- 1Departamento de Oceanografía Biológica, Centro de Investigación Científica y de Educación Superior de Ensenada, Ensenada, Mexico
- 2Departamento de Acuicultura, Centro de Investigación Científica y de Educación Superior de Ensenada, Ensenada, Mexico
- 3Instituto de Sanidad Acuícola, A.C. (ISA), Ensenada, Mexico
- 4Departamento de Biotecnología Marina, Centro de Investigación Científica y de Educación Superior de Ensenada, Ensenada, Mexico
In 2016 a mass mortality period (MMP) of cage cultured tuna occurred in the northwest coast of Baja California, Mexico. Nine die-offs occurred from May 31st to August 2nd in Todos Santos Bay, Salsipuedes Bay and Coronado Islands. The organisms were disoriented, gasping, swimming erratically, and died hours after these signs were detected. Necropsies and histopathological analyses were performed on dead organisms. Abundant mucus and congestion was observed in the gills. Histopathological analysis of the gills showed hyperplasia, fusion of gill filaments and lamellae, telangiectasia, edemas, increased numbers of mucus cells, and in some cases severe hemorrhage. Water samples were analyzed and a sampling campaign was implemented in some cultivation areas to evaluate the presence of ichthyotoxic microalgae. Chattonella spp. (mainly C. cf. marina) were detected in the water column during the MMP. At the end of May abundances of 5 × 103 cells L−1 were detected in sea surface samples and Chattonella spp. represented ~20% of the microphytoplankton community. Abundance of these species at surface increased to 33 × 103 cells L−1 in June and represented 85% of the phytoplankton community. No other environmental stressful variables were detected during the MMP. The presence of Chattonella spp. in the water column explains the dead of the tuna since behavior, necropsies, and histopathological analyses of the gills indicate a severe reaction to an environmental noxa that could be related to the characteristic toxic effect of these species. Before the MMP, ichthyotoxic species have not been reported in the phytoplankton community of the region. Accumulation of Chattonella spp. was probably associated with abnormally high temperatures present during the two previous years before the MMP. Surface temperature anomalies of 3°C were registered during 2015. Mesoscale oceanographic and atmospheric phenomena brought the environmental conditions for a change in the phytoplankton community in the region. Phytoplankton biomass was low and associated with a decrease in the abundance of diatoms and dinoflagellates. The absence of diatoms together with upwelling events followed by stratification before the MMP probably favored the accumulation of Chattonella spp. that affected importantly tuna ranching activities in Northwest Baja California.
Introduction
Harmful algal blooms (HABs) can affect economically important coastal activities. Among these, marine aquaculture is highly susceptible to HABs. Phycotoxin accumulation and mass mortalities are the two major noxious aspects of HABs on cultivated organisms. From an economic perspective, massive mortalities of fish is the main negative effect of HABs to marine aquaculture (Lewitus et al., 2012; Hallegraeff et al., 2017). Mortalities are associated with different causes but mainly with the presence of marine fish-killing microalgae species. For example, in 2016 more than 40,000 t of cultivated salmon in Chile were affected by the presence of the microalga Pseudochattonella cf. verruculosa (Clement et al., 2016; León-Muñoz et al., 2018). This is the most extraordinary mass mortality event of cultivated fish ever recorded, referred as the “Godzilla Red Tide” that caused an estimated loss of over 800M USD (Hallegraeff et al., 2017).
Ichthyotoxic microalgae belong to different taxonomic groups. Dinoflagellates, cyanobacteria, raphydophytes, chrysophytes, dictyochophytes (Silicoflagellates) have been implicated in wild and cultivate massive fish mortalities (Kim et al., 2007; Aoki et al., 2012; Imai and Yamaguchi, 2012; Kudela and Gobler, 2012). The rhaphydophytes is one of the most important group of ichthyotoxic microalgae that has caused significant economic losses worldwide. Mortalities have been associated mainly with blooms of Chattonella and Heterosigma akashiwo. This latter species has affected fish aquaculture principally in northern latitudes, while Chattonella species are distributed in tropical waters (Imai and Yamaguchi, 2012). In 1980, ~1,500 t of salmon cultivated in British Colombia were lost due to a H. akashiwo bloom (Bruslé, 1995; Lewitus et al., 2012; Esenkulova et al., 2014). The estimated loss was 35M USD (Rensel and Whyte, 2004; Hallegraeff et al., 2017). In 1990, H. akashiwo was again associated with another mass mortality of cultivated salmon in the same region (Black et al., 1991). Chattonella marina had been implicated in more fish mass mortalities than other raphydophytes species. Particularly, blooms of this microalga have affected importantly yellowtail finfish (Seriola quinqueradiata) aquaculture in Japan and Korea. The first registered mass mortality occurred in 1973 in the Japanese Seto Inland Sea (Imai et al., 1991). The estimated loss was 90M USD (Imai and Yamaguchi, 2012). In the 1970s and mid1980s, C. marina blooms caused other mass mortalities of yellowtail finfish (Imai et al., 2006). This species was also associated with a mass mortality of captive southern bluefin tuna Thunnus maccoyii in Australia (Munday and Hallegraeff, 1998). In April 1996, ~1700 t of captive tuna died in Boston Bay, South Australia (Munday and Hallegraeff, 1998). The association of the fish mortality with the presence of Chattonella was unclear since the microalga was present at much lower abundances (maximum 66 × 103 cells L−1) than the ones reported when yellowtail finfish mortalities occur. Mortalities of this species occurs when cell abundances are in the order of millions per liter (Imai and Yamaguchi, 2012). However, microalgal toxicosis due to C. marina was the most plausible explanation for the tuna die-off (Munday and Hallegraeff, 1998). This is the only report of a negative impact to the tuna ranching industry by an ichthyotoxic microalga.
Tuna cultivation of northern bluefin tuna (Thunnus thynnus orientalis) is an important aquaculture activity in Mexico. Tuna ranching was established in 2002 in northern Baja California and three companies operate currently close to Todos Santos bay. Cultivated tuna represents more than 90% of the aquaculture production of Baja California and it is the second largest (behind shrimp production) aquaculture activity in terms of revenue in Mexico. Annual production of the last 5 years fluctuated around 6,000 t per year (Boletines Estadísticos de la producción pesquera y acuícola de Baja California; http://www.sepescabc.gob.mx). In summer of 2016, this industry experienced a period of mass mortalities of organisms maintained in different areas in northern Baja California. First mass mortalities were registered in May 31 to June 2 (Figure 1). Eighty percent of the biomass of some culture pens located in Punta Banda was affected (Personal communication; Baja Aquafarms Co.). In June 14, a second outbreak occurred in Salsipuedes Bay followed by another mass mortality in Punta Banda after 2 days (Figures 1, 2 for the location of the cultivation areas). Some cultivation pens were relocated from Todos Santos Bay to Coronado Islands as a response to the registered events. However, mortalities also occurred in this location at the end of July. In total, nine die-offs were registered at different locations from May 30 to August 4 (Figure 1) hereafter, referred as the mass mortality period (MMP). This phenomenon has never been recorded before in the region. We implemented a sampling campaign during the MMP to identify the causes of the tuna mass mortalities. Water samples and hydrographic variables were analyzed. In addition, analyses on affected organisms were conducted. Here, we report that the tuna disease causative agent was raphydophyte species of the genus Chattonella, mainly C. marina. The presence of these species was probably associated with abnormal environmental conditions present in the region before the MMP. In addition, we describe the behavior and lesions of affected organisms caused by these microalgae.
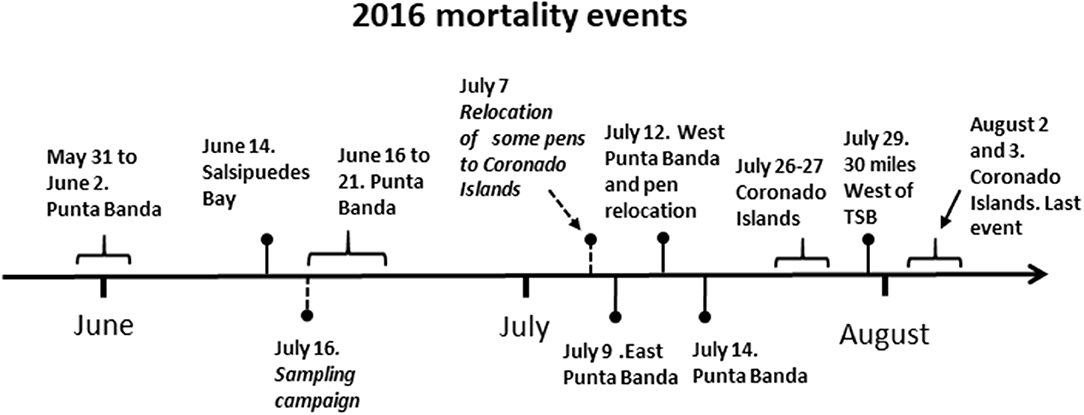
Figure 1. Time line of the events during the period of occurrence of mass mortalities of northern bluefin tuna Thunnus thynnus orientalis cultivated in the northwest coast of Baja California, Mexico.
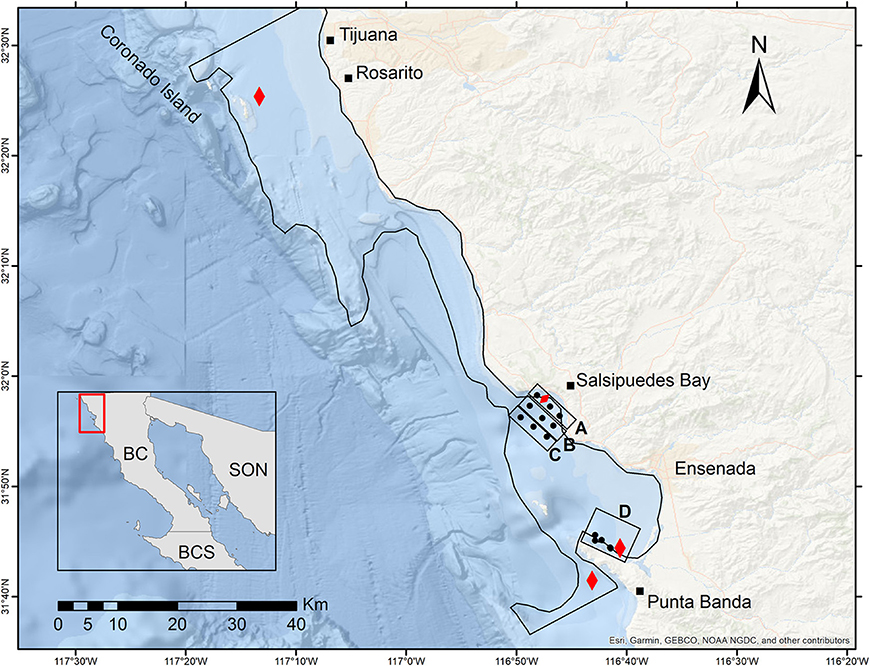
Figure 2. Location of tuna production areas (red symbols) in the northwest coast of Baja California, Mexico. The round closed symbols represent the stations covered during the sampling campaign implemented the 16 of June during the mass mortality period. The coastal (line A) and offshore (lines B and C) stations are indicated in the map. In addition, stations in Punta Banda area are indicated (D). The black line delimits the integration area A used in the calculation of VTN.
Materials and Methods
Description of the Study Area and Location of the Cultivation Zones
The study area is located in Todos Santos Bay (TSB) region on the Baja California Peninsula (31° 52′ N, 116° 37′ W). The bay has two mouths, which communicate with the Pacific Ocean. Two islands, called Todos Santos, divide the two communication mouths of the bay. Seventy five percent of the area presents depths < 50 m and 25% is part of a narrow submarine canyon located between Punta Banda peninsula and Todos Santos Islands (Figure 2). TBS is an area influenced by the California Current (CC) that transports water southward. Additionally, wind forcing causes coastal upwelling with a marked seasonal cycle (Durazo, 2009). The concessions for cage-cultivation of tuna are located mainly in Todos Santos Bay region (Punta Banda and Salsipuedes Bay). A systematic sampling was implemented in these locations (Figure 2).
Sample Campaign (June 16) and Data Collection
The sampling campaign was conducted aboard the research boat Rigel of the Center for Scientific Research and Higher Education at Ensenada (CICESE). Water samples were collected at 16 stations in TSB region. At each station, temperature, salinity, and pressure were measured with a continuous temperature, salinity depth profiler (Sontek, CastAway CTD). The water samples were collected at different depths with a 5-L Niskin bottle.
Phytoplankton Community Evaluation and Pigment Analysis
Of the water collected with the Niskin bottles, a 50 mL aliquot was placed in amber bottles (Nalgene type). The samples were fixed with a solution of paraformaldehyde, glutaraldehyde 1% with HEPES buffer (pH 7.4) and sucrose, as described by Katano et al. (2009). This solution preserved microalgae species with ichthyotoxic potential. The phytoplankton community was analyzed using the Utermöhl method (Sournia, 1978). Ten, 25, or 50 mL were sedimented and the complete area of the sedimentation chamber was analyzed with an inverted light microscope (Leica DM3000 model). Diatoms, dinoflagellates, raphidophytes, and other phytoplankton groups were identified up to genus level and in some cases to species level according to Tomas (1997) and Omura et al. (2012). Abundance is reported in cells L−1. In addition, the presence of Chattonella species is expressed as relative abundances in relation of the total abundance of the microphytoplankton community.
One liter of water was vacuum filtered to determine the concentration of phytoplankton pigments. The water was passed through GF/F (Whatman) glass fiber filters. The analysis of the pigments was performed by high-performance liquid chromatography (HPLC). The method used was the one described by Van-Heukelem and Thomas (2001) and modified by Almazán-Becerril and García-Mendoza (2017).
Analyses on Affected Organisms
Histological Procedures
During the MMP, 14 tunas (136.6 ± 19.5 cm of total length) were analyzed. Moribund animals were captured directly from the cage nets and sacrificed in situ. Pieces of gills were excised and fixed in 10% neutral buffered formalin, placed in histological cassettes and send to the laboratory. The tissues were decalcified with tetrasodium ethylenediaminotetracetate (EDTA) for a period of 3 to 10 days. The gills were dehydrated in an ethyl alcohol series of ascending concentrations, embedded in paraffin and sectioned at 5 mm. The tissue sections were stained with haematoxilin-eosin (HE), 3 sections of each tissue from each fish were examined by light microscope. The presence of histological alterations was recorded and photographed.
Analysis of Environmental Variables
Regional-Environmental Indicators
Long-term series of monthly anomalies of several physical and biological fields were constructed in order to describe the interannual variability of the environmental conditions close to the study area. The period for these series was from the year 2003 through year 2017, coincident with the availability of the satellite imagery used in this analysis. For all the variables, which are described below, those data points located over the continental shelf (shoreward of the 200-m isobath) and between Punta Banda Peninsula and Coronado Islands (see Figure 2) were averaged (or integrated) for each month in the series. A monthly-mean climatology was then calculated and subtracted to the monthly composites to obtain the anomalies for the series.
Satellite Imagery
Monthly composites of satellite-derived products were used in the long-term anomaly series. These products included the 4 km-resolution version of the 4 μ night-time sea surface temperature (SST) and default algorithm (OCI) based chlorophyll-a (CHL), taken from the Moderate Resolution Imaging Spectroradiometer (MODIS)—Aqua product. These data were obtained from the National Aeronautics and Space Administration (NASA) OceanColor Web: http://oceancolor.gsfc.nasa.gov.
Coastal Upwelling Index
To analyze the variability of the regional upwelling regime, a monthly coastal upwelling index (CUI) was calculated using the wind stress obtained from the 3-h 25 km-resolution wind vector at 10-m height from the North American Regional Reanalysis (NARR; Mesinger et al., 2006) and using parameterizations proposed by Smith (1988). This calculation was done according to the definition used by Bakun (1973) and Schwing et al. (1996), assuming a coast oriented 28° Clockwise from the north.
Numerical Oceanic Model
Numerical-model outputs were also included in the series to obtain information of the conditions of the water column during the anomalies of the surface fields described above. The numerical model used in this analysis was the regional physical-biogeochemical coupled model used in Cruz-Rico and Rivas (2018) but with some differences (described below). This biogeochemical model is a nutrients-phytoplankton-zooplankton-detritus (NPZD) model based on nitrogen (Powell et al., 2006). The total nitrogen at any point is partitioned between dissolved nitrogen (N), phototrophic phytoplankton (P), the herbivorous zooplankton (Z), and particulate nitrogen (D: Detritus). Major biological processes (i.e., photosynthetic growth and uptake of nitrogen by phytoplankton, grazing on phytoplankton by zooplankton, mortality of both phytoplankton and zooplankton, and sinking and remineralization of detritus), and physical processes (i.e., advection and mixing) affecting the biological components (N, P, Z, D) are included in the biochemical model which runs together with the physical model as one (Powell et al., 2006).
The differences between our model approach and that used by Cruz-Rico and Rivas (2018) are the boundary data and surface forcing. In our approach, monthly data from the Global Ocean Data Assimilation System (GODAS; e.g., Huang et al., 2008; Ravichandran et al., 2013) were used in the model's lateral open boundaries, and daily wind stress calculated from the NARR's wind and monthly heat fluxes from the GODAS were used in the model's free surface. The simulation period was also 2003–2017.
An indicator of the nutrient availability for phytoplankton growth was diagnosed from the numerical-model outputs and the vertical transport of nitrogen through the 50-m depth. This transport was calculated as
where w is the vertical velocity, N is the dissolved nitrogen (nitrate), and A is the area of the horizontal plane limited offshore by the 200-m isobath and extending from Todos Santos Bay to Coronado Islands located at 50-m depth; the total area A is 1.546 × 103 km2.
On the other hand, the Brunt-Väisälä frequency squared (BVF2) was calculated as an indicator of the stratification of the water column. The maximum of BVF2 (maxBVF2) and its depth were used to characterize the water-column conditions around the study area.
Teleconnection Index
A climatic index was also compared to the oceanographic variables around the study area in order to explore a possible relation between such variables and the large-scale climatic patterns. The Southern Oscillation Index (SOI) was used as an indicator of the El Niño activity around the study area. As reported in previous papers (e.g., Cruz-Rico and Rivas, 2018), the El Niño phenomenon modulates much of the dynamic over Baja California's shelf. Indeed, in particular for the warm anomaly occurred in the period 2013–2016, many of the effects are attributed to an intense El Niño event (e.g., McClatchie et al., 2016).
Results
Affectation of Organisms, Necropsy and Gill Histopathological Results
The first signs of distress of cultivated tuna were observed in May 31 (Figure 1). The organisms were disoriented, swimming slowly, and erratically, not moving in circles as in normal conditions. Some fishes were gasping, the opercula and mouth were open and the most affected organisms crashed against the nets of the pen (Video S1; Supplementary Material). The organisms died and sank to the bottom of the pens ~4 h after these signs were noticed.
Necropsies were performed in different organisms and organs were analyzed macroscopically and histopathologically. Liver and kidneys did not present signs of damage that represented a malfunction of the organs. In contrast, gills of the evaluated organisms were heavily affected. Excessive mucus and excretion of blood was observed in the gills of an organism analyzed in June 15. The observation of mucus under the microscope revealed the presence of microalgae species (Figure 1, Supplementary Material). Some cells of Tripos furca were visible and round or oval cells were also observed that probable were Chattonella spp., see below.
Histopathological analysis revealed the presence of diffuse congestion by erythrocytes of branchial lamellae (Figures 3A,B). Multifocal telangiectasia, which is the result of rupture of pillar cells in the distal part of the branchial lamellae was also observed and in some areas rupture of lamellae epithelia was evident releasing erythrocytes (Figures 3C,D). In some cases, multifocal telangiectasia occupied the entire branchial lamellae showing the magnitude of damage (Figures 3E,F). Severe inflammation and hyperplasia of both, gill filaments, and lamellae, including fusion and shortening of lamellae were common recorded (Figures 4A,B). In several cases, the total loose of gill lamellae with severe inflammation, hyperplasia, and necrosis were noted (Figures 4C,D). Additionally, detachment of lamellae epithelium, consistent with diffuse edema, and vacuolization were observed (Figures 4E,F). Hemorrhagic zones were observed over the gills filaments and lamellae (Figures 5A,B).
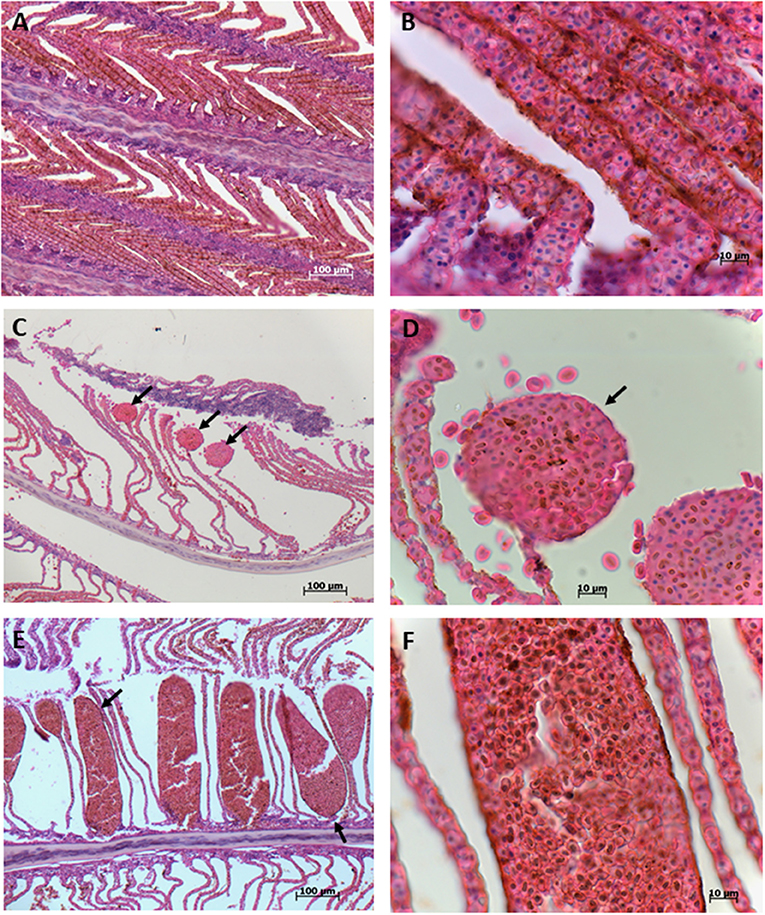
Figure 3. Gills of blue tuna (Thunnus thynnus orientalis), exposed to a harmful algal bloom of Chattonella marina. (A) The presence of diffuse congestion by erythrocytes of the branchial lamellae is observed. (B) Detail of erythrocyte accumulation (congestion) of the branchial lamellae. (C) Multifocal telangiectasia (arrows), resulted of rupture of pillar cells, in the distal part of branchial lamellae (aneurysm). (D) Detail of the top of branchial lamellae telangiectasia (arrow) where release of some erythrocytes is observed, evidencing rupture of lamellae epithelia. (E) Large multifocal telangiectasia of entire branchial lamellae plethoric of erythrocytes. (F) Close up of erythrocytes accumulated in branchial lamellae, note hemosiderin granules.
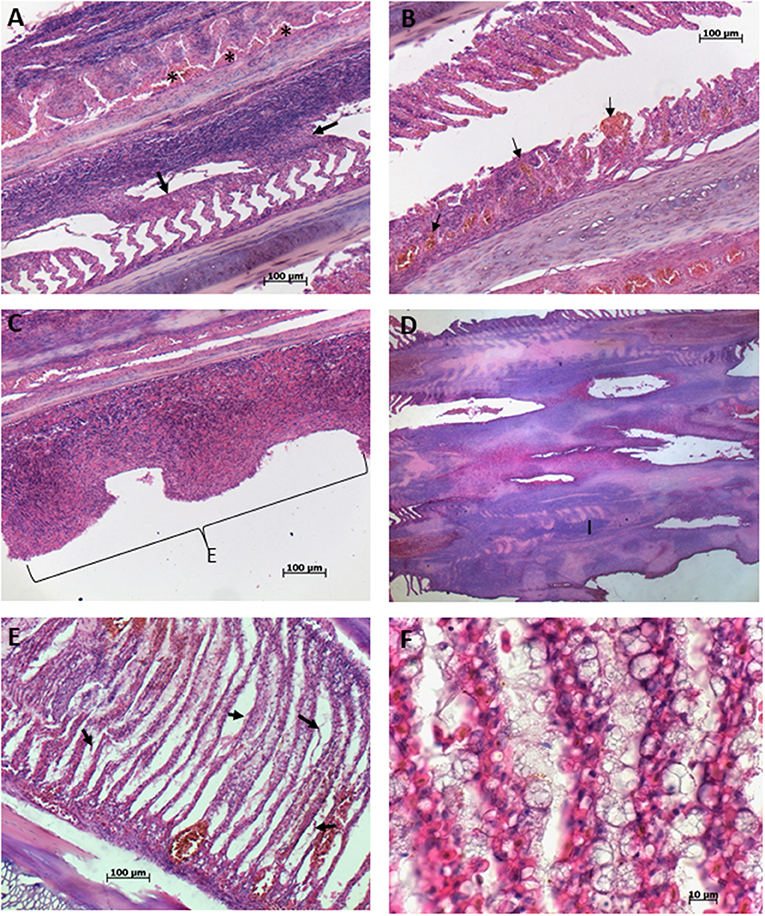
Figure 4. Gills of blue tuna (Thunnus thynnus orientalis), exposed to a harmful algal bloom of Chattonella marina. (A) Severe inflammation and hyperplasia of gill filaments and lamellae, showing fusion of lamellae (arrows), note accumulation of erythrocytes (asterisks) in the middle area of filament. (B) Fusion and shortening of gill lamellae with multifocal telangiectasia (arrows). (C) Total loose of gill lamellae with severe inflammation and necrosis of the gill filament. (D) Severe inflammation, hyperplasia, fusion, and necrosis of filament with almost the totality loose of gill lamellae. (E) Detachment of lamellae epithelium (arrow) consistent with diffuse edema and vacuolization. (F) Close up of gill lamellae severely vacuolated.
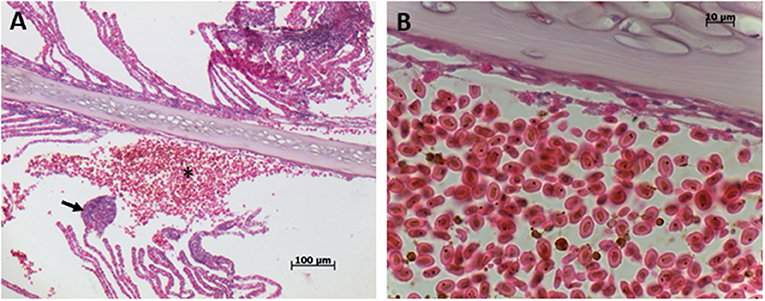
Figure 5. Gills of blue tuna (Thunnus thynnus orientalis), exposed to a harmful algal bloom of Chattonella marina. (A) General view of gill filament with hemorrhagic zone (asterisk) where all lamellae are loosed, note telangiectasia in the distal area of gill lamellae (arrow) of the contiguous filament. (B) Detail of hemorrhagic zone plenty of erythrocytes with hemosiderin granules, note necrosis of the basal epithelia close to the cartilage of the filament.
Identification of the Causative Agent
Analyses of water samples from Punta Banda and Salsipuedes Bay started after the report of the first die-off. Samples were collected at different days during the mass mortality period (MMP, Figure 1) and to the end of August in 2016. After this month, analysis of samples continued on weekly basis as part of the continuous monitoring programs of Baja Aquafarms Co. and CICESE. The ichthyotoxic microalgae Chattonella spp. were present in the tuna cultivation areas during the MMP. The species was identified as C. marina according to cell morphology of the organism observed in water samples without any fixative (Figures 6A,B). The cells presented a tear (oblong to ovoid) shape morphology and were 35 to 60 μm long. Several chloroplasts arranged close to the cell wall were evident (Figures 6A,B). Cells with different morphologies were also present in the samples (Figures 6C,D) but the most conspicuous and abundant organism was the one identified as C. marina. Raphydophytes are susceptible to some preservatives and cell morphology was affected by the addition of lugol-acetate to the samples (Figure 6E). Several fixatives were tested during the monitoring period. Using paraformaldehyde, 1% glutaraldehyde, HEPES and sucrose (Katano et al., 2009) proved to be adequate to preserve the samples with minor alterations of Chattonella cells (Figure 6F). Although it was possible to identify the organisms as Chattonella, the differentiation between morphotypes was not possible in preserved samples. Identification of Chattonella species using morphological characteristics is sometimes ambiguous. Therefore, several isolates were established from cells collected during the MMP. Cultures of two strains were established. The strains were identified with the D1/D2 large DNA subnunit sequences as Chattonella marina var. ovata (Figure 6G) and C. minima (Figure 6H) (Ahumada-Fierro, 2017). Therefore, different species (or varieties) of Chattonella were present during the die-off period and the abundance is presented as Chattonella spp.
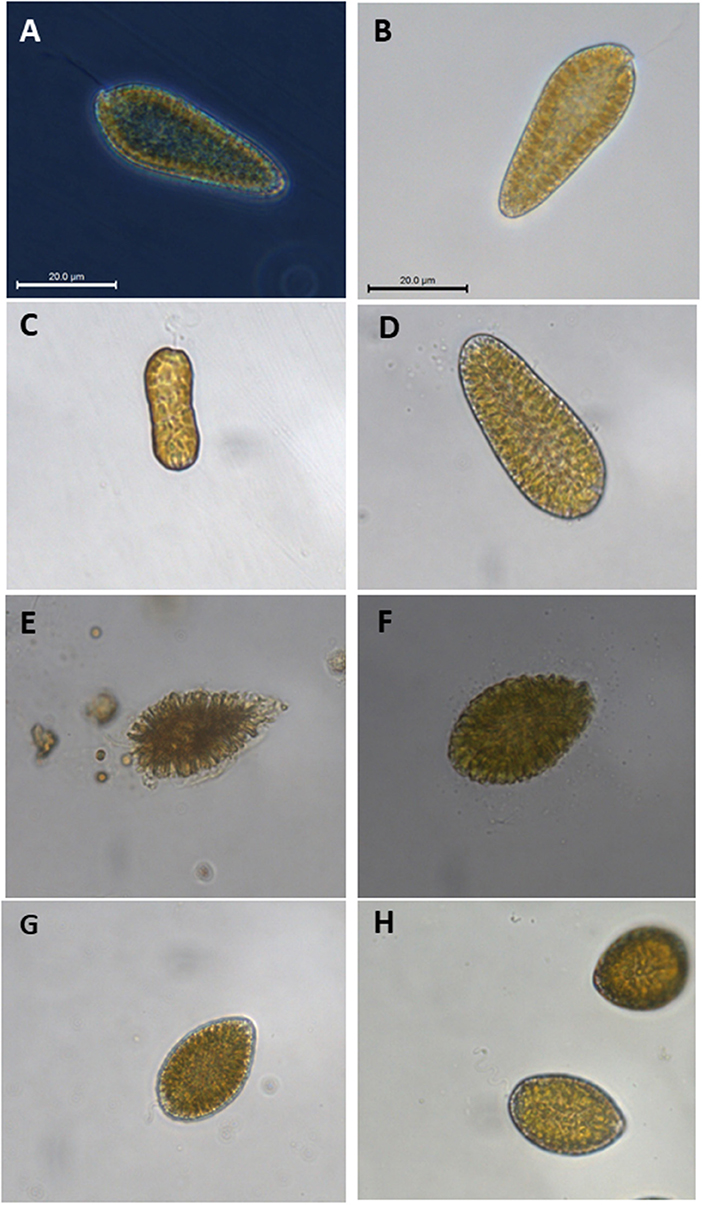
Figure 6. Chattonella spp. in water samples collected during the mass mortality period of northern bluefin tuna Thunnus thynnus orientalis cultivated in the northwest coast of Baja California, Mexico. Panels (A–D) shows cell observed in water samples without any fixative. Addition of Lugol-acetate affected significantly the morphology of the cells (E). Less affectation of Chattonella cells was observed (F) with paraformaldehyde, 1% glutaraldehyde, HEPES, and sucrose (Katano et al., 2009). Cultivated strains isolated during the (MMP) are also presented: Chattonella marina var. ovata (G) and C. minima (H).
The abundance of phytoplankton and Chattonella spp. in samples collected in Punta Banda and Salsipuedes from the end of May to December 2017 is presented in Figure 7. Chattonella spp. abundance at the end of May was ~5 × 103 cells L−1 and reached a maximum of 30 × 103 cells L−1 in the second week of June (Figure 7B). Chattonella spp. abundance was higher than 10 × 103 cells L−1 from June to the beginning of August, particularly in surface samples (Figure 7B). Cell abundance decreased after August and only an abundance above 10 × 103 cells L−1 was detected in one surface sample after this month. Low phytoplankton abundances (below 50 × 103 cells L−1) were registered during the period of the appearance of Chattonella spp. (Figure 7A). Therefore, these species were highly represented in the phytoplankton community. In some samples, relative abundances of Chattonella spp. were higher than 60% (maximum relative abundance of 83% by the middle of June) of the microphytoplankton community (Figure 7C). These species were not detected or were present at abundances lower than 1 × 103 cells L−1 after August. Phytoplankton abundance increased after this month (Figure 7A).
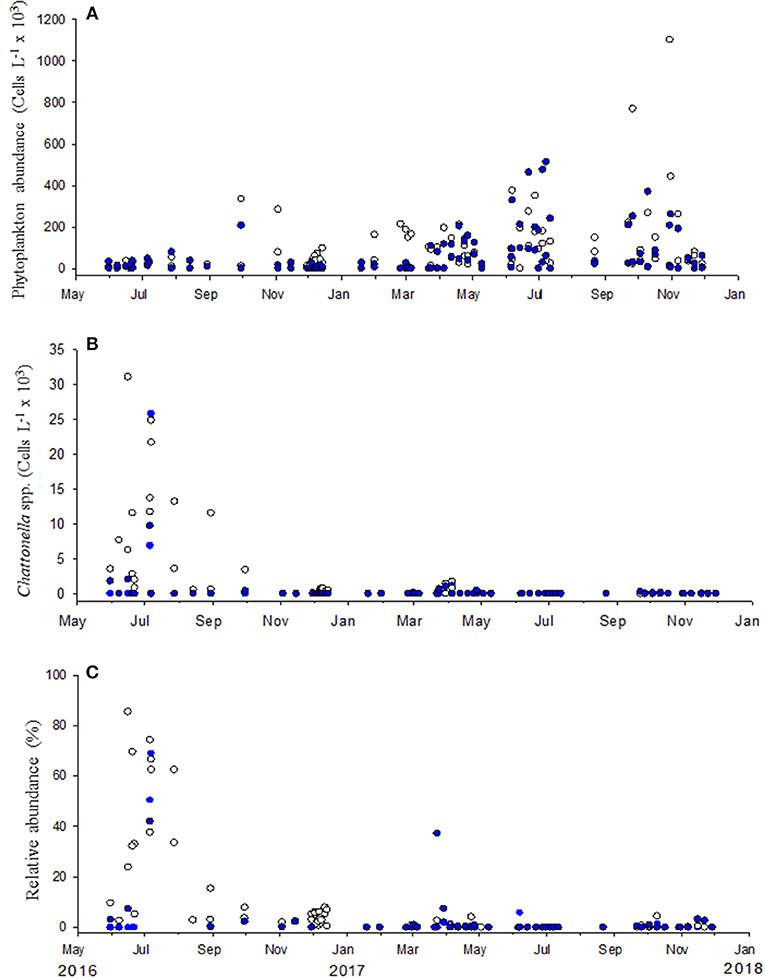
Figure 7. Phytoplankton (A) and Chattonella spp. (B) abundance in samples collected in Punta Banda and Salsipuedes from the end of May to December 2017. The relative abundance of these species is presented in (C) Abundances in surface samples are represented by empty symbols and blue circles are abundances detected at 10 m depth.
Environmental Conditions Associated With the Presence of Chattonella
To characterize the spatial distribution of ichthyotoxic species and environmental variables close to the tuna cultivation areas, a sampling campaign was implemented after the second mass mortality episode that occurred in Salsipuedes bay (Figure 1). In June 16, phytoplankton abundance was evaluated in nine sampling stations in this area (Figure 2, lines marked as A, B, and C) and in five stations in Punta Banda (Figure 2, area D). We found high abundances of Chattonella spp. in the surface and close to the coast in Salsipuedes bay. Abundances of ~30 × 103 cells L−1 were detected at surface in three stations located between 0.77 and 1.79 miles from the coast (Figure 8A). Chattonella abundance decreased to ~25 × 103 cells L−1 at 10 m and decreased significantly at 20 m sampling depth in these stations (Figure 8A). The abundance of this species at surface decreased to approximately to 10 × 103 cells L−1 and 5 × 103 cells L−1 in offshore stations (Figures 8B,C). In Punta Banda (Figure 8D) Chattonella spp abundance was lower than in Salsipuedes Bay. These species were detected from surface to ~15 m depth (Figure 8D).
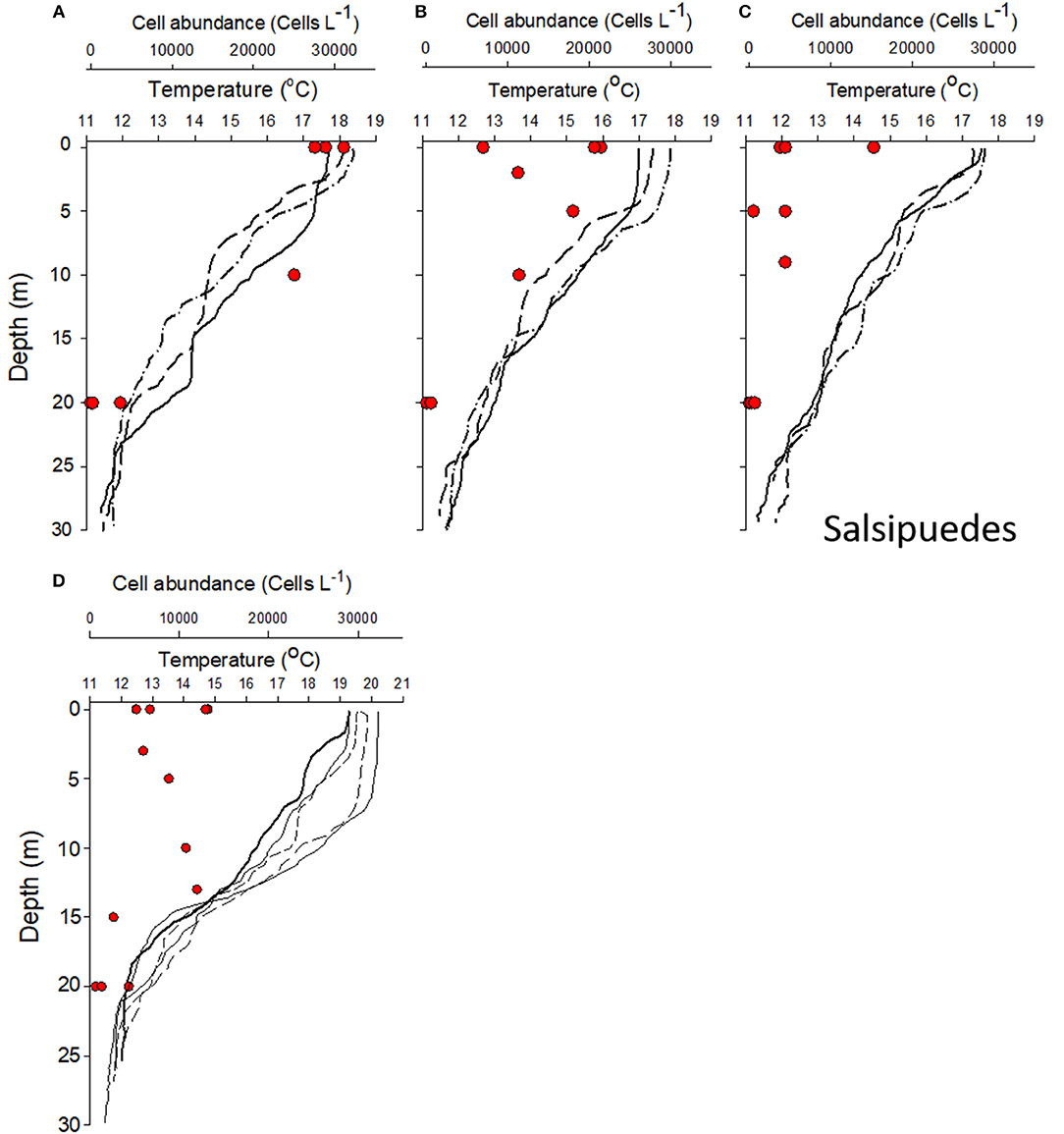
Figure 8. Chattonella spp. abundance (in cells L−1, red circles) and temperature distribution (lines) in the water column in tuna cultivation areas after a mass mortality episode that occurred in Salsipuedes Bay. (A) presents the abundance and temperature in three stations located close to the coast (see Figure 2 for the distribution of the stations). (B,C) shows the results of samples collected in offshore stations. (D) shows the results of registered in Punta Banda area (see Map in Figure 2 for the localization of sampling stations).
Phytoplankton pigments were also determined in samples collected during the campaign. CHL, fucoxanthin, violaxanthin, and chlorophyll C2 were highly represented in samples dominated by Chattonella spp. (Figure 2A, Supplementary Material). Pigment concentration was high relative to phytoplankton abundance registered in water samples. CHL concentration was ~3 μg L−1 in samples collected at surface in coastal stations. In these stations, microphytoplankton abundance was lower than 40 × 103 cells L−1 and Chattonella spp. represented ~80% of this abundance. Therefore, accumulation of CHL was associated with the presence of Chattonella spp. in the water column (Figure 2B, Supplementary Material).
There was not a clear relation between the distribution of Chattonella spp. and the thermal structure of the water column during the sampling campaign. Surface temperature was similar (mean of 17.8°C) in all Salsipuedes stations (Figures A–C). Also, a clear thermal stratification of the water column was not evident between coastal and offshore stations. There was no evident mixed layer and the temperature decreased monotonically with the depth in most of Salsipuedes stations (Figures 8A–C). In contrast, a well-defined mixed layer was evident in some stations of Punta Banda (inside TSB). The thermocline was evident at ~8 m depth in some stations (Figure 8D). Also, surface temperature in Punta Banda was higher than in Salsipuedes. The maximum surface temperature was 20.3°C in this area (Figure 8D). Under these conditions, Chattonella abundances were ~10 × 103 cells L−1 from surface to the bottom of the thermocline in Punta Banda (Figure 8D).
Chattonella species thrive in warm temperatures and blooms of this species have been reported when water temperature is above 20°C (Edvardsen and Imai, 2006; Imai and Yamaguchi, 2012). The presence of these species and its relation with the thermal conditions was not clear during the sampling campaign. Therefore, we analyzed the variation of the temperature measured continuously in-situ at various depths in Punta Banda since 2012. Two short upwelling pulses occurred at the beginning of the MMP (Figure 9). Thereafter, surface temperatures increased and ~23°C occurred in the Punta Banda region during the MMP. In addition, stratification was evident during this period and the 18°C isotherm was detected between 8 and 10 m depth. Only in few occasions, this temperature were registered below 10 m depth in the rest of the year (Figure 9). Most notably, temperatures above 18°C were registered from surface to the bottom of the water column in the two previous years of the MMP. Temperatures in the bottom of the water column above 18°C prevailed for ~5 months, from August to December in 2015 (Figure 9). This condition was not registered in other years.
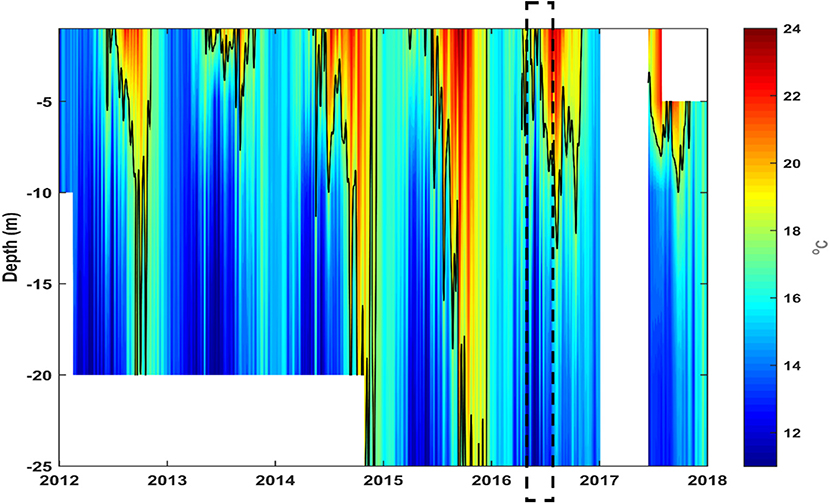
Figure 9. Water temperature measured continuously in-situ since 2012 at various depths in Punta Banda cultivation area. The mass mortality period is highlight. The data was obtained with a set of temperature data loggers (HOBO U22-001) placed at different depths in the water column.
In-situ thermal conditions indicate that abnormally warm years occurred in the region before 2016. The interannual variability of the environmental conditions in the region was analyzed to evaluate the anomaly of the conditions related to the MMP. This period (May-August 2016) was characterized by moderate La Niña conditions. Early 2016 and during the MMP the waters were anomalously warm (~1–2°C) but colder than in the previous months when the anomalies were higher than 2°C with a maximum exceeding 3°C in October 2015 (Figure 10B). During this year and in 2014 intense El Niño conditions were evident (Figure 10A). Associated with this conditions, almost 3 years of excessively low levels of CHL were registered in the region. Right after one of its lowest values in April 2016, the CHL levels became nearly normal (Figure 10C). In the months prior to the MMP the upwelling activity was intense, especially from December 2015 to March 2016, after that the upwelling was nearly normal with slightly intense short periods (Figure 10D). The strong positive upwelling anomaly was related to an intense vertical nutrient supply estimated by numerical-model outputs in the first months of 2016, especially in March, reaching lower values (but still over the normal) in the MMP (Figure 10E). From late 2015 (November and December) through the MMP the stratification became stronger, except in July-August 2016, with a thermocline (i.e., depth of the maxBVF2) becoming shallower and then nearly normal (Figure 10F). After the MMP, SST, and SOI, CHL concentration were nearly normal, positive or close to the climatological mean and the SOI was nearly normal (Figure 10).
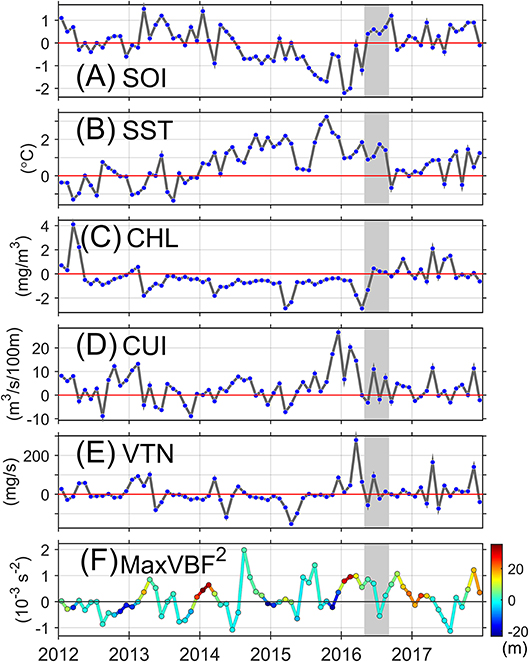
Figure 10. (A) Monthly values of the southern oscillation index (SOI), and monthly anomalies (with respect to the 2003–2017 climatology) of (B), surface temperature (SST); (C), chlorophyll-a concentration (CHL); (D), coastal upwelling index (CUI); (E), vertical transport of nutrients (VTN), and (F), magnitude and depth (line's color) of the maximum Brunt-Väisälä frequency (maxBVF2) for the period 2012–2017. The shaded area indicates the period mass morality period. Positive values of the SOI correspond to La Niña, whereas negative values correspond to El Niño. Positive values in the VTN correspond to an upward nitrogen supply.
Discussion
Here, we document for the first time mass mortalities of cage-reared tuna caused by raphytophytes species of the genus Chattonella in Northwest Baja California, Mexico. No other environmental stressful variable was detected during the mass mortality period (MMP). The presence of Chattonella species, but most probably C. marina, in the water column explains the death of the tuna since behavior of the organisms, and histopathological analyses of the gills indicate a severe reaction to an environmental noxa that could be related to the characteristic toxic effect of these ichthyotoxic raphydophytes.
The gills functions include not only respiration but also osmoregulation and excretion. Since the gills are in contact with the environment, they are particularly sensitive to presence of noxas in the water. The significant morphological changes of the gill tissue of tunas exposed to C. marina are characteristic of an exposure of fishes to an acute-type noxa or contaminants (Mallatt, 1985; Godoy, 2016). Changes such as hypertrophy, hyperplasia, epithelial lifting, partial fusion of some lamellae of gill epithelium constitute adaptive changes that can be reversed if the external noxa is eliminated. In contrast, other changes are more severe and if are extended, impairment of the normal functioning of the tissue and irreparable damage occurs, even with elimination of the noxa in the water. These changes correspond to lamellar telangiectasis (aneurysm), rupture of epithelial cells with hemorrhages and necrosis (Fernandez and Mazon, 2003; Camargo and Martinez, 2007; Godoy, 2016). These alterations were documented in all gill samples analyzed from organisms that died in different dates during the MMP. Similar alterations in the gills of fishes exposed to Chattonella spp. have been observed in yellow tail, Seriola quinqueradiata in Japan (Ishimatsu et al., 1996); Tilapia, Oreochromis mossambicus, from the Salton Sea, California (Tiffany et al., 2001), and the Atlantic salmon, Salmon salar in Chile (Godoy, 2016). Lethal effects associated with Chattonella spp. has been recorded in S. quinqueradiata in Japan, southern bluefin tuna (Thunnus maccoyi) in Australia, and salmon (S. salar) in Norway and Chile (Imai and Itoh, 1987; Munday and Hallegraeff, 1998; Godoy, 2016).
The precise affectation mechanisms of the gills by Chattonella is not clear. It was proposed (Ishimatsu et al., 1996) that oxygen radicals released from Chattonella, stimulate mucus cells in the gills, and the secreted mucus, possibly plus Chattonella cells trapped within the mucus, impedes the gas exchange capacity of the gills by shunting respiratory water current away from the lamellae. Khan et al. (1996) proposed that brevetoxins produced by C. marina in conjunction with superoxide radicals (ROS) cause gill epithelium to become swollen with massive mucous production resulting in fish suffocation. However, production of brevetoxins by Chattonella has been disputed since these phycotoxins have not been detected by LC-MS/MS analysis (Hallegraeff et al., 2017). What is clear is that ROS play an important role in the affectation of the gills since raphydophytes produce high amounts of these compounds and together with polyunsaturated fatty acids (PUFAs) produce other toxic compounds through lipid peroxidation by increasing superoxide dismutase activity and damage gill cell membranes (Dorantes-Aranda et al., 2015). Therefore, the toxicity of PUFAs increased synergistically in the presence of ROS (Marshall et al., 2003).
It well recognized that intense blooms of raphydophytes have been responsible for several mass mortalities of cultivated and wild fish and represent a threat to marine fish aquaculture (Imai and Yamaguchi, 2012). However, two main questions arise from the analysis of the extraordinary event reported in this work: (1) Why a relatively low abundance of Chattonella species has such a devastating effect on tuna? and (2) What caused the accumulation of Chattonella spp. since these species have not been reported before in the region?
A high toxic potential of Chattonella species during the bloom or (together with) a high susceptibility of affected organisms are possible answers for the first question. After the first report of the effect of Chattonella to cultured tuna in southern Australia, investigations on strains isolated from this region have been performed. These strains showed higher growth rates than Japanese strains at high irradiances (Marshall and Hallegraeff, 1999). Maximum growth rate of the Southern Australian strain was registered at 400 μmol quanta m−2 s−1 and they have been recognized as a high-light adapted microalga strains (Marshall and Hallegraeff, 1999; Hallegraeff et al., 2017). In addition, the C. marina Australian strain probed to be more toxic to fish gill cells due to an elevated production of the eicosapentaenoic fatty acid and superoxide anions (Dorantes-Aranda et al., 2013). The mortality of cage-reared tuna in the Todos Santos bay region can be related also to the presence of highly toxic Chattonella strains. Abundances were in the same order of magnitude during the MMP and the outbreak that occurred in Australia. We registered a maximum cell abundance of 33 × 103 cells L−1. However, a tuna ranching company reported (abundance was evaluated with Sedgwick-rafter chambers) a maximum abundance of 90 × 103 cells L−1. In comparison, cells abundances in the Australian outbreak were between 1 × 103 cells L−1 and a maximum of 66 × 103 cells L−1 (Munday and Hallegraeff, 1998). Toxic potential of the species from our region has to be investigated but it is clear from the only two reported mass mortalities of cultivated tuna that the lethal abundance for tuna is one order of magnitude lower than the one considered for other economically important fishes. Affectations to the yellow tail S. quinqueradiata are expected when Chattonella cell abundances are higher than 500 × 103 cells L−1.
Tuna susceptibility to ichthyotoxic species has not been investigated. Behavior and affectation of the gills of the organisms during the outbreak of Australia were similar to our observations. Therefore, it seems that tuna is particularly susceptible to ichthyotoxic microalgae. Shen et al. (2011) proposed that susceptibility of marine fish to C. marina appears to be inversely related to their tolerance to hypoxia. However, T. maccoyii is highly tolerant to conditions of low dissolved oxygen in the environment. The maintenance metabolism, routine swimming, and specific dynamic action of these species are not affected in moderate to severe hypoxia (Fitzgibbon et al., 2010). Probably, oxygen uptake adaptations that support the high metabolic demand of tuna such as a large gill surface area and thin gill epithelium (Korsmeyer and Dewar, 2001) make these organisms extremely susceptible to the ROS and PUFAs produced by ichthyotoxic species. This has to be corroborated but a probably high susceptibility of tuna is the most plausible explanation for the devastating effect of the noxious C. marina on these organisms. Striped bass is also cultivated in the region and there were no reports of affection to this species during the MMP.
What caused the accumulation of Chattonella spp. since these species have not been reported before? It is important to try to understand how the Chattonella spp. HAB developed in the region. We have been monitoring the phytoplankton community intermittently for more than 15 years at different sampling points in TSB and semicontinuously since 2010 in Rincón de Ballenas where bivalve mollusks are cultivated and it is located 2 miles south from Punta Banda (Figure 2). There are also other phytoplankton monitoring programs in the region and Chattonella spp. have not been reported before. After the MMP the presence of ichthyotoxic species were monitored and Chattonella spp were registered in several samples. Abundances lower than 1000 cells L−1 were registered during some periods of the year and relative contribution to total abundance was generally not higher than 5% (see Figure 7). This indicates that Chattonella spp. are common species in the phytoplankton community of the region and are present at low abundances. Probably, these species were not detected before the MMP since the monitoring programs were not focused on the detection of ichthyotoxic species and samples were preserved with solutions that affect fragile phytoplankton cells. If they were present, probably they represented the seeding population for the HAB that affected the cultivated tuna. Alternatively, Chattonella spp. were transported into the region from other areas or cysts in the sediment were probably the source of cells for the initiation of the HAB during the MMP. Cysts were detected in sediments after the 2016 HAB (data not shown).
The source of the seeding population that originated the HAB during the MMP remains elusive. We cannot prove that Chattonella spp. or cysts were present before the MMP. However, it is important to try to identify the conditions that favored the accumulation of Chattonella. This was an extraordinary event not documented before in the region. Extraordinary environmental conditions were also present in the region before the MMP. Abnormally warm conditions were present the two previous years before 2016 and temperatures above 18°C were detected in the entire water column close to Punta Banda. In addition, there were two upwelling periods before the rise of the temperature and stratification in the water column during the MMP. The mesoscale interannual variability analysis demonstrated that these abnormal conditions started 3 years before 2016. In most of these 3 years before the MMP the El Niño conditions dominated in the study area. Notably, upwelling conditions were close to the climatological mean but high SST and low levels of CHL were present on these years. By the end of the year 2015 an upwelling intensification occurred, associated with an atmospheric low-pressure anomaly over the northeastern Pacific and an intensification of the North Pacific High Pressure System (Figure 3, Supplementary Material). This condition affected the northwestern Baja California coast through the first months of 2016, which caused a weakening of the SST anomaly, although it did not reach its normal values and the CHL showed no increase. According to the numerical model results the enhanced upwelling caused a stronger nutrient supply into the surface waters over the shelf combined with an enhanced stratification. Thus, the environmental conditions during the MMP turned into La Niña colder conditions with a relatively intense upwelling, accompanied with high levels of nutrients according to the model, and a relatively strong stratification, which caused an increment of the CHL but not phytoplankton accumulation. Notably, during the MMP microphytoplankton counts were extremely low but concentrations higher than 3 μg L−1 of CHL were detected in some samples. The high chlorophyll concentration was associated with the presence of Chattonella. This species presents high CHL cellular concentrations, up to 250 ng CHL cell−1 had been reported for C. marina maintained under culture conditions (Marshall and Newman, 2002).
We propose that extraordinary environmental conditions before the MMP permitted Chattonella spp. to thrive in the region. These conditions caused a change in the phytoplankton community normally observed in the region. The abundance of diatoms and dinoflagellates decreased significantly. Specifically, diatom abundance was unusually low in the previous years before 2016 (data not shown) and particularly during the MMP (Figure 4, Supplementary Material). Blooms of Chattonella occur when diatoms are scarce (Imai, 1990; Onitsuka et al., 2011; Aoki et al., 2015). The change in the phytoplankton community during the abnormal period and conditions (upwelling events followed by stratification periods) before the MMP were the probable causes for the accumulation of Chattonella spp. according to the conceptual model for the formation of blooms of these species.
The ecological interaction between Chattonella and diatoms is explained by the “Diatom resting hypothesis” proposed by Imai and Yamaguchi (2012). According to this hypothesis, blooms of Chattonella spp. are formed when diatoms are scare in surface waters and there is an input of nutrients associated with mixing processes after a period of strong stratification. Chattonellas show lower growth rate than diatoms (Imai and Yamaguchi, 2012). However, Chattonellas can dominate the phytoplankton community when diatoms population is in a resting stage in the sediments or they are physiologically inactive in the water column. Some diatoms species form resting stages and sink when there is a strong stratification accompanied by depletion of surface nutrients. Formation of diatom resting stages are essential for the increase of Chattonella abundance when the stratification breaks and nutrient are pumped into surface waters (Imai and Yamaguchi, 2012).
The increase in Chattonella spp. abundances during the MMP fits to this conceptual model for HABs formation in Seto Inland Sea in Japan developed after the observation of recurrent events in this region (Imai and Yamaguchi, 2012). Probably in the BTS region, the decrease of diatom abundance during the long period of abnormally warm conditions and the upwelling events that occurred at the end of 2015 and before the MMP brought the conditions for the increase of Chattonella abundance. After the MMP, “normal conditions” returned in 2016 and 2017 and phytoplankton abundance increased accompanied with the representation of diatoms in the phytoplankton community. We related a HAB not registered before to extraordinary environmental conditions that were present in the region. These two phenomena must occur recurrently to validate the concept that they were related. Logically, these will not be favorable for the mariculture industry of the region.
Impact to the Industry and Management of the Problem
The MMP affected importantly tuna-ranching activities in Northwest Baja California. This event is one of the largest economical negative impact for marine aquaculture industry related to a microalgae bloom in Mexico. An estimated value of the loses in one of the companies of the region was 42 million dollars according to insurance records (https://www.abacoadjusters.com/referencia/mortandad-de-atun-2/). Ecological information of some noxious species has been gathered from other regions with a long affectation history. This information is essential to understand the development of extraordinary blooms in our region. It is important to continue the monitoring of the phytoplankton community together with the characterization of environmental conditions to understand the bloom dynamics of Chattonella or other noxious microalga. Risk indexes for the presence of the noxious species including environmental and ecological variables should be developed to implement management plans to mitigate the effect of the species to the industry especially, if extraordinary events will become normal conditions.
Author Contributions
EG-M, analyzed and interpreted the data, reviewed the data for accuracy and integrity, wrote, and edited this manuscript. JC-M and RV-Y processed and analyzed histopathological samples, interpreted the results, wrote, and edited the manuscript. DR analyzed and interpreted mesoscale interannual data of the region, wrote, and edited the manuscript. MF-M analyzed samples, interpreted the data, elaborated figures, and participate in the edition of the manuscript. YS-B, analyzed phytoplankton samples and interpreted the data. JM-E participated in editing the manuscript.
Funding
CONACyT scholarship to MF-M. FORDECYT—CONACyT project number 260040-2015; Red Temática sobre Florecimientos Algales Nocivos (RedFAN) CONACyT 2015-2017 projects. DR was funded by CICESE through internal Project 625118.
Conflict of Interest Statement
The authors declare that the research was conducted in the absence of any commercial or financial relationships that could be construed as a potential conflict of interest.
Acknowledgments
We thank Baja Aquafarms S.A. de C.V for the help, support, and collaboration with CICESE, especially with FICOTOX laboratory. Also, we thank the company for sharing important information of the event. Particularly, we greatly appreciate the help and support of Javier Vivanco-Ocampo and Andres Ortinez-Escorza.
Supplementary Material
The Supplementary Material for this article can be found online at: https://www.frontiersin.org/articles/10.3389/fmars.2018.00454/full#supplementary-material
Video S1. The video was recorded during a mass mortality event of organisms maintained in Punta Banda. Organisms were disoriented, swimming slowly and erratically, not moving in circles as in normal conditions. The video shows a severely affected tuna crashing against the nets of the pen (video courtesy of Baja Aquafarms Co.).
Figure S1. Mucus present in the gills of a dead northern bluefin tuna Thunnus thynnus orientalis analyzed in June 15 in FICOTOX laboratory of CICESE. Cells of Tripos furca and round or oval cells were also observed.
Figure S2. A chromatograph of a typical pigment profile of samples dominated by Chattonella spp. (A) station E1, 33,000 cells L−1. Chlorophyll a concentration and Chattonella spp. abundance relation (B). The data was fitted to a linear regression model (solid line) passing through the origin considering that when Chattonella is not present the chlorophyll concentration is associated to other phytoplankton groups.
Figure S3. Geopotential height anomaly (with respect to the 1979–1995 climatology) at 500 hPa for December 2015. Plot taken from https://www.esrl.noaa.gov/psd/data/histdata.
Figure S4. Diatom abundance in samples collected in Punta Banda and Salsipeudes bay from the end of May to December 2017. Abundances in surface samples are represented by empty symbols and blue circles are abundances detected at 10 m depth.
References
Ahumada-Fierro, V. (2017). Caracterización de Aislados de Chattonella spp. de la Bahía de Todos Santos, y Evaluación de su Efecto Sobre Estadios Tempranos del Ostión Crassostrea gigas. Unpublished Bachelor's dissertation, Universidad Autónoma de Baja California, Ensenada, Mexico.
Almazán-Becerril, A., and García-Mendoza, E. (2008). Maximum efficiency of charge separation of photosystem II of the phytoplankton community in the Eastern Tropical North Pacific off Mexico: a nutrient stress diagnostic tool? Ciencias Marinas 34, 29–43. doi: 10.7773/cm.v34i1.1151
Aoki, K., Onitsuka, G., Shimizu, M., Kuroda, H., Matsuyama, Y., Kimoto, K., et al. (2012). Factors controlling the spatio-temporal distribution of the 2009 Chattonella antiqua bloom in the Yatsushiro Sea, Japan. Estuarine Coast. Shelf Sci. 114, 148–155. doi: 10.1016/j.ecss.2012.08.028
Aoki, K., Onitsuka, G., Shimizu, M., Yamatogi, T., Ishida, N., Kitahara, S., et al. (2015). Chattonella (Raphidophyceae) bloom spatio-temporal variations in Tachibana Bay and the southern area of Ariake Sea, Japan: interregional displacement patterns with Skeletonema (Bacillariophyceae). Mar. Poll. Bull. 99, 54–60. doi: 10.1016/j.marpolbul.2015.07.063
Bakun, A. (1973). Coastal Upwelling Indices, West Coast of North America. 194671. NOAA Tech. Rep. NMFS SWFSC 671. Seattle, WA: NOAA.
Black, E. A., Whyte, J. N. C., Bagshaw, J. W., and Ginther, N. G. (1991). The effects of Heterosigma akashiwo on juvenile Oncorhynchus tshawytscha and its implications for fish culture. J. Appl. Ichthyol. 7, 168–175. doi: 10.1111/j.1439-0426.1991.tb00523.x
Bruslé, J. (1995). The Impact of Harmful Algal Blooms on Finfish: Mortality, Pathology and Toxicology. Brest: REPÈRES OCEAN.
Camargo, M. M. P., and, Martinez, C. B. R. (2007). Histopathology of gills, kidney and liver of a Neotropical fish caged in an urban stream. Neotrop. Ichthyol. 5, 327–336. doi: 10.1590/S1679-62252007000300013
Clement, A., Lincoqueo, L., Saldivia, M., Brito, C. G., Muñoz, F., Fernández, C., et al. (2016). Exceptional summer conditions and HABs Pseudochattonella in southern chile create record impacts on salmon farms. Harm. Algal News 53, 1–3.
Cruz-Rico, J., and Rivas, D. (2018). Physical and biogeochemical variability in Todos Santos Bay, northwestern Baja California, derived from a numerical NPZD model. J. Mar. Sys. 183, 63–75. doi: 10.1016/j.jmarsys.2018.04.001
Dorantes-Aranda, J. J., Nichols, P. D., Waite, T. D., and Hallegraeff, G. M. (2013). Strain variability in fatty acid composition of Chattonella marina (Raphidophyceae) and its relation to differing ichthyotoxicity toward rainbow trout gill cells. J. Phycol. 49, 427–438. doi: 10.1111/jpy.12053
Dorantes-Aranda, J. J., Seger, A., Mardones, J. I., Nichols, P. D., and Hallegraeff, G. M. (2015). Progress in understanding algal Bloom-mediated fish killing: the role of superoxide radicals, phycotoxins and fatty acid. PLoS ONE 10:e133549. doi: 10.1371/journal.pone.0133549
Durazo, R. (2009). Climate and upper ocean variability off Baja California, Mexico: 1997-2008. Prog. Ocean. 83, 361–368. doi: 10.1016/j.pocean.2009.07.043
Edvardsen, B., and Imai, I. (2006). “The ecology of harmful flagellates within Prymnesiophyceae and Raphidophyceae,” in Ecology of Harmful Algae, eds E. Granéli and J. T. Turner (Berlin, Heidelberg: Springer), 67–79.
Esenkulova, S., Haigh, N., Keddy, L. J., and Pearce, C. M. (2014). Germinating cysts of Heterosigma akashiwo from marine sediments of British Columbia, Canada. Harm. Algae News 48, 4–5.
Fernandez, M. N., and Mazon, A. F. (2003). “Environmental pollution and fish gill morphology,” in Fish Adaptations, eds A. L. Val and B. G. Kapoor (Enfield, CT: Science Publishers), 203–231.
Fitzgibbon, Q. P., Seymour, R. S., Buchanan, J., Musgrove, R., and Carragher, J. (2010). Effects of hypoxia on oxygen consumption, swimming velocity and gut evacuation in southern Bluefin tuna (Thunnus maccoyii). Environ. Biol. Fish 89, 59–69. doi: 10.1007/s10641-010-9690-1
Godoy, M. (2016). Patología en Acuicultura, 2016 Histopatología Branquial Asociada a la Exposición a Bloom por Chattonella sp. (Raphidophyceae) en Salmón del Atlántico (Salmo salar). Available online at: http://goo.gl/e0SdFI
Hallegraeff, G. M., Dorantes-Aranda, J. J., Mardones, J., and Seger, A. (2017). “Review of Progress in our understanding of fish-killing microalgae: implications for management and mitigation,” in Marine and Fresh-Waters Harmful Algae. Procedings of the 17th International Conference on Harmful Algae 2017, eds L. A. O. Proença and G. M. Hallegraeff, (International Society for the Study of Harmful Algae).
Huang, B., Xue, Y., and Behringer, D. W. (2008). Impacts of argo salinity in NCEP global ocean data assimilation system: the tropical Indian Ocean. J. Geophys. Res. 113:C08002. doi: 10.1029/2007JC004388
Imai, I. (1990). Distribution of diatom resting cells in sediments of Harima-Nada and northern Hiroshima Bay, the Seto Inland Sea, Japan. Bull. Coast. Oceanogr. 28, 75–84.
Imai, I., Itakura, S., and Itho, K. (1991). Life cycle strategies of the Red Tide causing flagellates Chattonella (Raphidophyceae) in the Seto Inland Sea. Mar. Poll. Bull. 23, 165–170. doi: 10.1016/0025-326X(91)90668-I
Imai, I., and Itoh, K. (1987). Annual life cycle of Chattonella spp., causative flagellates of noxious red tides in the Inland Sea of Japan. Mar. Biol. 94, 287–292. doi: 10.1007/BF00392942
Imai, I., and Yamaguchi, M. (2012). Life cycle, physiology, ecology and red tide occurrences of the fish-killing raphidophyte Chattonella. Harm. Algae 14, 46–70. doi: 10.1016/j.hal.2011.10.014
Imai, I., Yamaguchi, M., and Hori, Y. (2006). Eutrophication and occurrences of harmful algal blooms in the Seto Inland Sea, Japan. Plankt. Benthos. Res. 1, 71–84. doi: 10.3800/pbr.1.71
Ishimatsu, A., Sameshima, M., Tamura, A., and Oda, T. (1996). Histological analysis of the mechanisms of chattonella-induced hypoxemia in yellowtail. Fish. Sci. 62, 50–58. doi: 10.2331/fishsci.62.50
Katano, T., Yoshida, M., Lee, J., Han, M.-S., and Hayami, Y. (2009). Fixation of Chattonella antiqua and C. marina (Raphidophyceae) using Hepes-buffered paraformaldehyde and glutaraldehyde for flow cytometry and light microscopy. Phycologia 43, 473–479. doi: 10.2216/08-102.1
Khan, S., Arakawa, O., and Onoue, Y. (1996). A toxicological study of the marine phytoflagellate,Chattonella antiqua (Raphidophyceae). Phycologia 35, 239–244. doi: 10.2216/i0031-8884-35-3-239.1
Kim, S. Y., Seo, K. S., Lee, C. G., and Lee, Y. (2007). Diurnal modification of a red-tide causing organism Chattonella antiqua (Raphidophyceae) from Korea. Algae 22, 95–106. doi: 10.4490/ALGAE.2007.22.2.095
Korsmeyer, K. E., and Dewar, H. (2001). Tuna metabolism and energetics. Fish Physiol. 19, 35–78. doi: 10.1016/S1546-5098(01)19003-5
Kudela, R. M., and Gobler, C. J. (2012). Harmful dinoflagellates blooms caused by Cochlodinium sp.: global expansion and ecological strategies facilitating bloom formation. Harm. Algae 14, 71–86. doi: 10.1016/j.hal.2011.10.015
León-Muñoz, J., Urbina, M. A., Garreaud, R., and Iriarte, J. L. (2018). Hydroclimatic conditions trigger record harmful algal Bloom in western Patagonia (summer 2016). Sci. Rep. 8:1330. doi: 10.1038/s41598-018-19461-4
Lewitus, A. J., Horner, R. A., Caron, D. A., García-Mendoza, E., Hickey, B. M., Hunter, M., et al. (2012). Harmful algal blooms along the North American west coast region: history, trends, causes and impacts. Harm. Algae 19, 133–159. doi: 10.1016/j.hal.2012.06.009
Mallatt, J. (1985). Fish gill structural changes induced by toxicants and other irritants: a statistical review. Can. J. Fish Aquatic Sci. 42, 630–648. doi: 10.1139/f85-083
Marshall, J. A., and Hallegraeff, G. M. (1999). Comparative ecophysiology of the harmful alga Chattonella marina (Raphidophyceae) from South Australian and Japanese water. J. Plankton Res. 21, 1809–1822. doi: 10.1093/plankt/21.10.1809
Marshall, J. A., and Newman, S. (2002). Differences in photoprotective pigments production between Japanese and Australian strains of Chattonella marina (Raphidophyceae). J. Exp. Mar. Biol. Ecol. 272, 13–27. doi: 10.1016/S0022-0981(02)00034-5
Marshall, J. A., Nichols, P. D., Hamilton, B., Lewis, R. J., and Hallegraeff, G. M. (2003). Ichthyotoxicity of Chattonella marina (Raphidophyceae) to damselfish (Acanthochromis polycanthus): the synergistic role of reactive oxygen species and free fatty acids. Harmful Algae 2, 273–281. doi: 10.1016/S1568-9883(03)00046-5
McClatchie, S., Goericke, R., and Leising, A. W. (2016). State of the California Current 2015-16: Comparisons with the 1997-98 El Niño. California Cooperative Oceanic Fisheries Investigations Report, 57, 5–61.
Mesinger, F., DiMego, G., Kalnay, E., Mitchell, K., Shafran, P. C., Ebisuzaki, W., et al. (2006). North American regional reanalysis. Bull. Am. Meteor. Soc. 87, 343–360. doi: 10.1175/BAMS-87-3-343
Munday, B. L., and Hallegraeff, G. M. (1998). Mass mortality of captive southern bluefin tuna (Thunnus maccoyii) in April/May 1996 in Boston Bay, South Australia: a complex diagnostic problem. Fish Pathol. 33, 343–350. doi: 10.3147/jsfp.33.343
Omura, T., Iwataki, M., Borja, V. M., Takayama, H., and Fukuyo, Y. (2012). Marine Phytoplankton of the Western Pacific. Tokyo: Kouseisha Kouseikaku.
Onitsuka, G., Aoki, K., Shimizu, M., Matsuyama, Y., Kimoto, K., Matsuo, H., et al. (2011). Short-term dynamics of a Chattonella antiqua bloom in the Yatsushiro Sea, apan, in summer 2010: characteristics of its appearance in the southern area. Bull. Jpn. Soc. Fish Oceanogr. 75, 143–153.
Powell, T. M., Lewis, C. V. W., Curchister, E. N., Haidvogel, D. B., Hermann, A. J., and Dobbins, E. L. (2006). Results from a three-dimensional, nested biological-physical model of the California current system and comparisons with statistics from satellite imagery. J. Geophys. Res. 111:C07018. doi: 10.1029/2004JC002506
Ravichandran, M., Behringer, D., Sivareddy, S., Girishkumar, M. S., Chacko, N., and y Harikumar, R. (2013). Evaluation of the global ocean data assimilation system at INCOIS: the tropical Indian Ocean. Ocean Modell. 69, 123–135. doi: 10.1016/j.ocemod.2013.05.003
Rensel, J. E., and Whyte, J. N. C. (2004). “Finfish mariculture and harmful algal blooms,” in Manual on Harmful Marine Microalgae, eds G. M. Hallegraeff, D. M. Anderson, and A. D. Cembella (Landais: UNESCO Publishing), 693.
Schwing, F. B., Farrell, M. O., and Steger, J. M. (1996). Coastal Upwelling Indices West Coast of North America. NOAA Tech. Rep. NMFS SWFSC 231. Seattle, WA: NOAA.
Shen, M., Xu, J., Chan, A. K. Y., and Au, D.W.T. (2011). Susceptibility of fish to Chattonella marina is determined by its tolerance to hypoxia. Mar. Pollut. Bull. 63, 189–194. doi: 10.1016/j.marpolbul.2011.06.001
Smith, S. D. (1988). Coefficients for sea surface wind stress, heat flux, and wind profiles as a function of wind speed and temperature. J. Geophys. Res. 93, 15467–15472. doi: 10.1029/JC093iC12p15467
Tiffany, M. A., Barlow, S. B., Matey, V. E., and Hulbert, S. H. (2001). Chattonella marina (Raphidophyceae), a pontentially toxic alga in the Salton Sea, California. Hydrobiologia 466, 187–194. doi: 10.1023/A:1014503920898
Keywords: ichthyotoxic species, Chattonella marina, environmental noxa, gill damage, El Niño
Citation: García-Mendoza E, Cáceres-Martínez J, Rivas D, Fimbres-Martinez M, Sánchez-Bravo Y, Vásquez-Yeomans R and Medina-Elizalde J (2018) Mass Mortality of Cultivated Northern Bluefin Tuna Thunnus thynnus orientalis Associated With Chattonella Species in Baja California, Mexico. Front. Mar. Sci. 5:454. doi: 10.3389/fmars.2018.00454
Received: 05 August 2018; Accepted: 13 November 2018;
Published: 04 December 2018.
Edited by:
Marius Nils Müller, Federal University of Pernambuco, BrazilReviewed by:
Raphael M. Kudela, University of California, Santa Cruz, United StatesChui Pin Leaw, University of Malaya, Malaysia
Kazuhiro Aoki, Japan Fisheries Research and Education Agency (FRA), Japan
Copyright © 2018 García-Mendoza, Cáceres-Martínez, Rivas, Fimbres-Martinez, Sánchez-Bravo, Vásquez-Yeomans and Medina-Elizalde. This is an open-access article distributed under the terms of the Creative Commons Attribution License (CC BY). The use, distribution or reproduction in other forums is permitted, provided the original author(s) and the copyright owner(s) are credited and that the original publication in this journal is cited, in accordance with accepted academic practice. No use, distribution or reproduction is permitted which does not comply with these terms.
*Correspondence: Ernesto García-Mendoza, ZXJnYXJjaWFAY2ljZXNlLm14