- 1Dauphin Island Sea Lab, Dauphin Island, AL, United States
- 2Department of Marine Sciences, University of South Alabama, Mobile, AL, United States
Research on marine mammal occurrence in ship channels often focuses on large cetaceans in offshore shipping routes, while nearshore research largely addresses small vessel strikes. Marine mammals, such as the West Indian manatee, that reside in or migrate through nearshore areas, have potential to travel through a wide range of channel types, encountering a greater diversity of vessels than previously recognized. We tested the extent and conditions of ship channel use by manatees along the north-central Gulf of Mexico (nGoM) coast by combining data from telemetry-tracked individuals, opportunistic citizen-sourced sightings, and environmental attributes linked to manatee movements. Manatees used both nearshore boat channels (130 and 300 m wide) and open water fairways but used nearshore channels much more frequently, consistent with habitat requirements. Satellite-tracked individuals swam faster and moved more directly in all channel types, indicating use of these channels as migratory and travel corridors. Accordingly, generalized additive models revealed that manatees used channels most often during spring/early summer and fall and at temperatures coincidental with entry to and exit from the nGoM during migration. Manatees also occurred in ship channels when freshwater discharges were low, likely because timing of peak manatee occurrence in the nGoM coincides with seasonally low discharge periods. Expanding shipping activity world-wide is likely to increase interactions between marine mammals and a variety of vessel types, and these effects may be particularly impactful to migratory animals like manatees that use nearshore habitats at the interface of recreational boating and commercial shipping. Linking near- and offshore ship channel use to migration and habitat use will better aid risk-assessment for vessel collision and other shipping related activities for migratory marine species globally.
Introduction
Boating and shipping activity are ubiquitous to global oceans and coasts. The intensity of shipping has increased drastically during the last several decades to accommodate the expanding global economy (Tournadre, 2014). More than 41,000 large merchant vessels were in operation globally in 2016, and nearly 16 million recreational vessels were in use in the United States alone as of 2017
(USDOT, 2016; National Marine Manufacturers Association, 2017). Vessel traffic can negatively impact marine mammals and other marine fauna by generating chemical and noise pollution (Weilgart, 2007; Liubartseva et al., 2015; Pirotta et al., 2019) and causing direct injury and mortality via collisions (Carrillo and Ritter, 2010; van der Hoop et al., 2015; Pirotta et al., 2019). Most research on vessel interactions with marine megafauna focuses on large cetaceans in offshore shipping routes or recreational boat collisions in nearshore waters (Laist et al., 2001; Laist and Shaw, 2006; van der Hoop et al., 2015; Edwards et al., 2016; Crum et al., 2019). Pressure from shipping, however, is increasing in nearshore as well as offshore channels (Tournadre, 2014; Zhang et al., 2015) and will likely affect smaller cetaceans, pinnipeds, and sirenians that live in nearshore areas at the interface of recreational and commercial channels. Little research has focused on the use of nearshore channel types by marine mammals.
The West Indian manatee (Trichechus manatus) is a sirenian vulnerable to multiple types of vessel interactions in nearshore areas. The Florida (T. m. latirostris) subspecies (Domning and Hayek, 1986) inhabits estuarine and nearshore coastal waters of southeastern North America (Gannon et al., 2007; Hieb et al., 2017), where they frequently interact with humans (Figure 1). Vessel collisions are the primary anthropogenic cause of injury and mortality in Florida manatees (Ackerman et al., 1995; Wright et al., 1995; Aipanjiguly et al., 2003). Manatees are frequently exposed to potential vessel collisions while foraging in shallow water, using thermal refuges, and moving among essential habitats (Flamm et al., 2005; Bauduin et al., 2013). The risk of vessel collision is additionally complicated during warm seasons when some manatees leave thermal refuge sites and migrate to widely distributed foraging areas (Deutsch et al., 2003; Cummings et al., 2014; Hieb et al., 2017). Thus, understanding manatee movement and habitat use in areas of high boat traffic is essential to conservation and management efforts (Flamm et al., 2005; Calleson and Frohlich, 2007; Bauduin et al., 2013; Rycyk et al., 2018).
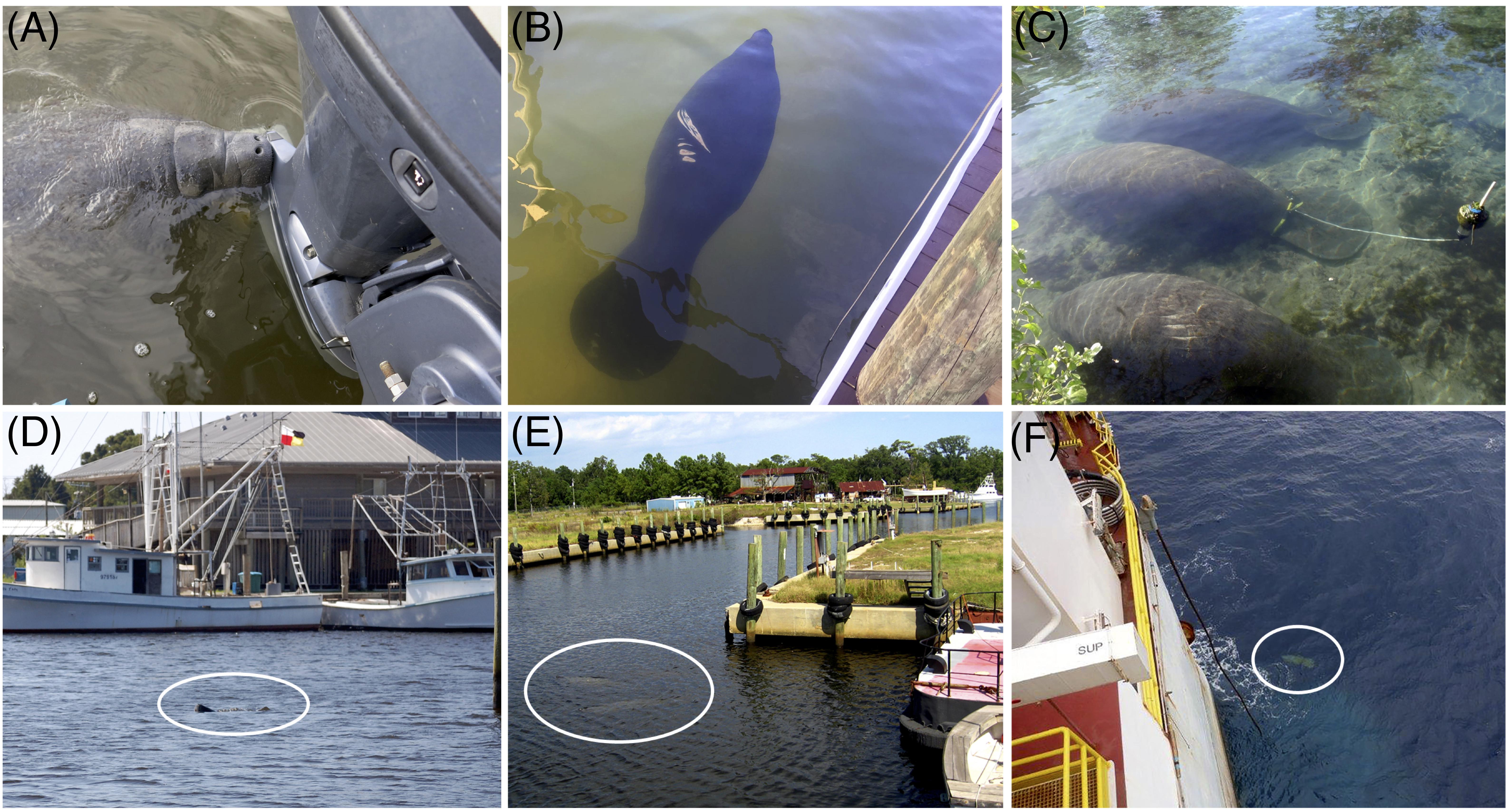
Figure 1. Typical examples of manatee interactions with vessels and channels. Manatee nudging a boat motor in Horseshoe Beach, FL 2013 (A). Fresh propeller scars on a manatee in Bayou St. John, AL 2018 (B) and healed propeller scars (bottom animal below tagged manatee) at Florida wintering ground 2012 (C). Manatees traveling in local commercial and recreational harbors in Apalachicola, FL in 2010 and Bayou La Batre, AL 2008, respectively (D,E). Manatee next to a seismic vessel in a fairway in the Gulf of Mexico 2013 (F). Photo credits: DISL/MSN staff and contributors.
While well-documented historically in the north-central Gulf of Mexico (nGoM; Powell and Rathbun, 1984; Fertl et al., 2005; Pabody et al., 2009), manatee sightings have drastically increased along the Alabama, Mississippi, and Louisiana coasts in warm months during the last several decades (Fertl et al., 2005; Pabody et al., 2009; Hieb et al., 2017). Increasing manatee population numbers in western and northwestern Florida may contribute to the increasing number of individuals migrating to the nGoM during recent years (Runge et al., 2004), but cold winter temperatures in the region limit year-round occupancy (Irvine, 1983; Bossart et al., 2003; Hieb et al., 2017). Cold-induced physiological problems can result in serious disease or death (Irvine, 1983; Bossart et al., 2003), forcing manatees in the United States to return to Florida to overwinter (Deutsch et al., 2003; Reep and Bonde, 2006). Thus, similar to many terrestrial, mammalian herbivores that migrate seasonally among foraging habitats, some manatee individuals move between winter ranges in peninsular Florida and summer ranges farther north (Deutsch et al., 2003; Ferguson and Elkie, 2004; White et al., 2010; Cummings et al., 2014), and other sirenian species follow similar migration patterns (Sheppard et al., 2006; Arraut et al., 2010; Castelblanco-Martínez et al., 2013).
Manatees, like other migratory species, face energetic costs during migrations and risk exposure to extreme conditions and dangerous situations (Sumich, 1983; Hein et al., 2012; Hopcraft et al., 2014; Le Corre et al., 2017). To reduce these costs, animals minimize the duration of migration by moving relatively fast and using direct routes (Sheppard et al., 2006; Tudorache et al., 2007; Åkesson et al., 2012). Animal trajectories during migration are often relatively straight, containing long step lengths (i.e., the distance between GPS pings) and small angles between steps that allow them to take the most direct migratory routes (Edelhoff et al., 2016; Michelot et al., 2017). These distinct movement patterns can be used to define areas and time periods of migratory and travel corridor use among tagged animals when direct observation is not possible.
The most direct migration routes for manatees in the nGoM overlap with an extensive network of ship channels (Gray et al., 2003; Zhang et al., 2015; U.S. Army Corps Of Engineers, 2018). This network of channels includes smaller, nearshore boat channels (Figures 1D,E) used by recreational boaters, commercial fisherman and shrimpers, barges, and other shipping vessels, as well as open water fairways (Figure 1F) that are mostly offshore and used by larger shipping freighters, seismic vessels, and cruise liners (Gray et al., 2003; Zhang et al., 2015). The Intracoastal Waterway (ICW), a heavily used route between Texas and Florida, and shipping fairways connect to inshore ports and rivers via overlapping boat channels and fairways and can convey larger vessels miles inshore. All of these channels pass through areas frequented by manatees (Hieb et al., 2017), potentially introducing them to various risks. The nearshore channels are typically used by smaller, faster vessels that allow less time for manatees to effectively react and avoid collisions (Nowacek et al., 2004; Laist and Shaw, 2006; Calleson and Frohlich, 2007; Rycyk et al., 2018). Larger vessels found in channels and fairways often move at slower speeds, but their large size may make collisions more lethal (Rommel et al., 2007; Rycyk et al., 2018). The collective use of these different ship channels is unknown but may put migratory species such as manatees at greater risk as species distributions and habitat change through time. Hence, defining the use of ship channels by manatees traveling in nearshore waters is necessary to assess risk from associated vessel interactions and support conservation for these and other migratory species.
We combined data from telemetry-tracked manatees and from opportunistic sightings to determine when and under what conditions manatees use the combination of ship channels in the nGoM. To determine if manatee movements were consistent with the use of channels as travel corridors and for migration, we compared swim speed, step lengths between GPS pings, and angles between steps for manatees in and outside channels (Michelot et al., 2017). We used generalized additive models (GAMs) to determine if nGoM water temperature, local air temperature, and freshwater discharge (as a proxy for salinity) affected manatee use of boat and ship channels at 2-week (fortnight) intervals year-round. This study has applications for ongoing management and recovery efforts for manatees and other species that occupy similar nearshore habitats, and it introduces a robust approach to help delineate the factors affecting channel use by many megafauna species.
Materials and Methods
Study Area
We examined manatee occurrence in ship channels along the nGoM, defined as eastern Louisiana, just west of Lake Pontchartrain (91°W) to western Florida at Pensacola Bay (87°W). Latitudinal boundaries for the study area were defined as the northern Mobile-Tensaw Delta (31°N) to the southeastern Louisiana coast (29.5°N). Longitudinal and latitudinal boundaries were based on regional manatee occurrence data and habitat requirements (Fertl et al., 2005; Pabody et al., 2009; Hieb et al., 2017). Channel and fairway locations in the nGoM were based on established GIS data layers from the US Department of Transportation National Transportation Atlas Databases (NTAD), U.S. Army Corps of Engineers Navigable Waterway Network, and the Bureau of Ocean Energy Management (BOEM) Data Center. We measured nearshore boat channels using ArcMap Version 10.3 (ESRI, 2014) following the NTAD “Navigable Waterways” GIS polyline layer with polygons at nGoM regional channel widths of 130 and 300 m (Gray et al., 2003; U.S. Army Corps Of Engineers, 2012; BIST, 2013). After initial analyses, we combined manatee occurrence data for the 130 and 300 m channels as representative of manatee occurrence in the nearshore boat channels throughout the region.
Data Collection
Telemetry Data
Manatee movement data were collected via GPS satellite telemetry, using established methods for capturing and tagging manatees (Bonde et al., 2012). We captured a total of 10 manatees during September 2009, August 2010, August 2012, and September 2014 (Table 1). Manatees were located via an aerial observer, and individual manatees were captured in a net deployed from a specialized capture boat (Bonde et al., 2012). Each manatee was then hauled aboard the boat and underwent a full health assessment onboard prior to fitting with a floating, tow-behind tagging platform (Telonics Inc., Mesa, AZ, United States; Bonde et al., 2012). Tags were attached to the peduncle with a belt and tether following standard tagging procedures for manatees (Deutsch et al., 1998; Weigle et al., 2001). Tags were programmed to record a GPS location every 15 or 30 min and locations were downloaded following tag recovery. Capture and release occurred at the same location, and manatees were typically released within 1 h of capture. Following initial tagging, some individuals lost tags, but retained the peduncle belts, and a new tag and tether combo was attached opportunistically at a later date by snorkeling or during a subsequent capture event. Tags were recovered by removing them at the end of their battery life and replacing with a new tag or when accidental loss from the animal occurred due to breaking at weak points in the belt or tether, which are designed to breakaway to protect against entanglement. Tag recovery was 98% because manatees use shallow, coastal systems and lost tags are easily located.
Continuous tagging duration was from 2 weeks to 13 months and non-continuous tagging (i.e., an individual tagged more than once) duration ranged from 4 to 22 months. We monitored animal locations using ARGOS service and with focal visual observations taken periodically while animals were tagged (Deutsch et al., 1998; Weigle et al., 2001). Data were downloaded directly from the tagging platforms and included standard GPS locations or quick-fix pseudoranging (QFP) positions accurate within 10 or 75 m, respectively. All locations with a successful GPS fix or a resolved QFP were included in the dataset, which were plotted in ArcMap 10.3 to verify accuracy of locations and all locations on land were removed (0.00006%). All work on manatees was performed under US Fish and Wildlife Service Permits MA107933-1 and MA37808A-0, Alabama Department of Conservation and Natural Resources Wildlife and Freshwater Fisheries Division annual permits, and University of South Alabama IACUC protocols 581568 and 1038636.
Sighting Data
We used manatee sighting data reported to the Dauphin Island Sea Lab’s Manatee Sighting Network (DISL/MSN), a citizen science program in the nGoM (Pabody et al., 2009). Sightings were compiled from DISL/MSN records to include live animal sightings obtained from public reports and targeted research efforts. Opportunistic, publicly reported sighting data were collected during 2007–2017 as described by Pabody et al. (2009) and Hieb et al. (2017). At a minimum, publicly reported sightings included the date, location, and number of manatees per sighting. Publicly reported sightings that did not provide sufficient location descriptions to derive GPS coordinates were excluded from the dataset. Duplicate sightings, which were defined as sightings reported by multiple observers but occurring at the same location, date, and time (within 20 min), were removed from the dataset. We augmented opportunistic sighting data with sightings during research efforts from 2009 to 2017 in Alabama, Mississippi, and Florida, including aerial observations during health assessments and surveys, boat-based monitoring by trained observers, and sightings of live animals during stranding response and rescue efforts.
Environmental Conditions
To examine relationships among manatee ship channel use and local environmental conditions, we divided the study area into five sub-regions defined longitudinally by major waterbodies: Lake Pontchartrain, Bay St. Louis, Pascagoula River, Mobile Bay, and Pensacola Bay (Supplementary Figure S1 and Table 2). For each sub-region, data for nGoM water temperature, local air temperature, and discharge were averaged for the week prior to each GPS ping or sighting date to represent conditions leading up to and during channel use. In cases when water temperature data were unavailable for the nGoM (14%), data were averaged for that fortnight period from other years the data were available. We obtained nGoM water temperature from 42040-Luke buoy located 63 nautical miles south of Dauphin Island, AL (29.208 N 88.226 W). We used data from the National Oceanic and Atmospheric Administration’s (NOAA) National Data Buoy Center to calculate average local air temperature from centrally located buoys within each sub-region (Table 2). Discharge data were obtained from the US Geological Survey (USGS) National Water Information System1 for major discharge sources in each sub-region (Table 2).
Statistical Analyses and Modeling
To determine differences in movement of tagged manatees inside versus outside of ship channels, we estimated manatee speeds, step lengths (i.e., the distance traveled between two GPS pings), and angles between step lengths (Edelhoff et al., 2016). To reduce error in our estimate of step length caused by large differences in the durations between GPS fixes, we only fit estimated step lengths for path segments where durations between GPS fixes were 25 – 35 min, and for tags that transmitted every 15 min, we only used fixes with 30-min durations (i.e., every other GPS fix). We estimated speed in kmh-1 as the step length between two successive GPS ping locations divided by the time between pings. We used Welch’s t-tests to determine differences in manatee speeds and natural-log transformed step lengths inside versus outside different channel types. Angles between step lengths were calculated using the ‘moveHMM’ package in R (Michelot et al., 2016), and we used a circular ANOVA, with the R package ‘circular’ (Agostinelli and Lund, 2013) to determine differences in angles inside versus outside of ship channels.
We used GAMs to determine how environmental variables—time-of-year (fortnight), nGoM water temperature, local air temperature, and freshwater discharge—affected manatee use of ship channels. We used fortnight because a 2-week period balanced a finer temporal resolution with the ability to compare detections and sightings at a seasonal scale. We constructed four sets of GAMs with the package mgcv in R (Wood, 2012); one set for 130 and 300 m boat channels and a second set for fairways for both tagged manatees and manatee sightings. To determine if collinearity existed among environmental variables, we used paired scatterplots and Pearson’s correlation statistics (Zuur, 2009) and found that nGoM water temperature, local air temperature, and discharge were all correlated with fortnight, but these correlations were too weak for collinearity to affect the results of the model (Dormann et al., 2013). Therefore, each GAM set included 20 models containing a combination of individual variables and their interactions (Supplementary Tables S1–S4). We used penalized thin plate smoothing splines to determine the main effects because they have been demonstrated to be the best smoother for these types of models (Wood, 2003). We used tensor smoothing to determine the interactions because this method is scale and unit invariant and can generalize between one- and multiple-dimensions (Wood et al., 2013).
For tagged manatees, we divided the number of GPS pings each day detected within each channel type (130 and 300 m channels and fairways) by the total number of pings from that day, resulting in the proportion of pings in each channel type per day. We used binomial models for tagged manatees with the logit link, as is standard with proportional data, and, because the total number of detected GPS pings varied each day, we used the total number of pings per day as an offset in the models (Zuur, 2009). For manatee sightings, we used binomial models with the logit link, as is standard with binomial data. To simplify models for sighting data, we excluded year if it was an insignificant predictor of ship channel use. We validated models graphically and tested the fit of the residuals.
To determine the best-fitting models for tagged manatees and manatee sightings in the 130 and 300 m channels and fairways, we used an information criterion approach (Johnson and Omland, 2004; Burnham et al., 2011). In this approach, we calculated Akaike information criterion (AIC) values as an indicator of model fit, where lower AIC values indicate better fit (Johnson and Omland, 2004). To measure the relative strength of each model, we calculated the normalized Akaike weights, w, for each model i, where , which is the probability that the given model is the best approximating model (Burnham et al., 2011; Symonds and Moussalli, 2011). Values of w > 0.9 indicate strong model support, while w < 0.9 indicate that other models may also fit the data well and should be considered in the analysis (Burnham et al., 2011; Symonds and Moussalli, 2011). To determine the relative importance of each variable, we calculated the normalized Akaike weight for each parameter wip, which is the sum of the wim values for all the models in which that variable was present (Symonds and Moussalli, 2011).
Results
Manatee Use of Ship Channels
Manatees broadly used ship channels across the nGoM, primarily occupying nearshore channels in rivers, canals, estuaries, and the ICW, with fewer manatees detected and sighted in offshore waters (Figure 2 and Table 3). Tagged and sighted manatees followed similar spatial patterns across the study area (Figure 2). For the 10 tagged manatees, GPS data included a total of 723 manatee days in the study area: 544 days in Alabama, 80 in Mississippi, 61 in Louisiana, and 37 in Florida. A total of 2,237 manatee sightings occurred from 2007 to 2017, including 1,945 sightings in Alabama, 205 in Mississippi, 79 in Florida, and 8 in Louisiana. Opportunistic, publicly reported sightings accounted for the majority of sighting data (93%) with an additional 150 sightings recorded during research efforts, including 137 sightings in Alabama, 12 in Mississippi, and 1 in Florida.
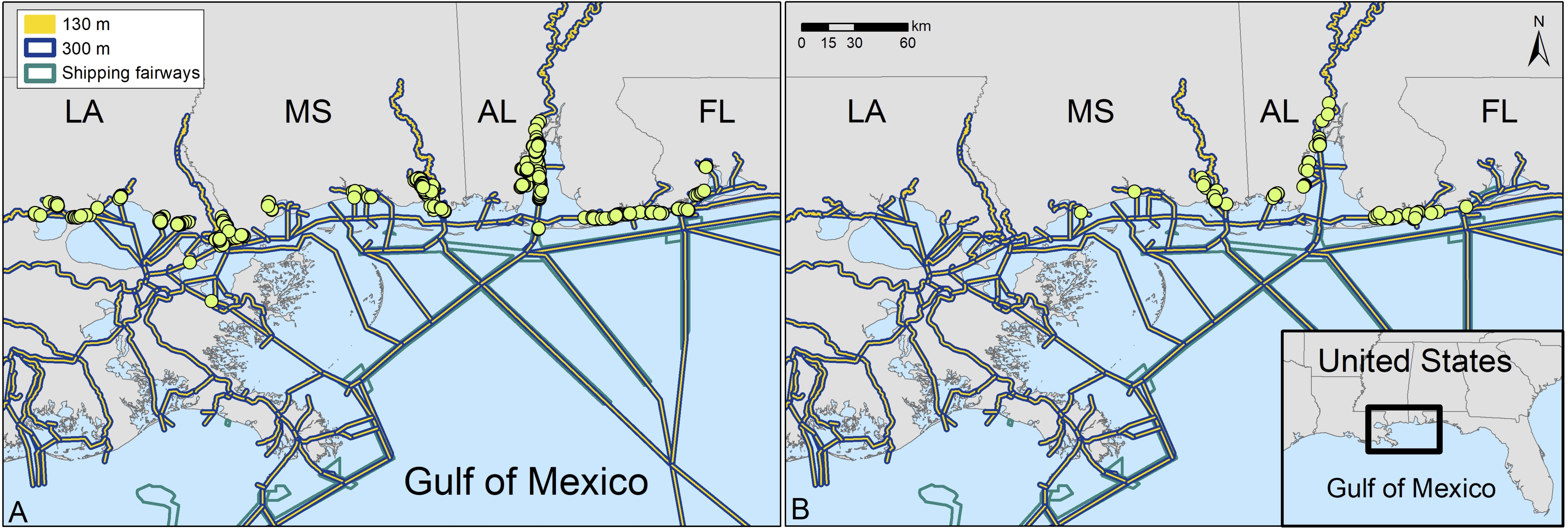
Figure 2. Maps of the nGoM with boat channels and fairways and locations of tagged (A) and sighted (B) manatees in ship channels.

Table 3. Number of tagged and sighted manatees in 130 and 300 m boat channels and shipping fairways (FW) and percentage of detected or sighted individuals in each type of channel.
Tagged manatees were detected in ship channels March – October (Figure 3A), and manatees were sighted in ship channels March – December (Figure 3E). Both tagged and sighted manatees occurred much more frequently in the 130 and 300 m boat channels than in fairways, including areas where fairways overlapped with boat channels (Figures 3A,E and Table 3). Tagged manatees occurred in ship channels when nGoM water temperature ranged from 22 to 32°C and local air temperature ranged from 12 to 31°C (Figures 3B,C), while manatees were sighted in ship channels when nGoM water temperatures ranged from 20 to 32°C and local air temperature ranged from 7 to 31°C (Figures 3F,G). Tagged and sighted manatees used ship channels when freshwater discharges ranged from 9 to 100,733⋅cfs-1 (Figure 3D) and 164 to 78,329⋅cfs-1 (Figure 3H), respectively. An example of these patterns can be seen in movements of a tagged manatee (TMA001, “Bama”) during fall 2009, when she used channels, including the Mobile Bay ship channel to access foraging sites in late October (nGoM water temperature = 26.8°C, local air temperature = 16.8°C, and discharge = 71,598⋅cfs-1), before exiting the region via the ICW between Bon Secour, AL and Pensacola, FL in early November (Supplementary Video S1).
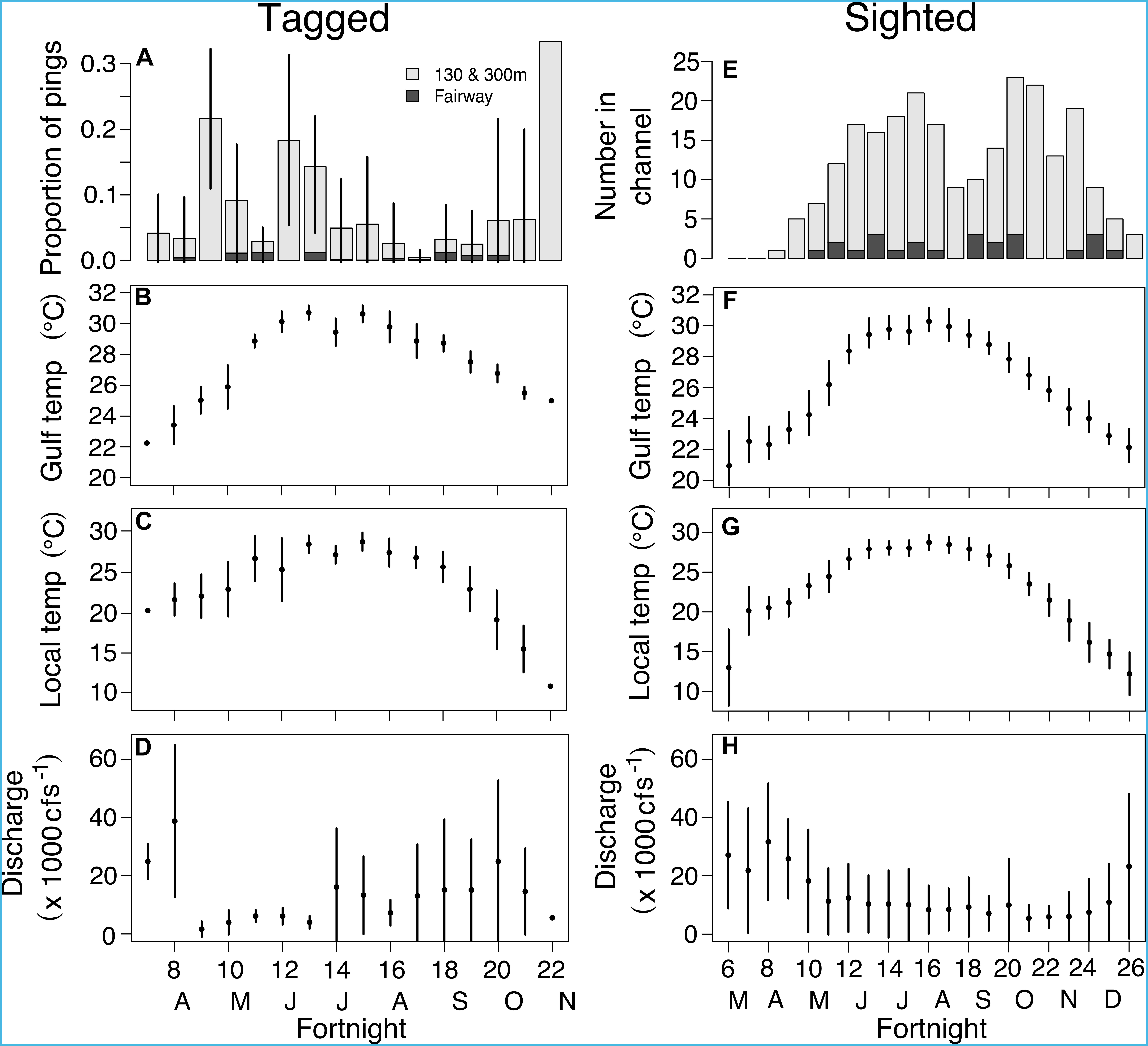
Figure 3. The average proportion of GPS pings (±SD) from tagged manatees (left column) in ship channels per fortnight period (A) and the sum of sighted manatees (right column) per fortnight period (E). The average (±SD) nGoM water temperature (B,F), local air temperature (C,G), and freshwater discharge of the nearest river (D,H) per fortnight for the periods of time that manatees were detected or sighted in ship channels.
Movements of tagged manatees were different inside compared to outside ship channels. We estimated that manatees swam faster inside the 130 and 300 m boat channels and fairways (130 and 300 m channels: t625.81= 4.19, p < 0.001; Fairways: t667.56= 4.72, p < 0.001; Figures 4A,C and Table 4), and step lengths were significantly longer inside the 130 and 300 m channels and fairways (130 and 300 m channels: t633.32= 9.75, p < 0.001; Fairways: t683.53= 11.20, p < 0.001; Figures 4B,D and Table 4). Step angles were not significantly different in or out of the 130 and 300 m channels or fairways (130 and 300 m channels: F1,26507 = 0.05, p = 0.83; Fairways: F1,26507 = 0.20, p = 0.65; Supplementary Figure S2). Tagged manatees also typically used ship channels for < 5 h (median: 2.36 and 1.60 h for 130 and 300 m channels and fairways, respectively); however, durations in ship channels up to 24 h were not uncommon, and, in several instances, manatees spent > 30 h in the channels (Figures 5A,C). Likewise, the distances that manatees traveled continuously in the ship channels were generally < 5 km (median: 3.08 and 1.62 km for 130 and 300 m channels and fairways, respectively), but distances up to 15 km were not uncommon and, in a few instances, manatees traveled up to 35 km in the channels (Figures 5B,D).
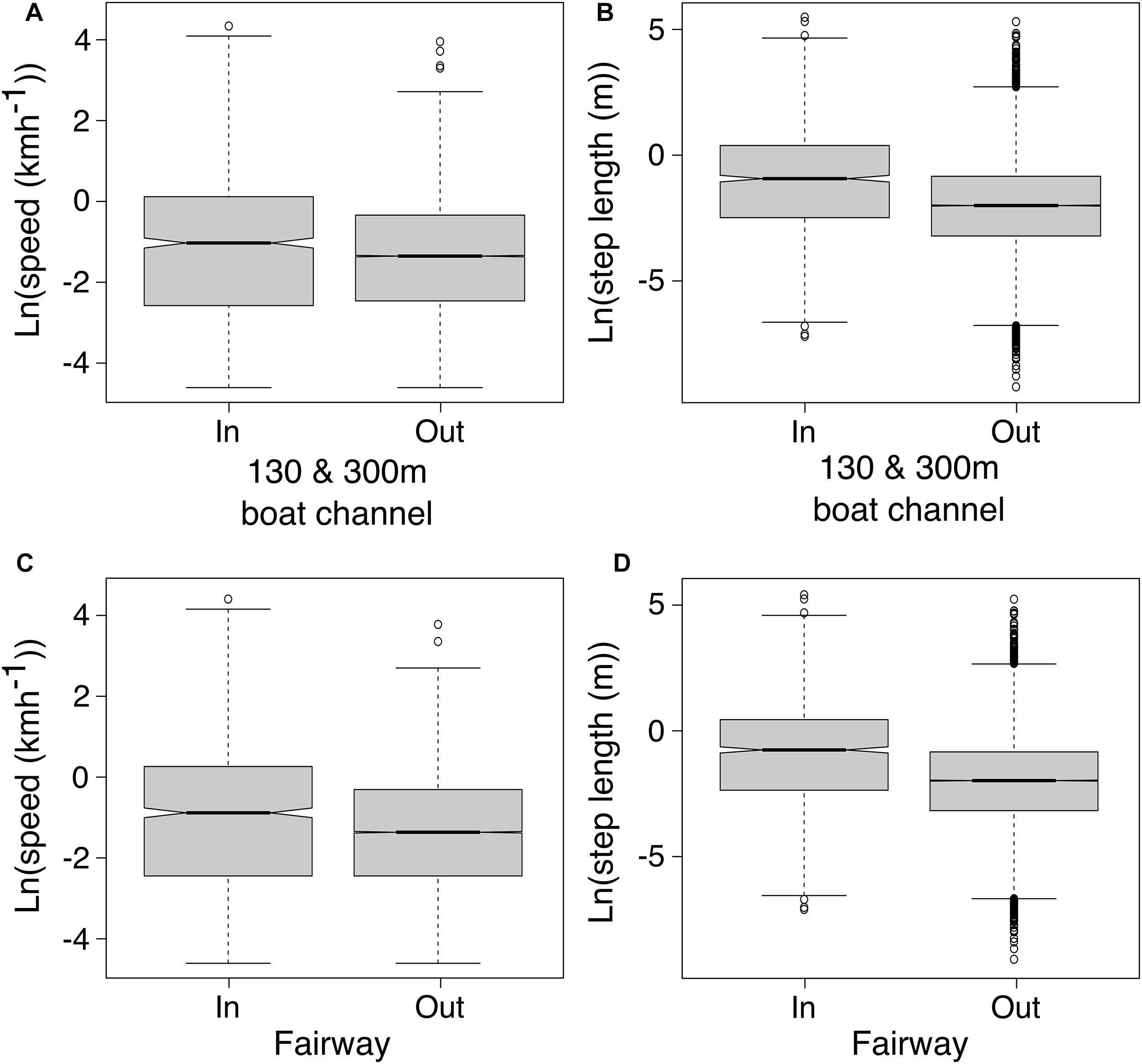
Figure 4. Estimated averages of natural log transformed manatee swimming speeds in and out of 130 and 300 m channels (A) and shipping fairways (C) and natural log transformed step lengths in and out of 130 and 300 m channels (B) and shipping fairways (D) for tagged manatees.
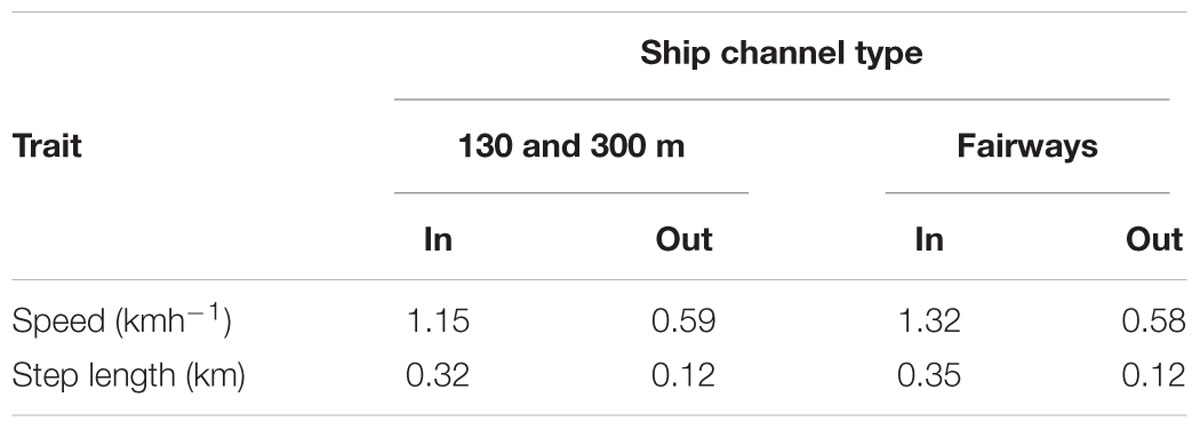
Table 4. Estimated average speed and step lengths of tagged manatees inside and outside of 130 and 300 m boat channels and shipping fairways.
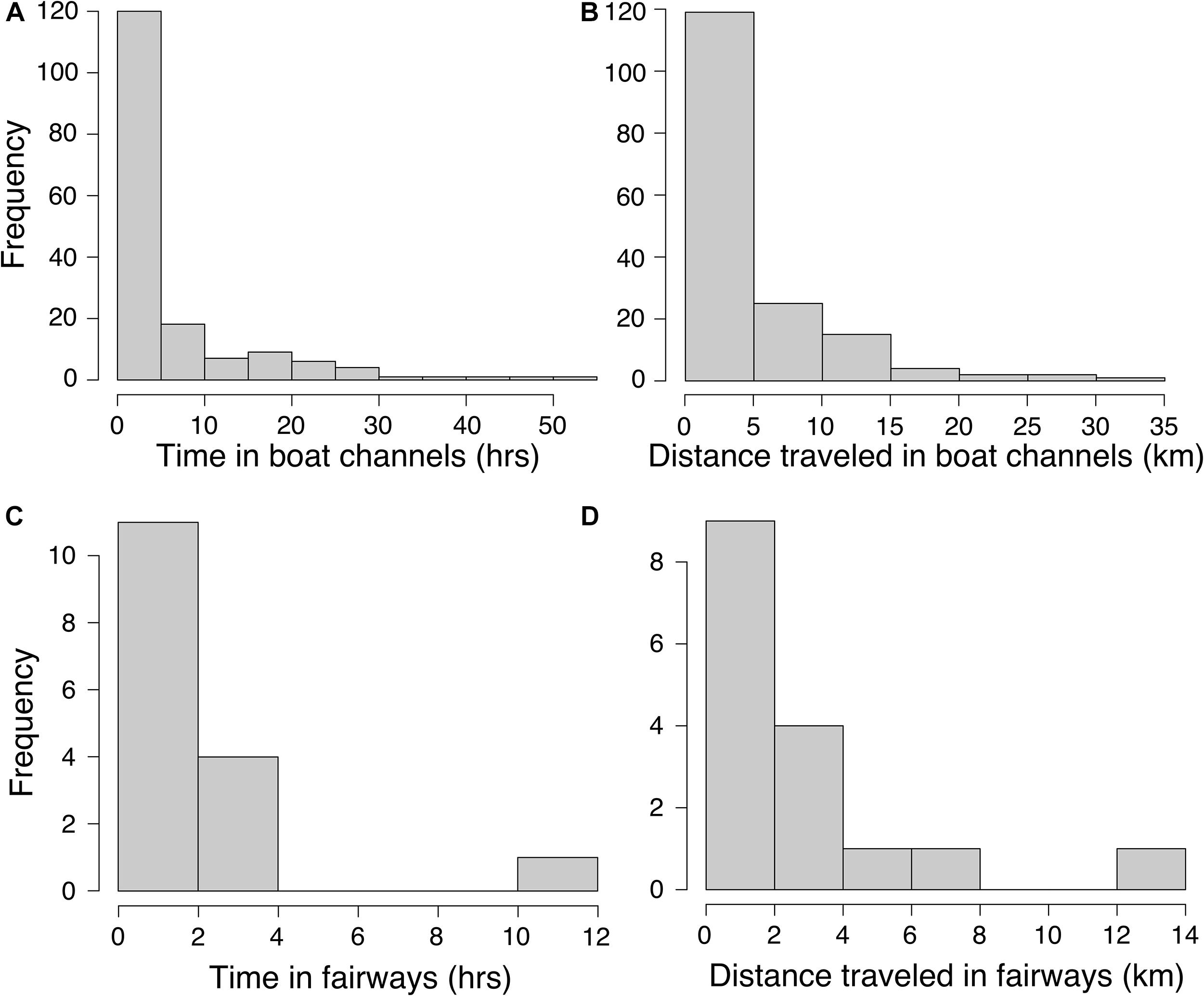
Figure 5. Histograms of continuous time durations that manatees spent in 130 and 300 m boat channels (A) and fairways (C) and associated distances traveled in the 130 and 300 m boat channels (B) and fairways (D).
Modeling of Tagged Manatees
The best-fitting model for telemetry-tracked manatees in the 130 and 300 m boat channels included fortnight, nGoM water temperature, and local air temperature and the interactions between fortnight and nGoM water temperature, local air temperature, and discharge (% Deviance Explained [R2] = 77%; Supplementary Table S1), which were all statistically significant predictors of ship channel use (Figures 6A–D, 7A–C and Table 5). The probability of detecting tagged manatees in the 130 and 300 m channels peaked May–June, decreased through the summer, and increased again September–October (Figure 6A). The probabilities of detections were highest when nGoM water temperatures were ∼24°C, corresponding to spring (May, June) and fall (October), and ∼31°C during the summer (July, August; Figures 6B, 7A). Results were similar when compared to local air temperatures, with peak use at 10–20°C during spring and fall with an additional peak at ∼31°C during the summer (Figures 6C, 7B). Tagged manatees were most likely to be detected in 130 and 300 m channels at low freshwater discharges during the spring and fall (Figures 6D, 7C) and at very high discharges during the spring (Figure 7C). All parameters had high weights except discharge (Supplementary Table S5), indicating that discharge had little effect on the fit of the model compared to other variables.
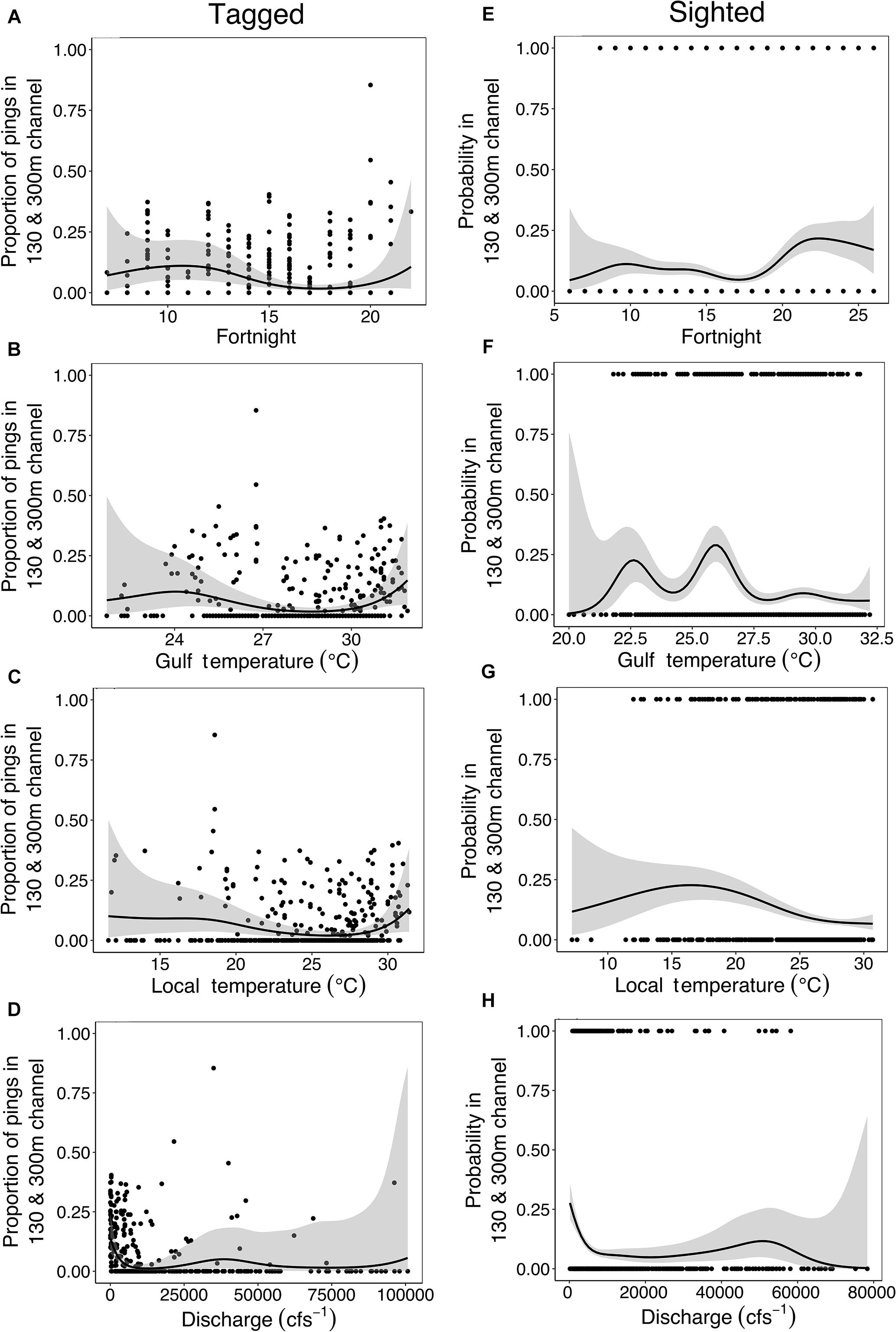
Figure 6. Smoothing curves for fortnight (A,E), nGoM water temperature (B,F), local air temperature (C,G), and freshwater discharge (D,H) from the generalized additive models for tagged (left column) and sighted (right column) manatees in the 130 and 300 m boat channels.
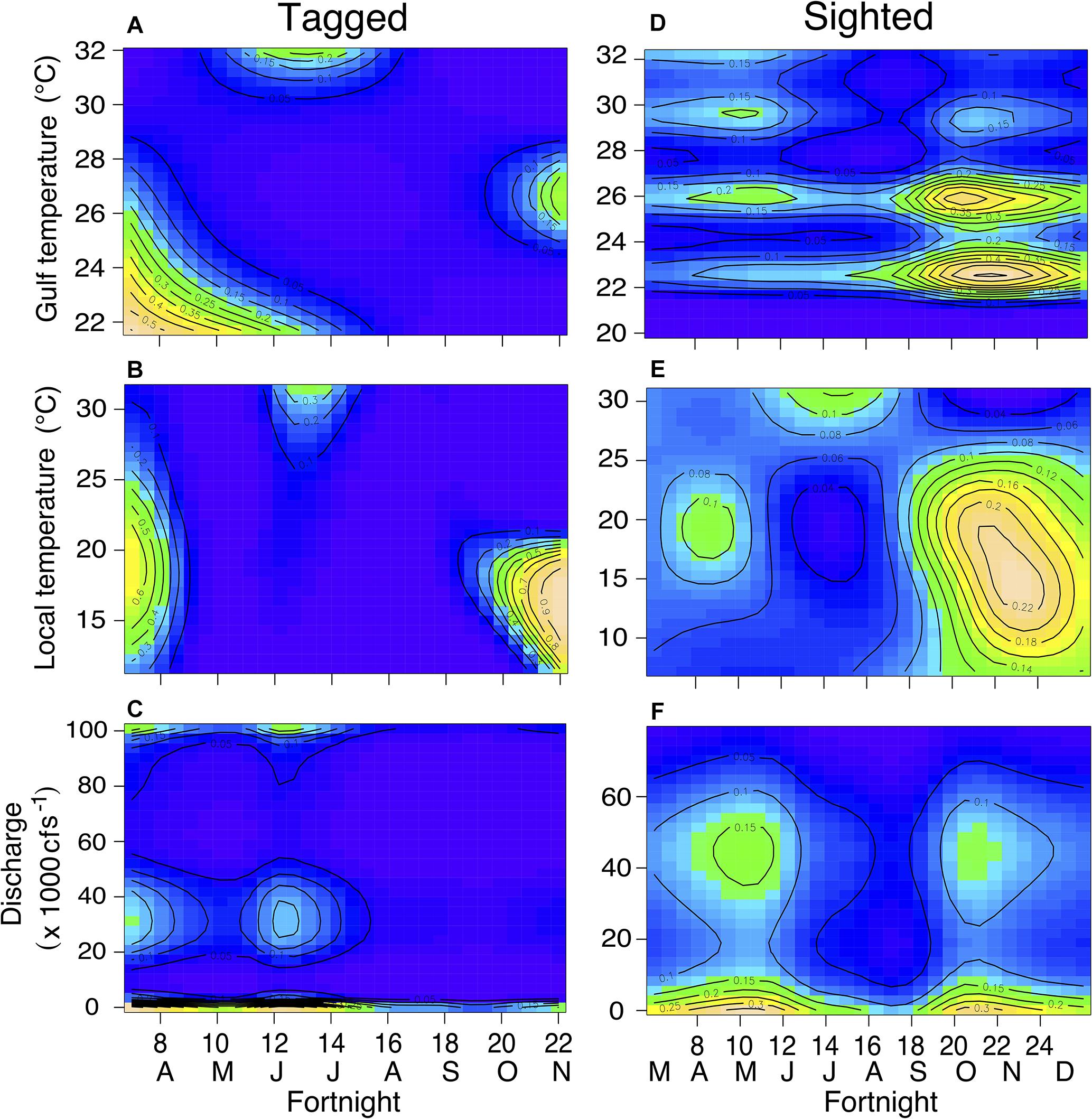
Figure 7. Contour plots displaying interactions between fortnight period and nGoM water temperature (A,D), local air temperature (B,E), and freshwater discharge (C,F) for tagged (left column) and sighted (right column) individuals in the 130 and 300 m ship channels. Cooler colors (e.g., blue, green) indicate lower probabilities while warmer colors (yellow, tan) indicate higher probabilities. Contour lines represent probabilities.
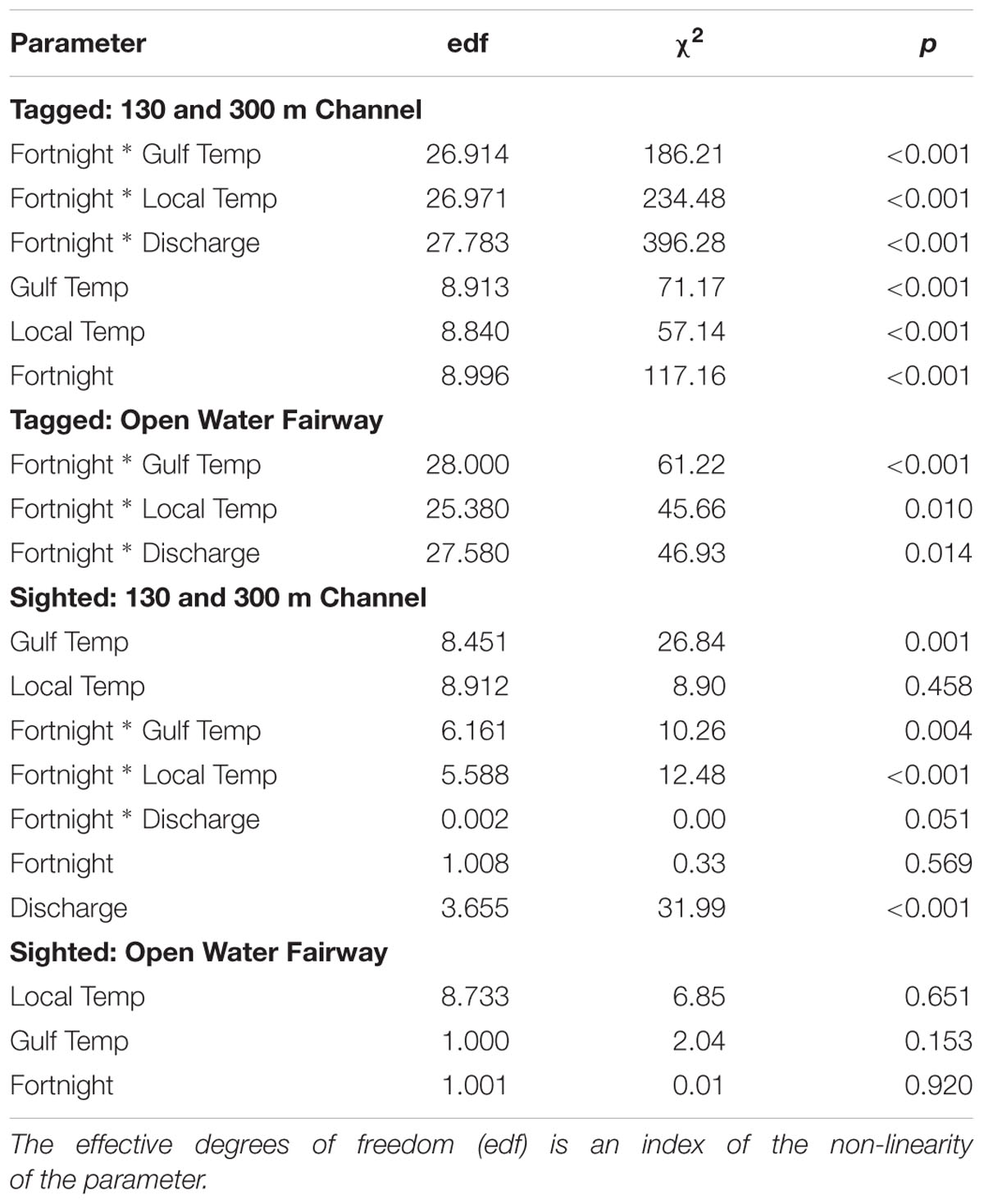
Table 5. Best-fitting models for tagged and sighted manatees in the 130 and 300 m boat channels and fairways.
The best-fitting model for tagged manatees in open water fairways included the three interactions (R2 = 65.3%; Supplementary Table S2), all of which were significant (Table 5). Manatees were most likely to be detected in fairways when nGoM water temperatures ranged from 24 to 31°C, generally in the late spring and early summer (Figure 8A), and local air temperatures ranged from 18 to 30°C, with peaks at ∼22°C in the spring, 26 and 30°C in the early and late summer, respectively, and at ∼19 and 14°C in the fall (Figure 8B). The probability of manatee detections in the fairways occurred at relatively low freshwater discharges in the late spring, early summer, and fall (Figure 8C).
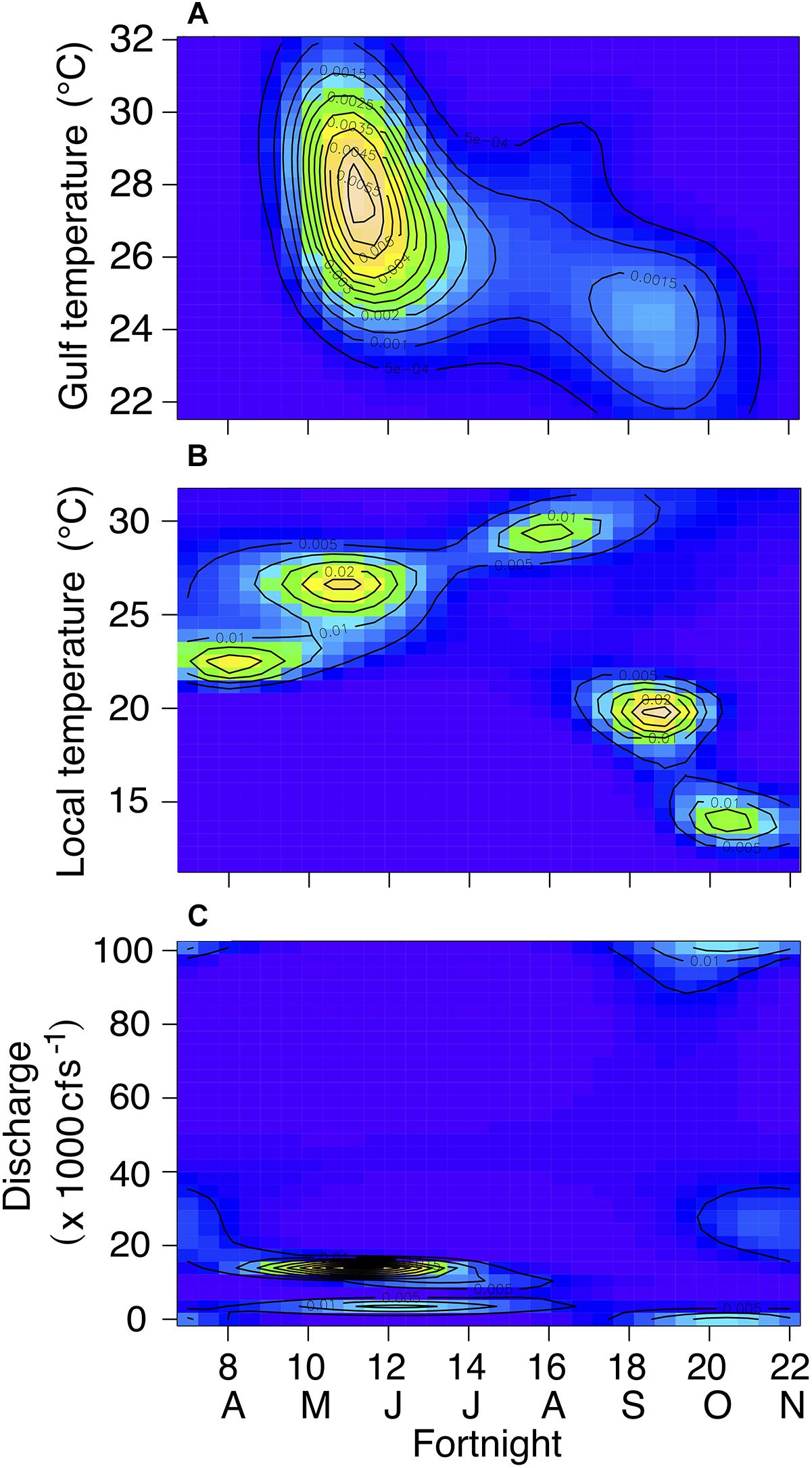
Figure 8. Contour plots displaying interactions between fortnight period and nGoM water temperature (A), local air temperature (B), and freshwater discharge (C) for tagged individuals in fairways. Cooler colors (e.g., blue, green) indicate lower probabilities while warmer colors (yellow, tan) indicate higher probabilities. Contour lines represent probabilities.
Modeling of Sighted Manatees
For sighted manatees, year was not a significant predicator of 130 and 300 m channel use (edf = 4.38, χ2 = 7.279, p = 0.225) and was not included in the model analysis. The best-fitting model included all four environmental variables (fortnight, nGoM water temperature, local air temperature, and freshwater discharge) and all three interactions (R2 = 12.5%; Supplementary Table S3), of which nGoM water temperature, discharge, and each interaction were statistically significant predictors of channel use (Figures 6F,H, 7D–F and Table 5). The probability of sighting manatees in the 130 and 300 m channels was relatively high April–June, decreased through the summer, and then increased September – December (Figure 6E). Manatees were most often sighted in any ship channels at nGoM water temperatures of 23°C (April–June) and 26°C (October–December) (Figures 6F, 7D) and when local air temperatures ranged between 10 and 25°C in the spring and fall (Figures 6G, 7E). Manatees were sighted most frequently in ship channels when freshwater discharge was low in the spring and fall (Figures 6H, 7F). Two other models, one that did not contain discharge and one that did not contain fortnight, but each contained all other factors and interactions, had relatively high model weights (Supplementary Table S3). The high weights of these models, as well as the lack of significance for fortnight and local air temperature and the low parameter weight for discharge (Supplementary Table S5), emphasize the relative importance of nGoM water temperature to manatee occurrence in the 130 and 300 m channels.
For manatees sighted in shipping fairways, year was not a significant predictor (edf = 1.003, χ2 = 2.83, p = 0.09) and was not included in the remaining model selection analysis. The best-fitting model included only nGoM water temperature, local air temperature, and fortnight (R2 = 8.94%; Supplementary Table S4); however, none of these factors were significant predictors of fairway use (Table 5). Furthermore, the model containing only local air temperature and another containing only the interaction between fortnight and local air temperature had high weights.
Discussion
Manatee Use of Ship Channels
Manatee movements in ship channels along the nGoM coast suggest they use these channels as migration routes and travel corridors among habitats. Manatees mostly used nearshore boat channels, moving east–west in the ICW, a heavily trafficked shipping route, and north-south in estuaries and rivers, consistent with known foraging habitats and other essential resources (Flamm et al., 2005; Cummings et al., 2014; Hieb et al., 2017). Manatees used the ICW most frequently between Gulf Shores, AL and Pensacola, FL during spring and fall, suggesting they use this channel to enter and exit the nGoM during seasonal migration. Accordingly, movements of tagged manatees in channels were typical of animals during migration or when moving among habitats, where manatees swam faster and had longer step lengths between GPS pings (Tudorache et al., 2007; Åkesson et al., 2012; Michelot et al., 2017). Speeds of manatees inside ship channels in this study were nearly identical to speeds of dugongs performing large-scale movements (Sheppard et al., 2006) but were faster than manatees migrating on the eastern coast of the United States (Deutsch et al., 2003). The similarity of angles between steps inside and outside of shipping channels may be explained by the temporal resolution of GPS pings (Wilson et al., 2013). The duration between GPS detections (∼30 min) could allow manatees moving in a discontinuous or meandering line outside of channels, but following a general heading, to appear to have slower speeds and shorter step lengths, but no difference in turn angle, as was seen in our analysis (Wilson et al., 2013). Additionally, manatee behavior can affect the quality of GPS fixes (e.g., swimming at depths and speeds that submerge the tag), which in turn can affect the step angle calculations. Despite these caveats, manatee movements in ship channels are consistent with use as travel corridors, particularly during seasonal migration, but finer temporal resolution of tagged individuals may reveal more detailed movements to explain differences in movements inside and outside channels.
Temperature is a migratory cue for manatees and dugongs (Deutsch et al., 2003; Sheppard et al., 2006; Cummings et al., 2014), and manatees used ship channels in our study at temperatures and times of the year associated with migration into or out of the region. Manatees and other sirenians that are susceptible to potentially fatal cold-stress typically migrate from summer ranges to wintering grounds (in peninsular Florida for manatees in the southern United States) when temperatures drop below ∼20°C, a threshold for healthy thermoregulation (Irvine, 1983; Bossart et al., 2003). In our study, manatees most frequently used ship channels during the spring/early summer as temperatures rose above ∼22°C, corresponding to entering the region, and the fall as temperatures dropped below ∼26°C, corresponding to exiting the region (Deutsch et al., 2003; Pabody et al., 2009; Hieb et al., 2017). Tagged manatees used open water fairways at similar times and temperatures as both tagged and sighted manatees in 130 and 300 m boat channels, but, overall, manatees were rarely detected in fairways, which are mostly located in offshore waters and less consistent with manatee habitat requirements (Reep and Bonde, 2006; Gannon et al., 2007; Marsh et al., 2011). These patterns of ship channel use by manatees in the nGoM are very similar to migratory patterns reported to-date along the southern United States Atlantic coast, including use of the ICW (Cummings et al., 2014), suggesting broad use of ship channels as migratory corridors by manatees throughout their United States range. Accordingly, temperature was the most significant predictor of manatee ship channel use in our models. While the interaction between fortnight and nGoM water and local air temperature were similar, local air temperature was consistently a few degrees lower than water temperature and a slightly weaker predictor of channel use. Because air temperature drives local water temperature in this study area (Park et al., 2007; Dzwonkowski et al., 2011), changes in air temperature can indirectly initiate manatee migration. Manatees have been documented to delay their north- or southward migrations during periods of unseasonably cold or warm air temperatures (Deutsch et al., 2003), which may explain some variability in relationships between temperature and manatee ship channel use detected in our study. Thus, while water temperature more directly influences manatee migrations, our data also indicate that air temperature has potential for use as a proxy in manatee movement studies when water temperature data are unavailable and broader relationships between water and air temperature are understood.
Manatees also used ship channels most frequently during periods of low freshwater discharge (<10,000⋅cfs-1). Low discharges are most common during the summer when manatees are present at higher numbers in the nGoM region; therefore, correlations between ship channel use and discharge may be coincidental to timing of manatee occurrence in the study area (Dzwonkowski et al., 2011; Hieb et al., 2017). Low discharge periods also correspond to higher summer temperatures, which could prompt manatees to seek refuge in cooler, deeper waters found in ship channels (Stith et al., 2011). Conversely, during periods of high discharge, salinity stratification in deeper channels such as the Mobile Bay ship channel (Hummell, 1990) results in more saline conditions that may drive manatees out of ship channels in favor of lower salinity waters. Manatees may also avoid ship channels during high discharge periods, particularly in large riverine-driven systems such as Mobile Bay, because movement may be more difficult and energetically costly when the direction of flow opposes the direction of travel (Fish, 1994). While discharge may not be as significant a factor as nGoM water or local air temperature in determining manatee use of ship channels, patterns of freshwater flow may act as a secondary factor mediating day-to-day use at local scales.
Combining datasets that used different methodologies helped to fill spatial and temporal data gaps and added confidence that the movement patterns we detected are meaningful for manatees in our study region. While sources of bias must be considered when using citizen-sourced data (e.g., uneven sampling effort or detection probability), these types of data are increasingly recognized as valuable contributions to long-term monitoring efforts and answering broad ecological questions (Dickinson et al., 2010; McKinley et al., 2017). In this study, the seasonal and spatial distribution of opportunistic manatee sightings both in and out of ship channels was highly consistent with patterns of manatee occurrence documented in the region during the last four decades (Fertl et al., 2005; Pabody et al., 2009; Hieb et al., 2017). Furthermore, we found very similar patterns in ship channel use between tagged and opportunistically sighted manatees. Tagged individuals provided a smaller set of shorter-term data but with high spatial resolution, while opportunistic, citizen-sourced sightings provided a large database that captured decadal-scale patterns of variation in manatee location and habitat use. Hence, our two datasets accentuate a common tradeoff between quantity and quality in ecological datasets on movement. Taken together, the consistency of results from these two independent datasets along with the similarity to patterns reported to-date along the southern United States Atlantic coast, including use of the ICW (Cummings et al., 2014), suggests broad use of ship channels as migratory corridors by manatees throughout their range in the United States.
Implications for Risk Assessment: Manatees and Other Marine Megafauna Species
Using ship channels as migration routes and travel corridors exposes manatees to a diversity of vessel types. Boat collisions are a leading cause of manatee mortality in Florida (Ackerman et al., 1995; Wright et al., 1995; Aipanjiguly et al., 2003), and manatee use of ship channels in the nGoM increases their risk of collision in waters west of Florida, where boat strike mortalities have been increasingly documented in recent years (Hieb et al., 2017). Boating speed limits and restricted access to critical manatee habitats in peninsular Florida have decreased collisions and contributed to population recovery (Laist and Shaw, 2006; Calleson and Frohlich, 2007; Gannon et al., 2007; Udell et al., 2018). However, increased use of habitats outside of Florida imposes new collision risks in areas with fewer protective regulations, many vessel types, and decreased awareness of manatees among boat operators. Accordingly, since 2013, three manatees deaths in the nGoM were attributed to blunt force trauma typical of vessel collision (no boat-related manatee mortality was documented prior to 2013 in our study area; Hieb et al., 2017). Although our data show manatees use boat channels more frequently than fairways, two of these mortalities occurred in or immediately adjacent to the Mobile Bay ship channel (Carmichael, 2017; Hieb et al., 2017), where boat channels overlap with a shipping fairway. These examples emphasize that manatees in areas with overlapping ship channel types potentially are at greater risk of collisions with a combination of recreational, commercial, passenger, and merchant vessels that are linked to manatee mortality (Calleson and Frohlich, 2007). Importantly, our data demonstrate that exposure to large vessels and associated mortality can occur even in inland harbors such as the Port of Mobile.
While most previous studies specifically evaluating collisions between vessels and manatees focus on recreational boats and other small vessels (Aipanjiguly et al., 2003; Laist and Shaw, 2006; Calleson and Frohlich, 2007; Bauduin et al., 2013), our study is unique in documenting use of multiple channel types and exposure to vessels at the intersection of boat channels and fairways. Globally, inland harbors are increasing in number and size to accommodate larger vessels (Walsh, 2012; Tournadre, 2014). For example, the main component of the Mobile Bay ship channel, which is currently 130 m wide, is planned to be expanded to 180m during the next few years (U.S. Army Corps Of Engineers, 2018), and similar harbor expansions are ongoing or planned globally (Wang, 2017). Additionally, exposure to increased noise and chemical pollution as well as increased habitat disturbance associated with shipping industries and channel construction and maintenance, such as dredging and channel widening, may have negative impacts on manatees (Todd et al., 2014; Liubartseva et al., 2015; Pirotta et al., 2019). As shipping activities continue to expand in nearshore areas, our data suggest potential for shipping traffic to pose increasing risks to manatees and other nearshore species such as small cetaceans, pinnipeds, and sea turtles.
Manatees, as well as other marine megafauna, face an uncertain future, with risks from human interactions (Ackerman et al., 1995; Wright et al., 1995; Aipanjiguly et al., 2003), habitat destruction (Laist and Reynolds, 2005; Marsh et al., 2011), and climate change (Marsh et al., 2011, 2017). Understanding ship channel use among species is crucial to evaluating risks and developing strategies to mitigate negative impacts (Carrillo and Ritter, 2010; van der Hoop et al., 2015; Martin et al., 2016; Crum et al., 2019; Pirotta et al., 2019). We show that GAMs populated with independently sourced datasets provide a powerful predictive tool that can help illuminate marine megafauna use of ship channels. Our modeling approach provides a complex evaluation of risk that can be tailored to different challenges based on type of species and their habitat requirements. Here, we focused primarily on nearshore channels (130 and 300 m) and some fairways, but our methods can easily be applied to either nearshore or offshore ship channels and fairways. While we chose variables based on manatee ecology—temperature, freshwater discharge, time-of-year (Deutsch et al., 2003; Reep and Bonde, 2006; Marsh et al., 2011)— other variables can easily be substituted based on the ecology of other marine species. Furthermore, we were able to combine telemetry and observational data to enhance occurrence data with information on movement and behavior of animals inside ship channels. We propose that this approach be used on a wider range of species among locations to help predict when and how marine megafauna use ship channels and to evaluate risks associated with channel use.
We provide new fundamental knowledge on movement ecology of a large, protected marine species and important information for managers, civil engineers, boaters, and the shipping industry to guide future conservation practices. Because manatee use of habitat in the nGoM is limited by temperature but increasing along with expanding vessel capacity and shipping activity, this region may represent the future landscape for temperature-dependent migratory and dispersing populations of many other megafauna species. The nGoM, and Mobile Bay in particular, can serve as a sentinel site that may be a ‘canary in the coal mine’ for assessing future boat-related risks to manatees and other migratory species. Using multiple datasets and a similar modeling approach, other researchers can evaluate nearshore ship channel use across a wider range of species and geographic distributions.
Data Availability
The datasets generated for this study are available on request to the corresponding author.
Ethics Statement
This study was carried out in accordance with the US Fish and Wildlife Service Permits MA107933-1 and MA37808A-0, Alabama Department of Conservation and Natural Resources Wildlife and Freshwater Fisheries Division annual permits. The protocol was approved by the University of South Alabama IACUC for protocols 581568 and 1038636.
Author Contributions
CC analyzed the data, wrote the main draft of the manuscript, and participated in editing the manuscript. EH contributed to the data collection and analysis for both tagged and sighted manatees, environmental data, and writing and editing the manuscript. MC contributed to collection and calculation of environmental data and editing the manuscript. KD contributed to the data collection for tagged manatees, determined coordinates of ship channels, prepared the SI video, and edited the manuscript. RC conceived the project, collected data for tagged and sighted manatees, contributed to data analysis and writing and editing the manuscript.
Funding
This project was funded in part by the NSF REU Program (OCE 1358873), Alabama Division of Wildlife and Freshwater Fisheries under traditional Section 6 of the US Fish and Wildlife Service, the Northern Gulf Institute, Mobile Bay National Estuary Program, and Dauphin Island Sea Lab.
Conflict of Interest Statement
The authors declare that the research was conducted in the absence of any commercial or financial relationships that could be construed as a potential conflict of interest.
Acknowledgments
We thank T. Gilbreath (Mobile Harbor Master) for providing information regarding channel widths, A. Aven (DISL/University of South Alabama) and E. Thompson (DISL/NSF-REU) for assistance in data acquisition, postprocessing from tagged manatees, and consultation on early data analysis, DISL staff and volunteers for assistance in collecting and managing sighting data, and the residents and visitors of the nGoM for reporting their sightings to DISL’s Manatee Sighting Network since 2007. We specially thank M. Ross and all the staff at Sea to Shore Alliance for their expert assistance coordinating and conducting manatee captures, tagging or retagging, and monitoring and collecting data from tagged animals.
Supplementary Material
The Supplementary Material for this article can be found online at: https://www.frontiersin.org/articles/10.3389/fmars.2019.00318/full#supplementary-material
Footnotes
References
Ackerman, B. B., Wright, S. D., Bonde, R., Odell, D., and Banowetz, D. (1995). Trends and Patterns in Mortality of Manatees in Florida. Population Biology of the Florida Manatee, Information and Technology Report I. Washington, DC: US Department of the Interior, 223–258.
Aipanjiguly, S., Jacobson, S. K., and Flamm, R. (2003). Conserving manatees: knowledge, attitudes, and intentions of boaters in Tampa Bay, Florida. Conserv. Biol. 17, 1098–1105. doi: 10.1046/j.1523-1739.2003.01452.x
Åkesson, S., Klaassen, R., Holmgren, J., Fox, J. W., and Hedenström, A. (2012). Migration routes and strategies in a highly aerial migrant, the common swift Apus apus, revealed by light-level geolocators. PLoS One 7:e41195. doi: 10.1371/journal.pone.0041195
Arraut, E., Marmontel, M., Mantovani, J., Novo, E., Macdonald, D., and Kenward, R. (2010). The lesser of two evils: seasonal migrations of Amazonian manatees in the Western Amazon. J. Zool. 280, 247–256. doi: 10.1111/j.1469-7998.2009.00655.x
Bauduin, S., Martin, J., Edwards, H. H., Gimenez, O., Koslovsky, S. M., and Fagan, D. E. (2013). An index of risk of co-occurrence between marine mammals and watercraft: example of the Florida manatee. Biol. Conserv. 159, 127–136. doi: 10.1016/j.biocon.2012.10.031
BIST (2013). iBoating: Marine Charts and GPS. Barcelona: Barcelona Institute of Science and Technology.
Bonde, R. K., Garrett, A., Belanger, M., Askin, N., Tan, L., and Wittnich, C. (2012). Biomedical health assessments of the Florida manatee in Crystal River—Providing opportunities for training during the capture, handling, and processing of this endangered aquatic mammal. J. Mar. Anim. Ecol. 5, 17–28.
Bossart, G. D., Meisner, R. A., Rommel, S., Ghim, S., and Jenson, A. B. (2003). Pathological features of the Florida manatee cold stress syndrome. Aquat. Mamm. 29, 9–17. doi: 10.1578/016754203101024031
Burnham, K. P., Anderson, D. R., and Huyvaert, K. P. (2011). AIC model selection and multimodel inference in behavioral ecology: some background, observations, and comparisons. Behav. Ecol. Sociobiol. 65, 23–35. doi: 10.1007/s00265-010-1029-6
Calleson, C. S., and Frohlich, R. K. (2007). Slower boat speeds reduce risks to manatees. Endanger. Spec. Res. 3, 295–304. doi: 10.3354/esr00056
Carmichael, R. H. (2017). The West Indian Manatee Population in Mobile Bay, AL and Surrounding Waters (1912–2017), Dauphin Island Sea Lab, AL: Data Management Center. Available at: http://cf.disl.org/datamanagement/metadata_folder/DISL-Carmichael-MSN-010-2016.xml
Carrillo, M., and Ritter, F. (2010). Increasing numbers of ship strikes in the Canary Islands: proposals for immediate action to reduce risk of vessel-whale collisions. J. Cetacean Res. Manag. 11, 131–138.
Castelblanco-Martínez, D. N., Padilla-Saldívar, J., Hernández-Arana, H. A., Slone, D., Reid, J., and Morales-Vela, B. (2013). Movement patterns of Antillean manatees in Chetumal Bay (Mexico) and coastal Belize: a challenge for regional conservation. Mar. Mamm. Sci. 29, E166–E182.
Crum, N., Gowan, T., Krzystan, A., and Martin, J. (2019). Quantifying risk of whale–vessel collisions across space, time, and management policies. Ecosphere 10, e02713. doi: 10.1002/ecs2.2713
Cummings, E. W., Pabst, D. A., Blum, J. E., Barco, S. G., Davis, S. J., Thayer, V. G., et al. (2014). Spatial and temporal patterns of habitat use and mortality of the Florida manatee (Trichechus manatus latirostris) in the mid-Atlantic states of North Carolina and Virginia from 1991 to 2012. Aquat. Mamm. 40:126. doi: 10.1578/am.40.2.2014.126
Deutsch, C. J., Bonde, R. K., and Reid, J. P. (1998). Radio-tracking manatees from land and space: tag design, implementation, and lessons learned from long-term study. Mar. Technol. Soc. J. 32, 18–29.
Deutsch, C. J., Reid, J. P., Bonde, R. K., Easton, D. E., Kochman, H. I., and O’Shea, T. J. (2003). Seasonal movements, migratory behavior, and site fidelity of West Indian manatees along the Atlantic coast of the United States. Wildlife Monogr. 67, 1–77.
Dickinson, J. L., Zuckerberg, B., and Bonter, D. N. (2010). Citizen science as an ecological research tool: challenges and benefits. Annu. Rev. Ecol. Evol. Syst. 41, 149–172. doi: 10.1146/annurev-ecolsys-102209-144636
Domning, D. P., and Hayek, L. A. C. (1986). Interspecific and intraspecific morphological variation in manatees (Sirenia: Trichechus). Mar. Mamm. Sci. 2, 87–144. doi: 10.1111/j.1748-7692.1986.tb00034.x
Dormann, C. F., Elith, J., Bacher, S., Buchmann, C., Carl, G., Carré, G., et al. (2013). Collinearity: a review of methods to deal with it and a simulation study evaluating their performance. Ecography 36, 27–46. doi: 10.1111/j.1600-0587.2012.07348.x
Dzwonkowski, B., Park, K., Ha, H. K., Graham, W. M., Hernandez, F. J., and Powers, S. P. (2011). Hydrographic variability on a coastal shelf directly influenced by estuarine outflow. Cont. Shelf Res. 31, 939–950. doi: 10.1016/j.csr.2011.03.001
Edelhoff, H., Signer, J., and Balkenhol, N. (2016). Path segmentation for beginners: an overview of current methods for detecting changes in animal movement patterns. Move. Ecol. 4:21. doi: 10.1186/s40462-016-0086-5
Edwards, H. H., Martin, J., Deutsch, C. J., Muller, R. G., Koslovsky, S. M., Smith, A. J., et al. (2016). Influence of manatees’ diving on their risk of collision with watercraft. PloS One 11:e0151450. doi: 10.1371/journal.pone.0151450
Ferguson, S. H., and Elkie, P. C. (2004). Seasonal movement patterns of woodland caribou (Rangifer tarandus caribou). Journal of Zoology 262, 125–134. doi: 10.1017/s0952836903004552
Fertl, D., Schiro, A., Regan, G., Beck, C. A., Adimey, N., Price-May, L., et al. (2005). Manatee occurrence in the northern Gulf of Mexico, west of Florida. Gulf Caribb. Res. 17, 69–94.
Fish, F. E. (1994). Influence of hydrodynamic-design and propulsive mode on mammalian swimming energetics. Aust. J. Zool. 42, 79–101.
Flamm, R. O., Weigle, B. L., Wright, I. E., Ross, M., and Aglietti, S. (2005). Estimation of manatee (Trichechus manatus latirostris) places and movement corridors using telemetry data. Ecol. Appl. 15, 1415–1426. doi: 10.1890/04-1096
Gannon, J. G., Scolardi, K. M., Reynolds, J. E. III, Koelsch, J. K., and Kessenich, T. J. (2007). Habitat selection by manatees in Sarasota Bay, Florida. Mar. Mamm. Sci. 23, 133–143. doi: 10.1111/j.1748-7692.2006.00096.x
Gray, W. O., Waters, J. K., Blume, A. L., and Landsburg, A. C. (2003). Channel design and vessel maneuverability: next steps. Mar. Technol. 40, 93–105.
Hein, A. M., Hou, C., and Gillooly, J. F. (2012). Energetic and biomechanical constraints on animal migration distance. Ecol. Lett. 15, 104–110. doi: 10.1111/j.1461-0248.2011.01714.x
Hieb, E. E., Carmichael, R. H., Aven, A., Nelson-Seely, C., and Taylor, N. (2017). Sighting demographics of the West Indian manatee Trichechus manatus in the north-central Gulf of Mexico supported by citizen-sourced data. Endanger. Species Res. 32, 321–332. doi: 10.3354/esr00817
Hopcraft, J. G. C., Morales, J. M., Beyer, H., Borner, M., Mwangomo, E., Sinclair, A., et al. (2014). Competition, predation, and migration: individual choice patterns of Serengeti migrants captured by hierarchical models. Ecol. Monogr. 84, 355–372. doi: 10.1890/13-1446.1
Hummell, R. L. (1990). Main Pass and the Ebb-Tidal Delta of Mobile Bay. Alabama, AL: Geological Survey of Alabama.
Irvine, A. B. (1983). Manatee metabolism and its influence on distribution in Florida. Biol. Conserv. 25, 315–334. doi: 10.1016/0006-3207(83)90068-x
Johnson, J. B., and Omland, K. S. (2004). Model selection in ecology and evolution. Trends Ecol. Evol. 19, 101–108. doi: 10.1016/j.tree.2003.10.013
Laist, D. W., Knowlton, A. R., Mead, J. G., Collet, A. S., and Podesta, M. (2001). Collisions between ships and whales. Mar. Mamm. Sci. 17, 35–75. doi: 10.1111/j.1748-7692.2001.tb00980.x
Laist, D. W., and Reynolds, J. E. (2005). Florida manatees, warm-water refuges, and an uncertain future. Coast. Manag. 33, 279–295. doi: 10.1080/08920750590952018
Laist, D. W., and Shaw, C. (2006). Preliminary evidence that boat speed restrictions reduce deaths of Florida manatees. Mar. Mamm. Sci. 22, 472–479. doi: 10.1111/j.1748-7692.2006.00027.x
Le Corre, M., Dussault, C., and Côté, S. D. (2017). Weather conditions and variation in timing of spring and fall migrations of migratory caribou. J. Mammal. 98, 260–271.
Liubartseva, S., De Dominicis, M., Oddo, P., Coppini, G., Pinardi, N., and Greggio, N. (2015). Oil spill hazard from dispersal of oil along shipping lanes in the Southern Adriatic and Northern Ionian Seas. Mar. Pollut. Bull. 90, 259–272. doi: 10.1016/j.marpolbul.2014.10.039
Marsh, H., Arraut, E. M., Diagne, L. K., Edwards, H., and Marmontel, M. (2017). “Impact of climate change and loss of habitat on Sirenians,” in Marine Mammal Welfare, ed. A. Butterworth (Cham: Springer), 333–357. doi: 10.1007/978-3-319-46994-2_19
Marsh, H., O’Shea, T. J., and Reynolds, J. E. III (2011). Ecology and Conservation of the Sirenia: Dugongs and Manatees. Cambridge: Cambridge University Press.
Martin, J., Sabatier, Q., Gowan, T. A., Giraud, C., Gurarie, E., Calleson, C. S., et al. (2016). A quantitative framework for investigating risk of deadly collisions between marine wildlife and boats. Methods Ecol. Evol. 7, 42–50. doi: 10.1111/2041-210x.12447
McKinley, D. C., Miller-Rushing, A. J., Ballard, H. L., Bonney, R., Brown, H., Cook-Patton, S. C., et al. (2017). Citizen science can improve conservation science, natural resource management, and environmental protection. Biol. Conserv. 208, 15–28.
Michelot, T., Langrock, R., Bestley, S., Jonsen, I. D., Photopoulou, T., and Patterson, T. A. (2017). Estimation and simulation of foraging trips in land-based marine predators. Ecology 98, 1932–1944. doi: 10.1002/ecy.1880
Michelot, T., Langrock, R., and Patterson, T. A. (2016). moveHMM: an R package for the statistical modelling of animal movement data using hidden Markov models. Methods Ecol. Evol. 7, 1308–1315. doi: 10.1111/2041-210x.12578
National Marine Manufacturers Association (2017). Recreational Boating Statistical Abstract. Chicago, IL: National Marine Manufacturers Association.
Nowacek, S. M., Wells, R. S., Owen, E. C., Speakman, T. R., Flamm, R. O., and Nowacek, D. P. (2004). Florida manatees, Trichechus manatus latirostris, respond to approaching vessels. Biol. Conserv. 119, 517–523. doi: 10.1016/j.biocon.2003.11.020
Pabody, C. M., Carmichael, R. H., Rice, L., and Ross, M. (2009). A new sighting network adds to 20 years of historical data on fringe West Indian manatee (Trichechus manatus) populations in Alabama waters. Gulf Mex. Sci. 27:6.
Park, K., Kim, C.-K., and Schroeder, W. W. (2007). Temporal variability in summertime bottom hypoxia in shallow areas of Mobile Bay, Alabama. Estuar. Coasts 30, 54–65. doi: 10.1007/bf02782967
Pirotta, V., Grech, A., Jonsen, I. D., Laurance, W. F., and Harcourt, R. G. (2019). Consequences of global shipping traffic for marine giants. Front. Ecol. Environ. 17:39–47. doi: 10.1002/fee.1987
Powell, J. A., and Rathbun, G. B. (1984). Distribution and abundance of manatees along the northern coast of the Gulf of Mexico. Gulf Mex. Sci. 7:1.
Reep, R., and Bonde, R. K. (2006). The Florida Manatee: Biology and Conservation. Gainesville, FL: University Press of Florida.
Rommel, S. A., Costidis, A. M., Pitchford, T. D., Lightsey, J. D., Snyder, R. H., and Haubold, E. M. (2007). Forensic methods for characterizing watercraft from watercraft-induced wounds on the Florida manatee (Trichechus manatus latirostris). Mar. Mamm. Sci. 23, 110–132. doi: 10.1111/j.1748-7692.2006.00095.x
Runge, M. C., Langtimm, C. A., and Kendall, W. L. (2004). A stage-based model of manatee population dynamics. Mar. Mamm. Sci. 20, 361–385. doi: 10.1111/j.1748-7692.2004.tb01167.x
Rycyk, A. M., Deutsch, C. J., Barlas, M. E., Hardy, S. K., Frisch, K., Leone, E. H., et al. (2018). Manatee behavioral response to boats. Mar. Mamm. Sci. 34, 924–962. doi: 10.1111/mms.12491
Sheppard, J. K., Preen, A. R., Marsh, H., Lawler, I. R., Whiting, S. D., and Jones, R. E. (2006). Movement heterogeneity of dugongs, Dugong dugon (Müller), over large spatial scales. J. Exp. Mar. Biol. Ecol. 334, 64–83. doi: 10.1016/j.jembe.2006.01.011
Stith, B. M., Reid, J. P., Langtimm, C. A., Swain, E. D., Doyle, T. J., Slone, D. H., et al. (2011). Temperature inverted haloclines provide winter warm-water refugia for manatees in southwest Florida. Estuar. Coasts 34, 106–119. doi: 10.1007/s12237-010-9286-1
Sumich, J. L. (1983). Swimming velocities, breathing patterns, and estimated costs of locomotion in migrating gray whales, Eschrichtius robustus. Can. J. Zool. 61, 647–652. doi: 10.1139/z83-086
Symonds, M. R., and Moussalli, A. (2011). A brief guide to model selection, multimodel inference and model averaging in behavioural ecology using Akaike’s information criterion. Behav. Ecol. Sociobiol. 65, 13–21. doi: 10.1007/s00265-010-1037-6
Todd, V. L., Todd, I. B., Gardiner, J. C., Morrin, E. C., MacPherson, N. A., DiMarzio, N. A., et al. (2014). A review of impacts of marine dredging activities on marine mammals. ICES J. Mar. Sci. 72, 328–340. doi: 10.1093/icesjms/fsu187
Tournadre, J. (2014). Anthropogenic pressure on the open ocean: the growth of ship traffic revealed by altimeter data analysis. Geophys. Res. Lett. 41, 7924–7932. doi: 10.1002/2014gl061786
Tudorache, C., Blust, R., and De Boeck, G. (2007). Swimming capacity and energetics of migrating and non-migrating morphs of three-spined stickleback Gasterosteus aculeatus L. and their ecological implications. J. Fish Biol. 71, 1448–1456. doi: 10.1111/j.1095-8649.2007.01612.x
U.S. Army Corps Of Engineers (2012). UFC-4-152-07 Design: small craft berthing facilities. Washington, DC: U.S. Army Corps Of Engineers.
U.S. Army Corps Of Engineers (2018). Mobile Harbor, Alabama general reevaluation report. Washington, DC: U.S. Army Corps of Engineers.
Udell, B. J., Martin, J., Fletcher, R. J. Jr., Bonneau, M., Edwards, H. H., Gowan, T. A., et al. (2018). Integrating encounter theory with decision analysis to evaluate collision risk and determine optimal protection zones for wildlife. J. Appl. Ecol. 56, 1050–1062. doi: 10.1111/1365-2664.13290
USDOT (2016). Data Statistics. Available at: https://www.maritime.dot.gov/data-reports/data-statistics/data-statistics (accessed February, 2019).
van der Hoop, J. M., Vanderlaan, A. S., Cole, T. V., Henry, A. G., Hall, L., Mase-Guthrie, B., et al. (2015). Vessel strikes to large whales before and after the 2008 Ship Strike Rule. Conserv. Lett. 8, 24–32. doi: 10.1111/conl.12105
Walsh, M. (2012). US Port and Inland Waterways Modernization: Preparing for Post-Panamax Vessels. Washington, DC: USACE-Institute for water resources.
Wang, M. (2017). The role of Panama Canal in global shipping. Marit. Bus. Rev. 2, 247–260. doi: 10.1108/mabr-07-2017-0014
Weigle, B. L., Wright, I. E., Ross, M., and Flamm, R. O. (2001). Movement of Radio-Tagged Manatees in Tampa Bay and Along Florida’s West Coast, 1991–1996 (St. Petersburg, FL: Florida Marine Research Institute TR-7), 156.
Weilgart, L. S. (2007). The impacts of anthropogenic ocean noise on cetaceans and implications for management. Can. J. Zool. 85, 1091–1116. doi: 10.1016/j.marpolbul.2018.01.014
White, P., Proffitt, K. M., Mech, L. D., Evans, S. B., Cunningham, J. A., and Hamlin, K. L. (2010). Migration of northern Yellowstone elk: implications of spatial structuring. J. Mammal. 91, 827–837. doi: 10.1644/08-mamm-a-252.1
Wilson, R., Griffiths, I., Legg, P., Friswell, M., Bidder, O., Halsey, L., et al. (2013). Turn costs change the value of animal search paths. Ecol. Lett. 16, 1145–1150. doi: 10.1111/ele.12149
Wood, S. (2012). mgcv: Mixed GAM Computation Vehicle with GCV/AIC/REML Smoothness Estimation. R package Version 1.7-17.
Wood, S. N. (2003). Thin plate regression splines. J. R. Stat. Soc. Ser. B 65, 95–114. doi: 10.1111/1467-9868.00374
Wood, S. N., Scheipl, F., and Faraway, J. J. (2013). Straightforward intermediate rank tensor product smoothing in mixed models. Stat. Comput. 23, 341–360. doi: 10.1007/s11222-012-9314-z
Wright, S., Ackerman, B., Bonde, R., Beck, C., and Banowetz, D. (1995). “Analysis of watercraft-related mortality of manatees in Florida, 1979–1991,” in Population Biology of the Florida Manatee, eds T. J. OShea, B. B. Ackerman, and H. F. Percival (Collins, CO: National Biological Service Information Report, Ft).
Zhang, Y., Li, S., and Guo, Z. (2015). The Evolution of the coastal economy: the role of working waterfronts in the alabama gulf coast. Sustainability 7, 4310–4322. doi: 10.3390/su7044310
Keywords: movement ecology, citizen science, generalized additive models, satellite telemetry, northern Gulf of Mexico, fairway, vessel strike
Citation: Cloyed CS, Hieb EE, Collins MK, DaCosta KP and Carmichael RH (2019) Linking Use of Ship Channels by West Indian Manatees (Trichechus manatus) to Seasonal Migration and Habitat Use. Front. Mar. Sci. 6:318. doi: 10.3389/fmars.2019.00318
Received: 18 February 2019; Accepted: 28 May 2019;
Published: 12 June 2019.
Edited by:
Joshua Nathan Smith, Murdoch University, AustraliaReviewed by:
Athena Rycyk, New College of Florida, United StatesDaryl Paul Domning, Howard University, United States
Copyright © 2019 Cloyed, Hieb, Collins, DaCosta and Carmichael. This is an open-access article distributed under the terms of the Creative Commons Attribution License (CC BY). The use, distribution or reproduction in other forums is permitted, provided the original author(s) and the copyright owner(s) are credited and that the original publication in this journal is cited, in accordance with accepted academic practice. No use, distribution or reproduction is permitted which does not comply with these terms.
*Correspondence: Carl S. Cloyed, Y2Nsb3llZEBkaXNsLm9yZw==