- 1School of Biological Sciences, University of Aberdeen, Aberdeen, United Kingdom
- 2School of Environmental Engineering, Technical University of Crete, Chania, Greece
The occurrence of priming effects (PEs) on the degradation of particulate terrestrial organic matter (OM) (13C-wheat detritus) was studied in marine sediments using phytoplankton detritus as priming inducer. Two scenarios, i.e., single-pulse vs. repetitive deposition of same amounts of algal detritus, were tested in sediment core incubation experiments. In the first case, a single pulse of phytodetritus emulated the sudden large input of phytoplanktonic material onto sediments following the decline of spring blooms in surface waters, whereas the repetitive deposition of small amounts of algal detritus represented the low, continuous flow of marine input of either pelagic or benthic origin that remains significant all year round. The mineralization rate of wheat detritus to 13CO2 in each treatment was measured on days 6, 8, 14, and 20 following the initiation of the experiment. No evidence of PEs on 13C-wheat mineralization upon the addition of labile phytodetritus could be detected in either scenario under the experimental conditions employed in this study.
Introduction
Ecosystems along the land-ocean continuum play a pivotal role in the transformation, sequestration and export of terrestrially derived terrigenous organic matter (OM) to the open ocean (Burdige, 2005; Cai, 2011; Bianchi et al., 2018); ∼55–80% of dissolved and particulate organic carbon flux from the continents is remineralized in the coastal zone, the remainder being preserved, buried and reincorporated into the longer-term components of the sedimentary cycle (Burdige, 2005; Blair and Aller, 2012). Terrigenous OM exported to the coastal ocean consists largely of vascular plant detritus, assumed to be relatively resistant to decay, which comes into contrast with its apparent high remineralization efficiency terrigenous OM in the coastal ocean (Bianchi, 2011). This contradiction has been one of the most persistent questions in global carbon cycling for the past 20 years (Hedges et al., 1997; Bianchi, 2011) but the mechanisms behind terrigenous OM processing on the land-ocean interface remain unclear (Bauer et al., 2013). The priming effect (PE), defined as a change in the microbial degradation of refractory OM upon addition of labile material, has been proposed as a possible mechanism facilitating the removal of terrigenous OM from marine ecosystems due to the co-presence of more easily degradable marine-derived OM (Guenet et al., 2010; Bianchi, 2011). Nevertheless, previous studies that have attempted to verify this hypothesis in freshwater and marine ecosystems have produced inconsistent results (Bengtsson et al., 2014; Wagner et al., 2014; Gontikaki et al., 2015; Ward et al., 2016).
Large pulsed inputs of marine OM in coastal and deep-water sediments occur following the decline of spring phytoplankton blooms in surface waters and usually cause an increase in benthic metabolism (Billen et al., 1990; Gooday, 2002). These pulsed depositions, however, are superimposed upon a low, steady level of marine OM input of either pelagic or benthic origin that remains significant all year round. Most priming studies in aquatic ecosystems have simulated single-pulse inputs that are usually characterized by the growth of a saprophytic population with r-strategist characteristics metabolizing easily accessible components of marine OM (van Nugteren et al., 2009; Gontikaki et al., 2013, 2015; Bianchi et al., 2015; Trevathan-Tackett et al., 2018). Under such conditions, apparent or negative PE due to preferential substrate utilization are likely to occur (Kuzyakov, 2002; Gontikaki et al., 2013). On the contrary, we hypothesized that the low, continuous supply of labile marine OM would maintain background levels of benthic metabolism with no disruption to the microbial community, thus causing real priming of terrigenous OM. We used pre-aged 13C-wheat detritus as a proxy for terrigenous OM and tested the effect of single-pulse vs. repetitive deposition of algal detritus to investigate the occurrence and magnitude of PE under different scenarios of marine OM input. To our knowledge, this is the first study to examine how temporal differences in marine OM input affect terrigenous OM degradation in marine sediments.
Materials and Methods
Sediment Sampling and Experimental Set-Up
Sediment was collected in Perspex cores (3.6 cm ID × 30 cm length) from the mudflats in the lower reach of the Ythan estuary, Aberdeenshire, Scotland, United Kingdom in February 2017 (Supplementary Figure S1). The cores were inserted 10 cm into the sediments by hand at low tide and kept in a temperature-controlled room at 10°C for the duration of the experiment. Core incubations were initiated following a 3-day acclimation period with continuous oxygenation. 13C-labeled wheat detritus (97 atom% grinded over a 1 mm sieve; IsoLife, Netherlands) was chosen as a proxy for terrigenous OM due to the fact that crop, mainly cereals, and grassland account for >95% of the Ythan River catchment area (Raffaelli, 1999). Wheat detritus was pre-aged in unfiltered seawater in the dark for 50 days prior to the incubation experiment to imitate natural degradation during the transition from land to sea. Freeze-dried unlabelled detritus of the diatom Thalassiosira pseudonana was used as a priming inducer (C and N composition of substrates are given in Supplementary Table S1). The amount of diatom detritus added was equivalent to the monthly deposition based on the mean annual daily phytoplankton deposition in the Ythan estuary (Baird and Milne, 1981). Four treatments – Ctrl, 13C-Ctrl, Pulse and Dose – were tested (Supplementary Table S2). The addition of wheat detritus marked the start of the experiment (day 0). Diatoms were added 1 week later, on day 7, in both Pulse and Dose treatments. Thereafter, diatom doses were added every other day in Dose treatment cores (Supplementary Table S2). Closed core incubations were performed on days 6, 8, 14, and 20 to measure production of dissolved inorganic carbon (total DIC and DI13C). Incubations lasted for 4–6 h. Details on the experimental set up and sampling procedure are given in Supplementary Information (see section “Supplementary Information on the Sampling Site, Experimental Set up and Sampling Procedure”, Supplementary Figure S2).
Sample Treatment and Analysis
Seawater samples were sterile-filtered and stored at 4°C in 3 ml exetainers (Labco, United Kingdom) with no headspace. The concentration of CO2 and carbon isotope ratio in the samples were measured at the University of California Davis Stable Isotope Facility1. δ13C values were expressed relative to the international standard V-PDB (Vienna PeeDee Belemnite). The mineralization of added wheat-derived carbon was calculated as the product of excess 13C and the total DIC concentration divided by the fractional abundance of 13C in wheat using well-established equations (Gontikaki et al., 2015).
Statistical Analysis
Statistical models to study the effect of treatment and incubation day (both categorical variables) on total and 13C-wheat mineralization were developed for each response variable using linear mixed-effect modeling (Zuur et al., 2009). Core identity was incorporated in the models as a random effect to impose a correlation structure on all observations within each core. Variance covariate terms were also included where data exploration revealed instances of unequal variance. Day 8 was excluded from analysis due to loss of 2 replicates from the Dose treatment on that sampling point. Due to the importance of this particular time point that followed diatoms addition on day 7, we also performed an analysis excluding the Dose treatment, but including day 8. The analysis was performed using the “nlme” package (Pinheiro et al., 2018) in the “R” programming environment (R Core Team, 2018). A detailed description of the statistical analysis is provided in Supplementary Information (see section “Supplementary Information on the Statistical Analysis”).
Results
Total and wheat mineralization per treatment and incubation day are shown in Figures 1, 2. Total mineralization was significantly affected by incubation day (L. ratio = 13.59, df1, p = 0.0011) and treatment (L. ratio = 12.28, df1, p = 0.0065) when days 6, 14, and 20 were tested [Supplementary Information see section “Model Output for the Total Mineralization (Total DIC) Data Analysis (Dataset Excluding Day 8)”, Supplementary Figure S3]. Total mineralization rate was lowest on day 14 in all treatments. Significantly higher total mineralization was observed in the Dose treatment compared to 13C-Ctrl and Pulse but none was significantly different from Ctrl (Supplementary Information see section “Model Output for the Total Mineralization (Total DIC) Data Analysis (Dataset Excluding Day 8)”, Supplementary Figure S3). When Dose treatment is excluded from the analysis (but day 8 included) the effect of treatment is lost (L. ratio = 1.01, df1, p = 0.6032) and total mineralization is only affected by incubation day (L. ratio = 16.13, df1, p = 0.0011) with highest rates measured on day 8 [Supplementary Information see section “Model Output for the Total Mineralization (Total DIC) Data Analysis Excluding Dose Treatment (Including Day 8)”, Supplementary Figure S4].
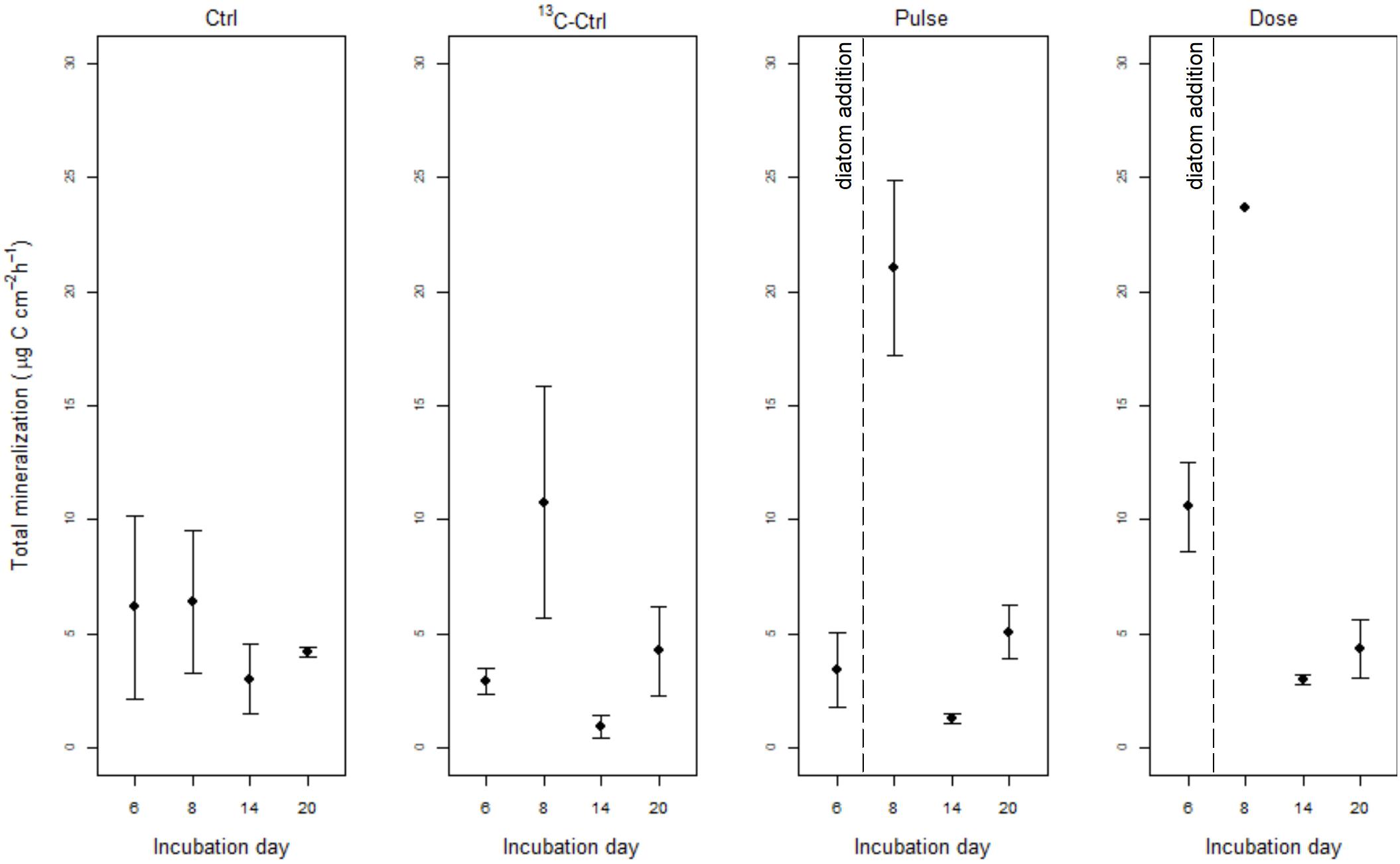
Figure 1. Total mineralization rate (μg C cm−2 h−1) (mean ± SE) in Ctrl (no addition), 13C-Ctrl (13C-wheat only), Pulse (13C-wheat + diatoms pulse), and Dose (13C-wheat + repeated addition of low doses of diatoms) treatments. Note that diatom detritus in Pulse and Dose treatments was added on day 7.
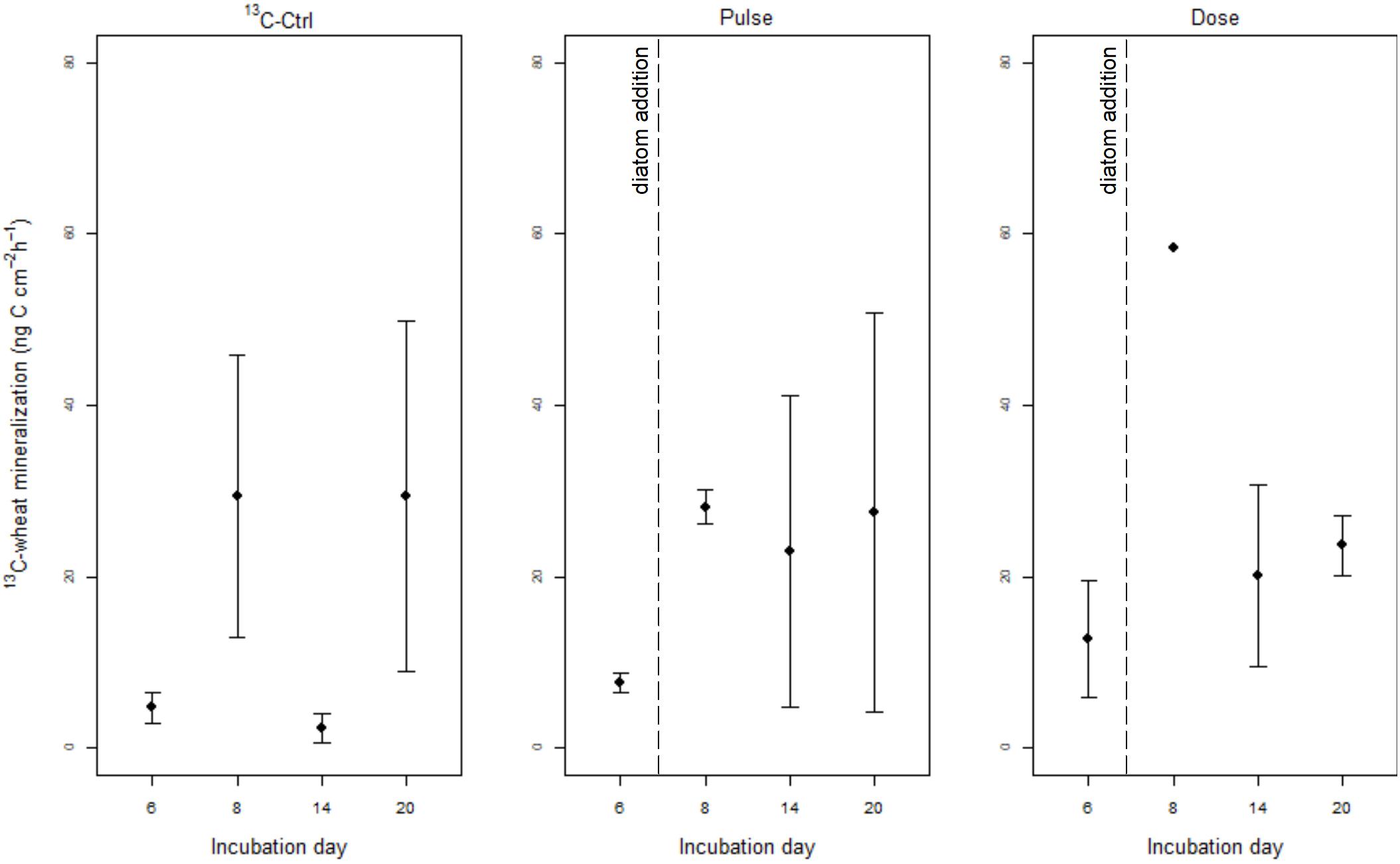
Figure 2. Mineralization rate of 13C-wheat (ng C cm−2 h−1) (mean ± SE) in 13C-Ctrl (13C-wheat only), Pulse (13C-wheat + diatoms pulse), and Dose (13C-wheat + repeated addition of low doses of diatoms) treatments. Note that diatom detritus in Pulse and Dose treatments was added on day 7.
Priming effect was evaluated as the difference in 13C-wheat mineralization between treatments with and without diatoms addition. If PE was present, we would expect addition of diatoms to the Pulse and Dose treatments on day 7 to result in higher 13C-wheat mineralization compared to 13C-Ctrl. It is reminded that on day 0, cores of all treatments apart from Ctrl had been amended with 13C-wheat only. 13C-wheat mineralization was not significantly different between treatments (L. ratio = 4.31, df1, p = 0.1158) or incubation day (L. ratio = 4.15, df1, p = 0.1254) when days 6, 14, and 20 were tested (Supplementary Information see section “Model Output for the 13C-Wheat Mineralization (DI13C) Data Analysis (Dataset Excluding Day 8)”). When day 8 is included in the analysis (but Dose treatment excluded), 13C-wheat mineralization was affected by incubation day (L. ratio = 20.38, df1, p < 0.0001) but not treatment (L. ratio = 2.49, df1, p = 0.1143). Highest 13C-wheat mineralization was observed on days 8 and 20 regardless of treatment (Supplementary Information see section “Model output for the 13C-Wheat Mineralization (DI13C) Data Analysis (dataset Excluding Dose Treatment (Including Day 8)”, Supplementary Figure S5). The full dataset used for the analysis can be found in Supplementary Table S3.
Discussion
The co-metabolic interactions between allochthonous and autochthonous marine-derived material were studied with the aim to uncover if PE is an important factor in the effective degradation of terrigenous OM in marine sediments. This study comes as a follow-up of Gontikaki et al. (2015) in which the occurrence of PE on terrigenous OM degradation was studied at the same location in sediment-water slurries with 13C-lignocellulose as proxy for terrigenous OM and a single pulse of marine OM. Gontikaki et al. (2015) reported differences in the timing but not overall quantity of terrigenous OM mineralization in marine OM-amended treatments compared to controls within 20 days. Due to the inconclusive results on PE occurrence in that study, we repeated a similar experiment but with improved methodology; the terrigenous OM proxy was naturally aged plant detritus, instead of extracted lignocellulose, and whole-core incubations that better represent the whole benthic community compared to sediment-water slurries were performed. Indeed, pre-aging resulted in a terrigenous OM substrate with higher C:N compared to lignocellulose (169 vs. 107, respectively) possibly indicating more complete removal of easily degradable components. This study further examined the effect of temporal differences in marine OM deposition, i.e., single large pulse vs. several lower inputs, which naturally occur in sedimentary environments but have not been addressed previously in the context of PE.
The pulse of marine OM on the sediments can result in the rapid growth of opportunistic diatom-degrading populations, observed as a surge in total mineralization within the first few days following addition. This, however, would complicate the observation of real PE due to the accelerated turnover of bacterial biomass that triggers apparent PE (Nottingham et al., 2009; Kuzyakov, 2010). In this study, total mineralization was not significantly different between cores that received diatoms compared to controls suggesting that a possible disturbance in the function of the benthic community by the sudden input of marine OM was avoided. PE, as evaluated by the difference in 13C-wheat mineralization between diatom-amended (Pulse and Dose) and wheat-only (13C-Ctrl) treatments, could not be detected under the experimental conditions of this study.
There is little agreement as to the importance of PE in aquatic systems based on the relatively few studies that have quantified this phenomenon in freshwater and marine ecosystems. Discrepancies could be attributed to a number of factors ranging from the type of ecosystem under study to purely experimental differences between studies, such as the “labile” priming agents used and the definition of “recalcitrant” primed OM. Priming of bulk sedimentary OM (C:N ∼10; Middelburg and Nieuwenhuize, 1998) by microalgal detritus as the priming agent has been previously reported in estuarine (van Nugteren et al., 2009) and in seagrass meadow sediments only when the priming agent used was the naturally predominant “labile” OM source i.e., seagrass detritus (Trevathan-Tackett et al., 2018). No clear evidence of PE on DOC degradation in lake waters of varying trophic states (oligotrophic to eutrophic) could be found under a suite of experimental conditions (Catalán et al., 2015) whereas low nutrient levels seemed to favor the occurrence of PE on leaf litter decomposition at the presence of a benthic diatom (Danger et al., 2013). Among studies that used the same methodology as here, i.e., addition of 13C-labeled terrigenous OM and unlabelled priming agents, Bengtsson et al. (2014) and Wagner et al. (2014) reported no PE in stream biofilms while Ward et al. (2016) measured positive PE in river water. Comparisons between ecosystems and methodologies is problematic, however, a meta-analysis of PE in aquatic studies showed only scarce potential for significant PE in aquatic systems; high heterogeneity across systems and within studies rendered the overall 12.6% positive PE insignificant (Bengtsson et al., 2018).
To conclude, this study focused on the occurrence of PEs during the degradation of terrestrial plant material after its deposition on coastal sediments due to the co-presence of easily degradable marine detritus. Two scenarios were tested in terms of priming inducer application; a single large pulse of diatoms emulating the deposition of phytodetritus following a phytoplankton bloom and the repeated addition of lower doses of diatom detritus emulating the daily algae deposition of pelagic or benthic origin. No strong evidence of priming on terrestrial plant detritus (13C-wheat) mineralization upon addition of marine OM could be detected in either scenario under the experimental conditions in this study. Low or insignificant PEs and high variability between replicates of the same treatment obstructed a solid conclusion on the occurrence of PEs in this case. Differences in macrofaunal abundance and bioturbation rate between cores could have contributed considerably to the observed heterogeneity. Higher numbers of replicate cores per treatment and greater core size should be considered in future experiments.
Author Contributions
EG and UW conceived the study. EG conducted the experiments and carried out data analysis. EG and UW co-wrote the manuscript.
Funding
EG was funded by the MASTS pooling initiative (The Marine Alliance for Science and Technology for Scotland). MASTS was funded by the Scottish Funding Council (Grant reference HR09011) and contributing institutions. EG is currently funded by the Hellenic Foundation for Research and Innovation (HFRI) (grant number 1874) and the General Secretariat for Research and Technology (GSRT). This study was funded by the MASTS Marine Biogeochemistry Forum small grants scheme and their support is gratefully acknowledged.
Conflict of Interest Statement
The authors declare that the research was conducted in the absence of any commercial or financial relationships that could be construed as a potential conflict of interest.
Acknowledgments
We thank Val Johnston for her assistance in sediment sampling in the Ythan estuary.
Supplementary Material
The Supplementary Material for this article can be found online at: https://www.frontiersin.org/articles/10.3389/fmars.2019.00327/full#supplementary-material
Footnotes
References
Baird, D., and Milne, H. (1981). Energy flow in the Ythan estuary, Aberdeenshire, Scotland. Estuar. Coast. Shelf Sci. 13, 455–472. doi: 10.1016/S0302-3524(81)80041-2
Bauer, J. E., Cai, W.-J., Raymond, P. A., Bianchi, T. S., Hopkinson, C. S., and Regnier, P. A. (2013). The changing carbon cycle of the coastal ocean. Nature 504, 61–70. doi: 10.1038/nature12857
Bengtsson, M. M., Attermeyer, K., and Catalán, N. (2018). Interactive effects on organic matter processing from soils to the ocean: are priming effects relevant in aquatic ecosystems? Hydrobiologia 822, 1–17. doi: 10.1007/s10750-018-3672-2
Bengtsson, M. M., Wagner, K., Burns, N. R., Herberg, E. R., Wanek, W., Kaplan, L. A., et al. (2014). No evidence of aquatic priming effects in hyporheic zone microcosms. Sci. Rep. 4:5187. doi: 10.1038/srep05187
Bianchi, T. S. (2011). The role of terrestrially derived organic carbon in the coastal ocean: a changing paradigm and the priming effect. Proc. Natl. Acad. Sci. U.S.A. 108, 19473–19481. doi: 10.1073/pnas.1017982108
Bianchi, T. S., Cui, X., Blair, N. E., Burdige, D. J., Eglinton, T. I., and Galy, V. (2018). Centers of organic carbon burial and oxidation at the land-ocean interface. Org. Geochem. 115, 138–155. doi: 10.1016/j.orggeochem.2017.09.008
Bianchi, T. S., Thornton, D. C. O., Yvon-Lewis, S. A., King, G. M., Eglinton, T. I., Shields, M. R., et al. (2015). Positive priming of terrestrially derived dissolved organic matter in a freshwater microcosm system. Geophys. Res. Lett. 42, 5460–5467. doi: 10.1002/2015GL064765
Billen, G., Joiris, C., Meyer-Reil, L., and Linderboom, H. (1990). Role of bacteria in the north sea ecosystem. Netherlands J. Sea Res. 26, 265–293. doi: 10.1016/0077-7579(90)90093-V
Blair, N. E., and Aller, R. C. (2012). The fate of terrestrial organic carbon in the marine environment. Ann. Rev. Mar. Sci. 4, 401–423. doi: 10.1146/annurev-marine-120709-142717
Burdige, D. J. (2005). Burial of terrestrial organic matter in marine sediments: a re-assessment. Glob. Biogeochem. Cycles 19, 1–7. doi: 10.1029/2004GB002368
Cai, W.-J. (2011). Estuarine and coastal ocean carbon paradox: CO2 sinks or sites of terrestrial carbon incineration? Ann. Rev. Mar. Sci. 3, 123–145. doi: 10.1146/annurev-marine-120709-142723
Catalán, N., Kellerman, A. M., Peter, H., Carmona, F., and Tranvik, L. J. (2015). Absence of a priming effect on dissolved organic carbon degradation in lake water. Limnol. Oceanogr. 60, 159–168. doi: 10.1002/lno.10016
Danger, M., Cornut, J., Chauvet, E., Chavez, P., Elger, A., and Lecerf, A. (2013). Benthic algae stimulate leaf litter decomposition in detritus-based headwater streams: a case of aquatic priming effect? Ecology 94, 1604–1613. doi: 10.1890/12-0606.1
Gontikaki, E., Thornton, B., Cornulier, T., and Witte, U. (2015). Occurrence of priming in the degradation of lignocellulose in marine sediments. PLoS One 10:e0143917. doi: 10.1371/journal.pone.0143917
Gontikaki, E., Thornton, B., Huvenne, V. A. I., and Witte, U. (2013). Negative priming effect on organic matter mineralisation in NE Atlantic slope sediments. PLoS One 8:e67722. doi: 10.1371/journal.pone.0067722
Gooday, A. J. (2002). Biological responses to seasonally varying fluxes of organic matter to the ocean floor: a review. J. Oceanogr. 58, 305–332. doi: 10.1023/A:1015865826379
Guenet, B., Danger, M., Abbadie, L., and Lacroix, G. (2010). Priming effect: bridging the gap between terrestrial and aquatic ecology. Ecology 91, 2850–2861. doi: 10.1890/09-1968.1
Hedges, J. I., Keil, R. G., and Benner, R. (1997). What happens to terrestrial organic matter in the ocean? Org. Geochem. 27, 195–212. doi: 10.1016/S0146-6380(97)00066-1
Kuzyakov, Y. (2002). Review: factors affecting rhizosphere priming effects. J. Plant Nutr. Soil Sci. 165, 382–396.
Kuzyakov, Y. (2010). Priming effects: interactions between living and dead organic matter. Soil Biol. Biochem. 42, 1363–1371. doi: 10.1016/j.soilbio.2010.04.003
Middelburg, J. J., and Nieuwenhuize, J. (1998). Carbon and nitrogen stable isotopes in suspended matter and sediments from the Schelde Estuary. Mar. Chem. 60, 217–225. doi: 10.1016/S0304-4203(97)00104-7
Nottingham, A. T., Griffiths, H., Chamberlain, P. M., Stott, A. W., and Tanner, E. V. J. (2009). Soil priming by sugar and leaf-litter substrates: a link to microbial groups. Appl. Soil Ecol. 42, 183–190. doi: 10.1016/j.apsoil.2009.03.003
Pinheiro, J., Bates, D., DebRoy, S., Sarkar, D., and R Core Team (2018). nlme: Linear and Nonlinear Mixed Effects Models. R Package Version 3.1–137.
R Core Team (2018). R: A Language and Environment for Statistical Computing. Vienna: R Foundation for Statistical Computing. Available at: https://www.r-project.org/ (accessed May 6, 2019).
Raffaelli, D. (1999). Nutrient enrichment and trophic organisation in an estuarine food web. Acta Oecologica. 20, 449–461. doi: 10.1016/S1146-609X(99)00123-X
Trevathan-Tackett, S. M., Thomson, A. C. G., Ralph, P. J., and Macreadie, P. I. (2018). Fresh carbon inputs to seagrass sediments induce variable microbial priming responses. Sci. Total Environ. 621, 663–669. doi: 10.1016/j.scitotenv.2017.11.193
van Nugteren, P., Moodley, L., Brummer, G. J., Heip, C. H. R., Herman, P. M. J., and Middelburg, J. J. (2009). Seafloor ecosystem functioning: the importance of organic matter priming. Mar. Biol. 156, 2277–2287. doi: 10.1007/s00227-009-1255-5
Wagner, K., Bengtsson, M. M., Besemer, K., Sieczko, A., Burns, N. R., Herberg, E. R., et al. (2014). Functional and structural responses of hyporheic biofilms to varying sources of dissolved organic matter. Appl. Environ. Microbiol. 80, 6004–6012. doi: 10.1128/AEM.01128-14
Ward, N. D., Bianchi, T. S., Sawakuchi, H. O., Gagne-Maynard, W., Cunha, A. C., Brito, D. C., et al. (2016). The reactivity of plant-derived organic matter and the potential importance of priming effects along the lower Amazon River. J. Geophys. Res. Biogeosci. 121, 1522–1539. doi: 10.1002/2016JG003342
Keywords: priming effects, aquatic, marine, sediment, terrestrial organic matter, degradation, bacteria, stable isotopes
Citation: Gontikaki E and Witte U (2019) No Strong Evidence of Priming Effects on the Degradation of Terrestrial Plant Detritus in Estuarine Sediments. Front. Mar. Sci. 6:327. doi: 10.3389/fmars.2019.00327
Received: 25 January 2019; Accepted: 28 May 2019;
Published: 14 June 2019.
Edited by:
Thomas S. Bianchi, University of Florida, United StatesReviewed by:
Christophe Rabouille, UMR8212 Laboratoire des Sciences du Climat et de l’Environnement (LSCE), FranceTomoko Komada, San Francisco State University, United States
Copyright © 2019 Gontikaki and Witte. This is an open-access article distributed under the terms of the Creative Commons Attribution License (CC BY). The use, distribution or reproduction in other forums is permitted, provided the original author(s) and the copyright owner(s) are credited and that the original publication in this journal is cited, in accordance with accepted academic practice. No use, distribution or reproduction is permitted which does not comply with these terms.
*Correspondence: Evangelia Gontikaki, ZWdvbnRpa2FraUBpc2MudHVjLmdy, ZXZpbmEuZ29udGlrYWtpQGdtYWlsLmNvbQ==; Ursula Witte, dXdpdHRlQGFiZG4uYWMudWs=