- Virginia Institute of Marine Science, College of William and Mary, Gloucester Point, VA, United States
Shoots in seagrass beds form canopies: structurally complex habitats that provide refuge for fauna and trap sediment particles by dampening water movement. Unfortunately, seagrasses are faced with continuing negative impacts to survival, including climate change and poor water quality. In areas where several seagrass species coexist, changing conditions may influence composition of beds so one species is favored over another. Two species found worldwide, Zostera marina and Ruppia maritima, are undergoing this shift: as Z. marina dies back, in some locations it is replaced by R. maritima, a smaller-form seagrass with shorter, thinner shoots. This process is occurring in Virginia, United States in the southern Chesapeake Bay, at intermediate depths where the species co-occur. Although changes in seagrass species abundance have previously been documented, few studies have measured the resulting effects on ecosystem functioning. We evaluated three sites to determine whether canopies of the two species displayed similar small epifaunal invertebrate animal assemblages and sediment properties, and found that Z. marina beds exhibited a greater amount of fine surface sediment than those of R. maritima, but found no effect of seagrass species on invertebrate assemblages. Epifaunal invertebrates were, however, more abundant and speciose with greater biomass, and more abundant with greater shoot density. This study provides baseline information from one summer for areas where the two seagrass species coexist. Although more research is needed, this study suggests in mixed beds, decline of Z. marina could result in coarsening of sediment, but dense R. maritima canopies could harbor similar small invertebrate assemblages.
Introduction
Seagrass canopies provide habitat for marine life, including small crustacean and gastropod invertebrates that are part of the diet of nearshore fishes (Orth et al., 1984; Valentine and Duffy, 2006). Seagrasses also attenuate waves and reduce currents, causing fine particles to be deposited and retained within beds, creating a positive feedback loop in which water clarity is improved for seagrass growth (Ward et al., 1984; Hansen and Reidenbach, 2013). Seagrasses are currently in decline due to decreased water quality and climate change (Cardoso et al., 2004; Orth et al., 2006), and beds in many areas could shift to being dominated by macroalgae or other seagrass species better able to cope with changing or degraded conditions (Armitage et al., 2011; van Tussenbroek et al., 2014). When a shift occurs between morphologically similar species, ecosystem functioning may not change (Christiaen et al., 2016), however, many co-occurring seagrasses have differing morphologies and life cycles. Several regions in theUnited States have experienced seagrass habitats transitioning from larger-form, spatially stable species to more opportunistic, smaller-form species (Fourqurean et al., 1995; Johnson et al., 2003; Bologna et al., 2007; Cho et al., 2009; Lopez-Calderon et al., 2010; Micheli et al., 2014).
In Chesapeake Bay, two seagrasses co-occur: Zostera marina and Ruppia maritima. Z. marina has taller canopies, wider strap-like leaves and spatially stable populations (Orth and Moore, 1988; Moore et al., 2014); comparatively, R. maritima has shorter canopies, smaller leaf areas, and unpredictable annual abundances (Kantrud, 1991; Orth et al., 2016). These species coexist in other regions of the mid-Atlantic United States, and in California and Maine (Orth and Moore, 1988; Kantrud, 1991; Johnson et al., 2003). Z. marina in Chesapeake Bay is in decline and its recovery problematic (Moore et al., 2012; Lefcheck et al., 2017).
Seagrass canopy structure may play a role in epifaunal community composition and sediment deposition (Orth et al., 1984; Fonseca and Callahan, 1992), but these effects are incompletely understood. In this study we compared surface sediment characteristics and small invertebrate assemblages in canopies of each seagrass to determine whether a shift from Z. marina to R. maritima could result in changes in seagrass bed features. We hypothesized that Z. marina would have greater canopy biomass than R. maritima over the summer sampling took place, and that Z. marina areas would contain more fine sediment and organic matter and provide refuge for more abundant and speciose invertebrates.
Materials and Methods
Study Locations
Three sites in the lower Chesapeake Bay (Supplementary Figure S1) with monotypic stands of Ruppia maritima and Zostera marina nearby to one another (50–300 m) in similar water depths (50 ± 10 cm MLLW) were sampled. The sites were physically diverse: one is located within an embayment with surrounding salt marsh, while the others are more exposed to prevailing winds and tidal currents, although all possess similar salinity and turbidity measurements1. Sites were within areas where an increase in R. maritima and decrease in Z. marina populations has occurred in recent years (Moore et al., 2014; Richardson et al., 2018).
Sample Collection Overview
Seagrass, sediment and invertebrate animal samples were taken in each of the habitat types (monospecific stands of Z. marina or R. maritima) during 2 months: June and August of 2013. Sampling timing was adapted from the NERRS (National Estuarine Research Reserve System) Vegetation Monitoring Protocols, which specify sampling within 2–3 weeks of peak biomass for seagrasses present (Moore, 2013). Here Z. marina increases in density during the late spring and senesces though mid-summer and into fall (Orth and Moore, 1986; Moore and Jarvis, 2008) and R. maritima has a similar trajectory, although it tends to peak in the fall (Moore et al., 2000). Replicate sampling areas were haphazardly chosen by throwing a meter-square quadrat within 10 m of a pole marking habitat type, samples were taken within the quadrat. HOBO temperature loggers (Onset Computer Corporation, Bourne, MA, United States) were anchored between the two habitat types at each site, where they recorded temperature every 15 min between 1 June and 26 August 2013.
Seagrass Biomass Sampling and Processing
Seagrass samples were taken with a 12 cm diameter acrylic core, sieved in the field to remove sediment, and transported back on ice to the laboratory where aboveground material was separated from roots and dried at 65°C until reaching a constant weight.
Sediment Sampling and Processing
Samples for grain size and sediment organic matter (SOM) were taken using a 7 cm diameter acrylic core, and transported back to the lab on ice, where the top (0–2 cm) layer of sediment was processed by homogenizing samples, separating sand from the sample, and using a pipetting method to determine fine (silt and clay) fractions (modification of Plumb, 1981). Silt and clay fractions were combined for a measurement of fine sediment, while the remaining sand represented coarse sediment. SOM was determined via the loss-on-ignition method (Erftemeijer and Koch, 2001) by drying samples at 65°C oven, then combusting at 500°C for 5 h.
Epifaunal Sampling and Processing
Samples were collected using a Virnstein grab (Virnstein and Howard, 1987), which closes over a 400 cm2 area of sediment surface, collecting seagrass shoots, any overlying macroalgae, and epifauna without collecting sediment. During processing, epifauna were separated from seagrass shoots and macroalgae, shoots were counted, animals were sieved using 0.5 mm mesh, and all invertebrates were identified to species or genus levels. Seagrass and macroalgae was dried at 65°C until reaching a constant weight.
Data Analyses
Seagrass core samples were used to compare mean biomass between Z. marina and R. maritima habitats and mean biomass between the 2 months sampled. Separate mixed models were used to test for differences in fine sediment content, sediment organic matter content, invertebrate richness, and invertebrate abundance between Z. marina and R. maritima habitats and between the 2 months sampled. The habitat and month during which a sample was taken were used as fixed factors, and site where a sample was taken was used as a random factor in the models. Richness and abundance of epifauna were normalized to biomass from the grab samples from which they came: both to seagrass biomass and total biomass including macroalgae. A one-way ANOVA and Tukey’s HSD test were used to determine the differences between daily means of temperature at the three sites. All data were inspected for normality and homogeneity of variance and transformed if assumptions were not met. Pearson’s correlation was used to test relationships between seagrass biomass, total biomass including macroalgae, or shoot density within grab samples and the richness or abundance of invertebrates (6 comparisons). All analyses were considered significant at the p < 0.05 level and were performed in the R programming language (R Core Team, 2015).
Results
Seagrass Biomass and Site Conditions
Water temperatures were higher in August than June, and mean temperatures were higher overall at the embayment site over the summer (p < 0.001 and p < 0.001, Supplementary Figure S2). Across sites, Z. marina had greater biomass than R. maritima during the months sampled (Supplementary Table S1), although at two sites in August, R. maritima biomass was greater. The greatest dieback of both R. maritima and Z. marina occurred at the embayment site, where seagrass biomass fell 90% between the beginning and end of the growing season.
Sediment Characterization
Zostera marina areas contained more fine sediment (silt and clay) than R. maritima areas (p = 0.027, Figure 1): Z. marina had an average of 86.8% sand and 13.2% fine sediment, compared to R. maritima with 89.4% sand and 10.6% fine sediment. The embayment site lost fine sediment from June to August, contrary to the other two sites, where the percentage of fine sediment increased. Mean organic content was greater, although not significantly so, in Z. marina sediments than R. maritima sediments in June, and means were similar in August (Figure 1). Mean organic content of sediments was greater in August than in June.
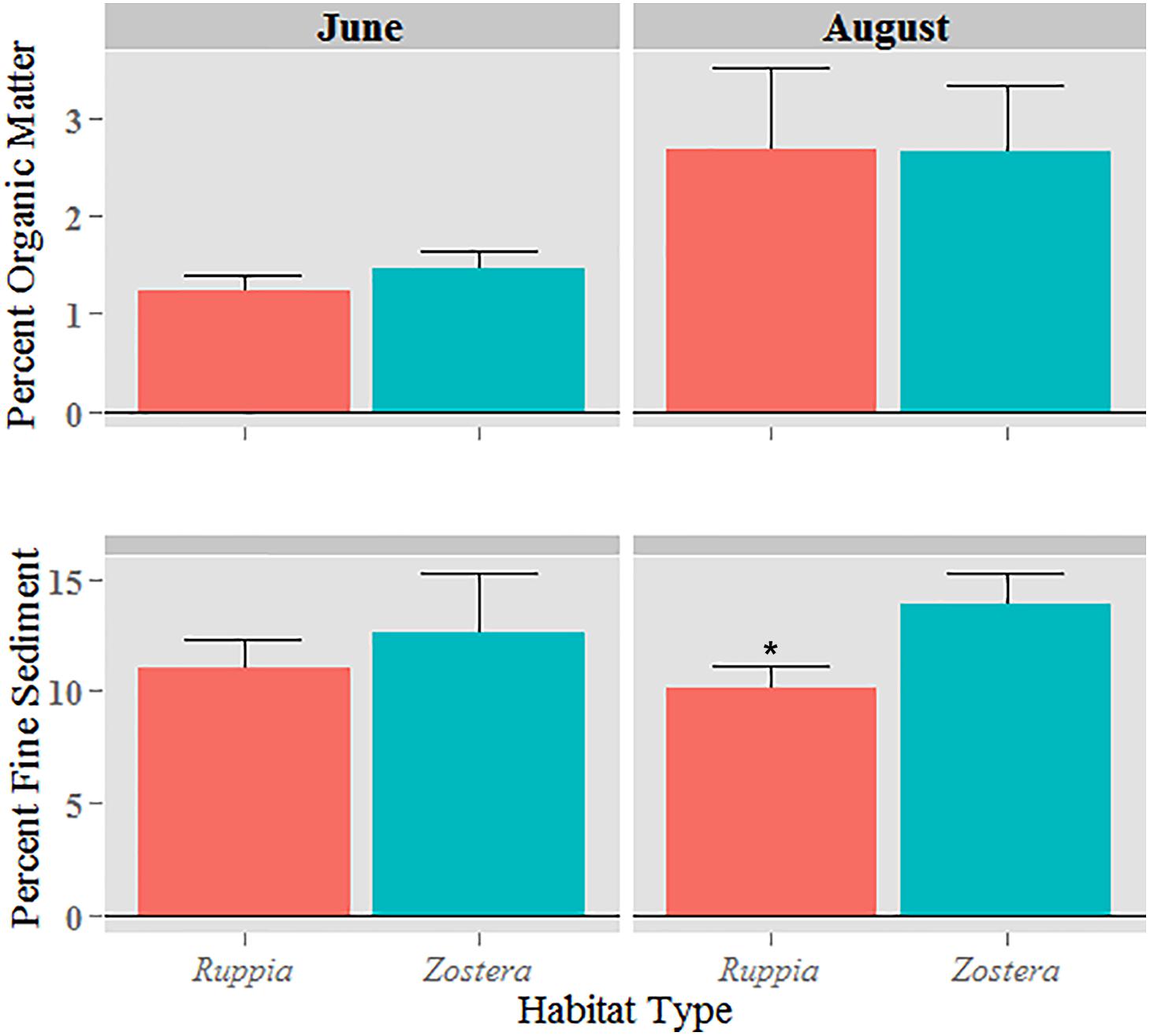
FIGURE 1. Mean fine content of sediment and organic matter ± standard error at each habitat during the sampling periods. Asterisk indicates mean values are significantly different.
Invertebrate Abundance and Diversity
Thirteen species of small invertebrates (<3 cm) were found. There was no effect of seagrass species on abundance of invertebrates. There was a significant effect of month (p = 0.001); between June and August, mean abundance decreased from 165.6 to 28 individuals per sample. There was no effect of seagrass species on richness of invertebrates, though there was a significant effect of month (p = 0.001); a mean richness of 5.5 species per sample in June fell to 3 in August (Supplementary Table S2).
Greater seagrass biomass was strongly associated with both greater richness and abundance of invertebrates (Table 1). Several of the grabs included the macroalgal species Gracilaria vermiculophylla, where present, it was added to seagrass biomass for total biomass present, which resulted in stronger relationships. Higher invertebrate abundances were associated with higher shoot densities (Table 1), but the relationship was not significant between shoot density and invertebrate richness.
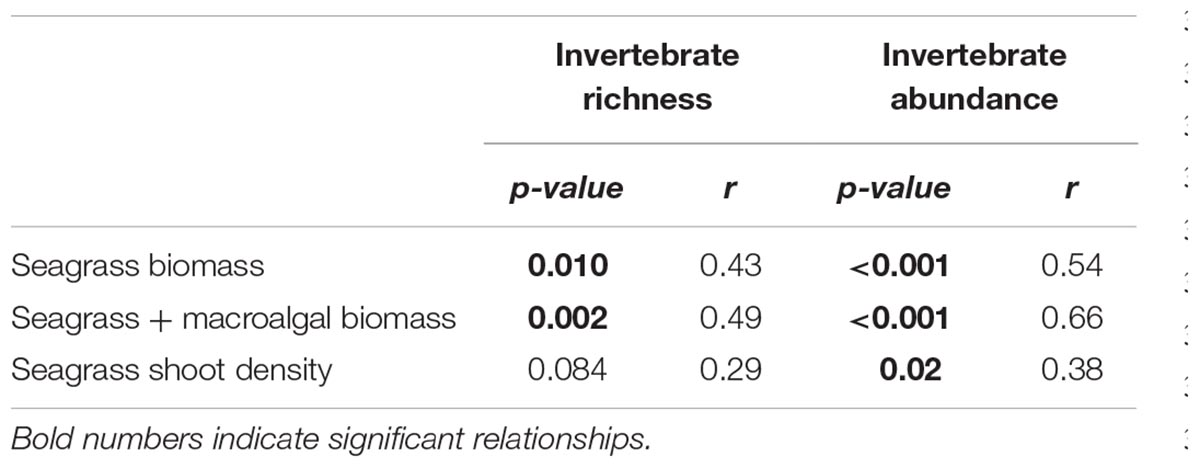
TABLE 1. Pearson’s correlation matrix of relationships between invertebrate abundance and richness with biomass and shoot density.
Discussion
This study demonstrated that mean biomass of Z. marina across sites was greater than the biomass of R. maritima, as we hypothesized, during both June and August of the summer we sampled when Z. marina typically dies back. Biomass decline of Z. marina is typical between June and August, however, the decline demonstrated in R. maritima between June and August is somewhat uncharacteristic (Wetzel and Penhale, 1983; Orth and Moore, 1988; Kantrud, 1991; Moore et al., 2014). It is possible that R. maritima only declined in the areas sampled in this study, intermediate depths where the seagrasses cooccur, and not the shallow depths at which R. maritima is typically more abundant (Kantrud, 1991). When examining site data individually, the embayment site stands out: it lost the most biomass of the three sites between June and August, and had the highest temperatures over the summer, making it likely that high temperatures caused seagrass dieback.
Greater fine sediment content in Z. marina habitats compared to R. maritima suggests that Z. marina may possess a more enhanced capability to trap sediment than R. maritima. Because greater wave attenuation occurs when shoots occupy more of the water column (Fonseca and Callahan, 1992), the taller Z. marina canopy may trap more fine material than R. maritima. Measuring sediment trapping by weighing the accumulation of fine vs. coarse sediments has limitations, thus the use of sediment traps would add valuable information to subsequent studies. However, fine-grained particles are more easily suspended than coarser particles (Ward, 1985); therefore, the increase of fine sediment from June to August in Z. marina beds is likely indicative of either settlement or retainment influenced by plant structure. The ubiquity of organic matter, including seagrass blade senescence and allochthonous sources, in the late summer could have contributed to the similar organic enrichment of sediments seen in August (Oreska et al., 2017).
Lack of differences in richness between seagrass species for small invertebrates (isopods, amphipods, decapods, and gastropods) could be attributable to their low richness in the lower Chesapeake Bay in general, and lack of differences in abundances could be attributable to the relative proximity of the habitat types in this study, or the mobility of invertebrates (Parker et al., 2001; Valentine and Duffy, 2006). Invertebrate richness and abundance were, however, greater in samples with higher shoot density, seagrass biomass, and total biomass including macroalgae. This intuitive relationship has been demonstrated in other studies (Orth et al., 1984 and sources therein; Douglass et al., 2010), and the present study supported this concept. Higher shoot density did not always correspond to higher biomass; most samples showed R. maritima had higher shoot density than Z. marina, while having less biomass. This suggests dense stands of R. maritima could harbor abundant epifauna. Abundance and richness of invertebrates decreased from June to August, this is likely due to the decrease in canopy biomass across all sites and both seagrasses.
This study highlights the need for further research in seagrass habitats transitioning from larger-form, stable species to smaller-form, opportunistic species. Recent research on seagrass populations in Chesapeake Bay show that Z. marina abundance is on a downward trajectory aligning in part with a warming climate (Lefcheck et al., 2017; Richardson et al., 2018), and the potential for long-term persistence of R. maritima populations warrants further research before concluding its canopies may provide ecosystem benefits comparable to that of Z. marina.
Author Contributions
This research was completed when EF was a graduate student at the Virginia Institute of Marine Science, School of Marine Science, College of William and Mary and KM was her graduate advisor and mentor.
Funding
This study received financial support from the Chesapeake Bay National Estuarine Research Reserve and from the Virginia Institute of Marine Science, College of William and Mary (contribution number 3790).
Conflict of Interest Statement
The authors declare that the research was conducted in the absence of any commercial or financial relationships that could be construed as a potential conflict of interest.
Acknowledgments
This manuscript was greatly improved through conversations with Andrew Johnson, Ashley Smyth, and Jonathan Lefcheck. We thank Erin Shields and Tavis Sparrer for assistance during field work and sample processing. We also thank the two reviewers whose comments significantly improved the manuscript.
Supplementary Material
The Supplementary Material for this article can be found online at: https://www.frontiersin.org/articles/10.3389/fmars.2018.00461/full#supplementary-material
FIGURE S1 | The three locations where the study took place in the lower Chesapeake Bay, VA, United States. Mobjack is the “embayment site” referred to in the methods section, and the two fringing marsh wave-exposed sites are Goodwin and Poquoson.
FIGURE S2 | Daily mean water temperatures at the three sites during the sampling events and throughout the summer. Mobjack is the “embayment site” referred to in the methods section, and the two fringing marsh wave-exposed sites are Goodwin and Poquoson.
TABLE S1 | Mean biomass of seagrass canopies ± standard error during the growing season. Mobjack is the “embayment site” referred to in the methods section, and the two fringing marsh wave-exposed sites are Goodwin and Poquoson.
TABLE S2 | Small epifaunal invertebrates (isopods, amphipods, gastropods and decapods) found in grab samples. Values are means (n = 3) ± standard error. G, M, and P correspond to the site names Goodwin, Mobjack and Poquoson. Mobjack is the “embayment site” referred to in the methods section, and the two fringing marsh wave-exposed sites are Goodwin and Poquoson.
Footnotes
References
Armitage, A. R., Frankovich, T. A., and Fourqurean, J. W. (2011). Long-term effects of adding nutrients to an oligotrophic coastal environment. Ecosystems 14, 430–444. doi: 10.1007/s10021-011-9421-2
Bologna, P. A. X., Gibbons-Ohr, S., and Downes-Gastrich, M. (2007). Recovery of eelgrass (Zostera marina) after a major disturbance event in little egg harbor, new jersey, usa. Bull. N. J. Acad. Sci. 52, 1–6.
Cardoso, P. G., Pardal, M. A., Lillebø, A. I., Ferreira, S. M., Raffaelli, D., and Marques, J. C. (2004). Dynamic changes in seagrass assemblages under eutrophication and implications for recovery. J. Exp. Mar. Biol. Ecol. 302, 233–248. doi: 10.1016/j.jembe.2003.10.014
Cho, H. J., Biber, P., and Nica, C. (2009). “The rise of Ruppia in seagrass beds: changes in coastal environment and research needs,” in Handbook on Environmental Quality, eds E. K. Drury and T. S. Pridgen (New York, NY: Nova Science), 1–15.
Christiaen, B., Lehrter, J. C., Goff, J., and Cebrian, J. (2016). Functional implications of changes in seagrass species composition in two shallow coastal lagoons. Mar. Ecol. Prog. Ser. 557, 111–121. doi: 10.3354/meps11847
Douglass, J. G., France, K. E., Richardson, J. P., and Duffy, J. E. (2010). Seasonal and interannual change in a chesapeake bay eelgrass community: insights into biotic and abiotic control of community structure. Limnol. Oceanogr. 55, 1499–1520. doi: 10.4319/lo.2010.55.4.1499
Erftemeijer, P. L. A., and Koch, E. W. (2001). “Chapter 18: Sediment geology methods for seagrass habitat,” in Global Seagrass Research Methods, eds F. T. Short and R. G. Coles (Berlin: Elsevier), 345–367. doi: 10.1016/B978-044450891-1/50019-0
Fonseca, M. S., and Callahan, J. A. (1992). A preliminary evaluation of wave attenuation by four species of seagrass. Estuar. Coast. Shelf Sci. 35, 565–576. doi: 10.1016/S0272-7714(05)80039-3
Fourqurean, J., Powell, G., Kenworthy, W., and Zieman, J. (1995). The effects of long-term manipulation of nutrient supply on competition between the seagrasses Thalassia testudinum and Halodule wrightii in florida bay. Oikos 72, 349–358. doi: 10.2307/3546120
Hansen, J. C. R., and Reidenbach, M. A. (2013). Seasonal growth and senescence of a Zostera marina seagrass meadow alters wave-dominated flow and sediment suspension within a coastal bay. Estuar. Coast. 36, 1099–1114. doi: 10.1007/s12237-013-9620-5
Johnson, M. R., Williams, S. L., Lieberman, C. H., and Solbak, A. (2003). Changes in the abundance of the seagrasses Zostera marina L. (eelgrass) and Ruppia maritima L. (widgeongrass) in san diego, california, following and El niño event. Estuaries 26, 106–115. doi: 10.1007/BF02691698
Kantrud, H. A. (1991). Wigeongrass (Ruppia maritima): a literature review. Fish Wildl. Res. 10, 1–58.
Lefcheck, J. S., Wilcox, D. J., Murphy, R. R., Marion, S. R., and Orth, R. J. (2017). Multiple stressors threaten the imperiled coastal foundation species eelgrass (Zostera marina) in chesapeake bay, usa. Glob. Change Biol. 23, 3474–3483. doi: 10.1111/gcb.13623
Lopez-Calderon, J., Riosmena-Rodríguez, R., Rodríguez-Baron, J. M., Carrión-Cortez, J., Torre, J., Meling-López, A., et al. (2010). Outstanding appearance of Ruppia maritima along baja california sur, méxico and its influence in trophic networks. Mar. Biodivers. 40, 293–300. doi: 10.1007/s12526-010-0050-3
Micheli, F., Bishop, M. J., Peterson, C. H., and Rivera, J. (2014). Alteration of seagrass species composition and function over two decades. Ecol. Monogr. 78, 225–244. doi: 10.1890/06-1605.1
Moore, K. A. (2013). NERRS SWMP vegetation monitoring protocol: long term monitoring of estuarine vegetation communities. Veg. Monitor. Workgroup 10–11.
Moore, K. A., and Jarvis, J. C. (2008). Eelgrass diebacks in the lower chesapeake bay: implications for long-term persistence. J. Coast. Res. 55, 135–147. doi: 10.2112/SI55-014
Moore, K. A., Shields, E. C., and Parrish, D. B. (2014). Impacts of varying temperature and light conditions on Zostera marina (Eelgrass) and its interactions with Ruppia maritima (Widgeongrass). Estuar. Coasts. 37, 20–30. doi: 10.1007/s12237-013-9667-3
Moore, K. A., Shields, E. C., Parrish, D. B., and Orth, R. J. (2012). Eelgrass survival in two contrasting systems: role of turbidity and summer water temperatures. Mar. Ecol. Prog. Ser. 448, 247–258. doi: 10.3354/meps09578
Moore, K. A., Wilcox, D. J., and Orth, R. J. (2000). Analysis of the abundance of submersed aquatic vegetation communities in the chesapeake bay. Estuar. Coast. 23, 115–127. doi: 10.2307/1353229
Oreska, M. P. J., Wilkinson, G. M., McGlathery, K. J., Bost, M., and Mckee, B. A. (2017). Non-seagrass carbon contributions to seagrass sediment blue carbon. Limnol. Oceano 63, S3–S18. doi: 10.1002/lno.10718
Orth, R. J., Carruthers, T. J. B., Dennison, W. C., Duarte, C. M., Fourqurean, J. W., Heck, K. L., et al. (2006). A global crisis for seagrass ecosystems. Bioscience 56, 987–996. doi: 10.1641/0006-3568(2006)56[987:AGCFSE]2.0.CO;2
Orth, R. J., Heck, K. L., and Montfrans, J. (1984). Faunal communities in seagrass beds: a review of the influence of plant structure and prey characteristics on predator-prey relationships. Estuaries 7, 339–350. doi: 10.2307/1351618
Orth, R. J., and Moore, K. A. (1986). Seasonal and year-to-year variations in the growth of Zostera marina L.(eelgrass) in the lower chesapeake bay. Aquat. Bot. 24, 335–341. doi: 10.1016/0304-3770(86)90100-2
Orth, R. J., and Moore, K. A. (1988). Distribution of Zostera marina and Ruppia maritima sensu lato along depth gradients in the lower chesapeake bay, usa. Aquat. Bot. 32, 291–305. doi: 10.1016/0304-3770(88)90122-2
Orth, R. J., Wilcox, D. J., Whiting, J. R., Nagey, L., Kenne, A. K., and Smith, E. R. (2016). 2015 Distribution of Submerged Aquatic Vegetation in Chesapeake Bay and Coastal Bays. Availble at: http://web.vims.edu/bio/sav/sav15/index.html [accessed October 20, 2018]
Parker, J. D., Duffy, J. E., and Orth, R. J. (2001). Plant species diversity and composition: experimental effects on marine epifaunal assemblages. Mar. Ecol. Prog. Ser. 224, 55–67. doi: 10.3354/meps224055
Plumb, R. H. (1981). Procedures for Handling and Chemical Analysis of Sediment and Water Samples. Technical Report EPA/CE-81-1. Great Lakes Laboratory, State University College at Buffalo, Buffalo, NY, for the U.S. Environmental Protection Agency/Corps of Engineers Technical Committee on Criteria for Dredged and Filled Material: Environmental Laboratory, U.S. Army Waterways Experiment Station, Vicksburg, MS. 1–403.
R Core Team (2015). R: A Language and Environment for Statistical Computing. Vienna: R Foundation for Statistical Computing. Available at: http://www.r-project.org/
Richardson, J. P., Lefcheck, J. S., and Orth, R. J. (2018). Warming temperatures alter the relative abundance and distribution of two co-occuring foundaional seagrasses in chesapeake bay, usa. Mar. Ecol. Porg. Ser. 599, 65–74. doi: 10.3354/meps12620
Valentine, J. F., and Duffy, J. E. (2006). “Grazing in seagrass ecosystems,” in Seagrasses: Biology, Ecology and Conservation, eds A. W. D. Larkum, R. J. Orth, and C. M. Duarte (Dordrecht: Springer), 463–501.
van Tussenbroek, B. I., Cortés, J., Collin, R., Fonseca, A. C., Gayle, P. M., Guzmán, H. M., et al. (2014). Caribbean-wide, long-term study of seagrass beds reveals local variations, shifts in community structure and occasional collapse. PLoS One 9:e90600. doi: 10.1371/journal.pone.0090600
Virnstein, R., and Howard, R. (1987). Motile epifauna of marine macrophytes in the indian river lagoon. Bull. Mar. Sci. 41, 1–12.
Ward, L. G. (1985). The influence of wind waves and tidal currents on sediment resuspension in the middle chesapeake bay. Geo Mar. Lett. 5, 71–75. doi: 10.1007/BF02629802
Ward, L. G., Kemp, W. M., and Boynton, W. R. (1984). The influence of waves and seagrass communities on suspended particulates in an estuarine embayment. Mar. Geol. 59, 85–103. doi: 10.1016/0025-3227(84)90089-6
Keywords: seagrass, eelgrass, Chesapeake, Chesapeake Bay, climate change, water quality, epifauna, widgeongrass
Citation: French E and Moore K (2018) Canopy Functions of R. maritima and Z. marina in the Chesapeake Bay. Front. Mar. Sci. 5:461. doi: 10.3389/fmars.2018.00461
Received: 01 September 2018; Accepted: 15 November 2018;
Published: 03 December 2018.
Edited by:
Susana Carvalho, King Abdullah University of Science and Technology, Saudi ArabiaReviewed by:
Juan Moreira Da Rocha, Universidad Autónoma de Madrid, SpainMarina Dolbeth, Centro Interdisciplinar de Investigação Marinha e Ambiental (CIIMAR), Portugal
Copyright © 2018 French and Moore. This is an open-access article distributed under the terms of the Creative Commons Attribution License (CC BY). The use, distribution or reproduction in other forums is permitted, provided the original author(s) and the copyright owner(s) are credited and that the original publication in this journal is cited, in accordance with accepted academic practice. No use, distribution or reproduction is permitted which does not comply with these terms.
*Correspondence: Emily French, ZW1pbHkuZC5mcmVuY2hAZ21haWwuY29t
†Present address: Emily French, Oak Ridge Institute for Science and Education, Environmental Protection Agency, Washington, DC, United States