- 1Escuela Superior Politécnica del Litoral, ESPOL, Facultad de Ingeniería Marítima y Ciencias del Mar, Guayaquil, Ecuador
- 2Instituto Oceanográfico de la Armada, INOCAR, Dirección de Oceanografía Naval, Guayaquil, Ecuador
- 3Escuela Superior Politécnica del Litoral, ESPOL, Facultad de Ciencias Naturales y Matemáticas, Guayaquil, Ecuador
- 4Galapagos Marine Research and Exploration Program (GMaRE), ESPOL-CDF Joint Research Program, Charles Darwin Research Station, Santa Cruz, Ecuador
- 5Escuela Superior Politécnica del Litoral, ESPOL, Centro Nacional de Acuicultura e Investigaciones Marinas, CENAIM, Guayaquil, Ecuador
Ocean climate drivers and phytoplankton life strategies interact in a complex dynamic to produce harmful algal blooms (HABs). This study aims to integrate historical biological data collected during “red tide” events along the Ecuadorian coast between 1997 and 2017 in relation to five ocean variables derived from satellite remote sensing data to explain the seasonal drivers of coastal processes associated with HABs dynamics. Seasonality of the occurrence of HABs was assessed in relation to oceanographic variables by applying multiple correspondence analysis (MCA) to the Ecuadorian central coast (Zone 1) and at the outer and inner Gulf of Guayaquil (Zone 2). Sixty-seven HABs events were registered between 1997 and 2017. From a total of 40 species of phytoplankton identified, 28 were identified as non-toxic and the remaining 12 are well known to produce toxins. Dinoflagellates were the taxonomic group most highly associated with potential HABs events along the entire Ecuadorian coast. HABs appear to be constrained by the Humboldt coastal upwelling, high precipitation, and associated coastal runoff, with higher biomass abundance in the Gulf of Guayaquil than in the central coast. Results from the MCA reveal that in the central Ecuadorian coast (oligotrophic system), toxic HABs occurred with low abundance of dinoflagellates, while in the Gulf of Guayaquil (eutrophic system), toxic HABs corresponded to a high abundance of dinoflagellates. In both cases, high values were found for sea surface temperature, precipitation, and irradiance—characteristic of wet seasons or El Niño years. Non-toxic HABs occurred with a high abundance of dinoflagellates, ciliates, and centric diatoms, corresponding to colder waters and low levels of precipitation and irradiance. These findings confirm that dinoflagellates display several strategies that enhance their productive capacity when ocean conditions are warmer, allowing them to produce toxins at high or at low concentrations. Considering that the Gulf of Guayaquil is essential to tourism, the shrimp industry, fisheries, and international shipping, these findings strongly suggest the need to establish an ecosystem health research program to monitor HABs and the development of a preventive policy for tourism and public health in Ecuador.
Introduction
For decades, global coastal waters have experienced events known as “red tides” that are related to phytoplankton blooms, micro-algal blooms, and toxic algal or potentially harmful algal blooms (HABs) (Hallegraeff, 1993; Anderson, 2014; Anderson et al., 2017). Blooms are described as occurrences of a high biomass of plankton and benthic species, while HABs have been broadly classified as potent natural toxin producers created either by attaining high biomass levels or even through low numbers of toxic species. HABs may take place when a group of microalgae—photosynthetic cells found in the sea, brackish water, and freshwater—grow to very high numbers, producing toxic or harmful effects on shellfish, fish, marine mammals, birds, and humans (Hallegraeff, 1993; Zingone and Enevoldsen, 2002; Fleming et al., 2006; Jiménez and Gualancañay, 2006; Pitcher et al., 2017).
High-density algal blooms may cause anoxia to marine life, killing it and blocking sunlight on the surface of the water column. However, international research on HABs has recognized that some species have toxic effects even at low cells densities (Reguera et al., 2012; Anderson, 2014). The reasons why an algal bloom becomes toxigenic, either at high or low concentrations, is still not well understood. However, oceanographic and climatic conditions play an important role in HAB occurrence, which may be constrained by upwelling systems and stratification of the water column provoked by low wind stress and marine heat waves (Hallegraeff, 2010; Díaz et al., 2013; Pitcher et al., 2017). HABs may also be exacerbated by climatic variability, extreme events, like El Niño–Southern Oscillation (ENSO) (Sellner et al., 2003), and climate change due to a long-term increase in sea surface temperatures (Wells et al., 2015; Miller et al., 2006; Pitcher et al., 2017). In addition, anthropogenic nutrient loading from agricultural and urban watersheds, ballast water discharges from vessels, and nutrients released from mariculture activities, among others, are factors that drive HABs in coastal waters (Moore et al., 2008; Hallegraeff, 2010; Davidson et al., 2014; Berdalet et al., 2016).
Currently, the development of ocean remote sensing-derived data makes possible the exploration of the synoptic climatology of regions, biogeography of the primary productivity and HABs biomass, spring bloom growth, and even the identification of species at a good scale of resolution in space and time (McClain et al., 2004; Nair et al., 2008; Wei et al., 2008; McKibben et al., 2015). Thus, HAB dynamics can be explained by using in situ biological data and remote sensing observations of oceanographic variables (Moisan et al., 2011; Anderson, 2014; Blondeau-Patissier et al., 2014).
South America’s Ecuadorian coast of the eastern tropical Pacific can be characterized by two biogeographical regions: the northern coast, influenced by the warm Panama Current, and the Gulf of Guayaquil, influenced by the Humboldt Current (Cucalon, 1989; Longhurst, 2006). Both regions are heavily affected by ENSO (Lee et al., 2014) and the eastern upwelling system, and these oceanographic structures can modify the ocean biogeochemistry periodically. All these oceanographic characteristics may trigger the occurrence of HABs along the Ecuadorian coast (Chavez et al., 2003; Pennington et al., 2006; Trainer et al., 2010). Following the global trend, the coast of Ecuador has experienced the occurrence of “red tides” for decades. Ecuadorian researchers have recorded approximately 132 events since 1968, resulting in reports of fish, juvenile shrimp, and shrimp larvae mortality (Torres, 2000, 2011, 2013, 2015, 2017; Torres and Tapia, 2002). Sixty-seven HABs events were registered between 1997 and 2017.
Despite their negative impacts on coastal activities, attempts to explain the oceanographic and climate drivers for the potential development of HABs are limited (Jiménez and Intriago, 2001; Jiménez and Gualancañay, 2006; Torres et al., 2017; Conde and Prado, 2018; Conde et al., 2018). Unfortunately, at this time there is no official HAB monitoring program for Ecuador that can analyze which toxins are generated by the phytoplankton species. Using a novel approach, this study intends to integrate ocean features, derived from synoptic remote sensing, and biological data, compiled from reports collected during “red tide” events, to identify which oceanographic conditions lead to the distribution and seasonal occurrence of HABs on the coast of Ecuador.
Materials and Methods
Study Area
The study area is situated along the center and south of the Ecuadorian coast (South America) and was divided into two zones (Figure 1): Zone 1, corresponding to the area situated between the coastal localities of Manta (80.8°W, 0.8°S) and La Libertad (81°W, 2°S); and Zone 2, which includes the coastal area of the Gulf of Guayaquil (outer estuary) and the Guayas River estuary (inner estuary). The Guayas River estuary is connected to the El Morro and Jambelí channels and is comprised of two main bodies of water: Estero Salado and the Guayas River (Figure 1). Zone 1 is characterized mostly by sandy beaches used for tourism, and it is the location of important international cargo ports and fishery activities. Zone 2 includes the largest estuarine system on the South America coast adjacent to the Pacific Ocean and is where the cities of Guayaquil and Machala are located (Figure 1). The main economic activities carried out in Zone 2 are connected to shrimp farming, international cargo ports, and artisanal fisheries. Climate seasonality of the Ecuadorian coast is characterized by a wet season (December–May), with a unimodal peak of warm temperatures (March–April), and a dry season (June–November) characterized by the absence of rainfall and lower temperatures than those experienced during the wet season (Cucalon, 1989; Bendix et al., 2011).
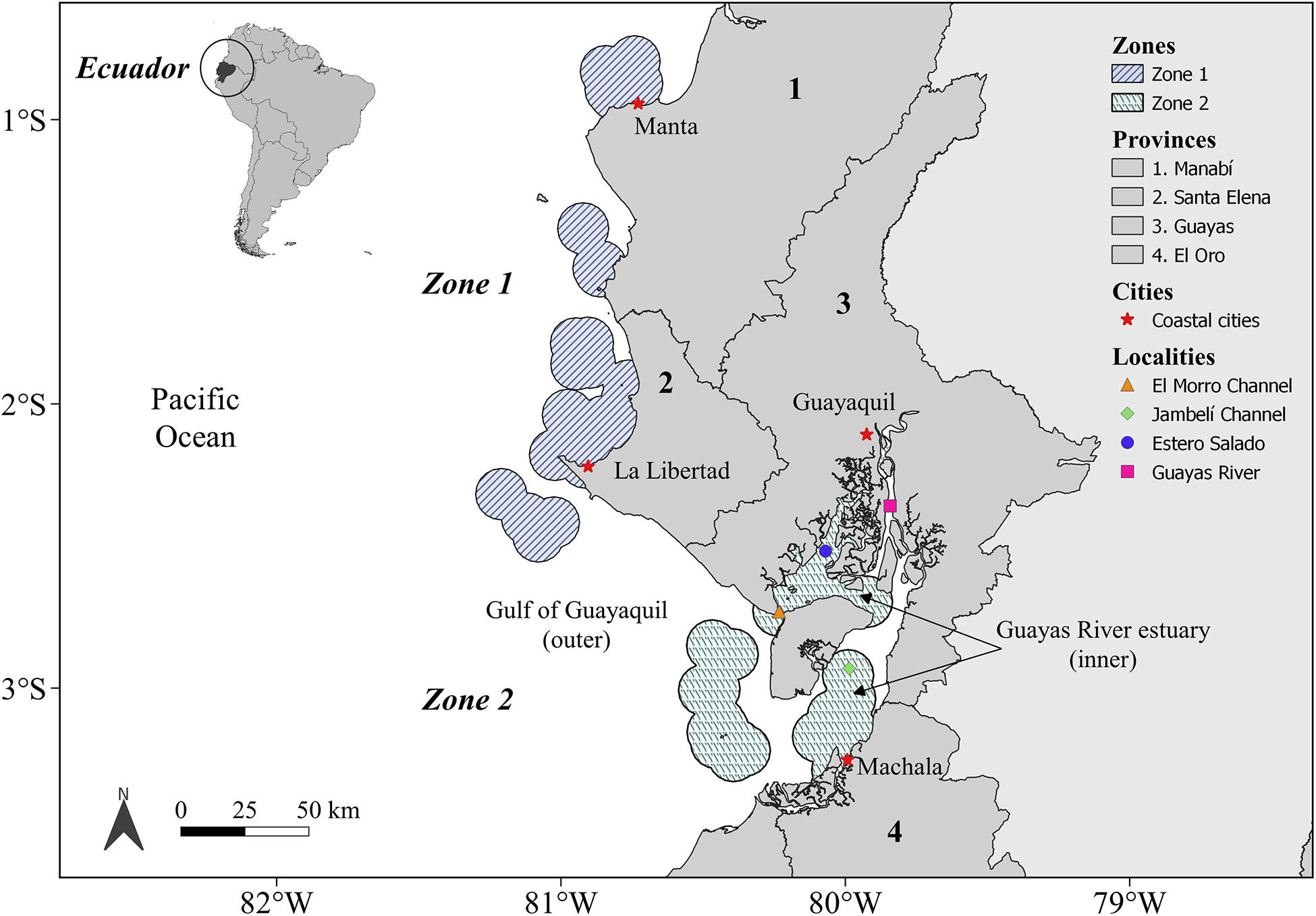
Figure 1. Study area. Zone 1 is situated between the coastal cities of Manta and La Libertad; Zone 2 is located around the outer and inner (Estero Salado and Jambelí Channel) Gulf of Guayaquil estuary.
Oceanographic and Atmospheric Data
Monthly averages of five variables recorded over the 1997–2017 period were derived from different satellite data compiled at ∼4 km of spatial resolution around Zones 1 and 2 on the Ecuadorian coast. Precipitation (PRE) was chosen as an atmospheric variable associated with climate seasonality (Nair et al., 2008; Shen et al., 2012) and therefore used as a proxy for seasonal atmospheric processes. In addition, seasonality of the region is strongly related to precipitation, which is also disturbed by the occurrence of El Niño and La Niña events in the equatorial eastern tropical Pacific (Pennington et al., 2006). The other four variables were oceanographic, representing select conditions of the ocean at specific times and places. Sea surface temperature (SST) is related to cold nutrient-rich currents and upwelling or with nutrient-poor warm currents (Pennington et al., 2006; Wei et al., 2008; Mélin and Hoepffner, 2011). Chlorophyll a (chl-a) was used as a proxy for phytoplankton abundance. Absorption due to phytoplankton at 443 nm (aph) represents the band of chlorophyll associated with the HABs peaks (Shen et al., 2012). Photosynthetically active radiation (PAR) was chosen as an indicator of the amount of light available for photosynthesis (Shi et al., 2014). Previously, SST and PRE have been related to the oceanographic and atmospheric processes of HABs, respectively (Wei et al., 2008). Satellite images were collected in NetCDF format and converted to GeoTiff files for the analyses using R software (version 3.5.0). Several satellites and sensors were used throughout the period of analysis (Table 1). Each variable was standardized with the respective global mean and standard deviation.
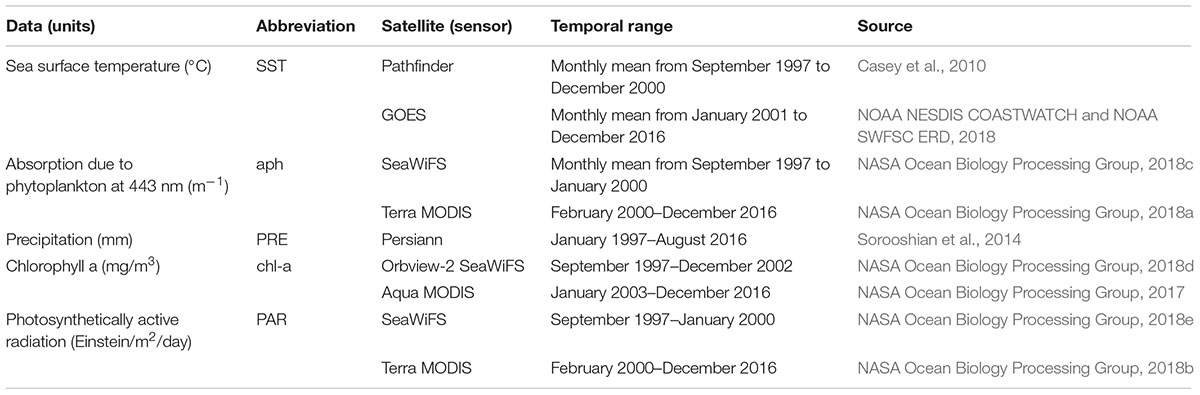
Table 1. Description of oceanographic (SST, aph, chl-a, PAR) and atmospheric (PRE) variables from remote sensors.
Phytoplankton Data
Phytoplankton data were compiled from 67 algal bloom events reported from 1997 to 2017 in the literature generated by the Ecuadorian Navy Army Oceanographic Institute (INOCAR), the National Institute of Fisheries, and reports from shrimp hatchery producers. Most of these data are included in the periodical research publications of INOCAR1. According to these reports, samples were collected from the sea surface. Data included taxa identification, abundance, location, and an observational description. This study also includes 8 unpublished reports from INOCAR and other researchers, increasing the original number of 59 registered events to 67 events in total. More detailed information on each event can be found in the publications and reports referenced in Table 2.
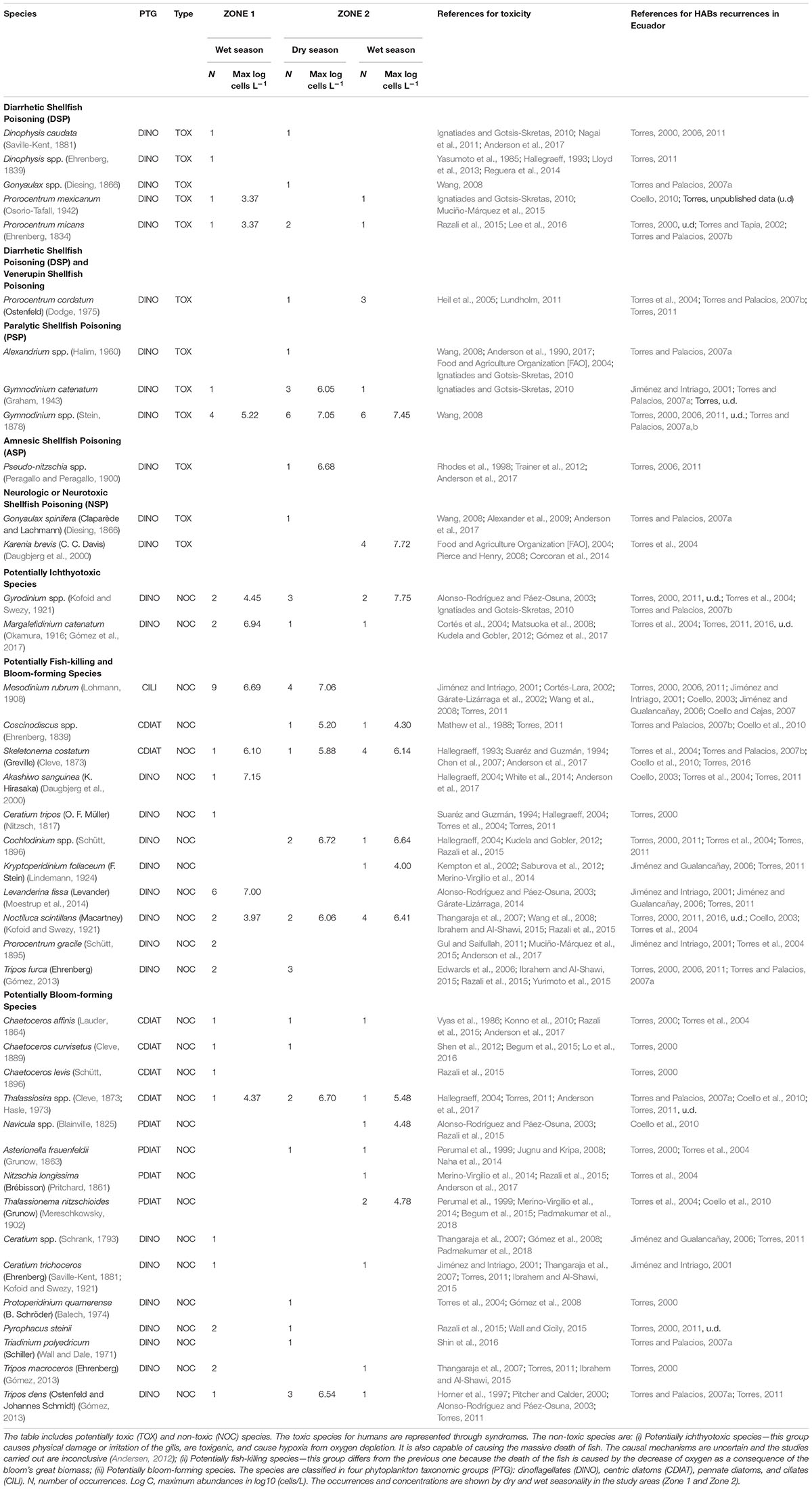
Table 2. List of potentially harmful algal blooms (HABs) recorded in events along the coast of Ecuador (1997–2017).
Some phytoplankton names have been updated according to the records in the World Register of Marine Species (WoRMS2). For the biological analysis performed in INOCAR, samples (a minimal volume of 250 mL of surface water) were collected from the “red tide” events and immediately transported to INOCAR’s laboratories. All surface samples were fixed with Lugol solution until they changed to a light brown color (8–10 drops). The quantitative analysis was carried out using the Utermohl method described in Reguera et al. (2012) and adapted by Torres (2017). Samples were briefly homogenized and placed in a sedimentation chamber (25 mL) for 24 h.
Phytoplankton identification and abundance was assembled up to the genus or species level according to Jiménez (1983); Pesantes (1983), Balech (1988); Taylor et al. (1995), and Reguera et al. (2012) using a Leica (DMIL) inverted microscope. Cell abundance estimations were performed in horizontal transects of the chamber at 400× magnification, with a 15% error margin. Data were converted into cells L-1. These data were further assembled in four phytoplankton taxonomic groups (PTG): dinoflagellates (DINO), centric diatoms (CDIAT), pennate diatoms (PDIAT), and ciliates (CILI), based on the GEOHAB criterion (WoRMS3; Pitcher et al., 2017). The species were categorized as toxic (TOX) or non-toxic (NOC) using the List of Harmful Microalgae (Moestrup et al., 2009).
Data Analysis
Each of four phytoplankton taxonomic groups, as well as the five remote sensing variables, were transformed into categorical variables (two categories: low and high) using the median as a cut-off. In addition, two categorical variables were also included: type of HAB event and season (identifying whether the event occurred during the wet or dry season) (Vila and Masó, 2005). All categorical variables were used for multi-correspondence analysis (MCA) for Zones 1 and 2. This analysis allowed for an investigation of the PTG patterns associated with the oceanographic and atmospheric variables in each zone.
Results
Oceanographic Characteristics Associated With Potential HABs Using Remote Sensing Data
In Zone 1, chlorophyll a showed a unimodal peak at the end of the wet season, in April–May (∼1.5 mg m-3), whereas a peak in April (∼5.0 mg m-3) and a lower sustained value (3.0 mg m-3) in September, during the dry season, were observed in Zone 2 (Figures 2A,B). The levels of chlorophyll a were higher in Zone 2 (2.5–5.0 mg m-3) when compared to Zone 1 (0.5–1.5 mg m-3).
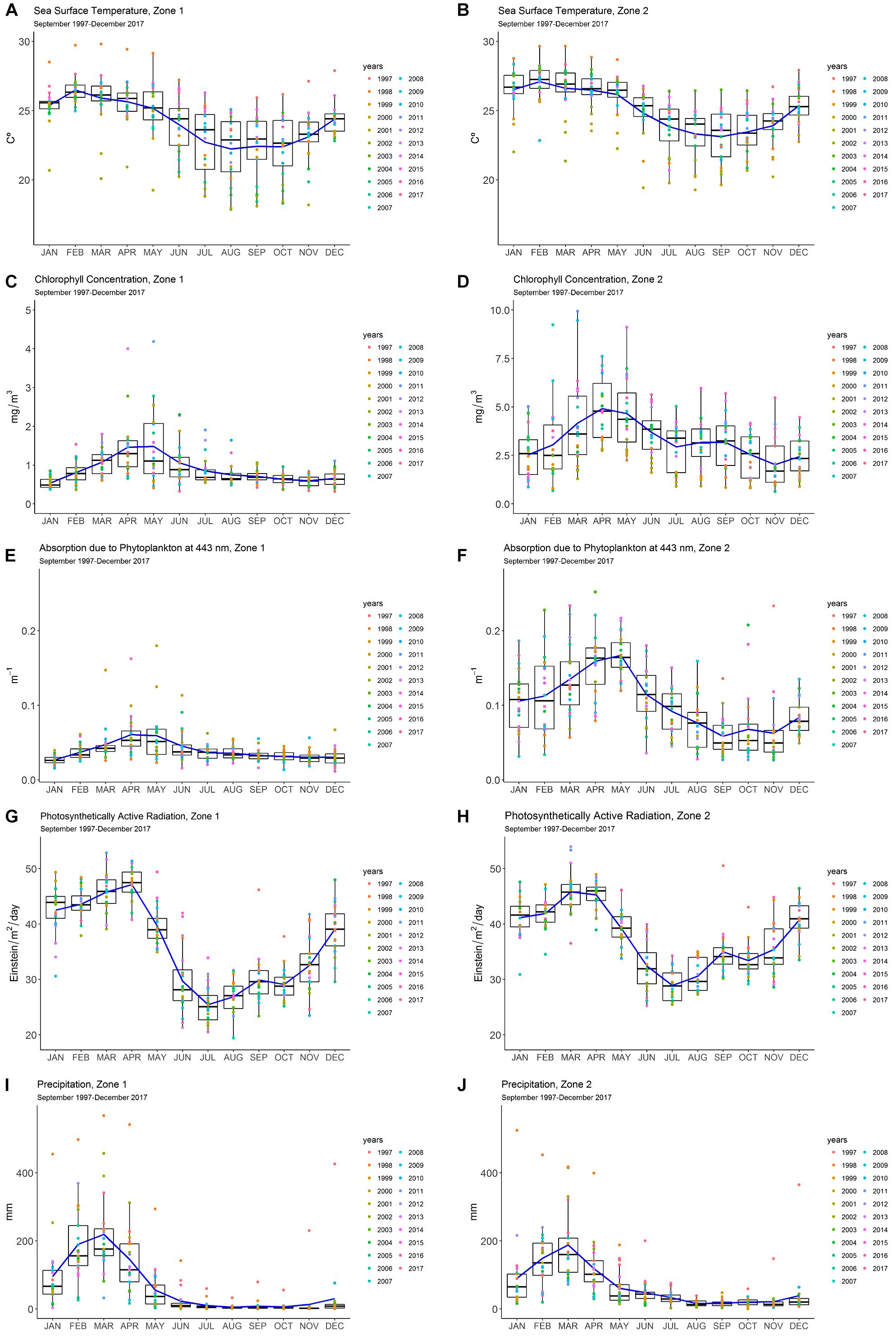
Figure 2. Panel diagram of the monthly means of the remote sensing data of oceanographic variables: (A,B) sea surface temperature (SST); (C,D) chlorophyll a (chl-a); (E,F) absorption due to phytoplankton at 443 nm (aph); (G,H) photosynthetically active radiation (PAR) and atmospheric variable; (I,J) precipitation (PRE) for Zones 1 and 2 on the Ecuadorian coast (1997–2017). Seasonal pattern and inter-annual variability are shown with the blue line and the colored dots, respectively.
A similar pattern was observed in the sea surface temperature (SST) monthly average in both zones, with a peak of 25–26°C in February, during the wet season, and lower values of 22.5–23°C in September (Figures 2C,D). Absorption due to phytoplankton at 443 nm (aph) showed a unimodal pattern, with high values in Zone 1 (peak in April; 0.05 m-1) and the highest value in Zone 2 (April–May; 0.15 m-1) (Figures 2E,F). Photosynthetically active radiation (PAR) presented a bimodal pattern in both zones. Zone 1 saw a maximum value in April (48 Einstein m-2 day-1) and a lower peak in September (30 Einstein m-2 day-1). In Zone 2, the maximum average value was registered in March (48 Einstein m-2 day-1) and a second peak in September (32 Einstein m-2 day-1) (Figures 2G,H). Precipitation showed a similar pattern for both zones, with an average peak in the wet season (March) both for Zone 1 (∼200 mm) and Zone 2 (180 mm) (Figures 2I,J).
Distribution and Seasonality of HAB and Phytoplankton Taxonomic Groups
A detailed checklist of the four PTGs is presented in Table 2 and includes 40 species, 67 HAB occurrences, maximum abundance levels according to category in log (log base 10) of cells L-1, HAB category (NOC or TOX), season (dry and wet), and the respective toxicity and reporting references (See Suplementary Tables S1, S2).
Harmful algal blooms showed strong seasonal characteristics: (a) there were 46 registered occurrences of PTG in Zone 2 during the dry season (Figure 3A; there were no data for Zone 1; see section “Materials and Methods”); and b) 48 (Zone 1) and 40 (Zone 2) PTG occurrences during the wet season (Figure 3B). Regarding the spatial distribution during the dry season, the chl-a ranged from ∼1–1.5 mg m-3 in Zone 1 (from Manta to La Libertad) to ∼2–3 mg m-3 in Zone 2 at the Gulf of Guayaquil, and it varied along with the clusters of PTG (Figure 3A). On the other hand, during the wet season, most of the PTG were DINO, both in Zones 1 and 2. In particular, PTGs coincided with higher chl-a (3–5 mg m-3) in Zone 2 at the Jambelí Channel (Figure 3B).
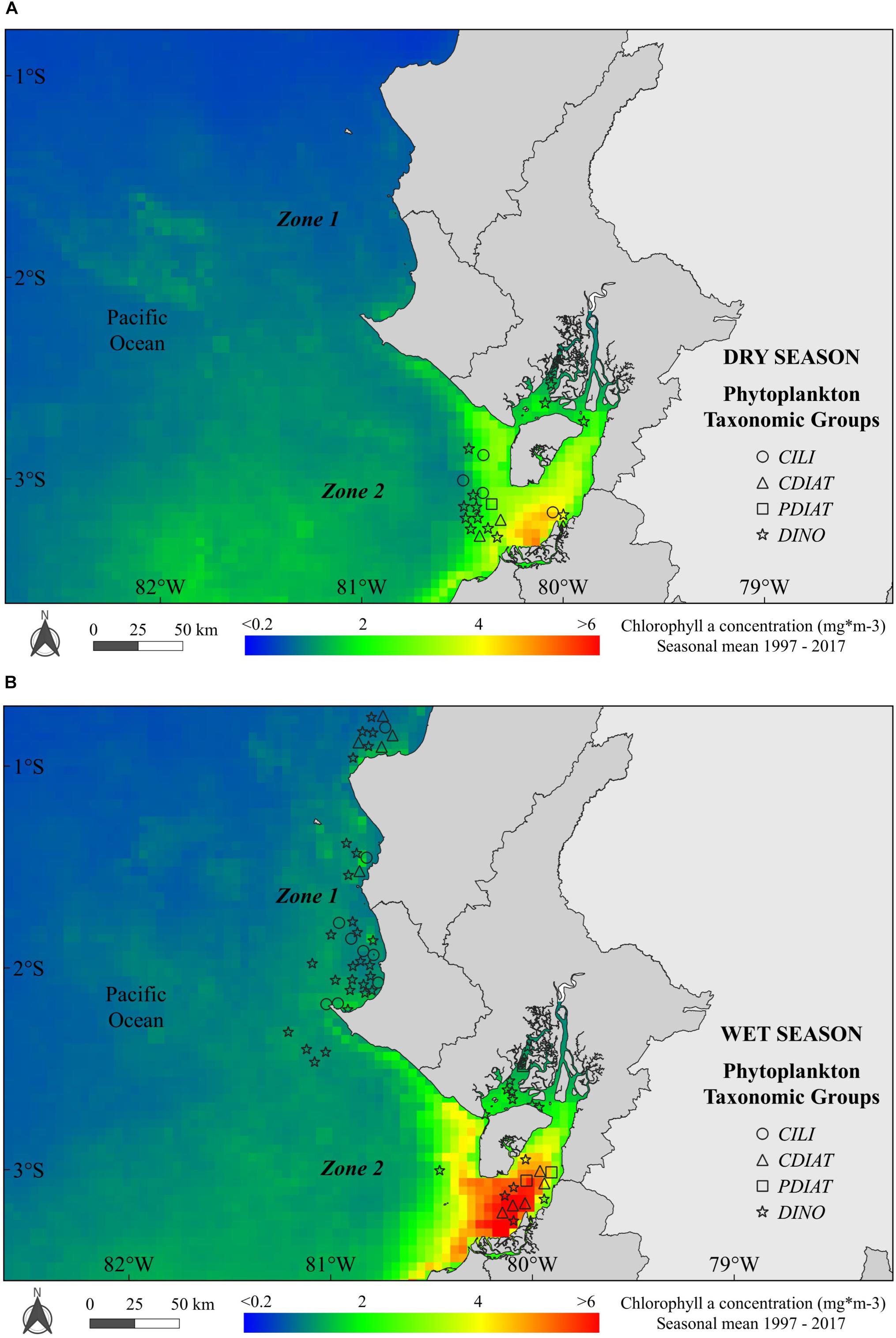
Figure 3. Spatial distribution of occurrences of phytoplankton taxonomic groups (PTGs: ciliates, centric diatoms, pennate diatoms and dinoflagellates) along the Ecuadorian coast during (A) the dry season—occurrences of PTGs and (B) the wet season—occurrences of PTGs in Zones 1 and 2 of the study area. Background colors show the seasonal mean of chlorophyll a for the dry and wet seasons.
During the dry season, in Zone 2, DINO were the most common PTG (72% of occurrences), followed by CDIAT (13%), PDIAT (4%), and CILI (11%) (Figure 4). On the other hand, during the wet season, in both zones DINO reached ∼70%, while CDIAT comprised only 10% in Zone 1 and 18% in Zone 2. PDIAT amounted to 13% in Zone 2 and 19% was CILI, but only in Zone 1 (Figure 4).
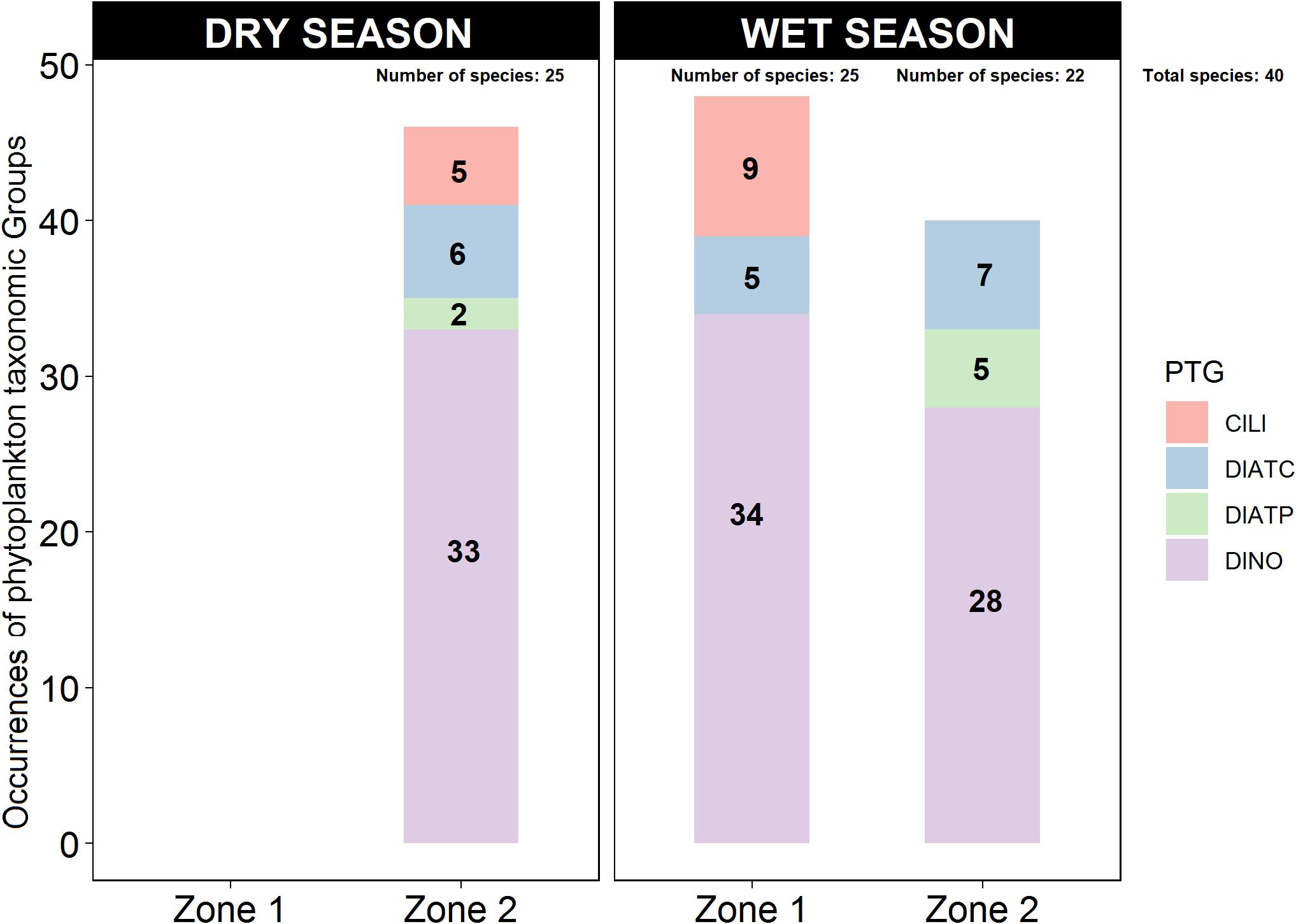
Figure 4. Occurrences of phytoplankton taxonomic groups (PTGs) in Zones 1 and 2 of the study area during the dry and wet seasons. For the period 1997–2017, a total of 46 PTG occurrences with 25 species were reported in the dry season in Zone 2, 48 PTG occurrences with 25 species were reported in Zone 1, and 40 PTG occurrences and 22 species were reported in Zone 2 in the wet season.
Regarding the abundance of PTG, dinoflagellates presented great variability in their abundance, ranging from 3.4 to 7.8 log of cells L-1 during both the dry and wet seasons, reaching a maximum in Zone 2 of the Gulf of Guayaquil. Centric diatoms were higher during the dry season (5.2–6.7 log of cells L-1) than in the wet season (4.3–6 log of cells L-1), reaching a maximum in Zone 2. Pennate diatoms were reported only 4 times in 20 years of data, with a maximum concentration of 6.7 log of cells L-1 (Figure 5).
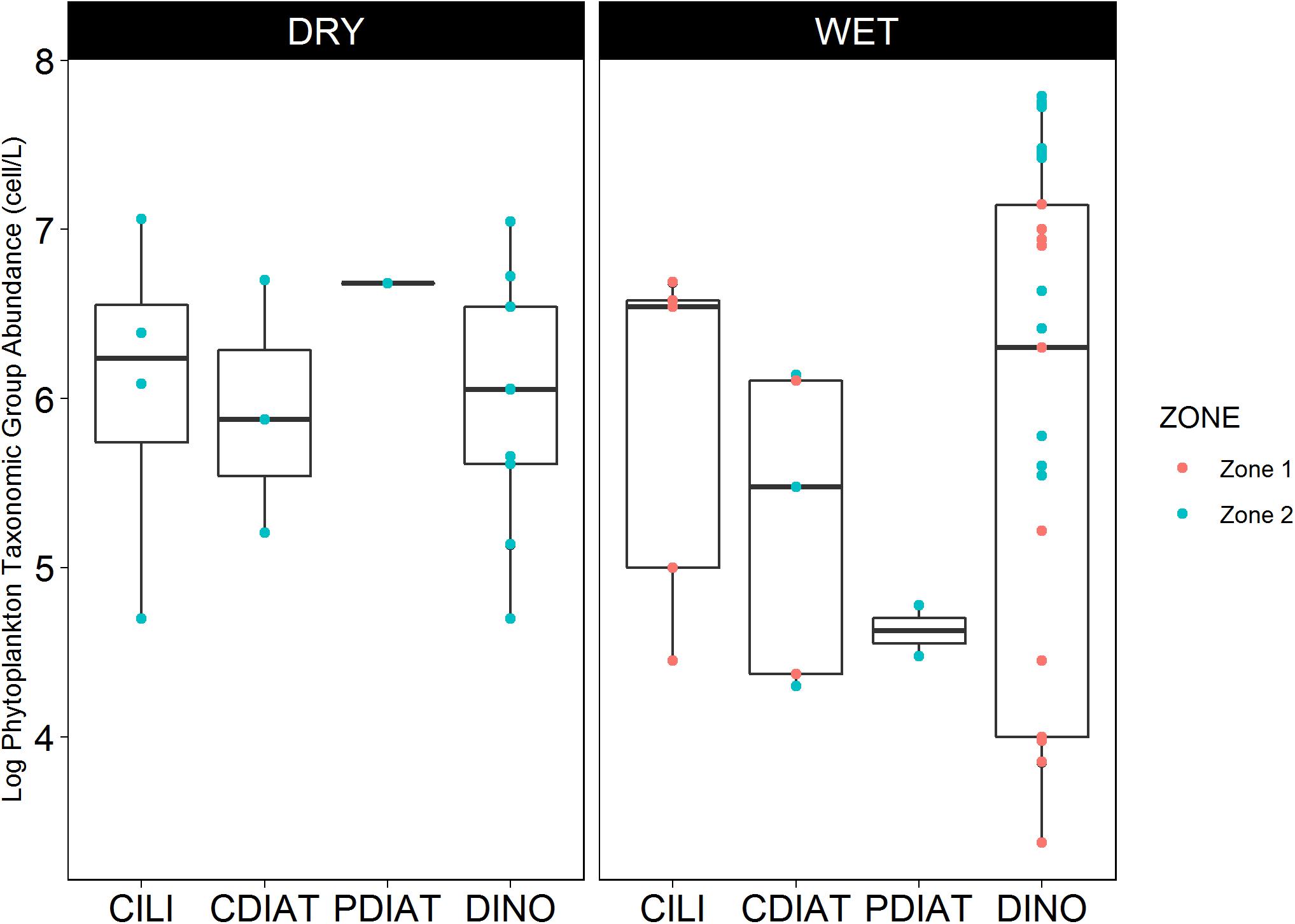
Figure 5. Seasonal abundance of phytoplankton taxonomic groups during HABs events in Zones 1 and 2 of the study area.
Harmful Algal Bloom Species Assembled Along the Ecuadorian Coast
Harmful algal blooms reports from 1997 to 2017 determined a total of 40 species of phytoplankton: 28 were identified as non-toxic, whereas the other 12 are well known to produce toxins (Table 2). The most recurrent genera were Gymnodinium (21 occurrences) and Prorocentrum (10). Other observed genera were Karenia (4), Dinophysis (3), and Pseudo-nitzschia (1). Gymnodinium catenatum (5 occurrences) was observed in the years 2000, 2004, 2008, and 2014 in several locations in Zone 1 and in the Jambeli Channel in Zone 2, with a maximum abundance of 6.05 log of cells L-1. Prorocentrum cordatum (4 occurrences) was observed in 2003 and 2004 in the Jambelí Channel and Estero Salado in Zone 2, but unfortunately without abundantly available data. Prorocentrum mexicanum (2 occurrences) with a maximum abundance of 3.4 log of cells L-1 in 2009 and 2016 was reported in Manta in Zone 1 and in Puerto Bolivar and the Jambelí Channel in Zone 2. Prorocentrum micans (4 occurrences), with a maximum abundance of 3.4 log of cells L-1, was reported in Manta in Zone 1 and in Isla Santa Clara, Puerto Bolivar, in Zone 2. Microphotographs of Lugol-preserved colonies of Gymnodinium catenatum are shown in Figures 6A,B. This sample was found in San Pedro (Zone 1) in March 2018. A potentially harmful, non-toxic Cochlodinium catenatum, a synonym of Margalefidinium catenatum, is shown in Figure 6C. This sample was found in La Libertad in March 2018. However, the HAB-forming dinoflagellate Cochlodinium catenatum needs further samplings/taxonomic studies to ensure a precise identification.
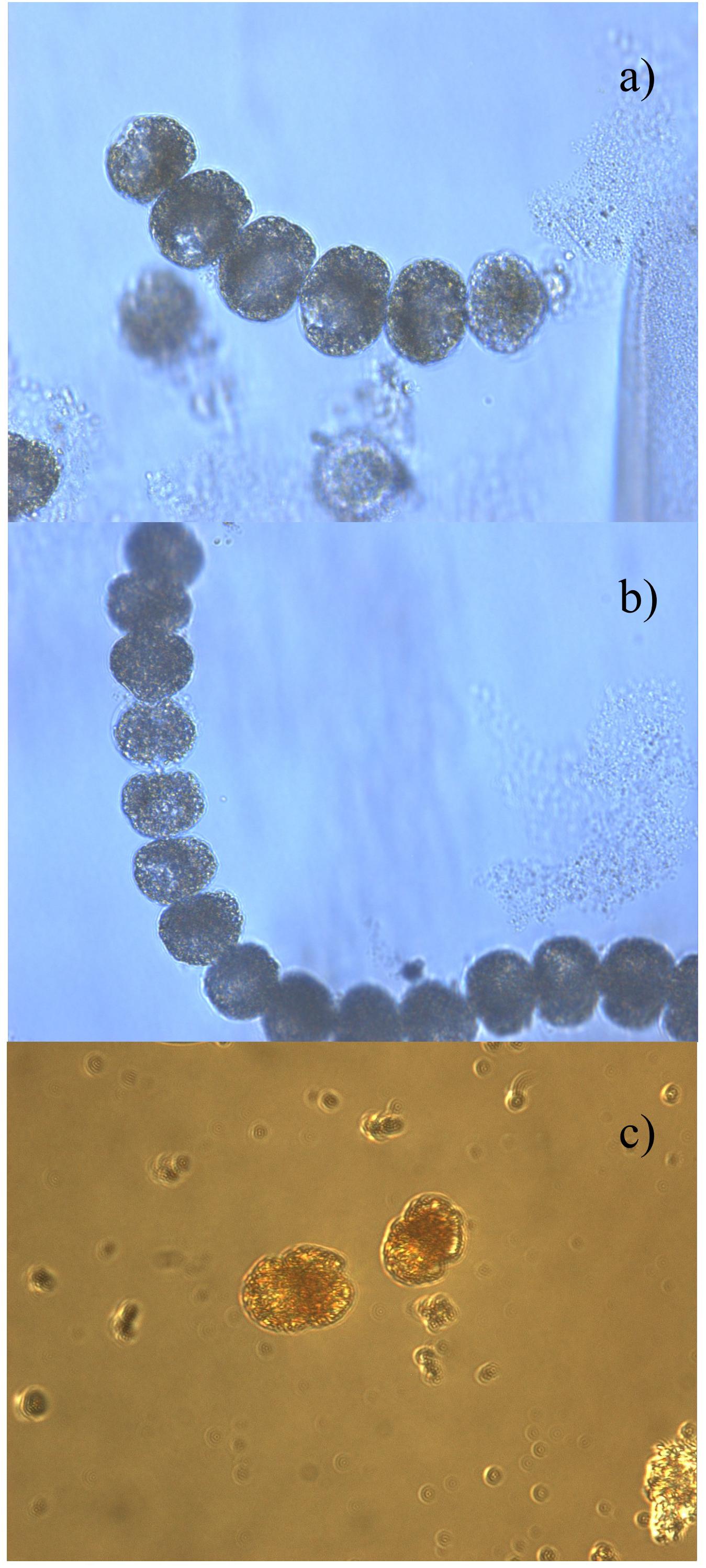
Figure 6. Microphotographs of Lugol-preserved colonies associated with toxic or non-toxic species collected on the Ecuadorian coast: (A,B) Gymnodinium catenatum (Graham, 1943) found in San Pedro (Zone 1) in 2018. This taxon is associated with a paralytic toxin; (C) Cochlodinium catenatum (Schütt, 1896), found in La Libertad in Zone 1 in March 2018.
Other groups of 28 species were categorized as non-toxic algal blooms in order of occurrence: 16 taxa of dinoflagellates, including Noctiluca scintillans, Gyrodinium spp., Levanderina fissa, Tripos dens, and Tripos furca, six centric diatoms, including Skeletonema costatum, Thalassiosira spp., and Chaetoceros affinis, four pennate diatoms, including Asterionella frauenfeldii, Thalassionema nitzschioides, Nitzschia longissima, and Navicula spp., and just one ciliate—Mesodinium rubrum (Table 2). The highest NOC species abundance was represented by Gyrodinium spp., attaining 7.75 log of cells L-1, found in Estero Salado (Zone 2) during 1999’s wet season. The highest abundance of Mesodinium rubrum was 7.06 log of cells L-1, found in Jambeli Channel (Zone 2) during the 2007 dry season. A greater variability in abundance was observed during the wet season for most of the PTG.
Multi-Correspondence Analysis of PTG and Remote Sensing Data
In Zone 1 (Figure 7), the occurrence of toxic HABs corresponded to low abundances of DINO and CDIAT. These biological features seem to be associated with high values of SST, PRE, and PAR and to low chl-a and aph values (443 nm). All these environmental features characterized the wet season (Figure 7). Conversely, non-toxic HABs corresponded to a high abundance of DINO and CILI and to low SST and PRE, but with high aph (Figure 7).
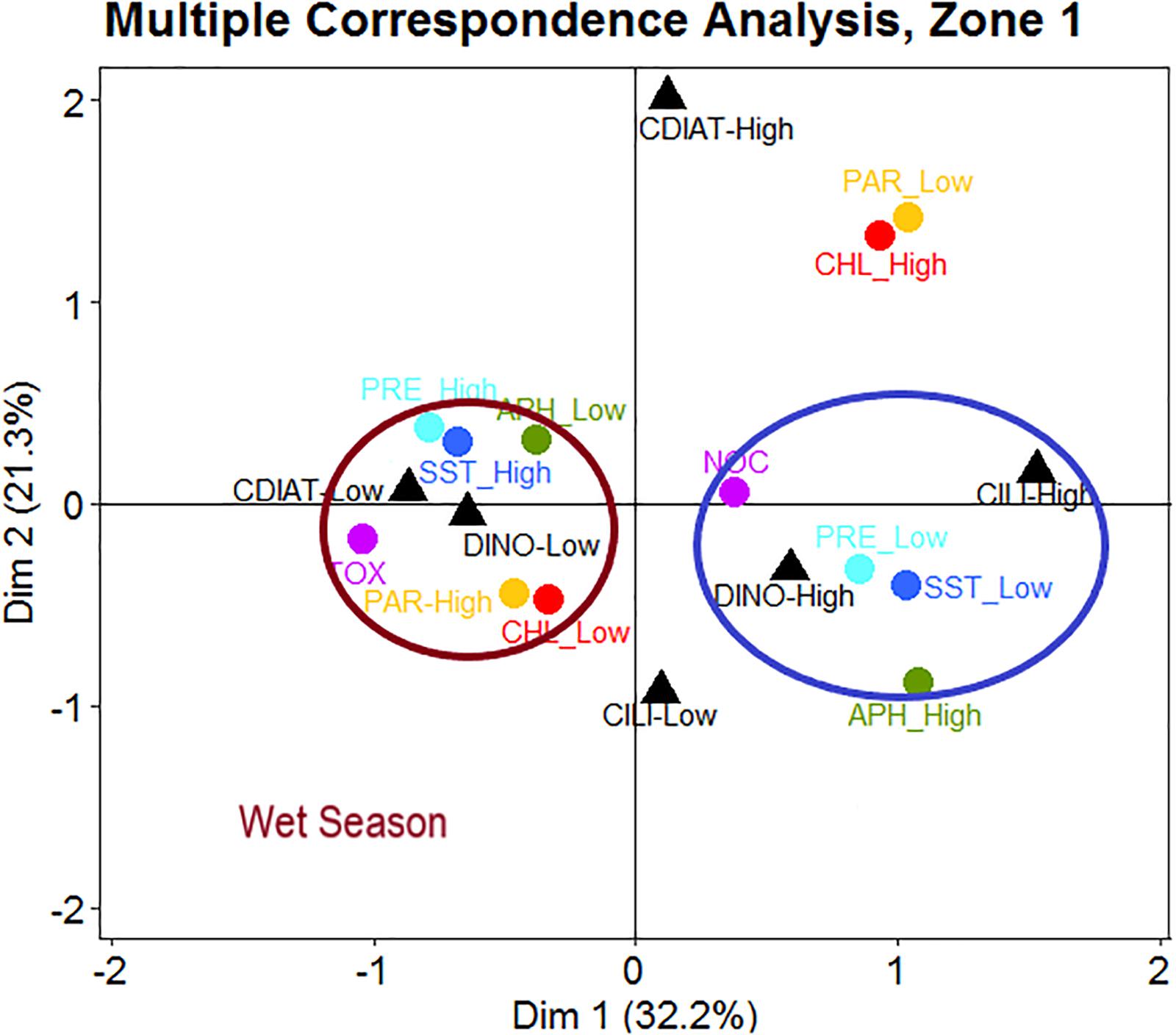
Figure 7. In Zone 1, occurrences of toxic HABs (TOX) linked to low concentrations of dinoflagellates (DINO) and centric diatoms (CDIAT) are associated with high levels of sea surface temperature (SST), precipitation (PRE), and photosynthetic active radiation (PAR) as well as low levels of absorption coefficient of phytoplankton at 443 nm (aph) and chlorophyll a (chl-a) appearing during the wet season. Events of non-toxic HABs (NOC) appearing with high concentrations of DINO and ciliates (CILI) are associated with low levels of SST and PRE, accompanied by high values of aph.
During the wet season in Zone 2 (Figure 8), TOX events corresponded to high biomass levels of DINO, coupled with high SST, PAR, and PRE. However, during the dry season, low PRE and PAR and colder waters were linked to a high abundance of CDIAT. These features are simultaneously related to NOC species.
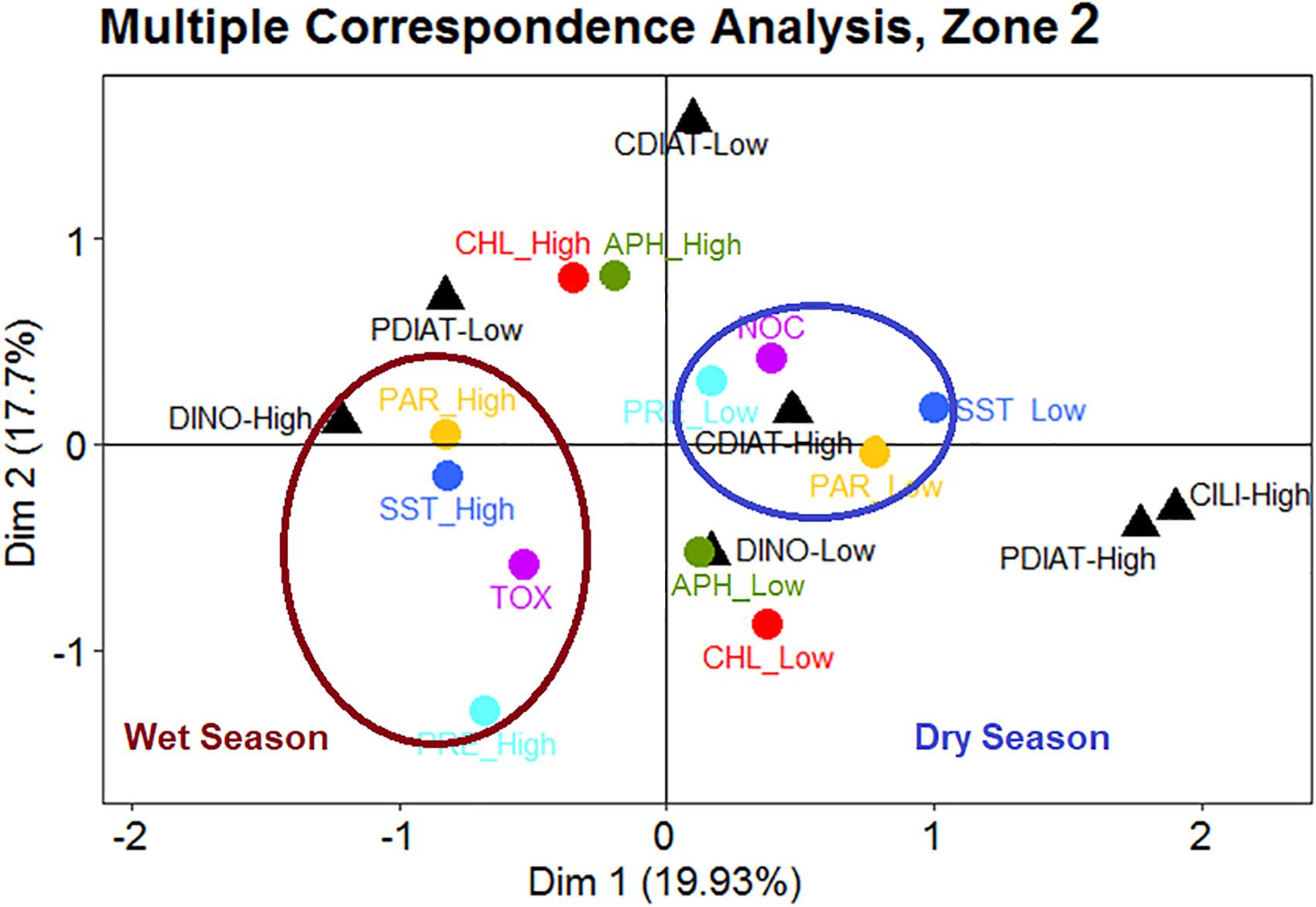
Figure 8. In Zone 2, occurrences of toxic HABs (TOX) are associated with high levels of sea surface temperature (SST), photosynthetic active radiation (PAR), and precipitation (PRE), accompanied by high concentrations of dinoflagellates during the wet season. Events of non-toxic HABs (NOC) seem to be associated with low levels of SST, PRE and PAR and high levels of centric diatoms during the dry season.
Discussion
Oceanography of HABs and Remote Sensing Data
This study confirms that HAB dynamics in the Ecuadorian coastal zone seem to be constrained by the Humboldt upwelling system, high precipitation, and terrestrial nutrients from the Guayas Basin, aligning with other HAB studies in coastal ecosystems and upwelling zones (Kudela et al., 2005; Vila and Masó, 2005; Borbor-Cordova et al., 2006; Anderson et al., 2017; Conde et al., 2018; Oyarzún and Brierley, 2018). In addition, high sea surface temperature during ENSO and ocean heat waves seem to affect all the aforementioned drivers, along with the adaptive strategies of the phytoplankton species and their community assemblage (Masó and Garcés, 2006; Anderson, 2014; Anderson et al., 2017).
The findings of this study reveal that a higher biomass of HABs was found in Zone 2 than in Zone 1 when considering eutrophic and oligotrophic systems, respectively, based on chl-a concentration. Dinoflagellates were the taxonomic group most highly associated with HABs all along the Ecuadorian coast. An interesting finding is that toxic species of DINO appeared either at low or high levels of abundance in Zone 1 or Zone 2, respectively, mostly during the wet season.
These results agree with the findings presented in previous research, demonstrating that dinoflagellate species that form blooms utilize diverse ecological strategies as colonists and r-strategists in nutrient-rich, disturbed environments, or are nutrient stress tolerant in oligotrophic systems (Smayda and Reynolds, 2003; Smayda and Trainer, 2010). Dinoflagellates use a C-strategy (as colonists) to adapt, reaching high levels of biomass and becoming potentially toxic species in nutrient-enriched, high irradiance, estuarine habitats, such as that found in Zone 2. Whereas in the oligotrophic Zone 1, with its highly stratified and high irradiance conditions, a nutrient stress-tolerant ensemble of dinoflagellate species occurred at very low biomass levels (Smayda and Reynolds, 2003; Suparna, 2005; Smayda and Trainer, 2010; Corcoran et al., 2014). For instance, Gymnodinium catenatum and Karenia brevis, considered toxic species, were found at high levels of abundance in Zone 2, mostly in the wet season, while Prorocentrum micans and Prorocentrum mexicanum were reported at low concentrations in the oligotrophic Zone 1, but also in Zone 2, in the wet season.
Considering the bloom behavior of diatoms, previous research found that they occur in coastal and upwelling zones and are annually recurrent and prolonged in duration, with high species diversity (Smayda and Reynolds, 2003). In our work, annual periodicity and high diversity were not evident, with only 7–5 occurrences of CDIAT (6 species) and PDIAT (5 species), respectively, mostly in the Jambeli Channel in Zone 2. Non-toxic HABs occurred with high abundance values of DINO, CILI, and CDIAT and were mostly associated with the cold water from the upwelling system, low precipitation, and irradiance, both in Zones 1 and 2 in environmental conditions more related to the dry season. This work suggests that there is a higher risk of HABs in the Gulf of Guayaquil, at the entrance of the Guayas Estuary (dry season), in the Jambeli Channel and Estero Salado (wet season), and in La Libertad and Manta in the dry season (Figures 2A,B).
However, the question remains as to the biological drivers of the presence of potentially toxic or non-toxic HABs on the Ecuadorian coast (in the eastern Pacific Ocean). It is known that species-specific adaptive strategies within the phytoplankton community enable adaption to specific habitats and climate conditions (e.g., ENSO) (Prado et al., 2015). Adaptive and behavioral adaptation strategies of HABs may include mobility behavior, such as vertical migration, swimming patterns, photo-taxis, and life cycle strategies as resting cysts, and phytoplankton development phases (Masó and Garcés, 2006; Anderson, 2014; Anderson et al., 2017). Future research on these aspects of the adaptive strategies of HABs are necessary for the region.
On the other hand, this study suggests that upwelling systems are related to HABs in the Gulf of Guayaquil. The Humboldt upwelling system is considered to be one of the most productive systems globally (Daneri et al., 2000; Taylor, 2008; Oyarzún and Brierley, 2018). Research groups, such as GEOHAB (Hallegraeff, 2004), have highlighted the potential impacts of HABs on upwelling systems in the context of climate change (Kudela et al., 2005; Edwards et al., 2006; Wei et al., 2008; Trainer et al., 2010; Díaz et al., 2016; Pitcher et al., 2017). In the case of Ecuador and Peru, the Humboldt upwelling system is the foundation of both countries’ fishery productivity and the economic benefits of the fishery industry, which can be impacted by emergent HABs (Pennington et al., 2006; Trainer et al., 2010). Considering that there are limited studies on the upwelling systems in the Gulf of Guayaquil region (Oyarzún and Brierley, 2018), understanding these dynamics is critical to assess the future impacts of a changing climate in this very productive ecosystem.
Harmful Algal Blooms and Human Health
Emergent issues concerning upwelling systems and HABs relate to the occurrence of phytoplankton species able to produce phycotoxins and jeopardize human health through paralytic shellfish poisoning, diarrhetic shellfish poisoning, and amnesic shellfish poisoning, as well as other harmful non-toxic blooms resulting in hypoxic and anoxic environments with potentially high negative impacts on fishery resources (Trainer et al., 2010; Pitcher et al., 2017). Phycotoxins can be transferred to human beings through the ingestion of contaminated seafood, thereby posing a potential risk to human health (Fleming et al., 2001; Alonso-Rodríguez and Páez-Osuna, 2003; Backer et al., 2003; Berdalet et al., 2016). Since the Gulf of Guayaquil and Zone 1 are very important for tourism activities, and are particularly known for their seafood, a HABs monitoring program, by research and academic institutions, and a preventive policy to protect tourism and public health should be developed as a priority.
A recent study on the risk perceptions of coastal managers, public health officers, and coastal communities in Ecuador revealed that there is limited knowledge of the health impacts of “red tides” and HABs (Borbor-Cordova et al., 2018). While there have not been any reports of human beings affected by HAB toxins in Ecuador to date, this may be explained by the lack of awareness of the syndromes they cause (Borbor-Cordova et al., 2018). Gymnodinium catenatum, Prorocentrum cordatum, Prorocentrum mexicanum, Prorocentrum micans, Karenia brevis, Alexandrium spp., Dinophysis caudata, and Pseudo-nitzschia spp. are species found along the Ecuadorian coast that are associated with syndromes related to seafood toxicity. The toxins associated with algal blooms and affected seafood for the aforementioned species are described in Supplementary Table S3. The main vectors of algal toxins to humans are filter-feeding bivalve mollusks and finfish that ingest toxic algae. The main shellfish toxic syndromes include: paralytic shellfish poisoning, associated with Gymnodinium catenatum, diarrhetic shellfish poisoning, associated with Dinophysis spp. and Prorocentrum spp., neurotoxic shellfish poisoning, associated with Karenia brevis, and amnesic shellfish poisoning, linked to Pseudo-nitzschia (Supplementary Table S3). Symptoms depend upon the toxin and can be temporary, chronic, or even lethal (Backer et al., 2003, 2005). A main limitation of this study is that no analysis of toxins was done during the HABs events, thus an important further step would be to implement a toxins analysis in Ecuador.
Finally, HAB research has shown that exotic species of dinoflagellates can be introduced into the environment through ballast water from shipping (Barry et al., 2008). The intense harbor activities carried out within the Gulf of Guayaquil, with its high volume of international traffic, makes it a site of great risk for the introduction of exotic harmful microalgae, which is therefore another important reason to develop a HABs monitoring program in Ecuador.
Management Implications for Monitoring Ecosystem Health on the Ecuadorian Coast
The findings of this study raise many questions about high levels of productivity and HABs, upwelling conditions and their interaction with extreme events, such as El Niño or La Niña, and the associated impact on human health on the Ecuadorian coast. There are many knowledge gaps in terms of how climate ocean drivers, vertical patterns of mixing and stratification, and upwelling nutrients trigger high levels of productivity, as well as HABs. Thus, this study recommends the design of an integrated ecosystem health research program for the Gulf of Guayaquil and the central Ecuadorian coast. This program would attempt to increase knowledge of the drivers of algal growth, analyze the effects of extreme climatic events and upwelling systems on phytoplankton communities, monitor eutrophication indicators, and identify toxins (Hallegraeff, 2010; Anderson et al., 2017). The program would also develop an early warning system for potentially toxic algal blooms and introduced HAB species. Such an ecosystem health research program would be based on a collaborative framework of stakeholders from the scientific community, public health institutions, and marine resources management organizations, such as scientific institutions, health promoters, residents, and the tourism industry—whoever is interested in participating in citizen science (Fleming et al., 2006; Anderson, 2014). Finally, considering the intrinsic relationship between ocean and human health in relation to HABs, it is important to develop policies related to seafood safety, sanitary controls of shellfish, and deploy a fully equipped monitoring system for a biotoxin analysis program in Ecuador.
Final Considerations
Using remote sensing information combined with in situ historical data might help increase understanding of the drivers of high biomass phytoplankton within Ecuadorian coastal sites and indicate when those high biomass events might become toxic, harmful algal blooms. In situ sampling is critical to determine the composition and distribution of phytoplankton taxonomic groups and biotoxins, thus an integrated ecosystem health research program to monitor HABs in the Gulf of Guayaquil needs be developed and implemented in the near future. Upwelling studies linked to the ecology of the HABs in the Gulf of Guayaquil and the Humboldt Current are critical to maintaining the productivity of the coastal zone and managing its estuarine resources.
Author Contributions
MB-C was responsible for the study design, developing an integrated framework, analyzing the data, and drafting the manuscript. GM-S and AC-T pre-processed and analyzed the data. GT collected and analyzed phytoplankton samples and analyzed the data. WR collected samples and reviewed all sections. Together, MB-C, BB, RB, and AC-T drafted the article. All authors discussed the results and reviewed and approved the final manuscript for submission.
Funding
This work was funded by the Escuela Superior Politécnica del Litoral (ESPOL) to the Transdisciplinary Project “Climate Variability and recurrence of harmful algae blooms and their impact on human health along with an estuarine-coastal gradient” (T2-DI-2014) and by the Instituto Oceanografico de la Armada del Ecuador (INOCAR).
Conflict of Interest Statement
The authors declare that the research was conducted in the absence of any commercial or financial relationships that could be construed as a potential conflict of interest.
Supplementary Material
The Supplementary Material for this article can be found online at: https://www.frontiersin.org/articles/10.3389/fmars.2019.00013/full#supplementary-material
Footnotes
References
Alexander, J., Benford, D., Boobis, A., Ceccatelli, S., Cravedi, J., Di Domenico, A., et al. (2009). Marine biotoxins in shellfish – Domoic acid scientific opinion of the Panel on Contaminants in the Food Chain adopted on 2 July 2009. EFSA J. 1181, 1–61. doi: 10.2903/j.efsa.2008.723
Alonso-Rodríguez, R., and Páez-Osuna, F. (2003). Nutrients, phytoplankton and harmful algal blooms in shrimp ponds: a review with special reference to the situation in the Gulf of California. Aquaculture 219, 317–336. doi: 10.1016/S0044-8486(02)00509-4
Andersen, G. N. (2012). Ichthyotoxic Algae and Their Effects on Fish. Ph.D. thesis, University of Copenhagen, Copenhagen.
Anderson, D. (2014). “HABs in a changing world: a perspective on harmful algal blooms, their impacts, and research and management in a dynamic era of climactic and environmental change,” in Proceedings of the 15th International Conference on Harmful Algae: October 29–November 2, 2012, eds H. G. Kim, B. Reguera, G. M. Hallegraeff, and C. K. Lee (Changwon: CECO), 3–17.
Anderson, D. M., Boerlage, S. F. E., and Dixon, M. B. (eds) (2017). Harmful Algal Blooms (HABs) and Desalination: A Guide to Impacts, Monitoring and Management. Paris: Intergovernmental Oceanographic Commission of UNESCO.
Anderson, D. M., Kulis, D. M., Sullivan, J. J., Hall, S., and Lee, C. (1990). Dynamics and physiology of saxitoxin production by the dinoflagellates Alexandrium spp. Mar. Biol. 104, 511–524. doi: 10.1007/BF01314358
Backer, L., Fleming, L. E., Rowan, A., and Baden, D. (2003). “Epidemiology and public health of human illnesses associated with harmful marine phytoplankton,” in UNESCO Manual on Harmful Marine Algae, eds G. M. Hallegraeff, D. M. Anderson, and A. D. Cembella (Geneva: WHO), 725–750.
Backer, L. C., Schurz, R. H., Fleming, L. E., Kirkpatrick, B., and Benson, J. (2005). “Phycotoxins in marine seafood,” in Chemical and Functional Properties of Food Components: Toxins in Food, ed. W. Dabrowski (Boca Raton, FL: CRC Press), 155–190.
Balech, E. (1974). El Género “Protoperidinium” Bergh. 1881 (“Peridinium” Ehrenberg, 1831, Partim). Rev. Museo Argentino Cienc. Nat. Inst. Nac. Inv. Cienc. Nat. 4, 1–79.
Barry, S. C., Hayes, K. R., Hewitt, C. L., Behrens, H. L., Dragsund, E., and Bakke, S. M. (2008). Ballast water risk assessment: principles, processes, and methods. ICES J. Mar. Sci. 65, 121–131. doi: 10.1093/icesjms/fsn004
Begum, M., Sahu, B. K., Das, A. K., Vinithkumar, N. V., and Kirubagaran, R. (2015). Extensive Chaetoceros curvisetus bloom in relation to water quality in Port Blair Bay, Andaman Islands. Environ. Monit. Assess. 187, 1–14. doi: 10.1007/s10661-015-4461-2
Bendix, J., Trachte, K., Palacios, E., Rollenbeck, R., Göttlicher, D., Nauss, T., et al. (2011). El Niño meets La Nina—anomalous rainfall patterns in the “traditional” El Niño region of Southern Ecuador. Erdkunde 65, 151–167. doi: 10.3112/erdkunde.2011.02.04
Berdalet, E., Fleming, L. E., Gowen, R., Davidson, K., Hess, P., Backer, L. C., et al. (2016). Marine harmful algal blooms, human health and wellbeing: challenges and opportunities in the 21st century. J. Mar. Biol. Assoc. U.K. 1, 61–91. doi: 10.1017/S0025315415001733
Blondeau-Patissier, D., Gower, J., Dekker, A., Phinn, S., and Brando, V. (2014). A review of ocean color remote sensing methods and statistical techniques for the detection, mapping and analysis of phytoplankton blooms in coastal and open oceans. Prog. Oceanogr. 123, 123–144. doi: 10.1016/j.pocean.2013.12.008
Borbor-Cordova, M., Boyer, E., Mcdowell, W., and Hall, C. (2006). “Nitrogen and phosphorus budgets for a tropical watershed impacted by agricultural land use: Guayas, Ecuador,” in Nitrogen Cycling in the Americas: Natural and Anthropogenic Influences and Controls SE – 7, eds L. Martinelli and R. Howarth (Dordrecht: Springer), 135–161. doi: 10.1007/978-1-4020-5517-1_7
Borbor-Cordova, M. J., Pozo-Cajas, M., Cedeno-Montesdeoca, A., Mantilla Saltos, G., Kislik, C., Espinoza-Celi, M. E., et al. (2018). Risk perception of coastal communities and authorities on harmful algal blooms in Ecuador. Front. Mar. Sci. 5:365. doi: 10.3389/fmars.2018.00365
Casey, K. S., Brandon, T. B., Cornillon, P., and Evans, R. (2010). “The past, present, and future of the AVHRR pathfinder SST program,” in Oceanography from Space, eds V. Barale, J. Gower, and L. Alberotanza (Dordrecht: Springer), 273–287. doi: 10.1007/978-90-481-8681-5_16
Chavez, F. P., Ryan, J., Lluch-Cota, S. E., and Miguel, N. (2003). From anchovies to sardines and back: multidecadal change in the Pacific Ocean. Science 299, 217–221. doi: 10.1126/science.1075880
Chen, G. F., Wang, G. C., Zhang, B. Y., and Fan, X. L. (2007). Morphological and phylogenetic analysis of Skeletonema costatum-like diatoms (Bacillariophyta) from the China Sea. Eur. J. Phycol. 42, 163–175. doi: 10.1080/09670260601149784
Cleve, P. T. (1873). Examintaion of diatoms found on the surface of the sea of Java. Bihang Till K. Svenska Vet. Akad. Handlingar. 11, 1–17.
Cleve, P. T. (1889). “Pelagisk Diatomeer från Kattegat,” in Det Videnskabelige Udbytte Af Kanonbaaden “Hauchs” Togter I de Danske Have Indefor Skagen, I. Aarene 1883-86, ed. C. G. J. Petersen (Kjøbenhavn: Andr. Fred. Høst & Sons Forlag), 53–56.
Coello, D. (2003). “Mareas rojas en el Ecuador”, in Proceedings of the Informe del Instituto Nacional de Pesca en Sexto Taller Regional de Planificación Científica sobre Floraciones de Algas Nocivas en Sudamérica, Guayaquil.
Coello, D. (2010). Marea Roja ocasionada por Scripsiella trochoidea en el Estuario del río Chone (mayo 2007). Rev. Ciencias Del Mar Limnol. 4, 93–10.
Coello, D., and Cajas, J. (2007). Plancton del Crucero Demersal. T07/06/02, junio de 2007. Reporte Interno. Instituto Nacional de Pesca, Guayaquil.
Coello, D., Cajas, J., Macías, P., and Lindao, J. (2010). Floración algal ocasionada por Prorocentrum mexicanum en el canal de Jambelí. Rev. Ciencias Del Mar Limnol. 4, 25–33.
Conde, A., Hurtado, M., and Prado, M. (2018). Phytoplankton response to a weak El Niño event. Ecol. Indic. 95, 394–404. doi: 10.1016/j.ecolind.2018.07.064
Conde, A., and Prado, M. (2018). Changes in phytoplankton vertical distribution during an El Niño event. Ecol. Indic. 90, 201–205. doi: 10.1016/j.ecolind.2018.03.015
Corcoran, A. A., Richardson, B., and Flewelling, L. J. (2014). Effects of nutrient-limiting supply ratios on toxin content of Karenia brevis grown in continuous culture. Harmful Algae 39, 334–341. doi: 10.1016/j.hal.2014.08.009
Cortés, C., Cortés, R., and Sierra, A. (2004). Presencia de Cochlodinium catenatum (Gymnodiniales: Gymnodiniaceae) en mareas rojas de Bahía de Banderas, Pacífico mexicano. Biol. Trop. 52, 35–49.
Cortés-Lara, M. C. (2002). Primer registro de marea roja del 2002. Bahía de Banderas Jalisco- Nayarit (Enero de 2002). Revista Biomédica 13, 229–230. doi: 10.32776/revbiomed.v13i3.321
Cucalon, E. (1989). “Oceanographic characteristics off the coast of Ecuador,” in A Sustainable Shrimp Mariculture Industry for Ecuador, eds S. Olsen and L. Arriaga (Narragansett, RI: Coastal Resources Center), 183–196.
Daneri, G., Dellarossa, V., Quiñones, R., Jacob, B., Montero, P., and Ulloa, O. (2000). Primary production and community respiration in the Humboldt current system off Chile and associated oceanic areas. Mar. Ecol. Prog. Ser. 197, 41–49. doi: 10.3354/meps197041
Daugbjerg, N., Hansen, G., Larsen, J., and Moestrup,Ø., (2000). Phylogeny of some of the major genera of dinoflagellates based on ultrastructure and partial LSU rDNA sequence data, including the erection of three new genera of unarmoured dinoflagellates. Phycologia 39, 302–317. doi: 10.2216/i0031-8884-39-4-302.1
Davidson, K., Gowen, R. J., Harrison, P. J., Fleming, L. E., Hoagland, P., and Moschonas, G. (2014). Anthropogenic nutrients and harmful algae in coastal waters. J. Environ. Manage. 146, 206–216. doi: 10.1016/j.jenvman.2014.07.002
Díaz, P. A., Reguera, B., Ruiz-Villarreal, M., Pazos, Y., Velo-Suárez, L., Berger, H., et al. (2013). Climate variability and oceanographic settings associated with interannual variability in the initiation of Dinophysis acuminata blooms. Mar. Drugs 11, 2964–2981. doi: 10.3390/md11082964
Díaz, P. A., Ruiz-Villarreal, M., Pazos, Y., Moita, T., and Reguera, B. (2016). Climate variability and Dinophysis acuta blooms in an upwelling system. Harmful Algae 53, 145–159. doi: 10.1016/j.hal.2015.11.007
Diesing, K. M. (1866). Revision der Prothelminthen. Abtheilung: Mastigophoren. Sitzungsberichte Der Kaiserlichen Akademie Der Wissenschaften. Mathematisch-Naturwissenschaftliche Classe. Abt. 1 Mineral. Botanik Zool. Anat. Geol. Paläontologie, 52, 287–401.
Dodge, J. D. (1975). The Prorocentrales (Dinophyceae). II. Revision of the taxonomy within the genus Prorocentrum. Bot. J. Linn. Soc. 71, 103–125. doi: 10.1109/ICTD.2009.5426693
Edwards, M., Johns, D. G., Richardson, A. J., Leterme, S. C., Svendsen, E., and Richardson, A. J. (2006). Regional climate change and harmful algal blooms in the northeast Atlantic. Limnol. Oceanogr. 51, 820–829. doi: 10.4319/lo.2006.51.2.0820
Ehrenberg, C. G. (1834). Dritter Beitrag Zur Erkenntniss Grosser Organisation in Der Richtung Des Kleinsten Raumes. Abh. Königl. Akad. Wiss. Berlin 1833, 145–336.
Ehrenberg, C. G. (1839). Über die Bildung der Kreidefelsen und des Kreidemergels durch unsichtbare Organismen. Abh. Königl. Akad. Wiss. Berlin 1838,59–147.
Fleming, L. E., Bean, J. A., Katz, D., and Hammond, R. (2001). “The epidemiology of seafood poisoning,” in Seafood and Environmental Toxins, eds Y. H. Hui, D. D. Kits, and P. S. Stanfield (Amsterdam: Marcel Dekker), 287–310.
Fleming, L. E., Broad, K., Clement, A., Dewailly, E., Elmira, S., Knap, A., et al. (2006). Oceans and human health: emerging public health risks in the marine environment. Mar. Pollut. Bull. 53, 545–560. doi: 10.1016/j.marpolbul.2006.08.012
Gárate-Lizárraga, I. (2014). Proliferation of Levanderina fissa and Polykrikos hartmannii (Dinophyceae: Gymnodiniales) in Bahia de la Paz, Gulf of California, Mexico. CICIMAR Oceán. 29, 25–35.
Gárate-Lizárraga, I., Band, C., Cervantes, R., and Escobedo, D. (2002). Mareas rojas de Mesodinium rubrum (Lohmann) Hamburger y Buddenbrock en el Golfo de California. Hidrobiológica 12, 15–20.
Gómez, F. (2013). Reinstatement of the dinoflagellate genus Tripos to replace Neoceratium, marine species of Ceratium(Dinophyceae, Alveolata). CICIMAR Oceán. 28, 1–22.
Gómez, F., Claustre, H., and Souissi, S. (2008). Rarely reported dinoflagellates of the genera Ceratium, Gloeodinium, Histioneis, Oxytoxum and Prorocentrum (Dinophyceae) from the open southeast Pacific Ocean. Rev. Biol. Mar. Oceanogr. 43, 25–40. doi: 10.4067/S0718-19572008000100004
Gómez, F., Richlen, M. L., and Anderson, D. M. (2017). Molecular characterization and morphology of Cochlodinium strangulatum, the type species of Cochlodinium, and Margalefidinium gen. nov. for C. polykrikoides and allied species (Gymnodiniales, Dinophyceae). Harmful Algae 63, 32–44. doi: 10.1016/j.hal.2017.01.008
Graham, H. W. (1943). Gymnodinium catenatum, a new dinoflagellate from the Gulf of California. Trans. Am. Microsc. Soc. 62, 259–261. doi: 10.2307/3223028
Grunow, A. (1863). Über einige neue und ungenugend bekkante Arten und Gattungen von Diatomaceen. Verh. Kaiserlich Königlichen Zool. Bot. Ges. Wien 13, 137–162.
Gul, S., and Saifullah, S. M. (2011). The dinoflagellate genus Prorocentrum (Prorocentrales, Prorocentraceae) from the North Arabian Sea. Pak. J. Bot. 43, 3061–3065.
Halim, Y. (1960). Alexandrium minutum, n. gen. n. sp. dinoflagellé provocant des “eaux rouges”. Vie Milieu 11, 102–105.
Hallegraeff, G. M. (1993). A review of harmful algal blooms and their apparent global increase. Phycologia 32, 79–99. doi: 10.2216/i0031-8884-32-2-79.1
Hallegraeff, G. M. (2004). “Harmful algal blooms: a global overview,” in Manual on Harmful Marine Microalgae (UNESCO), eds G. M. Hallegraeff, D. Anderson, and A. Cembella (Paris: IOC-UNESCO), 1–45. doi: 10.1016/B978-0-12-391499-6.00008-6
Hallegraeff, G. M. (2010). Ocean climate change, phytoplankton community responses, and harmful algal blooms: a formidable predictive challenge. J. Phycol. 46, 220–235. doi: 10.1111/j.1529-8817.2010.00815.x
Heil, C. A., Glibert, P. M., and Fan, C. (2005). Prorocentrum minimum (Pavillard) Schiller: a review of a harmful algal bloom species of growing worldwide importance. Harmful Algae 4, 449–470. doi: 10.1016/j.hal.2004.08.003
Horner, R. A., Garrison, D. L., and Plumley, F. G. (1997). Harmful algal blooms and red tide problems on the U.S. west coast. Limnol. Oceanogr. 42, 1076–1088. doi: 10.4319/lo.1997.42.5_part_2.1076
Ibrahem, H., and Al-Shawi, I. (2015). Potential harmful algae of Iraqi coastal marine waters. Int. J. Mar. Sci. 5, 1–6. doi: 10.5376/ijms.2015.05.0060
Ignatiades, L., and Gotsis-Skretas, O. (2010). A review on toxic and harmful algae in Greek coastal waters (E. Mediterranean Sea). Toxins 2, 1019–1037. doi: 10.3390/toxins2051019
Jiménez, R. (1983). Diatomeas y dinoflagelados del fitoplancton del Golfo de Guayaquil. Acta Oceanogr. Pac. 2, 1993–1282.
Jiménez, R., and Gualancañay, E. (2006). Floraciones de Mesodinium rubrum en los procesos de surgencia en el Pacífico Ecuatorial. Acta Oceanogr. Pac. 13, 65–72.
Jugnu, R., and Kripa, V. (2008). Prevalence of non toxic and harmful algal blooms along Kerala coast. Mar. Fish. Inform. Serv. 195, 3–5.
Kempton, J. W., Wolny, J., Tengs, T., Rizzo, P., Morris, R., Tunnell, J., et al. (2002). Kryptoperidinium foliaceum blooms in South Carolina: a multi-analytical approach to identification. Harmful Algae 1, 383–392. doi: 10.1016/S1568-9883(02)00051-3
Kofoid, C. A., and Swezy, O. (1921). The Free-Living Unarmored Dinoflagellata. Berkeley, CA: University of California Press.
Konno, S., Inoue, N., Hernández-Becerril, D. U., and Jordan, R. W. (2010). Chaetoceros affinis blooms in palauan meromictic marine lakes. Vie Milieu 60, 257–264.
Kudela, R., Pitcher, G., Probyn, T., Figueiras, F., Moita, T., and Trainer, V. (2005). Harmful algal blooms in coastal upwelling systems. Oceanography 18, 184–197. doi: 10.5670/oceanog.2005.53
Kudela, R. M., and Gobler, C. J. (2012). Harmful dinoflagellate blooms caused by Cochlodinium sp.: Global expansion and ecological strategies facilitating bloom formation. Harmful Algae 14, 71–86. doi: 10.1016/j.hal.2011.10.015
Lauder, H. S. (1864). Remarks on the marine Diatomaceae found at Hong Kong, with descriptions of new species. Trans. Microsc. Soc. Lond. New Ser. 12, 75–79.
Lee, K.-W., Yeh, S.-W., Kug, J.-S., and Park, J.-Y. (2014). Ocean chlorophyll response to two types of El Niño events in an ocean-biogeochemical coupled model. J. Geophys. Res. 119, 933–952. doi: 10.1002/2013JC009050
Lee, T. C. H., Fong, F. L. Y., Ho, K. C., and Lee, F. W. F. (2016). The mechanism of diarrhetic shellfish poisoning toxin production in Prorocentrum spp.: physiological and molecular perspectives. Toxins 8, 2–23. doi: 10.3390/toxins8100272
Lindemann, E. (1924). Der Bau der Hülle bei Heterocapsa und Kryptoperidinium foliaceum (Stein) n. nom. (Zugleich eine vorläufige Mitteilund). Bot. Arch. 5, 114–120.
Lloyd, J. K., Duchin, J. S., Borchert, J., Quintana, H. F., and Robertson, A. (2013). Diarrhetic shellfish poisoning, Washington, USA, 2011. Emerg. Infect. Dis. 19, 1314–1316. doi: 10.3201/eid1908.121824
Lo, W.-T., Hsu, P.-K., Fang, T.-H., Hu, J.-H., and Hsieh, H.-Y. (2016). Phytoplankton communities impacted by thermal effluents off two coastal nuclear power plants in subtropical areas of northern taiwan. Terr. Atmos. Ocean. Sci. 27, 107–120. doi: 10.3319/TAO.2015.09.24.01
Lohmann, H. (1908). Untersuchungen zur Feststellung des vollständigen Gehaltes des Meeres an Plankton. Wiss. Meeresunters. N. F. Abt. Kiel 10,129–370.
Lundholm, N. (2011). “Bacillariophyta,” in Taxonomic Reference List of Harmful Micro Algae, ed. N. Lundholm (Paris: IOC-UNESCO).
Masó, M., and Garcés, E. (2006). Harmful microalgae blooms (HAB): problematic and conditions that induce them. Mar. Pollut. Bull. 53, 620–630. doi: 10.1016/j.marpolbul.2006.08.006
Mathew, K. J., Thomas, P. A., George, R. M., Girijavallabhan, K. G., Siraimeetan, P., Naomi, T. S., et al. (1988). Plankton blooms along the Indian coasts, some highlights. Mar. Fish. Inf. Serv. Tech. Ext. Ser. 84, 11–13.
Matsuoka, K., Iwataki, M., and Kawami, H. (2008). Morphology and taxonomy of chain-forming species of the genus Cochlodinium (Dinophyceae). Harmful Algae 7, 261–270. doi: 10.1016/j.hal.2007.12.002
McClain, C. R., Feldman, G. C., and Hooker, S. (2004). An overview of the SeaWiFS project and strategies for producing a climate research quality global ocean bio-optical time series. Deep Sea Res.Part II 51, 5–42. doi: 10.1016/j.dsr2.2003.11.001
McKibben, S. M., Watkins-Brandt, K. S., Wood, A. M., Hunter, M., Forster, Z., Hopkins, A., et al. (2015). Monitoring Oregon coastal harmful algae: observations and implications of a harmful algal bloom-monitoring project. Harmful Algae 50, 32–44. doi: 10.1016/j.hal.2015.10.004
Mélin, F., and Hoepffner, N. (2011). “Case Study 6 - Monitoring phytoplankton productivity from satellite: an aid to marine resources management,” in Handbook of Satellite Remote Sensing Image Interpretation: Applications for Marine Living Resources Conservation and Management, eds J. Morales, V. Stuart, T. Platt, and S. Sathyendranath (Dartmouth: EU PRESPO and IOCCG), 79–93.
Merino-Virgilio, F. C., Okolodkov, Y. B., Aguilar-Trujillo, A. C., Osorio-Moreno, I., and Herrera-Silveira, J. A. (2014). “Florecimientos algales nocivos en las aguas costeras del norte de Yucatán (2001-2013),” in Golfo de México. Contaminación e Impacto Ambiental: Diagnóstico y Tendencias, eds A. V. Botello, J. Rendón von Osten, J. A. Benítez, and G. Gold-Bouchot (Mérida: CINVESTAV- Unidad Mérida).
Miller, P., Shutler, J., Moore, G., and Groom, S. (2006). SeaWiFS discrimination of harmful algal bloom evolution. Int. J. Remote Sens. 27, 2287–2301. doi: 10.1080/01431160500396816
Moestrup,Ø., Akselmann, R., Fraga, S., Hoppenrath, M., Iwataki, M., Komárek, J., et al. (2009). IOC-UNESCO Taxonomic Reference List of 427 Harmful Micro Algae. Available at: http://www.marinespecies.org/hab/
Moestrup,Ø., Hakanen, P., Hansen, G., Daugbjerg, N., and Ellegaard, M. (2014). On Levanderina fissa gen. & comb. nov. (Dinophyceae) (syn. Gymnodinium fissum, Gyrodinium instriatum, Gyr. uncatenum), a dinoflagellate with a very unusual sulcus. Phycologia 53, 265–292. doi: 10.2216/13-254.1
Moisan, J. R., Moisan, T. A. H., and Linkswiler, M. A. (2011). An inverse modeling approach to estimating phytoplankton pigment concentrations from phytoplankton absorption spectra. J. Geophys. Res. 116, 0148–0227. doi: 10.1029/2010JC006786
Moore, S. K., Trainer, V. L., Mantua, N. J., Parker, M. S., Laws, E. A., and Backer, L. C. (2008). Impacts of climate variability and future climate change on harmful algal blooms and human health. Environ. Health 7(Suppl. 2):S4. doi: 10.1186/1476-069X-7-S2-S4
Muciño-Márquez, R. E., Gárate-Lizárraga, I., and López-Cortés, D. J. (2015). Variación estacional del género Prorocentrum (Dinophyceae) en dos granjas atuneras en la Bahía de La Paz México. Acta Biol. Colomb. 20, 195–206. doi: 10.15446/abc.v20n1.42442
Nagai, S., Suzuki, T., Nishikawa, T., and Kamiyama, T. (2011). Differences in the production and excretion kinetics of okadaic acid, dinophysistoxin-1, and pectenotoxin-2 between cultures of Dinophysis acuminata and Dinophysis fortii isolated from western Japan. J. Phycol. 2011, 1326–1337. doi: 10.1111/j.1529-8817.2011.01076.x
Naha, S., Rakshit, D., Kumar, S., and Kumar, R. (2014). Impact of multispecies diatom bloom on plankton community structure in Sundarban mangrove wetland, India. Mar. Pollut. Bull. 85, 306–311. doi: 10.1016/j.marpolbul.2014.04.015
Nair, A., Sathyendranath, S., Platt, T., Morales, J., Stuart, V., Forget, M., et al. (2008). Remote sensing of phytoplankton functional types. Remote Sens. Environ. 112, 3366–3375. doi: 10.1016/j.rse.2008.01.021
Nitzsch, C. L. (1817). Beitrag zur Infusorienkunde oder Naturbeschreibung der Zerkarien und Bazillarien. Neue Schrift. Naturforsch. Ges. Halle 3, 1–128.
NASA Ocean Biology Processing Group (2017). MODIS-Aqua Level 3 Mapped Chlorophyll Data Version R2018.0 [Data Set]. Greenbelt, MD: NASA Ocean Biology DAAC. doi: 10.5067/aqua/modis/l3m/chl/2018
NASA Ocean Biology Processing Group (2018a). MODIS-Terra Level 3 Mapped Absorption Due to Phytoplankton at 443 nm Data Version R2018.0 [Data Set]. Greenbelt, MD: NASA Ocean Biology DAAC.
NASA Ocean Biology Processing Group (2018b). MODIS-Terra Level 3 Mapped Photosynthetically Available Radiation Data Version R2018.0 [Data Set]. Greenbelt, MD: NASA Ocean Biology DAAC. doi: 10.5067/orbview-2/seawifs/l3m/par/2018
NASA Ocean Biology Processing Group (2018c). SEAWIFS-ORBVIEW-2 Level 3 Mapped Absorption Due to Phytoplankton at 443 nm Data Version R2018.0 [Data Set]. Greenbelt, MD: NASA Ocean Biology DAAC.
NASA Ocean Biology Processing Group (2018d). SEAWIFS-ORBVIEW-2 Level 3 Mapped Chlorophyll Data Version R2018.0 [Data Set]. Greenbelt, MD: NASA Ocean Biology DAAC. doi: 10.5067/orbview-2/seawifs/l3m/chl/2018
NASA Ocean Biology Processing Group (2018e). SEAWIFS-ORBVIEW-2 Level 3 Mapped Photosynthetically Available Radiation Data Version R2018.0 [Data Set]. Greenbelt, MD: NASA Ocean Biology DAAC. doi: 10.5067/orbview-2/seawifs/l3m/par/2018
NOAA NESDIS COASTWATCH and NOAA SWFSC ERD (2018). Day and Night, Western Hemisphere Level 3 Sea Surface Temperature from the Geostationary Operational Environmental Satellites (GOES) Imager on the GOES-11 Satellite (GDS Version 1). Silver Spring, MD: National Oceanographic Data Center.
Okamura, K. (1916). Akashio ni Tsuite (On red-tides). Suisan Koushu Sikenjo Kenkyu Hokoku 12, 26–41.
Osorio-Tafall, B. F. (1942). Notas sobre algunos dinoflagelados planctónicos marinos de México con descripciön de nuevas especies. An. Esc. Nal. Cienc. Biol. 2, 435–447.
Oyarzún, D., and Brierley, C. M. (2018). The future of coastal upwelling in the Humboldt Current from model projections. Clim. Dyn. 51, 1–17. doi: 10.1007/s00382-018-4158-7
Padmakumar, K. B., Thomas, L. C., Salini, T. C., and Vijayan, A. (2018). Subsurface bloom of dinoflagellate Gonyaulax polygramma Stein in the shelf waters off Mangalore- South Eastern Arabian Sea. Int. J. Geo Mar. Sci. 47, 1658–1664.
Pennington, J. T., Mahoney, K. L., Kuwahara, V. S., Kolber, D. D., Calienes, R., and Chavez, F. P. (2006). Primary production in the eastern tropical Pacific: a review. Prog. Oceanogr. 69, 285–317. doi: 10.1016/j.pocean.2006.03.012
Peragallo, H., and Peragallo, M. (1900). Diatomées Marines de France et des Districts Maritimes Voisins (Grez-sur-Loing: M. J. Tempére), 73–80. doi: 10.5962/bhl.title.13501
Perumal, P., Sampathkumar, P., and Karuppasamy, P. K. (1999). Studies on the bloom-forming species of phytoplankton in the Vellar estuary, southeast coast of India. Indian J. Mar. Sci. 28, 400–403.
Pesantes, F. (1983). Dinoflagelados del fitoplancton del Golfo de Guayaquil. Acta Oceanogr. Pac. 2, 284–318.
Pierce, R. H., and Henry, M. S. (2008). Harmful algal toxins of the Florida red tide (Karenia brevis): Natural chemical stressors in South Florida coastal ecosystems. Ecotoxicology 17, 623–631. doi: 10.1007/s10646-008-0241-x
Pitcher, G. C., and Calder, D. (2000). Harmful algal blooms of the southern Benguela current: a review and appraisal of monitoring from 1989–1997. S. Afr. J. Mar. Sci. 22, 255–271. doi: 10.2989/025776100784125681
Pitcher, G. C., Jiménez, A. B., Kudela, R. M., and Reguera, B. (2017). Harmful algal blooms in eastern boundary upwelling systems: a GEOHAB Core Research Project. Oceanography 30, 22–35. doi: 10.5670/oceanog.2017.107
Prado, M., Trocholli, L., and Moncayo, E. (2015). Cambios estructurales del microfitoplancton en la zona 442 costera de la provincia El Oro-Ecuador en temporada seca. Bol. Inst. Oceanogr. Venez. 54, 139–152.
Pritchard, A. (1861). A History of Infusoria, Including the Desmidiaceae and Diatomaceae, British and Foreign, eds J. T. Arlidge, B. A. Lond, W. Archer, J. Ralfs, and W. C. Williamson (London: Whittaker & Co).
Razali, R. M., Leaw, C. P., Lim, H. C., Nyanti, L., Ishak, I., and Lim, P. T. (2015). Harmful microalgae assemblage in the aquaculture area of Aman Island, Northern Strait of Malacca. Malays. J. Sci. 34, 20–32. doi: 10.22452/mjs.vol34no1.3
Reguera, B., Riobó, P., Rodríguez, F., Díaz, P., Pizarro, G., Paz, B., et al. (2014). Dinophysis toxins: causative organisms, distribution and fate in shellfish. Mar. Drugs 12, 394–461. doi: 10.3390/md12010394
Reguera, B., Velo-Suárez, L., Raine, R., and Park, M. G. (2012). Harmful Dinophysis species: a review. Harmful Algae 14, 87–106. doi: 10.1016/j.hal.2011.10.016
Rhodes, L., Scholin, C., and Garthwaite, I. (1998). Pseudo-nitzschia in New Zealand and the role of DNA probes and immunoassays in refining marine biotoxin monitoring programmes. Natural Toxins 6, 105–111. doi: 10.1002/(SICI)1522-7189(199805/08)6:3/4<105::AID-NT13>3.0.CO;2-9
Saburova, M., Polikarpov, I., and Al-Yamani, F. (2012). First record of Kryptoperidinium foliaceum (Dinophyceae: Peridiniales) from a hypersaline environment in Kuwait, north-western Arabian Gulf. Mar. Biodivers. Records 5, 3–7. doi: 10.1017/S1755267212000838
Saville-Kent, W. (1881). A Manual of the Infusoria, Including a Description of all Known Flagellate, Ciliate, and Tentaculiferous Protozoa, British and Foreign and an Account of the Organization and Affinities of the Sponges. David Bogue: London, 289–720.
Schütt, F. (1895). Die peridineen der plankton-expedition. Ergeb. Plankton Exp. Humboldt Stift. 4, 1–170.
Schütt, F. (1896). “Bacillariales (Diatomeae),” in Die Natürlichen Pflanzenfamilien, eds A. Engler and K. Prantl (Leipzig: Verlag von Wilhelm Engelmann), 31–150.
Sellner, K. G., Doucette, G. J., and Kirkpatrick, G. J. (2003). Harmful algal blooms: causes, impacts and detection. J. Ind. Microbiol. Biotechnol. 30, 383–406. doi: 10.1007/s10295-003-0074-9
Shen, L., Xu, H., and Guo, X. (2012). Satellite remote sensing of harmful algal blooms (HABs) and a potential synthesized framework. Sensors 12, 7778–7803. doi: 10.3390/s120607778
Shi, K., Zhang, Y., Liu, X., Wang, M., and Qin, B. (2014). Remote sensing of diffuse attenuation coefficient of photosynthetically active radiation in Lake Taihu using MERIS data. Remote Sens. Environ. 140, 365–377. doi: 10.1016/j.rse.2013.09.013
Shin, H. H., Li, Z., Kim, E. S., Youn, J. Y., Jeon, S. G., Oh, S. J., et al. (2016). Morphology and phylogeny of Triadinium polyedricum (Pouchet) Dodge (Dinophyceae) from Korean coastal waters. Ocean Sci. J. 51, 647–654. doi: 10.1007/s12601-016-0049-1
Smayda, T. J., and Reynolds, C. S. (2003). Strategies of marine dinoflagellate survival and some rules of assembly. J. Sea Res. 49, 95–106. doi: 10.1016/S1385-1101(02)00219-8
Smayda, T. J., and Trainer, V. L. (2010). Dinoflagellate blooms in upwelling systems: seeding, variability, and contrasts with diatom bloom behaviour. Prog. Oceanogr. 85, 92–107. doi: 10.1016/j.pocean.2010.02.006
Sorooshian, S., Hsu, K., Braithwaite, D., Ashouri, H., and NOAA CDR Program (2014). NOAA Climate Data Record (CDR) of Precipitation Estimation from Remotely Sensed Information Using Artificial Neural Networks (PERSIANN-CDR), Version 1 Revision 1 [Data Set]. Asheville, NC: NOAA National Climatic Data Center. doi: 10.7289/v51v5bwq
Suaréz, B., and Guzmán, L. (1994). Floraciones de Algas Nocivas : Mareas Rojas y Toxinas Marinas. Santiago: Editorial Universitaria, 40–46.
Suparna, M. (2005). “Harmful phytoplankton blooms indicate the existence of allelopathic interactions,” in Proceedings of the 4th World Congress on Allelopathy, Wagga Wagga, 355–358.
Taylor, F. J. R., Fukuyo, Y., and Larsen, J. (1995). “Taxonomy of toxic dinoflagellates,” in Manual on Harmful Marine Microalgae, eds G. M. Hallegraeff, D. M. Anderson, and A. D. Cembella (Paris: UNESCO), 283–317.
Taylor, M. H. (2008). The Northern Humboldt Current Ecosystem and its Resource Dynamics: Insights from a Trophic Modeling and Time Series Analysis. Ph.D. dissertation, Bremen University, Bremen.
Thangaraja, M., Al-Aisry, A., and Al-Kharusi, L. (2007). Harmful algal blooms and their impacts in the middle and outer ROPME sea area. Int. J. Oceans Oceanogr. 2, 85–98.
Torres, G. (2000). Mareas Rojas durante 1989-1999 en aguas ecuatorianas. Acta Oceanogr. Pac. 10, 127–136.
Torres, G. (2006). Eventos de Mareas Rojas en el Pacífico Ecuatorial (Ecuador). Reporte en el Curso COI-AECI-IEO de Taxonomía y Biotoxinas de Fitoplancton toxico, Vigo-España, febrero 2006.
Torres, G. (2011). Eventos de Mareas Rojas: Estrategias de Manejo Preventivas en Ecuador. Master’s thesis, Universidad de Guayaquil, Guayaquil.
Torres, G. (2013). Eventos de Mareas Rojas: Estrategias de Manejo Preventivas en Ecuador. Master’s thesis, Universidad de Guayaquil, Guayaquil.
Torres, G. (2015). Evaluación de Mareas Rojas durante 1968-2009 en Ecuador. Acta Oceanogr. Pac. 20, 89–98.
Torres, G. (2017). Evaluacion del Fitoplacnton Como un Mecanismo Preventivo a la Ocurrencia de Bloom Algal Frente a las Costas de Esmeraldas, Manta, La Libertad y Puerto Bolivar en Ecuador 2013-2015. Ph.D. thesis, Universidad Nacional Mayor de San Marcos, Lima.
Torres, G., and Palacios, C. (2007a). Bloom de Noctiluca Scintillans y Ceratium dens en el Golfo de Guayaquil (2004). Acta Oceanogr. Pac. 14, 125–130.
Torres, G., and Palacios, C. (2007b). Calidad Ambiental del Blomm Algal en area urbana del Estero Salado (Ciudad de Guayaquil) en junio 2005. Acta Oceanogr. Pac. 14, 115–124.
Torres, G., Recalde, S., Narea, R., Renteria, W., Troccoli, L., and Tinoco, O. (2017). Variabilidad espacio temporal del fitoplancton y variables oceanográficas en El Golfo de Guayaquil durante 2013-15. Rev. Inst. Invest. 20, 70–79.
Torres, G., and Tapia, M. (2002). Distribución del fitoplancton en la región costera del mar ecuatoriano, durante diciembre 2000. Acta Oceanogr. Pac. 11, 63–72.
Torres, G., Tapia, M. E., Coello, D., and Jiménez, R. (2004). “Monitoreo de fitoplancton y eventos de mareas rojas en el Pacfico Ecuatorial (Ecuador),” in Sexto Taller Regional de Planificación Científica sobre Floraciones de Algas Nocivas en Sudamérica (Guayaquil: Comisión Oceanográfica Intergubernamental), 56–64.
Trainer, V. L., Bates, S. S., Lundholm, N., Thessen, A. E., Cochlan, W. P., Adams, N. G., et al. (2012). Pseudo-nitzschia physiological ecology, phylogeny, toxicity, monitoring and impacts on ecosystem health. Harmful Algae 14, 271–300. doi: 10.1016/j.hal.2011.10.025
Trainer, V. L., Pitcher, G. C., Reguera, B., and Smayda, T. J. (2010). The distribution and impacts of harmful algal bloom species in eastern boundary upwelling systems. Prog. Oceanogr. 85, 33–52. doi: 10.1016/j.pocean.2010.02.003
Vila, M., and Masó, M. (2005). Phytoplankton functional groups and harmful algal species in anthropogenically impacted waters of the NW Mediterranean Sea. Sci. Mar. 69, 31–45. doi: 10.3989/scimar.2005.69n131
Vyas, A. A., Chhaya, N. D., Buch, A. U., Bhaskaran, M., Patel, A. J., and Mohile, V. (1986). Mass culture of centric diatom Chaetoceros affinis (Lauder) - A live feed for prawn larvae. Indian J. Mar.Sci. 15:55.
Wall, D., and Dale, B. (1971). A reconsideration of living and fossil Pyrophacus Stein, 1883 (Dinophyceae). J. Phycol. 7, 221–235. doi: 10.1111/j.1529-8817.1971.tb01507.x
Wall, S., and Cicily, L. (2015). First report on a bloom of marine dinoflagellate Pyrophacus steinii (Schiller). Harmful Algae News 51, 8–9.
Wang, D. Z. (2008). Neurotoxins from marine dinoflagellates: a brief review. Mar. Drugs 6, 349–371. doi: 10.3390/md6020349
Wang, S. F., Tang, D. L., He, F. L., Fukuyo, Y., and Azanza, R. V. (2008). Occurrences of harmful algal blooms (HABs) associated with ocean environments in the South China Sea. Hydrobiologia 596, 79–93. doi: 10.1007/s10750-007-9059-4
Wei, G. F., Tang, D. L., and Wang, S. (2008). Distribution of chlorophyll and harmful algal blooms (HABs): a review on space based studies in the coastal environments of Chinese marginal seas. Adv. Space Res. 41, 12–19. doi: 10.1016/j.asr.2007.01.037
Wells, M. L., Trainer, V. L., Smayda, T. J., Karlson, B. S. O., Trick, C. G., Kudela, R. M., et al. (2015). Harmful algal blooms and climate change: learning from the past and present to forecast the future. Harmful Algae 49, 68–93. doi: 10.1016/j.hal.2015.07.009
White, A. E., Watkins-Brandt, K. S., McKibben, S. M., Wood, A. M., Hunter, M., Forster, Z., et al. (2014). Large-scale bloom of Akashiwo sanguinea in the Northern California current system in 2009. Harmful Algae 37, 38–46. doi: 10.1016/j.hal.2014.05.004
Yasumoto, T., Murata, M., Oshima, Y., Sano, M., Matsumoto, G. K., and Clardy, J. (1985). Diarrhetic shellfish toxins. Tetrahedron 41, 1019–1025. doi: 10.1016/S0040-4020(01)96469-5
Yurimoto, T., Aue-umneoy, D., Meeanan, C., and Tsutsui, I. (2015). Bloom of the two dinoflagellates Ceratium furca and Diplopsalis lenticula in a mangrove estuary of Thailand. Int. Aquat. Res. 7, 133–141. doi: 10.1007/s40071-015-0099-5
Keywords: remote sensing, phytoplankton taxonomic groups (PTG), Gulf of Guayaquil, dinoflagellates, upwelling, Humboldt Current
Citation: Borbor-Cordova MJ, Torres G, Mantilla-Saltos G, Casierra-Tomala A, Bermúdez JR, Renteria W and Bayot B (2019) Oceanography of Harmful Algal Blooms on the Ecuadorian Coast (1997–2017): Integrating Remote Sensing and Biological Data. Front. Mar. Sci. 6:13. doi: 10.3389/fmars.2019.00013
Received: 01 August 2018; Accepted: 14 January 2019;
Published: 19 February 2019.
Edited by:
Marius Nils Müller, Federal University of Pernambuco, BrazilReviewed by:
Maximo Jorge Frangopulos, University of Magallanes, ChileMárcio Silva de Souza, Fundação Universidade Federal do Rio Grande, Brazil
Copyright © 2019 Borbor-Cordova, Torres, Mantilla-Saltos, Casierra-Tomala, Bermúdez, Renteria and Bayot. This is an open-access article distributed under the terms of the Creative Commons Attribution License (CC BY). The use, distribution or reproduction in other forums is permitted, provided the original author(s) and the copyright owner(s) are credited and that the original publication in this journal is cited, in accordance with accepted academic practice. No use, distribution or reproduction is permitted which does not comply with these terms.
*Correspondence: Mercy J. Borbor-Cordova, bWVib3Jib3JAZXNwb2wuZWR1LmVj