- 1School of Ocean Science and Engineering, The University of Southern Mississippi, Ocean Springs, MS, United States
- 2Department of Earth and Environmental Sciences, Wright State University, Dayton, OH, United States
Lacustrine and coastal systems are vulnerable to the increasing number and intensity of tropical storms driven by climate change. Strong winds associated with tropical storms can mobilize nutrients in sediments and alter nitrogen and phosphorus cycling, leading to amplification of preexisting conditions, such as eutrophication and cyanobacterial blooms (cyanoHABs). In 2016, Florida declared a State of Emergency within and downstream of Lake Okeechobee (LO) due to toxic cyanobacterial blooms (primarily Microcystis). The blooms originated in LO, but flood control measures released water from LO to the brackish St. Lucie Estuary (SLE). In September 2017, Hurricane Irma traversed the Florida peninsula with sustained winds exceeding 160 km h–1, generating torrential rains over the watershed. We quantified ammonium () regeneration and potential uptake rates, and Microcystis toxin gene (mcyD) abundance in LO and SLE during the massive bloom in July 2016, the bloom in August 2017 (2 weeks before Irma), and 10 days after Hurricane Irma landfall. In 2016, cyanoHABs were present in both LO and SLE, and potential uptake rates were high in both systems. In 2017, the bloom was constrained to LO, potential uptake rates in LO exceeded those in SLE, and mcyD gene abundance was greater in LO than SLE. Post Hurricane Irma, potential uptake rates decreased significantly in LO and SLE, while mcyD gene abundance decreased in LO and increased slightly in SLE. Average regeneration rates could support 25–40% of water column potential demand in the lake and, when extrapolated to the entire LO water column, exceeded external nitrogen loading. These results emphasize the importance of internal recycling for bloom expansion and toxicity in the lake and downstream estuaries. In 2018, the cyanobacterial bloom in the Okeechobee region was one of the largest recorded and is presumed to be driven by the aftermath of Hurricane Irma. Large-scale blooms have also been observed in SLE, likely due to LO flushing and decreased salinity post-hurricane. Thus, results from this study support predictions that increased frequency and strength of tropical storms will lead to more intense blooms in aquatic systems.
Introduction
Anthropogenically-driven climate change has major effects on aquatic systems globally. Additionally, anthropogenic alterations to the nitrogen (N) cycle via synthetic fertilizer production (Haber–Bosch process) have quadrupled the amounts of chemically reduced N in the last 60 years (Erisman et al., 2015). Together, increased N runoff and climate change contribute to eutrophication and cyanobacterial harmful algal blooms (cyanoHABs; Paerl et al., 2016; Glibert, 2017) in freshwater and coastal systems. Internal loading of N and phosphorus (P) from sediments and recycling processes within the water column can enhance cyanoHABs (Havens et al., 2001a; McCarthy et al., 2007, 2016), especially in shallow lakes (Havens et al., 2001a; Moss et al., 2003; Jeppesen et al., 2007; James et al., 2009). However, external nutrient loading from non-point agricultural runoff and point sources is the major driver of internal nutrient loading/recycling and cyanoHABs in eutrophic lakes (Huisman et al., 2018).
Lake Okeechobee in southern Florida is a large (1800 km2), shallow lake (mean depth = 2.7 m) and has experienced cyanoHABs for decades (Havens et al., 1994). Lake water levels are closely monitored and managed by the US Army Corps of Engineers. These activities allow the lake to fill in the winter dry season, supplying water for agricultural and urban uses, and lake water is released in spring to increase storage capacity for the summer wet season (Julian and Osborne, 2018). The St. Lucie Estuary (SLE) is subjected to high freshwater and nutrient inputs from Lake Okeechobee through the South Fork of the estuary and Indian River Lagoon. Lake Okeechobee and the SLE receive high external N and P loads from agricultural runoff and anthropogenic activities (James et al., 2011; Phlips et al., 2012), and recent toxic cyanoHABs have been well-documented in both systems (e.g., Phlips et al., 2012; Kramer et al., 2018). In 2016, a large, toxic cyanobacterial bloom occurred in Lake Okeechobee and SLE, leading to a State of Emergency declaration in Florida (Kramer et al., 2018). Increased rainfall in 2016, corresponding with an El Niño event, led to increased N concentrations in the water column and decreased salinity in the estuary, conditions ideal for development of a Microcystis bloom (Kramer et al., 2018). Environmental degradation in SLE (e.g., cyanoHABs, fecal bacteria, degradation of nearshore reefs) has also been attributed to on-site sewage disposal systems (septic tanks; Lapointe et al., 2012, 2017), which have led to nutrient enrichment and microbial contamination (Lapointe et al., 2017).
Cyanobacteria community structure in Lake Okeechobee has shifted from N-fixing to non-N fixing taxa (Microcystis) in recent decades (Havens et al., 2003). Eutrophication management efforts in Lake Okeechobee included 40% reductions of P loading and reducing back-pumping from the Everglades Agricultural Area (EAA; James et al., 2011). The Okeechobee Basin Management Action Plan (BMAP) was also implemented in 2014 to reduce daily loads for total P (Zhang et al., 2016), and these approaches will likely also reduce N loads. However, non-point source pollution remains a problem in the Lake Okeechobee watershed, and concerns about water-level and flood control management activities persist (Kramer et al., 2018).
Microcystis is a potential toxin-producing cyanobacterial genus and strong competitor for chemically reduced forms of N (Blomqvist et al., 1994; Yang et al., 2017; Hampel et al., 2018), such as ammonium () and urea. Microcystin (MC), synthesized by Microcystis and other cyanobacteria, is a potent hepatotoxin and is N-rich (10 N atoms per molecule; Gobler et al., 2016). In fact, reduced forms of N can yield higher production of MC (Monchamp et al., 2014; Davis et al., 2015; Harke and Gobler, 2015). Ammonium is the preferred source of N for most primary producers, including non-N-fixing cyanobacteria (Blomqvist et al., 1994; Beversdorf et al., 2015). Thus, internal cycling of is of particular importance in lakes affected by non-diazotrophic cyanoHABs, and internal regeneration often sustains late summer blooms (Paerl et al., 2011; McCarthy et al., 2013; Hampel et al., 2019). turnover rates in Lake Okeechobee are rapid, while ambient concentrations are generally low, suggesting that is in high demand (McCarthy et al., 2009; James et al., 2011). Large discrepancies have been observed between inflow and outflow N in previous mass balance studies for Lake Okeechobee (Havens et al., 2001a), suggesting that internal N cycling processes play a critical role in supporting primary productivity and cyanoHABs (James et al., 2011).
Current climate change models forecast increased severity of extreme weather events, including hurricanes and typhoons (Bender et al., 2010; Knutson et al., 2010; Paerl et al., 2018). Hurricane events increase rainfall in affected areas, leading to higher runoff and nutrient concentrations (Havens et al., 2001b; James et al., 2008). Strong winds associated with tropical storms also enhance eutrophication by mobilizing nutrients in sediments via resuspension (Havens et al., 2001b; James et al., 2008; Ding et al., 2012). Previous studies in Lake Okeechobee reported doubled to quadrupled concentrations of total N (TN) and total phosphorus (TP), along with major increases in soluble reactive phosphorus (SRP), , and other forms of N, after hurricanes (James et al., 2008; Ding et al., 2012). Increased nutrient availability after these events leads to amplified productivity, biomass, and chlorophyll levels (Fogel et al., 1999; Paerl et al., 2001).
The objectives of this study were to (1) quantify water column dynamics during a severe cyanoHAB in 2016 that affected both Lake Okeechobee and the SLE and (2) compare those rates to those from 2017, when the cyanoHAB was restricted to Lake Okeechobee. We also aimed to (3) quantify these rates within 2 weeks after a major hurricane in September 2017 to examine post-hurricane cycling rates in both the lake and estuary. Finally, we (4) quantified MC synthetase gene (mcyD) abundance before and after the hurricane to investigate the effects of hurricane passage on MC producing cyanobacteria. We hypothesized that cycling rates in Lake Okeechobee would be higher during the large bloom in 2016 than in 2017 during the smaller bloom. We also hypothesized that uptake and regeneration rates in the estuary in 2016 would decrease along the salinity gradient and be higher than in 2017. Lastly, we anticipated that the hurricane would have a major effect on cycling and Microcystis in both systems, and that regeneration rates would increase post-disturbance, while uptake rates and abundance of MC synthetase would decrease.
Materials and Methods
Sample Collection
Lake Okeechobee (LO) and SLE water samples were collected on three occasions: July 25–27, 2016; August 22–24, 2017; and September 20–21, 2017. Sampling in July 2016 followed a major cyanobacterial bloom in LO that extended into SLE and reached the Atlantic Ocean (Kramer et al., 2018). The August 2017 sampling occurred shortly before Hurricane Irma passed over the lake as a Category 3 hurricane (sustained winds 178–208 km h–1 at landfall near Marco Island) on September 10. The September 2017 sampling occurred 10 days after Hurricane Irma passed through central Florida and the Okeechobee region (sustained winds ∼40 mph on September 10, DBHydro, SFWMD).
In July 2016, sampling was conducted at two stations in LO and four stations in SLE: L004 at surface and bottom (∼3 m) water depths; LZ40 at surface and bottom (∼4 m) water depths; and SLE80, SLE2, SLE4, and SLE8 (all SLE sampling was surface water; Figure 1). Bottom water samples were collected about 0.5 m above the sediment-water interface. L004 is located in eastern LO, ∼8.5 km from the St. Lucie Canal, and LZ40 is in the center of the lake. Stations in SLE followed a salinity gradient (Table 2), with SLE80 closest to the canal discharge lock (S308; Figure 1), and SLE8 closest to the Atlantic Ocean. In August 2017, due to poor weather, only L004 (surface and bottom) and a northern LO station (SAV 165, surface water only) were sampled. Similarly, SLE stations (SLE7, SLE5, and SLE80) could only be sampled from land due to poor weather conditions. In September 2017, stations SLE5 and SLE7 were sampled in SLE, and stations L004 (surface), LZ40 (surface), and LOBG in southeastern LO were sampled (Figure 1).
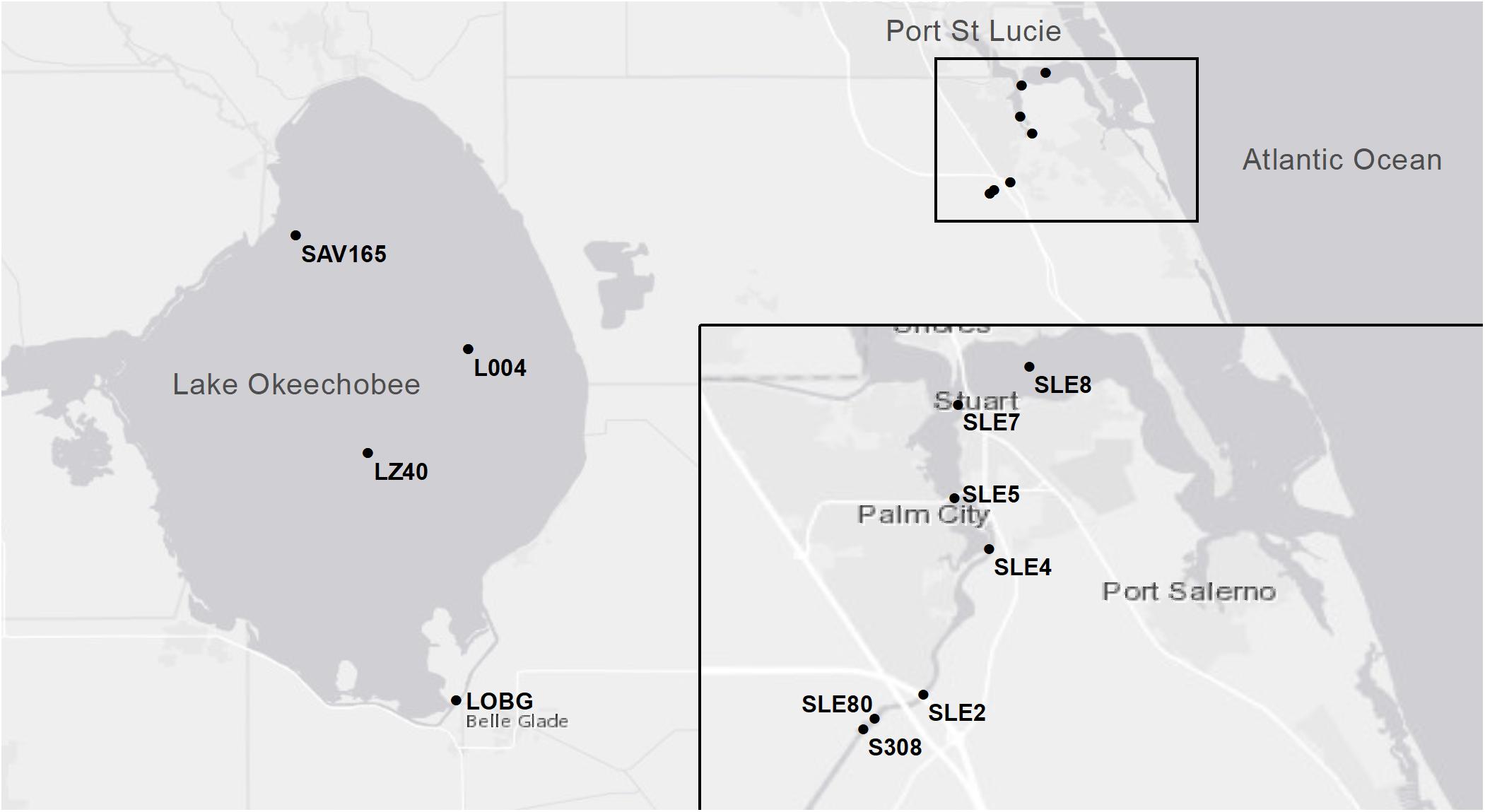
Figure 1. Map of sampling locations in Lake Okeechobee and St. Lucie Estuary during 2016 and 2017 sampling events. The inset shows the South Fork of St. Lucie Estuary.
Water for in situ nutrient analyses was filtered in the field using 0.2 μm syringe filters into 15 ml polypropylene tubes and frozen upon return to the laboratory. Geophysical parameters (temperature, dissolved oxygen, pH, salinity, and conductivity) were measured using a YSI multi-parameter sonde in July 2016 and August 2017 and a Manta 2 sonde (Eureka Waterprobes) in September 2017. Water for dynamics experiments was collected into pre-washed and sample-rinsed 6 L cubitainers and returned to the lab within 3 h for incubations.
Samples for chlorophyll a (Chl a), TN, and TP analyses were collected and analyzed by South Florida Water Management District (SFWMD) according to standard USEPA methods (as described in SFWMD, 2002). Dissolved nutrient analyses included , , , orthophosphate (OP), and urea and were analyzed using a Lachat Quikchem 8500 FIA nutrient analyzer according to the manufacturer’s instructions.
Regeneration and Potential Uptake
Water column regeneration and potential uptake experiments followed the protocol described in Hampel et al. (2018) with modifications for 15N analysis. Briefly, 1 L of water collected at each station was amended with 98% 15NH4Cl (Isotec; final concentration added: 16 μM for all stations except SAV165 = 32 μM). Amended water was mixed thoroughly and decanted into six, 125 ml clear polystyrene Nalgene bottles (triplicates for light and dark incubations). Initial samples were filtered (0.2 μm syringe filter) immediately after spiking (T0) into 15 ml clear, polypropylene tubes (for total ) and 12 ml gas-tight exetainers (for 15N analysis). Dark incubation bottles were wrapped in aluminum foil, and all samples were placed in a standard lab incubator (also used for algal cultures), at near-ambient temperature (29°C) and set to the appropriate diurnal light cycle, for 20 h. After incubation, final samples (Tf) were processed as described for the T0 samples and stored frozen until analysis. Total (14+15N) concentrations were determined using the Lachat Quikchem 8500 FIA nutrient analyzer, and concentrations were determined using the OX-MIMS method, combining membrane inlet mass spectrometry (MIMS; Kana et al., 1994) with reduction to N2 gas (Yin et al., 2014). Samples for OX-MIMS were treated with 200 μl of hypobromite iodine solution and immediately measured on the MIMS. concentrations were determined using the line equation from the standard curve (0.1–100 μM ) and total 15N2 production (Yin et al., 2014). Potential uptake and actual regeneration rates were calculated using the Blackburn/Caperon model (Blackburn, 1979; Caperon et al., 1979). Volumetric uptake rates (light and dark), but not regeneration rates (actual rates; Gardner et al., 2017), reported in this study are qualified as potential rates due to saturating additions of substrate, which can alter steady-state conditions (Glibert, 1988). However, in eutrophic systems, results from saturating- and tracer-level isotope additions tend to converge (Glibert, 1988).
DNA Collection and Extraction
Environmental DNA for gene abundance analysis was collected in August and September 2017 using 0.2 μm Sterivex filters (EMD Millipore, Burlington, MA, United States) and preserved with Ambion RNAlater (Invitrogen, Carlsbad, CA, United States). In August, approximately 120–240 ml of water was pushed through a Sterivex filter. However, due to highly turbid waters after the hurricane in September, only 45–60 ml of water were filtered for stations L004, LZ40, and SLE, and 300 ml for station LOBG. Preserved filters were frozen at −80°C. DNA was extracted using the Gentra PureGene kit (Qiagen, Inc., United States). Residual RNAlater in the Sterivex filters was removed by pushing 10 ml of Phosphate Buffer Saline 1X Solution (Fisher BioReagents, United States) through the filter. Lysis buffer (0.9 ml) and Proteinase K (10 μl) were added to the filters, followed by 1 h incubation at 55°C and 1 h incubation at 65°C (Newell et al., 2011). Concentration and purity of extracted DNA were measured spectrophotometrically (Nanodrop 2000, Thermo Scientific).
qPCR Analysis
The MC synthetase gene was amplified using the mcyD-F2 and mcyD-R2 primers, targeting a 298 bp region of the mcy operon (Kaebernick et al., 2000). qPCR standards were prepared by cloning the fragment of interest with the TOPO TA Cloning Kit (Invitrogen, United States) and inserting it into a competent cell (One Shot E. coli cells, Invitrogen, United States). The plasmid containing the mcyD gene was isolated using the UltraClean Standard Mini Plasmid Prep Kit (Mo Bio Laboratories, Inc., Carlsbad, CA, United States). The qPCR run included three negative controls (no template), triplicates of five standards generated from serial dilution, and the environmental DNA samples in triplicate. Each sample and standard received 10 μl of Luna qPCR Master Mix (New England Biolabs, United States), 1 μl of each 10 μM primer, and 20–30 ng of template DNA.
qPCR protocol followed a method modified from Davis et al. (2009) for mcyD (95°C initial denaturation for 2 min, 95°C denaturation for 15 s, 50°C annealing for 1 min, and 60°C extension for 1 min; 45 cycles) followed by the melting curve. Automatic settings for the thermocycler (Realplex, Eppendorf) were used to determine the threshold cycle (Ct values), efficiency (98%), and a standard curve with R2 values of 0.99. mcyD gene copies were calculated as (ng ∗ number mol–1)/(bp ∗ ng g–1 ∗ g mol–1 of bp) and is reported in gene copies ml–1 of sample water.
Statistical Analysis
All statistical analyses were performed using RStudio software (version 1.1.383). Environmental data were checked for normality using the Shapiro–Wilk normality test. After ensuring that the data were not normally distributed, the Kruskal–Wallis test for non-parametric data was used to determine significant differences between sites and time points. The Kendall correlation method for non-parametric data was used between environmental variables and geochemical rates and mcyD gene abundance. Kendall’s p-values are usually more accurate for datasets with small sample sizes than Spearman’s (Croux and Dehon, 2010). The correlation matrix presented below was constructed showing the Kendall’s tau correlation coefficient and p-values (p < 0.05 was considered statistically significant).
Results
Environmental Data
Lake Okeechobee
Environmental variables in LO varied between sampling events, with only temperature remaining stable (Table 1). DO was highest in July 2016 (10.9 ± 1.05 mg L–1; mean ± standard error) and lowest following the hurricane in September 2017 (5.5 ± 1.5 mg L–1). Similarly, pH was highest before the hurricane and decreased afterward. Chl a was also higher during the August 2017 bloom (55.6 ± 22.2 μg L–1) than during the July 2016 bloom (26.2 ± 0.9 μg L–1), and lowest values were observed post-Irma in September 2017 (13.8 ± 5.13 μg L–1). Conductivity was similar in July 2016 and August 2017 (372 ± 11.5 and 370 ± 2.00 μs cm–1, respectively) and increased in September 2017 (451 ± 64.7 μs cm–1).
In July 2016, ambient concentrations in the east–central lake (L004; 7.22 ± 0.53 μM) were significantly greater than in the lake center (LZ40; 0.47 ± 0.36 μM; p < 0.05). In September 2017, concentrations were also significantly greater at the shore station (LOBG; 19.1 μM) than at L004 (0.1 μM; p < 0.05). concentrations were not statistically different between sampling events and ranged from 0.06 to 1.15 μM. Ambient concentrations were similar in July 2016 (1.01 ± 0.31 μM) and August 2017 (1.9 ± 0.16 μM) and significantly increased after the hurricane (32.2 ± 10.8 μM). Similarly, OP and urea concentrations both increased after the hurricane (2.24 ± 1.34 and 3.22 ± 0.71 μM, respectively).
St. Lucie Estuary
Water temperatures in SLE also were consistent between sampling events (29.4–31.1°C; Table 2). Salinity in July 2016 increased from SLE80 (closest to the canal; 0.19) to SLE8 (closest to the Atlantic Ocean; 8.9), and the same general pattern was observed in August 2017, with lower salinity at SLE5 (4.6) than SLE7 (6.75). However, after the hurricane, salinity decreased significantly to 0.19 (SLE5) and 0.22 (SLE7; p < 0.05). Similar to LO, highest conductivity was observed in September 2017 and was significantly different from other sampling events (436 ± 30.5 μs cm–1; p < 0.001). Highest mean Chl a values were recorded in August 2017 (18 ± 1.9 μg L–1).
Ambient concentrations were highest in August 2017 (9.13 ± 1.52 μM) and decreased after the hurricane (1.23 ± 0.98 μM; Table 2). Similarly, concentrations were higher before the hurricane (August 2017: 3.15 ± 1.40 μM) and decreased afterward (September 2017: 0.71 ± 0.37 μM). In contrast, concentrations in SLE increased post-Irma (September: 34.9 ± 0.35 μM). OP and urea showed an opposite pattern than in LO and decreased after the hurricane (Table 2).
Potential Ammonium Uptake
Lake Okeechobee
As mentioned previously, light and dark uptake rates are qualified as potential rates because of added substrate. However, regeneration rates are considered actual rates because they represent turnover (Gardner et al., 2017). In July 2016, potential uptake rates in the light ranged from 0.54 to 2.24 μM h–1, and peak light uptake rates were observed at L004 in surface water (Figure 2A). Light uptake rates in August 2017 (mean = 2.88 ± 0.72 μM h–1; Figure 2B) were greater than in July 2016, with peak rates in the central–eastern part of the lake (L004; Figure 2B). After the hurricane (Figure 2C), light uptake rates significantly decreased (mean = 0.66 ± 0.27 μM h–1; p < 0.05) and showed spatial variability; highest light uptake was observed at the shore station (LOBG) and lowest in the central lake (LZ40).
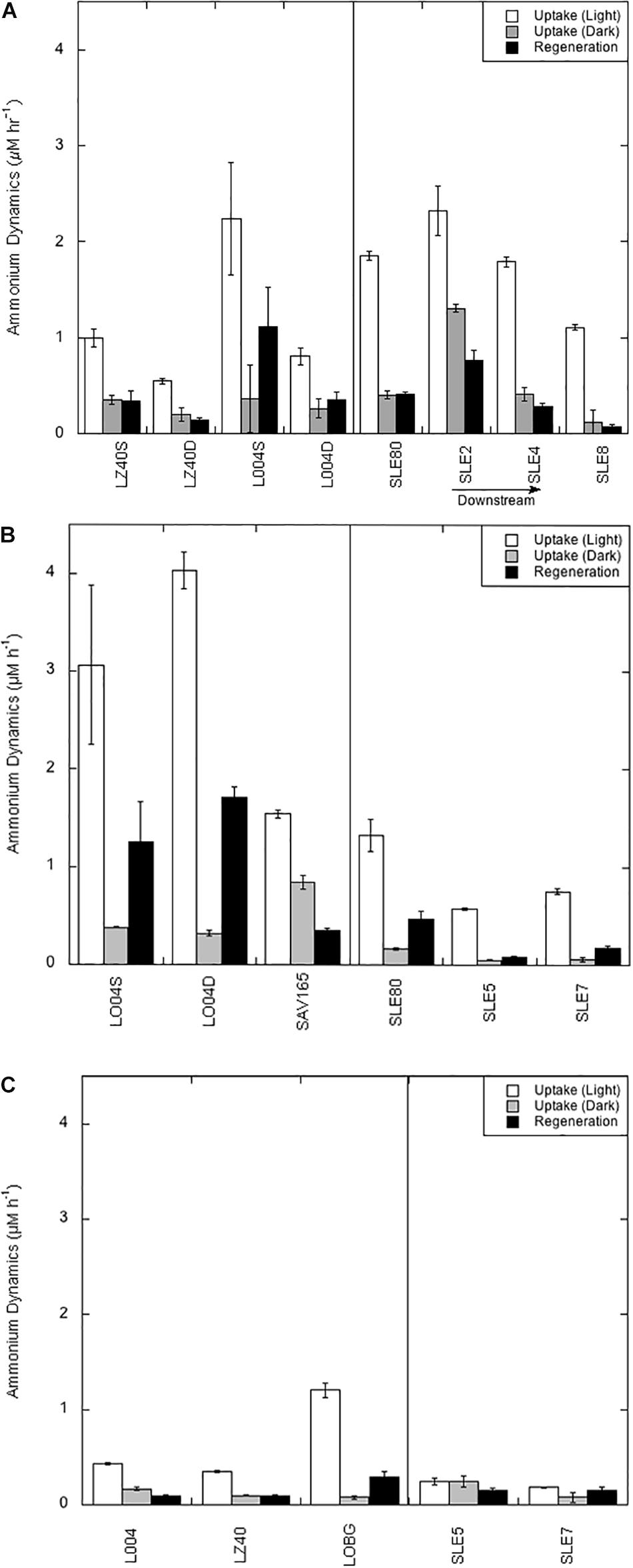
Figure 2. Ammonium regeneration and potential uptake rates in Lake Okeechobee and St. Lucie Estuary in July 2016 (A), August 2017 (B), and September 2017 (C). Values are averaged (three replicates) with error bars showing ± one standard error. Vertical line divides stations in the lake from stations in the estuary. S = surface water; D = bottom water.
Dark uptake rates were significantly lower than light rates on all occasions (p < 0.001). In July 2016, dark uptake rates were comparable between stations and depths (mean = 0.30 ± 0.05 μM h–1; Figure 2A). Dark rates in August 2017 were not statistically different from the July 2016 rates. However, dark uptake rates in September 2017 were significantly lower than in July 2016 (mean = 0.12 ± 0.02 μM h–1; Figure 2C), with highest dark uptake in the central–eastern lake (L004; Figure 2C) and lowest at the shore station (LOBG).
St. Lucie Estuary
Light uptake rates in the estuary were significantly different between sampling events and years (p < 0.05). Mean light uptake rates in the estuary were highest in July 2016 (mean = 1.76 ± 0.25 μM h–1), with highest rates close to the canal (SLE80 and SLE2) and decreasing toward the ocean (Figure 2A). In August 2017, light uptake rates were significantly lower (mean 0.89 ± 0.22 μM h–1; p < 0.05) but followed the same pattern, with peak uptake rates at the lock (SLE80) and decreasing at SLE5 and SLE7 (Figure 2B). After the hurricane, light uptake in the estuary decreased even further and was similar between stations SLE5 and SLE7 (mean = 0.22 ± 0.03 μM h–1; Figure 2C).
Dark uptake rates in the estuary were not statistically different between sampling events but were significantly lower than the light uptake rates (p < 0.001). In July 2016, dark rates in the estuary ranged from 0.12 to 1.31 μM h–1, with highest dark uptake near the lock at SLE2 and lowest further down the salinity gradient (SLE8; Figure 2A). In August 2017, dark uptake rates were lower than in 2016 (mean = 0.09 ± 0.03 μM h–1) but followed a similar pattern, with peak rates observed at SLE80 (Figure 2B). In contrast to light uptake rates, dark uptake rates in the estuary in September 2017 were slightly greater than before the hurricane, but not statistically different (0.16 ± 0.08 μM h–1; Figure 2C).
Light uptake rates in LO and SLE were positively correlated with ambient temperature and negatively correlated with , TN, and TP concentrations (Table 3). Dark uptake rates were positively correlated with DO concentration and mcyD abundance and negatively correlated with , OP, urea, and TP concentrations (Table 3).
Ammonium Regeneration
Lake Okeechobee
regeneration rates in LO (averaged light and dark rates) were not statistically different between July 2016 and August 2017 sampling events. Mean regeneration in July 2016 was 0.49 ± 0.22 μM h–1, and highest regeneration rates were observed in the east-central part of the lake (L004; Figure 2A). In August 2017, regeneration rates were higher than in July 2016 (mean = 1.10 ± 0.39 μM h–1), with highest rates at L004 (surface: 1.25 ± 0.40 μM h–1; deep: 1.70 ± 0.11 μM h–1) and lowest at SAV165 in surface water (0.35 ± 0.03 μM h–1). Following the hurricane, regeneration rates decreased significantly (mean = 0.16 ± 0.06 μM h–1; p < 0.05; Figure 2C), with very low values in the central and east-central lake (L004 and LZ40) and slightly higher rates nearshore (LOBG).
St. Lucie Estuary
regeneration rates in the estuary were not statistically different between sampling events, and peak regeneration rates were observed in July 2016 (mean = 0.38 ± 0.15 μM h–1; Figure 2A). regeneration rates followed the same pattern as uptake rates in July 2016, with highest values observed at sites closest to the canal (SLE2 and SLE80) and decreasing toward the Atlantic Ocean (SLE8). In August 2017, regeneration rates at the lock (SLE80; 0.46 ± 0.08 μM h–1) were similar to 2016 regeneration rates and decreased slightly along the salinity gradient (SLE5 and SLE7; Figure 2B). After the hurricane, regeneration rates at SLE5 and SLE7 (mean = 0.16 ± 0.01 μM h–1) remained similar to rates in August 2017. Ammonium regeneration rates in LO and SLE were positively correlated with temperature and Chl a and negatively correlated with salinity, , and TP concentrations (Table 3).
mcyD Abundance
Lake Okeechobee
Average abundance of the mcyD gene in August 2017 was 5.08 ± 1.45 × 106 copies ml–1 (Figure 3A), with highest mcyD gene abundance at L004 in bottom water (7.88 × 106 copies ml–1) and lowest at L004 in surface water (3.02 × 106 copies ml–1). mcyD abundance decreased after the hurricane (Figure 3B) and was significantly lower than in August (mean = 8.17 ± 6.36 × 104 copies ml–1; p < 0.05). After the hurricane, highest and lowest abundances of the mcyD gene were observed near shore at LOBG and in the east-central region (L004; Figure 3B), respectively. mcyD gene abundance was negatively correlated with and TP concentrations (Table 3; p < 0.05).
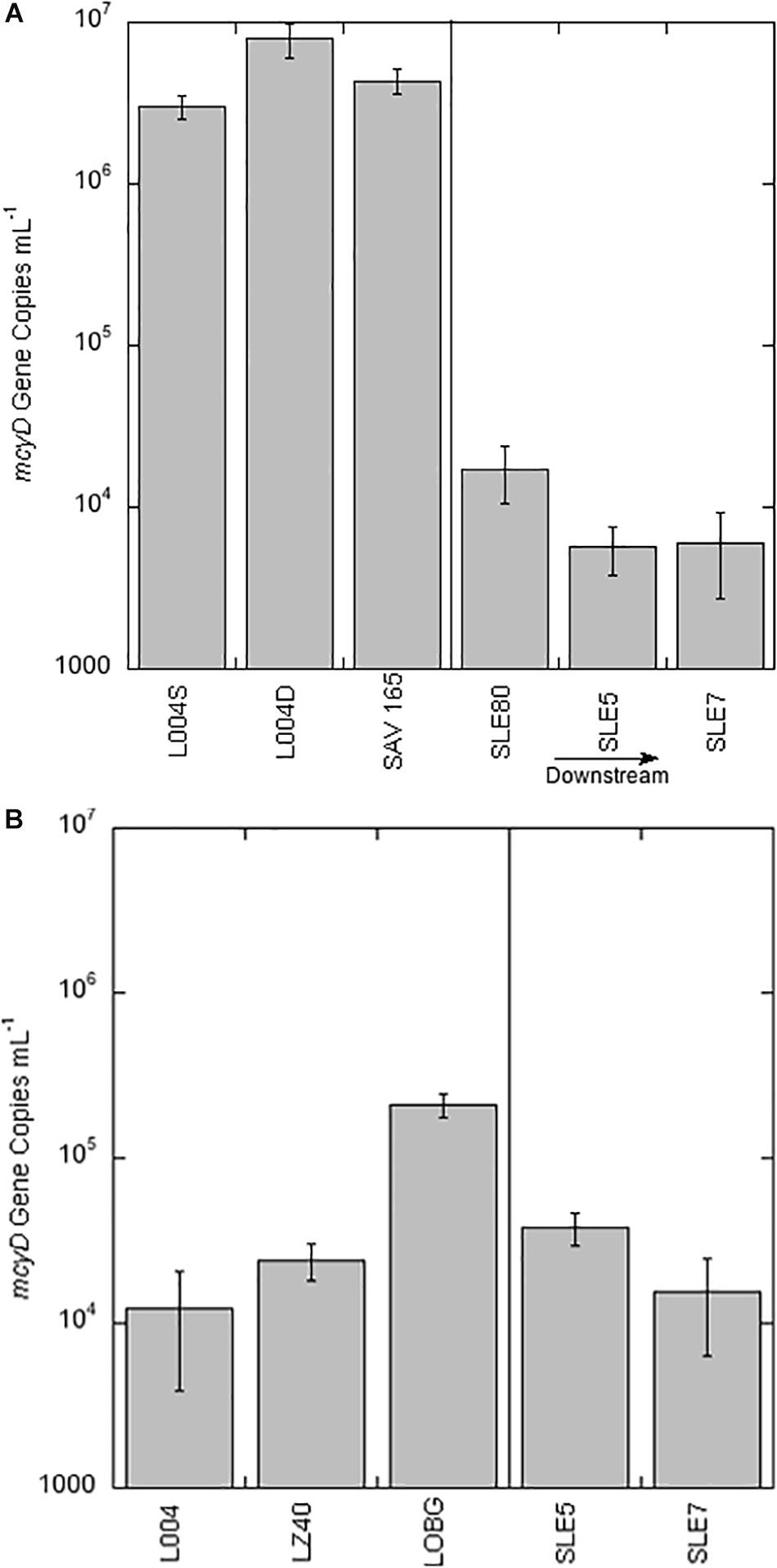
Figure 3. mcyD gene abundance ± one standard deviation in Lake Okeechobee and St. Lucie Estuary in August 2017 (A) and September 2017 (B). Vertical line divides stations in the lake from stations in the estuary. S = surface water; D = bottom water.
St. Lucie Estuary
Abundance of mcyD in SLE in August 2017 (mean = 9.67 ± 3.82 × 103 copies ml–1) was significantly lower than in LO (Figure 3A; p < 0.05). Highest abundance of mcyD in August in SLE was observed at SLE80 near the canal, while lowest abundance was observed further along the salinity gradient at SLE5 (Figure 3A). After the hurricane, mcyD abundance in SLE (mean = 2.69 ± 1.14 × 104 copies ml–1; Figure 3B) was slightly greater than in August, but not statistically different (p > 0.05).
Discussion
Uptake During 2016 and 2017 Microcystis Blooms
Increased rainfall in 2016, an El Niño year, led to increased water levels in LO, requiring the release of billions of gallons of lake water through the St. Lucie Canal into SLE (Figure 4A; Kramer et al., 2018). Release of high nutrient freshwater into the brackish estuary created ideal conditions for a Microcystis bloom to develop (Kramer et al., 2018). In 2017, the net flow of water from LO and SLE was lower until Hurricane Irma made landfall in September (Figure 4B). We compared cycling rates in LO and SLE during two summer blooms and 10 days after Hurricane Irma disturbance.
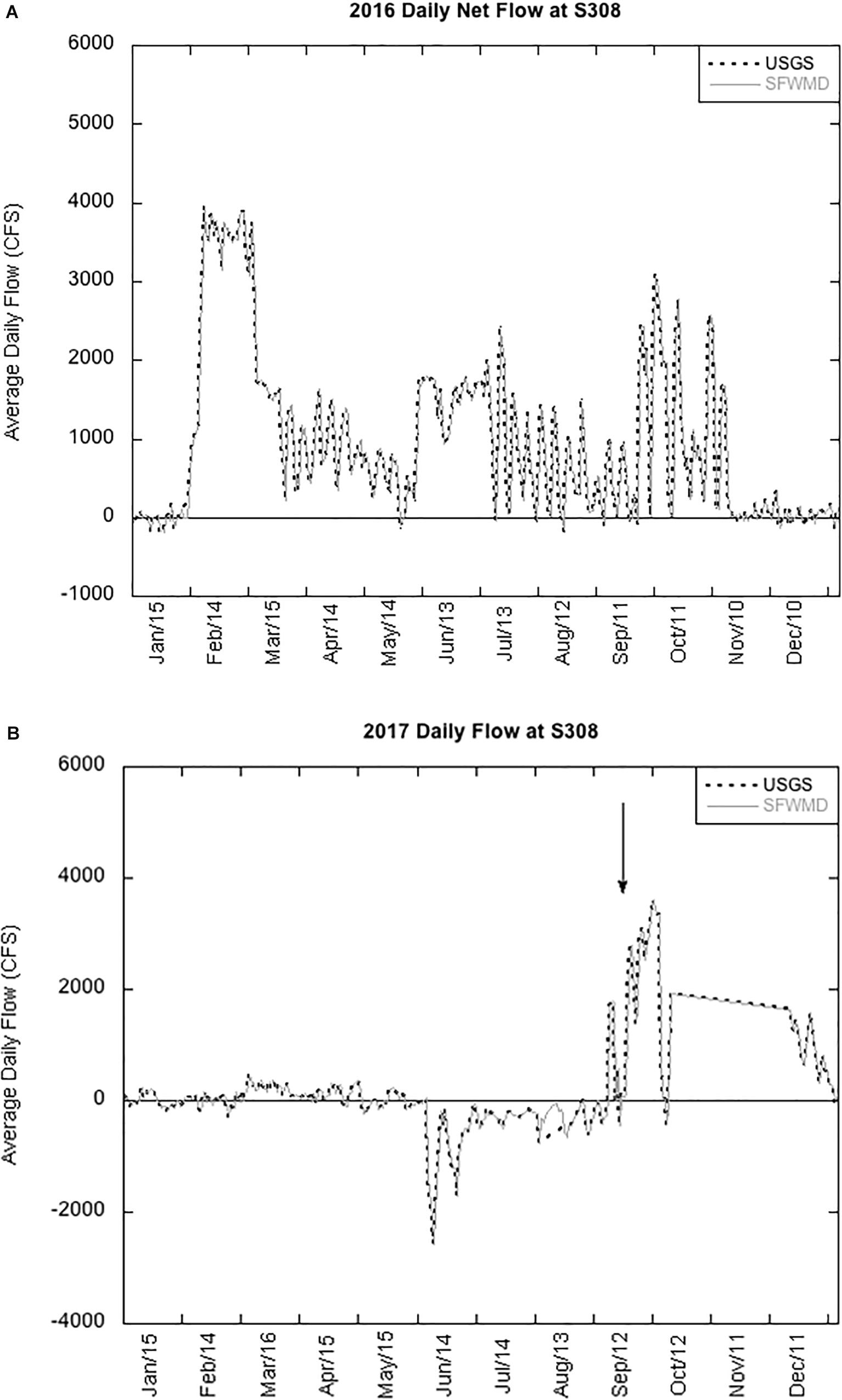
Figure 4. Daily flow rates at the S308 lock between Lake Okeechobee and St. Lucie Estuary in 2016 (A) and 2017 (B) measured by U.S. Geological Survey (USGS) and South Florida Management District (SFWMD). The arrow points to increased flow during the Hurricane Irma disturbance.
Potential uptake rates measured in LO and SLE were on par with rates reported in other eutrophic and hypereutrophic ecosystems (McCarthy et al., 2007; Paerl et al., 2011; Gardner et al., 2017; Hampel et al., 2018), but higher than those previously reported in LO (0.58 ± 0.01 μM h–1, James et al., 2011; 0.67 ± 0.15 μM h–1, Gu et al., 1997). uptake values in this study varied significantly based on bloom intensity and water flow (Figure 2). Peak bloom uptake rates in LO (August 2017, mean = 2.88 ± 1.25 μM h–1) were similar to rates reported during the annual Planktothrix bloom in Sandusky Bay, Lake Erie (Hampel et al., 2019), Microcystis blooms in hypereutrophic Lake Taihu (Paerl et al., 2011; Hampel et al., 2018), and in hypereutrophic Lake Maracaibo, Venezuela (Gardner et al., 1998).
In July 2016 (El Niño year), potential uptake rates were comparable between the lake and the estuary (Figure 2A). Bloom conditions were present in both systems, with high abundances of Microcystis (95% of algal biomass) and high MC and Chl a concentrations (Kramer et al., 2018). In the estuary, potential uptake decreased following a gradient from the lock (SLE80, SLE2) into the saline part of the estuary (SLE8; Figure 3A). This eastward decrease in dynamics corresponded with decreasing Chl a values in this study (Table 1), as well as decreased Chl a and MC concentrations and mcyE abundance reported previously (Kramer et al., 2018). Light uptake rates exceeded dark uptake rates in both systems, suggesting that demand was dominated by photoautotrophs.
In contrast, without water releases from LO in 2017 (Figure 4B), potential uptake rates in the lake were significantly higher than in the estuary (Figure 2B). However, uptake rates, Chl a concentrations, and mcyD abundances (Figure 3A) in SLE followed a similar pattern as in 2016, with highest values upstream (SLE80) and decreasing toward the coast. These results reflect the influence of water releases for flood control and support previous work showing that flood prevention practices in the Okeechobee region drive cyanoHABs in the downstream estuary, even when it may be beneficial for the trophic status of the lake (Lapointe et al., 2012; Phlips et al., 2012; Julian and Osborne, 2018).
Interestingly, mcyD abundance showed a strong, positive correlation with dark uptake rates. Microcystis has been shown to synthesize MC at night (Penn et al., 2014; Davenport et al., 2019), suggesting that MC has a role in Microcystis diurnal metabolism. Dark uptake rates observed here may be involved in MC production, but night time MC synthesis has not been extensively studied and merits future research, including diurnal transcriptomics and gene expression experiments.
Hurricane Irma
After Hurricane Irma, potential uptake rates in both systems significantly decreased (Figure 2C). Uptake rates at pelagic stations (L004 and LZ40) were the lowest measured in LO in this study (<0.5 μM h–1) and suggest decreased algal biomass and activity. In contrast, uptake rates close to the shore (LOBG) were significantly higher than in the central lake (1.2 ± 0.07 μM h–1), despite high ambient concentrations (Table 1). Algal biomass may have accumulated along shorelines, driven by winds and seiches. Post-hurricane abundance of mcyD in LO also decreased significantly, and mcyD was most abundant at the shoreline station (LOBG, Figure 3B), where peak uptake rates were also recorded (Figure 2C).
uptake rates and Chl a concentrations in SLE after the hurricane were significantly lower, but mcyD abundance remained unchanged (Figure 3B). Following Hurricane Wilma in 2005, the phytoplankton community in LO shifted toward diatoms (James et al., 2008). Under poor light conditions, such as high turbidity and low water column stability, diatoms may have outcompeted cyanobacteria (James et al., 2008). Indeed, turbidity and total suspended solids (TSSs) increased significantly after the hurricane in both LO and SLE (Supplementary Table 1). Cyanobacteria are also susceptible to flushing events (Paerl et al., 2018), and rapid flushing can also explain the lower Chl a concentrations in SLE (Table 2; Paerl et al., 2018). Net water flow at S308 increased after Hurricane Irma and remained high for the rest of the year (Figure 4B).
These results represent the short-term (10 days) response of a HAB to a major storm disturbance. Numerous other studies have reported long-term responses of cyanobacteria and phytoplankton to hurricanes/typhoons in other systems (Yannarell et al., 2007; Zhu et al., 2014; Paerl et al., 2018). These studies have reported that increased pulses of nutrient loading, along with wind-induced nutrient resuspension from the sediments, may ultimately stimulate HABs and amplify preexisting eutrophication (James et al., 2008; Zhu et al., 2014). The HAB in the LO and SLE region in 2018 was one of the largest recorded and may have been related to rainfall and high winds from Hurricane Irma and heavy rainfall in spring 2018 (Paerl et al., 2019). Our short-term lake and estuary responses to Hurricane Irma emphasize that studies focusing on both short and long-term effects are necessary for understanding hurricane impacts on shallow, eutrophic ecosystems and cyanoHAB dynamics.
Ammonium Regeneration
Actual regeneration rates in the lake and estuary followed the same general pattern as uptake rates. During the 2016 bloom, internal regeneration rates in the lake were similar to regeneration rates in SLE. In July 2016, actual regeneration could support 43% of the potential light uptake in LO. Similarly, during the 2017 bloom, 38% of light uptake could be supported by regeneration, despite higher uptake rates. Previous dynamics experiments in LO showed that ∼35% of light uptake could be supported by regeneration (James et al., 2011). Following the hurricane, water column regeneration decreased significantly in LO and SLE, and only 24% of uptake in LO could be supported by regeneration, likely due to flushing and decreased biomass post-disturbance.
Total nitrogen (TN) from external loading into LO is estimated at ∼6.36 × 103 tons yr–1 (James et al., 2009). LO can be divided into three distinct regions: littoral zone, nearshore (1–2 m depth), and pelagic zone (offshore; James et al., 2008). Acknowledging the temporal and spatial limitations of the data generated in this study, we extrapolated the measured regeneration rates in the pelagic zone of the lake (stations L004 and LZ40) and nearshore (stations SAV165 and LOBG from this study and L005 from James et al., 2011) to estimate how much may be regenerated in LO annually relative to external N loads. Extrapolating summer bloom values in the pelagic zone of LO (estimated volume = 2.85 km3), 2.86 × 105 tons of N yr–1 could be regenerated internally. This value is 45 times greater than the estimated external loading of TN (6.36 × 103 tons yr–1; James et al., 2009). Nearshore, regeneration could supply 7.94 × 103 tons of N yr–1. These extrapolations of rates measured in summer months are almost certainly an overestimate due to higher productivity and algal biomass. However, the same calculations, using significantly lower regeneration rates from September 2017, showed that 3.37 × 104 tons of N yr–1 can be regenerated in the pelagic water column after a major disturbance. While this value is much lower than those reported based on July and August rates, it is still five times greater than annual external TN loading. These results suggest that internal regeneration supplements external TN loading in LO to a large degree, even following a major disturbance, which led to significantly lower regeneration rates. This exercise also illustrates the importance of characterizing internal nutrient dynamics on more extensive temporal and spatial scales to more explicitly constrain annual nutrient budgets.
Results from this study, showing that internal N recycling in the pelagic zone of LO supports a large proportion of primary producer demand, are supported by other studies in hypereutrophic lakes experiencing cyanoHABs, including: Lake Balaton, Hungary (Présing et al., 2001); Lake Biwa, Japan (Haga et al., 1995; Takahashi et al., 1995); Missisquoi Bay, Lake Champlain, United States/Canada (McCarthy et al., 2013); Lake Taihu, China (Paerl et al., 2011; Hampel et al., 2018), and Lake Erie, United States/Canada (Hampel et al., 2019). This study contributes to the growing literature showing that rapid turnover rates can fuel and sustain HABs, despite low concentrations (McCarthy et al., 2007; Hampel et al., 2019). In hypereutrophic Lake Taihu, about 60% of potential light uptake was supported by regeneration during a mid-summer bloom (June), and all potential demand could be supported internally in late summer (August; Hampel et al., 2018). In Sandusky Bay (Lake Erie), summer regeneration in the water column provided bioavailable N equivalent to ∼77% of the annual N load and could support most of the primary producer demand during the HAB season (Hampel et al., 2019).
Conclusion
Results from this study provided insight into and HAB dynamics in Lake Okeechobee and St. Lucie Estuary during summer HABs and after Hurricane Irma. We emphasize the importance of cycling on HAB expansion in LO and downstream nutrient pollution in SLE. Results from this study support those from previous studies (Lapointe et al., 2012; Phlips et al., 2012; Kramer et al., 2018), which call for water management strategies that balance flood control and health of downstream estuaries. Previous management strategies (40% P reductions and reduced nutrient inflow from Everglades Agricultural Area; James et al., 2011) have not prevented cyanoHABs in the Okeechobee–St. Lucie system. We show that regeneration plays an important role in Lake Okeechobee in sustaining cyanoHABs, even after a major disturbance. High nutrient loading, combined with internal recycling and climate change, will likely continue to exacerbate cyanoHABs in the Okeechobee-St. Lucie and other freshwater-estuarine systems if further N and P reductions are not implemented. Lastly, short-term lake and estuary responses to Hurricane Irma emphasize that studies focusing on both short and long-term effects are necessary for understanding hurricane impacts on shallow, eutrophic ecosystems and cyanoHAB dynamics.
Author Contributions
SN, MM, and JH designed the study and analyzed and interpreted the data. JH and MR collected and analyzed the samples. JH primarily wrote the manuscript. All authors contributed to text and revised the manuscript.
Funding
Funding for this work was provided by Florida Sea Grant (Project No. PD-16-10 to MM and SN).
Conflict of Interest
The authors declare that the research was conducted in the absence of any commercial or financial relationships that could be construed as a potential conflict of interest.
Acknowledgments
We thank the South Florida Water Management District for providing lab space, boat access, and assistance with incubations. We especially thank Therese L. East for her extensive assistance with all aspects of the project. We also thank Captain David Lauer for boat access on Lake Okeechobee after Hurricane Irma, Daniel Hoffman, Justin Myers, and Ashlynn Boedecker for help with sample analysis, and Donnie Peterson for help with field sampling.
Supplementary Material
The Supplementary Material for this article can be found online at: https://www.frontiersin.org/articles/10.3389/fmars.2019.00640/full#supplementary-material
References
Bender, M. A., Knutson, T. R., Tuleya, R. E., Sirutis, J. J., Vecchi, G. A., Garner, S. T., et al. (2010). Modeled impact of anthropogenic warming on the frequency of intense Atlantic hurricanes. Science 327, 454–458. doi: 10.1126/science.1180568
Beversdorf, L. J., Miller, T. R., and McMahon, K. D. (2015). Long-term monitoring reveals carbon and nitrogen metabolism key to microcystin production in eutrophic lakes. Front. Microbiol. 6:456. doi: 10.3389/fmicb.2015.00456
Blackburn, T. H. (1979). Method for measuring rates of nh(4) turnover in anoxic marine sediments, using a N-NH(4) Dilution technique. Appl. Environ. Microb. 37, 760–765.
Blomqvist, P., Petterson, A., and Hyenstrand, P. (1994). Ammonium-nitrogen: a key regulatory factor causing dominance of non-nitrogen-fixing cyanobacteria in aquatic systems. Arch. Hydrobiol. 132, 141–164.
Caperon, J., Schell, D., Hirota, J., and Laws, E. (1979). Ammonium excretion rates in Kaneohe Bay, Hawaii, measured by a 15N isotope dilution technique. Mar. Biol. 54, 33–40. doi: 10.1007/BF00387049
Croux, C., and Dehon, C. (2010). Influence functions of the Spearman and Kendall correlation measures. Stat. Method. Appl. 19, 497–515. doi: 10.1007/s10260-010-0142-z
Davenport, E. J., Neudeck, M. J., Matson, P. G., Bullerjahn, G. S., Davis, T. W., Wilhelm, S. W., et al. (2019). Metatranscriptomic analyses of diel metabolic functions during a Microcystis bloom in western Lake Erie (USA). Front. Microbiol. 10:2081. doi: 10.3389/fmicb.2019.02081
Davis, T. W., Berry, D. L., Boyer, G. L., and Gobler, C. J. (2009). The effects of temperature and nutrients on the growth and dynamics of toxic and non-toxic strains of Microcystis during cyanobacteria blooms. Harmful Algae 8, 715–725. doi: 10.1016/j.hal.2009.02.004
Davis, T. W., Bullerjahn, G. S., Tuttle, T., McKay, R. M., and Watson, S. B. (2015). Effects of increasing nitrogen and phosphorus concentrations on phytoplankton community growth and toxicity during Planktothrix blooms in Sandusky Bay. Lake Erie. Environ. Sci. Technol. 49, 7197–7207. doi: 10.1021/acs.est.5b00799
Ding, Y., Qin, B., Zhu, G., Wu, T., Wang, Y., and Luo, L. (2012). Effects of typhoon Morakot on a large shallow lake ecosystem, Lake Taihu, China: effects of typhoon morakot on a large shallow lake ecosystem. Ecohydrology 5, 798–807. doi: 10.1002/eco.270
Erisman, J. W., Galloway, J. N., Dise, N. B., Sutton, M. A., Bleeker, A., Grizzetti, B., et al. (2015). Nitrogen: too Much of a Vital Resource. Available at: http://www.louisbolk.org/downloads/3005.pdf (accessed October 3, 2018).
Fogel, M. L., Aguilar, C., Cuhel, R., Hollander, D. J., Willey, J. D., and Paerl, H. W. (1999). Biological and isotopic changes in coastal waters induced by Hurricane Gordon. Limnol. Oceanogr. 44, 1359–1369. doi: 10.4319/lo.1999.44.6.1359
Gardner, W. S., Cavaletto, J. F., Bootsma, H. A., Lavrentyev, P. J., and Troncone, F. (1998). Nitrogen cycling rates and light effects in tropical Lake Maracaibo, Venezuela. Limnol. Oceanogr. 43, 1814–1825. doi: 10.4319/lo.1998.43.8.1814
Gardner, W. S., Newell, S. E., McCarthy, M. J., Hoffman, D. K., Lu, K., Lavrentyev, P. J., et al. (2017). community biological ammonium demand: a conceptual model for cyanobacteria blooms in eutrophic lakes. Environ. Sci. Technol. 51, 7785–7793. doi: 10.1021/acs.est.6b06296
Glibert, P. M. (1988). “Primary productivity and pelagic nitrogen cycling,” in Nitrogen Cycling in Coastal Marine Environments, eds T. H. Blackburn, and J. Sorensen (Chichester: John Wiley & Sons), 3–31.
Glibert, P. M. (2017). Eutrophication, harmful algae and biodiversity — Challenging paradigms in a world of complex nutrient changes. Mar. Pollut. Bull. 124, 591–606. doi: 10.1016/j.marpolbul.2017.04.027
Gobler, C. J., Burkholder, J. M., Davis, T. W., Harke, M. J., Johengen, T., Stow, C. A., et al. (2016). The dual role of nitrogen supply in controlling the growth and toxicity of cyanobacterial blooms. Harmful Algae 54, 87–97. doi: 10.1016/j.hal.2016.01.010
Gu, B., Havens, K. E., Schelske, C. L., and Rosen, B. H. (1997). Uptake of dissolved nitrogen by phytoplankton in a eutrophic subtropical lake. J. Plankton Res. 19, 759–770. doi: 10.1093/plankt/19.6.759
Haga, H., Nagata, T., and Sakamoto, M. (1995). Size-fractionated NH4+ regeneration in the pelagic environments of two mesotrophic lakes. Limnol. Oceanogr. 40, 1091–1099. doi: 10.4319/lo.1995.40.6.1091
Hampel, J. J., McCarthy, M. J., Gardner, W. S., Zhang, L., Xu, H., Zhu, G., et al. (2018). Nitrification and ammonium dynamics in Taihu Lake, China: seasonal competition for ammonium between nitrifiers and cyanobacteria. Biogeosciences 15, 733–748. doi: 10.5194/bg-15-733-2018
Hampel, J. J., McCarthy, M. J., Neudeck, M., Bullerjahn, G. S., McKay, R. M. L., and Newell, S. E. (2019). Ammonium recycling supports toxic Planktothrix blooms in Sandusky Bay, Lake Erie: evidence from stable isotope and metatranscriptome data. Harmful Algae 81, 42–52. doi: 10.1016/j.hal.2018.11.011
Harke, M. J., and Gobler, C. J. (2015). Daily transcriptome changes reveal the role of nitrogen in controlling microcystin synthesis and nutrient transport in the toxic cyanobacterium, Microcystis aeruginosa. BMC Genomics 16:1068. doi: 10.1186/s12864-015-2275-9
Havens, K. E., Fukushima, T., Xie, P., Iwakuma, T., James, R., Takamura, N., et al. (2001a). Nutrient dynamics and the eutrophication of shallow lakes Kasumigaura (Japan), Donghu (PR China), and Okeechobee (USA). Environ. Pollut. 111, 263–272. doi: 10.1016/S0269-7491(00)00074-9
Havens, K. E., Jin, K.-R., Rodusky, A. J., Sharfstein, B., Brady, M. A., East, T. L., et al. (2001b). Hurricane effects on a shallow lake ecosystem and its response to a controlled manipulation of water level. Sci. World J. 1, 44–70. doi: 10.1100/tsw.2001.14
Havens, K. E., Hanlon, C., and James, R. T. (1994). Seasonal and spatial variation in algal bloom frequencies in lake okeechobee, Florida, U.S.A. Lake Reserv. Manage. 10, 139–148. doi: 10.1080/07438149409354185
Havens, K. E., James, R. T., East, T. L., and Smith, V. H. (2003). N:P ratios, light limitation, and cyanobacterial dominance in a subtropical lake impacted by non-point source nutrient pollution. Environ. Pollut. 122, 379–390. doi: 10.1016/S0269-7491(02)00304-4
Huisman, J., Codd, G. A., Paerl, H. W., Ibelings, B. W., Verspagen, J. M. H., and Visser, P. M. (2018). Cyanobacterial blooms. Nat. Rev. Microbiol. 16, 471–483. doi: 10.1038/s41579-018-0040-1
James, R. T., Gardner, W. S., McCarthy, M. J., and Carini, S. A. (2011). Nitrogen dynamics in Lake Okeechobee: forms, functions, and changes. Hydrobiologia 669, 199–212. doi: 10.1007/s10750-011-0683-7
James, R. T., Havens, K., Zhu, G., and Qin, B. (2009). Comparative analysis of nutrients, chlorophyll and transparency in two large shallow lakes (Lake Taihu, P.R. China and Lake Okeechobee, USA). Hydrobiologia 627, 211–231. doi: 10.1007/s10750-009-9729-5
James, T. R., Chimney, M. J., Sharfstein, B., Engstrom, D. R., Schottler, S. P., East, T., et al. (2008). Hurricane effects on a shallow lake ecosystem, Lake Okeechobee, Florida (USA). Fund. Appl. Limnol. 172, 273–287. doi: 10.1127/1863-9135/2008/0172-0273
Jeppesen, E., Søndergaard, M., Meerhoff, M., Lauridsen, T. L., and Jensen, J. P. (2007). Shallow lake restoration by nutrient loading reduction—some recent findings and challenges ahead. Hydrobiologia 584, 239–252. doi: 10.1007/s10750-007-0596-7
Julian, P., and Osborne, T. Z. (2018). From lake to estuary, the tale of two waters: a study of aquatic continuum biogeochemistry. Environ. Monit. Assess. 190:90. doi: 10.1007/s10661-017-6455-8
Kaebernick, M., Neilan, B. A., Borner, T., and Dittmann, E. (2000). Light and the transcriptional response of the microcystin biosynthesis gene cluster. Appl. Environ. Microb. 66, 3387–3392. doi: 10.1128/AEM.66.8.3387-3392.2000
Kana, T. M., Darkangelo, C., Hunt, M. D., Oldham, J. B., Bennett, G. E., and Cornwell, J. C. (1994). Membrane inlet mass spectrometer for rapid high-precision determination of N2, O2, and ar in environmental water samples. Anal. Chem. 66, 4166–4170. doi: 10.1021/ac00095a009
Knutson, T. R., McBride, J. L., Chan, J., Emanuel, K., Holland, G., Landsea, C., et al. (2010). Tropical cyclones and climate change. Nat. Geosci. 3:157.
Kramer, B. J., Davis, T. W., Meyer, K. A., Rosen, B. H., Goleski, J. A., Dick, G. J., et al. (2018). Nitrogen limitation, toxin synthesis potential, and toxicity of cyanobacterial populations in Lake Okeechobee and the St. Lucie River Estuary, Florida, during the 2016 state of emergency event. PLoS One 13:e0196278. doi: 10.1371/journal.pone.0196278
Lapointe, B. E., Herren, L. W., and Bedford, B. J. (2012). Effects of hurricanes, land use, and water management on nutrient and microbial pollution: St. Lucie Estuary, Southeast Florida. J. Coastal Res. 285, 1345–1361. doi: 10.2112/JCOASTRES-D-12-00070.1
Lapointe, B. E., Herren, L. W., and Paule, A. L. (2017). Septic systems contribute to nutrient pollution and harmful algal blooms in the St. Lucie Estuary, Southeast Florida, USA. Harmful algae 70, 1–22. doi: 10.1016/j.hal.2017.09.005
McCarthy, M. J., Gardner, W. S., Lavrentyev, P. J., Moats, K. M., Jochem, F. J., and Klarer, D. M. (2007). Effects of hydrological flow regime on sediment-water interface and water column nitrogen dynamics in a great lakes coastal wetland (Old Woman Creek, Lake Erie). J. Great Lakes Res. 33, 219–231. doi: 10.3394/0380-1330(2007)33
McCarthy, M. J., Gardner, W. S., Lehmann, M. F., and Bird, D. F. (2013). Implications of water column ammonium uptake and regeneration for the nitrogen budget in temperate, eutrophic Missisquoi Bay, Lake Champlain (Canada/USA). Hydrobiologia 718, 173–188. doi: 10.1007/s10750-013-1614-6
McCarthy, M. J., Gardner, W. S., Lehmann, M. F., Guindon, A., and Bird, D. F. (2016). Benthic nitrogen regeneration, fixation, and denitrification in a temperate, eutrophic lake: effects on the nitrogen budget and cyanobacteria blooms: sediment N cycling in Missisquoi Bay. Limnol. Oceanogr. 61, 1406–1423. doi: 10.1002/lno.10306
McCarthy, M. J., James, R. T., Chen, Y., East, T. L., and Gardner, W. S. (2009). Nutrient ratios and phytoplankton community structure in the large, shallow, eutrophic, subtropical Lakes Okeechobee (Florida, USA) and Taihu (China). Limnology 10, 215–227. doi: 10.1007/s10201-009-0277-5
Monchamp, M. E., Pick, F. R., Beisner, B. E., and Maranger, R. (2014). Nitrogen forms influence microcystin concentration and composition via changes in cyanobacterial community structure. PLoS One 9:e85573. doi: 10.1371/journal.pone.0085573
Moss, B., Mckee, D., Atkinson, D., Collings, S. E., Eaton, J. W., Gill, A. B., et al. (2003). How important is climate? Effects of warming, nutrient addition and fish on phytoplankton in shallow lake microcosms: climate change and phytoplankton. J. Appl. Ecol. 40, 782–792. doi: 10.1046/j.1365-2664.2003.00839.x
Newell, S. E., Babbin, A. R., Jayakumar, A., and Ward, B. B. (2011). Ammonia oxidation rates and nitrification in the Arabian Sea: arabian sea ammonia oxidation and nitrification. Glob. Biogeochem. Cycles 25:4016. doi: 10.1029/2010GB003940
Paerl, H. W., Bales, J. D., Ausley, L. W., Buzzelli, C. P., Crowder, L. B., Eby, L. A., et al. (2001). Ecosystem impacts of three sequential hurricanes (Dennis, Floyd, and Irene) on the United States’ largest lagoonal estuary, Pamlico Sound, NC. Proc. Natl. Acad. Sci. U.S.A. 98, 5655–5660. doi: 10.1073/pnas.101097398
Paerl, H. W., Crosswell, J. R., Van Dam, B., Hall, N. S., Rossignol, K. L., Osburn, C. L., et al. (2018). Two decades of tropical cyclone impacts on North Carolina’s estuarine carbon, nutrient and phytoplankton dynamics: implications for biogeochemical cycling and water quality in a stormier world. Biogeochemistry 141, 307–322. doi: 10.1007/s10533-018-0438-x
Paerl, H. W., Gardner, W. S., Havens, K. E., Joyner, A. R., McCarthy, M. J., Newell, S. E., et al. (2016). Mitigating cyanobacterial harmful algal blooms in aquatic ecosystems impacted by climate change and anthropogenic nutrients. Harmful Algae 54, 213–222. doi: 10.1016/j.hal.2015.09.009
Paerl, H. W., Havens, K. E., Hall, N. S., Otten, T. G., Zhu, M., Xu, H., et al. (2019). Mitigating a global expansion of toxic cyanobacterial blooms: confounding effects and challenges posed by climate change. Mar. Freshw. Res. doi: 10.1071/mf18392
Paerl, H. W., Xu, H., McCarthy, M. J., Zhu, G., Qin, B., Li, Y., et al. (2011). Controlling harmful cyanobacterial blooms in a hyper-eutrophic lake (Lake Taihu, China): the need for a dual nutrient (N & P) management strategy. Water Res. 45, 1973–1983. doi: 10.1016/j.watres.2010.09.018
Penn, K., Wang, J., Fernando, S. C., and Thompson, J. R. (2014). Secondary metabolite gene expression and interplay of bacterial functions in a tropical freshwater cyanobacterial bloom. ISME J. 8, 1866–1878. doi: 10.1038/ismej.2014.27
Phlips, E. J., Badylak, S., Hart, J., Haunert, D., Lockwood, J., O’Donnell, K., et al. (2012). Climatic influences on autochthonous and allochthonous phytoplankton blooms in a subtropical estuary, St. Lucie Estuary, Florida, USA. Estuar. Coast. 35, 335–352. doi: 10.1007/s12237-011-9442-2
Présing, M., Herodek, S., Preston, T., and Vörös, L. (2001). Nitrogen uptake and the importance of internal nitrogen loading in Lake Balaton. Freshw. Biol. 46, 125–139. doi: 10.1046/j.1365-2427.2001.00622.x
Takahashi, M., Hama, T., Matsunaga, K., and Handa, N. (1995). Nitrogenous nutrient uptake by phytoplankton and ammonium regeneration by microbial assemblage in Lake Biwa. J. Plank. Res. 17, 1027–1037. doi: 10.1093/plankt/17.5.1027
Yang, J., Gao, H., Glibert, P. M., Wang, Y., and Tong, M. (2017). Rates of nitrogen uptake by cyanobacterially-dominated assemblages in Lake Taihu, China, during late summer. Harmful Algae 65, 71–84. doi: 10.1016/j.hal.2017.04.001
Yannarell, A. C., Steppe, T. F., and Paerl, H. W. (2007). Disturbance and recovery of microbial community structure and function following Hurricane Frances. Environ. Microbiol. 9, 576–583. doi: 10.1111/j.1462-2920.2006.01173.x
Yin, G., Hou, L., Liu, M., Liu, Z., and Gardner, W. S. (2014). A novel membrane inlet mass spectrometer method to measure 15 NH 4 + for isotope-enrichment experiments in aquatic ecosystems. Environ. Sci. Technol. 48, 9555–9562. doi: 10.1021/es501261s
Zhang, J., Burke, P., Baldwin, L., Mo, C., and Hill, S. (2016). Lake Okeechobee Watershed Tributary Nutrient Loading Trends WY2006-WY2015. West Palm Beach, FL: South Florida Water Management District.
Keywords: hurricane, nitrogen, cyanobacteria, Florida, Okeechobee, St. Lucie Estuary
Citation: Hampel JJ, McCarthy MJ, Reed MH and Newell SE (2019) Short Term Effects of Hurricane Irma and Cyanobacterial Blooms on Ammonium Cycling Along a Freshwater–Estuarine Continuum in South Florida. Front. Mar. Sci. 6:640. doi: 10.3389/fmars.2019.00640
Received: 07 February 2019; Accepted: 30 September 2019;
Published: 16 October 2019.
Edited by:
Christopher Osburn, North Carolina State University, United StatesReviewed by:
Richard Thomas James, South Florida Water Management District, United StatesCorianne Tatariw, The University of Alabama, United States
Copyright © 2019 Hampel, McCarthy, Reed and Newell. This is an open-access article distributed under the terms of the Creative Commons Attribution License (CC BY). The use, distribution or reproduction in other forums is permitted, provided the original author(s) and the copyright owner(s) are credited and that the original publication in this journal is cited, in accordance with accepted academic practice. No use, distribution or reproduction is permitted which does not comply with these terms.
*Correspondence: Justyna J. Hampel, anVzdHluYS5oYW1wZWxAdXNtLmVkdQ==