Fine-Scale Effects of Boat Moorings on Soft Sediment Communities Masked in Large-Scale Comparisons
- 1School of Biological, Earth and Environmental Sciences, University of New South Wales, Sydney, NSW, Australia
- 2Sydney Institute of Marine Science, Mosman, NSW, Australia
- 3Department of Environmental Sciences, Macquarie University, North Ryde, NSW, Australia
Population growth is driving the demand for recreational marine infrastructure, resulting in extensive coastal habitat modification. Boat moorings, for example, are popular for vessel storage and are known to damage seagrass communities, yet little is known about how they influence unvegetated sediment habitats. Here we investigate the effects of boat moorings on sediment infauna using metrics of community composition, diversity, total abundance and abundances of individual functional groups and dominant taxa. Metrics were compared at fine and larger spatial scales, to investigate how spatial variability affects the ecological assessments in soft-sedimentary environments. Fine-scale models revealed changes in community composition and mollusk abundance with the distance from moorings, while sediment grain size was also an important predictor for composition, bivalve and polychaete abundances, although the direction of effects varied. When the same metrics were compared at larger scales (i.e., boating infrastructure present or lacking) we found that spatial variability among locations was detected, but no effect for moorings. With increased urbanization and industrialization of coastal areas there is a clear need to account for the scale of potential ecological effects in investigations of coastal infrastructure developments.
Introduction
Human-induced ecological change has increased over recent decades (Glasson et al., 2013; Lewis and Maslin, 2015; Waters et al., 2016), prompting governments to use legislation to manage user-environment interactions (e.g., Christodoulou and Stamatelatou, 2016; Feng and Liao, 2016; Gunningham and Holley, 2016; Horne et al., 2016; Pettipas et al., 2016; Harvey et al., 2017). In order to conform to these legislative requirements, and to maintain a social license to operate, both industry and government are applying formal impact and monitoring assessments to existing and proposed developments (Glasson et al., 2013). During the past few decades such assessments have become more rigorous, more reliant on formal statistical principles, and are now better able to detect change (Downes et al., 2002; Briggs and Hudson, 2013; Drayson et al., 2017). However, refinement of analytical frameworks must continue and new methodologies and technologies should build on existing techniques to understand ecological changes (Morgan, 2012; Briggs and Hudson, 2013).
Ecological impact assessments (EcIAs) are a component of environmental impact assessments (EIAs) and provide a formal test for ecological change, at a scale relevant to a proposed or existing development (Glasson et al., 2013). EcIAs aim to (1) evaluate whether the activity in question impacts surrounding ecosystems and (2) if present, estimate the magnitude and scale of the disturbance (Downes et al., 2002). All infrastructure is expected to have some effect on natural systems (McKinney, 2002; Benítez-López et al., 2010; Dafforn et al., 2015), so it is often the magnitude and scale of impact that is more pertinent to policy development. The most appropriate EcIA approaches must therefore be designed to test, not only for Impact/No Impact (a simple binary test), but also for the scale (in both space and time) and direction (positive or negative) of such effects. Inappropriately designed assessments may fail to provide policy-makers and decision makers with the most appropriate information they need to manage marine infrastructure development (Legg and Nagy, 2006).
Studies that investigate human-induced ecological change are often geographically restricted, and consist of a few replicate observations taken at relatively large spatial scales, where potentially impacted and reference locations may be 1000s of meters apart (Piersma et al., 2001; Lewis et al., 2002; Dauvin et al., 2006; Wildsmith et al., 2011; Minshall et al., 2014). Such designs may not provide a representative description of the impacts associated with the disturbance in question; particularly the scale of its effects. These approaches also fail to consider important sources of variation (Schmitt and Osenberg, 1996; Downes et al., 2002) especially those occurring at small spatial scales. Many communities are known to exhibit natural small-scale spatial variability (Dexter, 1984; Thrush, 1991; James and Fairweather, 1996; Herman et al., 1999; Fraschetti et al., 2005), but if sampling to infer impact is assessed at larger spatial scales (100s to 1000s of meters), then important information on fine scale species distributions may remain hidden. Similarly, small-scale anthropogenic impacts (restricted developments) on population and/or community level measures will be obscured. EcIAs in these environments may benefit from assessing impact at multiple spatial scales.
Coastal developments that require EcIAs include the local and regional installation of infrastructure such as groins and breakwaters. These are relatively well studied (Connell and Glasby, 1999; Connell, 2001; Martin et al., 2005; Bulleri and Chapman, 2010; Johnston et al., 2011; Rivero et al., 2013; Clark et al., 2015; Sim et al., 2015), but there a paucity of research exists on the ecological and environmental changes associated with estuarine recreational boating infrastructure, such as swing moorings. The small body of research that does exist has primarily focused on seagrass communities (Walker et al., 1989; Hastings et al., 1995; Demers et al., 2013). Studies have shown that moorings negatively impact seagrass beds through scouring, consequently increasing meadow susceptibility to erosion and reducing productivity (Walker et al., 1989; Hastings et al., 1995; Demers et al., 2013). As a result, seagrass-friendly moorings have been used in some areas to reduce the scour and ecological impacts associated with conventional “swing” moorings (Demers et al., 2013), yet their installation is still relatively rare. Moorings have also been linked to changes in grain sizes, organic matter and contamination (Hedge et al., 2017 in a parallel study to this one), however, few studies have assessed the effect of marine infrastructure (reviewed by Heery et al., 2017) or specifically boat moorings on soft-sedimentary communities (but see Herbert et al., 2009; Collins et al., 2010). This is surprising given the large number of dense mooring fields, situated on soft-sedimentary habitats (e.g., >26,000 moorings in New South Wales, Transport for New South Wales, 2014).
In this study we analyze the fine (m) and large (km) scale spatial patterns in soft-sediment assemblages associated with boat moorings. We contrast the sensitivity of sampling at different spatial scales to detect ecological change associated with recreational boating infrastructure. Thus, we simultaneously develop our understanding of boating infrastructure impacts on coastal environments; and evaluate sampling designs for future impact assessments in soft-sedimentary systems.
Materials and Methods
Study Area
Sediments were sampled from Sydney Harbour, located on the SE coast of New South Wales, Australia (Figure 1). Recent data from Transport for NSW estimates registered recreational vessel numbers in Sydney Harbor at more than 20,000 with annual growth predicted at 2.9% (Transport for New South Wales, 2015). Four locations were chosen, two with large mooring fields and two reference locations containing no boating infrastructure. Clontarf (33°81′S, 151°25′E) and North Harbor (33°80′S, 151°27′E) contained a marina and extensive mooring fields. Quarantine Bay (33°81′S, 151°29′E) and East Rose Bay (33°86′S, 151°27′E) were selected as reference locations with no boating infrastructure within 500 m. All locations are protected from ocean waves and subject to diurnal tidal flushing.
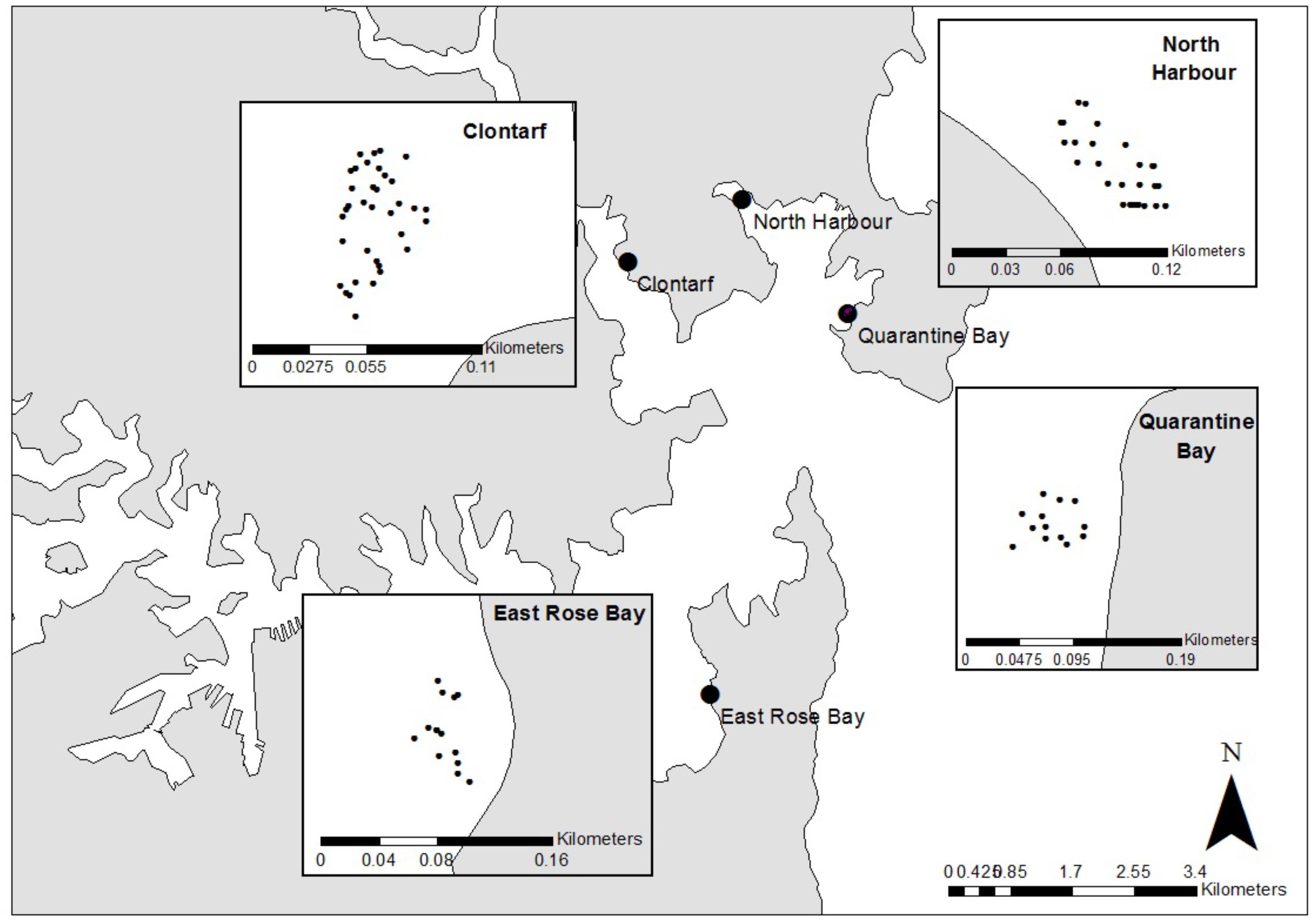
Figure 1. The location of sampling locations, and sites within locations, in Sydney Harbour, Australia. Moorings were present at Clontarf (n = 36 samples taken) and North Harbour (n = 28). The reference locations lacking moorings were East Rose Bay (n = 13) and Quarantine Bay (n = 13).
Sampling Design
A Generalized Random Tessellation Stratified (GRTS) sampling design (Stevens and Olsen, 2004) was used to select samples within all locations for consistency. Sampling was stratified by distance to moorings at boating infrastructure locations or unstratified at reference sites. A GRTS design allows for spatially balanced sampling, where sampling effort is distributed evenly across both environmental (distance from shore and moorings) and geographic space (Stevens and Olsen, 2004). This coverage is important when dealing with ecological communities that exhibit variation in spatial patterning (Thrush, 1991). Divers were positioned to collect samples on the seafloor using an Ultra Short Baseline (USBL) sub-sea positioning system (Sonardyne ScoutTM) and diver-to-vessel communication equipment. This enabled fine-scale sampling (<1 m intervals) in relation to distance from boating infrastructure.
Thirteen samples were collected from each of the mooring fields and reference locations, for a large-scale impact assessment. Additional samples were taken at the two mooring field locations (including the original 13 samples, n = 28 at North Harbor and n = 36 at Clontarf) for a finer-scale impact assessment within locations. All sampling was subtidal at between 3 and 8 m below MLWS. Samples were collected during February and March 2015. Each sample comprised 500 mL of sediment, collected from the top 5 cm of the seafloor using a 8 cm × 5 cm corer operated by SCUBA divers.
Analysis of Infauna and Sediment Grain Size
Sediment samples were wet sieved on site through steel sieves with mesh sizes of 2 mm (to remove debris) and 500 μm (to collect biota). To aid with invertebrate identification, samples were stained with Rose Bengal and preserved in 10% formaldehyde. Half the sample was sorted for infauna under a dissecting microscope. The sieving resulted in damage to the organisms in some samples. Further, while the staining aided in picking live material from the sample, it obscured some finer morphological features, preventing classification to species-level for some specimens. Therefore, organisms were identified to order and then classified into morphospecies. Polychaetes were further identified to family as they represent ∼70% of taxa within sedimentary ecosystems (Hutchings, 1998; Dafforn et al., 2013).
Subsamples (3–5 g freeze-dried sediment) were assessed using a Malvern laser particle size analyzer (Mastersizer 2000) to quantify grain size distributions (Hedge et al., 2017). The 10th percentile (D10) was used as a representative metric for grain size in further statistical analysis (Blott and Pye, 2001).
Statistical Analyses of Fine Scale Patterns
To test for fine-scale effects of moorings, we constructed generalized linear models (GLMs) to assess patterns in community structure, abundance and diversity (Baeten et al., 2014) within the two locations with mooring fields (Clontarf and North Harbor). Multivariate species composition was modeled as a function of distance to moorings, distance from shore, location, and grain size. Distance from shore was included as a proxy for depth (which was highly correlated) and for potential land-based influences, and grain size was included because of a previously described relationship between grain size and distance from moorings at these locations (Hedge et al., 2017).
Only abundant taxa (containing >10 observed individuals) were included in this analysis, effectively reducing the final taxa count from 144 to 44. This was done because taxa with less than ten individuals found throughout a study are rarely important to the multivariate analysis and may lead to zero-inflation issues during model specification (Zuur et al., 2009; Wang et al., 2012). Due to the presence of non-constant error variances in residuals, GLMs were constructed using a negative binomial distribution and log link in the mvabund package within the R Statistical Environment (Wang et al., 2012; R Core Team, 2014). Full details of this model-based multivariate approach can be found in Wang et al. (2012). Briefly, abundances of infauna taxon j at site i (Yij) were modeled as a negative binomial process where;
A multivariate test statistic (Wald value) was used to test for the effects of each factor with inference from bootstrap resampling with 999 samples.
The univariate response variables of total abundance per sample, abundance of each of the dominant higher taxa (polychaetes, crustaceans, and mollusks) and diversity, were also modeled with GLMs in mvabund with the same independent variables as above, and inference from bootstrap resampling. The abundances were modeled using a negative binomial distribution with a log link. Analyses of diversity (Shannons Diversity H’) used a gamma distribution and log link such that:
For both multivariate and univariate models, model validation was done by examining both residual plots and mean-variance plots in order to confirm if the choice of the distribution family for these analyses was appropriate. A correlation plot between grain size and distance from moorings revealed no correlation between the two variables confirming the validity of our model (P = 0.86).
Statistical Analyses of Large-Scale Patterns
To test for larger scale impacts of boating infrastructure, we contrasted species composition, abundance and diversity among all four locations with GLMs. Multivariate species composition was modeled in mvabund with location as the predictor variable and a negative binomial distribution. The total abundance per sample and the abundance of each of the dominant higher taxa (polychaetes, crustaceans and mollusks), were also modeled with GLMs, using a negative binomial distribution. Diversity was modeled with a gamma distribution. Statistical inference and model validation were done as described above, and for all analyses, 13 replicate samples were randomly selected from the mooring field locations to ensure a balanced data set. With two locations per impact category (mooring fields vs. reference), we considered location a fixed factor and tested for differences among these two categories by pair-wise tests among all locations (using the Holm method for adjusting for the inflated error rates associated with multiple tests) (Holm, 1979). Differences in the species composition among locations were visualized with multi-dimensional scaling using the Bray-Curtis similarity coefficient and square root transformed data.
Results and Discussion
Soft-sediment infaunal communities are important components of marine ecosystems, and critical to major ecosystem processes (Snelgrove, 1997; Lohrer et al., 2004; Douglas et al., 2017). Given their ecological significance, it is important that we understand how building coastal infrastructure, such as boat moorings, might affect these sediment communities and whether current impacts assessment methodologies have sufficient sensitivity to detect such effects. We found that comparisons at large scales failed to detect ecological change related to boat moorings, however, fine -scale effects of boating infrastructure on infauna were detected when considering environmental space (distance to moorings). Furthermore, while distance to moorings was found to be a predictor of ecological change, the response of each taxon varied considerably. No ‘one’ taxa were the main driver of this change, but rather, the effects of moorings manifested in a combination of small changes in several taxa of the communities at both locations with boat moorings present. This highlights the importance of sampling at multiple scales in impact assessments and considering taxon-specific responses.
Fine-Scale Effects of Boat Moorings
A significant multiplicative effect of distance to moorings, distance from shore, location and grain size was found on infaunal community composition (Table 1). While the communities nearer to the moorings were different than those found further away (Table 1), the direction and magnitude of this response for individual taxa varied considerably (Figure 2 and Supplementary Table S1). We observed similar fine scale impact of moorings on fish communities in a parallel study (Lanham et al., 2018). No single taxon could be identified as most strongly associated with these compositional changes. Nematodes were the most abundant in close proximity to moorings (Figure 2) and decreased with distance from moorings independent of spatial differences (Supplementary Table S1). They are often used as indicator species because of their differential sensitivity to disturbance and here their response may be related to greater physical disturbance close to the swing mooring change (Bongers and Ferris, 1999; Boyd et al., 2000). Among the species with the strongest relationship with distance from moorings (Figure 2), there were taxa that were more abundant at sites further away from moorings, but only at Clontarf (Orbiniid polychaetes, ostracod sp. 1), while other taxa were more abundant at sites closer to moorings but, again, only at Clontarf (ostracod sp. 6, bivalve sp. 1).
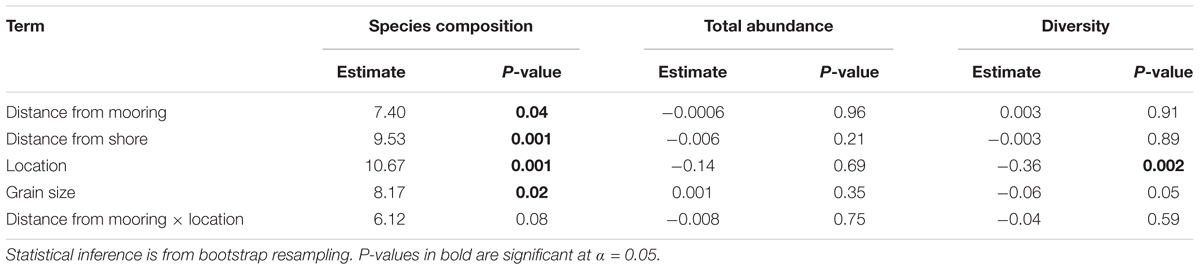
Table 1. Results from generalized linear models used to test for effects of distance to moorings, distance from shore, location and grain size on the multivariate composition of infaunal species, and the univariate total abundance per sample and diversity (Shannons Diversity H’).
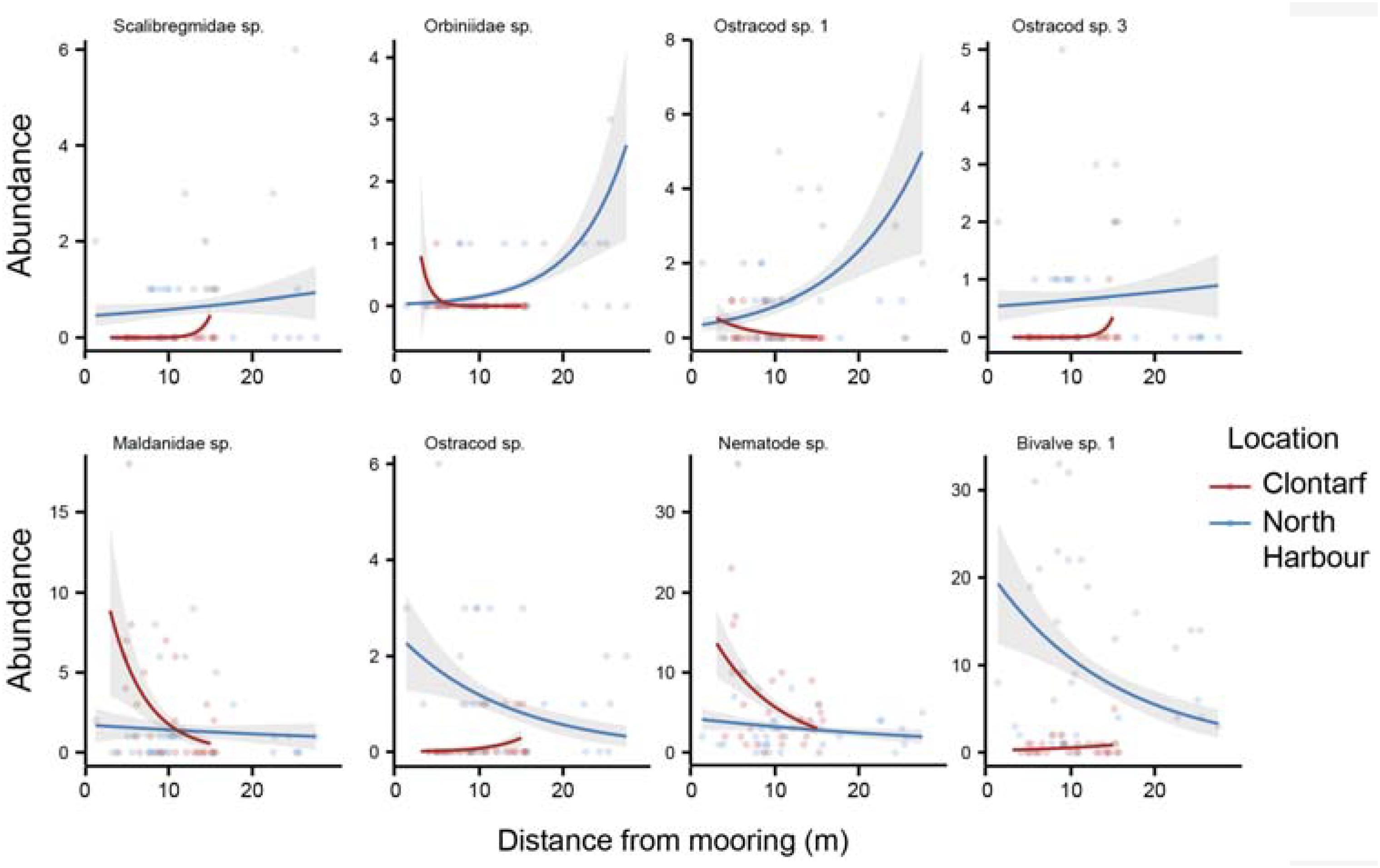
Figure 2. The relationship between infaunal abundance (count per sample) and distance from moorings (m) at the two locations with boating infrastructure (Clontarf, North Harbour). The taxa plotted are the eight morphospecies with the lowest p-values for the distance from moorings predictor variable in the generalized linear models (GLM). Fitted lines are derived from the negative binomial GLMs and shading represents 95% CI.
One potential physical change we expected from the swing moorings was a change in grain size due to scouring (Demers et al., 2013; Hedge et al., 2017). Overall the grain sizes were similar and sandy at all sites (Supplementary Figure S1). Clontarf sediments had ∼4% silt, while North Harbor sediments were composed of ∼6% silt. Both Quarantine Bay and East Rose Bay had around 3% silt. While we found significant relationships between infauna and grain size changes, these were independent of the distance from the mooring. The relationships between abundance and grain size differed among taxa, and between locations within taxa (Supplementary Figure S1 and Table S1). At Clontarf, some taxa, such as bivalve sp. 1 and ostracod sp. 1, decreased in abundance with increasing sediment grain size (Supplementary Figure S1), while Pectinariidae and Opheliidae (Polychaetes) increased in abundance with increasing sediment size (Supplementary Table S1). Infaunal communities are strongly influenced by sediment characteristics such as grain size (Simpson et al., 2005) and in particular, several polychaete families (Syllidae, Opheliidae, and Pectinariidae), and polychaetes as a group, increased as grain size increased, while mollusks, crustaceans and other taxa decreased overall. These changes were detected at a fine-scale, crucially allowing the effects of moorings on infauna to be distinguished from natural spatial variation in grain size.
When pooling species, the total abundance of infauna per sample did not significantly vary with distance to moorings, distance from shore, location or grain size (10th percentile, μm) (Table 1). Diversity per sample (Shannons Diversity H’) varied among locations, but there was no effect of distance to moorings, distance from shore or grain size (Table 1). When the species were aggregated to higher taxonomic levels, the abundance of mollusks declined with increasing distance from moorings and increasing grain size (Supplementary Table S2). The abundance of crustaceans varied with location, but not with the distance from moorings or with grain size (Supplementary Table S2) and the abundance of polychaetes was unrelated to the distance from moorings but increased with increasing grain size (Supplementary Table S2).
Large Scale Effects of Boat Moorings
In this study, the large-scale analysis incorporating (1) 13 replicates at two boating infrastructures and two reference locations and (2) randomized sample sites within the locations (i.e., no consideration given to environmental space) found no effect of boat moorings on infaunal communities. Specifically, the composition of infauna varied significantly among locations (Figure 3, multivariate GLM, deviance 907.1, P = 0.001), but there was no evidence that the two locations with boating infrastructure (Clontarf and North Harbor) differed consistently from those lacking boating infrastructure (Quarantine Bay and Rose Bay). In pairwise tests, the composition of infauna at each of the locations differed significantly from all other locations (multivariate GLMs, P = 0.006).
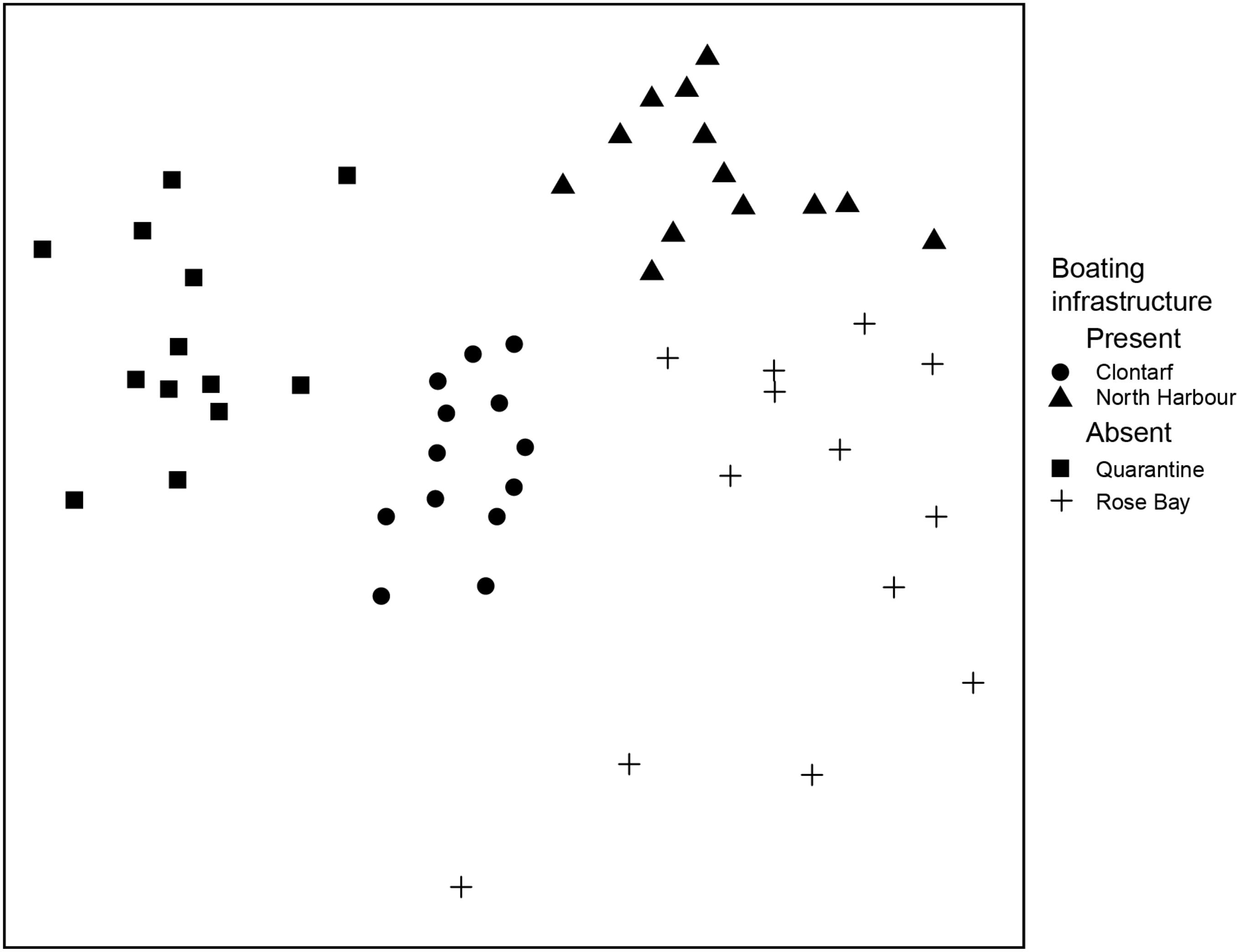
Figure 3. MDS ordination visualizing the differences in composition of the infaunal community for locations with boating infrastructure (Clontarf, North Harbour) and lacking boating infrastructure (Quarantine Bay, Rose Bay). The ordination used the Bray-Curtis index of similarity and square root transformed data (stress = 0.19).
The total abundance of infauna per sample varied significantly among locations (GLM, deviance = 37.32, P = 0.001), with no evidence for reduced abundance at the locations with boating infrastructure (Figure 4A). The two locations with boating infrastructure supported similar total abundances, with abundances both significantly lower (Rose Bay) and higher (Quarantine Bay) at the reference locations (Figure 4). Diversity (Shannons Diversity H’) also varied among locations (F3,48, P < 0.001), but not in the presence or absence of boating infrastructure (Figure 4B). Diversity was highest and similar at two sites that either had (Clontarf) or lacked (Quarantine Bay) boating infrastructure. North Harbor and Rose Bay, one of which had moorings, supported similar diversity, both significantly lower than the other two locations (Figure 4).
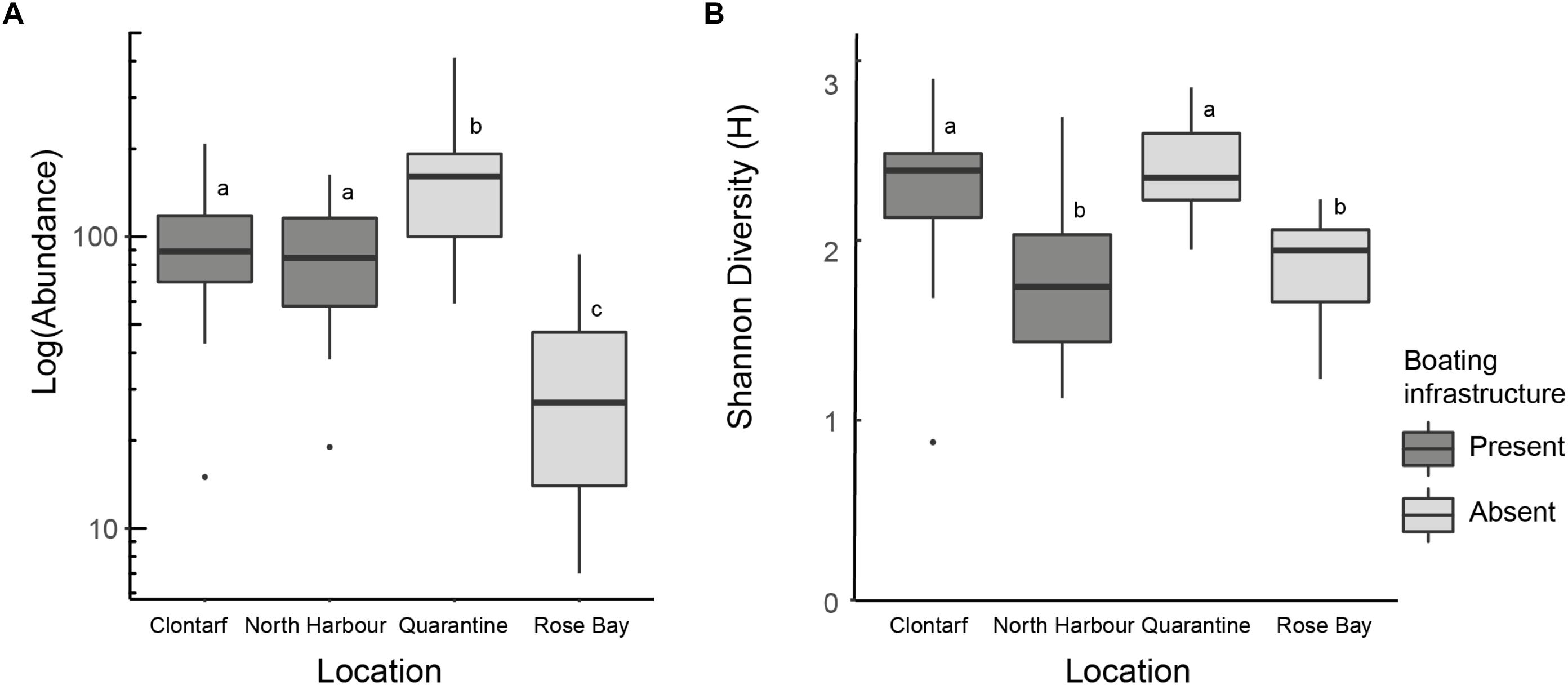
Figure 4. The (A) abundance and (B) Shannons Diversity (H′) for each of the locations with boating infrastructure (Clontarf, North Harbour) and lacking boating infrastructure (Quarantine Bay, East Rose Bay). Locations sharing a letter do not differ in post hoc tests following (A) a negative binomial GLM and (B) a linear model contrasting all locations. Boxes indicate the upper and lower quartiles around the median, whiskers represent scores outside the middle 50%.
The total abundance of each of the major taxa varied among locations, but the two locations with boating infrastructure did not differ consistently from the two locations lacking boating infrastructure (Supplementary Figure S2; GLMs, crustaceans, deviance 55.18, P < 0.001; mollusks, deviance 55.18, P < 0.001; polychaetes, deviance 55.32, P < 0.001). For crustaceans, one of the reference locations (Quarantine Bay) supported the highest abundance, while the other reference location (Rose Bay) did not differ significantly from one of the locations with moorings (North Harbor; Supplementary Figure S2A). For mollusks, abundance was also highest at Quarantine Bay, with the other reference location supporting lower abundance than both locations with moorings (Supplementary Figure S2B). For polychaetes, one of the reference locations (Rose Bay) supported lower abundance than all other locations (Supplementary Figure S2C).
Implications for the Design of Impact Assessments
Fine scale sampling designs have been successfully incorporated into EcIA frameworks in the past. Walker et al. (2008), for example, found effects of an artificial groin on the distribution of macrofauna communities. Here they contrasted macrofauna across 11 transects with proximities of 1, 3, 5, 10, 15, 25, 50, 100, 150, 200, and 250 m from each side of the groin. Similarly, Rivero et al. (2013) and Sim et al. (2015) investigated changes in epifaunal and infaunal communities, respectively, with distance from boating structures finding patterns in larval recruitment, reduced flow, and increased contamination closest to boating structures. Yet, despite the obvious benefits of understanding the scale of impact, rather than just testing if an impact occurs, studies such as these are relatively rare.
If the effects of boating infrastructure were only assessed at larger spatial scales (locations in this study) then changes in sediment communities could be overlooked. Clearly this could be of concern for policy makers and government bodies that use the advice of analysts to guide the development of infrastructure (Morgan, 2012; Clarke and Menadue, 2016). The failure to detect an impact appears, in large part, due to the fine-scale nature of the effects of moorings, which are not well assessed by the large-scale sampling approach. For the majority of marine developments, the potential scale of ecological impacts will be difficult to predict a priori with any great accuracy (Dafforn et al., 2015; Bishop et al., 2017; Heery et al., 2017). In such cases, it becomes prudent to add value to impact assessment frameworks by incorporating sampling programs that use multiple scales within potentially impacted locations. Advances in the accuracy and versatility of satellite positioning systems have greatly increased the feasibility of such an approach (Dafforn et al., 2016).
Our large-scale impact assessment approach had fewer replicates per location but more samples overall for processing (13 replicate samples × n = 4 locations = 52) relative the within location fine-scale approaches (n = 28 and n = 36 samples, respectively, per impact location). Interestingly, 13 replicates remain high for an EcIA in a marine ecosystem [e.g., see systematic review of contamination impacts by Johnston and Roberts (2009)]. Very few analyses have employed >10 replicate samples per impact and reference locations (e.g., Anderlini and Wear, 1992; Danovaro et al., 1995; Ward and Hutchings, 1996; Stark, 1998; Lasiak et al., 2006; Skilleter et al., 2006; Fukunaga et al., 2011). Lewis et al. (2002), for example, found effects of pipeline construction on benthic invertebrate distributions in Clonakilty Bay, Ireland. The study design consisted of three sediment cores taken at an impact location and three reference locations per sampling interval. Further to this, samples were randomly allocated within sites and scale was not considered. Sampling at finer spatial scales has the potential to reduce sampling efforts without reducing the sensitivity in detecting ecological changes.
Taxonomic Resolution
Where there are limitations to the taxonomic resolution that can be attained, results show that morphospecies can be used as surrogates for taxonomic species in ecological studies (Brind’Amour et al., 2014). Further, many impact assessment studies have recommended community analysis at the level of class or family to provide a taxonomic sufficiency to detect differences among locations (Warwick, 1988; Olsgard et al., 1997; Roach et al., 2001). With limited research undertaken on the impact of boat moorings on the distribution of infaunal communities, it is unknown as to whether results from this study would differ, had a species-level of taxonomic identification been possible. Certainly, at the scale of taxonomic resolution commonly applied in impact assessments (e.g., Sydney Water, 2014), we only detected ecological changes at finer spatial scales of sampling for some taxa. Future studies in this system could investigate a species-level approach to taxonomic identification or other taxonomic and functional groupings (Bennett et al., 2014; Jansen et al., 2018). This will allow for the comparison of results using differing methods to taxonomic identification and may highlight any loss of ecological information incurred by using one approach over another.
Conclusion
As the world’s population moves closer to the sea, those responsible for detecting impacts, approving development, and monitoring societal-environmental interactions require every tool at their disposal and must quickly adopt improved methodologies to assess potential change (Kennish, 2002; Halpern et al., 2007). There are thousands of moorings in Sydney harbor, the study area, – and in coastal ecosystems around the globe, with the potential to change soft sedimentary communities. While the impacts identified here may be fine scale – they will act to fragment infaunal assemblages, which might disrupt ecological processes at larger scales.
Data Availability
The datasets generated for this study are available on request to the corresponding author.
Author Contributions
AM conducted the fieldwork, sample processing, and data analysis. EJ, KD, LH, and AP conceived the experimental design. KD and LH assisted with fieldwork, sample processing, and statistical analysis. All authors contributed to writing and editing the manuscript.
Funding
This project was partly funded by the Australian Research Centre Linkage Project Grant LP140100855 awarded to EJ and AP. Further funding was supplied by Transport for NSW.
Conflict of Interest Statement
The authors declare that the research was conducted in the absence of any commercial or financial relationships that could be construed as a potential conflict of interest.
Acknowledgments
We would like to thank members of the Applied Marine and Estuarine Ecology laboratory and volunteers for their assistance.
Supplementary Material
The Supplementary Material for this article can be found online at: https://www.frontiersin.org/articles/10.3389/fmars.2019.00101/full#supplementary-material
References
Anderlini, V. C., and Wear, R. G. (1992). The effect of sewage and natural seasonal disturbances on benthic macrofaunal communities in Fitzroy Bay, Wellington, New Zealand. Mar. Pollut. Bull. 24, 21–26. doi: 10.1016/0025-326X(92)90312-T
Baeten, L., Warton, D. I., Van Calster, H., De Frenne, P., Verstraeten, G., Bonte, D., et al. (2014). A model-based approach to studying changes in compositional heterogeneity. Methods Ecol. Evol. 5, 156–164. doi: 10.1021/acs.analchem.7b00518
Benítez-López, A., Alkemade, R., and Verweij, P. A. (2010). The impacts of roads and other infrastructure on mammal and bird populations: a meta-analysis. Biol. Conserv. 143, 1307–1316. doi: 10.1016/j.biocon.2010.02.009
Bennett, J. R., Sisson, D. R., Smol, J. P., Cumming, B. F., Possingham, H. P., and Buckley, Y. M. (2014). Optimizing taxonomic resolution and sampling effort to design cost-effective ecological models for environmental assessment. J. Appl. Ecol. 51, 1722–1732. doi: 10.1111/1365-2664.12312
Bishop, M. J., Mayer-Pinto, M., Airoldi, L., Firth, L. B., Morris, R. L., Loke, L. H. L., et al. (2017). Effects of ocean sprawl on ecological connectivity: impacts and solutions. J. Exp. Mar. Biol. Ecol. 492, 7–30. doi: 10.1016/j.jembe.2017.01.021
Blott, S. J., and Pye, K. (2001). GRADISTAT: a grain size distribution and statistics package for the analysis of unconsolidated sediments. Earth Surf. Proc. Land. 26, 1237–1248. doi: 10.1002/esp.261
Bongers, T., and Ferris, H. (1999). Nematode community structure as a bioindicator in environmental monitoring. Trends Ecol. Evol. 14, 224–228. doi: 10.1016/S0169-5347(98)01583-3
Boyd, S. E., Rees, H. L., and Richardson, C. A. (2000). Nematodes as sensitive indicators of change at dredged material disposal sites. Estuar. Coast. Shelf Sci. 51, 805–819. doi: 10.1006/ecss.2000.0722
Briggs, S., and Hudson, M. D. (2013). Determination of significance in ecological impact assessment: past change, current practice and future improvements. Environ. Impact Assess. Rev. 38, 16–25. doi: 10.1016/j.eiar.2012.04.003
Brind’Amour, A., Laffargue, P., Morin, J., Vaz, S., Foveau, A., and Le Bris, H. (2014). Morphospecies and taxonomic sufficiency of benthic megafauna in scientific bottom trawl surveys. Cont. Shelf Res. 72, 1–9. doi: 10.1016/j.csr.2013.10.015
Bulleri, F., and Chapman, M. G. (2010). The introduction of coastal infrastructures as a driver of change in marine environments. J. Appl. Ecol. 47, 26–35. doi: 10.1111/j.1365-2664.2009.01751.x
Christodoulou, A., and Stamatelatou, K. (2016). Overview of legislation on sewage sludge management in developed countries worldwide. Water Sci. Technol. 73, 453–462. doi: 10.2166/wst.2015.521
Clark, G. F., Kelaher, B. P., Dafforn, K. A., Coleman, M. A., Knott, N. A., Marzinelli, E. M., et al. (2015). What does impacted look like? High diversity and abundance of epibiota in modified estuaries. Environ. Pollut. 196, 12–20. doi: 10.1016/j.envpol.2014.09.017
Clarke, B., and Menadue, T. (2016). Fit for purpose? Establishing the robustness of EIA screening systems for land-use planning using a case study from South Australia. J. Environ. Plann. Man. 59, 538–556. doi: 10.1080/09640568.2015.1024307
Collins, K. J., Suonpää, A. M., and Mallinson, J. J. (2010). The impacts of anchoring and mooring in seagrass, Studland Bay, Dorset, UK. Underwat. Technol. 29, 117–123. doi: 10.3723/ut.29.117
Connell, S. D. (2001). Urban structures as marine habitats: an experimental comparison of the composition and abundance of subtidal epibiota among pilings, pontoons and rocky reefs. Mar. Environ. Res. 52, 115–125. doi: 10.1016/S0141-1136(00)00266-X
Connell, S. D., and Glasby, T. M. (1999). Do urban structures influence local abundance and diversity of subtidal epibiota? A case study from Sydney Harbour, Australia. Mar. Environ. Res. 47, 373–387. doi: 10.1016/S0141-1136(98)00126-3
Dafforn, K. A., Glasby, T. M., Airoldi, L., Rivero, N. K., Mayer-Pinto, M., and Johnston, E. L. (2015). Marine urbanisation: an ecological framework for designing multifunctional artificial structures. Front. Ecol. Environ. 13:82–90. doi: 10.1890/140050
Dafforn, K. A., Johnston, E. L., Ferguson, A., Humphrey, C. L., Monk, W., Nichols, S. J., et al. (2016). Big data opportunities and challenges for assessing multiple stressors across scales in aquatic ecosystems. Mar. Freshw. Res. 67, 393–413. doi: 10.1071/MF15108
Dafforn, K. A., Kelaher, B. P., Simpson, S. L., Coleman, M. A., Hutchings, P. A., Clark, G. F., et al. (2013). Polychaete richness and abundance enhanced in anthropogenically modified estuaries despite high concentrations of toxic contaminants. PLoS One 8:e77018. doi: 10.1371/journal.pone.0077018
Danovaro, R., Fabiano, M., and Vincx, M. (1995). Meiofauna response to the Agip Abruzzo oil spill in subtidal sediments of the Ligurian Sea. Mar. Pollut. Bull. 30, 133–145. doi: 10.1016/0025-326X(94)00114-O
Dauvin, J. C., Desroy, N., Janson, A. L., Vallet, C., and Duhamel, S. (2006). Recent changes in estuarine benthic and suprabenthic communities resulting from the development of harbour infrastructure. Mar. Pollut. Bull. 53, 80–90. doi: 10.1016/j.marpolbul.2005.09.020
Demers, M.-C. A., Davis, A. R., and Knott, N. A. (2013). A comparison of the impact of ‘seagrass-friendly’ boat mooring systems on Posidonia australis. Mar. Environ. Res. 83, 54–62. doi: 10.1016/j.marenvres.2012.10.010
Dexter, D. (1984). Temporal and spatial variability in the community structure of the fauna of four sandy beaches in south-eastern New South Wales. Mar. Freshw. Res. 35, 663–672. doi: 10.1071/MF9840663
Douglas, E. J., Pilditch, C. A., Kraan, C., Schipper, L. A., Lohrer, A. M., and Thrush, S. F. (2017). Macrofaunal functional diversity provides resilience to nutrient enrichment in coastal sediments. Ecosystems 20, 1324–1336. doi: 10.1007/s10021-017-0113-4
Downes, B. J., Barmuta, L. A., Fairweather, P. G., Faith, D. P., Keough, M. J., Lake, P., et al. (2002). Monitoring Ecological Impacts: Concepts and Practice in Flowing Waters. Cambridge: Cambridge University Press. doi: 10.1017/CBO9780511542015
Drayson, K., Wood, G., and Thompson, S. (2017). An evaluation of ecological impact assessment procedural effectiveness over time. Environ. Sci. Policy 70, 54–66. doi: 10.1016/j.envsci.2017.01.003
Feng, L., and Liao, W. (2016). Legislation, plans, and policies for prevention and control of air pollution in China: achievements, challenges, and improvements. J. Clean. Prod. 112, 1549–1558. doi: 10.1016/j.jclepro.2015.08.013
Fraschetti, S., Terlizzi, A., and Benedetti-Cecchi, L. (2005). Patterns of distribution of marine assemblages from rocky shores evidence of relevant scales of variation. Mar. Ecol. Prog. Ser. 296, 13–29. doi: 10.3354/meps296013
Fukunaga, A., Anderson, M. J., and Webster-Brown, J. G. (2011). Assessing the nature of the combined effects of copper and zinc on estuarine infaunal communities. Environ. Pollut. 159, 116–124. doi: 10.1016/j.envpol.2010.09.012
Glasson, J., Therivel, R., and Chadwick, A. (2013). Introduction to Environmental Impact Assessment. London: Routledge.
Gunningham, N., and Holley, C. (2016). Next-generation environmental regulation: law, regulation, and governance. Annu. Rev. Law Soc. Sci. 12, 273–293. doi: 10.1146/annurev-lawsocsci-110615-084651
Halpern, B. S., Selkoe, K. A., Micheli, F., and Kappel, C. V. (2007). Evaluating and ranking the vulnerability of global marine ecosystems to anthropogenic threats. Conserv. Biol. 21, 1301–1315. doi: 10.1111/j.1523-1739.2007.00752.x
Harvey, N., Dew, R. E. C., and Hender, S. (2017). Rapid land use change by coastal wind farm development: Australian policies, politics and planning. Land Use Policy 61, 368–378. doi: 10.1016/j.landusepol.2016.11.031
Hastings, K., Hesp, P., and Kendrick, G. A. (1995). Seagrass loss associated with boat moorings at Rottnest Island, Western Australia. Ocean Coast. Manag. 26, 225–246. doi: 10.1016/0964-5691(95)00012-Q
Hedge, L. H., Dafforn, K. A., Simpson, S. L., and Johnston, E. L. (2017). Uncovering hidden heterogeneity: geo-statistical models illuminate the fine scale effects of boating infrastructure on sediment characteristics and contaminants. Mar. Pollut. Bull. 119, 143–150. doi: 10.1016/j.marpolbul.2017.04.044
Heery, E. C., Bishop, M. J., Critchley, L. P., Bugnot, A. B., Airoldi, L., Mayer-Pinto, M., et al. (2017). Identifying the consequences of ocean sprawl for sedimentary habitats. J. Exp. Mar. Biol. Ecol. 492, 31–48. doi: 10.1016/j.jembe.2017.01.020
Herbert, R. J. H., Crowe, T. P., Bray, S., and Sheader, M. (2009). Disturbance of intertidal soft sediment assemblages caused by swinging boat moorings. Hydrobiologia 625, 105–116. doi: 10.1007/s10750-008-9700-x
Herman, P., Middelburg, J., Van de Koppel, J., and Heip, C. (1999). Ecology of estuarine macrobenthos. Adv. Ecol. Res. 29, 195–240. doi: 10.1016/S0065-2504(08)60194-4
Horne, R., Strengers, Y., and Strempel, A. (2016). Policing and polluting: the role of practices in contemporary urban environmental pollution governance. Environ. Sci. Policy 66, 112–118. doi: 10.1016/j.envsci.2016.08.006
Hutchings, P. (1998). Biodiversity and functioning of polychaetes in benthic sediments. Biodivers. Conserv. 7, 1133–1145. doi: 10.1023/A:1008871430178
James, R. J., and Fairweather, P. G. (1996). Spatial variation of intertidal macrofauna on a sandy ocean beach in Australia. Estuar. Coast. Shelf Sci. 43, 81–107. doi: 10.1006/ecss.1996.0058
Jansen, J., Hill, N. A., Dunstan, P. K., Eléaume, M. P., and Johnson, C. R. (2018). Taxonomic resolution, functional traits, and the influence of species groupings on mapping Antarctic seafloor biodiversity. Front. Ecol. Evol. 6:81. doi: 10.3389/fevo.2018.00081
Johnston, E. L., Marzinelli, E. M., Wood, C. A., Speranza, D., and Bishop, J. D. D. (2011). Bearing the burden of boat harbours: heavy contaminant and fouling loads in a native habitat-forming alga. Mar. Pollut. Bull. 62, 2137–2144. doi: 10.1016/j.marpolbul.2011.07.009
Johnston, E. L., and Roberts, D. A. (2009). Contaminants reduce the richness and evenness of marine communities: a review and meta-analysis. Environ. Pollut. 157, 1745–1752. doi: 10.1016/j.envpol.2009.02.017
Kennish, M. J. (2002). Environmental threats and environmental future of estuaries. Environ. Conserv. 29, 78–107. doi: 10.1017/S0376892902000061
Lanham, B. S., Vergés, A., Hedge, L. H., Johnston, E. L., and Poore, A. G. (2018). Altered fish community and feeding behaviour in close proximity to boat moorings in an urban estuary. Mar. Poll. Bull. 129, 43–51. doi: 10.1016/j.marpolbul.2018.02.010
Lasiak, T., Underwood, A., and Hoskin, M. (2006). An experimental assessment of the potential impacts of longline mussel farming on the infauna in an open coastal embayment. Aquat. Conserv. 16, 289–300. doi: 10.1002/aqc.710
Legg, C. J., and Nagy, L. (2006). Why most conservation monitoring is, but need not be, a waste of time. J. Environ. Manage. 78, 194–199. doi: 10.1016/j.jenvman.2005.04.016
Lewis, L. J., Davenport, J., and Kelly, T. C. (2002). A study of the impact of a pipeline construction on estuarine benthic invertebrate communities. Estuar. Coast. Shelf Sci. 55, 213–221. doi: 10.1006/ecss.2001.0898
Lewis, S. L., and Maslin, M. A. (2015). Defining the anthropocene. Nature 519, 171–180. doi: 10.1038/nature14258
Lohrer, A. M., Thrush, S. F., and Gibbs, M. M. (2004). Bioturbators enhance ecosystem function through complex biogeochemical interactions. Nature 431, 1092–1095. doi: 10.1038/nature03042
Martin, D., Bertasi, F., Colangelo, M. A., de Vries, M., Frost, M., Hawkins, S. J., et al. (2005). Ecological impact of coastal defence structures on sediment and mobile fauna: evaluating and forecasting consequences of unavoidable modifications of native habitats. Coast. Eng. 52, 1027–1051. doi: 10.1016/j.coastaleng.2005.09.006
McKinney, M. L. (2002). Urbanization, biodiversity, and conservation. Bioscience 52, 883–890. doi: 10.1641/0006-3568(2002)052[0883:UBAC]2.0.CO;2
Minshall, G. W., Shafii, B., Price, W. J., Holderman, C., Anders, P. J., Lester, G., et al. (2014). Effects of nutrient replacement on benthic macroinvertebrates in an ultraoligotrophic reach of the Kootenai River, 2003–2010. Freshw. Sci. 33, 1009–1023. doi: 10.1086/677900
Morgan, R. K. (2012). Environmental impact assessment: the state of the art. Impact Assess. Proj. A. 30, 5–14. doi: 10.1080/14615517.2012.661557
Olsgard, F., Somerfield, P. J., and Carr, M. R. (1997). Relationships between taxonomic resolution and data transformations in analyses of a macrobenthic community along an established pollution gradient. Mar. Ecol. Prog. Ser. 149, 173–181. doi: 10.3354/meps149173
Pettipas, S., Bernier, M., and Walker, T. R. (2016). A Canadian policy framework to mitigate plastic marine pollution. Mar. Policy 68, 117–122. doi: 10.1016/j.marpol.2016.02.025
Piersma, T., Koolhaas, A., Dekinga, A., Beukema, J. J., Dekker, R., and Essink, K. (2001). Long-term indirect effects of mechanical cockle-dredging on intertidal bivalve stocks in the Wadden Sea. J. Appl. Ecol. 38, 976–990. doi: 10.1046/j.1365-2664.2001.00652.x
R Core Team (2014). R: A Language and Environment for Statistical Computing. Vienna: R Foundation for Statistical Computing.
Rivero, N. K., Dafforn, K. A., Coleman, M. A., and Johnston, E. L. (2013). Environmental and ecological changes associated with a marina. Biofouling 29, 803–815. doi: 10.1080/08927014.2013.805751
Roach, A. C., Jones, A. R., and Murray, A. (2001). Using benthic recruitment to assess the significance of contaminated sediments: the influence of taxonomic resolution. Environ. Pollut. 112, 131–143. doi: 10.1016/S0269-7491(00)00124-X
Schmitt, R. J., and Osenberg, C. W. (1996). Detecting Ecological Impacts: Concepts and Applications in Coastal Habitats. Cambridge, MA: Academic Press.
Sim, V. X. Y., Dafforn, K. A., Simpson, S. L., Kelaher, B. P., and Johnston, E. L. (2015). Sediment contaminants and infauna associated with recreational boating structures in a multi-use marine park. PLoS One 10:e0130537. doi: 10.1371/journal.pone.0130537
Simpson, S. L., Batley, G. E., Chariton, A. A., Stauber, J. L., King, C. K., Chapman, J. C., et al. (2005). Handbook for Sediment Quality Assessment. Sydney: CSIRO.
Skilleter, G. A., Pryor, A., Miller, S., and Cameron, B. (2006). Detecting the effects of physical disturbance on benthic assemblages in a subtropical estuary: a beyond BACI approach. J. Exp. Mar. Biol. Ecol. 338, 271–287. doi: 10.1016/j.jembe.2006.06.016
Snelgrove, P. V. R. (1997). The importance of marine sediment biodiversity in ecosystem processes. Ambio 26, 578–583.
Stark, J. S. (1998). Heavy metal pollution and macrobenthic assemblages in soft sediments in two Sydney estuaries, Australia. Mar. Freshw. Res. 49, 533–540. doi: 10.1071/MF97188
Stevens, D. L., and Olsen, A. R. (2004). Spatially balanced sampling of natural resources. J. Am. Stat. Assoc. 99, 262–278. doi: 10.1198/016214504000000250
Sydney Water (2014). Sewage Treatment System Impact Monitoring Program. Available at: https://www.sydneywater.com.au/web/groups/publicwebcontent/documents/document/zgrf/mdc4/~edisp/dd_078230.pdf
Thrush, S. F. (1991). Spatial patterns in soft-bottom communities. Trends Ecol. Evol. 6, 75–79. doi: 10.1016/0169-5347(91)90178-Z
Transport for New South Wales (2014). Moorings Review. Prepared for Transport for New South Wales by Maritime Management Centre, Sydney.
Transport for New South Wales. (2015). Regional Boating Plan, Sydney Harbour Region. Prepared for Transport for New South Wales by Maritime Management Centre, Sydney.
Walker, D. I., Lukatelich, R. J., Bastyan, G., and McComb, A. J. (1989). Effect of boat moorings on seagrass beds near Perth, Western Australia. Aquat. Bot. 36, 69–77. doi: 10.1016/0304-3770(89)90092-2
Walker, S. J., Schlacher, T. A., and Thompson, L. M. C. (2008). Habitat modification in a dynamic environment: the influence of a small artificial groyne on macrofaunal assemblages of a sandy beach. Estuar. Coast. Shelf Sci. 79, 24–34. doi: 10.1016/j.ecss.2008.03.011
Wang, Y., Naumann, U., Wright, S. T., and Warton, D. I. (2012). mvabund–an R package for model-based analysis of multivariate abundance data. Methods Ecol. Evol. 3, 471–474. doi: 10.1111/j.2041-210X.2012.00190.x
Ward, T. J., and Hutchings, P. A. (1996). Effects of trace metals on infaunal species composition in polluted intertidal and subtidal marine sediments near a lead smelter, Spencer Gulf, South Australia. Mar. Ecol. Prog. Ser. 135, 123–135. doi: 10.3354/meps135123
Warwick, R. M. (1988). The level of taxonomic resolution required to detect pollution effects on marne benthic communities. Mar. Poll. Bull. 19, 259–268. doi: 10.1016/0025-326X(88)90596-6
Waters, C. N., Zalasiewicz, J., Summerhayes, C., Barnosky, A. D., Poirier, C., Gałuszka, A., et al. (2016). The Anthropocene is functionally and stratigraphically distinct from the Holocene. Science 351:aad2622. doi: 10.1126/science.aad2622
Wildsmith, M. D., Rose, T. H., Potter, I. C., Warwick, R. M., and Clarke, K. R. (2011). Benthic macroinvertebrates as indicators of environmental deterioration in a large microtidal estuary. Mar. Pollut. Bull. 62, 525–538. doi: 10.1016/j.marpolbul.2010.11.031
Keywords: moorings, soft sediment communities, monitoring, spatial variability, marine infrastructure, boating
Citation: Macolino AC, Dafforn KA, Hedge LH, Poore AGB and Johnston EL (2019) Fine-Scale Effects of Boat Moorings on Soft Sediment Communities Masked in Large-Scale Comparisons. Front. Mar. Sci. 6:101. doi: 10.3389/fmars.2019.00101
Received: 01 December 2018; Accepted: 20 February 2019;
Published: 12 March 2019.
Edited by:
Toyonobu Fujii, Tohoku University, JapanReviewed by:
Juan Moreira Da Rocha, Autonomous University of Madrid, SpainRodrigo Riera, Catholic University of the Most Holy Conception, Chile
Copyright © 2019 Macolino, Dafforn, Hedge, Poore and Johnston. This is an open-access article distributed under the terms of the Creative Commons Attribution License (CC BY). The use, distribution or reproduction in other forums is permitted, provided the original author(s) and the copyright owner(s) are credited and that the original publication in this journal is cited, in accordance with accepted academic practice. No use, distribution or reproduction is permitted which does not comply with these terms.
*Correspondence: Katherine A. Dafforn, katherine.dafforn@mq.edu.au