- Center for Global Discovery and Conservation Science, Arizona State University, Tempe, AZ, United States
Coral reefs face an uncertain future and may not recover naturally from anthropogenic climate change. Coral restoration is needed to rehabilitate degraded reefs and to sustain biodiversity. There is a need for baseline data on global reef distribution, composition, and condition to provide targets for conservation and restoration. Remote sensing can address this issue and is currently underutilized in reef research and restoration. This synthesis integrates current capabilities of remote sensing with key reef restoration criteria, to facilitate coral restoration success. Research into the development of a spectral database for corals, linking habitat type and extent with predator abundance, and identification of species-specific acoustic signatures are needed to advance the use of remote sensing in reef restoration design and monitoring. Reciprocally, reef restoration efforts should innovate at ecosystem, regional, and global levels using remote sensing, to preserve as much of the coral reef biome as possible with continued ocean-climate change.
Introduction
Anthropogenic greenhouse gas emissions are causing major changes in the land and sea, with variable effects temporally and spatially (Le Quere et al., 2016). Consequentially, coral reefs are facing ecological collapse, where an estimated 70–90% of coral reefs could disappear by 2050 if emissions are not curbed (van Hooidonk et al., 2016; Hughes et al., 2018). Millions of people depend on intact reefs for various socioeconomic and ecological values, and thus these ecosystems are crucial to protect (de Groot et al., 2012; Ferrario et al., 2014).
Reefs may be unable to recover naturally, where active restoration can be used to rehabilitate degraded reefs (Goreau and Hilbertz, 2005; Mumby and Steneck, 2008). Many organizations undertake coral reef restoration, planting thousands of corals every year (Ladd et al., 2018). However, high spatial variability in reef condition limits our knowledge of where restoration is needed. Remote sensing can help to provide a global map of reef distribution and condition at high spatial resolution and temporal frequency, improving accuracy in determining the regional impacts of climate change on reefs.
A fusion of remote sensing and coral restoration science is needed to yield the most efficient approaches for determining where to apply restoration effort to maximize success as well as to increase the scalability. This review focuses on a merging of these two scales, beginning with a description of remote sensing and its application to coral reefs. Restoration protocols are then summarized. With a consideration of which remote sensing technology is most mature and developed, current technologies are matched to key restoration steps, with suggestions of potential future studies that will advance the use of remote sensing in coral reef restoration.
Remote Sensing of Coral Reefs
Remote sensing is the acquisition of physical characteristics of an area from a distance, through measuring its reflected and emitted radiation. Remote sensing is likely the only technology able to measure coral reef pressures from anthropogenic stressors at scales sufficiently large enough to capture widespread, often subtle change, or spatially- and temporally-localized, and episodic change (Hochberg et al., 2003; Hedley et al., 2016). Field-based monitoring of coral reefs is costly, time consuming and geographically limited, and some of the most substantial areas of reef are located in regions with poor access. Remote sensing greatly reduces the amount of time needed to survey an area (Mumby et al., 2004) and has also been shown to more accurately estimate habitat distribution in comparison to local environmental knowledge (Selgrath et al., 2016).
However, limitations in remote sensing exist. For example, incomplete remote sensing coverage can lead to inaccurate estimations of the coverage of different benthic types. Additionally, spectral mixing can sometimes limit the quantification of benthic cover. Lastly, accuracy of optical data is affected by depth and turbidity (Goodman et al., 2013; Hedley et al., 2016). Despite these limitations, current and forthcoming remote sensing technology provides an efficient way to monitor reefs with standardized measures and repeatable observations (Figure S1).
A broad suite of remote sensing technology exists (reviews: Goodman et al., 2013; Xu and Zhao, 2014; Hedley et al., 2016; Purkis, 2018). Passive remote sensing, which relies on reflected sunlight or emitted heat, includes photographic, multispectral, hyperspectral, and infrared imaging. Active remote sensing records a return signal from an emitted source, and includes laser-, radar-, and radio-based scanning approaches. These sensors can be deployed on various platforms, where the sensor and platform combination influence the spectral, spatial, and temporal resolution of the measurements (Table S1).
Current Remote Sensing Capabilities of Coral Reefs
Remote sensing is routinely used to examine coral reefs, with the Landsat series of sensors providing the longest time series available for some reefs, as far back as 1984 (Mumby and Edwards, 2002). Remote sensing can discriminate between different components of a reef ecosystem such as habitat type and physical structure (Asner et al., 2017; Roelfsema et al., 2018), measurements that are critical for reef restoration practitioners. It can also provide climate-relevant measurements such as temperature, salinity and wind energy, and biological estimates such as primary productivity (Purkis, 2018).
Various remote sensing technologies differ in measurement type as well as spatial, temporal and radiometric resolution, where different technologies use energy from different parts of the electromagnetic spectrum. Passive remote sensing approaches such as photographic, multispectral and hyperspectral imaging are best suited for assessing habitat characteristics in clear water <20 m depth. They are capable of fine spatial resolutions of <5 m up to moderate resolutions of 10–30 m (Table S1, Goodman et al., 2013).
Active remote sensing technologies are also suited for the measurement of various reef properties. Laser scanning, mostly centered on LiDAR (Light Detection and Ranging) techniques, is often used for measuring bathymetry, seafloor topography, and geomorphology in water <40 m depth with a resolution of around 5 m or coarser. Other active sensors such as radio and radar, which are best suited for measuring near-surface waves and currents, provide information at a resolution of 25 m to > 1 km. Thermal sensors, best suited for sea surface temperature (SST) typically provide more coarse mapping results at about 1 km resolution, as they are mainly deployed on satellites.
Acoustic sensing can be both passive and active. Passive sensors, such as hydrophones, convert underwater sound into electrical signals. Active sensors, such as SONAR (Sound Navigation and Ranging), combine a sound source with a hydrophone utilizing the concept of echolocation to sense the surrounding environment (Fornshell and Tesei, 2013). Acoustic sensing is mainly deployed from ships and provides measurements in both the shallowest and deepest environments of more than 100 m depth. Sonar provides information on depth, seafloor topography, geomorphic zone, general habitat, water velocity, and fish presence (Table S1).
Coral Reef Restoration
Widespread in the Caribbean, many conservationists grow and outplant coral fragments onto degraded reefs. Pioneered in the 1990s “coral gardening,” involves the collection and culture of coral fragments and the outplanting of fragments onto reefs (Rinkevich, 2005; Lirman and Schopmeyer, 2016) where restoration programs currently operate in more than 150 coral nurseries across 20 countries (Lirman and Schopmeyer, 2016). In reef restoration, there are several clear objectives: (1) restore foundational species, (2) increase live coral biomass, (3) provide coastal protection, (4) increase structural complexity, (5) provide physical structure for associated fauna, (6) enhance biodiversity, and (7) increase physical connectivity between colonies (Ladd et al., 2018).
The propagation stage of coral restoration methodology is well-developed with thousands of corals nursery-grown and outplanted every year (Lirman and Schopmeyer, 2016). On the other hand, the success of outplanted corals is mixed as there are many natural processes that can affect their survival (Lirman et al., 2014; Ladd et al., 2018). Outplanted corals can experience rapid mortality when placed on macroalgal overgrown reefs, areas with low herbivore densities and increased coral predator density, and areas with unfavorable water chemistry (Lirman and Schopmeyer, 2016; Muehllehner et al., 2016). Outplanting represents the largest expense and most time-consuming step of restoration and thus identifying appropriate outplanting regions will maximize outplanting survival.
Protocols for Coral Propagation and Outplanting
Coral fragments are grown in nurseries until they reach a size that is suitable for outplanting (Rinkevich, 2005). Nursery propagation is successful, with high survival and growth equal to or higher than wild rates (Schopmeyer et al., 2017). The majority of coral propagation has been through asexual reproduction, also known as fragmentation, which can occur naturally through wave activity (Barton et al., 2017). Fragments can also be harvested from established coral colonies where multiple colonies can be fragmented to increase the genetic diversity of outplants (Schopmeyer et al., 2017). Sexually-derived propagules can be collected in situ from spawning corals with non-invasive collection devices, and also from colonies in aquaria but is limited as spawning usually occurs once or twice a year (Babcock et al., 1986). In this case, artificial substrates must be provided in the nursery to allow the larvae to settle, and highly filtrated water is needed to reduce pests and pathogens.
The majority of restoration effort involves outplanting nursery-grown fragments. Corals can be attached directly to the reef using epoxy, cement, or ties or attached to platforms which, in turn, are attached to the reef. Outplanting of larger fragments is often more successful, with larger corals reaching reproductive maturity quicker and having greater fecundity than smaller ones (Herlan and Lirman, 2008; Lirman et al., 2010).
Larval seeding, by which large quantities of coral larvae are either released onto the reef or first settled onto structures that are lodged into the existing reef, are other methods which may reduce outplanting costs as well as the time and space involved in the maintenance of coral fragments (Chamberland et al., 2017; dela Cruz and Harrison, 2017). Success with this approach is, however, quite low, and outplanting coral fragments continues to be the mainstream outplanting approach (Edwards et al., 2015).
Parameters Considered by Restoration Practitioners for Outplanting
According to reef restoration practitioners, the three key factors considered when choosing which reef system to restore are: (1) existing coral cover, (2) available clean substrate, and (3) water depth (Ladd et al., 2018), factors which are all able to be remotely sensed. When considering which area within a reef to outplant, the three main factors are: (1) available substrate, (2) avoidance of benthic competitors, and (3) outplanting near herbivores (Ladd et al., 2018), with the full list of parameters considered in outplanting summarized in Table S2.
The best reef transplant design will fail in a poor location thus, site selection is key to successful restoration, establishment and growth of transplants. The use of remote sensing can optimize the process of determining the best site to outplant, by providing complete areal coverage of reef systems and assessments of multiple criteria considered to be key to successful restoration. Table S2 links all parameters considered by restoration practitioners to their ability to be remotely sensed.
Maintenance and Monitoring of Outplants
After outplanting, maintenance, and monitoring can represent the next biggest costs for practitioners, where outplants are susceptible to dislodgement, storm damage, disease, bleaching, and predation (Fabian et al., 2013; Drury et al., 2017; Ladd et al., 2017; Schopmeyer et al., 2017). Monitoring of restoration success is done through labor intensive field surveys and manual photography to assess traits such as coral growth and survival (Precht, 2006; Hein et al., 2018).
Of all restoration parameters, monitoring of transplantation success is least considered and due to constraints and costs, the majority of outplant projects (53% of peer reviewed studies) are monitored for 1 year or less (Fabian et al., 2013; Hein et al., 2018). To date, coral reef restoration has primarily focused on short-term experiments, with no consistent monitoring procedures in place (Meesters et al., 2015). Remote sensing can therefore play a key role in standardizing the protocol to evaluate restoration success.
In line with terrestrial restoration guidelines (Ruiz-Jaen and Aide, 2005), there are six ecological benchmarks recommended for evaluating coral restoration success: (1) benthic coral cover, (2) productivity/recruitment of outplants, (3) coral diversity, (4) coral health, (5) contribution of outplants to reef complexity, and (6) diversity and biomass of associated fauna supported by restored sites (Schopmeyer et al., 2017; Hein et al., 2018) where most of these factors can be remotely sensed (Table S2). Furthermore, restored sites should be compared to nearby control or reference sites (Hein et al., 2018) to determine whether outplants are driving the increase in reef health.
Scaling up Reef Restoration With Remote Sensing
Outplanting is the most labor-intensive and expensive part of the restoration effort (Fabian et al., 2013; Hein et al., 2018). As future climate change ensues, it is clear there needs to be a more methodical way to identify which reefs are worth restoring and which reefs have a heightened chance of surviving projected changes. Remote sensing is a key system in determining these locations (Tables S1, S2).
Over the past 30 years, certain remote sensing technologies have matured and are best suited to measure specific reef characteristics. For example, LiDAR has proven highly accurate for mapping reef bathymetry. Hyperspectral sensors are currently underutilized, with the potential to provide much more detailed benthic habitat information than traditional multispectral sensors (Caras et al., 2017). Lastly, the majority of the passive remote sensing approaches can be hindered by water turbidity, and other systems such as acoustic sensors can surpass such approaches in certain scenarios, particularly in water more than 50 m in depth (Table S1).
Selecting Suitable Technology for Coral Restoration Criteria
Certain reefs are projected to experience a lower level of climate change threat (Beyer et al., 2018), and perhaps outplanting efforts should be targeted to these areas. For other regions more likely to experience greater climate change threat, it is essential to outplant resilient genotypes, or genotypes with well-matched traits to ensure survival, where remote sensing can assist by providing information on various abiotic conditions (e.g., water quality, sedimentation, and temperature). Of the remote sensing technologies available, satellite-based multispectral technology is the most mature, tested and suited for determining the general distribution of reef geomorphology and benthic cover. With daily imaging available for some satellite technologies, e.g., Planet (Asner et al., 2017), multispectral technology can help to identify reefs to restore through classification of the size and extent of the coral reef (Roelfsema et al., 2014; Selgrath et al., 2016).
After deciding which reef to restore, the area within the reef best suited for outplanting must be identified. As 50% of reef outplanting sites cover an area of just 10–1,000 m2 (Fabian et al., 2013), spatial resolution finer than most satellite-based sensors is needed to provide detailed site characteristics relevant for outplanting. Airborne hyperspectral remote sensing (also known as imaging spectroscopy) is at the technological forefront of optical sensing, providing the most detailed benthic habitat maps (Goodman et al., 2013; Hedley, 2013; Caras et al., 2017) successfully classifying benthos into bottom-types such as fleshy algae, turf algae, seagrass, coral, and coral rubble (Hochberg et al., 2003). By accessing data over time, changes in benthic maps can inform changes in land use, coastal development and reef connectivity (Mumby et al., 2004; Raitsos et al., 2017) (Table 1; Table S2, Figure 1; Figure S1).
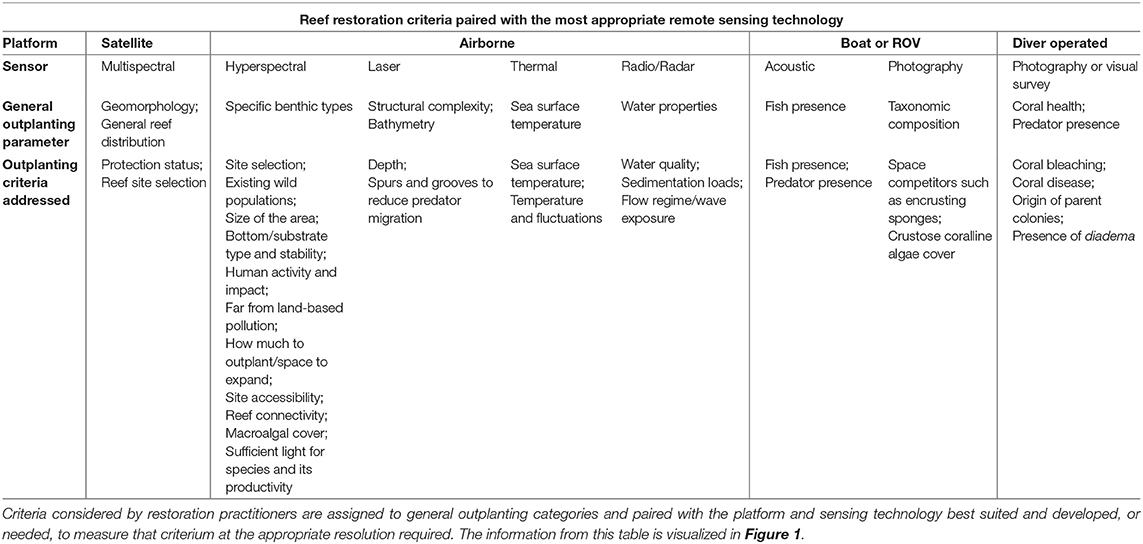
Table 1. Remote sensing technologies and their application to informing coral reef outplanting and restoration success.
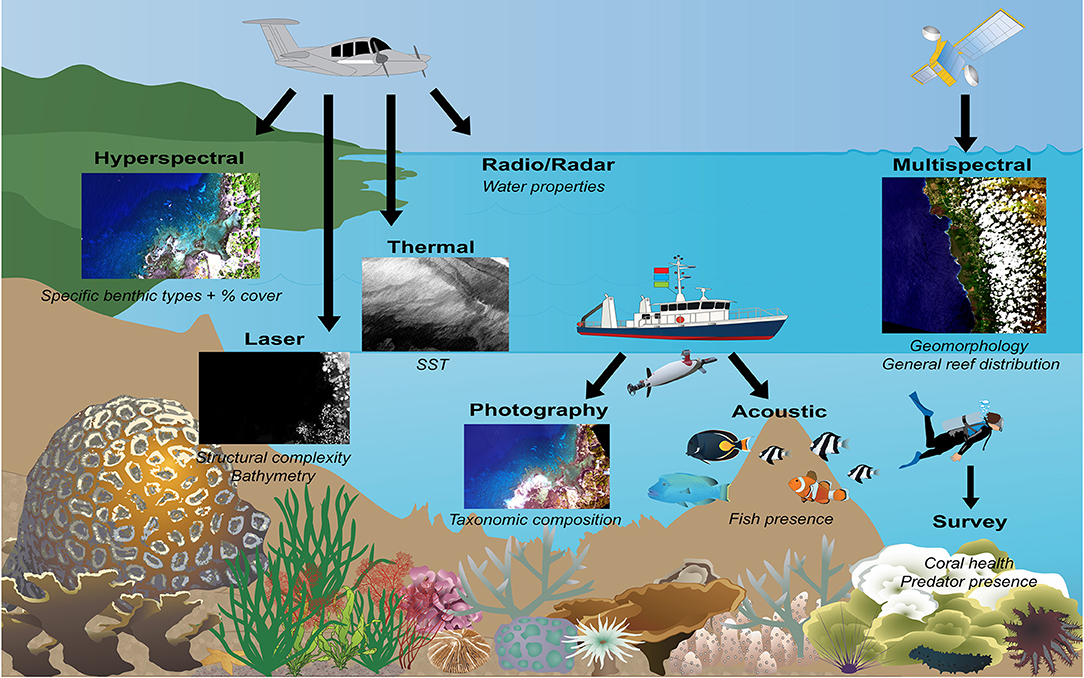
Figure 1. Remotely sensed measurements that can inform coral reef restoration activities. Key criteria considered by restoration practitioners are paired with the current platform and sensing technology that is most mature and developed, for the criterion at the required resolution to inform fragment collection, coral outplanting, and monitoring efforts. Remote sensing technology examples are provided by the Carnegie Airborne Observatory (Asner et al., 2012) where possible, for the same section of reef at representative resolutions. Symbols are courtesy of the Integration and Application Network, University of Maryland Center for Environmental Science (ian.umces.edu/symbols/).
Airborne laser scanners are currently the best demonstrated technology for providing detailed bathymetry and rugosity for water depths <50 m and surveys <100 km2 (Rohmann and Monaco, 2005) where LiDAR derived rugosity is highly aligned with in-situ measurements of rugosity (Wedding et al., 2008). Important biophysical properties of the water can be measured by airborne radiometer and radar sensors, where pollution, algal blooms and turbidity, all of which are important restoration considerations, can be inferred from the measured variables providing essential water quality parameters (Devlin et al., 2015; Petus et al., 2016). On the other hand, the use of thermal sensors has mainly been satellite based. To inform outplanting, a finer resolution is needed, which means coupling thermal sensors with airborne platforms.
For the finest resolutions of <0.1 m, boat-based, remotely operated vehicles (ROV) or low-flying aircraft (manned or unmanned aerial vehicle) approaches are needed (Table 1; Figure 1). Acoustic sensors deployed from boats can provide estimates of fish presence and abundance (Zwolinski et al., 2009). Photography is currently the best suited technology to assess taxonomic composition and requires a boat-based platform to provide information at the resolution needed for restoration. The main advantage of using boat-based platforms is the removal of surface reflected glint, a major confounding factor in remote sensing for above-water sensors (Garaba and Zielinski, 2013). Low-flying aircraft technologies, such as the CAO can currently map at 4 cm resolution (G.P. Asner, unpub. data), and with further development can provide maps at increased resolutions, which currently only boat-based sensors can provide.
Currently, in-water surveys are still needed to identify biotic variables such as coral disease and predator presence, where remote sensing technologies are currently unable to provide information on these parameters (Table S2). There is potential for remote sensing to detect healthy and diseased corals where these can be delineated based on spectral signatures (Holden and LeDrew, 2001; Anderson et al., 2013). Furthermore, with the development of acoustic sensors and interpretation of the data, there is the possibility of identifying and quantifying the presence and abundance of coral predators, eventually reducing the need for in-water surveys (Montgomery and Radford, 2017).
Lastly, remote sensing has an important role to play in monitoring restoration success, where many of the key ecological metrics of success such as coral cover, diversity, and structural complexity of restored sites are easily mapped with the techniques described above (Table S2). On the other hand, measuring reproductive output of outplants, coral health and diversity of associated fauna, remain difficult to detect with remote sensing techniques.
Coral reef restoration is expensive, with costs estimated from $1,717 up to $2,879,773 USD per hectare (Bayraktarov et al., 2015). This enormous range is due to the different phases of restoration and various methods employed where research suggests that current costs outweigh benefits (De Groot et al., 2013). The use of remotely sensed data can be economically incorporated into restoration activities as there is a plethora of freely available satellite data. For example, multispectral, 10 m spatial resolution data at 5-day intervals from satellites Sentinel-2A and 2B are freely available as well as Landsat data at 30 m resolution, which has been extensively used for coral reef applications (Palandro et al., 2008; Hedley et al., 2018). Furthermore, the Coral Reef Watch program by NOAA offers free ocean data resources including sea surface temperature, chlorophyll, winds, and ultraviolet radiation. For higher resolution data, airborne mapping of large areas (thousands to millions of hectares) costs ~$0.01 USD per hectare at non-profit rates (Asner et al., 2014). Thus, the integration of remote sensing with coral restoration is a cost-effective way to increase restoration success.
In sum, satellite and airborne remote sensing can provide information on which reef, and where within the reef to restore as well as reef extent and connectivity. Furthermore, these technologies provide a cost-effective way to determine sites with the highest water quality, maximal distance from land-based pollution and minimal site accessibility (Petus et al., 2016). A clear advantage of remote sensing is the ability to compare restored sites to natural reference reefs to fully determine whether reef recovery is driven by restoration efforts. When acute disturbances occur, restoring the coral soon after the disturbance could help to maintain associated biota already present (Dixson et al., 2014), and remote sensing provides a timely and efficient method to identify these regions. Using an approach informed by multi-platform remote sensing will greatly increase the chance of outplanting success, reduce the risk that restoration efforts are wasted, and help to achieve long-term coral conservation outcomes.
Potential Areas of Research
Sensor technology and processing algorithms continue to develop and drive forward the capability of mapping and monitoring of coral reefs, especially with respect to spatial resolution and biological detail (Purkis, 2018). Numerous parameters considered important for informing restoration work can be estimated remotely however, to maximize the use of the measurements provided, there are several areas of research needed to bridge the gap between reef restoration work and current remote sensing capabilities.
First, we need to develop spectral databases for different reef benthos in both healthy and unhealthy states, to increase the number of benthic types that hyperspectral and multispectral technologies can distinguish. This would be especially important for identifying species of crustose coralline algae, a substrate which induces the larval settlement of many organisms including coral (Hadfield and Paul, 2001). Distinguishing corals down to the species level, through various spectral features is underway (Torres et al., 2015), and if successful, will eventually reduce the need for diver- or boat-operated measurements.
Second, we need to develop empirical relationships between biotic factors and habitat characteristics that can help predict the presence and abundance of coral predators. Currently, diver-level surveys are needed to obtain this information. Determining a link between predator abundance and habitat type and extent would eliminate the need for in-water surveys.
Third, we need to understand density-dependent effects within and between coral colonies and entire reefs in order to determine the scale and resolution at which remote sensing can be most effective. A key goal of reef restoration is to facilitate self-seeding cascades and natural regeneration, however there is a lack of information on how outplanting influences reef connectivity and larval seeding. These types of studies would help to identify the ideal size, extent and position of an outplant area that will most likely facilitate the recovery of adjacent reefs.
Finally, the interpretation of acoustic data in a reef context requires continued development. Acoustic data, currently the technology that can be used in the deepest water, are relatively insensitive to turbid water, and have the potential to document both fish presence and abundance. Marine bioacoustics, the study of how marine animals use and perceive sound, is a growing field, with an aim to link reef fauna with reef-specific acoustic signatures (Montgomery and Radford, 2017). There is potential for acoustic technology to develop into the foremost remote sensing technology used to directly sense fauna, which might then be integrated with habitat data derived from other remote sensing techniques discussed.
An integrated use of multiple sensor technologies will provide a comprehensive view of the reef ecosystem. Research in these four areas will drive forward the use of remote sensing technologies in reef restoration efforts and many other important applications. While such integration may currently be impractical for reef restoration activities, subsets of the technology could be applied based on the relative importance of different restoration factors for any given reef system.
Conclusions
Widespread degradation of reef ecosystems is expected under climate change, and thus strategic planning and technology-assisted restoration are crucial to ensure the long-term survival of coral reefs. Investment into coral reef restoration will be insufficient if efforts are not spatially and temporally scalable. Remote sensing can provide a powerful avenue to help achieve reef restoration objectives. While remote sensing may not provide the fine level detail of a field survey, it can reveal both large- and small-scale patterns. Current needs include improved coral spectral remote sensing databases, species-specific acoustic signatures, identification of key landscape and animal interactions, and increased integration of multiple remote sensing technologies. While addressing the root causes of reef devastation such as climate change, overfishing and pollution remain essential, geospatially-informed restoration methods will improve the chance of preserving coral reef ecosystems far into the future.
Author Contributions
All authors listed have made a substantial, direct and intellectual contribution to the work, and approved it for publication.
Conflict of Interest Statement
The authors declare that the research was conducted in the absence of any commercial or financial relationships that could be construed as a potential conflict of interest.
Acknowledgments
This study was supported by the Leonardo DiCaprio Foundation, and is part of the Reefscape project1. We thank Nicholas Fabina and Nicholas Vaughn for helpful comments on the review and David Knapp for providing images for the figures. We also thank the two reviewers for their valuable comments which have greatly improved the manuscript.
Supplementary Material
The Supplementary Material for this article can be found online at: https://www.frontiersin.org/articles/10.3389/fmars.2019.00079/full#supplementary-material
Footnotes
References
Anderson, D. A., Armstrong, R. A., and Weil, E. (2013). Hyperspectral sensing of disease stress in the Caribbean reef-building coral, Orbicella faveolata – Perspectives for the field of coral disease monitoring. PLoS ONE 8:e81478. doi: 10.1371/journal.pone.0081478
Asner, G. P., Knapp, D. E., Boarman, J., Green, R. O., Kennedy-Bowdoin, T., Eastwood, M., et al. (2012). Carnegie airborne observatory-2: increasing science data dimensionality via high-fidelity multi-sensor fusion. Remote Sens. Environ. 124, 454–465. doi: 10.1016/j.rse.2012.06.012
Asner, G. P., Knapp, D. E., Martin, R. E., Tupayachi, R., Anderson, C. B., Mascaro, J., et al. (2014). Targeted carbon conservation at national scales with high-resolution monitoring. Proc. Natl. Acad. Sci. U.S.A. 111, E5016–E5022. doi: 10.1073/pnas.1419550111
Asner, G. P., Martin, R. E., and Mascaro, J. (2017). Coral reef atoll assessment in the South China sea using planet dove satellites. Remote Sensing Ecol. Conserv. 3, 57–65. doi: 10.1002/rse2.42
Babcock, R. C., Bull, G. D., Harrison, P. L., Heyward, A. J., Oliver, J. K., Wallace, C. C., et al. (1986). Synchronous spawnings of 105 scleractinian coral species on the Great Barrier Reef. Mar. Biol. 90, 379–394. doi: 10.1007/BF00428562
Barton, J. A., Willis, B. L., and Huston, K. S. (2017). Coral propagation: a review of techniques for ornamental trade and reef restoration. Rev. Aquacult. 9, 238–256. doi: 10.1111/raq.12135
Bayraktarov, E., Saunders, M. I., Abdullah, S., Mills, M., Beher, J., Possingham, H. P., et al. (2015). The cost and feasibility of marine coastal restoration. Ecol. Appl. 26, 1055–1074. doi: 10.1890/15-1077
Beyer, H. L., Kennedy, E. V., Beger, M., Chen, C. A., Cinner, J., Darling, E., et al. (2018). Long-term risk-sensitive planning for conserving coral reefs under rapid climate change. Conserv. Lett. 11:e12587. doi: 10.1111/conl.12587
Caras, T., Hedley, J., and Karnieli, A. (2017). Implications of sensor design for coral reef detection: upscaling ground hyperspectral imagery in spatial and spectral scales. Int. J. Appl. Earth Observ. Geoinform. 63, 68–77. doi: 10.1016/j.jag.2017.07.009
Chamberland, V. F., Petersen, D., Guest, J. R., Petersen, U., Brittsan, M., and Vermeij, M. J. A. (2017). New seeding approach reduces costs and time to outplant sexually propagated corals for reef restoration. Sci. Rep. 7:18076. doi: 10.1038/s41598-017-17555-z
De Groot, R. S., Blignaut, J., Van der Ploeg, S., Aronson, J., Elmqvist, T., and Farley, J. (2013). Benefits of investing in ecosystem restoration. Conserv. Biol. 27, 1286–1293. doi: 10.1111/cobi.12158
de Groot, R. S., Brander, L., van der Ploeg, S., Costanza, R., Bernard, F., Braat, L., et al. (2012). Global estimates of the value of ecosystems and their services in monetary units. Ecosyst. Serv. 1, 50–61. doi: 10.1016/j.ecoser.2012.07.005
dela Cruz, D. W., and Harrison, P. L. (2017). Enhanced larval supply and recruitment can replenish reef corals on degraded reefs. Sci. Rep. 7:13985. doi: 10.1038/s41598-017-14546-y
Devlin, M. J., Petus, C., Da Silva, E., Tracey, D., Wolff, N. H., Waterhouse, J., et al. (2015). Water quality and river plume monitoring in the Great Barrier Reef: an overview of methods based on ocean colour satellite data. Remote Sensing 7, 12909–12941. doi: 10.3390/rs71012909
Dixson, D. L., Abrego, D., and Hay, M. E. (2014). Chemically mediated behavior of recruiting corals and fishes: a tipping point that may limit reef recovery. Science 345, 892–897. doi: 10.1126/science.1255057
Drury, C., Manzello, D., and Lirman, D. (2017). Genotype and local environment dynamically influence growth, disturbance response and survivorship in the threatened coral, Acropora cervicornis. PLoS ONE 12:e0174000. doi: 10.1371/journal.pone.0174000
Edwards, A. J., Guest, J. R., Heyward, A. J., Villanueva, R. D., Baria, M. V., Bollozos, I. S. F., et al. (2015). Direct seeding of mass-cultured coral larvae is not an effective option for reef rehabilitation. Mar. Ecol. Prog. Ser. 525, 105–116. doi: 10.3354/meps11171
Fabian, R., Beck, M., and Potts, D. (2013). Reef Restoration for Coastal Defense: A Review. Santa Cruz, CA: University of California, Santa Cruz.
Ferrario, F., Beck, M. W., Storlazzi, C. D., Micheli, F., Shepard, C. C., and Airoldi, L. (2014). The effectiveness of coral reefs for coastal hazard risk reduction and adaptation. Nat. Commun. 5:3794. doi: 10.1038/ncomms4794
Fornshell, J. A., and Tesei, A. (2013). The development of SONAR as a tool in marine biological research in the twentieth century. Int. J. Oceanogr. 2013:678621. doi: 10.1155/2013/678621
Garaba, S., and Zielinski, O. (2013). Methods in reducing surface reflected glint for shipborne above-water remote sensing. J. Eur. Optical Soc. Rapid Publ. 8:13058. doi: 10.2971/jeos.2013.13058
Goodman, J. A., Purkis, S. J., and Phinn, S. R. (2013). Coral Reef Remote Sensing: A Guide for Multi-Level Sensing Mapping and Assessment. Dordrecht: Springer. doi: 10.1007/978-90-481-9292-2
Goreau, T. J., and Hilbertz, W. (2005). Marine ecosystem restoration: costs and benefits for coral reefs. World Resour. Rev. 17, 375–409.
Hadfield, M. G., and Paul, V. J. (2001). “Natural chemical cues for settlement and metamorphosis of marine invertebrate larvae.” Marine Chemical Ecology, eds J. McClintock and B. Baker (Boca Raton: CRC Press), 431–462. doi: 10.1201/9781420036602.ch13
Hedley, J. D. (2013). “Hyperspectral Applications in Goodman,” in Coral Reef Remote Sensing: A Guide for Multi-Level Sensing Mapping and Assessment, eds S. J. Purkis and S. R. Phinn (Dordrecht: Springer), 79–112.
Hedley, J. D., Roelfsema, C., Brando, B., Giardino, C., Kutser, T., Phinn, S., et al. (2018). Coral reef applications of Sentinel-2: Coverage, characteristics, bathymetry and benthic mapping with comparison to Landsat 8. Remote Sens. Environ. 216, 598–614. doi: 10.1016/j.rse.2018.07.014
Hedley, J. D., Roelfsema, C. M., Chollett, I., Harborne, A. R., Heron, S. F., Weeks, S., et al. (2016). Remote sensing of coral reefs for monitoring and management: a review. Remote Sens. 8, 118–157. doi: 10.3390/rs8020118
Hein, M. Y., Willis, B. L., Beeden, R., and Birtles, A. (2018). The need for broader ecological and socioeconomic tools to evaluate the effectiveness of coral restoration programs. Restor. Ecol. 25, 873–883. doi: 10.1111/rec.12580
Herlan, J., and Lirman, D. (2008). “Development of a coral nursery program for the threatened coral Acropora cervicornis in Florida,” in Proceedings of 11th International Coral Reef Symposium (Fort Lauderdale, FL), 1244–1427.
Hochberg, E. J., Atkinson, M. J., and Andrefouet, S. (2003). Spectral reflectance of coral reef bottom-types worldwide and implications for coral reef remote sensing. Remote Sens. Environ. 85, 159–173. doi: 10.1016/S0034-4257(02)00201-8
Holden, H., and LeDrew, E. (2001). Hyperspectral dsicrimnation of healthy versus stressed corals using in situ reflectance. J. Coastal Res. 17, 850–858. https://www.jstor.org/stable/4300244
Hughes, T. P., Anderson, K. D., Connolly, S. R., Heron, S. F., Kerry, J. T., Lough, J. M., et al. (2018). Spatial and temporal patterns of mass bleaching of corals in the anthropocene. Science 359, 80–83. doi: 10.1126/science.aan8048
Ladd, M. C., Miller, M. W., Hunt, J. H., Sharp, W. C., and Burkepile, D. E. (2018). Harnessing ecological processes to facilitate coral restoration. Front. Ecol. Environ. 16, 239–247. doi: 10.1002/fee.1792
Ladd, M. C., Shantz, A. A., Bartels, E., and Burkepile, D. E. (2017). Thermal stress reveals a genotype-specific tradeoff between growth and tissue loss in restored Acropora cervicornis. Mar. Ecol. Prog. Ser. 572, 129–139. doi: 10.3354/meps12169
Le Quere, C., Andrew, R. M., Canadell, J. G., Sitch, S., Korsbakken, J. I., Peters, G. P., et al. (2016). Global carbon budget 2016. Earth Syst. Sci. Data 8, 605–649. doi: 10.5194/essd-8-605-2016
Lirman, D., and Schopmeyer, S. (2016). Ecological solutions to reef degradation: optimizing coral reef restoration in the Caribbean and western Atlantic. PeerJ 4:e2597. doi: 10.7717/peerj.2597
Lirman, D., Schopmeyer, S., Galvan, V., Drury, C., Baker, A. C., and Baums, I. (2014). Growth dynamics of the threatened Caribbean staghorn coral Acropora cervicornis: influence of host genotype, symbiont identity, colony size, and environmental setting. PLoS ONE 9:e2597. doi: 10.1371/journal.pone.0107253
Lirman, D., Thyberg, T., Herlan, J., Hill, C., Young-Lahiff, C., Schopmeyer, S., et al. (2010). Propagation of the threatened staghorn coral Acropora cervicornis: methods to minimize the impacts of fragment collection and maximize production. Coral Reefs 29, 729–735. doi: 10.1007/s00338-010-0621-6
Meesters, H. W. G., Smith, S. R., and Becking, L. E. (2015). A Review of Coral Reef Restoration Techniques. Institute for Marine Resources (IMARES), Bremerhaven.
Montgomery, J. C., and Radford, C. A. (2017). Marine bioacoustics. Curr. Biol. 27, 502–507. doi: 10.1016/j.cub.2017.01.041
Muehllehner, N., Langdon, C., Venti, A., and Kadko, D. (2016). Dynamics of carbonate chemistry, production, and calcification of the florida reef tract (2009–2010): evidence for seasonal dissolution. Global Biogeochem. Cycles 30, 661–688. doi: 10.1002/2015GB005327
Mumby, P. J., and Edwards, A. J. (2002). Mapping marine environments with IKONOS imagery: enhanced spatial resolution can deliver greater thematic accuracy. Remote Sens. Environ. 82, 248–257. doi: 10.1016/S0034-4257(02)00041-X
Mumby, P. J., Hedley, J. D., Chisholm, J. R. M., Clark, C. D., Ripley, H., and Jaubert, J. (2004). The cover of living and dead corals from airborne remote sensing. Coral Reefs 23, 171–183. doi: 10.1007/s00338-004-0382-1
Mumby, P. J., and Steneck, R. S. (2008). Coral reef management and conservation in light of rapidly evolving ecological paradigms. Trends Ecol. Evolut. 23, 555–563. doi: 10.1016/j.tree.2008.06.011
Palandro, D. A., Andréfouët, S., Hu, C., Hallock, P., Muller-Karger, F., Dustan, P., et al. (2008). Quantification of two decades of shallow-water coral reef habitat decline in the Florida keys national marine sanctuary using landsat data (1984–2002). Remote Sens. Environ. 112, 3388–3399. doi: 10.1016/j.rse.2008.02.015
Petus, C., Devlin, M., Thompson, A., McKenzie, L., Teixeira da Silva, E., Collier, C., et al. (2016). Estimating the exposure of coral reefs and seagrass meadows to land-sourced contaminants in river flood plumes of the great barrier reef: validating a simple satellite risk framework with environmental data. Remote Sensing 8, 210–240. doi: 10.3390/rs8030210
Precht, W. F. (2006). Coral Reef Restoration Handbook. Boca Raton: CRC Press. doi: 10.1201/9781420003796
Purkis, S. (2018). Remote sensing tropical coral reefs: the view from above. Ann. Rev. Mar. Sci. 10, 149–168. doi: 10.1146/annurev-marine-121916-063249
Raitsos, D. E., Brewin, R. J. W., Zhan, P., Dreano, D., Pradhan, Y., Nanninga, G. B., et al. (2017). Sensing coral reef connectivity pathways from space. Sci. Rep. 7:9338. doi: 10.1038/s41598-017-08729-w
Rinkevich, B. (2005). Conservation of coral reefs through active restoration measures: recent approaches and last decade progress. Environ. Sci. Technol. 39, 4333–4342. doi: 10.1021/es0482583
Roelfsema, C., Kovacs, E., Ortiz, J. C., Wolff, N. H., Callaghan, D., Wettle, M., et al. (2018). Coral reef habitat mapping: a combination of object-based image analysis and ecological modelling. Remote Sens. Environ. 208, 27–41. doi: 10.1016/j.rse.2018.02.005
Roelfsema, C. M., Lyons, M., Kovacs, E. M., Maxwell, P., Saunders, M. I., Samper-Villarreal, J., et al. (2014). Multi-temporal mapping of seagrass cover, species and biomass: a semi-automated object based image analysis approach. Remote Sens. Environ. 150, 172–187. doi: 10.1016/j.rse.2014.05.001
Rohmann, S. O., and Monaco, M. E. (2005). Mapping Southern Florida's Shallow-Water Coral Ecosystems: An Implementation Plan. NOAA Technical Memorandum NOS NCCOS 19.
Ruiz-Jaen, M. C., and Aide, T. M. (2005). Restoration success: how is it being measured? Restorat. Ecol. 13, 569–577. doi: 10.1111/j.1526-100X.2005.00072.x
Schopmeyer, S. A., Lirman, D., Bartels, E., Gilliam, D. S., Goergen, E. A., Griffin, S. P., et al. (2017). Regional restoration benchmarks for Acropora cervicornis. Coral Reefs 36, 1047–1057. doi: 10.1007/s00338-017-1596-3
Selgrath, J. C., Roelfsema, C., Gergel, S. E., and Vincent, A. C. G. (2016). Mapping for coral reef conservation: comparing the value of participatory and remote sensing approaches. Ecosphere 7:e01325. doi: 10.1002/ecs2.1325
Torres, J. L., Guild, L. S., Armstrong, R. A., Corredor, J. E., Zuluaga-Montero, A., and Polanco, R. (2015). Relative pigment composition and remote sensing reflectance of Caribbean shallow-water corals. PLoS ONE 10:e0143709. doi: 10.1371/journal.pone.0143709
van Hooidonk, R., Maynard, J., Tamelander, J., Gove, J., Ahmadia, G., Raymundo, L., et al. (2016). Local-scale projections of coral reef futures and implications of the Paris Agreement. Sci. Rep. 6:39666. doi: 10.1038/srep39666
Wedding, L. M., Friedlander, A. M., McGranaghan, M., Yost, R. S., and Monaco, M. E. (2008). Using bathymetric lidar to define nearshore benthic habitat complexity: implications for management of reef fish assemblages in Hawaii. Remote Sens. Environ. 112, 4159–4165. doi: 10.1016/j.rse.2008.01.025
Xu, J., and Zhao, D. (2014). Review of coral reef ecosystem remote sensing. Acta Ecol. Sin. 24, 19–25. doi: 10.1016/j.chnaes.2013.11.003
Keywords: remote sensing, coral reef restoration, coral reef, management, monitoring
Citation: Foo SA and Asner GP (2019) Scaling Up Coral Reef Restoration Using Remote Sensing Technology. Front. Mar. Sci. 6:79. doi: 10.3389/fmars.2019.00079
Received: 30 November 2018; Accepted: 11 February 2019;
Published: 13 March 2019.
Edited by:
John A. Cigliano, Cedar Crest College, United StatesReviewed by:
Andrew A. Shantz, Pennsylvania State University, United StatesZac H. Forsman, University of Hawaii at Manoa, United States
Copyright © 2019 Foo and Asner. This is an open-access article distributed under the terms of the Creative Commons Attribution License (CC BY). The use, distribution or reproduction in other forums is permitted, provided the original author(s) and the copyright owner(s) are credited and that the original publication in this journal is cited, in accordance with accepted academic practice. No use, distribution or reproduction is permitted which does not comply with these terms.
*Correspondence: Shawna A. Foo, sfoo@asu.edu