- 1Department of Ecology and Hydrology, Regional Campus of International Excellence “Mare Nostrum,” University of Murcia, Murcia, Spain
- 2Murcia Institute for Research and Agricultural and Food Development – IMIDA, Murcia, Spain
- 3Department of Biodiversity, Ecology and Evolution, Complutense University of Madrid, Madrid, Spain
Coastal lagoons are considered among the marine habitats with the highest biological productivity, and support a great variety of human activities and pressures that make them especially vulnerable to trophic imbalances. While dystrophic crises are common in many lagoons, others like the Mar Menor show homeostatic mechanisms, high resilience, and clear waters. This paper analyses the water column descriptors dynamic during the last 22 years in this coastal lagoon, in the context of a eutrophication process produced by an increase in nutrient inputs, mainly derived from agriculture. Despite water column nitrate concentration increased by one order of magnitude, the lagoon maintained homeostatic regulation for two decades, keeping the water transparency and relatively low levels of nutrients and chlorophyll a (Prebreak phase), followed by a sudden change of state in 2016 with an abrupt increase in average nutrients and chlorophyll a concentration and loss of water transparency (Break phase), and a relatively rapid recovery after the reduction of nutrient discharges (Recovery phase). The activation of the regulation mechanisms seems to manifest through an ammonium production in the water column, as a consequence of the activity in the trophic web. The low correlation between chlorophyll a and nutrients concentration, mainly at small spatio-temporal scales, is in disagreement with eutrophication traditional models, and suggests a rapid response of primary producers to nutrient inputs and a zooplankton control in the short-term, which in turn is controlled by the rest of the trophic web components. Homeostatic properties that in the Mar Menor lagoon have provided resistance to eutrophication are based on several mechanisms: channeling its production toward the benthic system (maintaining high biomasses of primary producers, filter feeders, and detritivores), a top-down control of the pelagic trophic web exerted by ichthyoplankton and jellyfish, and exporting surplus production outside the system. Resilience of the system would be based on the high turnover in the species composition related to the restricted connectivity to the sea, the spatio-temporal variability of the environmental conditions, and the multiplicity of spatial–temporal scales involved in lagoon processes. TRIX index was sensitive to the trophic and water quality changes. However, in our study, its current score does not allow to anticipate or alert the eutrophication risk and the trophic breakpoint of the system.
Introduction
Eutrophication is among the main problems affecting the integrity and health status of coastal marine ecosystems. It is a process that, beyond causing contamination by nutrients or an increase of organic matter supply which enhance primary production of the ecosystem (European Environment Agency [EEA], 2001), represents a fundamental change in the energetic base of the same (Nixon, 2009). This may propagate through the system in various ways and produce a great variety of changes in the structure and dynamic of the ecosystem (Likens, 1972; Nixon, 1995, 2009; Gamito et al., 2005; Ferreira et al., 2011; Desmit et al., 2018).
In general, the eutrophication process leads to the progressive replacement of seagrasses and slow-growing macroalgae by fast-growing macroalgae and phytoplankton, with the final dominance of the latter at high nutrient loads (Gamito et al., 2005). When eutrophication is chronic, the effects include hypoxia events, chlorophyll and toxic algal blooms, foam events, nutrient imbalances, the massive death of benthic animals, and changes in species patterns and even in community structure (Desmit et al., 2018). Frequently, once the negative effects of eutrophication emerge, it is very difficult to stop or revert the process, and subsequent delivery of nutrients from bottom sediments may prolong it for years (Nienhuis, 1992; Duarte et al., 2013; McCrackin et al., 2016).
Under these conditions, periods of high photoautotrophic activity and accumulation of labile organic matter can be followed by heterotrophic phases with a strong imbalance of oxygen metabolism, leading to pulsed oxygen fluctuations from supersaturation to anoxia (D’Avanzo et al., 1996; Viaroli et al., 2001; Viaroli and Christian, 2003). These changes in trophic status can occur both at seasonal and daily time scales.
Coastal lagoons are especially vulnerable to suffer from the eutrophication process (Ménesguen and Lacroix, 2018). They are transitional ecosystems between proper transitional waters sensu EU Water Framework Directive (WFD) and coastal waters (Pérez-Ruzafa et al., 2011a) characterized by being shallow, relatively isolated from the sea, and with strong physical–chemical gradients in a constricted space (UNESCO, 1981; Tagliapietra et al., 2009; Pérez-Ruzafa et al., 2011b). These characteristics cause them to be among the marine habitats with the highest biological productivity (Alongi, 1998; Kennish and Paerl, 2010). They play an important ecological role by offering a variety of habitat types for many species, giving shelter to an important biodiversity (European Environment Agency [EEA], 2010; De Wit, 2011), and functioning as nursery areas and feeding grounds for many marine fishes and crustaceans that migrate from the sea to grow inside the lagoon, returning to the open sea for their reproduction (Yañez-Arancibia and Nugent, 1977; Clark, 1998; Vasconcelos et al., 2011). At the same time, they also generate an important number of other less evident, but with a high ecological value, ecosystem services such as flood control, the prevention of seawater intrusion, groundwater recharge, shoreline stabilization, storm protection, the retention and export of sediment and nutrients, the mitigation of climate change, water purification, or acting as reservoirs of genetic or species biodiversity. This translates into a remarkable socioeconomic role by providing a significative set of societal goods and benefits (Barbier et al., 1997; Ramsar Convention and Secretariat, 2006; Anthony et al., 2009; Pérez-Ruzafa et al., 2011b, 2019b; Basset et al., 2013; Barbier, 2015; Newton et al., 2018; Velasco et al., 2018).
Humans take advantage of the potential of coastal lagoons by developing important fishing and aquaculture activities that often coexist with other uses like tourism, nautical sports, swimming activities, saltworks, or medicinal uses (Pérez-Ruzafa et al., 2009, 2011c). But at the same time, and as a consequence of these characteristics, these natural systems are subject to a great variety of strains and transformations, human activities, and management actions (Pérez-Ruzafa et al., 2005b, 2009; Velasco et al., 2018). These pressures mainly have to do with the uses developed in the drainage basin of the lagoons, with special reference to agricultural uses, the extractive and tourist activities that take place in them, shore modifications and coastal works, the adaptation and maintenance of beaches and infrastructures and, more recently, with the potential effects of climate change. All of them involve energy inputs or alterations in the form of nutrients or organic matter inputs, resuspension of sediments, hydrodynamic modifications, disturbance of water balances and physical–chemical gradients, among others, facilitating in the last instance the process of eutrophication.
This makes eutrophication and dystrophic crises common threats in coastal lagoons, as widely described in literature (Amanieu et al., 1975; Boutiere et al., 1982; Reyes and Merino, 1991; Ferrari et al., 1993; Sfriso et al., 1995; Boynton et al., 1996; Viaroli et al., 1996, 2008, 2015; Giordani et al., 1997, 2009; Guyoneaud et al., 1998; Taylor et al., 1999; Bachelet et al., 2000; Lenzi et al., 2003, 2010; Newton et al., 2003; Sakka Hlaili et al., 2007; Specchiulli et al., 2009; Giusti et al., 2010; Solidoro et al., 2010; Facca et al., 2014; Kralj et al., 2016; Leruste et al., 2016; Martelloni et al., 2016; Rodríguez-Gallego et al., 2017; Vybernaite-Lubiene et al., 2017; Ménesguen and Lacroix, 2018).
However, despite this assumed vulnerability, the geomorphologic and ecological characteristics of coastal lagoons seem to allow them to develop more complex responses to nutrient inputs and eutrophication processes than those observed in other coastal aquatic ecosystems, such as bays or estuaries (Kjerfve and Magill, 1989; Taylor et al., 1999; Pérez-Ruzafa et al., 2002, 2011b). During the last decades, the Mar Menor has resisted to high nutrient loads, after the change in agricultural practices in its watershed in the early 1990s, without showing clear eutrophication symptoms in its water descriptors (Pérez-Ruzafa et al., 2002, 2005a; Salas et al., 2008). This resistance came suddenly to a halt in 2016, when an evident change in the quality of the waters was recorded, that caused high social concern and alarm with a strong impact on social media and with important socioeconomic consequences (Limón, 2016). In this paper, we analyze the evolution, behavior, and relationships of the main descriptors of the water quality in the Mar Menor during the eutrophication process, the sudden break of its ecological equilibrium, and the deterioration of its water quality, and its recovery after the interruption of the nutrient discharges into the lagoon.
Materials and Methods
Study Area
The Mar Menor is a choked coastal lagoon located in SE Spain, a semi-arid region of the SW Mediterranean (Figure 1). The mean annual rainfall in the area is less than 300 mm/year, and potential evapotranspiration is close to 900 mm/year (López Bermúdez et al., 1981). So, the net hydric balance attained an annual deficit above 600 mm/year (Pérez-Ruzafa et al., 2005a).
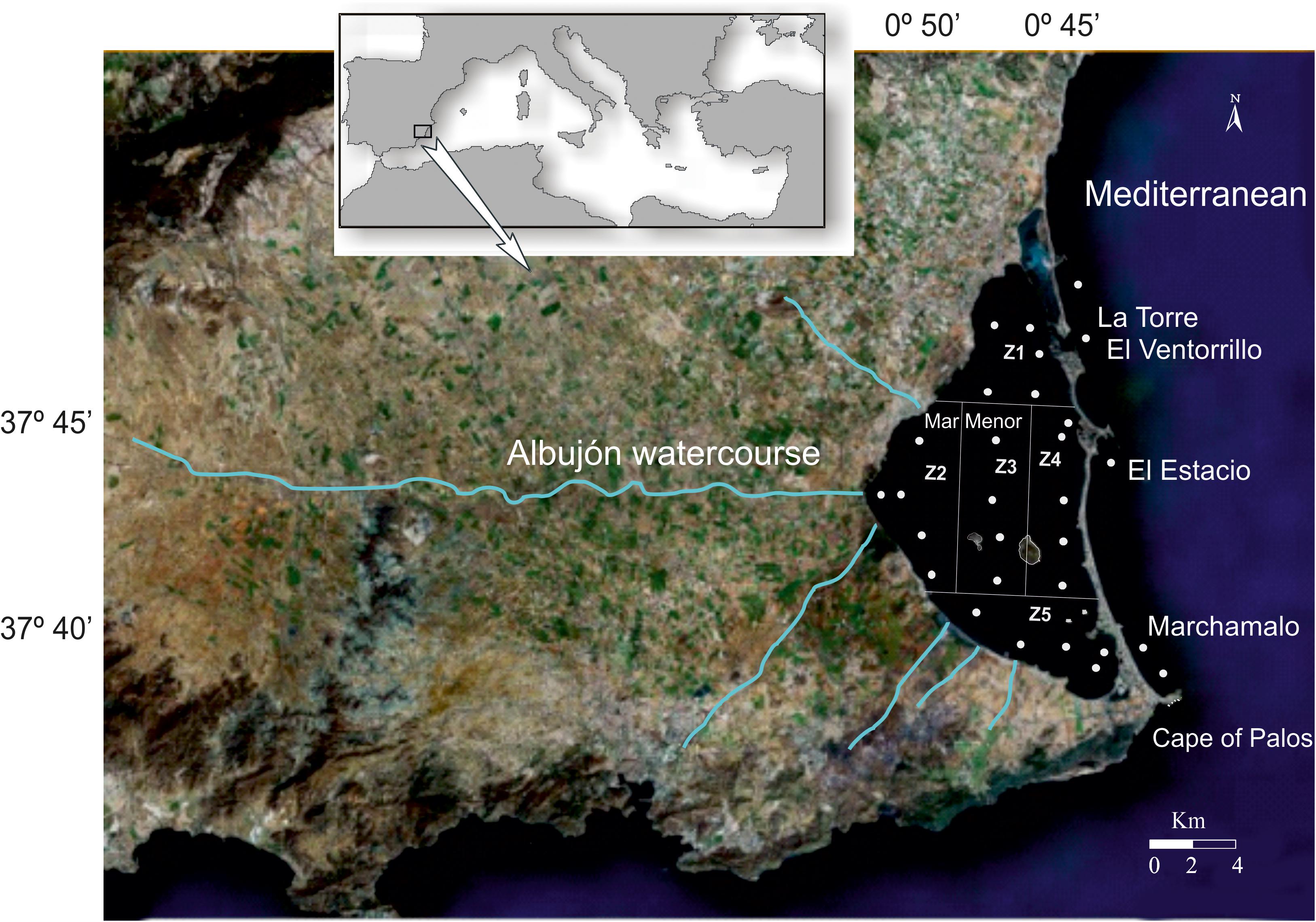
Figure 1. Location of the Mar Menor, its drainage basin that is delimited by the mountain ranges that extend along the north, west, and south limits of the image, and the main watercourses that flow into the lagoon. Points represent the network of sampling stations used in the monitoring studies.
Traditionally, the Mar Menor has been markedly oligotrophic, its main distinctive feature being the transparency of its waters compared to the great majority of coastal lagoons. The primary production was mainly benthic, based on microphytobenthos and on the phanerogam Cymodocea nodosa (Ucria) Ascherson (1870) as the main macrophyte. After the enlarging of one of its inlets (El Estacio) in 1973, benthic vegetation evolved toward a C. nodosa–Caulerpa prolifera (Forsskål) J.V. Lamouroux, 1809 mixed bed, with a biomass of approximately 280 gDW/m2 (Pérez-Ruzafa, 1989; Pérez-Ruzafa et al., 1989; Terrados and Ros, 1991). This high benthic biomass contrasted with the low phytoplankton density (Ros and Miracle, 1984) and water oligotrophy (Gilabert, 2001a). It was estimated that 63.18% of the total primary production of the lagoon was due to C. prolifera, 0.42% to C. nodosa, 0.24% to photophilic algae, 11.62% to microphytobenthos, and 24.53% to phytoplankton (Terrados and Ros, 1991).
Despite the existence of more than 20 cataclinal watercourses in its watershed, traditionally, none of them maintained a permanent flow toward the lagoon, conditioned by a sporadic and torrential rainfall regime (Figure 1). However, since the early 1990s, after changes in agricultural practices from dry farming to irrigation and related phreatic rising, the main collector in the drainage basin, the Albujón watercourse, has maintained a regular flux of water (Pérez-Ruzafa and Aragón, 2002). This watercourse is about 40 km long, draining an area of 556 km2. The flow of El Albujón was estimated to be around 0.02 m3/s at about 4 km from its mouth, reaching up to 10.5 m3/s during a typical storm event (return period of 5.75 years) (García-Pintado et al., 2007). The load of nutrients that flow down the watercourses toward the lagoon shows some temporal pattern, with the highest contents of nitrates (>200 mg /L) and ammonium (>30 mg /L) in periods of maximum agricultural activities and of phosphorus (>10 mg /L) in summer season (Álvarez-Rogel et al., 2006).
As a result of the progressive increase in nutrient inputs, the trophic status changed from oligotrophic to eutrophic. Consequently, the assemblages dominated by small flagellates (Rhodomonas spp. and Cryptomonas spp.) in winter, and diatoms and dinoflagellates from spring to autumn, were replaced by assemblages constituted by large diatoms (Coscinodiscus spp. and Asterionella spp.) present throughout the year, but still maintaining low phytoplankton biomass and without any significant increase in chlorophyll a concentration (Pérez-Ruzafa et al., 2002). The maintenance of good water quality was due to the proliferation, since 1995, of the jellyfish Rhizostoma pulmo (Macri, 1778) and Cotylorhiza tuberculata (Macri, 1778), whose population was estimated at 40 million individuals, exerting a top-down control of the trophic web (Pérez-Ruzafa et al., 2002). This situation had remained relatively stable for 20 years, when a change in the water quality occurred that became particularly evident, even at social level, in the spring of 2016. The water column became dominated by dense populations of Synechococcus spp., and the depth of visibility in the water column was reduced from around 6 m to less than 0.5 m, whereby the compensation depth was above the average depth of the lagoon (4.5 m), leading to the mortality of the C. prolifera–C. nodosa mixed meadows located at more than 2 m depth (Pérez-Ruzafa et al., 2018).
Sampling Design
Spatial and temporal variations of hydrological conditions, nutrients, and chlorophyll a concentration in the Mar Menor lagoon have been analyzed in different projects over the last 22 years, using a sampling stations network that spatially covered the lagoon and following the same protocols (Figure 1). In 1997, weekly surveys were conducted from February to December, while bi-monthly surveys were carried out from May 2002 to May 2003, from February 2006 to September 2013, and from February 2016 to November 2018. According to the terrestrial and marine influence, 20 sampling stations were established in the lagoon, grouped into five zones, so that each zone was represented by four replicate sampling units. Since 2009 eight new sampling stations were added, three in the inner mouth of the lagoon inlets and five in the Mediterranean. More water samples have been also obtained in the framework of the different projects. In total, 5780 water samples have been taken during this period.
Water samples were taken at an approximate depth of 1 m, by pumping or using a Niskin bottle, kept in the dark at 4°C in the field and stored at -28°C. Nitrate (N-), nitrite (N-), ammonium (N-), phosphate (P-), and silicate (Si-) were determined following the methods described by Parsons et al. (1984) and using a continuous flow autoanalyzer (SYSTEA μMAC-1000 and SEAL AutoAnalyzer 3 with a JASCO Fluorescence detector FP-2020 Plus). In 1997, salinity was determined with a Beckman RS 7B salinometer and chlorophyll a was analyzed with the spectrophotometric methods reported by Parsons et al. (1984) in all sampling stations. Since 2002–2003, in situ determinations of salinity, temperature, dissolved oxygen, and chlorophyll a were performed using a WTW Multiline F/Set3 multiple probe, and since 2016 a YSI 6600 multiparameter probe was used including also turbidity measures. Samples for chlorophyll a analysis, following Parsons et al. (1984) spectrophotometric methods, were maintained in nine sampling stations during each campaign for the calibration of probe data. The field campaigns for ichthyoplankton composition studies were carried out using a 500 μm-mesh net equipped with a digital flowmeter (General Oceanics 2030) fixed to the mouth to calculate the volume of filtered water. The methodology is described in detail in Pérez-Ruzafa et al. (2004, 2005a).
Data Analysis
Two matrices have been built, one with the data of all the sampling locations in each campaign (complete matrix) and the other averaging all sampling stations in each campaign (campaigns’ matrix). In both cases, the localities of the Mediterranean, outside the lagoon, have been excluded. Meteorological variables (global radiation, rainfall, and wind speed), obtained from the Spanish Meteorological Agency at the San Javier station on the Mar Menor coast, were added to the campaigns’ matrix. Climatic data, obtained with hourly resolution, were averaged per each sampling day and for the week prior to it.
Relationships between the hydrological, meteorological, and trophic variables were tested using Pearson’s correlation. Temporal differences throughout the process of eutrophication have been analyzed using a permutational multivariate analysis of the variance (PERMANOVA) (Anderson, 2001, 2005), considering three factors: Trophic status phase, Year, and Season. Trophic status phase was considered fixed with three levels, Prebreak from January 2010 to June 2012, Break from February 2016 to December 2017, and Recovery from January 2018 to November 2018. Year and Season were considered randomly, nested in Trophic status phase. By using permutations, the test does not require specific assumption concerning the number of variables or the nature of their individual distributions or correlations (Anderson, 2001). A random subset of 9999 permutations was used. Data were previously normalized and the analyses were performed on the campaigns’ matrix, using the Primer 6.1 package. Euclidean distances were used for the similarity matrix. Significant terms were investigated using a posteriori pairwise comparison with the PERMANOVA t-statistic and 9999 permutations. The contribution of each variable to average differences between factor levels was examined using Similarity percentage analysis (SIMPER) (Clarke et al., 2014).
Relationships among the considered variables and patterns during the eutrophication process were analyzed using a principal component analysis (PCA) and a multidimensional scaling analysis (MDS), using the campaigns’ matrix after square root and log(x+1) transformation of the environmental and ichthyoplankton variables, respectively.
The best linear model accounting for the observed variation of chlorophyll a and water transparency was analyzed using multiple linear regression models (GLM) with stepwise forward selection of variables (using P < 0.05 as the inclusion criterion) performed on the complete matrix.
As a standard reference for the evolution of ecological status in the water column, TRIX index was calculated for each sample according to the algorithm of Vollenweider et al. (1998) and compared with Secchi disc transparency data.
where DIN = N- + N- + N-.
Results
Meteorological and Hydrographic Conditions
The Mar Menor area is characterized by high global irradiation. In the three studied phases (Prebreak, Break, and Recovery), it showed the minima seasonal average of daily global irradiation in autumn, ranging between 3062.51 ± 174.81 and 3806.61 ± 272.18 wats/m2, and the maxima in spring and summer ranging between 6387.78 ± 421.33 and 7471.46 ± 291.74 wats/m2. On the contrary, rainfall is very low and usually concentrates in short intense rain events. Cumulated rainfall in the week previous to sampling days ranged between 0.18 ± 0.10 L/m2 in spring and 39.66 ± 32.81 L/m2 in autumn, both in the Break phase (Table 1). However, some sporadic peaks reached 100 or >200 L/m2 in 1 week, such as the event that occurred in September 2009 and December 2016 (Figure 2).
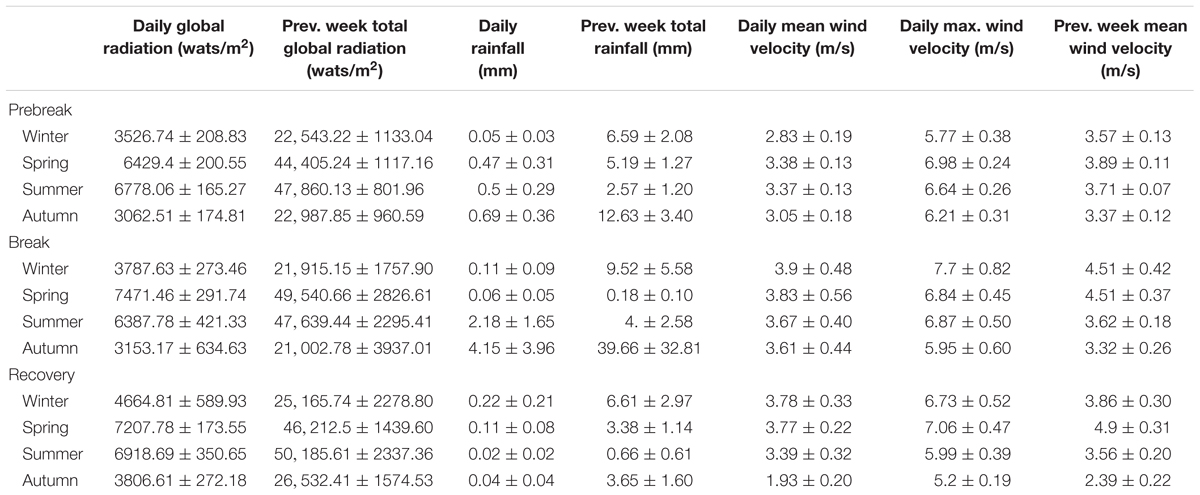
Table 1. Seasonal average of the main climatic variables measured for the previous week and the day of sampling in the Mar Menor area in the three periods considered for the eutrophication process in the lagoon.
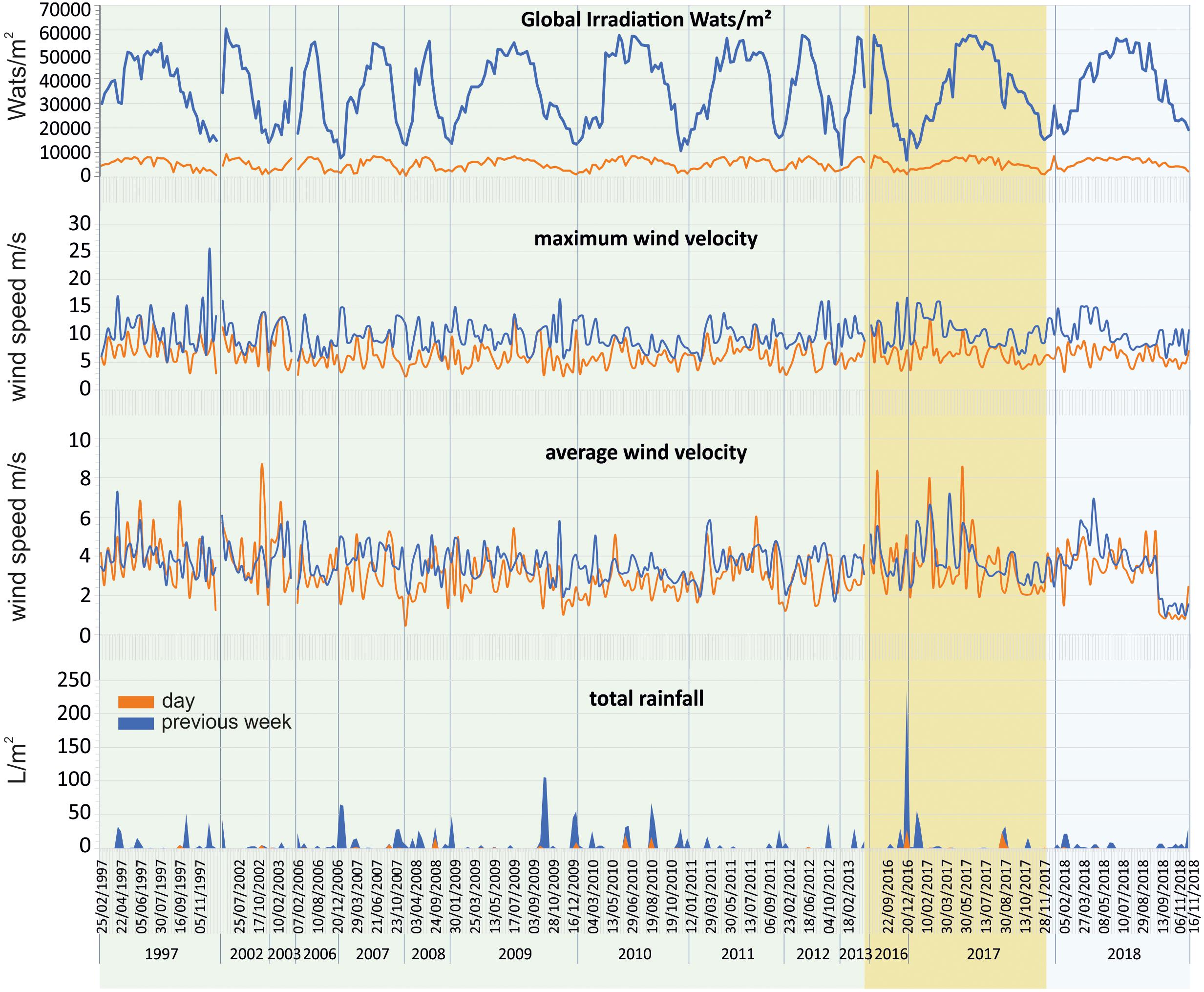
Figure 2. Evolution of the main meteorological variables during the study period in the Mar Menor area. Prebreak phase is highlighted in green, Break phase in ochre, and Recovery in light blue.
Water temperature showed a quite regular seasonal and interannual cycle (Figure 3). The minima seasonal average water temperatures were reached in winter, ranging between 12.78 ± 0.14 and 13.65 ± 0.09°C in the three periods considered, while the maxima were reached in summer, ranging between 27.99 ± 0.04 and 28.4 ± 0.09°C. Salinity showed, however, a very heterogeneous temporal dynamic (Figure 3), ranging between 41.48 ± 0.15 in winter and 45.23 ± 0.16 in autumn (Table 2).
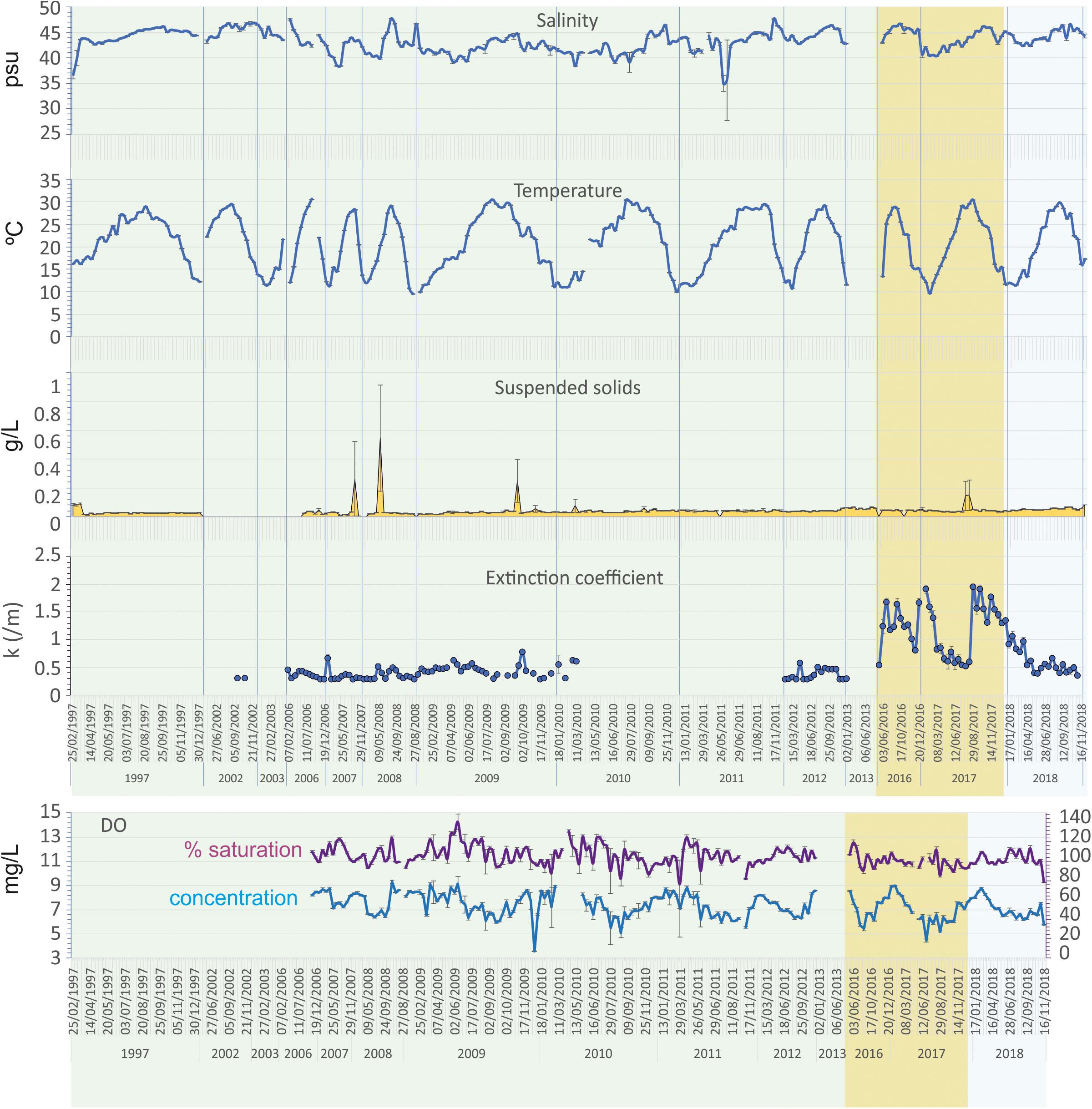
Figure 3. Evolution of the main hydrographic variables during the study period in the Mar Menor lagoon water column. Prebreak phase is highlighted in green, Break phase in ochre, and Recovery in light blue.
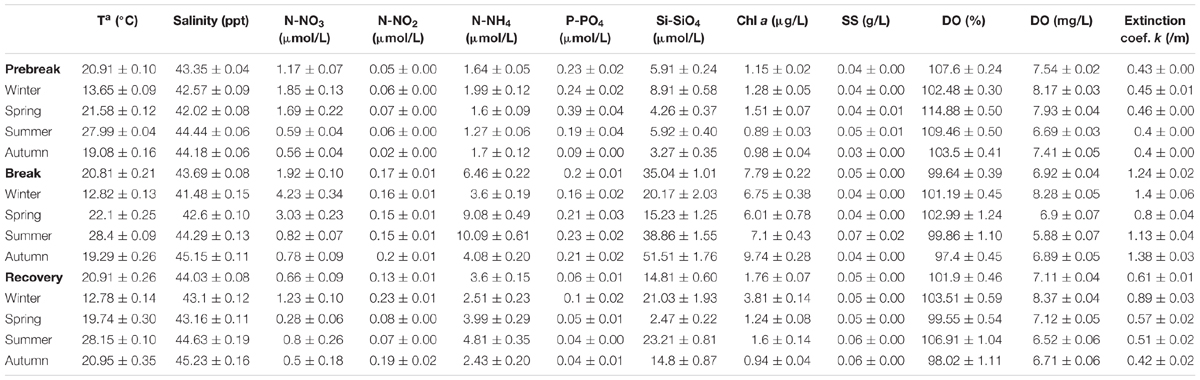
Table 2. Seasonal average of the main hydrographic and trophic variables measured in the water column in the 26 sampling stations in the Mar Menor lagoon for the three periods considered for the eutrophication process.
PERMANOVA analyses performed on meteorological and hydrographic (salinity and temperature) data on the campaigns’ matrix showed no significant differences in climatic or hydrographical conditions between the three trophic status phases, but with significant interannual and seasonal variability (Table 3).
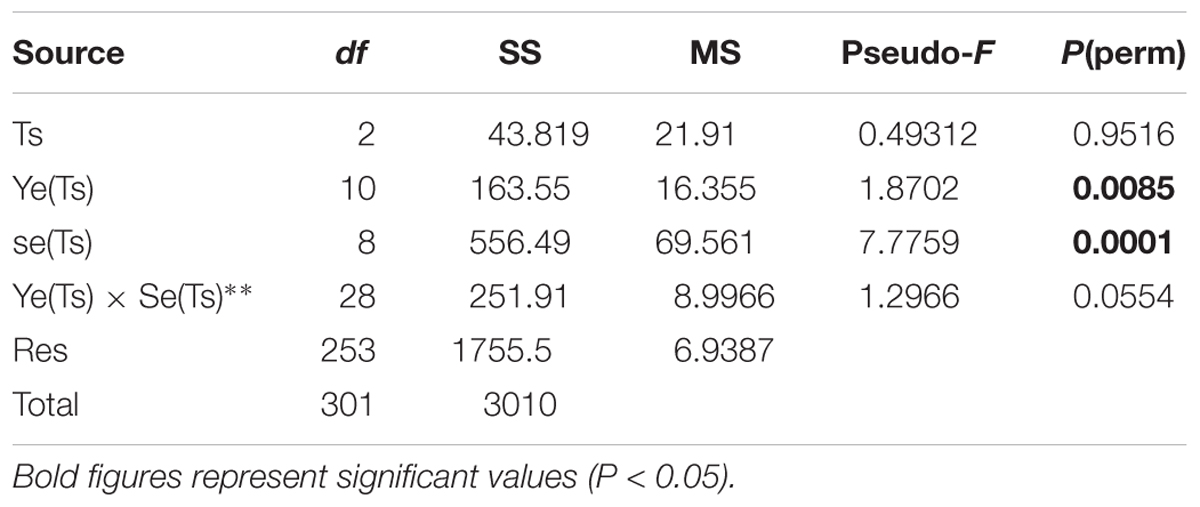
Table 3. Results of PERMANOVA analyses for the meteorological and hydrographic conditions, considering the factors Trophic status phase (Ts), Year (Ye), and Season (Se).
SIMPER analyses showed that the main differences between pairs of years were determined by the Maximum wind speed and Rainfall. The latter mainly determining the differences between 2007, 2008, and 2009. At the same time, high rainfall and low salinity determined the differences between 2010, 2011, and 2016 with respect to the remaining years. Although low salinity is usually associated with rainfall, in the case of Mar Menor it can also be determined by the Albujón watercourse wastes coming from irrigation, that are usually more intense in dry periods. Furthermore, the surplus of rain and irrigation recharges the phreatic, producing subsurface discharges in the coastal areas of the lagoon. This takes place with a temporary delay with respect to the rains and can last for long periods of time. That was the case after the strong rainfall occurred in December 2016 that produced a decrease in salinity maintained until May 2017 (Figures 2, 3). In fact, 2017 was characterized by low rainfall but at the same time low salinity during most of the year.
Trophic Status Variables
Trophic variables and ichthyoplankton assemblages in the water column showed very irregular patterns with very low correlation with climatic and hydrological parameters and between them (Figures 4, 5). The highest significant correlations were found between dissolved oxygen concentration in mg/L and irradiation and water temperature (-0.42; P = 0.00 and -0.64; P = 0.00, respectively), and between chlorophyll a and silicate (0.40; P = 0.00). Chlorophyll a and ammonium also showed a moderate correlation (0.40) but not a significant one (P = 0.43).
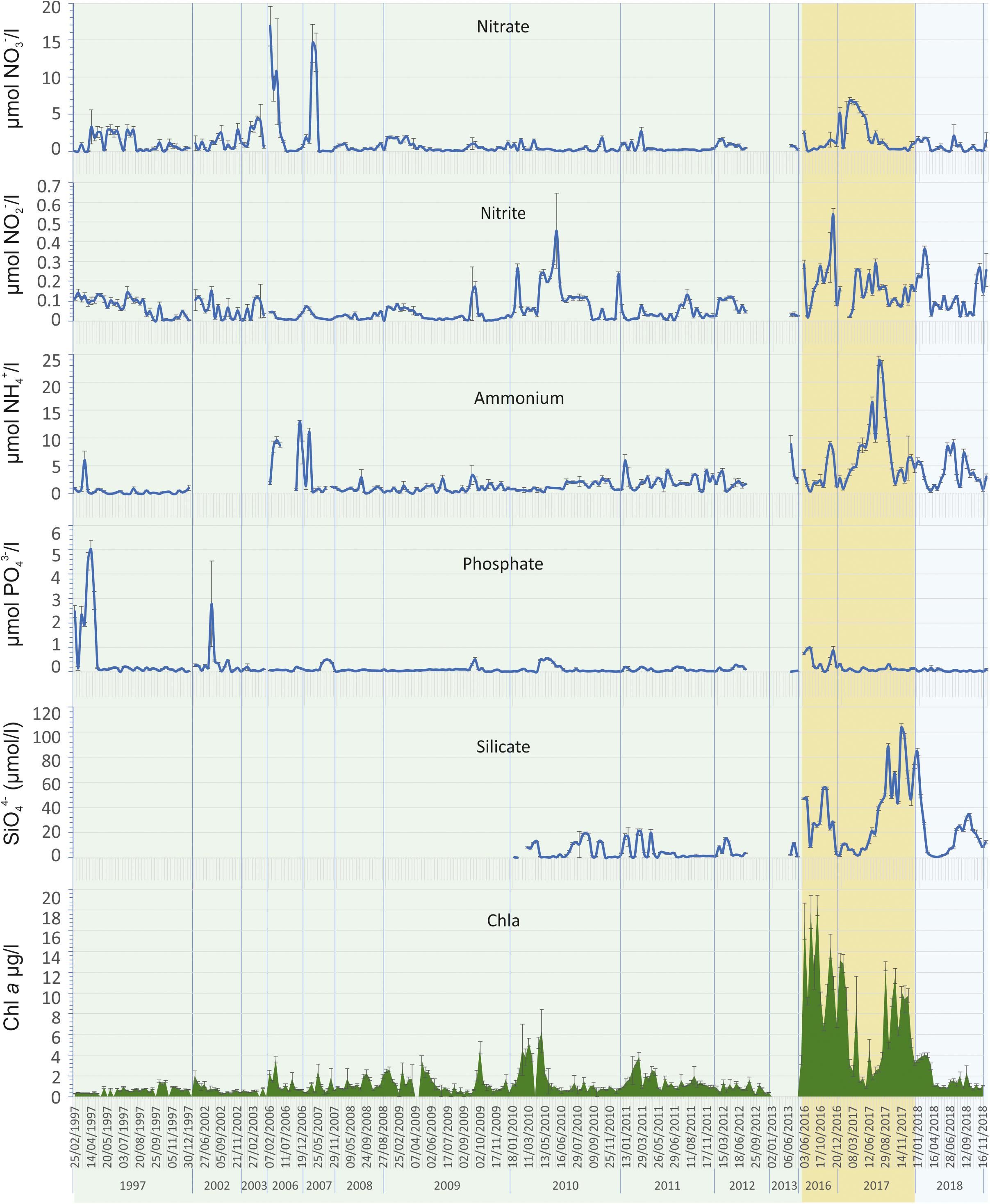
Figure 4. Evolution of the main chemical variables (nitrate, nitrite, ammonium, phosphate, and silicate) and chlorophyll a concentration characterizing the trophic status during the study period in the Mar Menor lagoon water column. Prebreak phase is highlighted in green, Break phase in ochre, and Recovery in light blue.
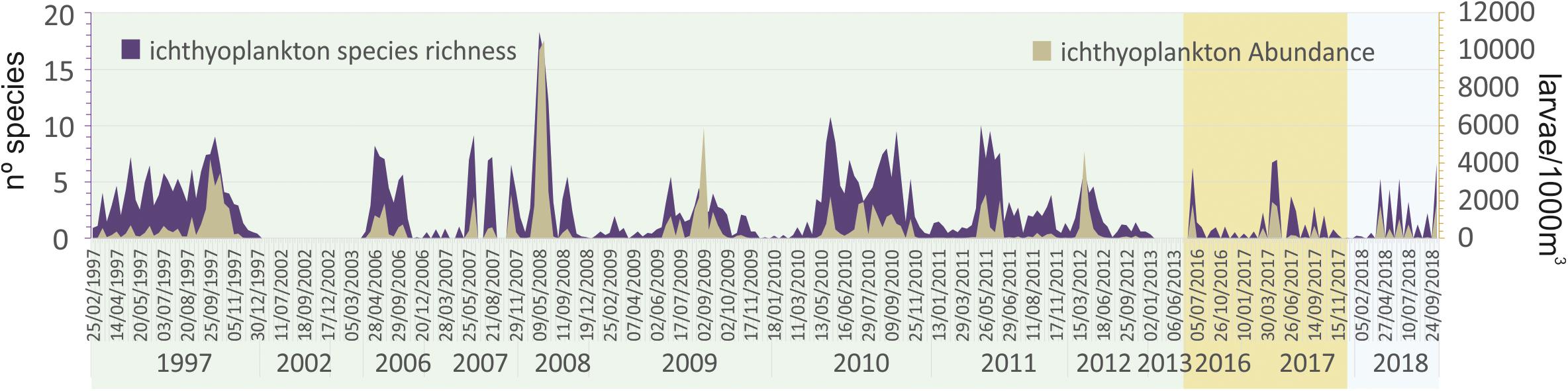
Figure 5. Evolution of the total abundance and species richness of ichthyoplankton assemblages during the study period in the Mar Menor lagoon. Prebreak phase is highlighted in green, Break phase in ochre, and Recovery in light blue.
While seasonal average nutrient values in the three trophic phases analyzed showed low-to-moderate concentrations (Figure 4, Table 2), all nutrients had peaks of greater or lesser intensity throughout the study period. Seasonal mean values were lower than 4.23 ± 0.34 μmol/L in the case of nitrate, 0.23 ± 0.01 μmol/L for nitrite, 10.09 ± 0.61 μmol/L for ammonium, and 0.39 ± 0.04 μmol/L for phosphate. The spatial distribution of the maximum values of nitrate and chlorophyll a showed very distinct patterns in the three trophic status phases (Figure 6). Nitrate reached 145.79 μmol/L in some lagoon localities in 2006 and up to 263.19 μmol/L close to the Albujón watercourse, both during the Prebreak period, while maximum values were always lower than 37.5 μmol/L during Break and Recovery phases. On the contrary, Chl a reached its maximum concentration during the Break phase (49.7 μg/L) and its values were lower than 27 and 13.5 μg/L during Prebreak and Recovery phases, respectively.
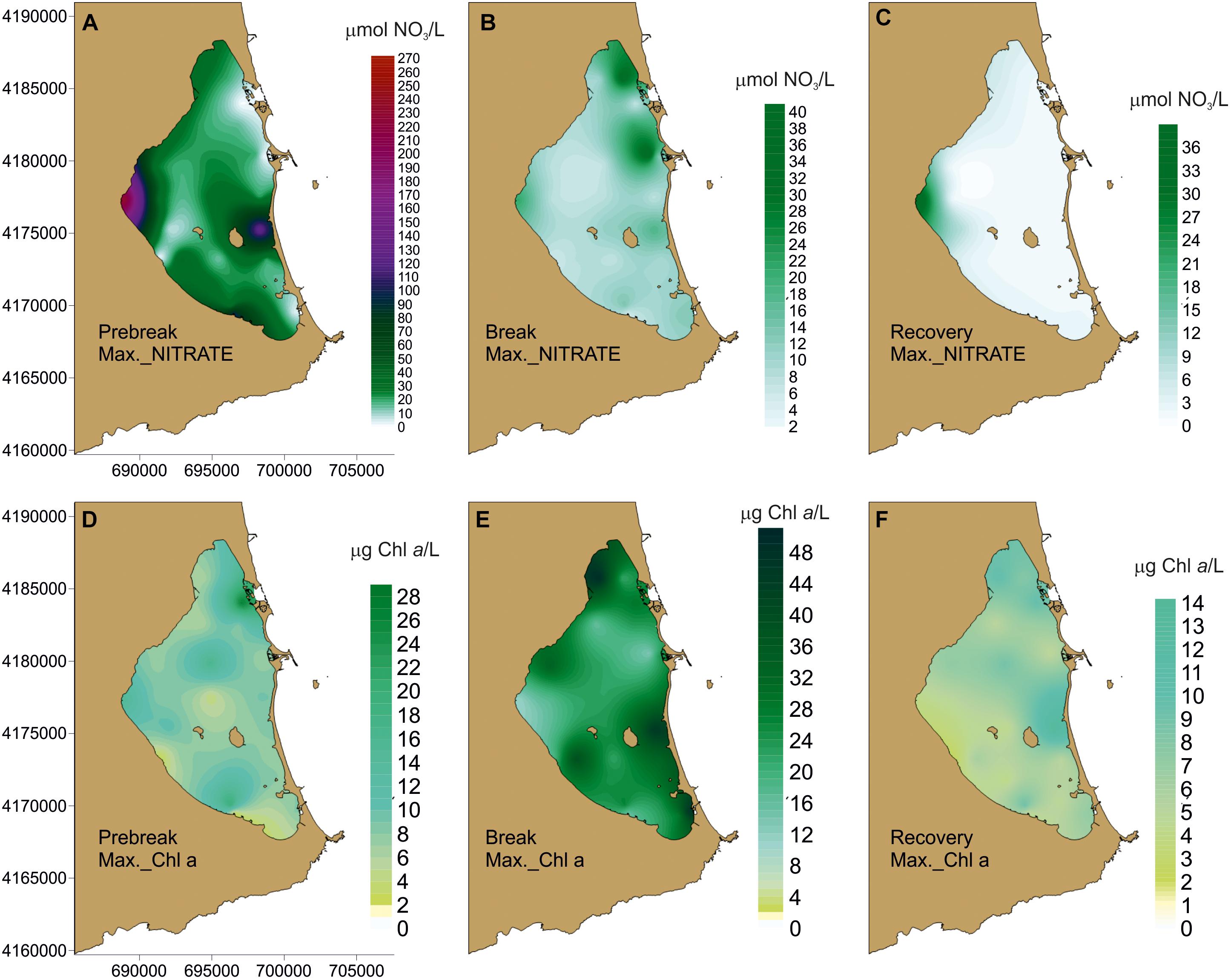
Figure 6. Spatial distribution of the maximum values of nitrate and chlorophyll a reached in the three trophic status phases considered: (A, D) Prebreak; (B, E) Break; and (C, F) Recovery.
The remaining nutrients also showed distinct patterns between periods. Nitrites maintained low values. They only exceeded 2 μmol/L during the Prebreak phase, in eight occasions, all of them in November–December 2006 and in spring and summer of 2007, all in coastal localities, mainly in the mouth of watercourses, reaching in three occasions values higher than 5 μmol/L (7.18, 32.97, and 47.61 μmol/L). The highest values of phosphate were reached early during the Prebreak period. In 1997, values between 2.20 and 8.74 μmol/L were found in most sampling localities, from the end of February to the end of April. In July 2002, still the early Prebreak phase, the highest punctual values of phosphate were reported, reaching 23.86 and 27.17 μmol/L. Silicate, on the other hand, reached the highest values during the Break and the Recovery phases with mean values of 35.04 ± 1.01 and 14.81 ± 0.60 μmol/L, respectively. Its highest values being at the end of the Break phase, reaching values frequently higher than 100 and up to 133.61 μmol/L. Ammonium reached values higher than 30 μmol/L only in two occasions, 40.42 and 44.63 μmol/L, in March and July 2007, respectively, close to the mouth of the Albujón watercourse during the Prebreak phase. However, it reached values between 30.35 and 37.25 μmol/L in several occasions in the inner lagoon localities in April, July, and August of 2017, during the Break period.
PERMANOVA analyses performed on the trophic and water quality variables, using the factors Trophic status phase (Ts) as a fixed factor, and Year and Season nested in Ts, showed significant differences between years and for the interaction Year × Season, precluding to detect differences between phases (Table 4). However, these differences became highly significant when the analysis was performed considering only the Trophic status phase factor (Table 5).
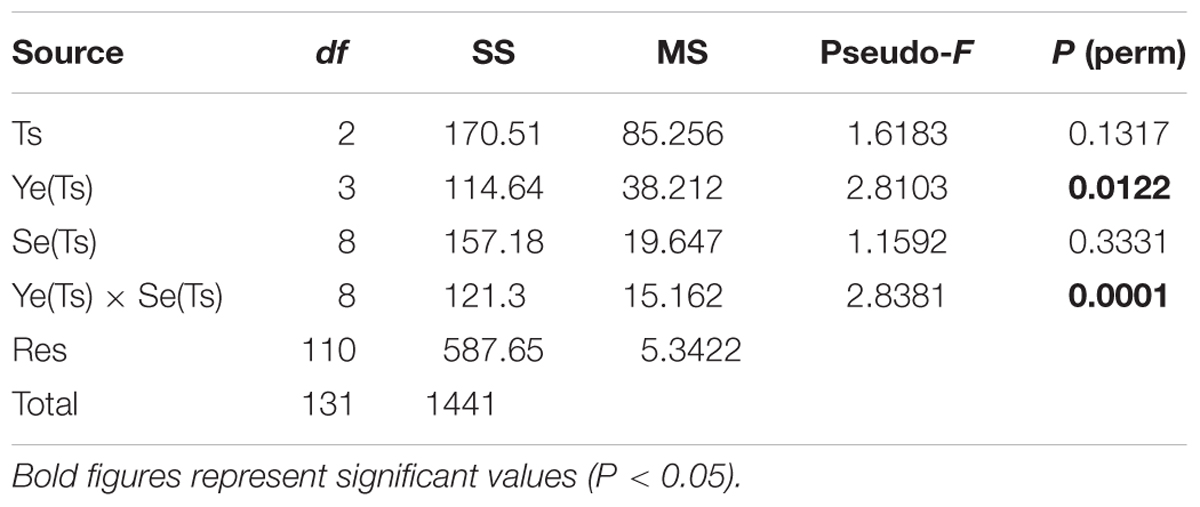
Table 4. Results of PERMANOVA analyses for the trophic variables considering the factors Trophic status phase (Ts), Year (Ye), and Season (Se).
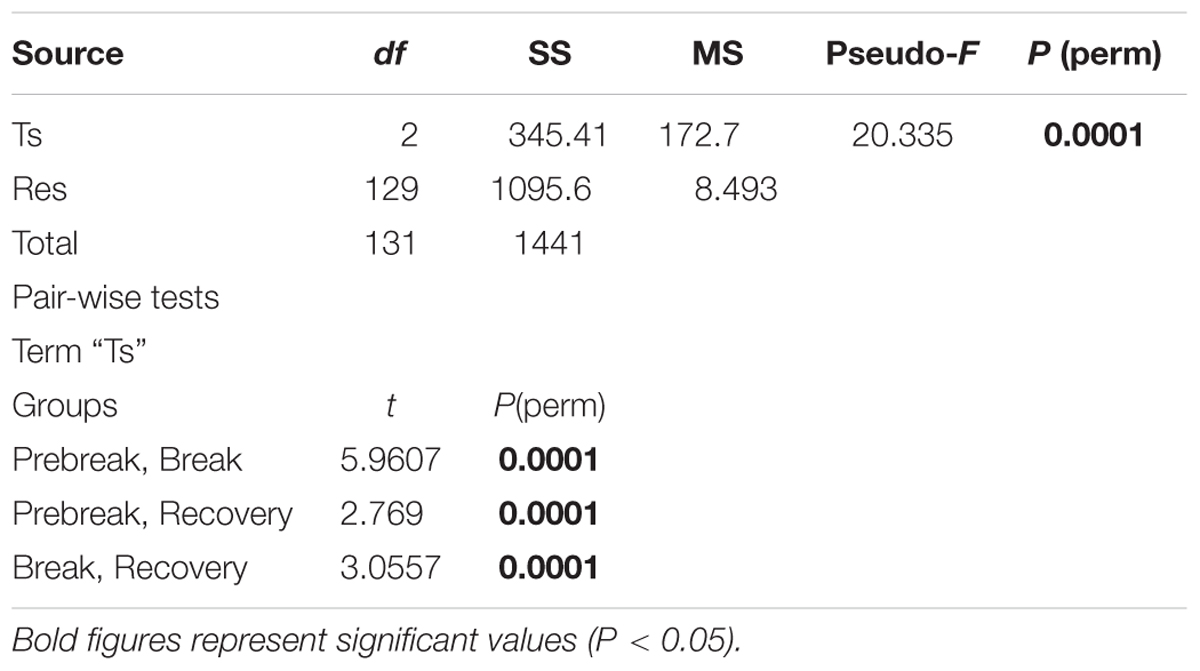
Table 5. Results of PERMANOVA analyses for the meteorological and hydrographic conditions considering the factors Trophic status phase (Ts).
MDS analysis performed on the same trophic and water quality variables from the campaigns’ matrix confirmed this result and showed the same samples distribution than PCA in Figure 7. ANOSIM analyses also confirmed significant differences between all pairwise comparisons of Trophic status phases (P < 0.003).
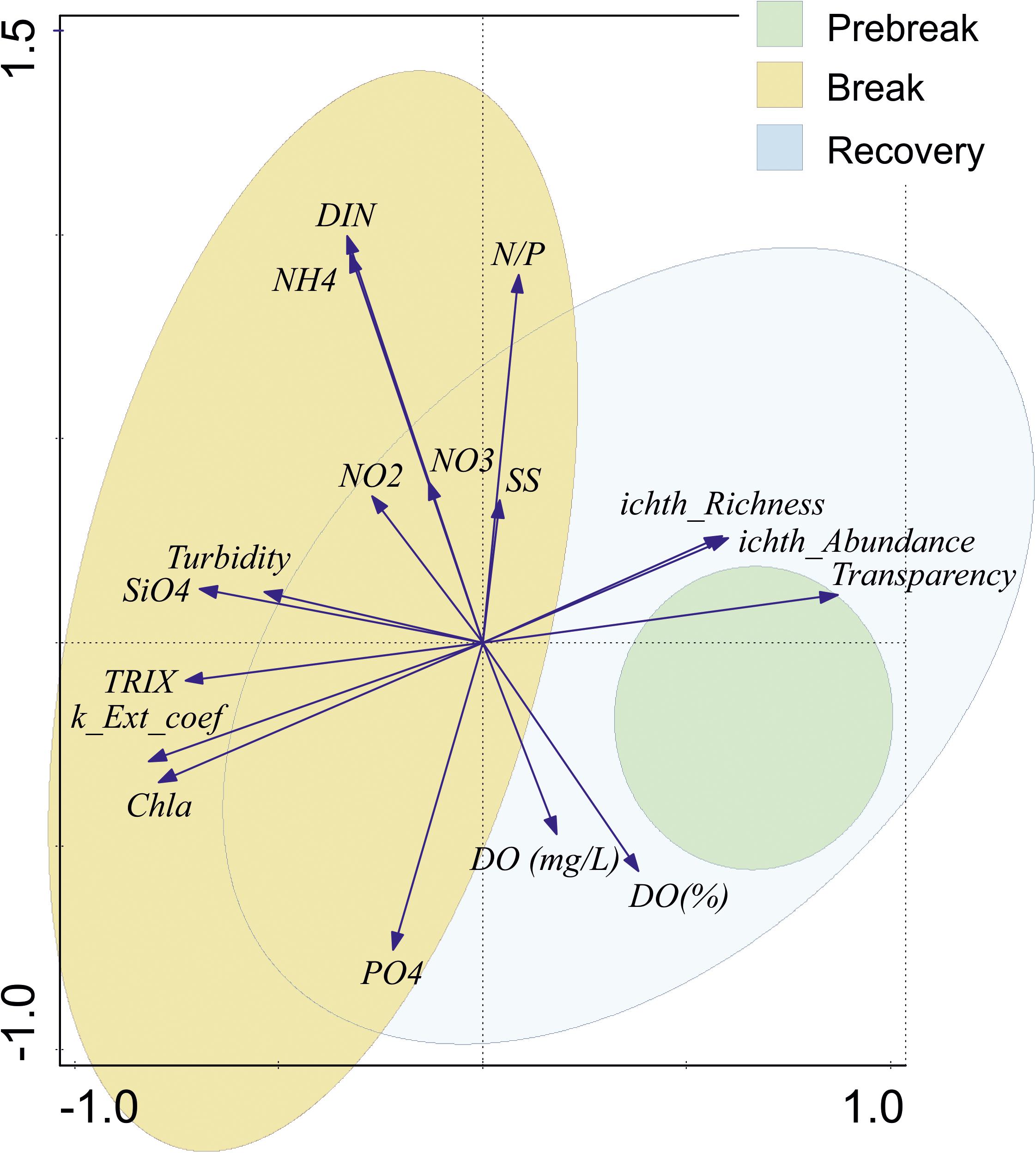
Figure 7. Biplot representations of the first two axes of the PCA performed on the campaigns’ matrix of trophic variables (averaged for the lagoon) sampled from 1997 to 2018 in the Mar Menor lagoon identifying the trophic status phase to which they correspond. Ellipses represent 95% confidence level for samples distribution.
Factors Explaining Chlorophyll a Concentration in the Water Column and Trophic Characterization of Trophic Status Phases
Multiple regression analyses with forward selection of variables showed that there is a low percentage of chlorophyll a concentration variance that is explained by meteorological, hydrological, or nutrient variables. This implies that there are other factors that determine the concentration of chlorophyll a or, more probably, that there are complex patterns and temporary lags in the response of chlorophyll a to them. This analysis, performed both on campaigns’ and complete matrices, showed a similar adjusted squared multiple R of 0.29 and 0.30, respectively (Tables 6, 7). The first case considered the averaged data for the whole lagoon in 151 campaigns carried out over the past 22 years, and the last one considered the 1324 sampling stations sampled in the same 151 campaigns and excluding the meteorological conditions. Although in both analyses , and showed a positive coefficient, indicating that chlorophyll a tends to increase with these nutrients, the response is not linear (Figure 8) and the concentration of chlorophyll increases mainly at low concentrations, but tends to decrease at higher concentrations of them. Ammonium produced the contrary pattern effect on chlorophyll a concentration. Chlorophyll a decreases when increasing ammonium at low concentrations, but tends to increase when this nutrient reached concentrations higher than 25 μmol/L. In fact, in the case of campaigns’ matrix, dissolved inorganic nitrogen (DIN) showed a negative, but not significant, coefficient, probably due to the influence of which in the complete matrix analyses was selected with a significant negative coefficient.
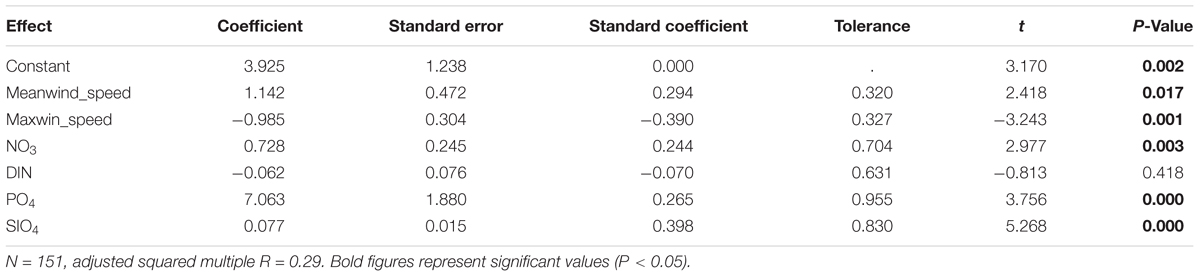
Table 6. Results of the multiple regression analyses with forward selection of variables performed on the campaigns’ matrix to explain Chl a concentration in the water column in the Mar Menor lagoon during the last 22 years (1997–2018), using meteorological, hydrographical, and nutrients as independent variables.
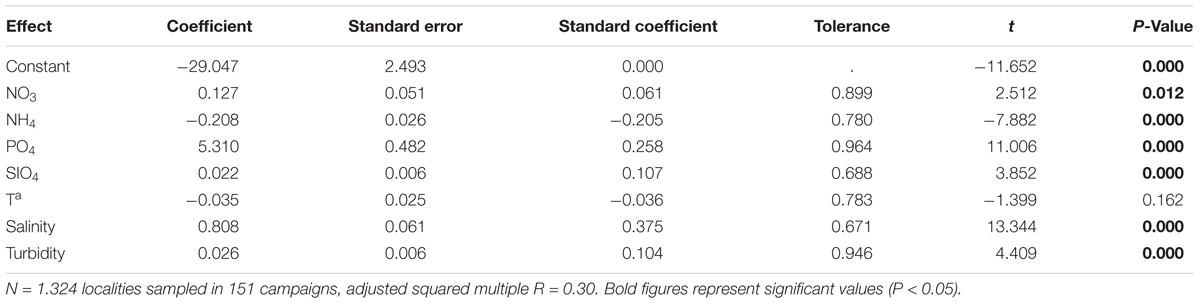
Table 7. Results of the multiple regression analyses with forward selection of variables performed on complete matrix of sampling stations to explain Chl a concentration in the water column in the Mar Menor lagoon during the last 22 years (1997–2018) using meteorological, hydrographical, and nutrients as independent variables.
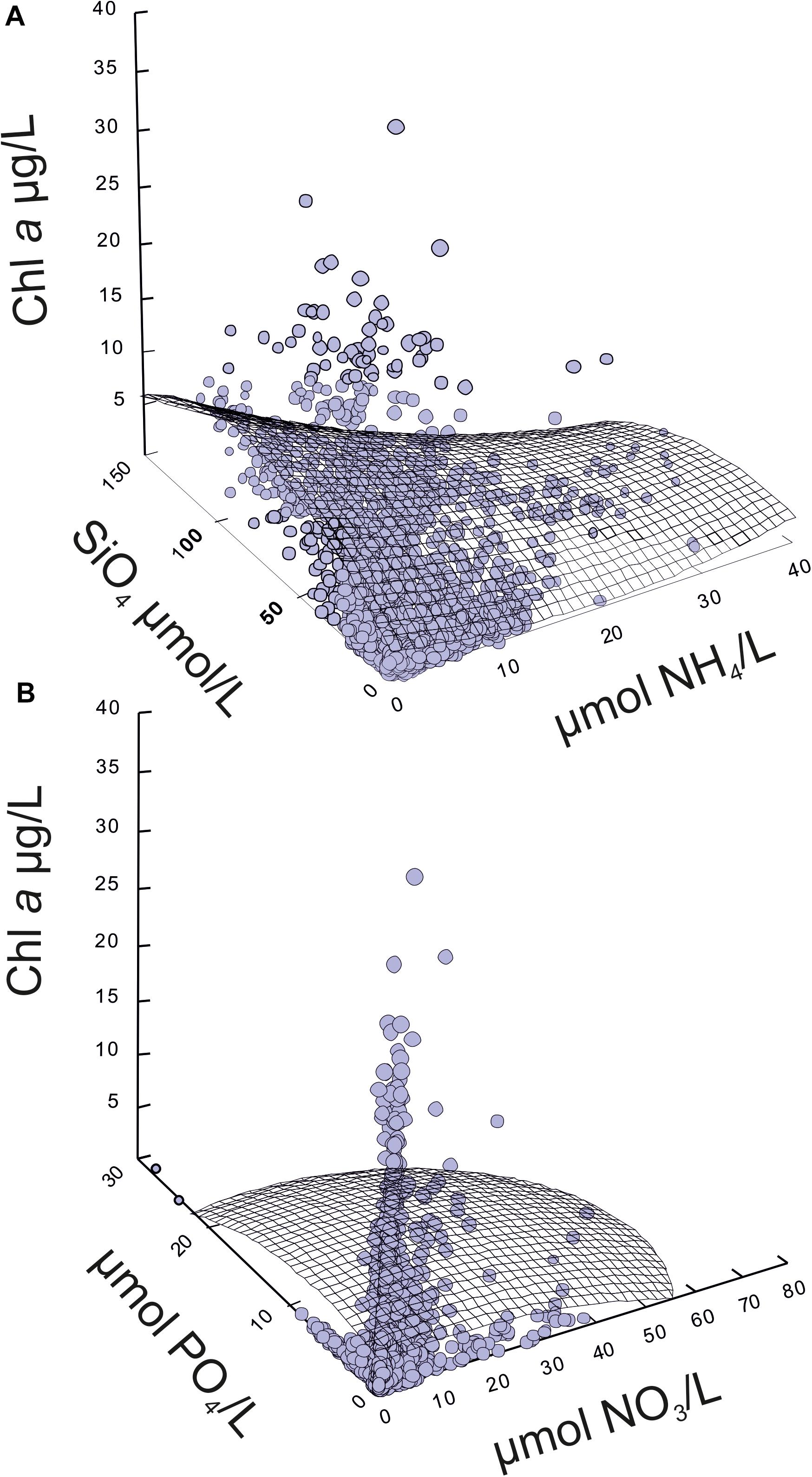
Figure 8. Relationship between chlorophyll a concentration and nutrients in the water column in the Mar Menor lagoon during the eutrophication process from 1997 to 2018. (A) Regression model using silicate and ammonium as independent variables. (B) Regression model using phosphate and nitrate as independent variables.
These complex patterns are consistent with trophic web regulations on chlorophyll a and suggests that zooplankton produces ammonium at while grazing on phytoplankton. However, when nitrate is low and the production of ammonium is high during the Recovery phase, the phytoplankton can grow using this last nutrient.
Regarding meteorological and hydrographical conditions affecting chlorophyll a concentration in the water column, only the average wind speed of the day of sampling showed positive influence, while maximum wind speed in the same day showed negative coefficient. This implies that while a moderate and sustained agitation of the water column favors planktonic primary production, wind storms produce the opposite effect. At the scale of localities, only salinity and turbidity showed a significant positive relationship with chlorophyll a. In the case of salinity, this is because the maximum chlorophyll a concentration tends to be higher in the confined areas, especially in the Break phase. Chlorophyll a shows especially low values in the areas of direct influence of the watercourses and El Estacio inlet, in which the salinity is low due to the inputs of fresh and less salty Mediterranean waters, respectively (Figures 6D–F). In the case of turbidity, the positive correlation can be explained by the direct effect of the chlorophyll itself on this parameter.
The first two axes of the PCA explain a cumulative 49.87% of the variance (Figure 7). PC1 axis shows 29.48% and is related to high water transparency and high ichthyoplankton species richness and abundance in the positive part, and high nutrient concentration, mainly , and Chl a in the negative one. It clearly separates Break and Prebreak phases. Recovery samples, being partially overlapping Break situation, are displaced toward Prebreak conditions, overlapping them. PC2 axis, showing an additional 20% of the variance, discriminates mainly winter samples, with situations with higher DIN, ammonium, and N/P ratios in the positive part and spring and summer situations with high Chl a and in the negative one.
The opposite situation of ichthyoplankton richness and abundance versus chlorophyll a concentration along the PC1 axis suggests a top down control of the trophic web during the Prebreak and Recovery phases.
The water column transparency is mainly constrained by Chl a concentration, that explain up to 55% of Secchi disc visibility at a given locality. In fact, chlorophyll a is the only variable selected by the GLM regression model with forward selection of variables performed using 1188 samples of the complete matrix (Figure 9A). This percentage increased up to 57% when considering the campaigns’ matrix.
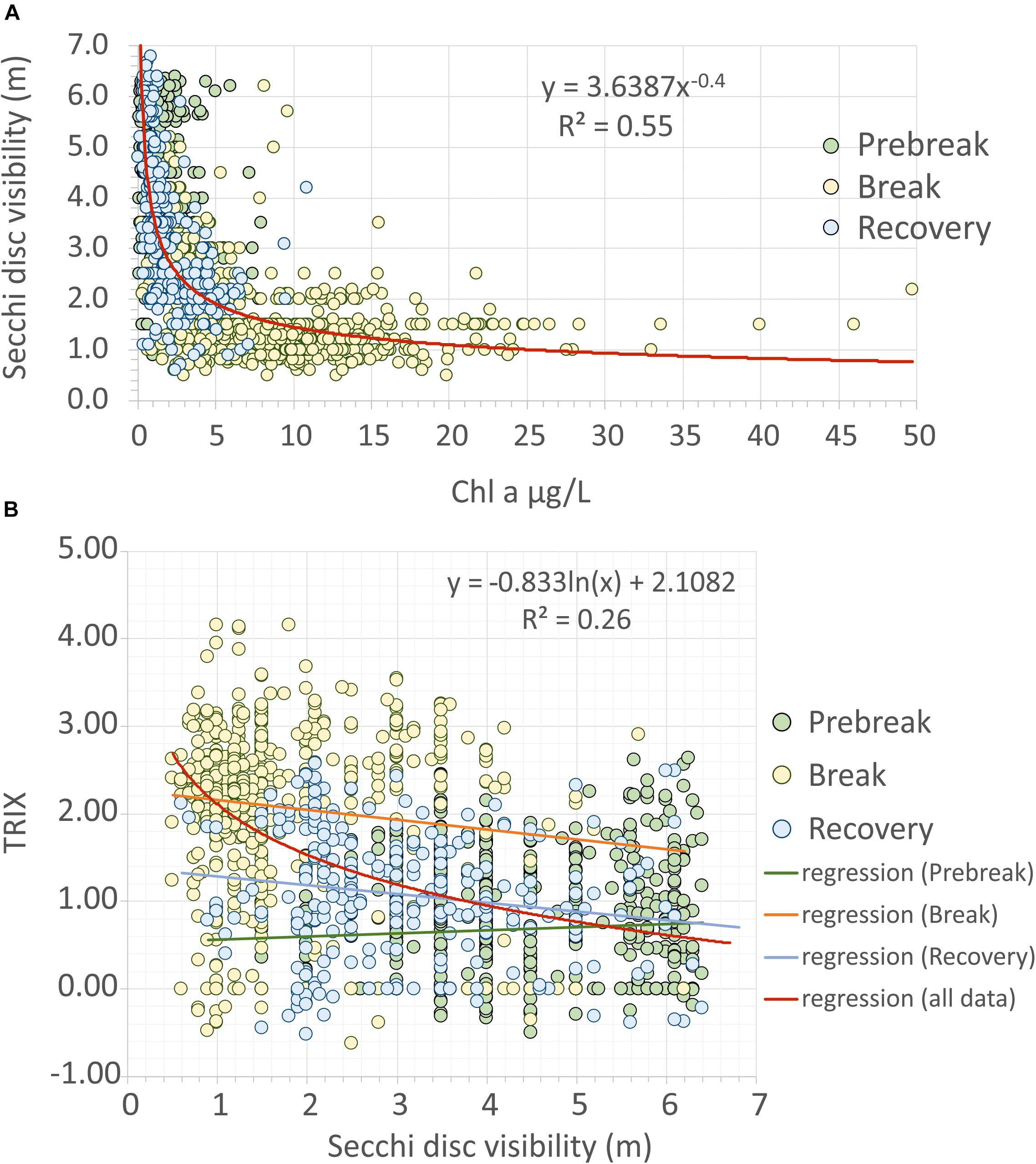
Figure 9. (A) Relationship between water column transparency measured by Secchi disc visibility depth and chlorophyll a concentration at each locality during the studied period (1997–2018); (B) relationship between TRIX trophic index and water column transparency using the same samples than in B. The color of the points represents the phase of the trophic state to which they belong. The red line corresponds to the regression model that best fits all the data. In figure B, the colored lines correspond to the linear regression considering the points belonging, respectively, to each of the phases of the trophic state.
In summary, Prebreak phase is characterized by high water transparency, the highest peaks of nitrates and phosphate and maximum values of nitrate, but also by the lowest concentrations of silicate, nitrites, ammonium, and chlorophyll a, despite the high nutrient loads in the Mar Menor. Break phase was characterized by the highest average nutrient and chlorophyll a concentration and, linked to this, the lowest average water transparency and the highest water extinction coefficient. It also showed the lowest average oxygen concentration. Finally, the Recovery phase has involved the lowest concentration of nitrate and phosphate and an improvement in the other water quality parameters compared to the Break phase. The activation of the regulation mechanisms seems to manifest through an ammonium production in the water column at the end of the Break phase and low phosphate concentration, probably as a consequence of the activity of ichthyoplankton and the rest of the trophic web.
Discussion
Phases of the Lagoon Eutrophication Process: Prebreak, Break of Water and Ecological Quality, and Recovery
Negative ecological and socioeconomic effects of eutrophication have been well known for a long time (Vollenweider, 1968; Vollenweider and Kerekes, 1981; United States Environmental Protection Agency [USEPA], 2001; Bricker et al., 2003; Smith, 2003; Jessen et al., 2015), and the concern for their consequences is increasing (Ménesguen and Lacroix, 2018). Moreover, recovery from an eutrophication process is usually difficult and long, and trajectories of impacted ecosystems tend not to be directly reversible, failing to return to the reference status even upon nutrient reduction (Duarte et al., 2008, 2013; Duarte, 2009; Carstensen et al., 2011; McCrackin et al., 2016).
In the Mar Menor, the process of eutrophication has shown three well-defined phases of very different duration that we have been able to observe and characterize (Figure 10).
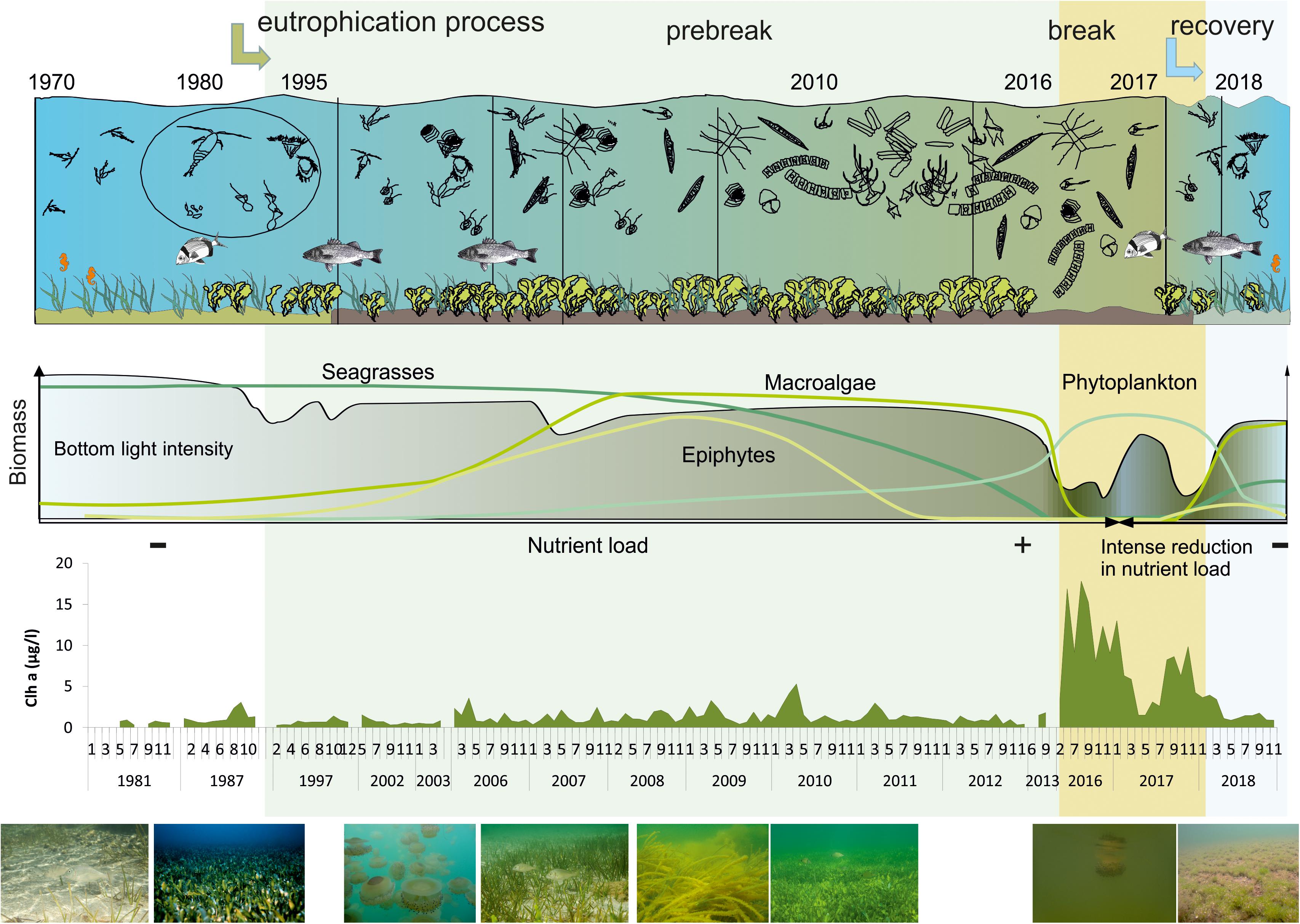
Figure 10. Synthesis of the evolution of the three phases of the eutrophication process in the Mar Menor lagoon and main changes occurred in the system during them.
The start of the process took place in the 1990s due to an increase in the entry of nutrients linked to the change of agricultural practices in the lagoon drainage basin (Pérez-Ruzafa et al., 2002). The comparison between data from 1988, prior to the start of the eutrophication process, and 1997 data, when the process was in its early stages with jellyfish proliferations, showed that the trophic status of the lagoon was changing. Nitrate concentration increased by one order of magnitude, from being always lower than 1 μM in 1988, to frequently having values higher than 4–6 μM in 1997, particularly during spring and summer (just at the harvest time, when the main irrigations occur in the drainage basin and larger amounts of fertilizers was used in the lagoon’s watershed) (Pérez-Ruzafa et al., 2005a). Furthermore, Pérez-Ruzafa et al. (2005a) found that, while previously to the eutrophication process the nitrate was mainly entering into the lagoon via runoff, as its concentration in the water column showed a positive and significant correlation with accumulated rainfall during the week prior to the sampling day, this positive relationship was lost during the eutrophication process, related to agricultural activity. Although peaks in nutrients inputs occurred, reaching up to 45 μM (Pérez-Ruzafa et al., 2005a), and still took place after runoff events, this general loss of correlation with rainfall is confirmed in our present work.
On the other hand, P concentrations suffered a sharp decline when the eutrophication process started compared with a decade before. This is explained by the fact that, at the end of 1990, a sanitation plan for urban wastes was established in the lagoon watershed, with the construction of a collector network and water treatment plants. This changed the input regime of this nutrient, moving from direct urban discards with a strongly seasonal pattern, associated with tourism and coast occupation during summer, to an occasional pattern, only when the torrential rains caused the closure of the wastewater treatment plants to avoid their overload (Pérez-Ruzafa et al., 2005a). At the same time, the increase in the nitrate load in the lagoon led to a shift from N- to P-limitation in primary production, maintaining phosphorous concentration permanently low due to its consumption by primary producers.
The highest nitrate concentrations were reached in the springs of 2006 and 2007 with average values exceeding 15 μmol /L, although the values returned to concentrations under 2 μmol /L during autumn and winter of the same years. The maximum concentrations of nutrients found in the lagoon water column at that moment were much lower than those measured in the runoff waters that discharge into the lagoon that account for a total input of 219 t N-/year, 30 t N-/year, and 52 t/year of total phosphorous (García-Pintado et al., 2007). This difference cannot be explained by an effect of dilution or export to the Mediterranean. The Mar Menor is a choked lagoon with a Water Renewal Time of 318 days (Umgiesser et al., 2014; Ghezzo et al., 2015; Pérez-Ruzafa et al., 2019a). Hydrodynamic models showed that in less than a year the Mar Menor waters would have reached 100% of the concentration contained in the Albujón watercourse waters, which during the Prebreak period represented el 50–60% of total discharges reaching the lagoon (Pérez-Ruzafa, 2010). Therefore, the difference in concentration must be largely attributed to nutrient incorporation into trophic networks through consumption by phytoplankton, macrophytes and microphytobenthos and the biogeochemical cycles inside the lagoon. In this way, surprisingly, despite the high inputs and the nitrate concentration values measured in 2006 and 2007, the mean concentration of chlorophyll a remained low during all the Prebreak phase (1.15 ± 0.02 μg/L) and similar to values recorded before starting the eutrophication process (1.02 ± 0.16 μg/L) (Gilabert, 2001b; Pérez-Ruzafa et al., 2005b; Figure 10).
When the nitrates began to enter in greater amounts in the 1990s, the role played by the jellyfish which proliferated at that time and by the ichthyoplankton resulted in a top-down control of the pelagic system maintaining the chlorophyll a concentration low (Pérez-Ruzafa et al., 2002, 2004, 2005a). This, added to the particular lagoon homeostatic mechanisms showed by the Mar Menor, stopped the main consequences of the eutrophication process for more than 20 years. Such mechanisms are based on (1) a high spatio-temporal hydrographical and biological heterogeneity (Pérez-Ruzafa et al., 2005a, 2007), induced by a restricted connectivity with the Mediterranean sea (Pérez-Ruzafa, 2015; Pérez-Ruzafa et al., 2018, 2019a), (2) a high benthic micro and macrophytes production and filter feeders, scavengers, and detritivores biomass (Pérez-Ruzafa, 1989), and (3) the accumulation of excess production in the sediments or its export out of the system through fisheries and migratory species (Pérez-Ruzafa et al., 2018).
Although some lesser events had already occurred in 2009 and 2010 with chlorophyll peaks exceeding 5 μg/L, the eutrophication crisis and Break phase became evident in 2016 with the sudden increase in chlorophyll a concentration and the generalized loss of water quality in the whole lagoon. During this Break phase, mean chlorophyll a concentration was 7.79 ± 0.22 μg/L, exceeding frequently 10 μg/L.
This translated in a drastic increase of the light extinction coefficient, reaching during the Break phase an average value of 1.24 ± 0.02 /m, that led to the loss of 81% of the macrophyte meadows below 2 m depth compared to the areas estimated in 2008 and 2014 (Pérez-Ruzafa et al., 2012; Belando et al., 2017). This produced a strong social concern and political sensitivity (Limón, 2016) that resulted in the adoption of urgent measures to stop spills of agricultural origin.
According to reported data (Esamur, 2018), from April 2017 to October 2018, the average flow to the Mar Menor through the Albujón water course was reduced to 5.89 ± 1.32 L/s and a load of 38.76 t /year, representing a reduction of about 97.55% of the flow and 82.3% of nitrate loads regarding the Prebreak and Break periods (García-Pintado et al., 2007; Pérez-Ruzafa, 2010).
At the end of the Recovery phase, mean chlorophyll a concentration was back to levels lower than 2 μg/L (0.94 ± 0.04 μg/L in the last sampled season, Autumn 2018, when light extinction coefficient was 0.42 ± 0.02 /m).
The start of regulation mechanisms in the trophic web, both precluding the chlorophyll a proliferation in 2009 and 2010, and helping the system recover after the 2016 crises, seems to manifest through an ammonium production in the water column, probably as a consequence of the activity of the herbivores and the highest levels of the trophic web. In fact, during the eutrophication process, fishing yields tended to increase in the Mar Menor (Marcos et al., 2015).
The climatic conditions, so far, have not had a major influence on the overall process. Although there are seasonal and inter-annual significant differences in the meteorological and hydrographic parameters, these do not show significant differences between the Prebreak, Break, and Recovery phases of the eutrophication process. Only mean wind velocity in the sampling day had some positive influence on chlorophyll a concentration while wind reaching 15–25 m/s has negative influence. The low or negative relationship between chlorophyll a and nutrients concentration, mainly at small spatio-temporal scales and high nutrient concentrations, is in disagreement with the traditional eutrophication models in which a positive response of chlorophyll a concentration is expected when nutrient concentration increases. This suggests the existence of complex patterns and regulatory mechanisms as proposed by Cloern (2001) or Nixon et al. (2001).
Detecting Eutrophication in Coastal Lagoons
The use of indicators of ecological status in coastal lagoons faces several difficulties. As they are subject to frequent environmental fluctuations and extreme conditions, their functioning in pristine conditions may resemble that of systems under anthropic pressure. Therefore, they are expected to be dominated by opportunistic and r strategist species, giving rise to what has been called the “estuarine quality paradox” (Dauvin, 2007; Elliott and Quintino, 2007; Dauvin and Ruellet, 2009). In any case, this approach for estuaries may not be fully applicable in coastal lagoons (Pérez-Ruzafa et al., 2011b,c, 2013). At the same time, the dynamics of chlorophyll a and nutrients in coastal lagoons can show a high spatio-temporal variability that depends on the observation scale, with a significant space × time interaction and with frequent lags, which complicates the design of sampling programs (Pérez-Ruzafa et al., 2005a, 2007).
Furthermore, these ecosystems show a higher than expected homeostatic capability, showing frequently negative or complex relationships between chlorophyll a concentration and nutrients. So, it has already been established that, contrary to freshwater ecosystems, nutrient concentration is not a good indicator of eutrophication in coastal lagoons, and the same would happen for estuaries and marine coastal areas (Cloern, 2001; Nixon et al., 2001; Boesch, 2002; Bricker et al., 2003; Pérez-Ruzafa et al., 2005a).
This makes it essential to find indicators that detect the critical points of the eutrophication process: (1) in the phases in which the ecosystem is subject to pressures but the functioning of homeostatic mechanisms prevents finding evident deviations in traditional indicators like nutrients or chlorophyll a concentration and (2) in the vicinity of the break points, before the whole system shifts to an alternative steady state and therefore it would be too late to take management measures (Ferreira et al., 2011; Pérez-Ruzafa and Marcos, 2015; Pérez-Ruzafa et al., 2018).
The TRIX index, proposed by Vollenweider et al. (1998), is widely used as an indicator of eutrophication. According to Giovanardi and Vollenweider (2004) and Penna et al. (2004), values ranging from 0 to 4 correspond to high-quality, 4–5 to good, 5–6 to moderate, and 6–10 to degraded conditions. Following these authors, values lower than 4 TRIX units are instead associated with scarcely productive coastal waters, while values lower than 3 are usually found in the open sea. In our work, although this index showed a negative relationship with water transparency (Figure 9B), from 3363 sampling data during the three phases of the eutrophication process, only four samples showed values higher than 4 (ranging between 4.11 and 4.16), therefore indicating high water quality in all the lagoon during the period considered. These highest values were reached in November 2016, just after the starting of the Break phase. This is evidence that, according to the current score scale, it was not able to detect the pressures during the previous 20 years (Salas et al., 2008) nor to anticipate the break point.
Factors of Risk, Resistance, and Resilience to Eutrophication in Coastal Lagoons
Extended shallow enclosed water bodies that have long water residence times are often mentioned among the ecosystems with the highest risk to suffer eutrophication processes (Ferreira et al., 2011). Coastal lagoons have these exact characteristics. In addition, they tend to have multiple and intense freshwater influences, directly through rivers, watercourses, or surface runoff and by direct or indirect anthropic discharges, without forgetting the possible influence of groundwater, which may be especially important in many lagoons as a promoting and/or mitigating agent (Jiménez-Martínez et al., 2016; Erostate et al., 2018; Rodellas et al., 2018). All these vectors are capable of transporting nutrients derived from human activity. In addition, it should be noted that the reduction of salinity can limit the influence of marine species in the trophic webs and that this can affect the homeostatic capabilities of the ecosystem (Pérez-Ruzafa et al., 2011c). Furthermore, it must be taken into account that freshwater ecosystems usually have positive proportional and direct responses to the entry of nutrients, increasing quickly their chlorophyll a concentration (Cloern, 2001; Nixon et al., 2001).
However, some of these risk conditions must be reviewed and put into the context of the operation of complex systems. The Mar Menor has a very high water residence time in comparison with other coastal lagoons (Umgiesser et al., 2014; Ghezzo et al., 2015). Lagoons like Venice, with an area of 436 km2, have a water residence time of only 12 days, Curonian in the Baltic (1584 km2) reaches 152 days, while in the Mar Menor, with only 136 km2, it reaches 318 days. Despite this, the Mar Menor traditionally showed a great resistance and resilience to the process of eutrophication, and it is one of the few lagoons in which the transparency of the waters is part of its idiosyncrasy and is one of the key elements of its tourist appeal.
In the Mar Menor lagoon, Pérez-Ruzafa et al. (2005a) already found that correlations between chlorophyll a concentrations and environmental variables were in disagreement with traditional eutrophication models (Vollenweider, 1968; Vollenweider and Kerekes, 1981; Vollenweider et al., 1998) in which a positive response of chlorophyll a concentration is expected when nutrient concentration increases. Relationships between chlorophyll a and nutrients found in Pérez-Ruzafa et al. (2005a) were negative when daily data were considered in the analyses. However, at longer time scales (monthly or seasonal means) or when the analyses considered time lags, the relationships between chlorophyll a and nutrients became positive. This suggests a very rapid response of primary producers to nutrient enrichment in the short-term (days to week) when phytoplankton consume and maintain low nutrient concentrations, but framed in a general context where nutrient inputs favor primary production and determine chlorophyll concentration and algal biomass in the long-term.
In the present study, with larger temporal data series, chlorophyll a showed a general positive relationship with nitrate and phosphate concentration, but this positive correlation only happens at very low concentration of these nutrients, turning this relation to negative at higher concentrations of the same (Figure 8).
Considering two different years at the start of the eutrophication process, in the Prebreak phase, Pérez-Ruzafa et al. (2005a) also found a significant correlation between chlorophyll a concentration and fish larvae density, suggesting a top-down control of fish larvae on main planktonic grazers in a seasonal limit cycle. In the present work, where a longer time period that includes the three described phases of the eutrophication process is considered, this relationship becomes antagonist in the ordination diagrams (Figure 7), linking chlorophyll a to high nutrient concentrations and low water quality, while ichthyoplankton is in the opposite part of the first axis, linked to the highest water quality and transparency.
The lack of relationship between the nutrients input and the concentration of chlorophyll a in the water column is, more than an exception, a common situation in coastal lagoons and shallow coastal habitats (Fitzpatrick and Meyers, 2000; Cloern, 2001; Nixon et al., 2001), where most of the primary production is carried out by phanerogams, epiphytic algae, drift and attached macroalgae, and epibenthic microalgae, rather than by phytoplankton (Nixon et al., 2001). Differences in the response of chlorophyll a concentration to nutrient loads between freshwater and estuarine and coastal ecosystems have been attributed to the higher hydrological complexity (Smith, 2003) and the spatial–temporal heterogeneity (Pérez-Ruzafa et al., 2005a) of the last ones. This does not preclude that total system production increases with nutrient enrichment at very low rates of input, and that even moderate levels of fertilization negatively affect seagrass (Nixon et al., 2001), or even that general rules can be applicable in any case (Smith, 2003). It becomes more evident if the response of chlorophyll a to nutrients is considered at large temporal scales (Pérez-Ruzafa et al., 2005a).
The homeostatic regulations that permit the coexistence of a high productivity and, at the same time, good water quality, and that provide this resistance in some coastal lagoons to show the effects of eutrophication, are probably based on two main mechanisms.
The first mechanism consists of channeling the production and main fluxes toward the benthic system, maintaining high biomass of primary producers (including macrophytes, cyanophycean, and benthic diatoms), filter feeders (sponges, cnidarians, polychaetes, bivalves, ascidians), and scavengers and detritivores (gastropods, polychaetes, crustaceans, holothurians) that remove organic matter and organisms from the water column and the sediment, exerting a top-down control over the pelagic community. In fact, it has long been recognized that filter feeding animals may exert “top down” controls that sometimes causes some systems to depart from the traditional simple eutrophication model maintaining low chlorophyll a concentration in the water column despite having high loads of DIN (Carpenter et al., 1987; Meeuwig et al., 1998; Nixon et al., 2001; Bruschetti et al., 2018). In the Mar Menor lagoon, during the Prebreak phase, this role was complemented from the pelagic system by the ichthyoplankton and jellyfish (Pérez-Ruzafa et al., 2002, 2005a). Furthermore, during the Recovery phase it has been observed a conspicuous increase in benthic species like the suspension feeders Filograna implexa (Berkeley, 1835), Branchiomma boholense (Grube, 1878), or Zoobotryon verticillatum (Delle Chiaje, 1822); the scavengers Hexaplex trunculus (Linnaeus, 1758) and Cyclope neritea (Linnaeus, 1758); and detritivorous like Holothuria (Roweothuria) poli (Delle Chiaje, 1824). Although it would be expected that, despite these promising results, the recovery of pelagic and benthic communities will take longer to occur, the fact is that this recovery had also begun to be evident at the end of our study. The Caulerpa prolifera meadow has begun to recover on part of the bottoms from which it had disappeared. Even in the areas that have not yet been recolonized by C. prolifera, other communities have settled, such as dense meadows of the new colonizer algae Dasycladus vermicularis (Scopoli) Krasser 1898, or large areas of Acetabularia calyculus J.V. Lamouroux, 1824 facies on gravel beds and sciaphilic communities under the pillars of the piers, which had almost disappeared from the Mar Menor for decades after the opening of El Estacio inlet or due to the dredging and dumping of sand on the beaches before the eutrophication process started (Supplementary Figure 1).
The second key mechanism is to export the surplus production outside the system, and this is done through the migration of numerous species that use the lagoons to grow, then returning to the open sea for reproduction, and the extraction of biomass by the aquatic birds and fisheries, the latter being one of the main human activities in coastal lagoons over the world taking advantage of these migratory patterns (Pérez-Ruzafa and Marcos, 2012).
There is a high heterogeneity in the ecosystems’ response to management actions to reduce eutrophication. Although in most coastal areas where nutrient inputs have been reduced the trends are mixed or responses are delayed (Boesch, 2002), in some coastal lagoons recovery times after cessation of nutrient inputs may be faster than expected but still responding to complex behavior at different time scales (Lenzi et al., 2003; Leruste et al., 2016). Chl a, TN, and TP can decrease strongly and quickly, while the planktonic community restructuring can take more time (Leruste et al., 2016). This may depend on how long the lagoon has been under eutrophic conditions. Coastal lagoons are spatially and temporally heterogeneous environments and their populations depend on colonization rates from the open sea through communication channels (Pérez-Ruzafa and Marcos, 1992, 1993). This is confirmed by the low rate of repetition of species when comparing lagoons (Basset et al., 2006; Pérez-Ruzafa et al., 2011c) and even in the composition of the species of the same lagoon. The evidence shows that the lagoon assemblages, both planktonic and benthic, change their species composition from one year to the next by 40–50% (Sigovini, 2011; Quispe, 2014). This confers a great resilience to the ecosystem if the key species that constitute the fixed elements have not disappeared completely during a too long stress process, as species colonizing each year have not suffered the environmental stress of previous years. In this context, the spatio-temporal variability of the environmental conditions and the multiplicity of spatial–temporal scales at which the processes take place in some lagoons suggests a greater probability that the effects of a crisis do not extend simultaneously to the entire ecosystem (Pérez-Ruzafa et al., 2005a). This functioning complexity would be related to restrictions on water flows and organisms imposed by communication channels that preclude homogeneity and would justify that relatively large lagoons, with low renewal rates, can maintain efficient homeostatic mechanisms (Pérez-Ruzafa, 2015).
Some authors have insisted that, in freshwater lakes, the only method that has proven successful in reducing the eutrophication is reducing input of phosphorus (Schindler, 2012; Schindler et al., 2016). Said authors even extend this claim to the coastal and estuarine ecosystems and insist that there is no documented case history of reducing nitrogen being successful. In the Mar Menor, nitrate and phosphorous seemed to alternate as limiting factors throughout the annual cycle (Pérez-Ruzafa et al., 2005a). In our long-term study, 53.8% of the samples showed N/P ratios higher than 16 and 19.8% higher than 100 (Figure 11). In these conditions, our study shows that decreasing nitrogen discharges is an effective measure to allow the start of the recovery of the ecological integrity and water quality of the lagoon ecosystem.
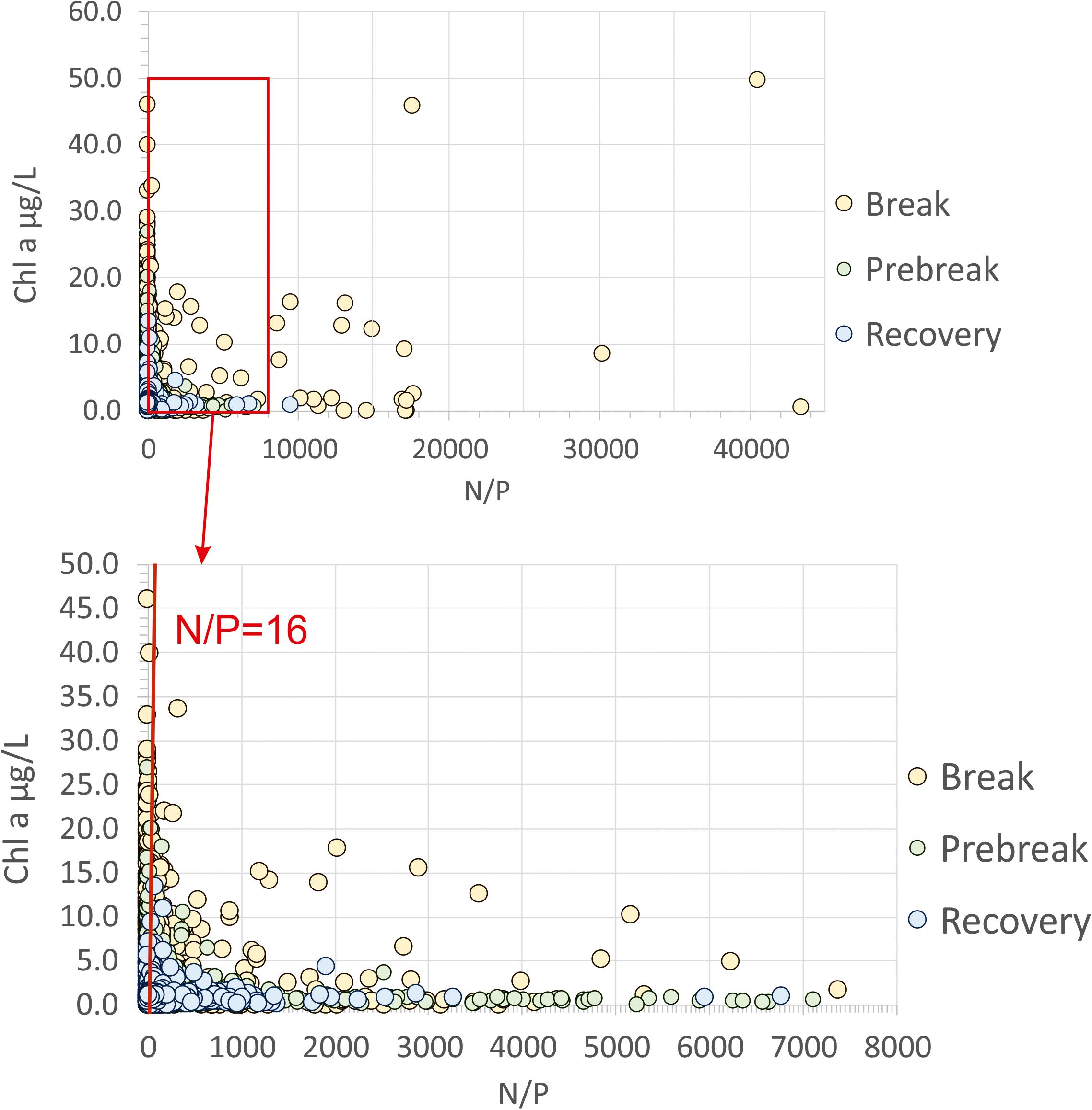
Figure 11. Distribution of chlorophyll a concentration data against N/P ratio values in the Mar Menor lagoon during the three phases of the eutrophication process (1997–2018). There is no significant relationship.
Reducing nutrient inputs could not be enough to reverse eutrophication if the organic matter accumulated for years in the sediments can release them continuously, prolonging the process (Nienhuis, 1992). However, there is evidence that this release may be scarce or, at least, lower than those required by biota, depending on lagoon conditions and that the anthropic discharges that tend to persist in spite of the management restrictions remain more important than the processes in the sediment. Orthophosphate may not be available for the primary producers as it can be absorbed by the carbonate skeletons and structures of benthic organisms, detritus, and clays (Dodge et al., 1984; De Jonge and Villerius, 1989; Lenzi et al., 1998), but P adsorption will decrease in conditions of organic matter accumulation, low redox potential, and sulfide accumulation in sediments (Lenzi et al., 1998; McGlathery et al., 2007). In warm coastal lagoons like the Mar Menor, high temperatures favor carbonate precipitation, helping to maintain low P concentration in the water column and retaining it in the sediments. In fact, during the eutrophication break, a strong proliferation of F. implexa has taken place occupying large extensions on muddy and sandy areas. At the same time, while after the dredging of El Estacio inlet in the early 1970s the quick expansion of C. prolifera meadows led to a rapid accumulation of organic matter, anoxia, and hydrogen sulfide production in the sediments (Pérez-Ruzafa et al., 2012), probably favoring the release of phosphorus, now, the strong regression of the C. prolifera meadows after the loss of water transparency in the summer of 2016 allowed the oxygenation of the surface layer of the sediments and its colonization by Cyanophyceae and benthic diatoms facilitating the retention of phosphorus and maintaining the nutrient uptake capacity of the vegetation. To understand in detail the distinct behavior of different coastal lagoons in response to management actions to activate oligotrophication, we need a detailed knowledge of the biogeochemical processes in the upper layers of the sediment under different environmental, hydrodynamic, and biological conditions, as well as of the balance between the nutrient fluxes from sediment to water column and the nutrient removal by benthic vegetation.
Conclusion
Although coastal lagoons are highly productive ecosystems, and considered simple, naturally stressed and prone to suffer dystrophic crises, some lagoons such as the Mar Menor are able to maintain high water quality for decades despite receiving a high load of nutrients. However, when the pressures are excessive, the system collapses and loses its equilibrium abruptly, following the traditional steps of the eutrophication process. This implies not only the loss of water quality, but the disappearance of the macrophytes meadows in the deep zones (Havens et al., 2001). The recovery after an eutrophication process is usually considered difficult and long, and not directly reversible even upon nutrient reduction (Duarte et al., 2008, 2013; Duarte, 2009; Carstensen et al., 2011; McCrackin et al., 2016). However, in the Mar Menor, after the adoption of measures leading to the reduction of nutrients input 1 year after the system breaks, the recovery of equilibria in the ecosystem has been faster than expected. After less than 2 years the quality of the conditions in the water column had recovered.
The change from oligotrophic status to the start of eutrophication process (Prebreak phase) was characterized by a shift in the planktonic system from assemblages dominated by small flagellates in winter, and diatoms and dinoflagellates the rest of the year, to assemblages constituted by large diatoms present throughout the year, but still maintaining low phytoplankton biomass and without any significant increase in chlorophyll a concentration. The maintenance of good water quality was due to a top-down control of the trophic web exerted through the proliferation of jellyfish. During the Break phase, the water column became dominated by dense populations of Synechococcus spp., and the depth of visibility in the water column was reduced from around 6 m to less than 0.5 m, whereby the compensation depth was above the average depth of the lagoon (4.5 m), leading to the mortality of the C. prolifera–C. nodosa mixed meadows located at more than 2 m depth.
Throughout the process of eutrophication and recovery, the system was alternatively limited by N and P, with a certain seasonal pattern and with a domain of limitation by phosphorus. This P limitation is determined by the scarce inputs from the watershed and the quick consume by the primary producers of that inputs and of any possible flows from the sediment. Despite this, the reduction in nitrogen load has been sufficient for the system to initiate an oligotrophication process.
The low ratio between chlorophyll a and nutrients concentration, mainly at small spatio-temporal scales, contrasts with the traditional eutrophication models, and suggests a rapid response of primary producers to nutrient inputs and a zooplankton control in the short-term, which in turn is controlled by the rest of the trophic web components.
Beyond the recovery of nutrient and chlorophyll a levels and water transparency, the good ecological status must involve the recovery of the structure of the pelagic and benthic communities. Although this process is usually slower, a significant recovery of those communities has also been observed in the Mar Menor and it is in the process of being studied (Supplementary Figure 1).
The mechanisms that favor homeostatic regulation, resilience, and rapid recovery involve top-down control of the trophic webs exerted by ichthyoplankton and jellyfish in the pelagic system and by the benthic food web. The efficiency of such mechanisms may be related to some of the characteristics of coastal lagoons. In the first place, the restricted connectivity between the lagoon and sea, determined by the inlets, prevents the homogenization of the system and favors a high hydrodynamic and biological heterogeneity and a high turnover in species composition, that in coastal lagoons is highly dependent from colonization rates, at multiple spatial and temporal scales. Furthermore, the primary production of the Mar Menor is mainly based on macrophytes and benthic microphytes, and the trophic network channels the production to the benthic system that maintains very high biomass of filter feeders, detritivores, and scavengers. Finally, excess production is accumulated in the sediment or exported out of the system through exploitation by water birds, and through migratory species, which are the main targets of fishing activity. All this favors that the nutrients, both those coming from external sources and from the biogeochemical cycles in the sediment, are quickly captured by the benthic macrophytes and the microphytobenthos, avoiding the proliferation of phytoplankton. In addition, any growth of phytoplankton based on any surplus is controlled by the pelagic trophic web and by the benthic filter feeders, thus maintaining low levels of nutrients and chlorophyll.
The need to detect changes in the trophic status of the ecosystem and, above all, to anticipate them, requires indicators that are sensitive both to gradual changes and breakpoints of equilibria. Although the assessment of the good ecological status of coastal lagoons in the context of the EU WFD also includes indicators of the structure of the communities, these tend to manifest themselves when the deterioration has already occurred. In this sense, the indicators of the water column and the pelagic system can be more agile and sensitive to detect changes and anticipate consequences. However, although the TRIX index shows some sensitivity to the trophic and water quality changes, its current score does not allow to anticipate or alert the eutrophication risk and the trophic breakpoint of the system. Therefore, it would be necessary to find other indicators that allow performing this function. Furthermore, to conclude about reversion ecological status of coastal lagoon ecosystems, it is advisable to use more than one bioindicator, covering different hierarchic levels of organization and benthic and pelagic systems as they can be complementary and important to understand the eutrophication and oligotrophication processes.
Author Contributions
SC, JF-P, AG-L, MG-O, HI, PCN-M, JQ-B, AS-M, and OS performed most of the field work and laboratory analyses. MP-M and IP-R have been involved, together with the other authors, in the analysis and interpretation of the results. AP-R and CM coordinated the different projects involved in this work, designed and supervised the study, performed the analyses of data, and wrote the draft of the manuscript together with the contributions of all other authors.
Funding
This work has been funded by the Spanish Ministry of Economy and Competitiveness project ConnectMar CTM 2014-56458-R. Data acquisition during the last 22 years has been partially supported by several projects including Eutrocost project CGL2004-06891 (National R&D Program, Ministry for Education and Science, Spain) and different agreements with distinct departments of the Región de Murcia Government, among others: “Sistema de Monitorización Costera para el Mar Menor” (Murcia Region Science and Technology Program 2007–2010) and the contract “Monitorización y modelado de la calidad de aguas y estado ecológico del Mar Menor y prevención de impactos” (Regional Ministry of Employment, Universities, Business and Environment of the Murcia Region).
Conflict of Interest Statement
The authors declare that the research was conducted in the absence of any commercial or financial relationships that could be construed as a potential conflict of interest.
Acknowledgments
We thank the referees for their constructive and stimulating comments that have helped to significantly improve the manuscript. We also thank to the Club Náutico de Lo Pagán for harbor facilities and the Agencia Española de Meteorología (AEMet) and Organismo Público Puertos del Estado for the meteorological and hydrographical data provided, respectively.
Supplementary Material
The Supplementary Material for this article can be found online at: https://www.frontiersin.org/articles/10.3389/fmars.2019.00026/full#supplementary-material
FIGURE S1 | Aspect of some benthic communities found in the bottoms of Mar Menor in summer and autumn of 2018, at the end of the Recovery phase. (a) Cymodocea nodosa meadows highly epiphyted. (b) Caulerpa prolifera meadow on mixed substrata with Alsidium corallinum and Chondrophycus tenerrimus. (c) Mixed meadow of C. prolifera and C. nodosa on sand. (d) Dense meadows of the algae Dasycladus vermicularis recently appeared in the Mar Menor during the Recovery phase after the regression of the C. prolifera meadows. (e) Low epiphyted C. nodosa meadows on sand. (e) Monospecific C. prolifera meadow colonizing infralittoral rock. (f) Monospecific C. prolifera meadow with colonies of Zoobotrion verticillatum. (g) Facies of Acetabularia calyculus on infralittoral sand, pebbles, and gravel. (i–q) Sciaphilic communities with sponges, cnidarians, polychaetes, bryozoans, and ascidians on protected shallow infralittoral rock, poorly illuminated under the pillars of piers.
References
Álvarez-Rogel, J., Jiménez-Cárceles, F. J., and Nicolás, C. E. (2006). Phosphorus and Nitrogen content in the water of a coastal wetland in the Mar Menor lagoon (SE Spain): relationships with effluents from urban and agricultural areas. Water Air Soil Pollut. 173, 21–38. doi: 10.1007/s11270-005-9020-y
Amanieu, M., Baleux, B., Guelorguet, O., and Michel, P. (1975). Etude biologique et hydrologique d’une crise dystrophique (malaigue) dans l’étang du Prévost a Palavas (Hérault). Vie Milieu 25, 175–204.
Anderson, M. J. (2001). A new method for non-parametric multivariate analysis of variance. Austral Ecol. 26, 32–46.
Anderson, M. J. (2005). PERMANOVA: a FORTRAN Computer Program for Permutational Multivariate Analysis of Variance. Auckland: University of Auckland, 24.
Anthony, A., Atwood, J., August, P., Byron, C., Cobb, S., Foster, C., et al. (2009). Coastal lagoons and climate change: ecological and social ramifications in U.S. Atlantic and Gulf coast ecosystems. Ecol. Soc. 14:8. doi: 10.5751/ES-02719-140108
Bachelet, G., De Montaudouin, X., Auby, I., and Labourg, P. J. (2000). Seasonal changes in macrophyte and macrozoobenthos assemblages in three coastal lagoons under varying degrees of eutrophication. ICES J. Mar. Sci. 57, 1495–1506. doi: 10.1006/jmsc.2000.0902
Barbier, E. B. (2015). Valuing the storm protection service of estuarine and coastal ecosystems. Ecosyst. Serv. 11, 32–38. doi: 10.1016/j.marpolbul.2016.01.033
Barbier, E. B., Acreman, M. C., and Knowler, D. (1997). Valoración Económica de los Humedales – Guía Para Decisores y Planificadores. Gland: Oficina de la Convención de Ramsar.
Basset, A., Elliott, M., West, R. J., and Wilson, J. G. (2013). Estuarine and lagoon biodiversity and their natural goods and services. Estuar. Coast. Shelf Sci. 132, 1–4. doi: 10.1016/j.ecss.2013.05.018
Basset, A., Galuppo, N., and Sabetta, L. (2006). Environmental heterogeneity and benthic macroinvertebrate guilds in Italian lagoons. Transit. Water Bull. 1, 48–63.
Belando, M. D., Bernardeau-Esteller, J., García-Muñoz, R., Ramos-Segura, A., Santos-Echeandía, J., García-Moreno, P., et al. (2017). Evaluación del Estado de Conservación de las Praderas de Cymodocea nodosa en la Laguna Costera del Mar Menor. 2014-2016. Murcia: Instituto Español de Oceanografía y Asociación de Naturalistas del Sureste, 157.
Boesch, D. F. (2002). Challenges and opportunities for science in reducing nutrient over-enrichment of coastal ecosystems. Estuaries 25, 886–900. doi: 10.1007/bf02804914
Boutiere, H., Bovee, F., Delille, D., Fiala, M., Gros, C., and Jacques, G. (eds). (1982). Effect D’une Crise Dystrophique dasn l’étang de Salses-Leucate. Oceanol. Acta, Actes Symposium International sur les Lagunes Cotieres. Bordeaux: UNESCO, 31–242.
Boynton, W. R., Murray, L., Hagy, J. D., Stokes, C., and Kemp, W. M. (1996). A comparative analysis of eutrophication patterns in a temperate coastal lagoon. Estuaries Coast. 19, 408–421. doi: 10.2307/1352459
Bricker, S., Ferreira, J., and Simas, T. (2003). An integrated methodology for assessment of estuarine trophic status. Ecol. Modell. 169, 39–60. doi: 10.1016/s0304-3800(03)00199-6
Bruschetti, C. M., Addino, M., Luppi, T., and Iribarne, O. (2018). Effects of nutrient enrichment and grazing by an invasive filter feeder on phytoplankton biomass in a South West Atlantic coastal lagoon. Biol. Invasions 20, 2245–2256. doi: 10.1007/s10530-018-1699-y
Carpenter, S. R., Kitchell, J. F., Hodgson, J. R., Cochran, P. A., Elser, J. J., Elser, M. M., et al. (1987). Regulation of lake primary productivity by food web structure. Ecology 68, 1863–1876. doi: 10.2307/1939878
Carstensen, J., Sánchez-Camacho, M., Duarte, C. M., Krause-Jensen, D., and Marbà, N. (2011). Connecting the dots: responses of coastal ecosystems to changing nutrient concentrations. Environ. Sci. Technol. 45, 9122–9132. doi: 10.1021/es202351y
Clarke, K. R., Gorley, R. N., Somerfield, P. J., and Warwick, R. M. (2014). Change in Marine Communities: an Approach to Statistical Analysis and Interpretation, 3nd Edn. Plymouth: PRIMER-E.
Cloern, J. E. (2001). Our evolving conceptual model of the coastal eutrophication problem. Mar. Ecol. Prog. Ser. 210, 223–253. doi: 10.3354/meps210223
Dauvin, J. C. (2007). Paradox of estuarine quality: benthic indicators and indices, consensus or debate for the future. Mar. Pollut. Bull. 55, 271–281. doi: 10.1016/j.marpolbul.2006.08.017
Dauvin, J. C., and Ruellet, T. (2009). The estuarine quality paradox: is it possible to define an ecological quality status for specific modified and naturally stressed estuarine ecosystems? Mar. Pollut. Bull. 59, 38–47. doi: 10.1016/j.marpolbul.2008.11.008
D’Avanzo, C., Kremer, J. N., and Wainright, S. C. (1996). Ecosystem production and respiration in response to eutrophication in shallow temperate estuaries. Mar. Ecol. Prog. Ser. 141, 263–274. doi: 10.3354/meps141263
De Jonge, V. N., and Villerius, L. A. (1989). Possible role of carbonate dissolution in estuarine phosphate dynamics. Limnol. Oceanogr. 34, 332–340. doi: 10.4319/lo.1989.34.2.0332
De Wit, R. (2011). “Biodiversity of coastal lagoon ecosystems and their vulnerability to global change,” in Ecosystems Biodiversity, eds O. Grillo and G. Venora (Rijeka: InTech), 29–40.
Desmit, X., Thieu, V., Billen, G., Campuzano, F., Dulière, V., Garnier, J., et al. (2018). Reducing marine eutrophication may require a paradigmatic change. Sci. Total Environ. 635, 1444–1466. doi: 10.1016/j.scitotenv.2018.04.181
Dodge, R. E., Jickells, T. D., Knap, A. H., Boyd, S., and Bak, R. P. M. (1984). Reef-building coral skeletons as chemical pollution (phosphorus) indicators. Mar. Pollut. Bull. 15, 178–187. doi: 10.1016/0025-326x(84)90317-5
Duarte, C. M. (2009). Coastal eutrophication research: a new awareness. Hydrobiologia 629, 263–269. doi: 10.1007/s10750-009-9795-8
Duarte, C. M., Borja, A., Carstensen, J., Elliott, M., Krause-Jensen, D., and Marbà, N. (2013). Paradigms in the recovery of estuarine and coastal ecosystems. Estuar. Coasts 38, 1202–1212. doi: 10.1007/s12237-013-9750-9
Duarte, C. M., Conley, D. J., Carstensen, J., and Sánchez-Camacho, M. (2008). Return to neverland: shifting baselines affect eutrophication restoration targets. Estuar. Coasts 32, 29–36. doi: 10.1007/s12237-008-9111-2
Elliott, M., and Quintino, V. (2007). The estuarine quality paradox, environmental homeostasis and the difficulty of detecting anthropogenic stress in naturally stressed areas. Mar. Pollut. Bull. 54, 640–645. doi: 10.1016/j.marpolbul.2007.02.003
Erostate, M., Huneau, F., Garel, E., Lehmann, M. F., Kuhn, T., Aquilina, L., et al. (2018). Delayed nitrate dispersion within a coastal aquifer provides constraints on land-use evolution and nitrate contamination in the past. Sci. Total Environ. 644, 928–940. doi: 10.1016/j.scitotenv.2018.06.375
Esamur (2018). Realización de Aforos y Determinación del Contenido en Nitratos en la Desembocadura de la Rambla del Albujón. Available at: http://www.canalmarmenor.es/web/canalmarmenor/seguimiento-ambiental/historico/aforos
European Environment Agency [EEA] (2001). Eutrophication in Europe’s Coastal Waters. Topic Report 7. Copenhagen.
European Environment Agency [EEA] (2010). Ecosystem Accounting and the Cost of Biodiversity Losses. The Case of Coastal Mediterranean wetlands. EEA Technical Report 3/2010. Copenhagen.
Facca, C., Ceoldo, S., Pellegrino, N., and Sfriso, A. (2014). Natural recovery and planned intervention in coastal wetlands: Venice lagoon (Northern Adriatic Sea, Italy) as a case study. Sci. World J. 2014:968618. doi: 10.1155/2014/968618
Ferrari, I., Ceccherelli, V. U., Naldi, M., and Viaroli, P. (1993). Planktonic and benthic communities in a shallow-water dystrophic lagoon. Verh. Int. Ver. Limnol. 25, 1043–1047. doi: 10.1080/03680770.1992.11900318
Ferreira, J. G., Andersen, J. H., Borja, A., Bricker, S. B., Camp, J., Cardoso da Silva, M., et al. (2011). Overview of eutrophication indicators to assess environmental status within the European Marine Strategy Framework Directive. Estuar. Coast. Shelf Sci. 93, 117–131. doi: 10.1016/j.ecss.2011.03.014
Fitzpatrick, J. J., and Meyers, M. B. (2000). A critical look at criteria - A review of methodologies that determine nutrient criteria for coastal and estuarine systems. Water Environ. Technol. 35–59.
Gamito, S., Gilabert, J., Marcos, C., and Pérez-Ruzafa, A. (2005). “Effects of changing environmental conditions on lagoon ecology,” in Coastal Lagoons: Ecosystem Processes and Modeling for Sustainable Use and Development, eds I. E. Gönenç and J. P. Wolflin (Boca Ratón, FL: CRC Press), 193–229.
García-Pintado, J., Martínez-Mena, M., Barberá, G. G., Albaladejo, J., and Castillo, V. M. (2007). Anthropogenic nutrient sources and loads from a Mediterranean catchment into a coastal lagoon: mar Menor, Spain. Sci. Total Environ. 373, 220–239. doi: 10.1016/j.scitotenv.2006.10.046
Ghezzo, M., De Pascalis, F., Umgiesser, G., Zemlys, P., Sigovini, M., Marcos, C., et al. (2015). Connectivity in three European coastal lagoons. Estuar. Coasts 38, 1764–1781. doi: 10.1007/s12237-014-9908-0
Gilabert, J. (2001a). Seasonal plankton dynamics in a Mediterranean hypersaline coastal lagoon: the Mar Menor. J. Plankton Res. 23, 207–217. doi: 10.1093/plankt/23.2.207
Gilabert, J. (2001b). Short-term variability of the planktonic size structure in a Mediterranean coastal lagoon. J. Plankton Res. 23, 219–226. doi: 10.1093/plankt/23.2.219
Giordani, G., Azzoni, R., Bartoli, M., and Viaroli, P. (1997). Seasonal variations of sulphate reduction rates, sulphur pools and iron availability in the sediment of a dystrophic lagoon (Sacca di Goro, Italy). Water Air Soil Pollut. 99, 363–371. doi: 10.1007/BF02406876
Giordani, G., Zaldivar, J. M., and Viaroli, P. (2009). Simple tools for assessing water quality and trophic status in transitional water ecosystems. Ecol. Indic. 9, 982–991. doi: 10.1016/j.ecolind.2008.11.007
Giovanardi, F., and Vollenweider, R. A. (2004). Trophic conditions of marine coastal waters: experience in applying the Trophic Index TRIX to two areas of the Adriatic and Tyrrhenian seas. J. Limnol. 63, 199–218. doi: 10.4081/jlimnol.2004.199
Giusti, E., Marsili-Libellia, S., Renzi, M., and Focardi, S. (2010). Assessment of spatial distribution of submerged vegetation in the Orbetello lagoon by means of a mathematical model. Ecol. Modell. 221, 1484–1493. doi: 10.1016/j.ecolmodel.2010.02.019
Guyoneaud, R., De Wit, R., Matheron, R., and Caumette, P. (1998). Impact of macroalgal dredging on dystrophic crises and phototrophic bacterial blooms (red waters) in a brackish coastal lagoon. Oceanol. Acta 21, 551–561. doi: 10.1016/S0399-1784(98)80038-8
Havens, K. E., Hauxwell, J., Tyler, A. C., Thomas, S., McGlathery, K. J., Cebrian, J., et al. (2001). Complex interactions between autotrophs in shallow marine and freshwater ecosystems: implications for community responses to nutrient stress. Environ. Pollut. 113, 95–107. doi: 10.1016/S0269-7491(00)00154-8
Jessen, C., Bednarz, V. N., Rix, L., Teichberg, M., and Wild, C. (2015). “Marine eutrophication,” in Environmental Indicators, eds R. H. Armon and O. Hänninen (Dordrecht: Springer), 177–203.
Jiménez-Martínez, J., García-Aróstegui, J. L., Hunink, J. E., Contreras, S., Baudron, P., and Candela, L. (2016). The role of groundwater in highly human-modified hydrosystems: a review of impacts and mitigation options in the Campo de Cartagena-Mar Menor coastal plain (SE Spain). Environ. Rev. 24, 377–392. doi: 10.1139/er-2015-0089
Kennish, M. J., and Paerl, H. W. (2010). “Coastal lagoons. critical habitats of environmental change,” in Coastal Lagoons. Critical Habitats of Environmental Change, eds M. J. Kennish and H. W. Paerl (Boca Ratón, FL: CRC Press), 1–15. doi: 10.1201/EBK1420088304
Kjerfve, B., and Magill, K. E. (1989). Geographic and hydrographic characteristics of shallow coastal lagoons. Mar. Geol. 88, 187–199. doi: 10.1016/0025-3227(89)90097-2
Kralj, M., De Vittor, C., Comici, C., Relitti, F., Auriemma, R., Alabiso, G., et al. (2016). Recent evolution of the physical-chemical characteristics of a Site of National Interest – the Mar Piccolo of Taranto (Ionian Sea) – and changes over the last 20 years. Environ. Sci. Pollut. Res. 23, 12675–12690. doi: 10.1007/s11356-015-5198-8
Lenzi, M., Birardi, F., Calzolai, R., Finoia, M. G., Marcone, F., Nocciolini, S., et al. (2010). Hypertrophic lagoon management by sediment disturbance. Mar. Pollut. Bull. 61, 189–197. doi: 10.1016/j.marpolbul.2010.02.012
Lenzi, M., Costa, A., and Giannerini, S. (1998). Variazioni di dominanza nei popolamenti algali della laguna di Orbetello. Un’ipotesi che ne individua le cause. Atti della Societá toscana di Scienze Natuali. Mem. Ser. B 105, 35–43.
Lenzi, M., Palmieri, R., and Porrello, S. (2003). Restoration of the eutrophic Orbetello lagoon (Tyrrhenian Sea, Italy): water quality management. Mar. Pollut. Bull. 46, 1540–1548. doi: 10.1016/S0025-326X(03)00315-1
Leruste, A., Malet, N., Munaron, D., Derolez, V., Hatey, E., Collos, Y., et al. (2016). First steps of ecological restoration in Mediterranean lagoons: Shifts in phytoplankton communities. Estuar. Coast. Shelf Sci. 180, 190–203. doi: 10.1016/j.ecss.2016.06.029
Limón, R. (2016). El Mar Menor, al Borde del Colapso. La Fiscalía Investiga la Contaminación de la Zona, que se Encuentra en Situación Límite. El Pais, 07/07/2016. Available at: https://elpais.com/politica/2016/06/15/actualidad/1466007368_066035.html
López Bermúdez, F., Ramírez-Díaz, L., and Martín de Agar, P. (1981). Análisis integral del medio natural en la planificación territorial: el ejemplo del Mar Menor. Murcia 18, 11–20.
Marcos, C., Torres, I., López-Capel, A., and Pérez-Ruzafa, A. (2015). Long term evolution of fisheries in a coastal lagoon related to changes in lagoon ecology and human pressures. Rev. Fish Biol. Fish. 25, 689–713. doi: 10.1007/s11160-015-9397-7
Martelloni, T., Tomassetti, P., Gennaro, P., Vani, D., Persia, E., Persiano, M., et al. (2016). Artificial soft sediment resuspension and high density opportunistic macroalgal mat fragmentation as method for increasing sediment zoobenthic assemblage diversity in a eutrophic lagoon. Mar. Pollut. Bull. 110, 212–220. doi: 10.1016/j.marpolbul.2016.06.060
McCrackin, M. L., Jones, H. P., Jones, P. C., and Moreno-Mateos, D. (2016). Recovery of lakes and coastal marine ecosystems from eutrophication: a global meta-analysis. Limnol. Oceanogr. 62, 507–518. doi: 10.1002/lno.10441
McGlathery, K. J., Sundbäck, K., and Anderson, I. C. (2007). Eutrophication in shallow coastal bays and lagoons: the role of plants in the coastal filter. Mar. Ecol. Prog. Ser. 348, 1–18. doi: 10.3354/meps07132
Meeuwig, J. J., Rasmussen, J., and Peters, R. H. (1998). Turbid waters and clarifying mussels: their moderation of Chl: nutrient relations in estuaries. Mar. Ecol. Prog. Ser. 171, 139–150. doi: 10.3354/meps171139
Ménesguen, A., and Lacroix, G. (2018). Modelling the marine eutrophication: a review. Sci. Total Environ. 636, 339–354. doi: 10.1016/j.scitotenv.2018.04.183
Newton, A., Brito, A. C., Icely, J. D., Delorez, V., Clara, I., Angus, S., et al. (2018). Assessing, quantifying and valuing the ecosystem services of coastal lagoons. J. Nat. Conserv. 44, 50–65. doi: 10.1016/j.jnc.2018.02.009
Newton, A., Icely, J. D., Falcao, M., Nobre, A., Nunes, J. P., Ferreira, J. G., et al. (2003). Evaluation of eutrophication in the Ria Formosa coastal lagoon, Portugal. Cont. Shelf Res. 23, 1945–1961. doi: 10.1016/j.csr.2003.06.008
Nienhuis, P. H. (1992). Eutrophication, water management, and the functioning of Dutch estuaries and coastal lagoons. Estuaries 15, 538–548. doi: 10.2307/1352396
Nixon, S., Buckley, B., Granger, S., and Bintz, J. (2001). Responses of very shallow marine ecosystems to nutrient enrichment. Hum. Ecol. Risk Assess. 7, 1457–1481. doi: 10.1080/20018091095131
Nixon, S. W. (1995). Coastal marine eutrophication: a definition, social causes and future concerns. Ophelia 41, 199–219. doi: 10.1080/00785236.1995.10422044
Nixon, S. W. (2009). Eutrophication and the macroscope. Hydrobiologia 629, 5–19. doi: 10.1007/s10750-009-9759-z
Parsons, T. R., Maita, Y., and Lalli, C. M. (1984). A Manual of Chemical and Biological Methods for Sea-Water Analysis. Oxford: Pergamon Press, 173.
Penna, N., Capellacci, S., and Ricci, F. (2004). The influence of the Po River discharge on phytoplankton bloom dynamics along the coastline of Pesaro (Italy) in the Adriatic Sea. Mar. Pollut. Bull. 48, 321–326. doi: 10.1016/j.marpolbul.2003.08.007
Pérez-Ruzafa, A. (1989). Estudio Ecológico y Bionómico de los Poblamientos Bentónicos del Mar Menor (Murcia, SE de España). Doctoral thesis, Universidad de Murcia, Murcia.
Pérez-Ruzafa, A. (2010). Estudio de Dispersión de los Vertidos Existentes en la Laguna Costera del Mar Menor y los Análisis Coste-Eficacia de la no Aplicación de las Medidas Correctoras Oportunas Para la Mejora de la Calidad de las Aguas en Esta Laguna. Murcia: Consejeria de Agricultura y Agua.
Pérez-Ruzafa, A. (2015). El papel de la conectividad restringida en la construcción de los ecosistemas marinos semiaislados: el ejemplo de las lagunas costeras y los archipiélagos. Rev. Acad. Canaria Cienc. 27, 411–456.
Pérez-Ruzafa, A., and Aragón, R. (2002). “Implicaciones de la gestión y el uso de las aguas subterráneas en el funcionamiento de la red trófica de una laguna costera,” in Conflictos Entre el Desarrollo de las Aguas Subterráneas y la Conservación de los Humedales: Litoral Mediterráneo, eds J. M. Fornés and M. R. Llamas (Madrid: Fundación Marcelino Botín- Ediciones Mundi-Prensa), 215–245.
Pérez-Ruzafa, A., De Pascalis, F., Ghezzo, M., Quispe, J. I., Hernández-García, R., Muñoz, I., et al. (2019a). Connectivity between coastal lagoons and sea: asymmetrical effects on assemblages’ and population’s structure. Estuar. Coast. Shelf Sci. 216, 171–186. doi: 10.1016/j.ecss.2018.02.031
Pérez-Ruzafa, A., Fernández, A. I., Marcos, C., Gilabert, J., Quispe, J. I., and García-Charton, J. A. (2005a). Spatial and temporal variations of hydrological conditions, nutrients and chlorophyll a in a Mediterranean coastal lagoon (Mar Menor, Spain). Hydrobiologia 550, 11–27. doi: 10.1007/s10750-005-4356-2
Pérez-Ruzafa, A., Gilabert, J., Gutiérrez, J. M., Fernández, A. I., Marcos, C., and Sabah, S. (2002). Evidence of a planktonic food web response to changes in nutrient input dynamics in the Mar Menor coastal lagoon, Spain. Hydrobiologia 475/476, 359–369. doi: 10.1023/A:1020343510060
Pérez-Ruzafa, A., and Marcos, C. (1992). Colonization rates and dispersal as essential parameters in the confinement theory to explain the structure and horizontal zonation of lagoon benthic assemblages. Rapp. Commun. Int. Mer Médit. 33:100.
Pérez-Ruzafa, A., and Marcos, C. (1993). La teoría del confinamiento como modelo para explicar la estructura y zonación horizontal de las comunidades bentónicas en las lagunas costeras. Publ. Espec. Inst. Esp. Oceanogr. 11, 347–358.
Pérez-Ruzafa, A., and Marcos, C. (2012). Fisheries in coastal lagoons: an assumed but poorly researched aspect of the ecology and functioning of coastal lagoons. Estuar. Coast. Shelf Sci. 110, 15–31. doi: 10.1016/j.ecss.2012.05.025
Pérez-Ruzafa, A., and Marcos, C. (2015). “Monitoring heterogeneous and quick-changing environments: coping with spatial and temporal scales of variability in coastal lagoons and transitional waters,” in Coastal Ecosystems: Experiences and Recommendations for Environmental Monitoring, ed. M. Sebastiá (New York, NY: Nova Science Publishers, Inc.), 89–116.
Pérez-Ruzafa, A., Marcos, C., Bernal, C. M., Quintino, V., Freitas, R., Rodrigues, A. M., et al. (2012). Cymodocea nodosa vs. Caulerpa prolifera: causes and consequences of a long-term history of interaction in macrophyte meadows in the Mar Menor coastal lagoon (Spain, southwestern Mediterranean). Estuar. Coast. Shelf Sci. 110, 101–115. doi: 10.1016/j.ecss.2012.04.004
Pérez-Ruzafa, A., Marcos, C., and Gilabert, J. (2005b). “The ecology of the Mar Menor coastal lagoon: a fast-changing ecosystem under human pressure,” in Coastal Lagoons: Ecosystem Processes and Modeling for Sustainable Use and Development, eds I. E. Gönenç and J. P. Wolflin (Boca Ratón, FL: CRC Press), 392–422.
Pérez-Ruzafa, A., Marcos, C., Pérez-Marcos, M., and Pérez-Ruzafa, I. (2013). Are coastal lagoons physically or biologically controlled ecosystems? Revisiting r vs K strategies in coastal lagoons and estuaries. Estuar. Coast. Shelf Sci. 132, 17–33. doi: 10.1016/j.ecss.2012.04.011
Pérez-Ruzafa, A., Marcos, C., and Pérez-Ruzafa, I. M. (2009). “30 años de estudios en la laguna costera del Mar Menor: de la descripción del ecosistema a la comprensión de los procesos y la solución de los problemas ambientales,” in El Mar Menor. Estado Actual del Conocimiento Científico, ed. Instituto Euromediterráneo del Agua (Murcia: Fundación Instituto Euromediterráneo del Agua), 17–46.
Pérez-Ruzafa, A., Marcos, C., and Pérez-Ruzafa, I. M. (2011a). Mediterranean coastal lagoons in an ecosystem and aquatic resources management context. Phys. Chem. Earth 36, 160–166. doi: 10.1016/j.pce.2010.04.013
Pérez-Ruzafa, A., Marcos, C., and Pérez-Ruzafa, I. M. (2011b). Recent advances in coastal lagoons ecology: evolving old ideas and assumptions. Transit. Waters Bull. 5, 50–74.
Pérez-Ruzafa, A., Marcos, C., Pérez-Ruzafa, I. M., and Pérez-Marcos, M. (2011c). Coastal lagoons: “transitional ecosystems” between transitional and coastal waters. J. Coast. Conserv. 15, 369–392. doi: 10.1007/s11852-010-0095-2
Pérez-Ruzafa, A., Marcos, C., and Pérez-Ruzafa, I. M. (2018). “When maintaining ecological integrity and complexity is the best restoring tool: the case of the Mar Menor lagoon,” in Management and Restoration of Mediterranean Coastal Lagoons in Europe, Vol. 10, eds X. Quintana, D. Boix, S. Gascón, and J. Sala (Girona: Càtedra d’Ecosistemes litorals Mediterrànis i LIFE Pletera), 67–95.
Pérez-Ruzafa, A., Marcos, C., Pérez-Ruzafa, I. M., Barcala, E., Hegazi, M. I., and Quispe, J. (2007). Detecting changes resulting from human pressure in a naturally quick-changing and heterogeneous environment: spatial and temporal scales of variability in coastal lagoons. Estuar. Coast. Shelf Sci. 75, 175–188. doi: 10.1016/j.ecss.2007.04.030
Pérez-Ruzafa, A., Pérez-Ruzafa, I. M., Newton, A., and Marcos, C. (2019b). “Coastal lagoons: environmental variability, ecosystem complexity and goods and services uniformity,” in Coasts and Estuaries, the Future, eds E. Wolanski, J. Day, M. Elliott, and R. Ramesh (New York, NY: Elsevier), 253–276. doi: 10.1016/b978-0-12-814003-1.00015-0
Pérez-Ruzafa, A., Quispe-Becerra, J. I., García-Charton, J. A., and Marcos, C. (2004). Composition, structure and distribution of the ichthyoplankton in a Mediterranean coastal lagoon. J. Fish Biol. 64, 202–218. doi: 10.1111/j.1095-8649.2004.00301.x
Pérez-Ruzafa, A., Ros, J., Marcos, C., Ballester, R., and Pérez-Ruzafa, I. M. (1989). “Distribution and biomass of the macrophyte beds in a hypersaline coastal lagoon (the Mar Menor, SE Spain), and its recent evolution following major environmental changes,” in International Workshop on Posidonia Beds, Vol. 2, eds C. F. Bouderesque, A. Meinesz, E. Fresi, and V. Gravez (Marseille: GIS Posidonie), 49–62.
Quispe, J. I. (2014). Dinámica Espacio-Temporal del Ictioplancton del Mar Menor (SE España) y Factores Ambientales Asociados. Doctoral thesis, Universidad de Murcia, Murcia.
Ramsar Convention and Secretariat (2006). Manual de la Convención de Ramsar: Guía a la Convención Sobre los Humedales (Ramsar, Irán, 1971). Gland: Secretaría de la Convención de Ramsar.
Reyes, E., and Merino, M. (1991). Diel dissolved-oxygen dynamics and eutrophication in a shallow, well-mixed tropical lagoon (Cancun, Mexico). Estuaries 14, 372–381. doi: 10.2307/1352262
Rodellas, V., Stieglitz, T. C., Andrisoa, A., Cook, P. G., Raimbault, P., Tamborski, J. J., et al. (2018). Groundwater-driven nutrient inputs to coastal lagoons: the relevance of lagoon water recirculation as a conveyor of dissolved nutrients. Sci. Total Environ. 642, 764–780. doi: 10.1016/j.scitotenv.2018.06.095
Rodríguez-Gallego, L., Achkar, M., Defeo, O., Vidal, L., Meerhoff, E., and Conde, D. (2017). Effects of land use changes on eutrophication indicators in five coastal lagoons of the Southwestern Atlantic ocean. Estuar. Coast. Shelf Sci. 188, 116–126. doi: 10.1016/j.ecss.2017.02.010
Ros, M., and Miracle, R. M. (1984). Variación estacional del fitoplancton del Mar Menor y su relación con la de un punto próximo en el Mediterráneo. Limnética 1, 32–42.
Sakka Hlaili, A., Grami, B., Hadj Mabrouk, H., Gosselin, M., and Hamel, D. (2007). Fate of phytoplankton production in a restricted Mediterranean lagoon. Rapp. Commun. Int. Mer Médit. 38:391.
Salas, F., Teixeira, H., Marcos, C., Marques, J. C., and Pérez-Ruzafa, A. (2008). Applicability of the trophic index TRIX in two transitional ecosystems: the Mar Menor lagoon (Spain) and the Mondego estuary (Portugal). ICES J. Mar. Sci. 65, 1442–1448. doi: 10.1093/icesjms/fsn123
Schindler, D. W. (2012). The dilemma of controlling cultural eutrophication of lakes. Proc. R. Soc. B 279, 4322–4333. doi: 10.1098/rspb.2012.1032
Schindler, D. W., Carpenter, S. R., Chapra, S. C., Hecky, R. E., and Orihel, D. M. (2016). Reducing phosphorus to curb lake eutrophication is a success. Environ. Sci. Technol. 50, 8923–8929. doi: 10.1021/acs.est.6b02204
Sfriso, A., Pavoni, B., and Marcomini, A. (1995). Nutrient distributions in the surface sediment of the central lagoon of Venice. Sci. Total Environ. 172, 21–35. doi: 10.1016/0048-9697(95)04714-X
Sigovini, M. (2011). Multiscale Dynamics of Zoobenthic Communities and Relationships with Environmental Factors in the Lagoon of Venice. Venezia: Universitá Ca’Foscari.
Smith, V. H. (2003). Eutrophication of freshwater and coastal marine ecosystems a global problem. Environ. Sci. Pollut. Res. 10, 126–139. doi: 10.1065/espr2002.12.142
Solidoro, C., Bandelj, V., Aubry Bernardi, F., Camatti, E., Ciavatta, S., Cossarini, G., et al. (2010). “Response of Venice lagoon ecosystem to natural and anthropogenic pressures over the last 50 years,” in Coastal Lagoons: Systems of Natural and Anthropogenic Change, eds H. Paerl and M. Kennish (Taylor: CRC Press), 483–511. doi: 10.1201/EBK1420088304-c19
Specchiulli, A., D’Adamo, R., Renzi, M., Vignes, F., Fabbrocini, A., Scirocco, T., et al. (2009). Fluctuations of physicochemical characteristics in sediments and overlying water during an anoxic event: a case study from Lesina lagoon (SE Italy). Transit. Waters Bull. 3, 15–32.
Tagliapietra, D., Sigovini, M., and Volpi-Ghirardini, A. (2009). A review of terms and definitions to categorize estuaries, lagoons and associated environments. Mar. Freshw. Res. 60, 497–509. doi: 10.1071/MF08088
Taylor, D. I., Nixon, S. W., Granger, S. L., and Buckley, B. A. (1999). Responses of coastal lagoon plant communities to levels of nutrient enrichment: a mesocosm study. Estuaries 22, 1041–1056. doi: 10.2307/1353082
Terrados, J., and Ros, J. (1991). Production dynamics in a macrophyte-dominated ecosystem: the Mar Menor coastal lagoon (SE Spain). Oecol. Aquat. 10, 255–270.
Umgiesser, G., Ferrarin, C., Cucco, A., De Pascalis, F., Bellafiore, D., Ghezzo, M., et al. (2014). Comparative hydrodynamics of 10 Mediterranean lagoons by means of numerical modeling. J. Geophys. Res. Oceans 119, 2212–2226. doi: 10.1002/2013JC009512
United States Environmental Protection Agency [USEPA] (2001). Nutrient Criteria Technical Guidance Manual Estuarine and Coastal Marine Waters. Washington, DC: USEPA.
Vasconcelos, R. P., Reis-Santos, P., Costa, M. J., and Cabral, H. N. (2011). Connectivity between estuaries and marine environment: integrating metrics to assess estuarine nursery function. Ecol. Indic. 11, 1123–1133. doi: 10.1016/j.ecolind.2010.12.012
Velasco, A. M., Pérez-Ruzafa, A., Martínez-Paz, J. M., and Marcos, C. (2018). Ecosystem services and main environmental risks in a coastal lagoon (Mar Menor, Murcia, SE Spain): the public perception. J. Nat. Conserv. 43, 180–189. doi: 10.1016/j.jnc.2017.11.002
Viaroli, P., Azzoni, R., Bartoli, M., Giordani, G., and Tajé, L. (2001). “Evolution of the trophic conditions and dystrophic outbreaks in the Sacca di Goro lagoon (northern Adriatic Sea),” in Structure and Processes in the Mediterranean Ecosystems, eds F. M. Faranda, L. Guglielmo, and G. Spezie (Milan: Springer Verlag Italia), 443–451.
Viaroli, P., Bartoli, M., Bondavalli, C., Christian, R., Giordani, G., and Naldi, M. (1996). Macrophyte communities and their impact on benthic fluxes of oxygen, sulphide and nutrients in shallow eutrophic environment. Hydrobiologia 329, 93–103. doi: 10.1007/BF00034550
Viaroli, P., Bartoli, M., Giordani, G., Naldi, M., Orfanidis, S., and Zaldivar, J. M. (2008). Community shifts, alternative stable states, biogeochemical controls and feedback in eutrophic coastal lagoons: a brief overview. Aquat. Conserv. Mar. Freshw. Ecosyst. 18, S105–S117. doi: 10.1002/aqc.956
Viaroli, P., and Christian, R. R. (2003). Description of trophic status, hyperautotrophy and dystrophy of a coastal lagoon through a potential oxygen production and consumption index - TOSI: trophic oxygen status index. Ecol. Indic. 3, 237–250. doi: 10.1016/j.ecolind.2003.11.001
Viaroli, P., Nizzoli, D., Pinardi, M., Soana, E., and Bartoli, M. (2015). Eutrophication of the Mediterranean Sea: a watershed-cascading filter approach. Rend. Lincei Sci. Fis. Nat. 26, 13–23. doi: 10.1007/s12210-014-0364-3
Vollenweider, R. A. (1968). Scientific Fundamentals of the Eutrophication of Lakes and Flowing Waters, With Particular Reference to Nitrogen and Phosphorus as Factors in Eutrophication. OECD Technical Report DAS/CS/ 68.27. Paris.
Vollenweider, R. A., Giovanardi, F., Montanari, G., and Rinaldi, A. (1998). Characterization of the trophic conditions of marine coastal waters with special reference to the NW Adriatic Sea: proposal for a trophic scale, turbidity and generalized water quality index. Environmetrics 9, 329–357. doi: 10.1002/(SICI)1099-095X(199805/06)9:3<329::AID-ENV308>3.0.CO;2-9
Vollenweider, R. A., and Kerekes, J. J. (1981). Background and Summary Results of the OECD Cooperative Program on Eutrophication. In: Restoration of Lakes and Inland Waters. International Symposium on Inland Waters and Lake Restoration. EPA440/5-81-11. Portland, 25–36.
Vybernaite-Lubiene, I., Zilius, M., Giordani, G., Petkuviene, J., Vaiciute, D., Bukaveckas, P. A., et al. (2017). Effect of algal blooms on retention of N, Si and P in Europe’s largest coastal lagoon. Estuar. Coast. Shelf Sci. 194, 217–228. doi: 10.1016/j.ecss.2017.06.020
Keywords: coastal lagoons, transitional ecosystems, Mar Menor, eutrophication, resistance, resilience, recovery, oligotrophication
Citation: Pérez-Ruzafa A, Campillo S, Fernández-Palacios JM, García-Lacunza A, García-Oliva M, Ibañez H, Navarro-Martínez PC, Pérez-Marcos M, Pérez-Ruzafa IM, Quispe-Becerra JI, Sala-Mirete A, Sánchez O and Marcos C (2019) Long-Term Dynamic in Nutrients, Chlorophyll a, and Water Quality Parameters in a Coastal Lagoon During a Process of Eutrophication for Decades, a Sudden Break and a Relatively Rapid Recovery. Front. Mar. Sci. 6:26. doi: 10.3389/fmars.2019.00026
Received: 16 October 2018; Accepted: 21 January 2019;
Published: 11 February 2019.
Edited by:
Rodrigo Riera, Catholic University of the Most Holy Conception, ChileReviewed by:
Marcos Mateus, Universidade de Lisboa, PortugalFrancisco Leitão, Centro de Ciências do Mar (CCMAR), Portugal
Copyright © 2019 Pérez-Ruzafa, Campillo, Fernández-Palacios, García-Lacunza, García-Oliva, Ibañez, Navarro-Martínez, Pérez-Marcos, Pérez-Ruzafa, Quispe-Becerra, Sala-Mirete, Sánchez and Marcos. This is an open-access article distributed under the terms of the Creative Commons Attribution License (CC BY). The use, distribution or reproduction in other forums is permitted, provided the original author(s) and the copyright owner(s) are credited and that the original publication in this journal is cited, in accordance with accepted academic practice. No use, distribution or reproduction is permitted which does not comply with these terms.
*Correspondence: Angel Pérez-Ruzafa, YW5nZWxwckB1bS5lcw==