- 1NIVA Denmark Water Research, Copenhagen, Denmark
- 2Baltic Nest Institute, Baltic Sea Centre, Stockholm University, Stockholm, Sweden
- 3Department of Bioscience, Aarhus University, Roskilde, Denmark
- 4Baltic Nest Institute Denmark, Roskilde, Denmark
- 5Department of Geology, Lund University, Lund, Sweden
- 6Tvärminne Zoological Station, University of Helsinki, Helsinki, Finland
We modelled and assessed the past, present and predicted future eutrophication status of the Baltic Sea. The assessment covers a 350-year period from 1850 to 2200 and is based on: (1) modelled concentrations of dissolved inorganic nitrogen (DIN), dissolved inorganic phosphorous (DIP), chlorophyll-a, Secchi depth, and oxygen under four different of nutrient input scenarios and (2) the application of a multi-metric indicator-based tool for assessment of eutrophication status: HEAT 3.0. This tool was previously applied using historical observations to determine eutrophication status from 1901 to 2012. Here we apply HEAT 3.0 using results of a biogeochemical model to reveal significant changes in eutrophication status from 1850 to 2200. Under two scenarios where Baltic Sea Action Plan (BSAP) nutrient reduction targets are met, we expect future good status will be achieved in most Baltic Sea basins. Under two scenarios where nutrient loads remain at 1997–2003 levels or increase, good status will not be achieved. The change from a healthy state without eutrophication problems in the open waters took place in the late 1950s and early 1960s. Following introduction of the first nutrient abatement measures, recovery began in some basins in the late 1990s, whilst in others it commenced in the beginning of the 21st century. Based on model results, we expect that the first basin to achieve a status without eutrophication will be Arkona, between 2030 and 2040. By 2060–2070, a status without eutrophication is anticipated for the Kattegat, Bornholm Basin and Gulf of Finland, followed by the Danish straits around 2090. For the Baltic Proper and Bothnian Sea, a good status with regard to eutrophication is not expected before 2200. Further, we conclude that two basins are not likely to meet the targets agreed upon and to attain a status unaffected by eutrophication, i.e., the Gulf of Riga and Bothnian Bay. These results, especially the prediction that some basins will not achieve a good status, can be used in support of continuous development and implementation of the regional ecosystem-based nutrient management strategy, the HELCOM Baltic Sea Action Plan.
Introduction
The causes, process and effects of nutrient enrichment and eutrophication in the Baltic Sea are well understood and well documented (Larsson et al., 1985; Rönnberg and Bonsdorff, 2004; Vahtera et al., 2007; Andersen et al., 2011; Gustafsson et al., 2012; Fleming-Lehtinen et al., 2015; Savchuk, 2018). There is no commonly agreed definition of eutrophication, but there is a conceptual understanding of what the consequences of nutrient enrichment are (Andersen et al., 2006; HELCOM, 2009). Discharges, losses and inputs of nutrients from upstream catchments, atmosphere, the North Sea and nutrient regeneration from sediment pools lead to elevated concentration of nutrients in seawater. In most parts of the Baltic Sea, the direct consequences of elevated nutrient concentrations are increased primary production and phytoplankton biomass (Richardson and Heilmann, 1995; Wasmund et al., 2008), and in some areas manifested as blue-green algal blooms (Finni et al., 2001). The increased production of organic matter has negative consequences in most parts of the Baltic Sea. The enhanced sedimentation of organic matter has led to significantly reduced oxygen concentrations and hypoxia has become a large-scale problem (Conley et al., 2011; Carstensen et al., 2014). Subsequently, reduced oxygen concentrations have affected not only benthic invertebrates (Villnäs and Norkko, 2011) but also the spawning success rate of cod, a commercially important fish species (MacKenzie et al., 2000; Köster et al., 2001). The turnover of phosphorus in seabed sediments increases with expanding hypoxia which further amplifies primary production and consequently oxygen demand. The so-called vicious circle (Vahtera et al., 2007), is an important indirect effect of eutrophication.
Baltic Sea countries have been working for decades to reduce nutrient inputs and improve eutrophication status, primarily under the umbrella of the Baltic Marine Environment Protection Commission – Helsinki Commission (HELCOM). With the adoption of the Baltic Sea Action Plan (BSAP) in 2007 (HELCOM, 2007; Backer et al., 2010), this work entered a new phase with reductions based on numerical target values and model calculations for basin-wise Maximum Allowable Inputs and Country-wise Allocated Reduction Targets.
With the 2013 update of the BSAP’s eutrophication segment, the Baltic Sea states not only implement the Ecosystem Approach to management of human activities but also set a new standard for the development of an adaptive and evidence-based nutrient management strategy (HELCOM, 2013b). The environmental objectives of the BSAP are to attain, by 2020, a healthy Baltic Sea unaffected by eutrophication, including (1) concentrations of nutrients close to natural levels, (2) clear water, (3) natural levels of algae blooms, (4) natural distribution and occurrence of plants and animals, and (5) natural oxygen levels. These objectives were quantified into numerical targets that were subsequently used to calculate Maximum Allowable Inputs that, if achieved, will lead to reaching the objectives. Achieving these ambitious objectives by 2020 is unrealistic given the long retention time of water (30 years, Stigebrandt and Gustafsson, 2003) and nutrients (9–50 years, Gustafsson et al., 2017) in the Baltic Sea. Indeed, the Maximum Allowable Inputs were quantified with the prerequisite that the targets will be met when the Baltic Sea has adjusted to a new steady state. The mismatch between the policy goal of 2020 and the practical implementation is well known, and even acknowledged in the 2013 HELCOM Ministerial Declaration (HELCOM, 2013b). Nevertheless, it is still highly relevant to determine the most likely time frames, on a regional basis, for reaching a Baltic Sea unaffected by eutrophication.
The objectives of this study are: (1) to use results of a biogeochemical model to classify eutrophication status of nine Baltic Sea basins for the period 1850–2200 and (2) to identify the basins, which are likely to see improvement to a status not affected by eutrophication, and those basins which are not expected to achieve this status.
Materials and Methods
This study represents a meeting of two processes: (1) the regular assessment of eutrophication status in the Baltic Sea region using indicator-based eutrophication assessment tools (i.e., the HEAT tool) and (2) the implementation of the BSAP, particularly the expected future reduction in nutrient inputs from land-based sources and the atmosphere.
Study Area
The Baltic Sea is an inland sea in northern Europe surrounded by Sweden, Finland, Russia, Estonia, Latvia, Lithuania, Poland, Germany and Denmark, covering a surface area of 415,200 km2 (Table 1). The Baltic Sea is usually divided into several basins separated by sills, including a transition zone to the North Sea including the Kattegat and the Danish Straits (Figure 1 and Table 1).
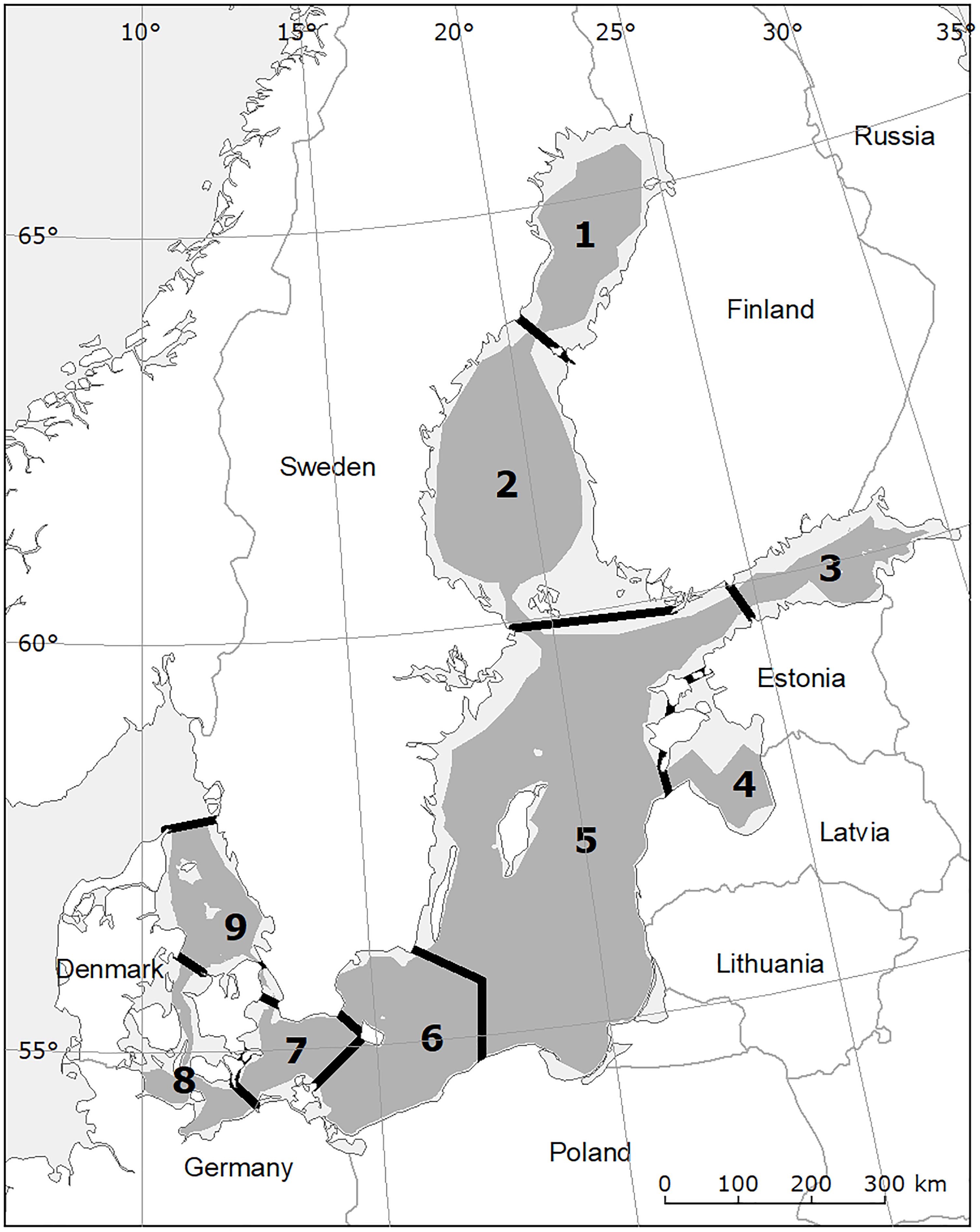
Figure 1. Map of the Baltic Sea. The numbers 1 to 9 identify the basins, as shown in Table 1 below. The darker shading indicates the open water parts of the subdivisions. The BALTSEM model simulates conditions in the open parts, not including coastal waters.
The basins vary substantially regarding ice cover, temperature, salinity, maximum depth and residence times. There is also a wide variation in composition of the benthic biota between basins. More information about Baltic Sea characteristics can be found in Bonsdorff (2006), Johannesson and André (2006), Österblom et al. (2007), and Leppäranta and Myrberg (2009). Nutrient enrichment and eutrophication signals within the study area are very well studied and documented (HELCOM, 2009; Andersen et al., 2011; Carstensen et al., 2014; Fleming-Lehtinen et al., 2015). The root causes, inputs and fluxes of nitrogen and phosphorus are, in general, well understood and documented (Vahtera et al., 2007; HELCOM, 2009).
Actions to improve the ecosystem health of the Baltic Sea, including the currently impaired status regarding eutrophication, are under way as part of the Baltic Sea Action Plan (HELCOM, 2007) and the EU Marine Strategy Framework Directive (Anon, 2008). With the most recent update of the Baltic Sea Action Plan, the countries bordering the Baltic Sea have agreed on a comprehensive ecosystem-based nutrient management strategy (HELCOM, 2013b).
Data Sources
The Baltic sea Long-Term large-Scale Eutrophication Model (BALTSEM: Gustafsson et al., 2012; Savchuk et al., 2012) is a coupled physical-biogeochemical model of the Baltic Sea. It represents the complex topography through 13 basin-specific 1D models of high vertical resolution that are linked horizontally. For the current study, model results from the 13 BALTSEM basins were aggregated into the nine basins used for regional eutrophication status assessment. The version of the BALTSEM model which was used to generate the results used in this study explicity describes the dynamics of nitrogen, phosphorus and silica in separate pools. The model simulates three groups of phytoplankton: diatoms, cyanobacteria and a third group including dinoflagellates and all other phytoplankton. Nutrients are taken up by phytoplankton for growth and are subsequently regenerated by heterotroph organisms in the water column. The model further simulates the transport of nutrients from the water column to bottom sediment in the form of detritus, where the organic nutrient pools are slowly remineralized. Oxygen consumption is coupled to all mineralization processes. BALTSEM has been validated against field data and other models (Eilola et al., 2011; Gustafsson et al., 2012, 2017; Savchuk et al., 2012; Meier et al., 2018a,b). It has been used to simulate the change in ecological indicators (Meier et al., 2012; Neumann et al., 2012) and was applied to calculate the Maximum Allowable Inputs of nutrients to the Baltic Sea in the revision of the Baltic Sea Action Plan (HELCOM, 2013a).
The past eutrophication status of the Baltic Sea in 1850–2006 was simulated by forcing the BALTSEM model with reconstructed nutrient inputs and atmospheric conditions as described in Gustafsson et al. (2012). Its future status was then assessed by extending the model runs for another 194 years under different nutrient scenarios, while hydrodynamics were driven by a statistical representation of the present climate. Nutrient load scenarios included continuation of present nutrient inputs, as well as declining and increasing nutrient inputs. “Present” inputs (PLC5.5) correspond to the loads observed in the BSAP reference period 1997–2003 as described in the review of the 5th HELCOM Pollution Load Compilation (HELCOM, 2013c). Load reduction scenarios simulate nutrient inputs according to the 2013 update of the BSAP’s eutrophication segment, implemented either instantaneously (BSAP0; Figure 2) or with a linear decrease in loads over 30 years (BSAP30). Further, a high nutrient input scenario (BAU30) represents potential increases in nutrient supply associated with future intensified agriculture in the Eastern Baltic States (Meier et al., 2011; Hägg et al., 2014) with a 30-year transition from present inputs (Figure 2). Details about the scenarios and modelled trajectories for the parameters used as indicators can be found as Supplementary Material.
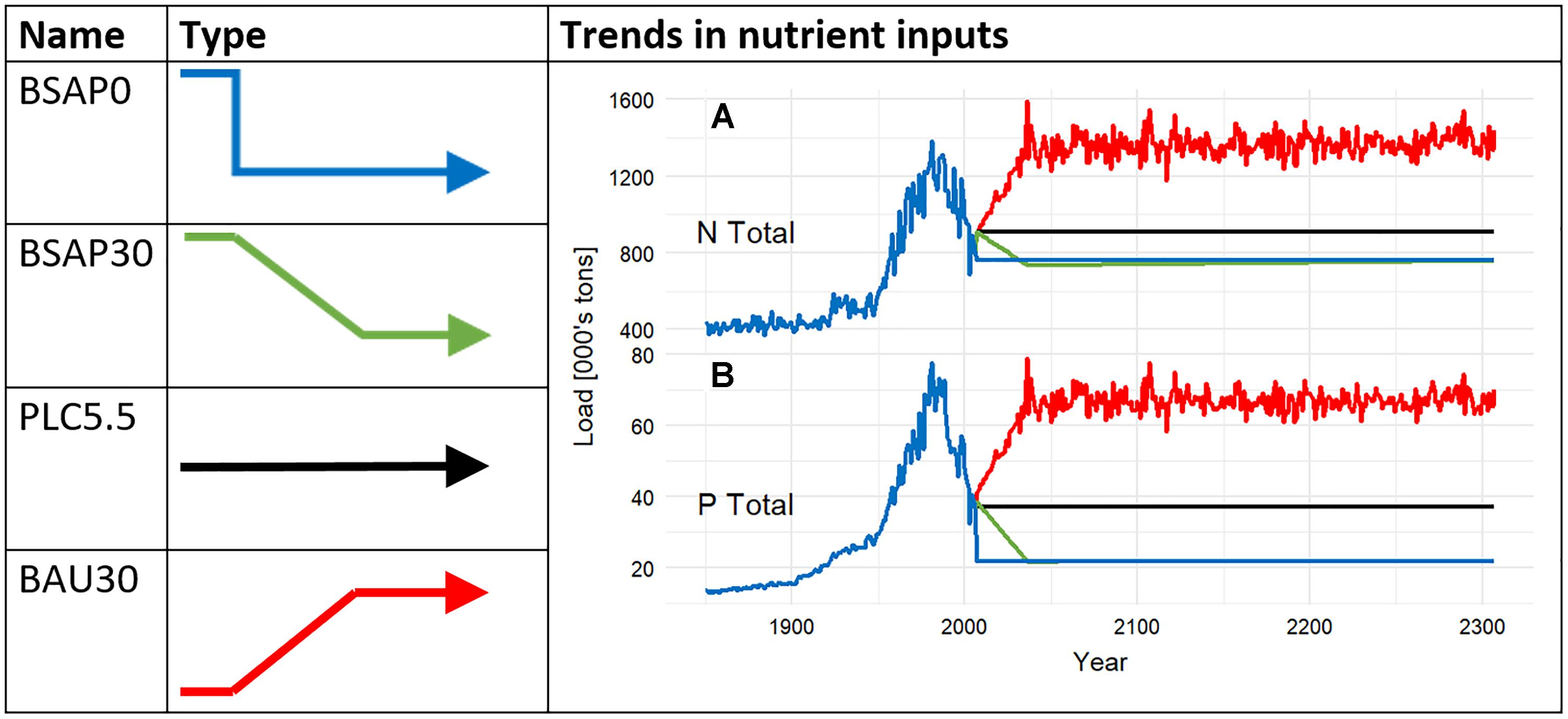
Figure 2. Overview of the nutrient supply scenarios. The four considered nutrient input scenarios with simulated temporal variations based on a variable climate forcing.
HEAT 3.0
In this study, we apply the recent version of the HELCOM Eutrophication Assessment Tool (HEAT 3.0), which has been used for assessing eutrophication in the Baltic Sea for the periods 2007–2011 (Fleming-Lehtinen et al., 2015) and 1901–2012 (Andersen et al., 2017). HEAT 3.0 is a multimetric indicator-based assessment tool which compares the values of several indicator parameters with threshold values, which define the boundary between eutrophic and non-eutrophic status. The ratios of observed and threshold values are averaged within the categories (1) Nutrients, (2) Direct effects, and (3) Indirect effects. The worst (highest) ratio from the three categories determines the overall Eutrophication Ratio (ER). An ER value greater than 1.0 indicates a eutrophic status whilst values less than 1.0 indicate a good status.
For a detailed description of the assessment principles and methods, please confer with the above references including the Supplementary Material to these. Additional information on the development of the tool and earlier versions can be found in Andersen et al. (2010; 2011; 2014) and Fleming-Lehtinen et al. (2015). For convenience, the HEAT3.0 method as described in Andersen et al. (2017) is reproduced in the Supplementary Material to this study.
The target values applied in HEAT 3.0, for the indicators DIN, DIP, chlorophyll-a, Secchi depth, oxygen debt, are taken from Fleming-Lehtinen et al. (2015). An overview of these values, which are also identical to those applied in the study of temporal trends in eutrophication status of the Baltic Sea 1901–2012 (Andersen et al., 2017), is given in Table 2. This also shows the categories for indicator aggregation as described above. Andersen et al. (2017) also included an indicator for benthic invertebrates but since this is not modelled by BALTSEM, this indicator is not used in the model-based HEAT calculations.
Results
Long-term temporal and spatial trends in eutrophication status of the Baltic Sea were obtained by taking data originating from modelling and applying the HEAT tool to these model results. As a first step, we compared the HEAT classifications for the period 1901–2012, which are based on BALTSEM model results with HEAT classifications based on observations for the same period. The rationale was to check the strength of the similarity of the two assessments to assess if model-based HEAT assessments (this study) are comparable with previously published observation-based assessments (from Andersen et al., 2017). Observation and model based HEAT values increased from 0.6 to 0.8 in 1900–1920 to approximately 1.0 in the 1930s. In the 1960s observed HEAT values reached 1.5, modelled 1.3. HEAT values increased even further and reached 2.0 based on observations and about 1.5 for modelled values in the 1990s. The relation between the two assessments show good agreement (Figure 3), therefore we have carried out integrated assessments of eutrophication status based on four different input scenarios all nine regional basins (Figures 4A–I).
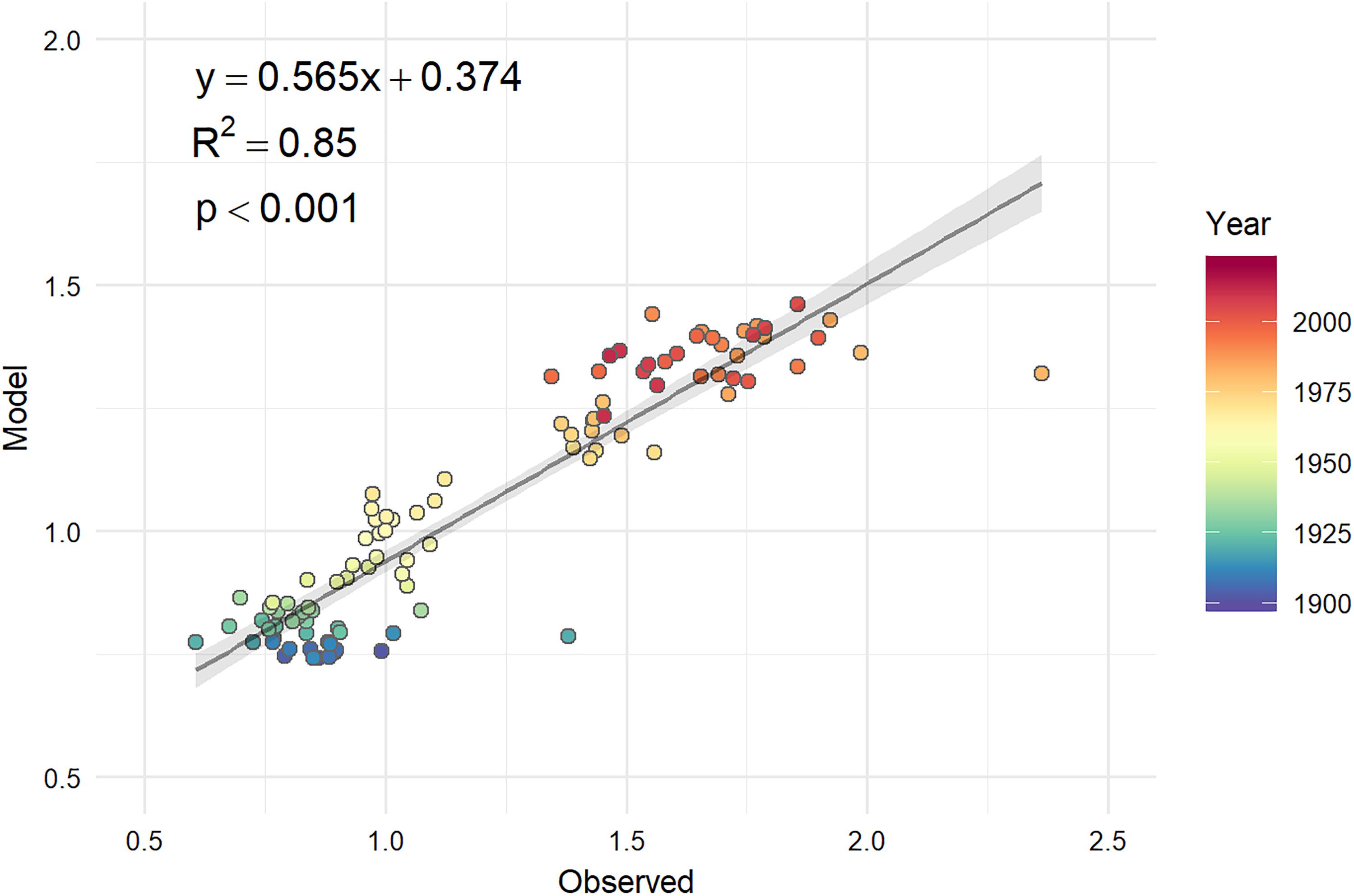
Figure 3. Comparison of model-based and observation-based HEAT classifications. Regression of Baltic average Eutrophication Ratio from BALTSEM model vs. Eutrophication Ratio based on observations for the period 1901–2012. The gray shading indicates the 95% confidence interval.
Increasing loads (BAU30 scenario) lead to a worsening of the eutrophication status in all basins. In some basins, i.e., the Baltic Proper (Figure 4E) and Gulf of Finland (Figure 4G), ER values can potentially reach 2.5, indicating a bad status with significant deviations of indicators from target values. Eutrophication status will improve in the PLC5.5 scenario with maintained nutrient inputs, but the target of a Baltic Sea unaffected by eutrophication will not be reached. In this scenario, the only basin likely to meet the BSAP objectives is the Arkona Basin (Figure 4C), although eutrophication status in the Kattegat will approach the target (Figure 4A).
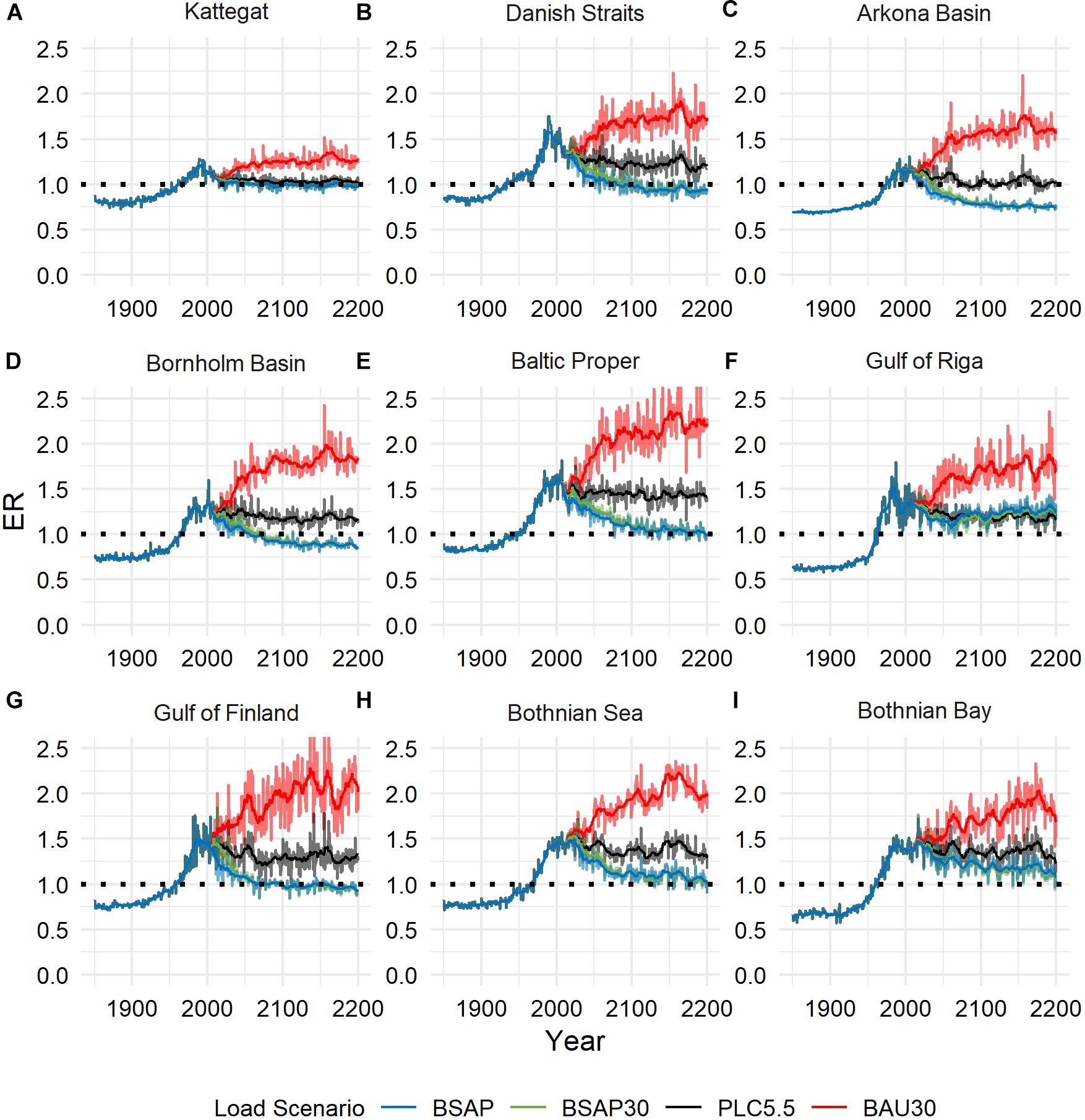
Figure 4. Model-based HEAT assessment results. Eutrophication Ratio (ER) from 1850 to 2200 in the nine Baltic basins (A–I) are shown for four load scenarios. For each scenario the lighter shaded line shows the annual result and the darker line the 10-year moving average result. The dotted line marks ER = 1.0, values below which indicate achievement of good eutrophication status.
The two load reduction scenarios (BSAP0 and BSAP30) may potentially result in oligotrophication sensu Nixon (2009) and thus give a better future eutrophication status in most of the Baltic Sea basins. However, even in these best-case scenarios, some basins are still unlikely to attain a good status according to the HEAT classification with ER values below 1.0, i.e., the Gulf of Riga and Bothnian Bay. BSAP0 and BSAP30 scenarios result in a good status in 7 out of 9 basins, whereas attaining ER = 1.0 in the Gulf of Riga and the Bothnian Sea seems unattainable.
Based on the assessment of the individual basins (Figure 4), we can identify the year when the targets are met for each basin. Since there is some year-to-year variation in ER, we define that the objective of good status in a basin is met when the moving average of ER over a 10-year period falls below 1.0. Using this criterion, the BSAP0 scenario predicts that Arkona Basin is the first to achieve good status, in 2024, followed by the Kattegat and Bornholm Basin in 2057, then the Gulf of Finland and Danish Straits in 2064 and 2080, respectively. Good status is achieved in the Baltic Proper and Bothnian Sea around 2200, just within the time scale of the model simulations.
The BSAP30 scenario describes a similar recovery pathway with basins achieving good status in the same order as for the BSAP0 scenario, however, as might be expected, with somewhat delayed responses. Good status is achieved 9 years later in the Arkona Basin (in 2033) and 8 years later in the Danish Straits (in 2088). The Baltic Proper and the Bothnian Sea just manage to achieve good status before 2200. As described above, Arkona is the only basin expected to return to a good status in the PLC5.5 scenario in 2079, whereas good status will not be achieved for any basin with the BAU30 scenario.
Discussion
The current eutrophication status in the Baltic Sea is far from the objectives agreed upon in the BSAP (HELCOM, 2013b). This is well documented as shown here and in Fleming-Lehtinen (2016) and Andersen et al. (2017). However, earlier trends of increasing eutrophication have been reversed and the Baltic Sea has entered a phase of recovery (Andersen et al., 2017). Examples of oligotrophication and partial recovery have been documented in many coastal waters, e.g., in Denmark (Riemann et al., 2016; Staehr et al., 2017), in Sweden (Walve et al., 2018), in the North Sea (Andersen et al., 2016; OSPAR, 2017; van Beusekom et al., 2018) and in United States (Bricker et al., 2008; Oviatt et al., 2017; Zhang et al., 2018).
Comparison of observation-based HEAT classifications and model-based HEAT classifications for entire Baltic for the period 1902–2012 shows a reasonable relation between the two methods of assessing eutrophication status. A model essentially gives a smoothed representation of the data and cannot describe the micro-variability and measurement errors associated with monitoring data, and henceforth affecting basin average values. Therefore, it is not unexpected that the slope of the regression (0.565) shows that the model-based HEAT results vary less than the observation-based HEAT results. The fact that the HEAT assessment based on observed data also includes a benthic invertebrate quality index could possibly explain some of the differences between model-based and observation-based HEAT results. However, both are in reasonable agreement on where the status changes between eutrophic and good. And since the model-based HEAT results capture 85% of the variation seen in the observation-based HEAT results, we conclude that using the model-based assessments provides an important way forward to assess the potential effects of the BSAP into the future.
Our study predicts how future eutrophication status will improve under different scenarios of nutrient reduction. The time required to achieve good status varies from decades in some basins to centuries in others. The time needed for recovery could be reduced with a faster implementation of nutrient reductions. In most basins, there are substantial delays between implementation of measures leading to reduced loads and ecological responses. Lagged responses of marine ecosystems are well-known (Carstensen et al., 2011) also due to large-scale changes associated with global climate and increasing human stress on coastal ecosystems.
Very few similar studies from other regions assessing the temporal trends in eutrophication status have been published. An example from the North Sea is OSPAR (2017), which applies a harmonized assessment framework for the third time and concludes that the spatial extent of ‘problem areas’ in terms of eutrophication has decreased from approximately 169.000 km (1990–2001) to 119.000 km2 (2001–2005) and to 100.000 km2 for the period 2006–2014. An assessment of the effects of nutrient enrichment in United States estuaries (n = 58) from the early 1990s to the early 2000s (Bricker et al., 2008) concludes that conditions had remained the same over this period in most systems (32), whilst they had worsened in 13 and improved in 13. In the future, conditions were predicted to improve in 19% of the assessed systems. However, in 65% of the estuaries conditions were expected to worsen, due to projected increases in nutrient loads with increasing population density.
There are limited numbers of assessments of eutrophication status in EU Member States and even fewer studies that describe how ecological status improves in eutrophied marine waters. In coastal waters susceptible to high nutrient loads, assessment of ‘ecological status’ according to the EU Water Framework Directive (WFD) can be considered equivalent to a eutrophication status assessment. Accepting this, Member States’ so-called Initial Assessments can provide an indirect indication of whether conditions are improving in coastal waters. Based on a meta-study of national reporting, a recent pan-European assessment (Kristensen et al., 2018) concludes that the overall ecological status of surface waters has not improved. In some coastal waters, the assessed status is even worsening, despite River Basin Management Plans in place to improve water quality.
To attain a Baltic Sea unaffected by eutrophication, reaching the load reduction targets of the ecosystem-based nutrient management strategy (the BSAP’s eutrophication segment, HELCOM, 2007, 2013b) is required. Decision-makers and the wider public should be aware of the current poor situation and should be well informed on the time-scales of Baltic ecosystem recovery. Improving the communication between decision-makers and the scientific society should of course be anchored in scientific studies and literature, but the primary means of communication is not scientific papers and complicated graphs. There is in our opinion a need for simplification, where complex information is synthesized in info-graphics, where messages can be more easily understood. For example, the trend information in multiple graphs in Figure 4 can be summarized as a single graph (Figure 5), where the eutrophication status, and the basin-wise trends are presented using simple colour classes. How the Baltic Sea changed from a system unaffected by eutrophication at the beginning of the 20th century to its present eutrophic state is now expressed in a single graph using an intuitive colour scale. The same graph can further show what we can expect for the future: the likely consequence of the agreed load reductions (BSAP0 and BSAP30 scenarios), once implemented, will cause significant improvements and ultimately a Baltic Sea unaffected by eutrophication in most basins.
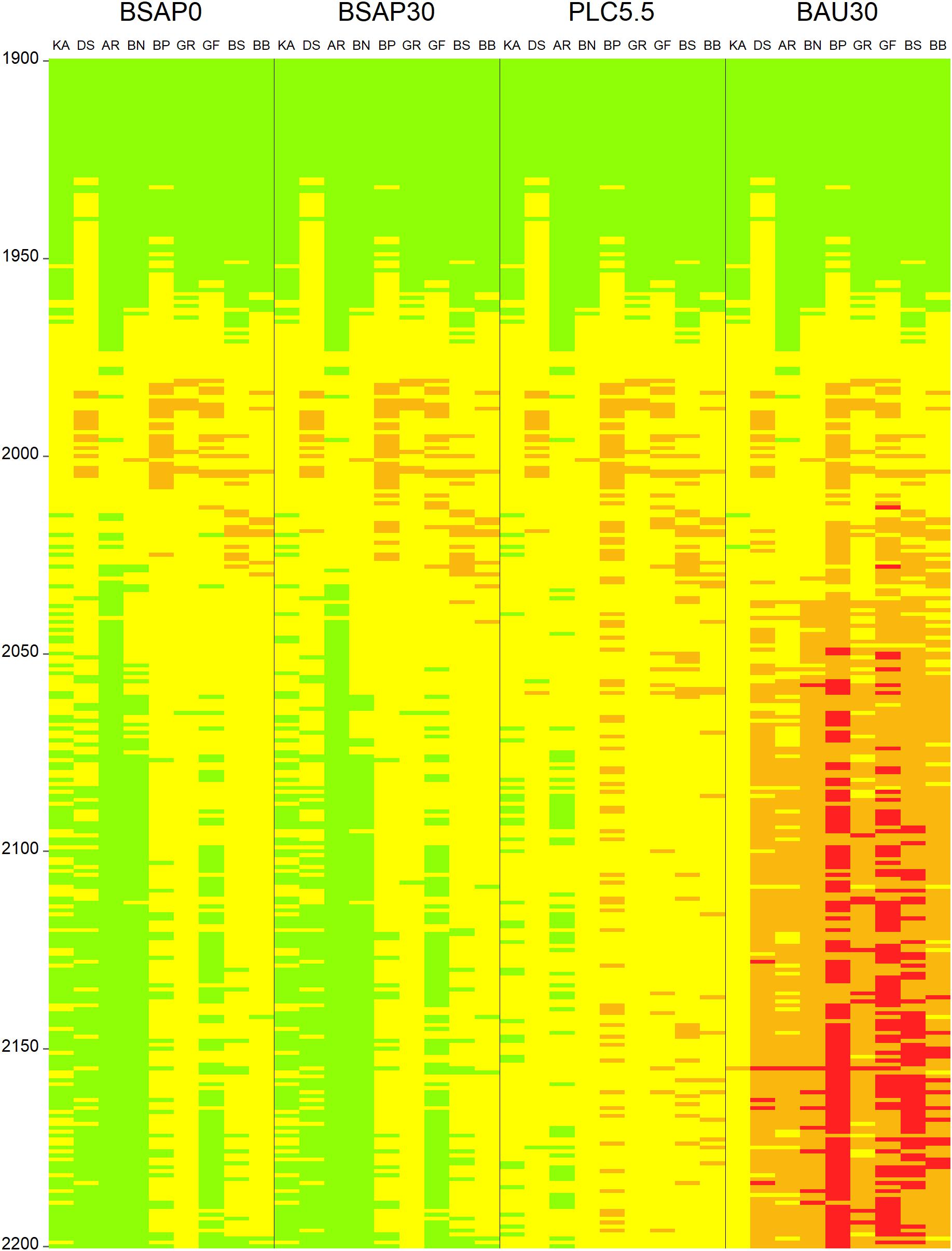
Figure 5. Integrated assessment of eutrophication status the period 1900–2200. The colours green, yellow, orange, red indicate, respectively, “Good” (0.5 ≤ ER < 1.0), “Moderate” (1.0 ≤ ER < 1.5), “Poor” (1.5 ≤ ER < 2.0), or “Bad” (ER > 2.0) eutrophication status. Basin abbreviations are KA, Kattegat; DS, Danish Straits; AR, Arkona Basin; BN, Bornholm Basin; BP, Baltic Proper; GR, Gulf of Riga; GF, Gulf of Finland; BS, Bothnian Sea; BB, Bothnian Bay. The HEAT tool uses a fifth class “High” (ER < 0.5) but none of the BALTSEM assessments returned this result.
Andersen et al. (2017) combined the status classifications from the nine basins into an overall Baltic Sea. In this way, data for several indicators representing different features of the ecosystem are synthesized into a single value. In a similar manner, this study presents the overall Baltic Sea eutrophication status classifications for four future nutrient load scenarios (Figure 6). The results for individual basins (Figure 4) are thus integrated, giving a result which reflect the overall long-term trends in eutrophication status resulting from differences between nutrient inputs scenarios. This integration supports the interpretation of the classification presented in Figure 5 and reveal, not surprisingly, that PLC5.5 and BAU30 scenarios do not lead to a Baltic Sea unaffected by eutrophication, whilst BSAP0 and BSAP30 scenarios will both, after a considerable number of years, meet the overall objective of a healthy Baltic Sea.
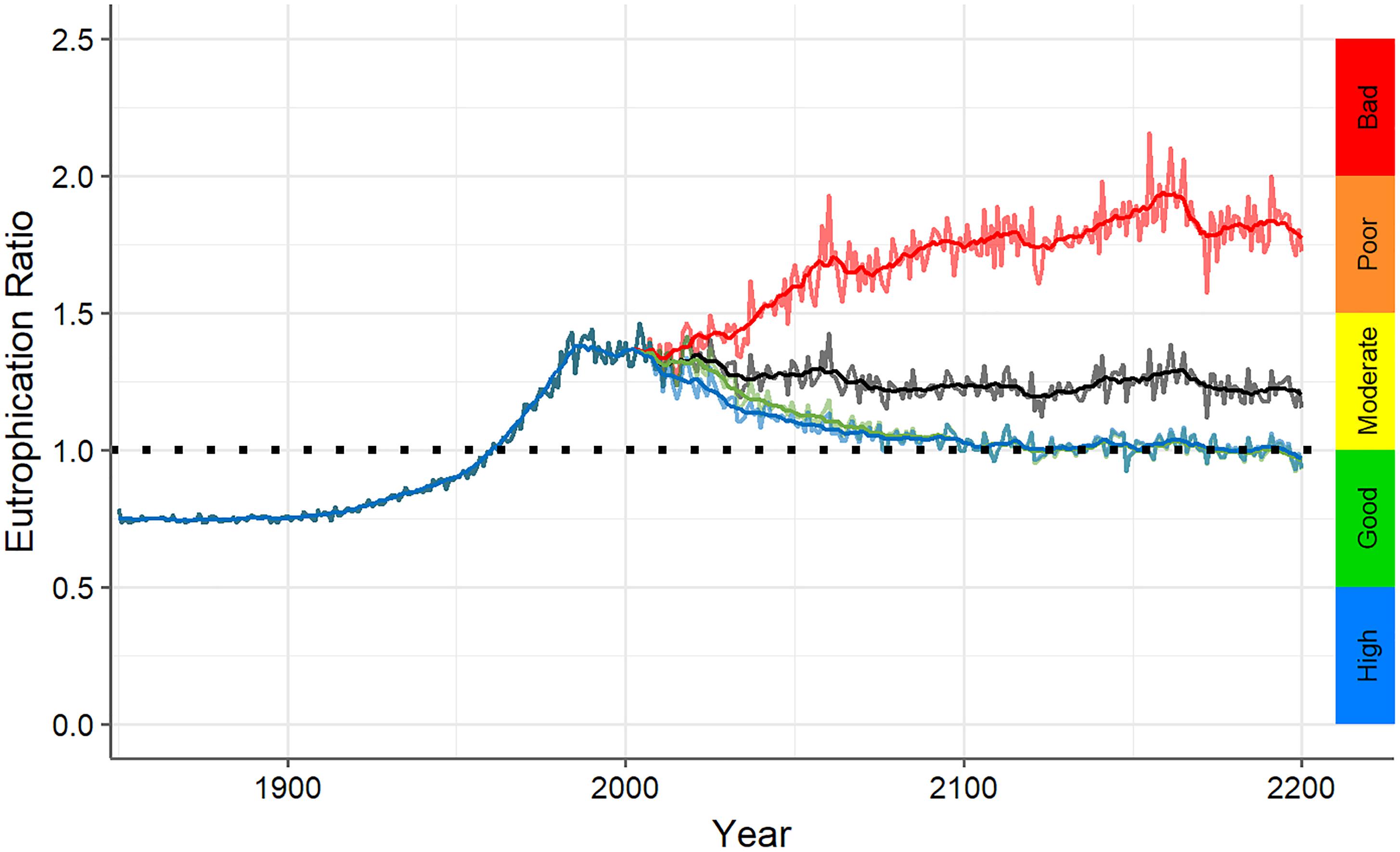
Figure 6. Integrated Baltic Sea-wide HEAT assessment. Averaging the nine basin ER values to a single value representing the entire Baltic Sea. For each scenario, the lighter shaded line shows the annual result and the darker line shows the 10-year moving average. The dotted line marks ER = 1, the boundary for good eutrophication status. The figure also indicates the ER ranges for colours in Figure 5.
The long-term trends in loads, indicators and eutrophication status follow distinctive trajectories for the four scenarios, though the differences between BSAP0 and BSAP30 scenarios narrow continuously. Communicating the links between human activities, the loads to the Baltic Sea, the responses in selected indicators, the time lags and ultimately the overall implication with respect to eutrophication status, to decision makers is important. One of many ways of synthesizing the results of this Baltic Sea-wide study is to compare the trends for selected indicators and for the worst-case (BAU30 scenario) and best-case scenario (BSAP0 scenario). By doing so, we illustrate the difference between implementing a state-of-the-art ecosystem-based nutrient management strategy (BSAP) and doing nothing at all (Figure 7).
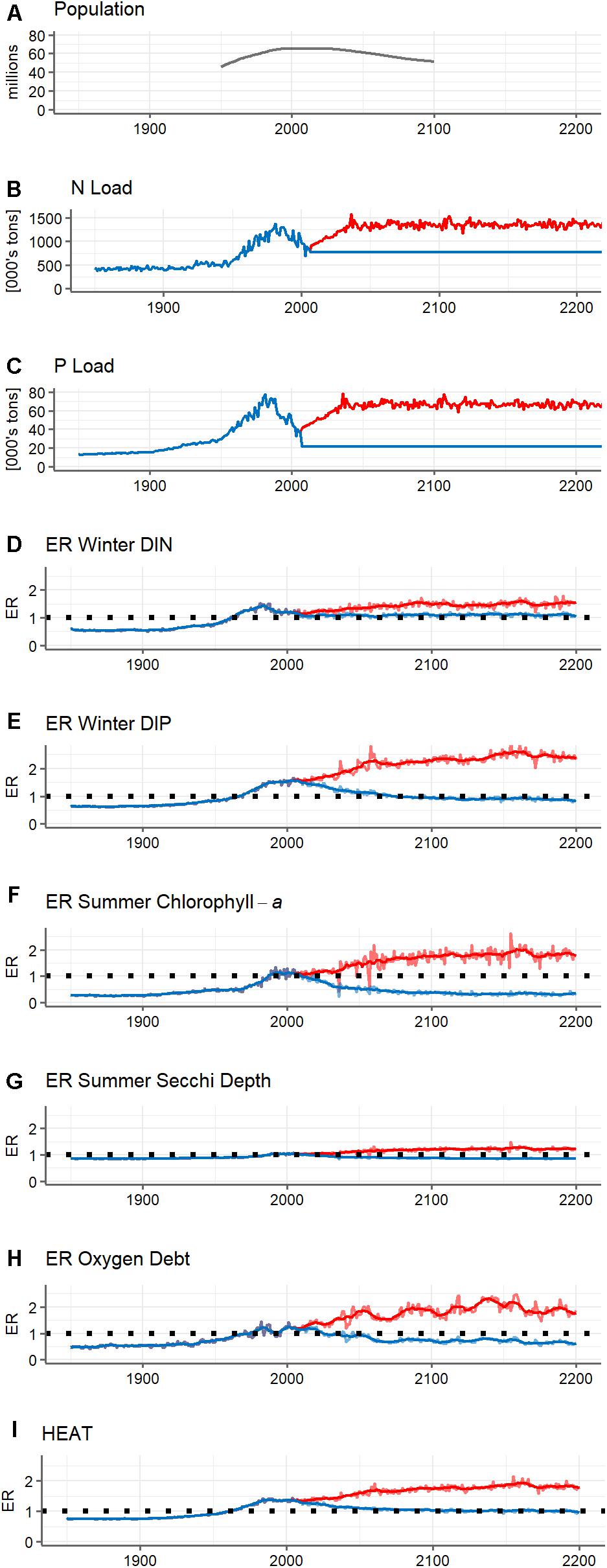
Figure 7. Long-term temporal trends for the Baltic Sea. (A) Estimated (1950–2015) and predicted (2015–2100) total population of seven Baltic countries∗, (B,C) total loads of N and P, (D–H) modelled trajectories of Eutrophication Ratio (ER) for dissolved inorganic nitrogen (DIN), dissolved inorganic phosphorous (DIP), chlorophyll-a, Secchi depth, and Oxygen Debt averaged over the nine basins, for the best- (BSAP0: blue) and worst-case (BAU30: red) load scenarios, and (I) the integrated assessment of eutrophication status. For ER values (D–I), annual values are shown with thin lines and the 10-year moving averages with thick lines. ∗Denmark, Estonia, Finland, Latvia, Lithuania, Sweden, and Poland (source: United Nations Department of Economic Social Affairs Population Division, 2017).
An interesting finding from the long-term trends is that the biological responses (chlorophyll-a, Secchi depth and oxygen debt) return to a good status with respect to eutrophication earlier than indicators for DIN and DIP concentrations. In part, different degrees of model bias contribute to the difference in timing. While BALTSEM captures phytoplankton trends, it tends to underestimate biomass and therefore chlorophyll-a concentrations (Supplementary Figure S8). Since phytoplankton biomass enters Secchi depth calculations, Secchi depth is slightly overestimated (Supplementary Figure S9), whereas nutrient and oxygen concentrations are simulated with little bias (Supplementary Figures S6, S7, S10). On the other hand, target values for biological variables, nutrient and oxygen levels have been developed by applying statistical change point detection methods to each time-series individually (HELCOM, 2013a). Thus, they do not necessarily reflect a single year in the eutrophication trajectory of the Baltic Sea. That targets might not be met simultaneously and might even be unachievable in individual basins was also taken into account in the 2013 Baltic Sea Action Plan revision. For example, the winter DIN target in the Gulf of Riga was disregarded (Gustafsson and Mörth, 2013), since increasing phosphorus limitation tended to increase DIN concentrations in the Gulf (see also Müller-Karulis and Aigars, 2011), while other indicators like phytoplankton biomass and oxygen debt reached their ecological targets. Both the Gulf of Riga and the Bothnian Bay are exceptionally phosphorous limited and becoming more so. This explains why the revised Baltic Sea Action Plan does not include nitrogen reductions in these basins even though nitrogen concentration targets are exceeded.
In other words, considering biological response indicators alone, it is likely that something resembling a Baltic Sea unaffected by eutrophication would be achieved earlier than when all indicators are used in the assessment. The responses of nutrient indicators to the load reductions (Figures 7D,E) do not appear to be lag behind the responses of biological indicators (Figures 7F,G) or oxygen debt (Figure 7H). However, they take longer to reach the target and do not reach as close to or as far under the target values.
Considering each of the 39 separate combinations of indicator and basin (See Supplementary Figures S1–S5) a similar pattern is seen. For BSAP0 and BSAP30, predicted concentrations of chlorophyll-a in 2200 are less than 50% of the target value in all but one of the nine basins, in some cases far less. For winter DIN in the BSAP0 scenario, the best case is in Arkona Basin where the concentration has fallen under the target and reached approximately 70% of the target value by 2200. For five of the other basins (Kattegat, Danish Straits, Bornholm Basin, Baltic Proper, and Bothnian Sea), DIN concentrations in 2200 lie close to the target values and for the three remaining basins (Gulf of Riga, Gulf of Finland, and Bothnian Bay) they are clearly above target values. Thus, according to the model the target values for nutrients are significantly more stringent than those for chlorophyll-a, Secchi depth and oxygen debt. However, since the biological indicators are dependent on nutrients and not the other way around, this situation can be seen in a positive light, in that it ensures that the nutrient targets are sufficiently ambitious to achieve the desired changes in biological indicators.
Conclusion and Perspectives
The BSAP may, according to model predictions, be an efficient driver regarding reduction of nutrient loads. However, this requires commitments from all HELCOM Contracting Parties to meet BSAP load reduction targets. Without a collective and strong commitment, we risk failing to attain a Baltic Sea unaffected by eutrophication.
This study indicates that a good status with respect to eutrophication will be met for most parts of the Baltic Sea, if the BSAP nutrient reductions are fully implemented. This recovery has already started but the ultimate effects will not be visible soon, but in a much longer perspective. An encouraging result of the study is that it concludes that the overall objective of a healthy Baltic Sea is within reach. Patience is required, as well as a continuation of the load reductions achieved so far, such that the load reduction targets set by BSAP are met. An interesting and positive finding is that the indicators representing biological responses do seem to respond faster to load reduction than indicators representing nutrient concentrations. This implies that the visual appearance of the Baltic will reach a good status earlier than the integrated assessment based on the HEAT tool and the full range of indicators.
On a less positive note, we should remember that none of the scenarios take into account climate change, where elevated sea temperatures are of concern. Thus, there seems to be an urgent need to include climate change in future updates of the BSAP and to update the projected development in eutrophication status.
Author Contributions
CM and JA conceived the study. BM-K and BG provided modelled trajectories from the BALTSEM model. JC and BG provided observed indicator trajectories. CM did the integration calculations. CM, JA, BG, and BM-K wrote a preliminary version of the manuscript. All authors discussed and reworked the manuscript.
Funding
This study is a Baltic Nest Institute activity co-funded by Aarhus University (AU) and Stockholm University (SU). Parts of the analyses were funded by EEA ETC ICM 2016 task 1.6.1.g (‘Eutrophication in Europe’s seas’).
Conflict of Interest Statement
The authors declare that the research was conducted in the absence of any commercial or financial relationships that could be construed as a potential conflict of interest.
Acknowledgments
We thank all those who have participated in development, testing, or application of various versions of the HEAT tool over the past decade. This study is dedicated to the memory of Prof. Fredrik Wulff.
Supplementary Material
The Supplementary Material for this article can be found online at: https://www.frontiersin.org/articles/10.3389/fmars.2019.00002/full#supplementary-material
References
Andersen, J. H., Axe, P., Backer, H., Carstensen, J., Claussen, U., Fleming-Lehtinen, V., et al. (2011). Getting the measure of eutrophication in the Baltic Sea: towards improved assessment principles and methods. Biogeochemistry 106, 137–156. doi: 10.1007/s10533-010-9508-4
Andersen, J. H., Carstensen, J., Conley, D., Dromph, K., Fleming-Lehtinen, V., Gustafsson, B. G., et al. (2017). Long-term temporal and spatial trends in eutrophication status of the Baltic Sea. Biol. Rev. 92, 135–149. doi: 10.1111/brv.12221
Andersen, J. H., Fossing, H., Hansen, J. W., Manscher, O. H., Murray, C., and Petersen, D. L. (2014). Nitrogen inputs from agriculture: towards better assessments of eutrophication status in marine waters. Ambio 43, 906–913. doi: 10.1007/s13280-014-0514-y
Andersen, J. H., Kallenbach, E., Murray, C., and Ledang, A. B. (2016). Eutrophication in the Danish parts of the North Sea, Skagerrak and Kattegat 2006-2014. A Literature-Based Assessment. NIVA Denmark Report. Available at: https://brage.bibsys.no/xmlui/handle/11250/2406499.
Andersen, J. H., Murray, C., Kaartokallio, H., Axe, P., and Molvær, J. (2010). A simple method for confidence rating of eutrophication status assessments. Mar. Pollut. Bull. 60, 919–924. doi: 10.1016/j.marpolbul.2010.03.020
Andersen, J. H., Schlüter, L., and Ærtebjerg, G. (2006). Coastal eutrophication: recent developments in definitions and implications for monitoring strategies. J. Plankton Res. 28, 621–628. doi: 10.1093/plankt/fbl001
Anon (2008). Directive 2008/56/EC of the European Parliament and of the Council of 17 June 2008 establishing a framework for community action in the field of marine environmental policy (Marine Strategy Framework Directive). Off. J. Eur. Commun. L 26, 136–157.
Backer, H., Leppänen, J. M., Brusendorff, A. C., Forsius, K., Stankiewicz, M., Mehtonen, J., et al. (2010). HELCOM Baltic Sea Action Plan: a regional program of measures for the marine environment based on the Ecosystem Approach. Mar. Pollut. Bull. 60, 642–649. doi: 10.1016/j.marpolbul.2009.11.016
Bonsdorff, E. (2006). Zoobenthic diversity-gradients in the Baltic Sea: continuous post-glacial succession in a stressed ecosystem. J. Exp. Mar. Biol. Ecol. 330, 283–391. doi: 10.1016/j.jembe.2005.12.041
Bricker, S. B., Longstaff, B., Dennison, W., Jones, A., Boicourt, K., Wicks, C., et al. (2008). Effects of nutrient enrichment in the nation’s estuaries: a decade of change. Harmful Algae 8, 21–32. doi: 10.1016/j.hal.2008.08.028
Carstensen, J., Gustafsson, B. G., Andersen, J. H., and Conley, D. J. (2014). Deoxygenation of the Baltic Sea during the last century. Proc. Natl. Acad. Sci. U.S.A. 111, 5628–5633. doi: 10.1073/pnas.1323156111
Carstensen, J., Sánchez-Camacho, M., Duarte, C. M., Krause-Jensen, D., and Marbà, N. (2011). Connecting the dots: responses of coastal ecosystems to changing nutrient concentrations. Environ. Sci. Technol. 45, 9122–9132. doi: 10.1021/es202351y
Conley, D. J., Carstensen, J., Aigars, J., Axe, P., Bonsdorff, E., Eremina, T., et al. (2011). Hypoxia is increasing in the coastal zone of the Baltic Sea. Environ. Sci. Technol. 45, 6777–6783. doi: 10.1021/es201212r
Eilola, K., Gustafsson, B. G., Kuznetsov, I., Meier, H. E. M., Neumann, T., and Savchuk, O. P. (2011). Evaluation of biogeochemical cycles in an ensemble of three state-of-the-art numerical models of the Baltic Sea. J. Mar. Syst. 88, 267–284. doi: 10.1016/j.jmarsys.2011.05.004
Finni, T., Kononen, K., Olsonen, R., and Wallström, K. (2001). The history of cyanobacterial blooms in the Baltic Sea. Ambio 30, 172–178. doi: 10.1579/0044-7447-30.4.172
Fleming-Lehtinen, V. (2016). Secchi Depth in the Baltic Sea – an Indicator of Eutrophication. Ph.D. dissertation, University of Helsinki, Helsinki.
Fleming-Lehtinen, V., Andersen, J. H., Carstensen, J., Łysiak-Pastuszak, E., Murray, C., Pyhälä, M., et al. (2015). Recent developments in assessment methodology reveal that the Baltic Sea eutrophication problem is expanding. Ecol. Indic. 48, 380–388. doi: 10.1016/j.ecolind.2014.08.022
Gustafsson, B. G., and Mörth, C. M. (2013). Draft Scientific Background on Revision of the Maximum Allowable Inputs and Country Allocation Scheme of the Baltic Sea Action Plan V.4, document 2-43, HELCOM Heads of Delegation Meeting No 41. Helsinki. Available at: https://portal.helcom.fi/Archive/Shared%20Documents/HOD%2041-2013_2-43%20Draft%20Scientific%20background%20report%20on%20revision%20of%20MAI%20and%20CART.pdf
Gustafsson, B. G., Schenk, F., Blenckner, T., Eilola, K., Meier, H. E. M., Müller-Karulis, B., et al. (2012). Reconstructing the development of Baltic Sea Eutrophication 1850–2006. Ambio 41, 534–548. doi: 10.1007/s13280-012-0318-x
Gustafsson, E. O., Savchuk, O. P., Gustafsson, B. G., and Müller-Karulis, B. (2017). Key processes in the coupled carbon, nitrogen, and phosphorus cycling of the Baltic Sea. Biogeochemistry 134, 301–317. doi: 10.1007/s10533-017-0361-6
Hägg, H. E., Lyon, S. W., Wällstedt, T., Mörth, C.-M., Claremar, B., and Humborg, C. (2014). Future nutrient load scenarios for the baltic sea due to climate and lifestyle changes. Ambio 43:337. doi: 10.1007/s13280-013-0416-4
HELCOM (2007). HELCOM Baltic Sea Action Plan. HELCOM Ministerial Meeting. Available at: http://www.helcom.fi/Documents/Baltic%20sea%20action%20plan/BSAP_Final.pdf (accessed November 15, 2007).
HELCOM (2009). Eutrophication in the Baltic Sea. An Integrated Thematic Assessment of Eutrophication in the Baltic Sea Region. Baltic Sea Environmental Proceedings No. 115B. Helsinki Commission, p. 148. Available at: http://www.helcom.fi/Lists/Publications/BSEP115B.pdf
HELCOM (2013a). Approaches and Methods for Eutrophication Target Setting in the Baltic Sea Region. Baltic Sea Environment Proceedings 133, p. 147. Available at: http://www.helcom.fi/Lists/Publications/BSEP133.pdf
HELCOM (2013b). HELCOM Copenhagen Ministerial Declaration. Taking Further Action to Implement the Baltic Sea Action Plan – Reaching Good Environmental Status for a Healthy Baltic Sea. Available at: http://www.helcom.fi/Documents/Ministerial2013/Ministerial%20declaration/2013%20Copenhagen%20Ministerial%20Declaration%20w%20cover.pdf (accessed October 3, 2013).
HELCOM (2013c). Review of the Fifth Baltic Sea Pollution Load Compilation for the 2013 HELCOM Ministerial Meeting. Baltic Sea Environment Proceedings 141, p. 54. Available at: http://helcom.fi/Lists/Publications/BSEP141.pdf
Johannesson, K., and André, C. (2006). Life on the margin: genetic isolation and diversity loss in a peripheral marine ecosystem, the Baltic Sea. Mol. Ecol. 15, 2013–2029. doi: 10.1111/j.1365-294X.2006.02919.x
Köster, F. W., Hinrichsen, H., St. John, M. A., Schnack, D., MacKenzie, B. R., Tomkiewicz, J., et al. (2001). Developing Baltic cod recruitment models. II. Incorporation of environmental variability and species interaction. Can. J. Fish. Aquat. Sci. 58, 1534–1556. doi: 10.1139/f01-093
Kristensen, P., Whalley, C., Nery, F., Zal, N., and Christiansen, T. (2018). “European waters – assessment of status and pressures 2018,” in EEA Report No. 7/2018, (Copenhagen: European Environment Agency), 85.
Larsson, U., Elmgren, R., and Wulff, F. (1985). Eutrophication and the Baltic Sea: causes and consequences. Ambio 14, 9–14.
Leppäranta, M., and Myrberg, K. (2009). Physical Oceanography of the Baltic Sea. New York, NY: Springer-Verlag, 378.
MacKenzie, B. R., Hinrichsen, H., Plikshs, M., Wieland, K., and Zezera, A. S. (2000). Quantifying environmental heterogeneity: habitat size necessary for successful development of cod gadus morhua eggs in the Baltic Sea. Mar. Ecol. Prog. Ser. 193, 143–156. doi: 10.3354/meps193143
Meier, H. E. M., Andersson, H. C., Eilola, K., Gustafsson, B. G., Kuznetsov, I., Müller-Karulis, B., et al. (2011). Hypoxia in future climates: a model ensemble study for the Baltic Sea. Geophys. Res. Lett. 38:L24608. doi: 10.1029/2011GL049929
Meier, H. E. M., Edman, M. K., Eilola, K. J., Placke, M., Neumann, T., Andersson, H. C., et al. (2018a). Assessment of eutrophication abatement scenarios for the Baltic sea by multi-model ensemble simulations. Front. Mar. Sci. 5:44. doi: 10.3389/fmars.2018.0044
Meier, H. E. M., Eilola, K., Almroth-Rosell, E., Schimanke, S., Kniebusch, M., Höglund, A., et al. (2018b). Disentangling the impact of nutrient load and climate changes on Baltic Sea hypoxia and eutrophication since 1850. Clim. Dynam. 1–3. doi: 10.1007/s00382-018-4296-y
Meier, H. E. M., Müller-Karulis, B., Andersson, H. C., Dieterich, C., Eilola, K., Gustafsson, B. G., et al. (2012). Impact of climate change on ecological quality indicators and biogeochemical fluxes in the Baltic Sea: a multi-model ensemble study. Ambio 41, 558–573. doi: 10.1007/s13280-012-0320-3
Müller-Karulis, B., and Aigars, J. (2011). Modeling the long-term dynamics of nutrients and phytoplankton in the Gulf of Riga. J. Mar. Syst. 87, 161–176. doi: 10.1016/j.jmarsys.2011.03.006
Neumann, T., Eilola, K., Gustafsson, B., Müller-Karulis, B., Kuznetsov, I., Meier, H. E. M., et al. (2012). Extremes of temperature, oxygen and blooms in the Baltic sea in a changing climate. Ambio 41, 574–585. doi: 10.1007/s13280-012-0321-2
Nixon, S. W. (2009). Eutrophication and the macroscope. Hydrobiologia 629, 5–19. doi: 10.1007/s10750-009-9759-z
OSPAR (2017). Eutrophication Status of the OPSAR Maritime Area. Third Integrated report on the Eutrophication Status of the OPSAR Maritime Area. Eutrophication Series. London: OSPAR Commission, 164.
Österblom, H., Hansson, S., Larsson, U., Hjerne, F., Wulff, F., Elmgren, R., et al. (2007). Human-induced trophic cascades and ecological regime shifts in the Baltic Sea. Ecosystems 10, 877–889. doi: 10.1007/s10021-007-9069-0
Oviatt, C., Smith, L., Krumholz, J., Coupland, C., Stoffel, H., Keller, A., et al. (2017). Managed nutrient reduction impacts on nutrient concentrations, water clarity, primary production, and hypoxia in a north temperate estuary. Estuar. Coast. Shelf Sci. 199, 25–34. doi: 10.1016/j.ecss.2017.09.026
Richardson, K., and Heilmann, J. P. (1995). Primary production in the Kattegat: past and present. Ophelia 41, 317–328. doi: 10.1080/00785236.1995.10422050
Riemann, B., Carstensen, J., Dahl, K., Fossing, H., Hansen, J. W., Jakobsen, H. H., et al. (2016). Recovery of Danish coastal ecosystems after reductions in nutrient loading: trends and time lags. Estuar. Coasts 39, 82–97. doi: 10.1007/s12237-015-9980-0
Rönnberg, C., and Bonsdorff, E. (2004). Baltic Sea eutrophication: area-specific ecological consequences. Hydrobiologia 514, 227–241. doi: 10.1023/B:HYDR.0000019238.84989.7f
Savchuk, O. P. (2018). Myths of the Baltic Sea eutrophication. J. Aquac. Mar. Biol. 7, 92–93. doi: 10.15406/jamb.2018.07.00190
Savchuk, O. P., Gustafson, B. G., and Müller-Karulis, B. (2012). BALTSEM - A Marine Model for Decision Support within the Baltic Sea Region (No. Technical Report No. 7). Vienna: Baltic Nest Institute.
Staehr, P. A., Testa, J., and Carstensen, J. (2017). Decadal changes in water quality and net productivity of a shallow Danish estuary following significant nutrient reductions. Estuar. Coasts 40, 63–79. doi: 10.1007/s12237-016-0117-x
Stigebrandt, A., and Gustafsson, B. G. (2003). Response of the Baltic Sea to climate change—Theory and observations. J. Sea Res. 49, 243–256. doi: 10.1016/S1385-1101(03)00021-2
United Nations Department of Economic Social Affairs Population Division (2017). World Population Prospects: The 2017 Revision, DVD Edition. Available at: https://population.un.org/wpp/DVD/Files/1_Indicators%20(Standard)/EXCEL_FILES/1_Population/WPP2017_POP_F01_1_TOTAL_POPULATION_BOTH_SEXES.xlsx.
Vahtera, E., Conley, D. J., Gustafsson, B. G., Kuosa, H., Pitkänen, H., Savchuk, O. P., et al. (2007). Internal ecosystem feedbacks enhance nitrogen-fixing cyanobacteria blooms and complicate management in the Baltic Sea. Ambio 36, 186–194. doi: 10.1579/0044-7447(2007)36[186:IEFENC]2.0.CO;2
van Beusekom, J. E. E., Carstensen, J., Kolbe, K., Lenhart, H.-J., Pätsch, J., and Rick, J. (2018). “Wadden Sea Eutrophication: long-term trends and regional differences,” in EUTRO 2018: 4th International Symposium on Research and Management of Eutrophication in Coastal Ecosystems: Programme and Book of Abstracts, ed. J. H. Andersen, Nyborg, 30.
Villnäs, A., and Norkko, A. (2011). Benthic diversity gradients and shifting baselines: implications for assessing environmental status. Ecol. Appl. 21, 2172–2186. doi: 10.1890/10-1473.1
Walve, J., Rolff, C., and Tidlund, A. (2018). Svealandskusten 2018. (Report) Svealands kustvattenvårdsförbund. Available at: https://www.havet.nu/dokument/Svealandskusten2018.pdf
Wasmund, N., Göbel, J., and Von Bodungen, B. (2008). 100-years-changes in the phytoplankton community of Kiel Bight (Baltic Sea). J. Mar. Syst. 73, 300–322. doi: 10.1016/j.jmarsys.2006.09.009
Zhang, Q., Murphy, R. R., Tian, R., Forsyth, M. K., Trentacoste, E. M., Keisman, J., et al. (2018). Chesapeake Bay’s water quality condition has been recovering: insights from a multimetric indicator assessment of thirty years of tidal monitoring data. Sci. Total Environ. 637–638, 1617–1625. doi: 10.1016/j.scitotenv.2018.05.025
Keywords: eutrophication, Baltic Sea, nutrient loads, modelling, scenarios, integrated assessment, status classification
Citation: Murray CJ, Müller-Karulis B, Carstensen J, Conley DJ, Gustafsson BG and Andersen JH (2019) Past, Present and Future Eutrophication Status of the Baltic Sea. Front. Mar. Sci. 6:2. doi: 10.3389/fmars.2019.00002
Received: 01 October 2018; Accepted: 07 January 2019;
Published: 25 January 2019.
Edited by:
Dongyan Liu, State Key Laboratory of Estuarine and Coastal Research (ECNU), ChinaReviewed by:
Hsiao-Chun Tseng, National Taiwan Ocean University, TaiwanMarco Uttieri, Stazione Zoologica Anton Dohrn, Italy
Copyright © 2019 Murray, Müller-Karulis, Carstensen, Conley, Gustafsson and Andersen. This is an open-access article distributed under the terms of the Creative Commons Attribution License (CC BY). The use, distribution or reproduction in other forums is permitted, provided the original author(s) and the copyright owner(s) are credited and that the original publication in this journal is cited, in accordance with accepted academic practice. No use, distribution or reproduction is permitted which does not comply with these terms.
*Correspondence: Ciarán J. Murray, Y2ptQG5pdmEtZGsuZGs=