid and Tropical warm), and “site,” nested within “subprovinces” factor, 19 random levels (see PERMANOVA; Anderson et al., 2008). Statistical significance was tested using 9,999 permutations of the residuals in a reduced model (according to Freedman and Lane, 1983) and the Type III (partial) sums of squares (see Anderson et al., 2008). In these analyses, the PERMANOVA was run on a Bray-Curtis similarity matrix, calculated from the fourth-root transformed biological data and a Euclidean similarity matrix for the environmental data (see Anderson, 2001; McArdle and Anderson, 2001). When the results were significant, post-hoc pair-wise contrasts were performed to establish where the differences in assemblage structure and environmental variables were found. In addition to the PERMANOVA, we conducted a Permutational Analysis of Multivariate Dispersions (PERMDISP) on the same Bray–Curtis matrix to test for differences in the multivariate dispersions of the “subprovinces” and “sites.”
The Similarity Percentages – species contributions (SIMPER; Clarke and Warwick, 2001) procedure was applied to reveal which fish species contributed most to the multivariate community patterns within the “subprovinces.” A constrained ordination technique, the Canonical Analysis of Principal Coordinates (CAP; Anderson and Robinson, 2003; Anderson and Willis, 2012), was performed to test the null hypothesis, given that no differences were found in the species composition among the “subprovinces” established a priori. A Spearman correlation of over 0.7 was used as an arbitrary limit for the display of the potential correlations between fish densities by feeding category and the canonical axes.
A Permutational Distance-based Linear Modeling (PERMDISP; Anderson, 2001; McArdle and Anderson, 2001) was used to test the relationship between fish assemblages and environmental variables. This procedure consists of a multivariate multiple regression method in which the ordination axes from a resemblance matrix of the response dataset (species composition) are regressed on a matrix of explanatory variables (the 23 environmental variables). A stepwise DISTLM routine was also run with 9,999 permutations selected using the Bayesian Information Criterion, BIC (Kuha, 2004). A distance-based redundancy analysis (dbRDA) was used as a constrained ordination technique to visualize the results of the DISTLM (Anderson et al., 2008).
Results
Environmental Variables
The PCA revealed a clear segregation of the four proposed intertidal subprovinces (Figure 2). Five of the components of the environmental data modeled approximately 61% of the total variation. The first principal component (PC1) explained 21.8% of the total variation, and clearly separated the equatorial (AE and NM) from the tropical (NS and TW) subprovinces, based on salinity, rainfall, and the environmental features of the tidepools. Except for rainfall and rock coverage, the environmental data were negatively correlated with PC2, explaining 13.8% of the total variation. Similarly, the PERMANOVA indicated significant differences among sites (p = 0.0001) and subprovinces (p = 0.0001; Supplementary Table S2), where “site” was the main effect, explaining 37.2% of the variability in the data, followed by “subprovince,” which explained 30.6%.
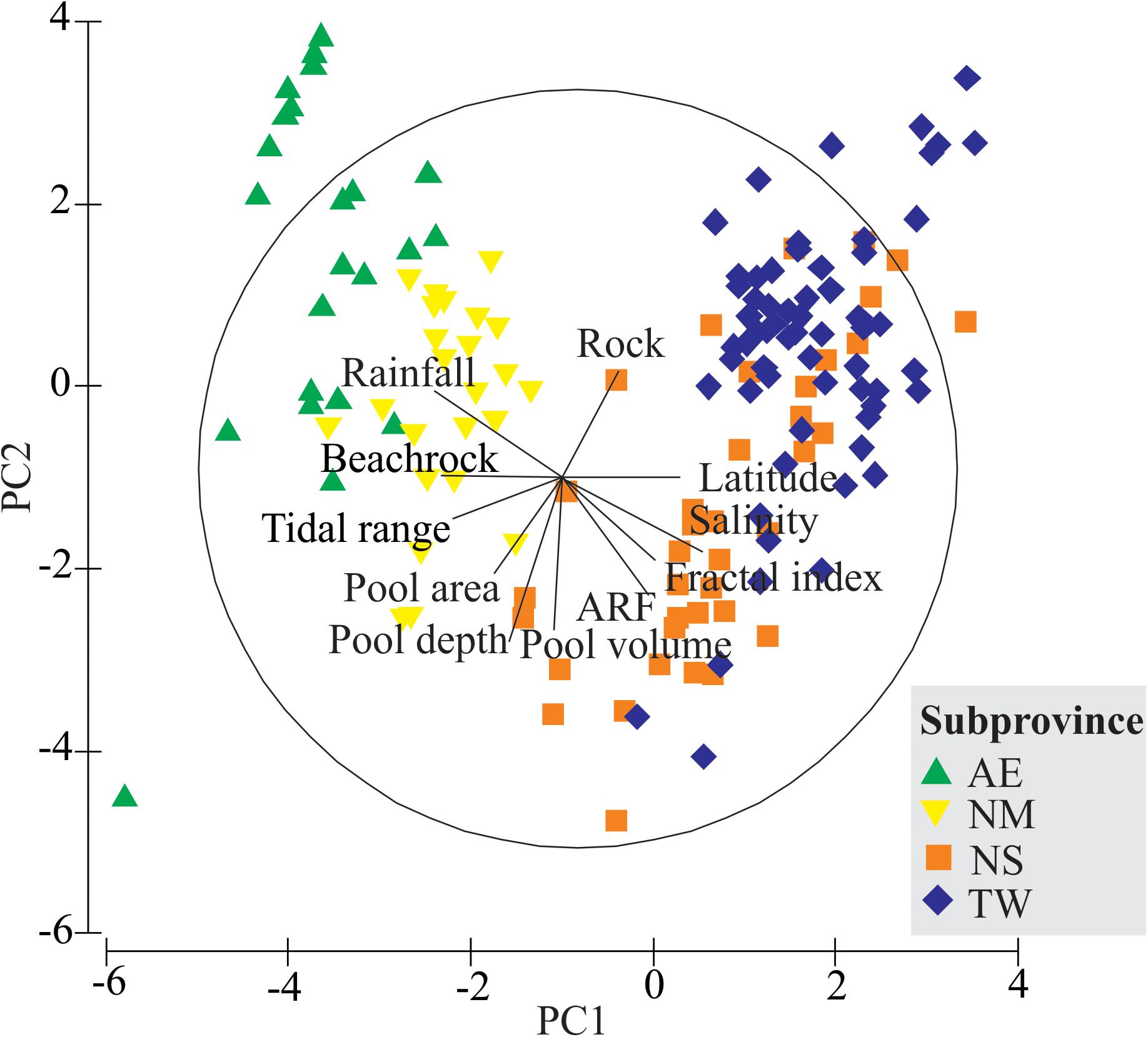
FIGURE 2. Principal Components Analysis (PCA) ordination constructed from the environmental data (Supplementary Tables S1, S2) of 149 tidepools sampled at 19 sites along the Brazilian coast. Only the variables with the highest loadings, positive or negative, are shown. The length and direction of the vectors represent the strength and direction of the relationship. The samples with no fish data (N = 3) were omitted from the analysis. Subprovinces: AE, Amazon estuary; NM, Northern mangrove; NS, Northeastern semiarid; TW, Tropical warm.
Tidepool Fish Fauna
A total of 5,113 fish, representing 67 taxa belonging to 27 families was sampled from the 152 tidepools studied (Supplementary Table S3). No fish were recorded in three tidepools. Scartella cristata was the most abundant species, accounting for 33.5% of all the individuals collected (1,309 ind.), followed by Bathygobius soporator, with 21.4% (899 ind.), and Bathygobius geminatus with 9.3% (388 ind.). The most representative families were Blenniidae (38.8%) and Gobiidae (14.7%), and only three families (Blenniidae, Gobiidae, and Batrachoididae) were recorded in all subprovinces (Supplementary Table S4). Species richness increased gradually from the AE to the TW subprovince, although a larger number of local endemics and exclusive species were recorded in the AE in comparison with the other subprovinces (Supplementary Table S5). Four species (B. soporator, B. geminatus, Labrisomus nuchipinnis, and S. cristata) were broadly distributed in the intertidal zone of the Brazilian coast, occurring in 17, 11 and 10 (both L. nuchipinnis and S. cristata) sites, respectively. By contrast, 28 taxa were restricted to a single site. Two species were endemic to subprovince AE (Gobiosoma sp. and Eleotris sp.), and one to NS (Paraclinus sp.).
The PERMANOVA explained 32.5% of the variation at the local scale (“site”) and 22.5% at the regional scale (“subprovince”), demonstrating the strong influence of local-scale factors shaping fish assemblages along the tropical Brazilian coast (p-values < 0.001; Supplementary Table S6). The PERMDISP analysis also revealed that the dispersion of the fish assemblages in tidepools varied significantly (F = 4.3169, p = 0.0003) across sites. The results of the SIMPER analysis indicated that B. soporator is typical of all four subprovinces, contributing most to similarity with the AE (94.79%). The most representative fish species in NM subprovince were Gobiesox barbatulus, Omobranchus punctatus, Barbulifer enigmaticus, Bathygobius soporator, Mugil curema, Genyatremus luteus, and Thalassophryne nattereri, whereas Scartella cristata, B. soporator, Abudefduf saxatilis, Lutjanus alexandrei, and Bathygobius geminatus were typical of the NS province. The TW subprovince was characterized by B. geminatus, S. cristata, Labrisomus nuchipinnis, B. soporator, Stegastes fuscus, and Ctenogobius boleosoma (Figure 3). Mean dissimilarity between subprovinces was 88.1, ranging from 81.8 for AE vs. NS to 96.2 for NM vs. TW.
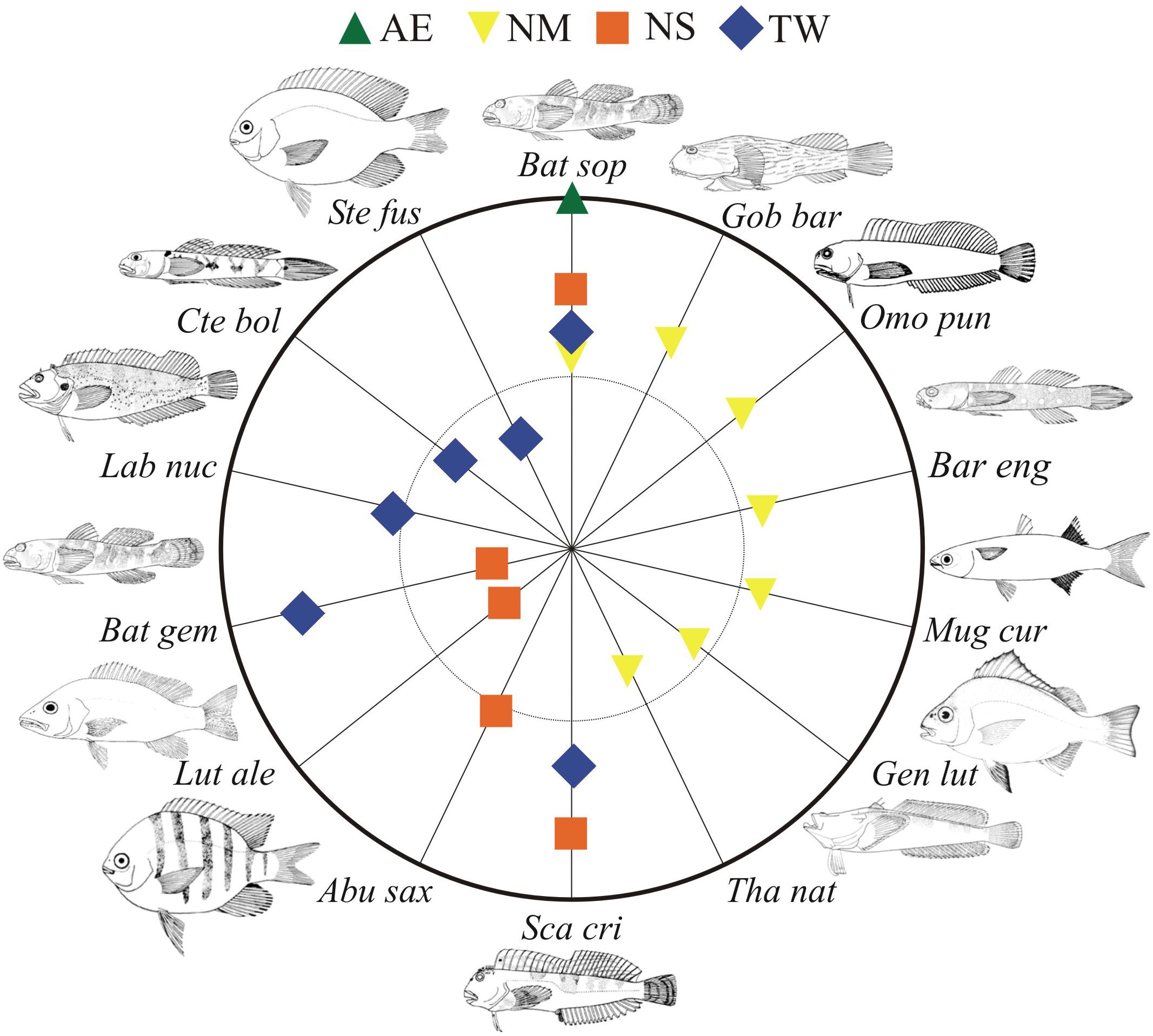
FIGURE 3. Percentage contributions (log scale; 0 – center, 10 – inner circle and 100 – outer circle) of the most important fish species to the typical “subprovinces,” based on the SIMPER analysis. Subprovinces: AE, Amazon estuary; NM, Northern mangrove; NS, Northeastern semiarid; TW, Tropical warm. Bat sop, Bathygobius soporator; Gob bar, Gobiesox barbatulus; Omo pun, Omobranchus punctatus; Bar eng, Barbulifer enigmaticus; Mug cur, Mugil curema; Gen lut, Genyatremus luteus; Tha nat, Thalassophryne nattereri; Sca cri, Scartella cristata; Abu sax, Abudefduf saxatilis; Lut ale, Lutjanus alexandrei; Bat gem, Bathygobius geminatus; Lab nuc, Labrisomus nuchipinnis; Cte bol, Ctenogobius boleosoma; Ste fus, Stegastes fuscus.
The Canonical Analysis of Principal coordinates (CAP; Figure 4) revealed a clear spatial trophic structure, with the fish taxa grouped in the four subprovinces (δ21 = 0.80, p = 0.0001). Overall, the leave-one-out allocation success was 82.5% (i.e., misclassification error of only 17.5%) for the “subprovince” factor. The CAP axis scores indicated higher herbivore densities associated with the TW and NS. Omnivorous fish were correlated with the NM and carnivores with the AE (Table 2).
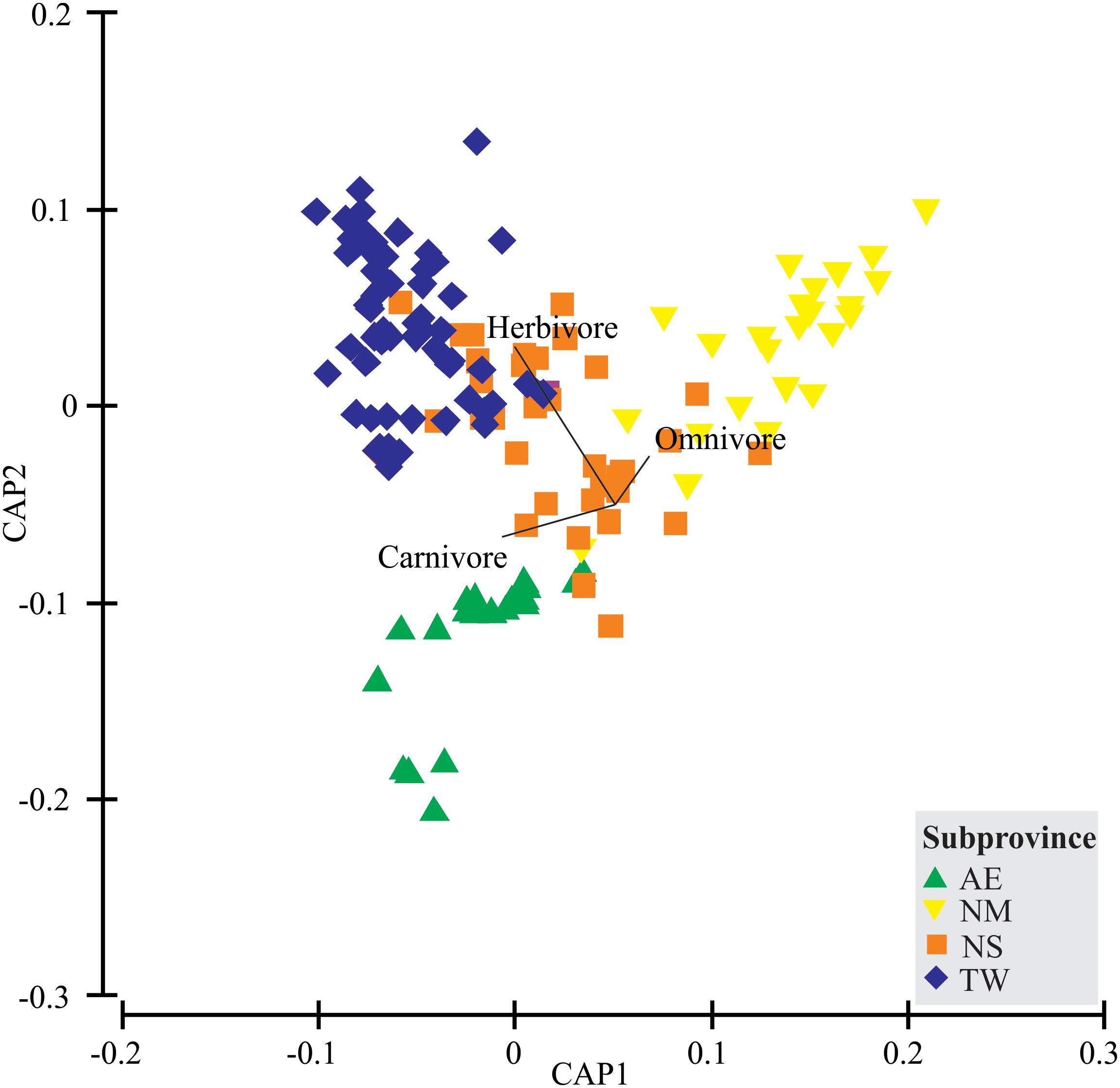
FIGURE 4. Plot of the canonical analysis of principal coordinates (CAP) generated from the Bray-Curtis similarity matrix of species composition using “subprovince” as the predictor factor. Vector overlay denotes feeding categories with a Spearman rank correlation of above 0.7. Subprovinces: AE, Amazon estuary; NM, Northern mangrove; NS, Northeastern semiarid; TW, Tropical warm.
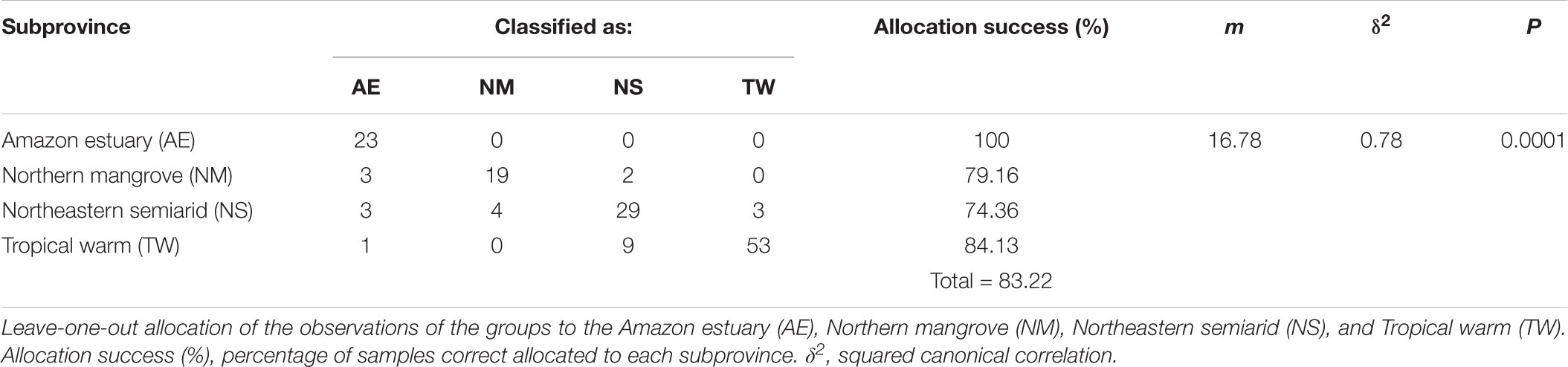
TABLE 2. Results of the Canonical Analysis of Principal coordinates (CAP), which examines the effect of the “subprovince” factor on the tidepool fish assemblages.
Habitat Variables Driving Fish Assemblage Structure
Distance based Linear Model (DistLM) identified water salinity explaining the largest proportion (11.3%) of the variation in the fish assemblages. Similarly, 20 of the other 23 predictive environmental variables tested were also significant (p < 0.05) in the marginal tests, with the only non-significant results being recorded for rugosity, temperature, and percentage sand cover (Supplementary Table S7). The optimal model solution consisted of the combination of seven environmental variables: salinity, tidal range, the shape of the rocky shore formation (SRF), percentage algal cover, distance to the subtidal area (DSA), latitude, and rainfall (Supplementary Table S7 and Figure 5). The results plotted in the RDA ordination indicated the segregation of AE and NM from the TW and NS samples (Figure 5). The most important variables contributing to the first axis of the dbRDA plot, explaining 44.4% of the fitted variation, were related to the DSA, salinity, rainfall, algal cover, and SRF. On the second axis, which explained 19.4% of the fitted variation, tidal range and latitude were the principal environmental variables.
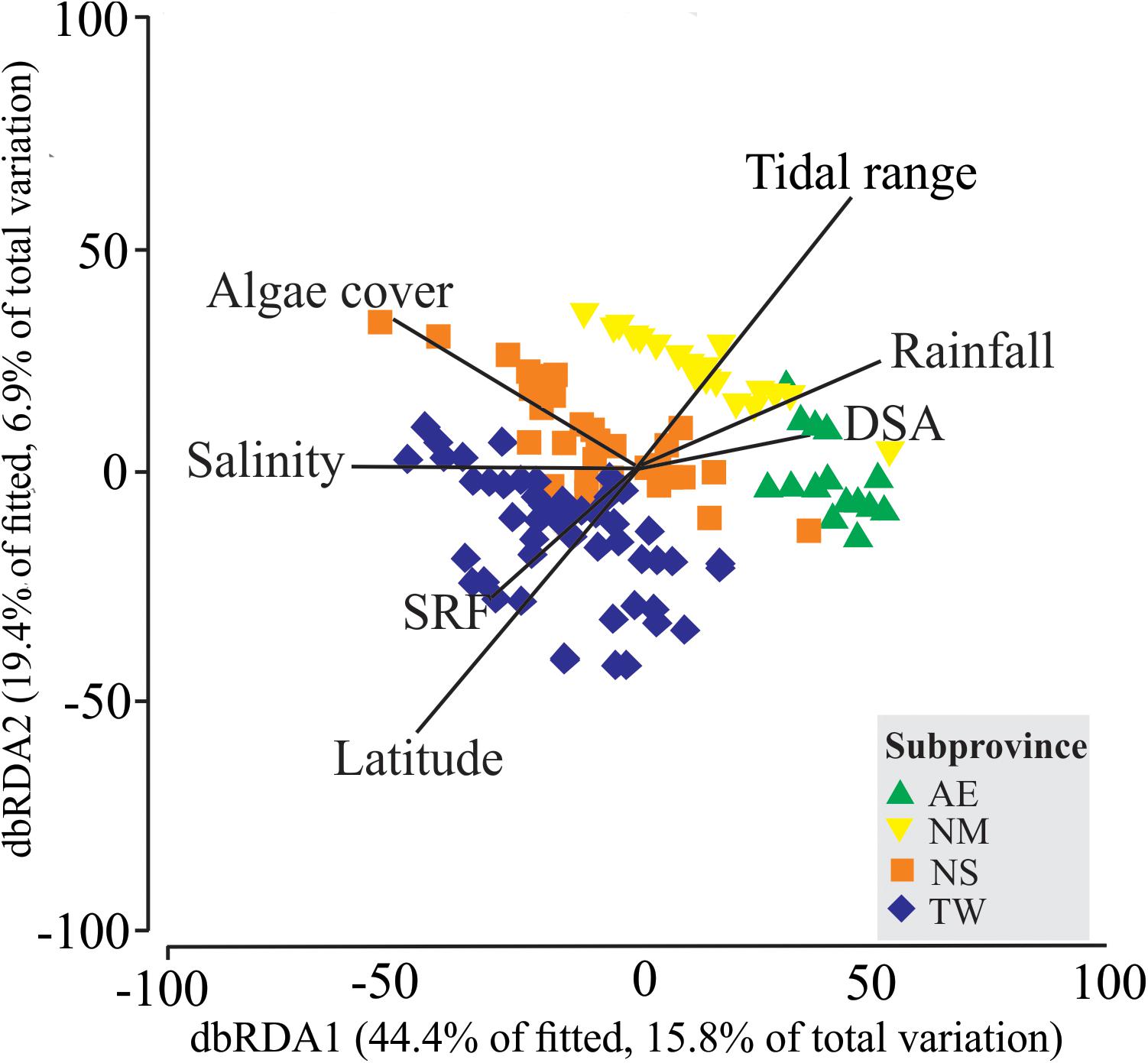
FIGURE 5. Biplot of the results of the redundancy analysis of the biological and environmental data from 149 tidepools sampled at 19 sites in the four Brazilian coastal subprovinces. Samples with no fish data (N = 3) were omitted from this analysis. SRF, Shape of Rocky shore Formation; DSA, Distance to Subtidal Area. Subprovinces: AE, Amazon estuary; NM, Northern mangrove; NS, Northeastern semiarid; TW, Tropical warm.
Discussion
The Brazilian coast is part of a single marine biogeographic region, the Brazilian Province (Briggs, 1974; Floeter et al., 2008; Briggs and Bowen, 2012; Pinheiro et al., 2018), although this province presents a number of local environmental, ecological, and evolutionary features, which characterize distinct biogeographic subprovinces (Pinheiro et al., 2018). In the specific case of reef fishes, Pinheiro et al. (2018) proposed two tropical coastal subprovinces, the north and north-east Brazil subprovince, and the east and south-east Brazil subprovince, although this division does not coincide exactly with our proposal of four coastal subprovinces, in the case of intertidal fishes. Clearly, despite the taxonomic and ecological proximity between fishes from intertidal reef environments (tidepools) and subtidal reefs, the subprovinces proposed for these two groups are related to the specific evolutionary, geological, oceanographic, and ecological processes that are characteristic of each ecosystem. This implies that distinct management and conservation strategies are necessary for the two groups of fishes.
The four coastal subprovinces proposed here added more precision on the previously known three coastal ecoregions (Amazonia, Northeastern Brazil and Eastern Brazil), which were inserted into two provinces (North Brazil Shelf and Tropical Southwestern Atlantic) defined by Spalding et al. (2007) and on the two regions (North-eastern and South-eastern Brazil Areas) resulted from distribution of prosobranch gastropods (Barroso et al., 2016). In addition, our results are partially aligned with the tropical marine geomorphological sectors proposed by Leão and Dominguez (2000), although our study also included equatorial latitudes (0°–2°). Given this, present study provides a more definitive proposal for the delimitation of the subprovinces of the tropical Brazilian coast, based on biological (tidepool fish diversity) and environmental data (23 in total) of the intertidal seascape. We further discuss below how the environmental and biological data support the proposed subprovinces from the perspective of the tropical Brazilian intertidal fishes.
Environmental Variables Influencing Intertidal Fish Distribution
Subprovinces evidenced here clearly shape intertidal fish communities. The main drivers acting on the spatial distribution of the fish were low salinity and high tidal ranges in the northernmost subprovinces (AE and NM), and high algal cover (NS and TW) and latitude (TW) toward the south. While the substrate has been identified as an important variable structuring intertidal fish communities (Arakaki et al., 2014), local-scale factors (the mouth of the Amazon, mangroves, and low-salinity estuarine waters) were the main factors defining the coastal subprovinces (e.g., AE and NM). However, regional-scale latitudinal trends were also key factors determining the configuration of the Tropical West subprovince. Local and regional drivers also act synergistically to shape subprovinces in intertidal and subtidal reefs worldwide (Rivadeneira et al., 2002; Floeter et al., 2008; Travers et al., 2010; Arakaki et al., 2014).
The spatial segregation of the geomorphological and oceanographic characteristics observed in the present study, together with the positive correlations between physical tidepool features (e.g., salinity, DSA, algal cover) and community variables further support the subprovinces proposed here. In this case, the established Brazilian Province (Briggs and Bowen, 2012; Pinheiro et al., 2018) can be divided into at least four distinct coastal subprovinces, based on the tropical intertidal seascapes. No strong correlations were found between physical tidepool features (e.g., pool volume and rugosity) and community variables, however, although a number of other studies have identified the area and volume of tidepools among the principal factors that determine fish density and diversity (Prochazka and Griffiths, 1992; Mahon and Mahon, 1994; Prochazka et al., 1999; Meager et al., 2005). The analytical approach we adopted allowed us to incorporate local and regional variables in a step-wise procedure, which indicated that species distributions and its composition may be the result of responses to the predominant environmental gradient. This contrasts with local-scale studies, which point to factors such as competition, foraging, and predator avoidance strategies, as principal drivers of fish assemblage structuring, which is the typical perspective in other marine environments (Martino and Able, 2003). In particular, intertidal reefs in the low latitude subprovinces (AE and NM) represent relatively harsh environments for marine fish, given their high rainfall and reduced salinity, emphasizing the influence of estuarine features (see Crabtree and Dean, 1982). In northern Brazil, the influence of the Amazon and other estuaries on coastal ecosystems is fundamental to the distribution and organization of marine species (Barletta et al., 2003; Rocha, 2003; Giarrizzo et al., 2010). For example, many of the intertidal ecotones of these two subprovinces are located within estuaries (Giarrizzo and Krumme, 2008; Oliveira et al., 2016), which not only determines the presence of a specific intertidal fish community, but also provides habitats for relatively large populations in comparison with the subprovinces located at higher latitudes (see Supplementary Table S3). Crabtree and Dean (1982) also found that estuarine tidepools harbor high fish densities. However, the stability of the environmental variables in the NS and TW subprovinces is demonstrated by the closer grouping of their samples in PCA ordination in comparison with the AE and NM, highlighting the heterogeneity of the intertidal habitats in these subprovinces, which also likely affects population density. In fact, Oliveira et al. (2016) observed three distinct intertidal habitats (rocky shore, mangrove, and salt marsh) within one coastal bay in the AE subprovince.
In general, the paucity of consistent significant effects of the local and structural environmental variables on the abundance and distribution of intertidal fishes is surprising given the apparent prevalence and importance micro-habitat arrangements in other habitats, terrestrial or marine (Pickett and White, 1985; Hector and Bagchi, 2007). However, the approach adopted in the present study provides alternative insights through a broader analysis of the factors regulating the biogeographical processes that mold intertidal reef fish communities, that is, the large-scale environmental factors that influence the distribution of fish most intensely. Further research incorporating data on the life cycle of the species that inhabit tidepools (i.e., residents and non-residents) will contribute to a better understanding of the micro- and meso-scale regulatory factors, which will be necessary to determine the exact nature of the processes that determine fish distribution patterns.
Biogeographic Patterns
The plume of the Amazon River forms a natural biogeographic barrier to many marine fish species, limiting the passage between the Caribbean and Brazilian provinces (Gilbert, 1973; Collette and Rützier, 1977; Rocha, 2003; Floeter et al., 2008). However, this barrier is considered to be semi-porous, allowing many marine species to cross when permitted by specific environmental conditions (e.g., Amazon run-off, sedimentation rates) and the ecological traits of the species, e.g., reproductive mode and body size (Joyeux et al., 2001; Rocha, 2003; Floeter et al., 2008; Pinheiro et al., 2018). Highly mobile species of large body size, with pelagic spawning (e.g., the Carangidae and Serranidae), and with capacity to tolerate a diversity of conditions will generally have a much greater chance of crossing the Amazon plume than small-sized species with restrict ranges, demersal spawning, and parental care, e.g., the Gobiidae (Luiz et al., 2012; Pinheiro et al., 2018). In this context, the dispersal capacity of species resident in tidepools is limited by their ecological traits, which include small body size, as well as their reduced swimming mobility, demersal spawning, and cryptic behavior (Barlow, 1999; DeMartini, 1999; Macieira et al., 2015).
In the present study, the AE and NM subprovinces limit the presence of many resident species of both the Caribbean and the NS–TW subprovinces. This spatial pattern is expressed through (i) number of families/species that are permanent residents in the AE–NM vs. the NS–TW subprovinces (Supplementary Tables S3, S4); (ii) the absence of permanent marine resident species in the AE subprovince (Supplementary Table S4), and (iii) the exclusive and endemic species of the AE subprovince (Supplementary Table S5). Considering the two adjacent pairs of subprovinces, the AE–NM and NS–TW, only six species – Barbulifer enigmaticus, Bathygobius soporator, Ctenogobius boleosoma, Gobiesox barbatulus, Microdesmus bahianus, and Omobranchus punctatus – occur in both pairs of subprovinces, and as O. punctuatus is an invasive species, it does not represent a true biogeographic pattern. Low species richness to the north (i.e., the Amazon Estuary; Supplementary Table S5) may be related to the same environmental filters (i.e., the Amazon plume and sedimentation rates, with low salinity and turbid waters) that limit the presence of typical marine species.
The environmental filters of the subprovinces are best exemplified by the distribution of the resident tidepool frillfin gobies, B. soporator and B. geminatus, which each present distinct habitat requirements and niche. Bathygobius soporator was distributed in all subprovinces, but at particularly high mean densities at the AE sites (225.9 individuals per m3), which is likely related to its preference for tidepools with estuarine features (Macieira and Joyeux, 2011; Oliveira et al., 2016). By contrast, B. geminatus, which is distributed between Florida and the TW subprovince, typically inhabits classical marine tidepools (Macieira and Joyeux, 2011; Andrades et al., 2018), and is absent from the AE and NM sites, while increasing in density toward the south. The subprovincial endemism of tidepool fishes in the AE also highlights the local features that act as barriers. Van Tassell et al. (2015) reported two resident species of Gobiosoma (denominated here as Gobiosoma spp.) endemic to intertidal areas of the Amazon estuary, as we observed in Eleotris (Guimarães-Costa et al., 2016), which has two endemic intertidal resident species (currently undescribed) in the AE and NM subprovinces, one of which (from the NM) was not record in the present study. This high degree of endemism may be accounted for by sympatric speciation events, through which the local drivers (e.g., environmental filters) of a subprovince may promote scenarios favorable to speciation at a local-scale (Doebeli and Dieckmann, 2003; Rocha et al., 2005; Bowen et al., 2013).
One other process that should be considered is ephemeral ecological speciation (Cutter and Gray, 2016; Pinheiro et al., 2017), given the observation of one new Gobiosoma species closely related to Gobiosoma alfiei (Van Tassell et al., 2015), and the other two new, cryptic Eleotris species (Guimarães-Costa et al., 2016), which probably evolved in the context of dynamic changes in the characteristics of the Amazon barrier (run-off, sedimentation rates) over geological time (Joyeux et al., 2001; Rocha, 2003). This incipient speciation would be the result of intermittent dispersal and colonization events (Cutter and Gray, 2016; Pinheiro et al., 2017). Pinheiro et al. (2017) concluded that the fusion process between nascent species and source area populations explains why most marine species are not differentiated without strong genetic selection. This further reinforces the role of the Amazon River as a biogeographic barrier for several tidepool species, which may be related to the high degree of endemism recorded in the AE subprovince. A second barrier highlighted by Pinheiro et al. (2018) is the South Equatorial Current bifurcation, where the Brazil Current flows to the south and the North Brazil Current flows to the north-west, between 10 and 14°S (Rodrigues et al., 2007), which may also constrain population movements and dispersal. Although, the São Francisco River run-off cited as possible barrier in this same study, it’s not helps to define our intertidal coastal subprovinces.
In general, herbivores are expected to prevail at lower latitudes and carnivores at higher ones in the marine realm, due to the species’ physiological constraints and the relative availability of primary resources in the tropical region (Choat, 1991; Ferreira et al., 2004; Floeter et al., 2005). In the present study, however, the observed trophic patterns did not correspond exactly to latitudinal trends, although we did identify local and regional patterns of trophic group distribution, with carnivores (AE) and omnivores (NM) dominating the subprovinces at lower latitudes. This can be at partly accounted for by the turbid waters and high river discharge of this region, which restrict algal development, reducing the availability of resources for herbivores in the AE and NM subprovinces. The carnivore and omnivore trophic structures of assemblages were also influenced strongly by the high densities of B. soporator and O. punctatus in the AE and NM subprovinces, respectively. The presence of these species and other estuarine-related species, such as Mugil curema, Genyatremus luteus, and Thalassophryne nattereri, further reinforces the role of local-scale factors (e.g., river discharge) in the structuring of tidepool assemblages in these subprovinces. At the nine TW sites, herbivores were the predominant group structuring the intertidal communities, which is expected in shallow reef habitats of tropical regions (Steneck et al., 2017).
Conservation Issues and Conclusion
From a conservation perspective, the subprovince profiles observed in the present study represent an essential step toward the more effective management of Brazilian coastal zones. Overall, the Amazon Estuary subprovince appeared to be the most vulnerable, due to its endemism and environmental particularities. Conservation priority actions in the Amazon coastal region were also suggested by Vilar et al. (2017) for estuarine fishes. Currently, oil drilling and coastal urban development are the principal threats to the marine biota of the Brazilian Province (Buruaem et al., 2012; Moura et al., 2016). Urban development, in particular, not only causes habitat losses, but also favors biological invasions through the discharge of ballast water by international shipping (Carlton, 1996; Wonham et al., 2001), which invariably occurs close to intertidal areas. All the Brazilian subprovinces identified here have already undergone invasions from species such as the sun corals, Tubastrea spp. (Creed, 2006), and the muzzled blenny Omobranchus punctatus (Lasso-Alcalá et al., 2011), and ongoing invasions, such as that of the lionfish, Pterois spp., are even more preoccupying (Luiz et al., 2013). We recorded O. punctatus at seven sites. Potential niche overlap and competition with native intertidal species seems to be unavoidable, and will be harmful, given that the ecological traits and habitat requirements of this species are highly similar to those of endemic species of the Brazilian Province, such as Entomacrodus vomerinus, Ophioblennius trinitatis, and Gobiosoma alfiei.
Currently, while Marine Protected Areas contemplate 26% of Brazilian marine territory, less than 2% of these areas include coastal zones and intertidal seascapes. This leaves these coastal zones extremely vulnerable to countless impacts (oil and gas exploration, tourism, mariculture, fisheries, and port activities), which could be minimized through more participative management, firm intention and concerted actions by environmental agencies (Jablonski and Filet, 2008). In this context, we would recommend an integrated approach, involving environmental agencies (at federal, state, and municipal levels), research institutions (universities and research centers), and general society (community leaders and NGOs), for the establishment of priorities for the use and conservation of coastal zones. While it will be impossible to eliminate conflicts altogether, collaborative networks have led the way to effective management and conservation in large-scale territories under multiple jurisdictions (Wyborn and Bixler, 2013).
Ethics Statement
This study was conducted in accordance with all ethical guidelines and Brazilian legislation, including authorization to collect fishes from Brazilian Environmental Protection Agency (ICMBio; license number 35625). Also, this study was carried out in accordance with the recommendation of Ethics Committee (CEUA; #29/2016).
Author Contributions
TG conceived and designed this study. FM and TG collected the data. All authors discussed and analyzed the data. RA, TG, and FM wrote the first draft. All authors revised and contributed to the final version.
Funding
RA received a Ph.D. scholarship from CAPES-DS, CAPES-PDSE (Edital 19/2016, process 88881.132520/2016-01) and PDJ scholarship (process 151121/2018-4; CNPq), FM received a master’s scholarship from CAPES, RM thanks CAPES for his PNPD grant and TG receives a productivity grant from the Conselho Nacional de Desenvolvimento Científico e Tecnológico [National Council for Scientific and Technological Development (CNPq; process 310299/2016-0)]. All specimen collection was conducted under license from the Brazilian Environmental Protection Agency ICMBio.
Conflict of Interest Statement
The authors declare that the research was conducted in the absence of any commercial or financial relationships that could be construed as a potential conflict of interest.
Acknowledgments
We are grateful to R. Oliveira, A. Carvalho-Filho, and J-C. Joyeux, who provided field assistance and commented on an earlier draft.
Supplementary Material
The Supplementary Material for this article can be found online at: https://www.frontiersin.org/articles/10.3389/fmars.2018.00412/full#supplementary-material
References
Abell, R., Thieme, M. L., Revenga, C., Bryer, M., Kottelat, M., Bogutskaya, N., et al. (2008). Freshwater ecoregions of the world: a new map of biogeographic units for freshwater biodiversity conservation. Bioscience 58, 403–414. doi: 10.1641/B580507
Ahmadia, G. N., Tornabene, L., Smith, D. J., and Pezold, F. L. (2018). The relative importance of regional, local, and evolutionary factors structuring cryptobenthic coral-reef assemblages. Coral Reefs 37, 279–293. doi: 10.1007/s00338-018-1657-2
Alvares, C. A., Stape, J. L., Sentelhas, P. C., Gonçalves, J. L. M., and Sparovek, G. (2013). Köppen’s climate classification map for Brazil. Meteorol. Z. 22, 711–728. doi: 10.1127/0941-2948/2013/0507
Anderson, M., and Willis, T. (2012). Canonical analysis of principal coordinates: a useful method of constrained ordination for ecology. Ecology 84, 511–525. doi: 10.1890/0012-96582003084
Anderson, M. J. (2001). Permutation tests for univariate or multivariate analysis of variance and regression. Can. J. Fish. Aquat. Sci. 58, 626–639. doi: 10.1139/f01-004
Anderson, M. J., Gorley, R. N., and Clarke, K. R. (2008). PERMANOVA + for PRIMER: guide to Software and Statistical Methods. Plymouth: PRIMER-E, 274.
Anderson, M. J., and Robinson, J. (2003). Generalised discriminant analysis based on distances. Aust. N. Z. J. Stat. 45, 301–318. doi: 10.1111/1467-842X.00285
Andrades, R., Joyeux, J.-C., Gasparini, J. L., Reis-Filho, J. A., Macieira, R. M., and Giarrizzo, T. (2017). Fringe on the brink: intertidal reefs at risk. Science 357:261. doi: 10.1126/science.aao0403
Andrades, R., Reis-Filho, J. A., Macieira, R. M., Giarrizzo, T., and Joyeux, J.-C. (2018). Endemic fish species structuring oceanic intertidal reef assemblages. Sci. Rep. 8:10791. doi: 10.1038/s41598-018-29088-0
Arakaki, S., and Tokeshi, M. (2011). Analysis of spatial niche structure in coexisting tidepool fishes: null models based on multi-scale experiments. J. Anim. Ecol. 80, 137–147. doi: 10.1111/j.1365-2656.2010.01749.x
Arakaki, S., Tsuchiya, M., and Tokeshi, M. (2014). Testing latitudinal patterns of tidepool fish assemblages: local substrate characteristics affect regional-scale trends. Hydrobiologia 733, 45–62. doi: 10.1007/s10750-013-1768-2
Barletta, M., Barletta-Bergan, A., Saint-Paul, U., and Hubold, G. (2003). Seasonal changes in density, biomass, and diversity of estuarine fishes in tidal mangrove creeks of the lower Caeté Estuary (northern Brazilian coast, east Amazon). Mar. Ecol. Prog. Ser. 256, 217–228. doi: 10.3354/meps256217
Barlow, G. W. (1999). “Fish life on the littoral edge,” in Behaviour and Conservation of Littoral Fishes, eds V. C. Almada, R. F. Oliveira, and E. J. Gonçalves (Lisboa: ISPA), 3–32.
Barroso, C. X., da Cruz Lotufo, T. M., and Matthews-Cascon, H. (2016). Biogeography of Brazilian prosobranch gastropods and their Atlantic relationships. J. Biogeogr. 43, 2477–2488. doi: 10.1111/jbi.12821
Bennett, B. A., and Griffiths, C. L. (1984). Factors affecting the distribution, abundance and diversity of rock-pool fishes on the Cape Peninsula, South Africa. S. Afr. J. Zool. 19, 97–104. doi: 10.1080/02541858.1984.11447865
Bernardes, M., Knoppers, B., Rezende, C., Souza, W., and Ovalle, A. (2012). Land-sea interface features of four estuaries on the South America Atlantic coast. Braz. J. Biol. 72, 761–774. doi: 10.1590/S1519-69842012000400011
Bowen, B. W., Rocha, L. A., Toonen, R. J., and Karl, S. A. (2013). The origins of tropical marine biodiversity. Trends Ecol. Evol. 28, 359–366. doi: 10.1016/j.tree.2013.01.018
Briggs, J. C., and Bowen, B. W. (2012). A realignment of marine biogeographic provinces with particular reference to fish distributions. J. Biogeogr. 39, 12–30. doi: 10.1111/j.1365-2699.2011.02613.x
Burrough, P. A. (1981). Fractal dimensions of landscapes and other environmental data. Nature 294, 240–242. doi: 10.1038/294240a0
Buruaem, L. M., Hortellani, M. A., Sarkis, J. E., Costa-Lotufo, L. V., and Abessa, D. M. S. (2012). Contamination of port zone sediments by metals from Large Marine Ecosystems of Brazil. Mar. Pollut. Bull. 64, 479–488. doi: 10.1016/j.marpolbul.2012.01.017
Campos, E. J. D., Lorenzzetti, J. A., Stevenson, M. R., Stech, J. L., and De Souza, R. B. (1996). Penetration of waters from the Brazil-Malvinas confluence region along the South American continental shelf up to 23°S. An. Acad. Bras. Cienc. 68, 49–58.
Carlton, J. T. (1996). Pattern, process, and prediction in marine invasion ecology. Biol. Conserv. 78, 97–106. doi: 10.1016/0006-3207(96)00020-1
Castellanos-Galindo, G. A., Giraldo, A., and Rubio, E. A. (2005). Community structure of an assemblage of tidepool fishes on a tropical eastern Pacific rocky shore, Colombia. J. Fish Biol. 67, 392–408. doi: 10.1111/j.1095-8649.2005.00735.x
Chaudhary, C., Saeedi, H., and Costello, M. J. (2016). Bimodality of latitudinal gradients in marine species richness. Trends Ecol. Evol. 31, 670–676. doi: 10.1016/j.tree.2016.06.001
Choat, J. H. (1991). “The biology of herbivorous fishes on coral reefs,” in The Ecology of Fishes on Coral Reefs, ed. P. Sale (San Diego, CA: Academic Press), 120–155. doi: 10.1016/B978-0-08-092551-6.50011-8
Clarke, K. R., and Ainsworth, M. (1993). A method of linking multivariate community structure to environmental variables. Mar. Ecol. Prog. Ser. 92, 205–219. doi: 10.3354/meps092205
Clarke, K. R., and Warwick, R. M. (2001). Change in Marine Communities: an Approach to Statistical Analysis and Interpretation, 2nd Edn. Plymouth: PRIMER-E.
Collette, B., and Rützier, K. (1977). “Reef fishes over sponge bottoms off the mouth of the Amazon River,” in Proceedings of the 3rd International Coral Reef Symposium, (Miami, FL: University of Miami), 305–310.
Cox, T. E., Baumgartner, E., Philippoff, J., and Boyle, K. S. (2011). Spatial and vertical patterns in the tidepool fish assemblage on the island of O‘ahu. Environ. Biol. Fishes 90, 329–342. doi: 10.1007/s10641-010-9744-4
Crabtree, R. E., and Dean, J. M. (1982). The structure of two South Carolina estuarine tide pool fish assemblages. Estuaries 5, 2–9. doi: 10.1007/BF02803950
Creed, J. C. (2006). Two invasive alien azooxanthellate corals, Tubastraea coccinea and Tubastraea tagusensis, dominate the native zooxanthellate Mussismilia hispida in Brazil. Coral Reefs 25:350. doi: 10.1007/s00338-006-0105-x
Cutter, A. D., and Gray, J. C. (2016). Ephemeral ecological speciation and the latitudinal biodiversity gradient. Evolution 70, 2171–2185. doi: 10.1111/evo.13030
DeMartini, E. E. (1999). “Intertidal spawning,” in Intertidal Fishes: Life in Two Worlds, eds M. H. Horn, K. L. M. Martin, and M. A. Chotkowski (San Diego, CA: Academic Press), 143–164. doi: 10.1016/B978-012356040-7/50009-3
Doebeli, M., and Dieckmann, U. (2003). Speciation along environmental gradients. Nature 421, 259–264. doi: 10.1038/nature01274
Dominguez, J. M. L. (2009). “The coastal zone of Brazil,” in Geology and Geomorphology of Holocene Coastal Barriers of Brazil, eds S. R. Dillenburg and P. A. Hesp (Berlin: Springer-Verlag), 17–51. doi: 10.1007/978-3-540-44771-9_2
Ekau, W., and Knoppers, B. (1999). An introduction to the pelagic system of the north-east and east Brazilian shelf. Arch. Fish. Mar. Res. 47, 113–132.
Elahi, R., O’Connor, M. I., Byrnes, J. E. K., Dunic, J., Eriksson, B. K., Hensel, M. J. S., et al. (2015). Recent trends in local-scale marine biodiversity reflect community structure and human impacts. Curr. Biol. 25, 1938–1943. doi: 10.1016/j.cub.2015.05.030
Ferreira, C. E. L., Floeter, S. R., Gasparini, J. L., Ferreira, B. P., and Joyeux, J.-C. (2004). Trophic structure patterns of Brazilian reef fishes: a latitudinal comparison. J. Biogeogr. 31, 1093–1106. doi: 10.1111/j.1365-2699.2004.01044.x
Floeter, S. R., Behrens, M. D., Ferreira, C. E. L., Paddack, M. J., and Horn, M. H. (2005). Geographical gradients of marine herbivorous fishes: patterns and processes. Mar. Biol. 147, 1435–1447. doi: 10.1007/s00227-005-0027-0
Floeter, S. R., Rocha, L. A., Robertson, D. R., Joyeux, J. C., Smith-Vaniz, W. F., Wirtz, P., et al. (2008). Atlantic reef fish biogeography and evolution. J. Biogeogr. 35, 22–47. doi: 10.1111/j.1365-2699.2007.01790.x
Freedman, D., and Lane, D. (1983). A nonstochastic interpretation of reported significance levels. J. Bus. Econ. Stat. 1, 292–298. doi: 10.1080/07350015.1983.10509354
Freire, K. M. F., Christensen, V., and Pauly, D. (2008). Description of the East Brazil Large Marine Ecosystem using a trophic model. Sci. Mar. 72, 477–491. doi: 10.3989/scimar.2008.72n3477
Giarrizzo, T., and Krumme, U. (2008). Heterogeneity in intertidal fish fauna assemblages along the world’s longest mangrove area in northern Brazil. J. Fish Biol. 72, 773–779. doi: 10.1111/j.1095-8649.2007.01728.x
Giarrizzo, T., Krumme, U., and Wosniok, W. (2010). Size-structured migration and feeding patterns in the banded puffer fish Colomesus psittacus (Tetraodontidae) from north Brazilian mangrove creeks. Mar. Ecol. Prog. Ser. 419, 157–170. doi: 10.3354/meps08852
Gibson, R. N., and Yoshiyama, R. M. (1999). “Intertidal fish communities,” in Intertidal Fishes: Life in Two Worlds, eds M. M. Horn, K. L. M. Martin, and M. A. Chotkowski (Cambridge, MA: Academic Press), 264–296. doi: 10.1016/B978-012356040-7/50014-7
Gilbert, C. R. (1973). Characteristics of the western Atlantic reef-fish fauna. Q. J. Florida Acad. Sci. 35, 130–144. doi: 10.1111/j.1095-8649.2011.03138.x
Griffiths, S. (2000). The use of clove oil as an anaesthetic and method for sampling intertidal rockpool fishes. J. Fish Biol. 57, 1453–1464. doi: 10.1006/jfbi.2000.1406
Griffiths, S. P. (2003). Rockpool ichthyofaunas of temperate Australia: species composition, residency and biogeographic patterns. Estuar. Coast. Shelf Sci. 58, 173–186. doi: 10.1016/S0272-7714(03)00073-8
Griffiths, S. P., Davis, A. R., and West, R. J. (2006). Role of habitat complexity in structuring temperate rockpool ichthyofaunas. Mar. Ecol. Prog. Ser. 313, 227–239. doi: 10.3354/meps313227
Guimarães-Costa, A., Vallinoto, M., Giarrizzo, T., Pezold, F., Schneider, H., and Sampaio, I. (2016). Molecular evidence of two new species of Eleotris (Gobiiformes: Eleotridae) in the western Atlantic. Mol. Phylogenet. Evol. 98, 52–56. doi: 10.1016/j.ympev.2016.01.014
Halpern, B. S., Walbridge, S., Selkoe, K. A., Kappel, C. V., Micheli, F., D’Agrosa, C., et al. (2008). A global map of human impact on marine ecosystems. Science 319, 948–952. doi: 10.1126/science.1149345
Harasti, D., McLuckie, C., Gallen, C., Malcolm, H., and Moltschaniwskyj, N. (2016). Assessment of rock pool fish assemblages along a latitudinal gradient. Mar. Biodivers. 48, 1147–1158. doi: 10.1007/s12526-016-0560-8
Hector, A., and Bagchi, R. (2007). Biodiversity and ecosystem multifunctionality. Nature 448, 188–190. doi: 10.1038/nature05947
Heffernan, J. B., Soranno, P. A., Angilletta, M. J., Buckley, L. B., Gruner, D. S., Keitt, T. H., et al. (2014). Macrosystems ecology: understanding ecological patterns and processes at continental scales. Front. Ecol. Environ. 12:5–14. doi: 10.1890/130017
Helmuth, B., Harley, C. D. G., Halpin, P. M., O’Donnell, M., Hofmann, G. E., and Blanchette, C. A. (2002). Climate change and latitudinal patterns of intertidal thermal stress. Science 298, 1015–1017. doi: 10.1126/science.1076814
Helmuth, B., Mieszkowska, N., Moore, P., and Hawkins, S. J. (2006). Living on the edge of two changing worlds: forecasting the responses of rocky intertidal ecosystems to climate change. Annu. Rev. Ecol. Evol. Syst. 37, 373–404. doi: 10.1146/annurev.ecolsys.37.091305.110149
Horn, M. H., and Ojeda, F. P. (1999). “Herbivory,” in Intertidal Fishes: Life in Two Worlds, eds M. H. Horn, K. L. M. Martin, and M. A. B. T.-I. F. Chotkowski (San Diego, CA: Academic Press), 197–222. doi: 10.1016/B978-012356040-7/50012-3
Horn, M. M., Martin, K. L. M., and Chotkowski, M. A. (1999). Intertidal Fishes: Life in Two Worlds. San Diego, CA: Elsevier.
Jablonski, S., and Filet, M. (2008). Coastal management in Brazil - A political riddle. Ocean Coast. Manag. 51, 536–543. doi: 10.1016/j.ocecoaman.2008.06.008
Joyeux, J.-C., Floeter, S. R., Ferreira, C. E. L., and Gasparini, J. L. (2001). Biogeography of tropical reef fishes: the South Atlantic puzzle. J. Biogeogr. 28, 831–841. doi: 10.1046/j.1365-2699.2001.00602.x
Kjerfve, B., Perillo, G. M. E., Gardner, L. R., Rine, J. M., Dias, G. T. M., and Mochel, F. R. (2002). “Morphodynamics of muddy environments along the Atlantic coasts of North and South America,” in Muddy Coasts of the World: Processes, Deposits and Functions, eds T. R. Healy, Y. Wang, and J.-A. Healy (Amsterdam: Elsevier), 479–532. doi: 10.1016/S1568-2692(02)80094-8
Kuha, J. (2004). AIC and BIC: comparisons of assumptions and performance. Soc. Methods Res. 33, 188–229. doi: 10.1177/0049124103262065
Lasso-Alcalá, O., Nunes, J. L. S., Lasso, C., Posada, J., Robertson, R., Piorski, N. M., et al. (2011). Invasion of the Indo-Pacific blenny Omobranchus punctatus (Perciformes: Blenniidae) on the Atlantic Coast of Central and South America. Neotrop. Ichthyol. 9, 571–578. doi: 10.1590/S1679-62252011000300010
Leão, Z., and Dominguez, J. M. L. (2000). Tropical coast of Brazil. Mar. Pollut. Bull. 41, 112–122. doi: 10.1016/S0025-326X(00)00105-3
Luiz, O. J., Floeter, S. R., Rocha, L. A., and Ferreira, C. E. L. (2013). Perspectives for the lionfish invasion in the South Atlantic: are Brazilian reefs protected by the currents? Mar. Ecol. Prog. Ser. 485, 1–7. doi: 10.3354/meps10383
Luiz, O. J., Madin, J. S., Robertson, D. R., Rocha, L. A., Wirtz, P., and Floeter, S. R. (2012). Ecological traits influencing range expansion across large oceanic dispersal barriers: insights from tropical Atlantic reef fishes. Proc. R. Soc. B Biol. Sci. 279, 1033–1040. doi: 10.1098/rspb.2011.1525
Machado, F. S., Carvalho-Filho, A., and Giarrizzo, T. (2017). Redescription and range extension of the endangered Paiva’s blenny Lupinoblennius paivai (Perciformes: Blenniidae). J. Fish Biol. 90, 2394–2401. doi: 10.1111/jfb.13322
Macieira, R. M., and Joyeux, J.-C. (2011). Distribution patterns of tidepool fishes on a tropical flat reef. Fish. Bull. 109, 305–315.
Macieira, R. M., Simon, T., Pimentel, C. R., and Joyeux, J.-C. (2015). Isolation and speciation of tidepool fishes as a consequence of Quaternary sea-level fluctuations. Environ. Biol. Fishes 98, 385–393. doi: 10.1007/s10641-014-0269-0
Mahon, R., and Mahon, S. D. (1994). Structure and resilience of a tidepool fish assemblage at Barbados. Environ. Biol. Fishes 41, 171–190. doi: 10.1007/BF02197843
Martino, E. J., and Able, K. W. (2003). Fish assemblages across the marine to low salinity transition zone of a temperate estuary. Estuar. Coast. Shelf Sci. 56, 969–987. doi: 10.1016/S0272-7714(02)00305-0
McArdle, B. H., and Anderson, M. J. (2001). Fitting multivariate models to community data: a comment on distance-based redundancy analysis. Ecology 82, 290–297. doi: 10.1890/0012-9658 (2001)082[0290:FMMTCD]2.0.CO;2
McGarigal, K., and Marks, B. (1995). FRAGSTATS: Spatial Pattern Analysis Program for Quantifying Landscape Structure. Portland, OR: U.S. Department of Agriculture, 122. doi: 10.2737/PNW-GTR-351
Meager, J. J., Williamson, I., and King, C. R. (2005). Factors affecting the distribution, abundance and diversity of fishes of soft-substrata tidal pools within Moreton Bay, Australia. Hydrobiologia 537, 71–80. doi: 10.1007/s10750-004-2308-x
Milne, B. T. (1988). Measuring the fractal geometry of landscapes. Appl. Math. Comput. 27, 67–79. doi: 10.1016/0096-3003(88)90099-9
Mittelbach, G. G., Schemske, D. W., Cornell, H. V., Allen, A. P., Brown, J. M., Bush, M. B., et al. (2007). Evolution and the latitudinal diversity gradient: speciation, extinction and biogeography. Ecol. Lett. 10, 315–331. doi: 10.1111/j.1461-0248.2007.01020.x
Moura, R. L., Amado-Filho, G. M., Moraes, F. C., Brasileiro, P. S., Salomon, P. S., Mahiques, M. M., et al. (2016). An extensive reef system at the Amazon River mouth. Sci. Adv. 2, 1–11. doi: 10.1126/sciadv.1501252
Navarrete, A. H., Lagos, N. A., and Ojeda, F. P. (2014). Latitudinal diversity patterns of Chilean coastal fishes: searching for causal processes. Rev. Chil. Hist. Nat. 87:2. doi: 10.1186/0717-6317-1-2
Nieder, J. (1993). Distribution of juvenile blennies (Pisces, Blenniidae) in small tide-pools: result of flow-tide lottery strategic habitat selection? Bonn. Zool. Beitr. 44, 133–140.
Oliveira, R. R. S., Macieira, R. M., and Giarrizzo, T. (2016). Ontogenetic shifts in fishes between vegetated and unvegetated tidepools: assessing the effect of physical structure on fish habitat selection. J. Fish Biol. 89, 959–976. doi: 10.1111/jfb.13013
Olson, D. M., Dinerstein, E., Wikramanayake, E. D., Burgess, N. D., Powell, G. V. N., Underwood, E. C., et al. (2001). Terrestrial ecoregions of the world: a new map of life on earth. Bioscience 51, 933–938. doi: 10.1641/0006-3568 (2001)051[0933:TEOTWA]2.0.CO;2
Pecl, G. T., Araújo, M. B., Bell, J. D., Blanchard, J., Bonebrake, T. C., Chen, I. C., et al. (2017). Biodiversity redistribution under climate change: impacts on ecosystems and human well-being. Science 355:eaai9214. doi: 10.1126/science.aai9214
Perry, G. D., Duffy, P. B., and Miller, N. L. (1996). An extended data set of river discharges for validation of general circulation models. J. Geophys. Res. Atmos. 101, 21339–21349. doi: 10.1029/96JD00932
Peterson, R. G., and Stramma, L. (1991). Upper-level circulation in the South-Atlantic Ocean. Prog. Oceanogr. 26, 1–73. doi: 10.1016/0079-6611(91)90006-8
Pickett, S. T. A., and White, P. S. (1985). The Ecology of Natural Disturbances and Patch Dynamics. New York, NY: Academic Press.
Pinheiro, H. T., Bernardi, G., Simon, T., Joyeux, J.-C., Macieira, R. M., Gasparini, J. L., et al. (2017). Island biogeography of marine organisms. Nature 549, 82–85. doi: 10.1038/nature23680
Pinheiro, H. T., Rocha, L. A., Macieira, R. M., Carvalho-Filho, A., Anderson, A. B., Bender, M. G., et al. (2018). South-western Atlantic reef fishes: zoogeographical patterns and ecological drivers reveal a secondary biodiversity centre in the Atlantic Ocean. Divers. Distrib. 24, 951–965. doi: 10.1111/ddi.12729
Prochazka, K., Chotkowski, M. A., and Buth, D. G. (1999). “Biogeography of rocky intertidal fishes,” in Intertidal Fishes: Life in Two Worlds, eds M. H. Horn, K. L. M. Martin, and M. A. Chotkowski (San Diego, CA: Academic Press), 332–355. doi: 10.1016/B978-012356040-7/50016-0
Prochazka, K., and Griffiths, C. L. (1992). The intertidal fish fauna of the west coast of South Africa: species, community and biogeographic patterns. S. Afr. J. Zool. 27, 115–120. doi: 10.1080/02541858.1992.11448271
Raffaelli, D., and Hawkins, S. (1996). Intertidal Ecology, 1st Edn. London: Chapman & Hall. doi: 10.1007/978-94-009-1489-6
Ricklefs, R. (1987). Community diversity: relative roles of local and regional processes. Science 235, 167–171. doi: 10.1126/science.235.4785.167
Rivadeneira, M., Fernández, M., and Navarrete, S. (2002). Latitudinal trends of species diversity in rocky intertidal herbivore assemblages: spatial scale and the relationship between local and regional species richness. Mar. Ecol. Prog. Ser. 245, 123–131. doi: 10.3354/meps245123
Rocha, L. A. (2003). Patterns of distribution and processes of speciation in Brazilian reef fishes. J. Biogeogr. 30, 1161–1171. doi: 10.1046/j.1365-2699.2003.00900.x
Rocha, L. A., Robertson, D. R., Roman, J., and Bowen, B. W. (2005). Ecological speciation in tropical reef fishes. Proc. R. Soc. B Biol. Sci. 272, 573–579. doi: 1098/2004.3005
Rodrigues, R. R., Rothstein, L. M., and Wimbush, M. (2007). Seasonal variability of the South Equatorial Current bifurcation in the Atlantic Ocean: a numerical study. J. Phys. Oceanogr. 37, 16–30. doi: 10.1175/JPO2983.1
Rosa, R. S., Rosa, I. L., and Rocha, L. A. (1997). Diversidade da ictiofauna de poças de poças de maré da praia do Cabo Branco, João Pessoa, Paraíba, Brasil. Rev. Bras. Zool. 14, 201–221. doi: 10.1590/S0101-81751997000100019
Schemske, D. W., Mittelbach, G. G., Cornell, H. V., Sobel, J. M., and Roy, K. (2009). Is there a latitudinal gradient in the importance of biotic interactions? Annu. Rev. Ecol. Evol. Syst. 40, 245–269. doi: 10.1146/annurev.ecolsys.39.110707.173430
Souza-Filho, P. W. M. (2005). Costa de manguezais de macromaré da Amazônia: cenários morfológicos, mapeamento e quantificação de áreas usando dados de sensores remotos. Rev. Bras. Geofis. 23, 427–435. doi: 10.1590/S0102-261X2005000400006
Spalding, M. D., Fox, H. E., Allen, G. R., Davidson, N., Ferdaña, Z. A., Finlayson, M. A. X., et al. (2007). Marine ecoregions of the world: a bioregionalization of coastal and shelf areas. Bioscience 57, 573–583. doi: 10.1641/B570707
Steneck, R. S., Bellwood, D. R., and Hay, M. E. (2017). Herbivory in the marine realm. Curr. Biol. 27, R484–R489. doi: 10.1016/j.cub.2017.04.021
Thompson, R. C., Crowe, T. P., and Hawkins, S. J. (2002). Rocky intertidal communities: past environmental changes, present status and predictions for the next 25 years. Environ. Conserv. 29, 168–191. doi: 10.1017/S0376892902000115
Travers, M. J., Potter, I. C., Clarke, K. R., Newman, S. J., and Hutchins, J. B. (2010). The inshore fish faunas over soft substrates and reefs on the tropical west coast of Australia differ and change with latitude and bioregion. J. Biogeogr. 37, 148–169. doi: 10.1111/j.1365-2699.2009.02183.x
Van Tassell, J. L., Joyeux, J.-C., Macieira, R. M., and Tornabene, L. (2015). Status of Gobiosoma (Teleostei: Gobiidae) from Brazil: description of a new species, redescription of G. hemigymnum, molecular phylogeny of the genus, and key to Atlantic species. Zootaxa 4007, 451–480. doi: 10.11646/zootaxa.4007.4.1
Vilar, C. C., Joyeux, J. C., Giarrizzo, T., Spach, H. L., Vieira, J. P., and Vaske-Junior, T. (2013). Local and regional ecological drivers of fish assemblages in Brazilian estuaries. Mar. Ecol. Prog. Ser. 485, 181–197. doi: 10.3354/meps10343
Vilar, C. C., Joyeux, J. C., and Spach, H. L. (2017). Geographic variation in species richness, rarity, and the selection of areas for conservation: an integrative approach with Brazilian estuarine fishes. Estuar. Coast. Shelf Sci. 196, 134–140. doi: 10.1016/j.ecss.2017.06.022
Vinagre, C., Leal, I., Mendonça, V., Madeira, D., Narciso, L., Diniz, M. S., et al. (2016). Vulnerability to climate warming and acclimation capacity of tropical and temperate coastal organisms. Ecol. Indic. 62, 317–327. doi: 10.1016/j.ecolind.2015.11.010
White, G. E., Hose, G. C., and Brown, C. (2015). Influence of rock-pool characteristics on the distribution and abundance of inter-tidal fishes. Mar. Ecol. 36, 1332–1344. doi: 10.1111/maec.12232
Wiens, J. J. (2015). Explaining large-scale patterns of vertebrate diversity. Biol. Lett. 11:20150506. doi: 10.1098/rsbl.2015.0506
Wisser, D., Fekete, B. M., Vörösmarty, C. J., and Schumann, A. H. (2010). Reconstructing 20th century global hydrography: a contribution to the Global Terrestrial Network- Hydrology (GTN-H). Hydrol. Earth Syst. Sci. 14, 1–24. doi: 10.5194/hess-14-1-2010
Wonham, M., Walton, W., Ruiz, G., Frese, A., and Galil, B. (2001). Going to the source: role of the invasion pathway in determining potential invaders. Mar. Ecol. Prog. Ser. 215, 1–12. doi: 10.3354/meps215001
Wyborn, C., and Bixler, R. P. (2013). Collaboration and nested environmental governance: scale dependency, scale framing, and cross-scale interactions in collaborative conservation. J. Environ. Manage. 123, 58–67. doi: 10.1016/j.jenvman.2013.03.014
Keywords: tidepool, rockpool, reef fish, Brazil, endemism, biogeography
Citation: Andrades R, Machado FS, Reis-Filho JA, Macieira RM and Giarrizzo T (2018) Intertidal Biogeographic Subprovinces: Local and Regional Factors Shaping Fish Assemblages. Front. Mar. Sci. 5:412. doi: 10.3389/fmars.2018.00412
Received: 25 August 2018; Accepted: 16 October 2018;
Published: 06 November 2018.
Edited by:
Gabriel Machovsky-Capuska, The University of Sydney, AustraliaReviewed by:
Jan Marcin Weslawski, Institute of Oceanology (PAN), PolandRodrigo Riera, Catholic University of the Most Holy Conception, Chile
Copyright © 2018 Andrades, Machado, Reis-Filho, Macieira and Giarrizzo. This is an open-access article distributed under the terms of the Creative Commons Attribution License (CC BY). The use, distribution or reproduction in other forums is permitted, provided the original author(s) and the copyright owner(s) are credited and that the original publication in this journal is cited, in accordance with accepted academic practice. No use, distribution or reproduction is permitted which does not comply with these terms.
*Correspondence: Ryan Andrades, cnlhbmFuZHJhZGVzQGdtYWlsLmNvbQ==