- 1National Institute of Water and Atmospheric Research, Wellington, New Zealand
- 2School of Biological Sciences, Victoria University of Wellington, Wellington, New Zealand
The resilience and recovery dynamics of deep-sea habitats impacted by bottom trawling are poorly known. This paper reports on a fishing impact recovery comparison based on four towed camera surveys over a 15-year period (2001–2015) on a group of small seamounts on the Chatham Rise, east of New Zealand, on which pre-disturbance benthic communities are dominated by thicket-forming scleractinian corals. The six seamounts studied encompass a range of trawl histories, including one with high and persistent levels of trawling throughout the survey period, two with intermittent and intermediate levels of trawling, two which were low/untrawled, and one, ‘Morgue’, which was closed to trawling in 2001, having been heavily trawled up to that point. Still photographs from all surveys were analyzed for the identification and abundance of all visible benthic fauna with effort made to ensure consistency of data among surveys. Because increases in image resolution and quality over time resulted in a persistent trend of increasing abundances, analyses were concentrated on comparisons among seamounts within surveys and how these relationships changed with time. The abundance, species richness, and diversity of benthic communities were higher on low/untrawled seamounts than on those that had been trawled. Multivariate community structure showed similar patterns at each survey point, the low/untrawled seamounts being strongly dissimilar to the persistently trawled seamount, with the others ranged between these extremes, broadly in accordance with their cumulative trawl histories. Community structure on the persistently trawled seamount was less variable than on the other seamounts throughout the study period, possibly because of regular ‘re-setting’ of the community by disturbance from trawling. Although there was some variability in results between whole seamount and summit sector analyses, in general communities on Morgue remained similar to those on the persistently trawled seamount, showing little indication of steps toward recovery to its pre-disturbance state following its closure. These results indicate low resilience of benthic communities on the seamounts to the effects of bottom trawling.
Introduction
The question of whether biotic communities are resilient to the impacts of human activities is important for resource management. Ecological resilience includes the concepts of resistance (how much disturbance can be absorbed before the community undergoes a change), recovery (the rate at which the community reverts toward a pre-disturbance state), and reversibility (whether the community is capable of returning to its original state) (see review by Gollner et al., 2017). The importance of understanding the resilience of biotic communities to impacts from human activities, in the context of ecosystem approaches to resource and environmental management, has led to an increasing focus on recovery studies in both terrestrial and marine ecosystems in recent years (Jones and Schmitz, 2009; Lotze et al., 2011; Lambert et al., 2014). The speed and nature of recovery can vary greatly, depending upon the type and duration of impact, the physical and life-history characteristics of the species making up the communities, and conservation and management measures applied during the recovery period (Lotze et al., 2011).
Biotic communities of many marine ecosystems, especially those associated with commercial trawl fisheries, have been subjected to significant disturbance (Worm et al., 2006; Clark et al., 2007; FAO, 2016). Historical depletion of fisheries, and the ability of fish populations to recover from overexploitation, have received considerable attention in the fisheries literature (Worm et al., 2009; Froese and Proelss, 2010; FAO, 2016). However, equally important in fisheries research is understanding the effects of bottom trawling and the ability of benthic invertebrate communities to recover from disturbance (Collie et al., 2000; Kaiser and Jennings, 2002). There is an extensive body of research now dealing with the nature of impacts of trawling and subsequent changes in benthic communities following cessation of trawling. Many of these studies are from shallow water or continental shelf environments (Collie et al., 1997; Kaiser et al., 2000, 2002; Bolam et al., 2006; Asch and Collie, 2008; Sheehan et al., 2013), including important meta-analyses based on available published data on changes in benthic communities (Collie et al., 2000; Kaiser et al., 2006; Curtis et al., 2012; Hiddink et al., 2017).
However, there have been comparatively few studies on the impacts of bottom trawling on deep-sea benthic communities. Although the type of impacts on such communities are generally thought to be similar to those of trawling in shallower waters (Clark et al., 2016), recovery dynamics are poorly understood. Deep-sea fauna are less adapted to natural disturbance, with species being often slower-growing and less productive than inshore and continental shelf equivalents, and recruitment can be variable (Young, 1983; Smith, 1994; Koslow, 1997; Lacharite and Metaxas, 2013; Clark et al., 2016), and hence recovery times might be expected to be longer. Althaus et al. (2009) reported no obvious recovery in coral fauna from previously trawled seamount features (5 years post-closure) off Tasmania but that some resilient species were more abundant (higher densities) than on actively trawled seamounts. Williams et al. (2010) compared benthic community structure on New Zealand and Australian seamounts after 5 and 10 years, respectively, following fisheries closures and concluded that benthic community structure may take decades or longer to return to a pre-disturbed state in the deep sea because of slow growth rates and high longevity of a number of major invertebrate taxa, including corals.
The Graveyard Knolls are a group of small seamount features off New Zealand (Figure 1) that provide a “natural experiment” to study the nature and extent of impacts caused by bottom trawling and assess the resilience of benthic communities. The seamount group comprises 28 small volcanic cone features east of New Zealand, each typically between 100 and 400 m high, rising from basal depths of 1050–1200 m to summit depths of 750–1000 m (Clark et al., 2010c). Deep-sea seamounts around New Zealand are commonly characterized by the presence of cold-water corals (Tracey et al., 2011b). As in many of the world’s oceans, such corals can produce extensive reef-like structures that provide habitat for many other benthic invertebrate and fish species (D’Onghia et al., 2010; Rowden et al., 2010; Baillon et al., 2012). However, these are also fragile species and thus vulnerable to impacts from heavy bottom trawl gear (Koslow et al., 2001; Hall-Spencer et al., 2002; Clark and Rowden, 2009).
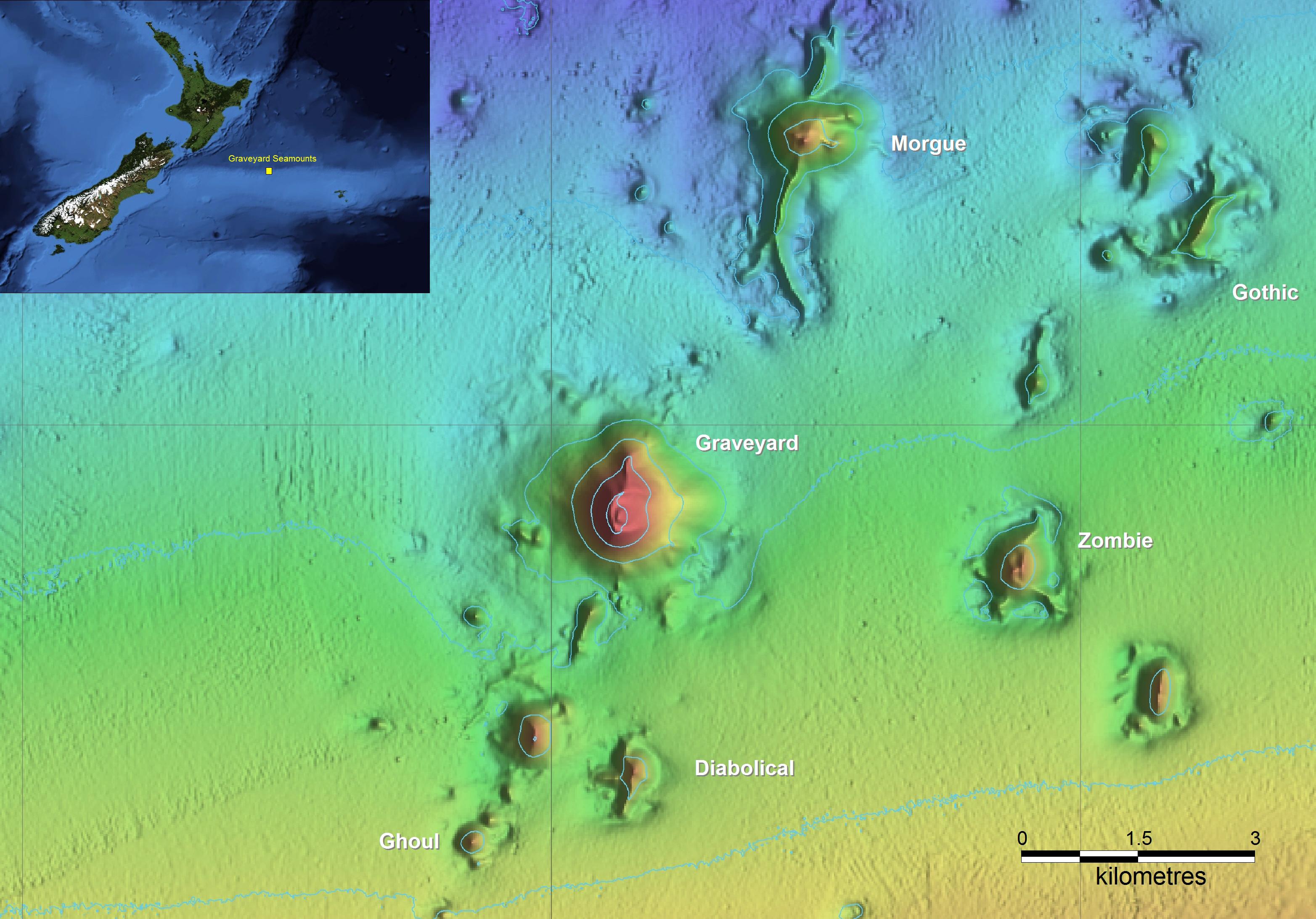
Figure 1. The New Zealand region showing the location of the Chatham Rise, and the Graveyard Knolls, a cluster of small seamounts of which the six labeled have been repeatedly surveyed.
Around New Zealand, major deepwater fisheries occur on seamount features for orange roughy (Hoplostethus atlanticus), oreos (black oreo Allocyttus niger and smooth oreo Pseudocyttus maculatus), black cardinalfish (Epigonus telescopus), and alfonsino (Beryx splendens) (Clark, 1999; Clark and O’Driscoll, 2003). A trawl fishery for spawning orange roughy began on the Graveyard Knolls in the early 1990s, with most effort on two features, “Morgue” and “Graveyard,” with others in the group largely unfished. In 2001, recognizing the importance of protecting some of these features from the effects of bottom trawling, the then Ministry of Fisheries closed 19 seamount features to trawling and dredging, including “Morgue” (Brodie and Clark, 2003). Because the Graveyard Knolls are in close geographic proximity to each other, of a broadly similar size, depth range, and elevation, and have been fished to varying degrees, they provided an ideal opportunity to establish a monitoring study to investigate the processes of resilience, with a particular emphasis on determining the mechanisms and rates of recovery of benthic fauna.
Seabed habitats and fauna have been surveyed on six features in the Graveyard Knolls on four occasions since 2001, and early results were included in a combined New Zealand and Australian analysis comparing changes in benthic community composition (Williams et al., 2010). However, at the time of that analysis, data from only two surveys were available (2001 and 2006). Since then, further surveys have been conducted in 2009 and 2015. Here we present analyses using data from all four surveys, which span a total of 14 years and enable a longer-term perspective of potential recovery post-closure to fishing disturbance. The central hypothesis of the present study is that benthic communities on Morgue Seamount will have changed following its closure to bottom trawling in 2001; becoming less similar to those on neighboring seamounts on which trawling has continued and more similar to those on unfished or lightly trawled seamounts. Implicit in this hypothesis are assumptions that seamounts which continued to be trawled annually, and those that had never been trawled, would maintain essentially constant community structures throughout the period of the study, or if there were temporal changes they would all be in the same ‘direction.’
Materials and Methods
Seamount Surveys
Surveys were carried out, in 2001, 2006, 2009, and 2015, with all four surveys occurring between March and June. Six seamounts were surveyed consistently as a ‘core’ group for each survey (Table 1). The methodology was the same in each survey, with seabed photographic transects started at the summit and run down the flanks to the base, using a towed camera system. At least eight transects were attempted on each seamount, planned to run along the cardinal and intercardinal points of the compass (i.e., N, NE, E, SE, S, SW, W, and NW), following the tracks from earlier surveys wherever possible (see Supplementary Figure S1).
In 2001, a camera frame was used housing a black and white video and color still camera that captured images of 1.5 megapixel resolution triggered manually at about 1 min intervals, with seabed image location determined by matching depths recorded in situ with vessel track and seabed topography. From 2006 onward, surveys used NIWA’s Deep Towed Imaging System, DTIS (Hill, 2009). DTIS is a battery-powered towed camera frame that records high definition (HD 1080i) color digital video with simultaneous high definition still images at 15 s intervals. Still image camera resolution was 5 megapixel (Nikon Coolpix E5000) in 2006 but was upgraded to 10 megapixel (Canon EOS 400D) for surveys in 2009 and 2015. Full resolution video and still images were recorded in-camera at the seabed, and downloaded on return to the surface. A low-resolution video image with overlaid depth and altitude data was transmitted to the surface in real time enabling control of camera altitude and initial evaluation of seabed substratum types and benthic communities. The seabed position of DTIS was monitored by an acoustic ultra-short baseline (USBL) transponder system (Kongsberg HiPAP) and plotted in real time against detailed bathymetric maps using OFOP (Ocean Floor Observation Protocol) software. The target speed for both camera systems was 0.5 to 1.0 knots, at a height of 2–3 m above the seafloor.
Image Data
In total, 22,514 seabed images were collected at the Graveyard Knolls during voyages in 2001 (Time-step 1 or ‘T1’), 2006 (Time-step 2 or ‘T2’), 2009 (Time-step 3 or ‘T3’), and 2015 (Time-step 4 or ‘T4’) and collated for these analyses. Prior to initial analyses, a subset of these images was selected based on two criteria: being within the basal polygon of each seamount (see below) and being of acceptable image quality.
Image transects were first truncated at the basal polygon of each seamount as defined in the NIWA SEAMOUNTS database (Rowden et al., 2008 and see Supplementary Figure S1), in a Geographic Information System (ArcGIS v10.4). Images taken along the southwest spur of Morgue Seamount were also excluded. This part of the seamount is too steep for trawlers to operate on, (as evidenced by extensive stands of scleractinian coral reef along its ridge and western slope (Clark et al., 2010a), and thus has a very different disturbance history from the rest of the seamount and its inclusion could bias any signal of recovery on the main, trawled, summit.
All selected images were then assessed for acceptable exposure, focus, and visibility of the seabed [see Clark et al. (2010a) for details of criteria]. In total, 10,980 seabed images from the surveys were evaluated, yielding a subset of 7,366 images of sufficient quality for quantitative analysis. With the resources available, it was not practicable to analyze all these images, so, for all surveys except T1 (in which there were relatively few images), every fourth acceptable image along each transect was selected. This compromise ensured that the full length of each transect was represented in analyses.
The seabed area (in m2) framed by each image was measured [in ImageJ software (Rasband, 2016)] by reference to a pair of fixed parallel laser points, 20 cm apart, projected onto the seabed from the camera vehicle. Substrate type, as one of eight categorical descriptors (bedrock, boulders, cobbles, gravel, sand, muddy sediment, ‘coral rubble’ (broken coral fragments and remains covering the seabed), and ‘intact coral matrix’ (upright intact matrix structure) was recorded (in ImageJ) as percentage cover of seabed imaged area. All visible benthic invertebrate fauna were identified to the finest taxonomic level possible from the imagery and recorded as counts of individuals. The average time taken for each image was 4–5 min. Colonial reef-forming scleractinian corals were recorded as both a substrate type (intact coral matrix) and as counts of individual ‘live heads’, which are distinguishable through their distinct coloration (see Williams et al., 2010). However, because all areas in which the underlying substrate was obscured by intact coral matrix were assumed to be bedrock, the intact coral matrix substrate type was replaced by bedrock in subsequent data analyses. Taxonomic determinations were made by reference to both the camera imagery, reference photographic sheets and guides (Tracey et al., 2011a), and physical specimens that were collected during the surveys using an epibenthic sled and photographed live under controlled conditions at sea. Specialist taxonomists were consulted wherever necessary to confirm identifications (see Acknowledgments for key specialists for major taxa). Where identifications changed or were confirmed with new imagery over time, earlier data were updated, and to ensure that identifications and counts were consistent over time, protocol checks were made by the image processor at the start of each new survey analysis. Counts of individual fauna were standardized, for each image, to numbers of individuals per 5 m2 of seabed. Where there were uncertainties in taxonomic identifications across the four time-steps, they were pooled to coarser taxonomic level identification. For example, branching scleractinian corals and sponges were often identified to species or class, but ultimately were amalgamated into Scleractinia and Porifera, respectively. The amalgamation of multiple species into higher groupings is an unfortunate reality with image data, where different images of the same species may have different angles, allowing definite identification in those images where distinguishing characteristics are evident, but not in others.
Eleven taxa that either were represented by fewer than ten individuals across the entire dataset or were planktonic rather than benthic were omitted from analyses and the remaining taxa were aggregated to a uniform taxonomic level by iterative matching across the full data set.
Individual images were categorized by seamount name, the time step at which they were collected (T1, T2, T3, or T4), and their spatial location on the feature. Initially, spatial location was defined by nine ‘Sectors’ on each seamount: eight 45° sectors, each centered on one of the cardinal or intercardinal points of the compass (“N,” “NE,” “E,” and “SE,” etc.), and a circle of radius 0.3 x seamount elevation centered on the seamount summit (‘Summit’). Although the factor ‘Sector’ in this form is useful, in theory at least, for comparisons among time steps, only the ‘Summit’ sector is useful for comparisons within time steps. This is because the summit is comparable in its topographic form across all seamounts whereas differences in aspect, topographic form, and fishing intensity between other sectors confound comparisons among them. Because trawl fishers run their gear from the summit downward over the flanks, the summit sector is likely to be the most highly impacted area on all seamounts and because we ran camera transects in the same way, it also has both the highest density of image coverage and the greatest spatial overlap of coverage between time steps. Thus, the summit sector is an area in which hypothesized effects of trawling are most likely to be detected. Stratifying by substrate type, following Williams et al. (2010) was also explored but some substrate types were not represented on some seamounts or in some time-steps, and the number of images in each Seamount x Substrate-type x Time-step pool was often too low to allow viable statistical analyses, particularly for T1 and on smaller seamounts.
In initial analyses it also became apparent that the comparability of the photographic data among time steps was compromised because there was a progressive increase in image quality from T1 to T4, corresponding with increases in camera sensor resolution and the use of dynamic positioning in 2015, which enabled more consistent speed, altitude, and direction to be maintained. The height of the camera above the seafloor affects the size of organisms that can be identified. We examined the frequency of occurrence of images of different seabed areas across the four time- steps (Figure 2), and the influence of imaged area on the numbers of taxa and individuals recorded (Figure 3). This assessment led to us decide that restricting analyses to images with seabed area in the range of 1.5 to 8 m2 would minimize differences in resolution between time steps, thus enabling consistent animal identification, while retaining adequate numbers of images within each time step.
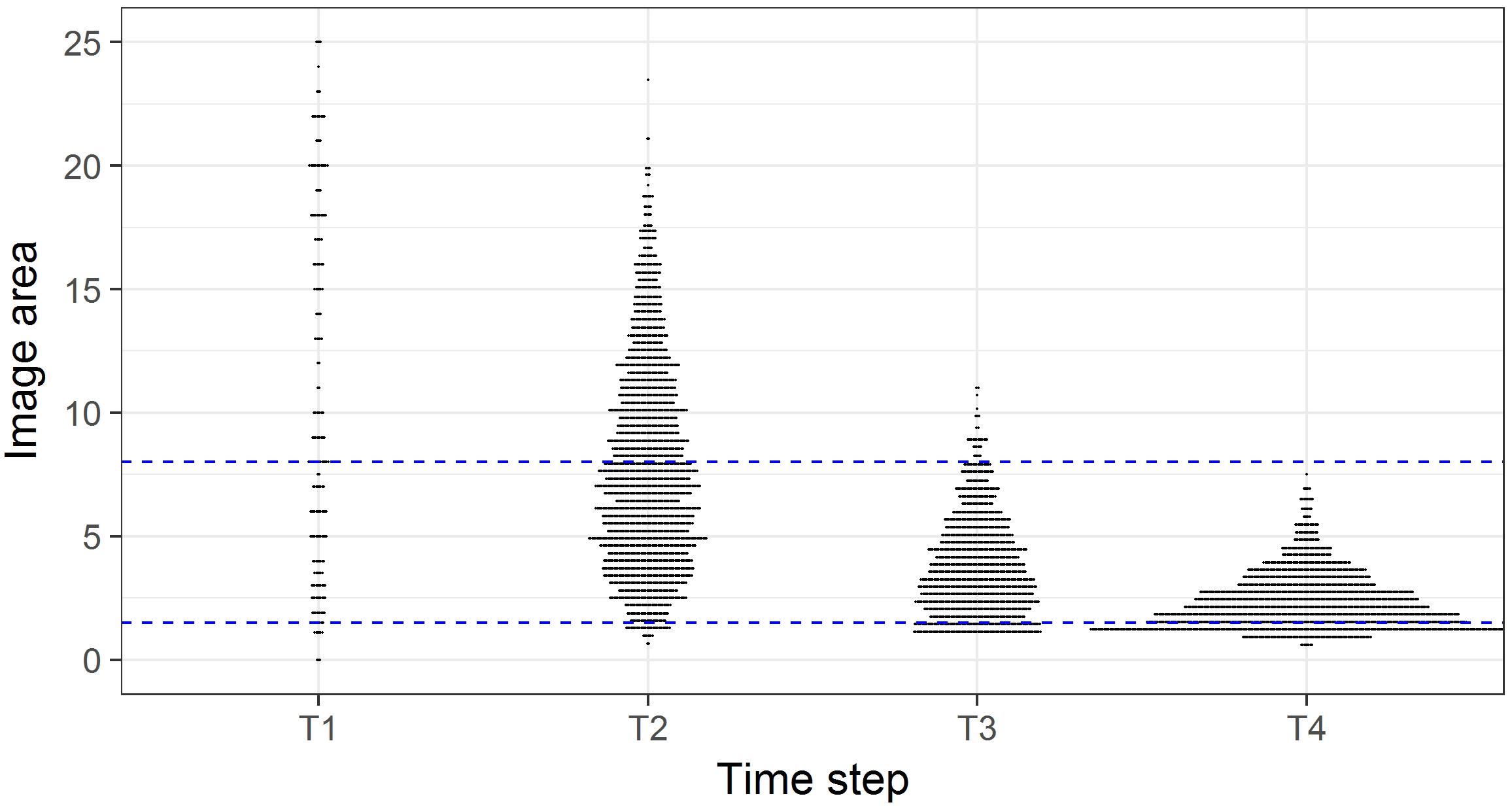
Figure 2. Image area (m2) in each survey of the time steps. Horizontal plot widths indicate the number of images of a given seabed area. Blue dashed lines indicate the subset of images with seabed areas in the range 1.5 to 8 m2.
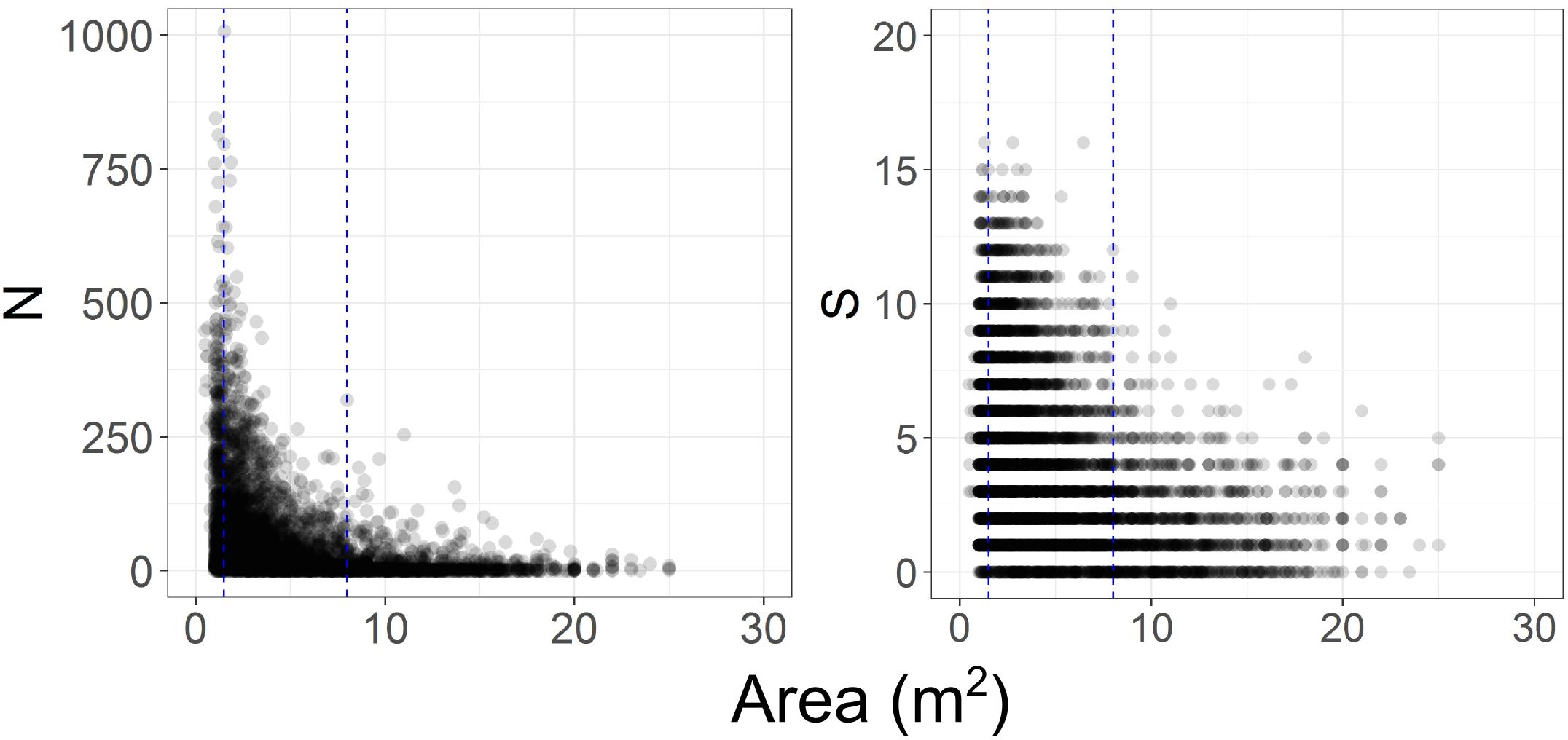
Figure 3. Effect of variations in imaged seabed area on the numbers of benthic invertebrate individuals (N) and taxa (S) recorded. Blue dashed lines indicate the subset of images with seabed areas ranging from 1.5 to 8 m2.
Thus, our final data set for analysis consisted of images with seabed areas between 1.5 and 8 m2 and categorized by three factors: Seamount (Diabolical, Ghoul, Gothic, Graveyard, Morgue, Zombie); Time-step (T1, T2, T3, T4), and Sector (Basal polygon, Summit) (Table 2). We ran all subsequent analyses twice; first on the full data set (‘Whole seamount’, i.e., all image data from within the seamount basal polygons), and second, on a subset of data from Summit sector images only.
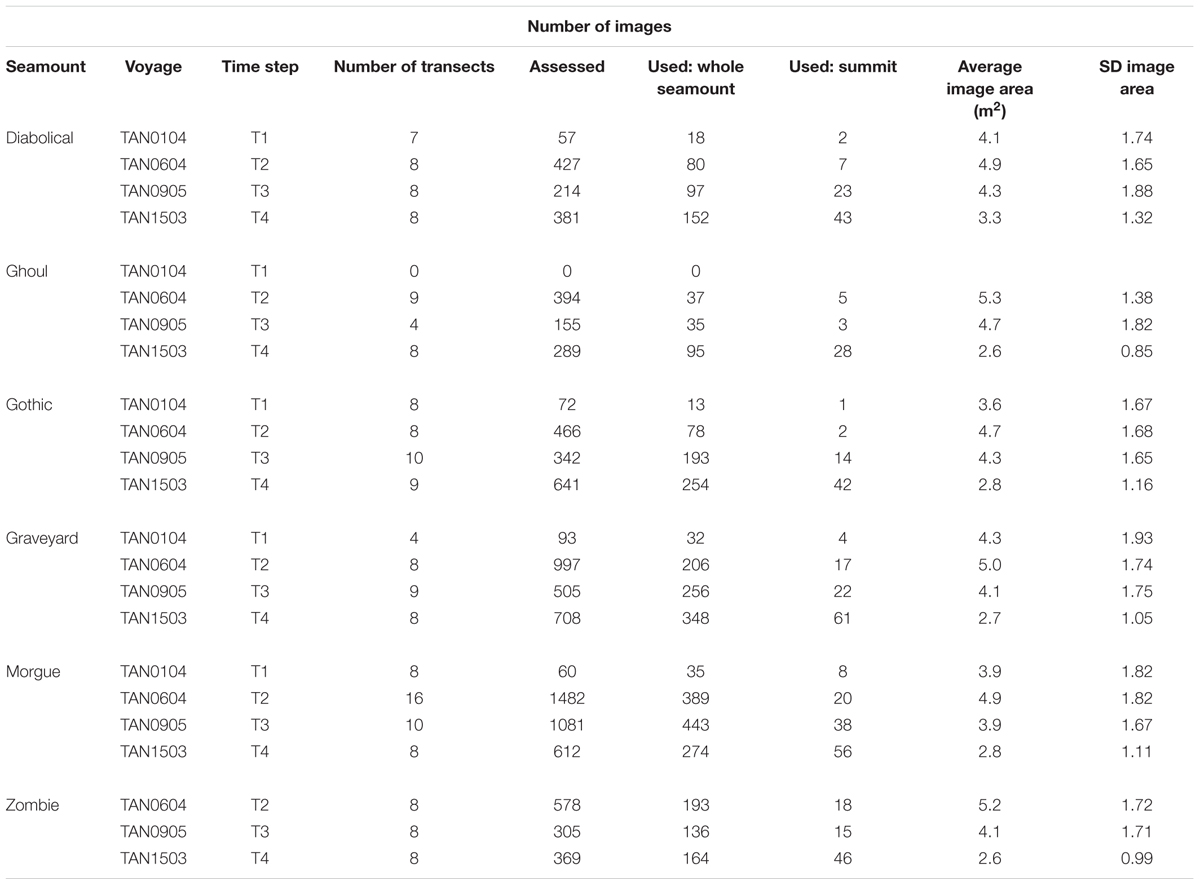
Table 2. Seabed images: “Images used” shows total numbers of images within basal polygons, with acceptable image quality, and with imaged seabed area in the range 1.5 m2 to 8.0 m2.
Fishing Intensity
Commercial fisheries bottom trawl data for all years up to and including 2015 were sourced from the Ministry for Primary Industries trawl data base. Trawls were assigned to individual seamounts on the basis of start position, direction, and duration, and the intensity of trawling on each seamount was then calculated as the Fishing Effects Index (FEI) (Clark and O’Driscoll, 2003), which summarizes information on the density of trawling on a seamount, taking into account the proportion of its seabed area affected and the number of tows in a given direction. FEI was calculated for each seamount in each of the four inter-survey periods separately, i.e., 1994 to 2001 (up to T1), 2001 to 2006 (T1 to T2), 2006 to 2009 (T2 to T3), and 2009 to 2015 (T3 to T4) (Table 3). On the basis of earlier analyses where the relationship between FEI and percentage of coral cover was investigated (Clark et al., 2010a), we also summarized trawling impacts by FEI categories which reflected the clustering of FEI values between seamounts with differing fishing histories, as well as a gradient of likely impact on coral: High (FEI > 50); Intermediate (FEI 0.01 to 50); Low/untrawled (FEI < 0.01); and Closed to fishing (FEI reduced toward 0).
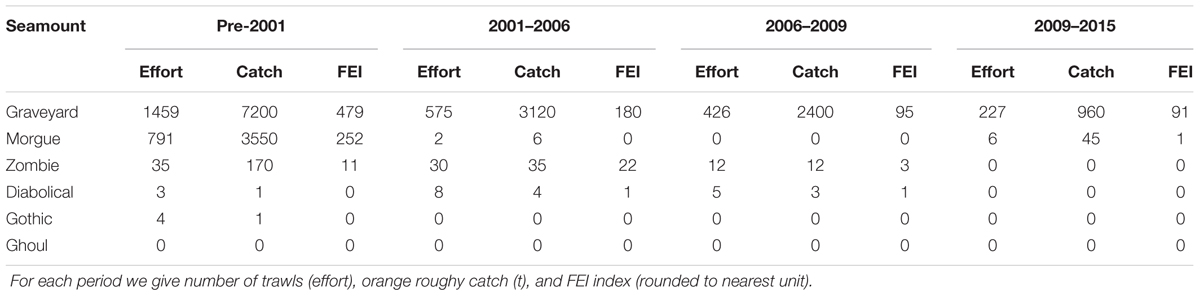
Table 3. Summary of fishing effort and catch levels on the six seamounts, showing the changes over the time period of the surveys.
Analyses
Univariate and multivariate statistical procedures [using routines in PRIMER (Clarke and Gorley, 2006) and R (R Core Team, 2013)]; were used to explore and test the study hypothesis, that benthic communities would over time become less similar to those on neighboring seamounts on which trawling has continued, and more similar to those on unfished or lightly trawled seamounts. The emphasis in the analyses has been on quantifying differences in community structure (species composition and abundance) among seamounts within a given time-step, and how these differences changed from one time-step to the next. This approach of evaluating trends internal to each survey avoided issues associated with changes in realized image resolution between time-steps.
Abundance (N) was calculated as the total number of individuals 5 m-2 and taxon richness as the expected number of taxa in a random sample of 50 individuals (ES50, based on actual counts of individuals rather than the standardized values). Values of these metrics were compared among seamounts within time-steps using boxplots and one-way ANOVA with Tukey HSD multiple means comparisons at 95% confidence level (R function TukeyHSD). There were too few samples (images) at T1 to support formal statistical comparisons.
Comparisons of multivariate community structure were based on Bray-Curtis similarities among individual images, calculated from square-root transformed standardized abundance data. To enable visualization of similarities in two-dimensional ordinations, the matrix of Bray-Curtis similarities was used to calculate multivariate centroids for sets of images within each of two combinations of factors: (1) Seamount basal polygon x Time-step and (2) Seamount summit sector x Time-step. Non-metric multidimensional scaling (nMDS) ordinations were then produced for each of these sets of centroids, to visualize similarities among communities on each seamount within and between time-steps, and with fishing intensity, as FEI, superimposed as bubble plots to illustrate relationships between community similarity and fishing history.
Results
Image Analysis Data
The subset of images selected for use in analyses provided good representation of most summit and flank areas of each seamount in each time step, with the exception of Ghoul Seamount in 2001 (Supplementary Figure S1). In total, 167 taxa were recorded during image analyses, at identification levels ranging from species-level to coarser taxonomic levels (Class, Phylum, etc.) and operational taxonomic descriptors (e.g., colonial and solitary scleractinian corals). The final data set for analyses consisted of quantitative counts of 38 taxa (Table 4).
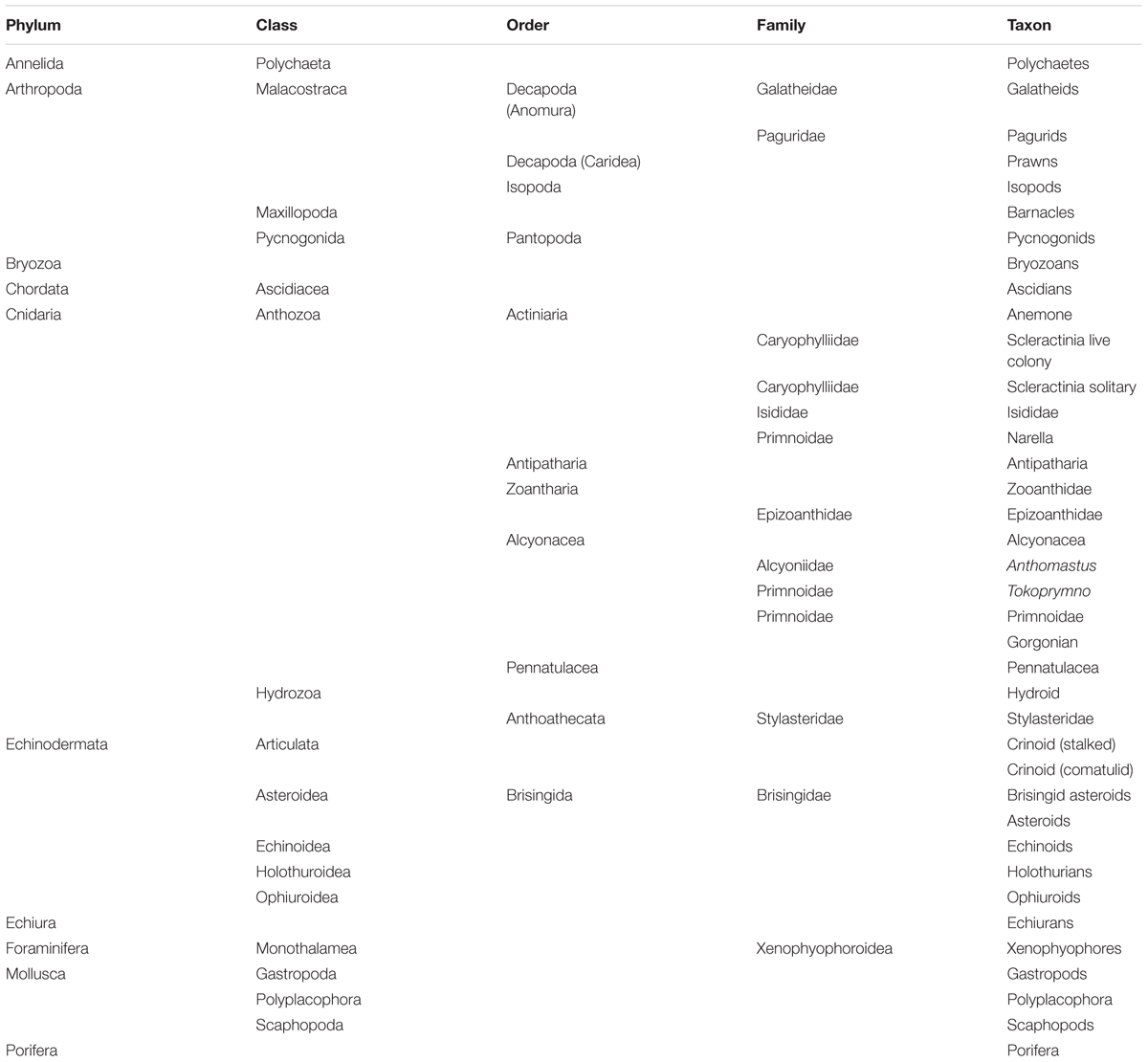
Table 4. The benthic invertebrate taxa used in analyses of seabed photographs across the four surveys.
Most taxa on all seamounts showed an increase in abundance over time (Supplementary Figure S2). As noted in the Methods, it is likely that the general increase in measured abundance is an artifact resulting from increases in image quality and consistency over the study period. These improvements in image quality result in more taxa being identified (i.e., likely taxa accumulation over time) and more individuals of a given taxon being recorded (i.e., likely abundance accumulation over time). Changes in abundance were noted for taxa that are unlikely to have increased in abundance because of their very slow growth rates (e.g., Antipatharia, Hydrozoa, Zooanthidae, Primnoidae).
Univariate Community Analyses
In whole-seamount analyses, abundance (N), and taxon richness (ES50) were significantly higher on the two low/untrawled seamounts (Ghoul and Gothic) than on trawled or previously trawled seamounts at all time steps from T2 to T4 (Figure 4). This pattern was similar for summit areas only, where abundance and richness were significantly higher on Ghoul and Gothic than on other seamounts at T3 and T4, and for Ghoul also at T2. On Morgue (high trawling, then closed to fishing after T1), values for both metrics were generally the same as, or lower than, those on Graveyard (persistently high trawling), although at T4 abundance, and richness (whole seamount only), were significantly higher than on Graveyard, and similar to levels of intermediate-trawling seamounts (Diabolical, Zombie).
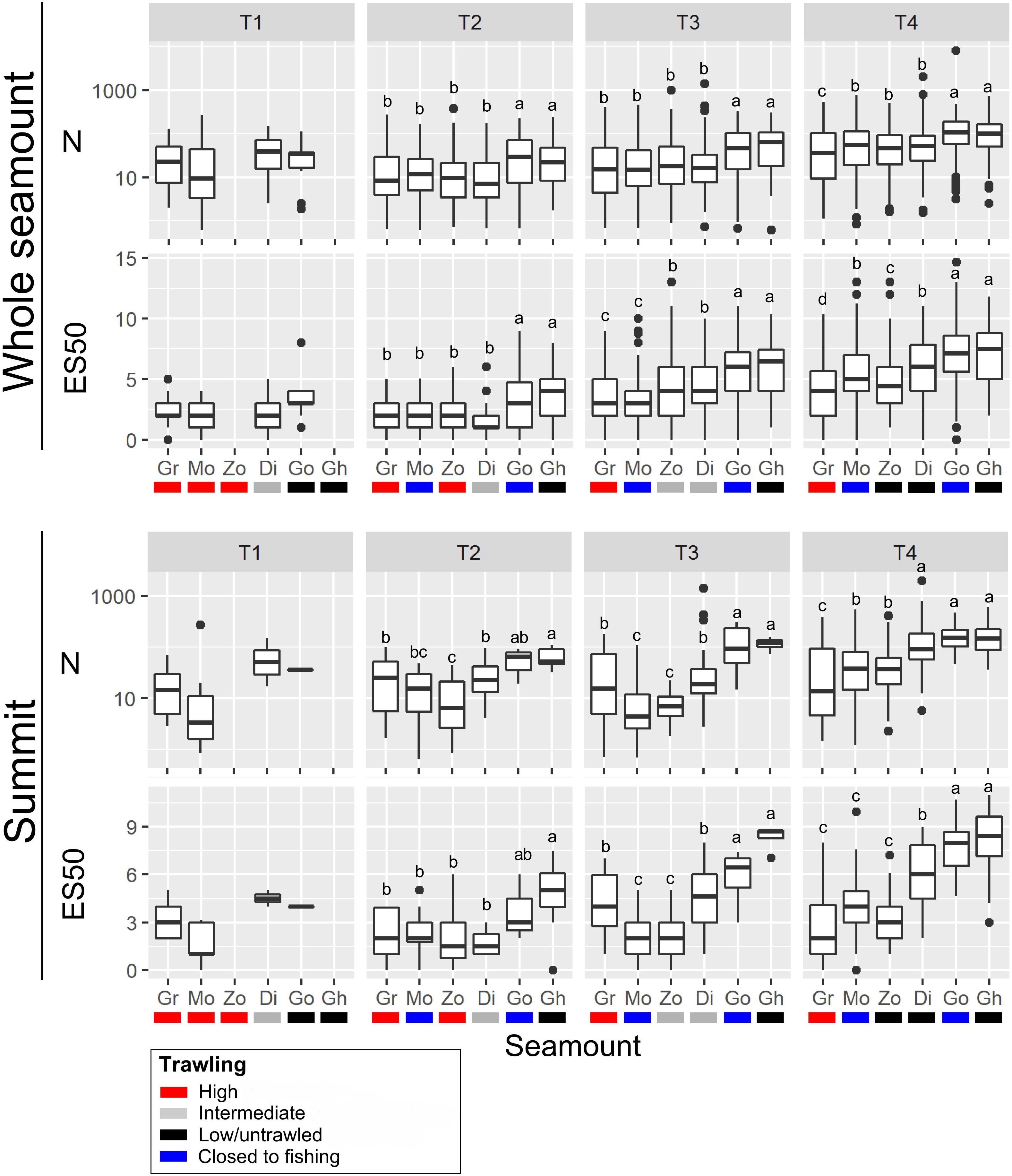
Figure 4. Univariate metrics of community structure; abundance (N) and taxon richness (ES50), calculated for all images within seamount basal polygons (‘Whole seamount’) and images within summit polygons only (‘Summit’) at each of the four time-steps (T1 to T4). Seamounts are: Diabolical (Di), Ghoul (Gh), Gothic (Go), Graveyard (Gr), Morgue (Mo), and Zombie (Zo). Box-and-whisker plots show median (central bar) and 25th and 75th percentiles (‘inter-quartile range’, top and bottom bars of boxes), whiskers extend to the largest and smallest data value no more than 1.5 times the interquartile range, and dots show values outside of this range (outliers). Letters above each plot indicate results of one-way analysis of variance (ANOVA) tests for differences between seamounts at each time step: for seamounts sharing the same letter, metrics are not significantly different (P > 0.05).
Multivariate Community Analyses
nMDS ordinations based on centroids of benthic community structure for each seamount at each time-step show similar patterns for both whole-seamount and summit centroid analyses (Figure 5). At each time-step, the two low/untrawled seamounts, Gothic and Ghoul, occupy one side of the ordination space, with the high FEI, persistently trawled feature, Graveyard, at the opposite side. The two seamounts with intermittent trawling (intermediate or high FEI from T1 to T3, then low at T4), Zombie and Diabolical, lie between these extremes. Morgue, on which high trawling ceased after T1, lies with Diabolical and Zombie in the whole-seamount analysis but closer to Graveyard in the summit-sector analysis. This result suggests that no recovery is occurring on Morgue, where a shift closer to the low/untrawled seamounts would be expected if recovery was occurring.
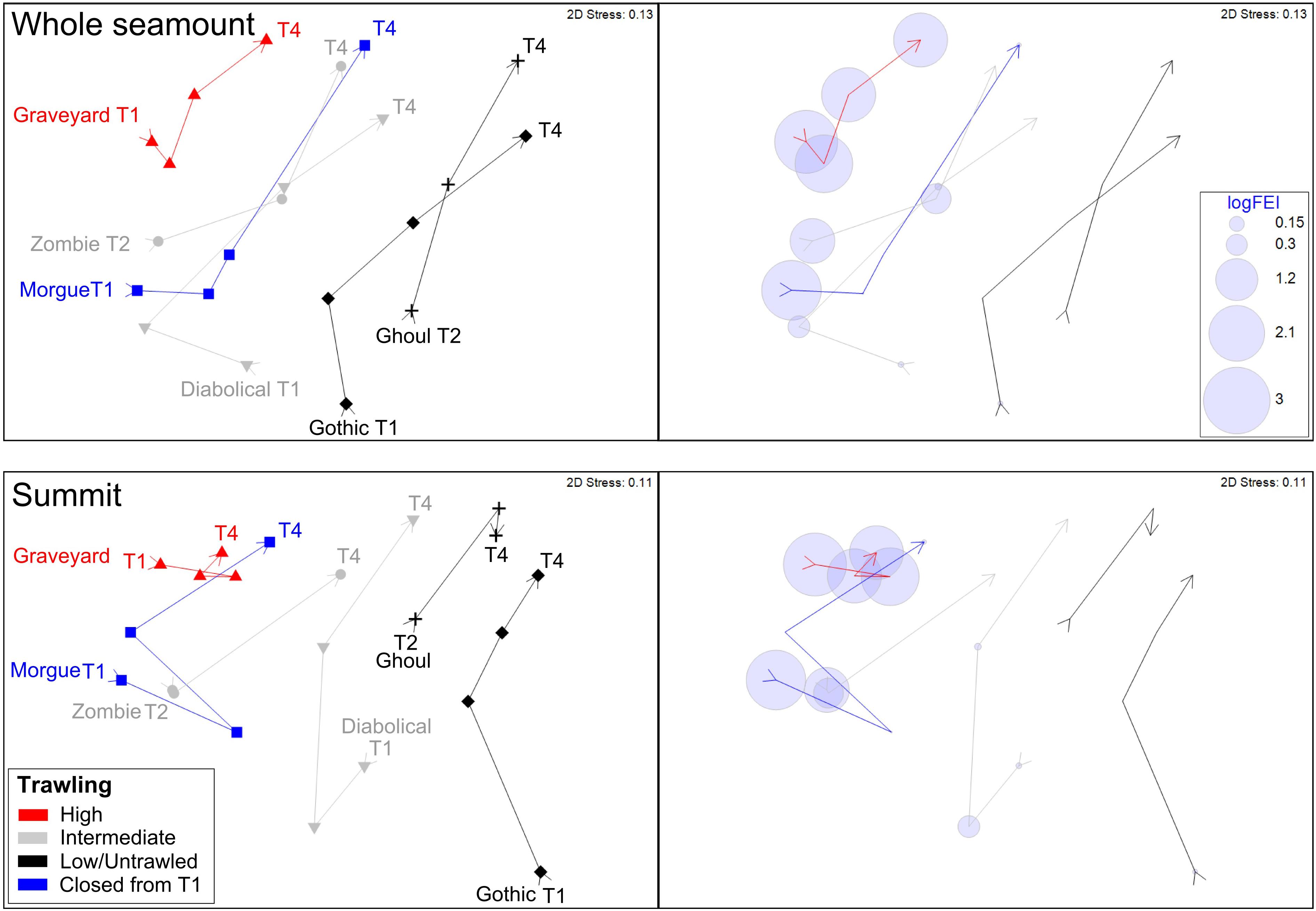
Figure 5. Ordinations (nMDS) illustrating benthic community similarities over time steps T1 to T4 and in relation to relative trawl history (see legend): Top panel, ordination using centroids of all images within each seamount basal polygon (‘whole seamount’); bottom panel, ordination using centroids of images within only the summit sector. Right and left windows in each panel show the same ordination: left indicates seamount identities, time-steps, and categorical indication (color) of overall trawl histories, right shows trawling intensity as the Fishing Effects Index (FEI) superimposed as bubble plots for each seamount at each time step. Ordinations represent Bray-Curtis similarities calculated from square-root transformed abundance data.
The ordination pattern matches well the gradient in fishing effort index (FEI) plotted in the right-hand panel. It is also conspicuous in these ordinations that the benthic community structure of Graveyard is less variable through the T1 to T4 trajectory than is the case for the other seamounts; its community centroids occupying much the same part of the ordination space throughout the time series while those of the other seamounts show substantial shifts between time-steps.
The occurrence of intact scleractinian coral matrix (as percent cover in individual images) declined markedly with increases in cumulative FEI across all time-steps (Figure 6). The untrawled (low FEI) seamounts Ghoul and Gothic had consistently higher cover of intact coral matrix than the highly trawled Graveyard (high FEI throughout) and Morgue (high FEI before closure) seamounts, with Diabolical and Zombie seamounts (intermediate FEI) between these two extremes.
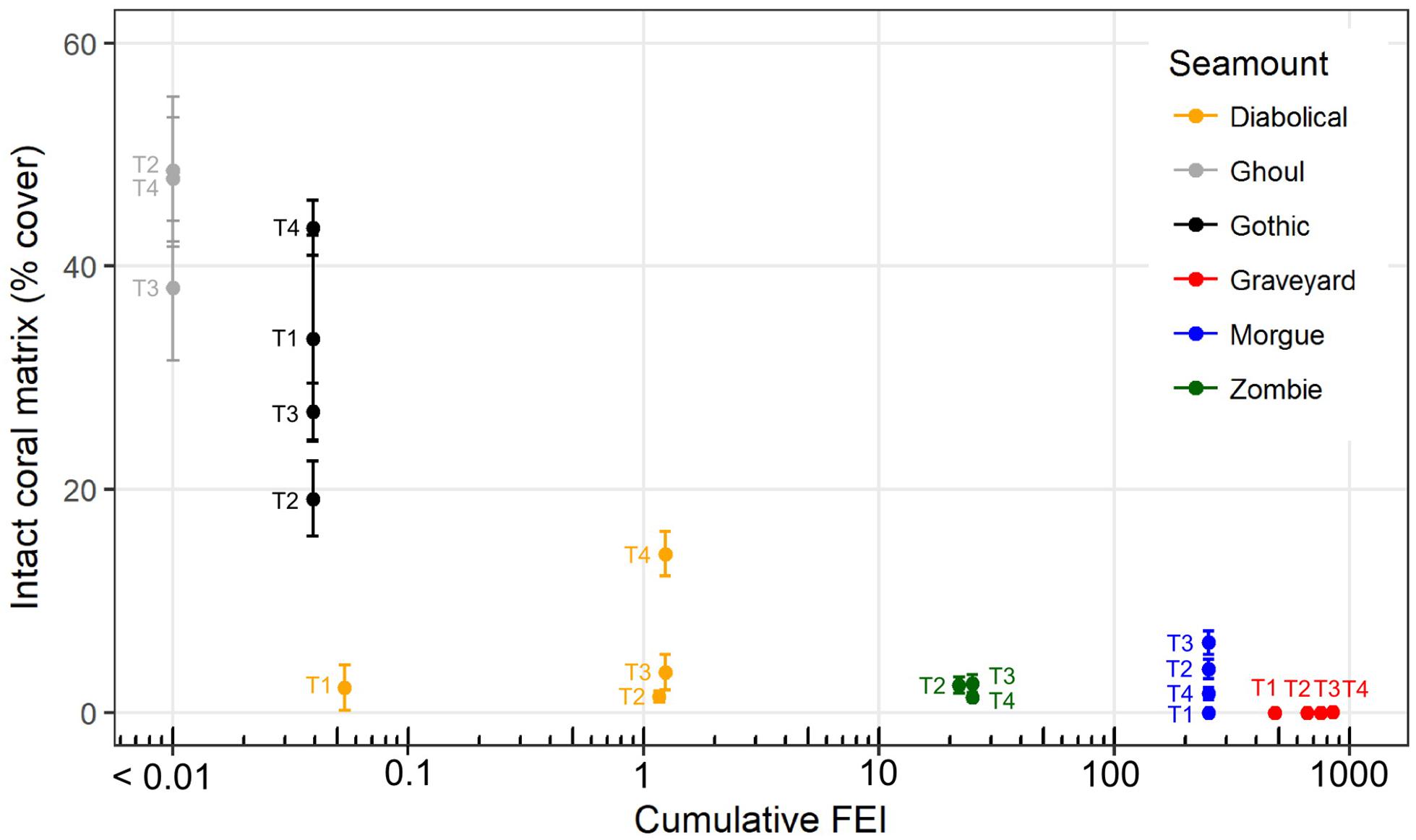
Figure 6. Intact coral matrix, as mean percent cover measured in individual seabed photographs, on six seamounts at each of four survey times (2001, ‘T1’; 2006, ‘T2’; 2009, ‘T3’ and 2015, ‘T4’) in relation to cumulative trawling impact (Fishing Effects Index, FEI, all years up to and including the year of each survey). Error bars show ± 1 SE. Plotted x-values are log10(FEI) with back-transformed axis labels, but because zero values cannot be plotted on a log scale, FEI values for Ghoul seamount (zero at all time steps) are plotted as log10(FEI + 0.01).
Discussion
This study extends previous descriptions of time-series data on New Zealand seamounts (Clark et al., 2016) to almost 15 years after management action that closed one feature to bottom trawling. However, despite the longer time period covered, there are no clear signals in our analyses to support the hypothesis that benthic communities on the seamount that was closed in 2001, Morgue, are recovering from disturbance caused by trawling. There are variations in results between some time periods and whole seamount versus summit, but in general the analyses of community metrics summarizing abundance and species richness, and multivariate analyses of community similarity indicate that benthic communities on Morgue are still more like those on its highly and persistently trawled neighbor, Graveyard, and seamounts with intermediate intensities of trawling than those on seamounts that have low levels or have never been trawled.
Methodological Issues and Checks Relating to the Detection of Recovery
Extensive efforts were made to produce a directly comparable dataset between surveys and maintain a consistent protocol for level of taxonomic identification. The same image analyst was used for all surveys, and there were regular checks on the consistency of processing and identification. However, a limitation of the final data set was the necessity to amalgamate various taxonomic levels of identification for some groups where species or genus could not be consistently resolved (where distinguishing characteristics were not always evident). This is common to many image-based surveys, but with this study means that there is some potential for species-specific differences in response to impact to be masked by the lumping into higher categories. Although we don’t think the relative balance of species or operational taxonomic units within the larger groups has changed over time, the possibility of it introduces a level of uncertainty into results that should be kept in mind.
The overall quality of the imagery improved with each survey as camera resolution increased and improvements in camera vehicle tracking (USBL and OFOP systems in 2009) and deployment methods (vessel dynamic position system in 2015) were introduced. This improvement meant that direct quantitative comparisons between surveys were likely to be confounded by directional trends in, for instance, the number of taxa identified and their abundances. To allow for this trend, we developed an analytical approach based on quantifying relationships between benthic communities on seamounts within individual surveys, and then looking at how these relationships changed with time from one survey to the next. An alternative approach of reducing the resolution of the later camera imagery to match earlier surveys was considered but such deliberate “fuzzying” would have lost much additional fine-scale information, which would have reduced confidence in faunal identifications and compromised our ability to detect small animals that might represent recent recruitment of juveniles during the early stages of recolonization.
The analysis approach used was only viable because of the number of surveys completed; patterns being evident over four surveys and 15 years that might not have been with only two surveys (see Williams et al., 2010). This situation highlights the importance of maintaining regular surveys for monitoring of recovery in the deep sea, and the value of sampling multiple seamounts and sites to be able to differentiate between real patterns and sampling artifacts. The combination here of four surveys of multiple seamounts with different fishing histories, allows us to have more confidence in the interpretation of patterns and the overall conclusion that recovery is not occurring than would have been the case with fewer surveys focussed on Morgue alone; the seamount of primary interest.
The surveys were all carried out in southern hemisphere autumn-winter months to avoid potentially confounding seasonal differences. However, to assess whether the general trend of increasing faunal abundance that we have attributed to improvements in image quality might be a real ecological trend driven by longer-term environmental changes, we examined records of surface temperature (SST, from satellite data) and temperature at depth (from ARGO data) at the location of the Graveyard Knolls. SST data were available dating back to 1981 but ARGO data only from 2004 (Figure 7). SST data indicated some oscillations but were generally very consistent over the 2001–2015 period. Temperature at depth from regional ARGO profiles show a seasonal variability of 0.2–0.4°C but at the time of each survey values were relatively consistent at 6.8–6.9°C. There might be time lags in any effects of temperature fluctuations on benthic fauna, but we have no reason to believe environmental changes (for temperature, at least) would have had any influence on the abundance of benthic taxa over the study period.
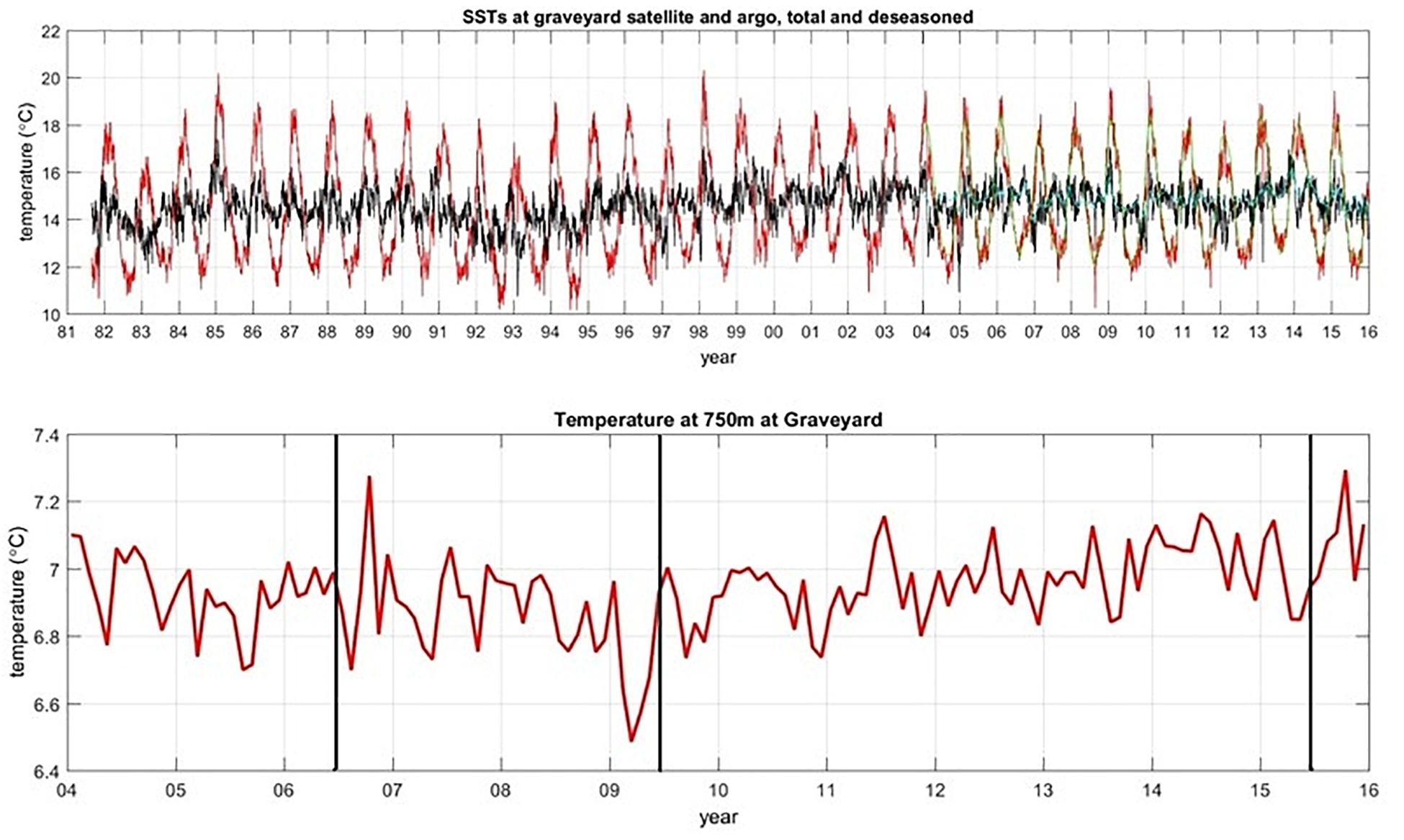
Figure 7. Time series plot of SST data (top panel), with red lines showing the raw data with seasonal variability, and black lines being smoothed by adjusting for seasons. Bottom panel shows temperature from ARGO floats at 750 m depth. Vertical lines indicate timing of T2, T3, and T4.
Experimental studies on disturbance impacts and small-scale comparisons are often difficult to scale-up appropriately to represent the extent of impact from commercial fishing (Hinz et al., 2009). In this study, we chose relatively small seamounts that are representative of many of the knolls, hills, and pinnacles around New Zealand that are trawled for orange roughy. The image transects also covered the same habitats on the seamounts as a bottom trawl would; from the summit to the base. Hence, we are confident that the results are meaningful at the scale of fishing impacts, despite differences in the spatial coverage of a camera image (2–3 m wide field of view) compared to a deepwater trawl (20–25 m ground gear contact).
The distribution of benthic communities at small spatial scales on seamounts can be highly variable, driven by the multiple habitat-types present in these complex features (Rowden et al., 2005; Boschen et al., 2015; Davies et al., 2015; Du Preez et al., 2016; Rogers, 2018; Victorero et al., 2018). This variability is a challenge for survey design, with the need to ensure sufficient area or habitat types within the seamount are sampled, and thus that potential recovery by taxa across a range of habitat types are captured. Our survey design covered different spatial aspects of each seamount, and also different types of habitat (ridge, valley, summit, etc) within each seamount, as determined by topographic analysis of the Graveyard seamounts (Clark et al., 2010a). This approach does not preclude missing small pockets of recovery by benthic communities, but we believe makes it unlikely that we would have failed to capture any substantial change events.
Resistance to Trawling Impacts
Multivariate analyses of community data across all seamounts showed a similar relationship among seamounts at each time-step, with the persistently highly trawled Graveyard along with the previously highly trawled and now closed Morgue seamount at one end of the ordination space, the low/untrawled Gothic and Ghoul at the other end, and intermittently trawled seamounts lying in-between. This order matches the gradient in impact from commercial fishing (expressed as the FEI index).
The level of impact on benthic communities resulting from a given amount of trawling effort has not been well researched in deep sea habitats, and the most recent and extensive review on depletion and recovery rates (Hiddink et al., 2017) did not include rock substrate. However, available data at several hundred meters depth suggest that a single trawl may not necessarily damage everything in its path, but repeated trawling can reduce populations of benthic fauna to low levels (Sainsbury et al., 1997; Freese et al., 1999). The present study (see Figure 6), as well as Clark et al. (2010a) indicate that as few as 10 trawls (which generated the FEI values > 1) in these deep-sea environments can reduce average coral cover from 15 to 20% down to zero (no visible cover). Because such substantial impacts can result from a very small number of trawls, subsequent trawling, even at high intensity may result in there being no observed increase in impact in subsequent surveys, and thus studies based on correlations of gradients in fishing effort over time with benthic impacts may detect only limited signals (Curtis et al., 2012; Yesson et al., 2017). Nevertheless, it is to be expected that repeated trawling over time has some degree of cumulative effect, and subtle cumulative effects may only become apparent over long time periods or large spatial scales (Hinz et al., 2009). In this regard it is of interest that community structure on Graveyard seamount was more constant over the four surveys than were those on the other seamounts, with consistently lower abundance and taxon richness. We suggest that this is likely to be an indication of regular ‘re-setting’ of the community by disturbance from trawling and thus that, in terms of the concepts associated with resilience, benthic communities on the Graveyard seamounts show no evidence of being resistant to disturbance.
Recovery From Trawling
We expected that 15 years after trawling had ceased on Morgue it would be possible to detect changes in the abundance, taxon richness, and species composition of benthic communities that would be suggestive of recovery when viewed in comparison to low/untrawled seamounts. However, the biodiversity measures we looked at gave little indication that communities on Morgue were converging with those on low/untrawled or intermittently trawled seamounts and after 15 years, Morgue was essentially indistinguishable from Graveyard; the most highly and persistently trawled seamount in the group. This result was strong for the summit analysis, which is the sector where all bottom trawling is likely to have had an impact. At the whole seamount scale the contrast is not as great relative to the intermittently trawled seamounts (Zombie, Diabolical) which may reflect a patchier distribution of impact down the flanks.
The nature of recovery in biotic communities after disturbance is uncertain. There are many accounts of the recovery characteristics of benthic communities of inshore and continental shelf sedimentary substrates (Kaiser et al., 2002, 2006; Asch and Collie, 2008; Hiddink et al., 2017), and coastal reefs (Schloder et al., 2013; Sheehan et al., 2013), but few for deep-sea habitats. Studies at depths of several hundred to 1500 m have shown potentially higher recovery of infaunal communities in soft sediment over periods of 1–10 years (Montagna et al., 2017; Yesson et al., 2017), but the recovery dynamics of hard-bottom mega-epifauna in bathyal slope depths are poorly understood. Experience from volcanic eruptions at active deep-sea hydrothermal vents on ridges and seamounts suggests communities at these habitats are fairly resilient and adapted to such natural disturbances. While abundance and biomass overall revert to pre-disturbance levels within 5 to 10 several years at these locations, community structure often follows a sequence of faunal succession (Gollner et al., 2017) that can be slower and more complicated at areas away from the active vent sites (Gollner et al., 2015). Experience from studies on changes in benthic communities at 200–300 m depth associated with iceberg disturbance in the Antarctic suggest a succession of faunal recolonization is likely, with first colonizers within 10 years consisting of bryozoans, sponges, polychaetes, gorgonians and ascidians, followed by longer-lived biogenic habitat forming sponges and slow growing coral species (Teixido et al., 2004). Patterns of recolonization and recovery in a number of shelf environments also appear to show a strong correlation with life history characteristics of certain taxa, with bushy bryozoans being early dominants in successional stages within 2 years, and longer-lived and slower-growing species becoming more predominant over time (up to 6 years) (Asch and Collie, 2008). Although we cannot compare densities of such taxa over the four time periods on each seamount, these will be important taxa to monitor on the Graveyard Knolls in future.
It has been speculated that early recolonisers on the Graveyard Knolls might include stylasterid hydrocorals (Clark and Rowden, 2009) and comatulid crinoids, and similarly observations on some Tasmanian seamounts have suggested that both stylasterids (Williams et al., 2010) and anemones (Clark et al., 2010b) might be early colonizers. However, it is often inconclusive whether recent recruitment has occurred, or whether these taxa were remnants in areas inaccessible to, or missed by, trawling. Furthermore, despite relatively rapid turnover by some colonial taxa, the spatial extent of recolonization may be small given many such species have limited larval dispersal (of the order of 10s meters) (Bradshaw et al., 2002).
A recent US survey on seamounts of the northwestern Hawaiian Ridge and southern Emperor Seamount Chain indicates there could be some recovery from effects of trawling in the 1970s (Amy Baco, Florida State University, pers.comm). Several areas appear to have been recolonized by some coral groups, as well as patches of fauna occur that are assumed to be remnant from the period when the seamounts were fished. The survey findings suggest that changes in benthic communities might begin to occur within 40 years after trawling disturbance. In comparison, it is likely that a similar temporal recovery of benthic communities on the Graveyard Knolls may not occur because of differences in the coral species and their depth distribution. Benthic communities on Morgue, with a summit depth of 890 m, have a deeper distribution than those on the North Pacific seamounts where the summit depths are 300–500 m. Growth rates of deeper coral species could be expected to be slower given lower temperatures and food availability. Furthermore, the North Pacific seamounts were originally dominated by individual growth form octocoral species in contrast to the reef-building scleractinian corals on the Graveyard Knolls, and different community patterns may affect recolonization and growth rates.
Reversibility From Trawling
Over the course of 15 years, it is unrealistic to expect a full recovery of benthic communities to their original untrawled state. Biogenic scleractinian coral reef-like structures such as those on low/untrawled Ghoul and Gothic seamounts are likely to have been growing for several hundred, perhaps thousands, of years, and growth rates of individual colonies are low at around 1 mm year-1 (Fallon et al., 2014). However, it is clear that long-term monitoring studies such as the one here on the Graveyard seamounts, and a similar program off Tasmania (Althaus et al., 2009; Williams et al., 2010) can play an important role in documenting the time required to see substantial changes in benthic communities, and to improve our understanding of resilience. There are other studies that are necessary to understand the resilience of benthic communities to trawling impacts. The environmental drivers of successful dispersal, settlement and recolonization of disturbed habitat are largely unknown, and this element was recognized recently as being a key knowledge need for determining best spatial management practices to mitigate environmental impacts.
Recruitment
Whereas immigration of mobile fauna has been noted as a major factor in recovery in some studies (Collie et al., 2000; Jennings et al., 2001) larger-scale areas or more isolated habitats such as seamounts will be expected to have different recovery dynamics because larval recruitment and growth may be the main sources of recovery (Lambert et al., 2014). The localized and concentrated nature of trawling on Morgue and Graveyard, with untrawled and low trawled seamounts distributed throughout the Graveyard Knolls group means we have more a “Type-2” disturbance, where recolonization is unlikely to occur through immigration from surrounding areas, but will proceed by the slower process of larval settlement with dispersal from the unaffected patches (Asch and Collie, 2008).
The spatial scale and distribution of likely sources of recruits is an important consideration in recovery dynamics. Reproductive and dispersal characteristics in the deep sea can be a limiting factor if disturbed areas are widely separated from colonizer pools, resulting in potentially low larval supply to impacted areas (Lacharite and Metaxas, 2013). Lambert et al. (2014) demonstrated that the recovery of large species was faster when conspecifics were relatively close (6 km in their case in the waters off the United Kingdom), and hence resilience of a community would be higher if there were unfished patches within a wider fished area. This situation occurs naturally with the Graveyard Seamounts, and this suggests the possibility of recruitment from remnant areas such as the SSW spur of Morgue which has an extensive untrawled area of scleractinian coral (Solenosmilia variabilis) reef (Clark et al., 2010a, 2016), or from neighboring low/untrawled Gothic and Ghoul seamounts which are about 5 km distant from Morgue and Graveyard, respectively. However, while this distance appears small for potential broadcast spawners such as many of the scleractinian coral species found on the seamounts, the nature of their reproduction may play a critical role in recovery potential. Miller and Gunasekera (2017) used genetic techniques on corals on Tasmanian seamounts and found differing dispersal patterns for two coral species that also occur on the Graveyard seamounts. The solitary coral Desmophyllum dianthus had widespread dispersal across neighboring seamounts, but the reef-forming Solenosmilia variabilis appeared to favor asexual reproduction with negligible dispersal of sexually produced larvae, resulting in isolated populations. This finding suggests that it is difficult to predict the recovery timeframes for the coral reef-forming species that is a key component of the benthic communities on the Graveyard Knolls, and makes it important that we obtain in situ information about realized recruitment in order to inform the design of spatial management measures.
In 2010 a camera mooring was deployed near the summit of Morgue Seamount to capture acoustic and image data on orange roughy. Before deployment, the mooring weight (a 250 kg railway wheel of 0.5 m diameter) was fitted with six unglazed ceramic tiles as an opportunistic settlement plate study of the potential for settlement by invertebrate larvae. In the 2015 survey (T4) a camera tow located this mooring and imaged the weight and plates after 5 years on the seafloor (Figure 8). There was no visible sign of faunal recruitment on the settlement plates or wheel.
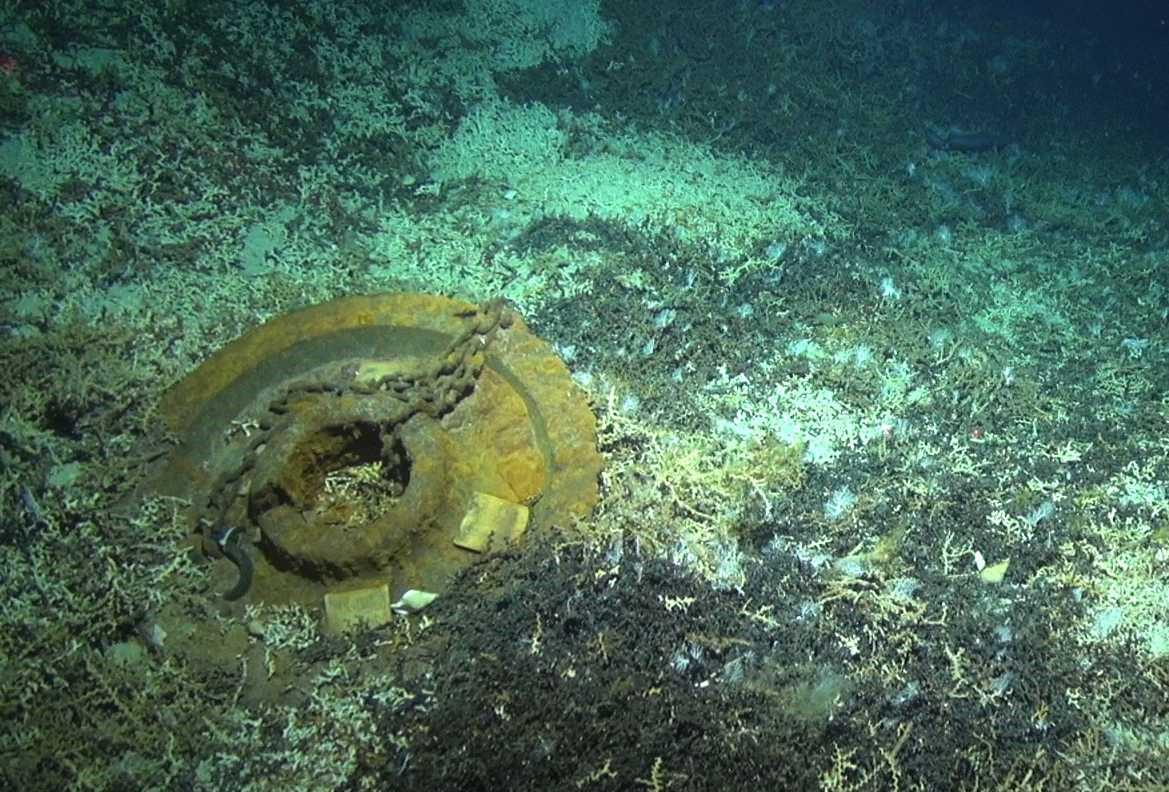
Figure 8. Frame-grab of video from DTIS, showing the wagon wheel in amongst coral rubble. The surface of the wheel, as well as the visible settlement plates (white-yellow squares) do not show signs of any recruitment.
Although this is only one settlement unit, the clear absence of fauna indicates that recruitment events for benthic species may take > 5 years or be patchy in space and/or time. Although the growth rates of many deep-sea species of coral are slow, a cross-section of seamount benthic species reported in Clark et al. (2016) showed axial growth of several to 10s of mm per year. This growth rate suggests that if settlement had occurred post-2010 the animals could be at least 1–2 cm high, which should be visible to our camera systems. The situation was similar on many exposed bedrock surfaces on Morgue, where there was little or no indication of recent recruitment by benthic invertebrates.
Management Implications
Benthic communities associated with biogenic habitats formed by deep-sea corals and sponges on seamounts are amongst the most susceptible to fishing impacts because their resilience is apparently low (Clark et al., 2016). This study, together with that off Tasmania (Williams et al., 2010) shows that recovery times of benthic communities on seamounts may be long, at least in the order of several decades. This situation means that restoration concepts are unachievable in the short term, and are anyway likely to be prohibitively expensive in the deep sea (Van Dover et al., 2014).
A variety of management actions have been taken to date with seamount fisheries, including regulating fishing methods and gear types; specifying the depths fished; limiting the volumes of by-catch or limiting catch; move-on rules; and closing areas of particular habitat and individual seamounts (Probert et al., 2007; Morato et al., 2010). The growing literature on the extent of bottom trawling (Amoroso et al., 2018) together with a greater recognition of the potential severity of human impacts in the deep-sea, and long recovery periods from such impacts, support the contention that spatial management is likely to be the most effective strategy to conserve benthic communities of seamount ecosystems (Clark and Dunn, 2012; Schlacher et al., 2014). This approach is best achieved by restricting the distribution of fishing effort, and putting in place a network of open and closed areas, with closure of unfished seamounts where benthic communities occur in their natural state (Johnston and Santillo, 2004; Clark et al., 2016).
Data Availability
The datasets generated for this study are available on request to the corresponding author.
Author Contributions
MC and AR designed the surveys. MC, AR, DB, and RS performed the surveys. RS processed the images. DB, MC, and AR analyzed the data. DB, MC, AR, and RS contributed to the writing and review.
Funding
We acknowledge the multiple funding sources that have enabled this work to be carried out since 2001: the Foundation for Research, Science and Technology “Ecology of Seamounts” program (Contracts CO1X0028, CO1X0224, and CO1X0508), the Ministry for Business, Innovation and Employment “Vulnerable Deep-Sea Communities” project (Contract CO1X0906) with supplementary support from the NZ government Oceans 2020 program, NIWA Coasts and Oceans Centre, the Ministry of Fisheries (Contracts ZBD2000-04 and ZBD2008-50), and Ministry for Primary Industries (MPI; project BEN2014-02). The support of Martin Cryer, Rich Ford, and Mary Livingston (all MPI) is acknowledged.
Conflict of Interest Statement
The authors declare that the research was conducted in the absence of any commercial or financial relationships that could be construed as a potential conflict of interest.
The reviewer MC declared a past co-authorship with one of the authors DB to the handling Editor.
Acknowledgments
Thanks are due to the captain, officers and crew of the R. V. Tangaroa for their skills and expertise in carrying out the at times demanding survey schedules, and the support and hard work of the scientific teams on each of the surveys. A special thanks to Steve George for supporting the DTIS platform over many years. A large team of taxonomists supported biodiversity components of the above research projects and enabled reliable identification of the epifaunal invertebrates in the image data. There are too many to name them all, but key specialists for some of the major taxa include: Steven Cairns (scleractinian and octocorals), Dennis Opresko (antipatharian corals; both Smithsonian Institution, United States); Juan Sanchez (Universidad de los Andes, Colombia; octocorals); Dennis Gordon (bryozoans, general), Michelle Kelly (sponges), Di Tracey (corals), Karen Schnabel (crustaceans), Owen Anderson (echinoids), Niki Davey (holothurians), Kate Neill (asteroids), Alan Hart and Sadie Mills (general; all NIWA, New Zealand). Phil Sutton (NIWA) extracted and analyzed ARGO data over the period of the surveys. Comments on an earlier version of the manuscript by Martin Cryer (MPI), and the suggestions of journal reviewers are appreciated.
Supplementary Material
The Supplementary Material for this article can be found online at: https://www.frontiersin.org/articles/10.3389/fmars.2019.00063/full#supplementary-material
References
Althaus, F., Williams, A., Schlacher, T., Kloser, R., Green, M. A., Barker, B. A., et al. (2009). Impacts of bottom trawling on deep-coral ecosystems of seamounts are long-lasting. Mar. Ecol. Prog. Ser. 397, 279–294. doi: 10.3354/meps08248
Amoroso, R. O., Pitcher, C. R., Rijnsdorp, A. D., McConnaughey, R. A., Parma, A. M., Suuronen, P., et al. (2018). Bottom trawl fishing footprints on the world’s continental shelves. Proc. Natl. Acad. Sci. U.S.A. 115, E10275–E10282. doi: 10.1073/pnas.1802379115
Asch, R. G., and Collie, J. S. (2008). Changes in a benthic megafaunal community due to disturbance from bottom fishing and the establishment of a fishery closure. Fish. Bull. 106, 438–456.
Baillon, S., Hamel, J. F., Wareham, V. E., and Mercier, A. (2012). Deep cold-water corals as nurseries for fish larvae. Front. Ecol. Environ. 10, 351–356. doi: 10.1890/120022
Bolam, S. G., Schratzberger, M., and Whomersley, P. (2006). Macro- and meiofaunal recolonisation of dredged material used for habitat enhancement: temporal patterns in community development. Mar. Pollut. Bull. 52, 1746–1755. doi: 10.1016/j.marpolbul.2006.07.010
Boschen, R. E., Rowden, A. A., Clark, M. R., Barton, S. J., Pallentin, A., and Gardner, J. P. A. (2015). Megabenthic assemblage structure on three New Zealand seamounts: implications for seafloor massive sulfide mining. Mar. Ecol. Prog. Ser. 523, 1–14. doi: 10.3354/meps11239
Bradshaw, C., Veale, L. O., and Brand, A. R. (2002). The role of scallop-dredge disturbance in long-term changes in Irish Sea benthic communities: a re-analysis of an historical dataset. J. Sea Res. 47, 161–184. doi: 10.1016/S1385-1101(02)00096-5
Brodie, S., and Clark, M. R. (2003). “The New Zealand seamount management strategy – steps towards conserving offshore marine habitat,” in Aquatic Protected Areas: What Works Best and How do we Know? Proceedings of the World Congress on Aquatic Protected Areas, Cairns, Australia, August 2002, eds J. P. Beumer, A. Grant, and D. C. Smith (Cairns, QLD: Australian Society of Fish Biology).
Clark, M. R. (1999). Fisheries for orange roughy (Hoplostethus atlanticus) on seamounts in New Zealand. Oceanol. Acta 22, 593–602. doi: 10.1016/S0399-1784(00)88950-1
Clark, M. R., Althaus, F., Schlacher, T. A., Williams, A., Bowden, D. A., and Rowden, A. A. (2016). The impacts of deep-sea fisheries on benthic communities: a review. ICES J. Mar. Sci. 73, i51–i69. doi: 10.1371/journal.pone.0022588
Clark, M. R., Bowden, D. A., Baird, S. J., and Stewart, R. (2010a). Effects of fishing on the benthic biodiversity of seamounts of the “Graveyard” complex, northern Chatham Rise. N. Z. Aquat. Environ. Biodiv. Rep. 46, 1–40.
Clark, M. R., Rowden, A. A., Schlacher, T., Williams, A., Consalvey, M., Stocks, K. I., et al. (2010b). The ecology of seamounts: structure, function, and human impacts. Ann. Rev. Mar. Sci. 2, 253–278. doi: 10.1146/annurev-marine-120308-081109
Clark, M. R., Rowden, A. A., Wright, I., and Consalvey, M. (2010c). Graveyard seamounts. Oceanography 23, 146–147. doi: 10.1371/journal.pone.0036897
Clark, M. R., and Dunn, M. R. (2012). Spatial management of deep-sea seamount fisheries: balancing sustainable exploitation and habitat conservation. Environ. Conserv. 39, 204–214. doi: 10.1017/S0376892912000021
Clark, M. R., and O’Driscoll, R. L. (2003). Deepwater fisheries and aspects of their impact on seamount habitat in New Zealand. J. Northwest Atlant. Fish. Sci. 31, 441–458. doi: 10.2960/J.v31.a34
Clark, M. R., and Rowden, A. A. (2009). Effect of deep water trawling on the macro-invertebrate assemblages of seamounts on the Chatham Rise, New Zealand. Deep Sea Res. 56, 1540–1554. doi: 10.1016/j.dsr.2009.04.015
Clark, M. R., Vinnichenko, V. I., Gordon, J. D. M., Beck-Bulat, G. Z., Kukharev, N. N., and Kakora, A. F. (2007). “Large-scale distant-water trawl fisheries on seamounts,” in Seamounts: Ecology, Fisheries & Conservation, eds T. J. Pitcher, T. Morato, P. J. B. Hart, M. R. Clark, N. Haggan, and R. S. Santos (Oxford: Blackwell). doi: 10.1002/9780470691953.ch17
Collie, J. S., Escanero, G. A., and Valentine, P. C. (1997). Effects of bottom fishing on the benthic megafauna of Georges bank. Mar. Ecol. Prog. Ser. 155, 159–172. doi: 10.3354/meps155159
Collie, J. S., Hall, S. J., Kaiser, M. J., and Poiner, I. R. (2000). A quantitative analysis of fishing impacts on shelf-sea benthos. J. Anim. Ecol. 69, 785–798. doi: 10.1046/j.1365-2656.2000.00434.x
Curtis, J. M. R., Poppe, K., and Wood, C. C. (2012). Indicators, Impacts and Recovery of Temperate Deepwater Marine Ecosystems Following Fishing Disturbance. Canadian Science Advisory Secretariat Research Document. Ottawa: Fisheries and Oceans Canada.
Davies, J. S., Stewart, H. A., Narayanaswamy, B. E., Jacobs, C., Spicer, J., Golding, N., et al. (2015). Benthic assemblages of the anton dohrn seamount (NE Atlantic): defining deep-sea biotopes to support habitat mapping and management efforts with a focus on vulnerable marine ecosystems. PLoS One 10:e0124815. doi: 10.1371/journal.pone.0124815
D’Onghia, G., Maiorano, P., Sion, L., Giove, A., Capezzuto, F., Carlucci, R., et al. (2010). Effects of deep-water coral banks on the abundance and size structure of the megafauna in the Mediterranean Sea. Deep Sea Res. Part II Topic. Stud. Oceanogr. 57, 397–411. doi: 10.1016/j.dsr2.2009.08.022
Du Preez, C., Curtis, J. M. R., and Clarke, M. E. (2016). The structure and distribution of benthic communities on a shallow seamount (Cobb Seamount, Northeast Pacific Ocean). PLoS One 11:e0165513. doi: 10.1371/journal.pone.0165513
Fallon, S. J., Thresher, R. E., and Adkins, J. (2014). Age and growth of the cold-water scleractinian Solenosmilia variabilis and its reef on SW Pacific seamounts. Coral Reefs 33, 31–38. doi: 10.1007/s00338-013-1097-y
FAO (2016). The State of World Fisheries and Aquaculture 2016: Contributing to Food Security and Nutrition for all. Rome: Food & Agriculture Organization.
Freese, L., Auster, P. J., Heifetz, J., and Wing, B. L. (1999). Effects of trawling on seafloor habitat and associated invertebrate taxa in the Gulf of Alaska. Mar. Ecol. Prog. Ser. 182, 119–126. doi: 10.3354/meps182119
Froese, R., and Proelss, A. (2010). Rebuilding fish stocks no later than 2015: will Europe meet the deadline? Fish Fish. 11, 194–202. doi: 10.1111/j.1467-2979.2009.00349.x
Gollner, S., Govenar, B., Arbizu, P. M., Mills, S., Le Bris, N., Weinbauer, M., et al. (2015). Differences in recovery between deep-sea hydrothermal vent and vent-proximate communities after a volcanic eruption. Deep Sea Res. Part I Oceanogr. Res. Papers 106, 167–182. doi: 10.1016/j.dsr.2015.10.008
Gollner, S., Kaiser, S., Menzel, L., Jones, D. O. B., Brown, A., Mestre, N. C., et al. (2017). Resilience of benthic deep-sea fauna to mining activities. Mar. Environ. Res. 129, 76–101. doi: 10.1016/j.marenvres.2017.04.010
Hall-Spencer, J., Allain, V., and Fossa, J. H. (2002). Trawling damage to Northeast Atlantic ancient coral reefs. Proc. R. Soc. Lond. Ser. B Biol. Sci. 269, 507–511. doi: 10.1098/rspb.2001.1910
Hiddink, J. G., Jennings, S., Sciberras, M., Szostek, C. L., Hughes, K. M., Ellis, N., et al. (2017). Global analysis of depletion and recovery of seabed biota after bottom trawling disturbance. Proc. Natl. Acad. Sci. U.S.A. 114, 8301–8306. doi: 10.1073/pnas.1618858114
Hill, P. (2009). Designing a deep-towed camera vehicle using single conductor cable. Sea Technol. 50, 49–51.
Hinz, H., Prieto, V., and Kaiser, M. J. (2009). Trawl disturbance on benthic communities: chronic effects and experimental predictions. Ecol. Appl. 19, 761–773. doi: 10.1890/08-0351.1
Jennings, S., Dinmore, T. A., Duplisea, D. E., Warr, K. J., and Lancaster, J. E. (2001). Trawling disturbance can modify benthic production processes. J. Anim. Ecol. 70, 459–475. doi: 10.1046/j.1365-2656.2001.00504.x
Johnston, P. A., and Santillo, D. (2004). Conservation of seamount ecosystems: application of a marine protected areas concept. Arch. Fish. Mar. Res. 51, 305–319.
Jones, H. P., and Schmitz, O. J. (2009). Rapid recovery of damaged ecosystems. PLoS One 4:e5653. doi: 10.1371/journal.pone.0005653
Kaiser, M. J., Clarke, K. R., Hinz, H., Austen, M. C. V., Somerfield, P. J., and Karakassis, I. (2006). Global analysis of response and recovery of benthic biota to fishing. Mar. Ecol. Prog. Ser. 311, 1–14. doi: 10.3354/meps311001
Kaiser, M. J., Collie, J. S., Hall, S. J., Jennings, S., and Poiner, I. R. (2002). Modification of marine habitats by trawling activities: prognosis and solutions. Fish Fish. 3, 114–136. doi: 10.1046/j.1467-2979.2002.00079.x
Kaiser, M. J., and Jennings, S. (2002). Ecosystem Effects of Fishing. Hoboken, NJ: Blackwell Publishing. doi: 10.1002/9780470693919.ch16
Kaiser, M. J., Ramsay, K., Richardson, C. A., Spence, F. E., and Brand, A. R. (2000). Chronic fishing disturbance has changed shelf sea benthic community structure. J. Anim. Ecol. 69, 494–503. doi: 10.1046/j.1365-2656.2000.00412.x
Koslow, J. A., Gowlett-Holmes, K., Lowry, J. K., O’Hara, T., Poore, G. C. B., and Williams, A. (2001). Seamount benthic macrofauna off southern Tasmania: community structure and impacts of trawling. Mar. Ecol. Prog. Ser. 213, 111–125. doi: 10.3354/meps213111
Lacharite, M., and Metaxas, A. (2013). Early life history of deep-water gorgonian corals may limit their abundance. PLoS One 8:e65394. doi: 10.1371/journal.pone.0065394
Lambert, G. I., Jennings, S., Kaiser, M. J., Davies, T. W., and Hiddink, J. G. (2014). Quantifying recovery rates and resilience of seabed habitats impacted by bottom fishing. J. Appl. Ecol. 51, 1326–1336. doi: 10.1111/1365-2664.12277
Lotze, H. K., Coll, M., Magera, A. M., Ward-Paige, C., and Airoldi, L. (2011). Recovery of marine animal populations and ecosystems. Trends Ecol. Evol. 26, 595–605. doi: 10.1016/j.tree.2011.07.008
Miller, K. J., and Gunasekera, R. M. (2017). A comparison of genetic connectivity in two deep sea corals to examine whether seamounts are isolated islands or stepping stones for dispersal. Nat. Sci. Rep. 7:46103. doi: 10.1038/srep46103
Montagna, P. A., Baguley, J. G., Cooksey, C., and Hyland, J. L. (2017). Persistent impacts to the deep soft-bottom benthos one year after the deepwater horizon event. Integr. Environ. Assess. Manag. 13, 342–351. doi: 10.1002/ieam.1791
Morato, T., Pitcher, T. J., Clark, M. R., Menezes, G., Tempera, F., Porteiro, F., et al. (2010). Can we protect seamounts for research? A call for conservation. Oceanography 23, 190–199. doi: 10.5670/oceanog.2010.71
Probert, P. K., Christiansen, S., Gjerde, K. M., Gubbay, S., and Santos, R. S. (2007). “Management and conservation of seamounts,” in Seamounts: Ecology, Fisheries & Conservation, eds T. J. Pitcher, T. Morato, P. J. B. Hart, M. R. Clark, N. Haggan, and R. S. Santos (Hoboken, NJ: Blackwell Publishing Ltd).
R Core Team (2013). R: A Language and Environment for Statistical Computing. Vienna: R Foundation for Statistical.
Rogers, A. D. (2018). The biology of seamounts: 25 years on. Adv. Mar. Biol. 79, 137–223. doi: 10.1016/bs.amb.2018.06.001
Rowden, A. A., Clark, M. R., and Wright, I. C. (2005). Physical characterisation and a biologically focused classification of “seamounts” in the New Zealand region. N. Z. J. Mar. Freshw. Res. 39, 1039–1059. doi: 10.1080/00288330.2005.9517374
Rowden, A. A., Oliver, M., Clark, M. R., and MacKay, K. (2008). New Zealand’s “SEAMOUNT” Database: Recent Updates and its Potential Use for Ecological Risk Assessment. New Zealand Aquatic Environment and Biodiversity Report No. 27. Wellington: Ministry of Fisheries Wellington.
Rowden, A. A., Schlacher, T. A., Williams, A., Clark, M. R., Stewart, R., Althaus, F., et al. (2010). A test of the seamount oasis hypothesis: seamounts support higher epibenthic megafaunal biomass than adjacent slopes. Mar. Ecol. 31, 95–106. doi: 10.1111/j.1439-0485.2010.00369.x
Sainsbury, K. J., Campbell, R. A., Lindholm, R., and Whitelaw, A. W. (1997)). “Experimental management of an Australian multispecies fishery: examining the possibility of trawl-induced habitat modification,” in Global Trends: Fisheries Management. American Fisheries Society Symposium 20, eds E. L. Pikitch, D. D. Huppert, and M. P. Sissenwine (Rockville, MD: Bethesda).
Schlacher, T. A., Baco, A. R., Rowden, A. A., O’Hara, T. D., Clark, M. R., Kelley, C., et al. (2014). Seamount benthos in a cobalt-rich crust region of the central Pacific: conservation challenges for future seabed mining. Div. Distribut. 20, 491–502. doi: 10.1111/ddi.12142
Schloder, C., O’Dea, A., and Guzman, H. M. (2013). Benthic community recovery from small-scale damaage on marginal Caribbean reefs: an example from Panama. Bull. Mar. Sci. 89, 1003–1013.
Sheehan, E. V., Stevens, T. F., Gall, S. C., Cousens, S. L., and Attrill, M. J. (2013). Recovery of a temperate reef assemblage in a marine protected area following the exclusion of towed demersal fishing. PLoS One 8:e83883. doi: 10.1371/journal.pone.0083883
Smith, C. R. (1994). Tempo and mode in deep-sea benthic ecology - punctuated equilibrium revisited. Palaios 9, 3–13. doi: 10.2307/3515074
Teixido, N., Garrabou, J., Gutt, J., and Arntz, W. E. (2004). Recovery in Antarctic benthos after iceberg disturbance: trends in benthic composition, abundance and growth forms. Mar. Ecol. Prog. Ser. 278, 1–16. doi: 10.3354/meps278001
Tracey, D. M., Anderson, O. F., and Naylor, J. R. (2011a). A Guide to Common Deepsea Invertebrates in New Zealand Waters. New Zealand Aquatic Environment and Biodiversity Report 86. Wellington: Ministry of Fisheries.
Tracey, D. M., Rowden, A. A., Mackay, K. A., and Compton, T. (2011b). Habitat-forming cold-water corals show affinity for seamounts in the New Zealand region. Mar. Ecol. Prog. Ser. 430, 1–22. doi: 10.3354/meps09164
Van Dover, C. L., Aronson, J., Pendleton, L., Smith, S., Arnaud-Haond, S., Moreno-Mateos, D., et al. (2014). Ecological restoration in the deep sea: desiderata. Mar. Pol. 44, 98–106. doi: 10.1016/j.marpol.2013.07.006
Victorero, L., Robert, K., Robinson, L. F., Taylor, M. L., and Huvenne, V. A. I. (2018). Species replacement dominates megabenthos beta diversity in a remote seamount setting. Sci. Rep. 8:4152. doi: 10.1038/s41598-018-22296-8
Williams, A., Schlacher, T. A., Rowden, A. A., Althaus, F., Clark, M. R., Bowden, D. A., et al. (2010). Seamount megabenthic assemblages fail to recover from trawling impacts. Mar. Ecol. 31, 183–199. doi: 10.1111/j.1439-0485.2010.00385.x
Worm, B., Barbier, E. B., Beaumont, N., Duffy, J. E., Folke, C., Halpern, B. S., et al. (2006). Impacts of biodiversity loss on ocean ecosystem services. Science 314, 787–790. doi: 10.1126/science.1132294
Worm, B., Hilborn, R., Baum, J. K., Branch, T. A., Collie, J. S., Costello, C., et al. (2009). Rebuilding global fisheries. Science 325, 578–585. doi: 10.1126/science.1173146
Yesson, C., Fisher, J., Gorham, T., Turner, C. J., Arboe, N. H., Blicher, M. E., et al. (2017). The impact of trawling on the epibenthic megafauna of the west Greenland shelf. ICES J. Mar. Sci. 74, 866–876.
Keywords: trawling impact, ecological resilience, recovery, time series, benthic fauna, deep sea, New Zealand
Citation: Clark MR, Bowden DA, Rowden AA and Stewart R (2019) Little Evidence of Benthic Community Resilience to Bottom Trawling on Seamounts After 15 Years. Front. Mar. Sci. 6:63. doi: 10.3389/fmars.2019.00063
Received: 10 November 2018; Accepted: 05 February 2019;
Published: 26 February 2019.
Edited by:
Ricardo Serrão Santos, Universidade dos Açores, PortugalReviewed by:
Philippe Maurice Cury, Institute for Research and Development, Sri LankaMarina R. Cunha, University of Aveiro, Portugal
Copyright © 2019 Clark, Bowden, Rowden and Stewart. This is an open-access article distributed under the terms of the Creative Commons Attribution License (CC BY). The use, distribution or reproduction in other forums is permitted, provided the original author(s) and the copyright owner(s) are credited and that the original publication in this journal is cited, in accordance with accepted academic practice. No use, distribution or reproduction is permitted which does not comply with these terms.
*Correspondence: Malcolm R. Clark, TWFsY29sbS5DbGFya0BuaXdhLmNvLm56