Native Herbivores Improve Sexual Propagation of Threatened Staghorn Coral Acropora cervicornis
- 1Program in Fisheries and Aquatic Sciences, School of Forest Resources and Conservation, Institute of Food and Agricultural Sciences, University of Florida, Gainesville, FL, United States
- 2Center for Conservation, The Florida Aquarium, Apollo Beach, FL, United States
Staghorn coral Acropora cervicornis was once spatially dominant on Caribbean reefs but is now threatened throughout its range. In recent years, advancements in ex situ sexual propagation of Caribbean corals have increased the viability of this management strategy. Thus, improving culture methods for sexually propagated corals is important to bolster the overall coral restoration portfolio and increase genetic diversity in restored populations. In both natural systems and culture scenarios, algae proliferation negatively impacts coral growth and survival. Growing coral with native herbivores may represent a strategy for increased efficiency. We tested A. cervicornis recruits raised in replicate aquaria with identical densities of juvenile Lithopoma americanum or juvenile Batillaria minima snails plus a no-snail control. Each of three replicates per treatment contained tiles with similar numbers of recently settled, visually healthy, A. cervicornis. Tiles were photographed every 3 weeks for 5 months and coral growth, survivability, turf algae cover, and crustose coralline algae (CCA) cover were quantified. Labor time for cleaning was carefully recorded for each treatment. Results indicated improved growth and survival when A. cervicornis recruits were raised with either snail species in comparison to a no-herbivore control. Further, including snails decreased labor and eliminated turf algae cover. Interestingly, L. americanum significantly reduced CCA cover relative to the other treatments. We report some of the highest survival rates observed to date for sexually propagated Atlantic corals. Ultimately, results suggest that rearing sexually propagated A. cervicornis with native herbivores could improve the ability to employ these corals in reef restoration.
Introduction
Persistent global coral reef degradation continues to amplify the need to improve understanding of these ecosystems and develop new methodologies to aid in their restoration. Coral reefs are extremely valuable as they provide a multitude of environmental and economic benefits including coastal protection and stability, tourism, and a source of food (Massel and Gourlay, 2000; Burke et al., 2011; Cinner, 2014). In southeast Florida alone, reefs generate $3.3 billion a year of economic impact and support 70,000 jobs (Johns et al., 2013). Anthropogenic and environmental factors including climate change, disease, pollutants, and extreme weather events continue to place these vulnerable ecosystems on the edge of loss (Goreau and Hilbertz, 2005; Young et al., 2012). Many Caribbean reefs have reached a point at which natural processes are no longer capable of fully recovering the habitat (Goreau and Hilbertz, 2005). For this reason, active reef restoration is a vital tool for the future of coral reefs (Rinkevich, 2008; Van Oppen et al., 2017). Given limited time and resources (Mumby and Steneck, 2008), it is important to identify the most effective techniques to grow and out-plant corals quickly and affordably (Lirman et al., 2010). Scaling-up out-planting capabilities and reducing associated costs are critical to the success of active reef restoration (Young et al., 2012).
Staghorn coral Acropora cervicornis was one of the spatially dominant reef building species in south Florida and is distributed throughout the Western Atlantic (Goldberg, 1973; Aronson and Precht, 2001). This species has declined by 97% over the last 30 years throughout south Florida and parts of the Caribbean (Acropora Biological Review Team, 2005). In 2006, A. cervicornis and its congener A. palmata became the first corals listed under the U.S. Endangered Species Act (National Marine Fisheries Service [NMFS], 2006). Subsequently, A. cervicornis has become the species most commonly cultured for restoration (Young et al., 2012), with most efforts relying on coral fragments produced asexually in in situ coral nurseries from a limited number of genotypes. Recovery of A. cervicornis will require scalable sexual reproduction to foster genetic diversity within restored populations (Hunt and Sharp, 2014). In recent years, great advancements in ex situ sexual propagation of corals have made this a viable restoration strategy (Nakamura et al., 2011; Chamberland et al., 2016; Craggs et al., 2019). Thus, improving grow-out methods for sexual recruits in land-based nurseries is becoming increasingly relevant to the active restoration of A. cervicornis and maintenance of genetic diversity in out-planted populations (Hunt and Sharp, 2014). Although there is still much to be learned, the improvements made toward the process of collecting wild spawn and settling Acropora larvae in aquaria are substantial (Chamberland et al., 2015, 2016; Patterson et al., 2016). Increased settlement and higher survival rates mean more corals to care for and a higher cost to do so in land-based nurseries. Therefore, decreasing cost and reducing labor hours are essential to scaling land-based culture of sexually propagated corals to ecologically relevant levels.
Given the complexities of land-based coral propagation, it is important to continually refine this process and identify the most effective techniques when rearing A. cervicornis ex situ. Like ocean nurseries, the purpose of land-based nurseries is to grow corals in an environment reasonably free of stressors including disease, predators, algae, etc. (Griffin et al., 2012). One of the most laborious aspects of land-based propagation is controlling fleshy algae, which can quickly overgrow coral recruits with negative consequences. Algae stresses recruits and inhibits growth, making it a factor that must be addressed in culture scenarios (Toh et al., 2013). Grazing herbivores may help control algae to maintain systems and improve coral development (Serafy et al., 2013; Toh et al., 2013; Villanueva et al., 2013; Craggs et al., 2019). This interaction mirrors natural systems, where herbivores play an important role in controlling benthic algal succession by maintaining small epilithic algae in a grazed state (Lirman, 2001; Suchley and Alvarez-Filip, 2017). By replicating conditions encountered in the wild, rearing juvenile corals with herbivores may reduce the amount of time spent cleaning aquaria. This could reduce the potential for negative impacts of increased handling and improve overall coral culture efficiency in land-based systems.
For biosecurity reasons, any organism used in co-culture with corals intended for reintroduction must be of similar known origin. Therefore, our study tested two native Caribbean herbivorous snail species collected from South Florida waters to determine their utility for growing A. cervicornis sexual recruits ex situ. Both snail species naturally occur in the subtropical waters of southern Florida and grow to an ideal size for land-based co-culture. Snails selected were Lithopoma americanum, a gastropod in the family Turbinidae, and Batillaria minima a gastropod in the family Batillaridae. Both snail species are common and commercially available and are thus practical options in culture scenarios. For example, L. americanum has been identified as one of the four most common macroinvertebrates in a Biscayne Bay, Florida seagrass bed (Maciá, 2000). Juvenile snails of <1 cm shell width were used due to concerns over large adult snails damaging coral recruits. Ultimately, this study seeks to determine whether the addition of herbivorous snails will increase survival and growth while decreasing labor involved in raising sexually propagated A. cervicornis in land-based systems. Furthermore, study design will allow quantification of differences in performance, if any, between snail species.
Materials and Methods
Gamete Collection and Settlement
Experimental activities and A. cervicornis gamete collection were permitted under National Oceanic and Atmospheric Administration’s National Marine Sanctuaries permit number FKNMS-2015-133-A2 to The Florida Aquarium. Gametes were collected from August 2 to August 4, 2018. Nineteen colonies from 10 unique A. cervicornis genets from the Coral Restoration Foundation’s Tavernier nursery were staged in land-based flow through systems at the Keys Marine Laboratory in Long Key, FL, United States. Colonies were monitored until egg and sperm bundles were released. Bundles were then collected and mixed as a single batch to achieve fertilization. Fertilized eggs were rinsed in 1-micron filtered seawater and separated and then allowed to develop for 72 h before transport to The Florida Aquarium’s Center for Conservation greenhouses in Apollo Beach. Upon arrival, larvae were allocated into 15 settlement containers suspended in a 2270-L recirculating aquarium. Settlement containers were made of 25 × 40 cm plastic wash basins with four 10 cm holes on the sides covered with 150-micron mesh to allow water flow while containing free swimming larvae. Filtered water was continuously supplied to the basins. Each wash basin contained conditioned 3 × 3 cm ceramic settlement tiles and a small amount of ground crustose coralline algae (CCA) to facilitate larval settlement. Larvae were allowed to settle over a 7-day period, with tiles being removed and replaced when several recruits were observed. Tiles with settled coral recruits were placed in shallow (244 L × 86 W × 20 H cm) rectangular trays with shading and higher flow. Coral recruits developed until they were determined to be visually healthy and initial signs of zooxanthellae uptake were observed. This period lasted approximately 2 weeks. A total of 90 tiles, each containing three to four recently settled corals on the top surface, were selected for the study.
Recirculating System and Husbandry
A single recirculating system containing 1140 L of water was used for the experiment. Nine replicate 38-L glass aquaria were set-up to test three treatments: juvenile L. americanum snails, juvenile B. minima snails (both ∼1-cm shell width at one snail per 46 cm2 tank surface area), and a no-snail control. Each treatment was randomly assigned to three replicate aquaria each containing 10 tiles with three to four recently settled A. cervicornis per tile, as described in the section “Gamete Collection and Settlement.” Divider screens were used to restrict surface area accessible by snails in each replicate aquarium to 2,530 cm2. A 21-day sampling interval was established for the duration of the 126-day experiment. Throughout the experiment, aquaria glass and divider screens were regularly cleaned for adequate water flow and sunlight to mimic the conditions of standard shallow propagation trays. Tiles were only cleaned periodically as will be described in the section “Tile Cleaning and Photographs.” Dead snails were removed and replaced with live snails after the 21- and 42-day sampling intervals to account for any mortality due to transfer stress. After day 42, dead snails were not replaced. Reverse osmosis deionized (RO-DI) water brought to a salinity of 36 ppt with a commercially available salt mixture (Pro-Reef Sea Salt, Tropic Marin, Wartenberg, Germany) was used for coral culture. Water temperatures were maintained between 25 and 26°C. Salinity, alkalinity, total calcium, phosphates, nitrate, and pH were monitored weekly. Water changes (20% of total system volume) were conducted weekly and RO-DI water was added to compensate for evaporation as needed. Alkalinity and total calcium were maintained with sodium bicarbonate and calcium chloride dosing, respectively. Commercial diets were fed to all aquaria in equal amounts three times weekly. Aquaria were initially shaded with 50% shade cloth and gradually acclimated to 30% shade cloth.
Tile Cleaning and Photographs
All experimental tiles were cleaned by hand at the end of each 21-day sampling interval. A standardized cleaning method was used in which all turf algae was removed from the tile and a 3-mm section of CCA was removed around the perimeter of each recruit. A timer was started at the beginning of cleaning each tile and stopped upon completion. The total time to clean the tiles in each replicate aquarium was divided by the number of coral recruits, yielding cleaning time per recruit in seconds. Cleaning was performed by the same two individuals at each sampling interval.
A DSLR camera (EOS 5D Mark IV, Canon, Melville, NY, United States) mounted to a dissecting microscope was used to capture scaled photos of every tile at the conclusion of each 21-day sampling interval. Photos of each tile were taken before and after algae cleaning. Photos were used to generate data as described in the sections “Measuring Coral Growth and Survival,” “Quantifying Turf Algae and CCA,” and “Statistical Analyses.”
Measuring Coral Growth and Survival
The image analysis software Image J (Rasband W, NIH) was used to measure coral coverage in scaled photos by tracing the margins of each recruit and calculating area in cm2. Dead recruits were recorded at this time and not traced. The total area of all recruits on each tile was divided by the number of recruits on the tile to determine mean area per recruit. Tiles were considered subsamples of each replicate aquarium. After each sampling interval, all tiles and snails (if present) were rotated into the adjacent aquarium to control for tank effects.
Quantifying Turf Algae and CCA
Turf algae and CCA were quantified separately at each sampling interval by the same reader estimating percent coverage in 10% increments. Photos taken before cleaning were used to estimate turf algae coverage and photos taken after the removal of turf algae were used to estimate CCA coverage. CCA removed around the periphery of recruits during cleaning was considered present for the purposes of estimating percent coverage. Percent coverage of turf algae and CCA was averaged across all subsamples for each replicate.
Statistical Analyses
All statistical tests were performed using R software (v. 3.5.0, R Core Team, 2014) and conducted at a significance level of α = 0.05. Means are presented as mean ± standard error (SE). Coral survival was calculated at the level of individual recruits and compared among treatments throughout the 126-day study using Kaplan–Meier survival analysis in the R package “survival” (Therneau, 2015). Data were assessed for normality and homogeneity of variance using visual assessment of residual plots and Bartlett’s tests, respectively. Percentage data were arcsine square-root transformed prior to analysis. Non-percentage data were log transformed and reassessed when ANOVA assumptions were not met. Repeated measures data were tested for sphericity and corrected using the Greenhouse–Geisser correction. A linear mixed effects model was used with treatment and time as fixed effects and replicate tank as the random effect. The Tukey’s HSD post hoc test was employed when significant differences were detected.
Results
Survival
Coral recruit survival patterns differed significantly among herbivore treatments (Figure 1, Kaplan–Meier X2 = 7.3, df = 2, p = 0.03). Replicate aquaria containing L. americanum, B. minima, and no-snail controls began the experiment with 33.3 ± 0.9, 34.7 ± 1.2, and 35.7 ± 1.2 coral recruits, respectively. After 126 days, L. americanum replicate aquaria had 25.3 ± 2.6 live corals (∼76% survival), B. minima replicate aquaria had 24.0 ± 0.7 live corals (∼69% survival), and no-snail control replicate aquaria had 20.0 ± 2.1 live corals (∼56% survival).
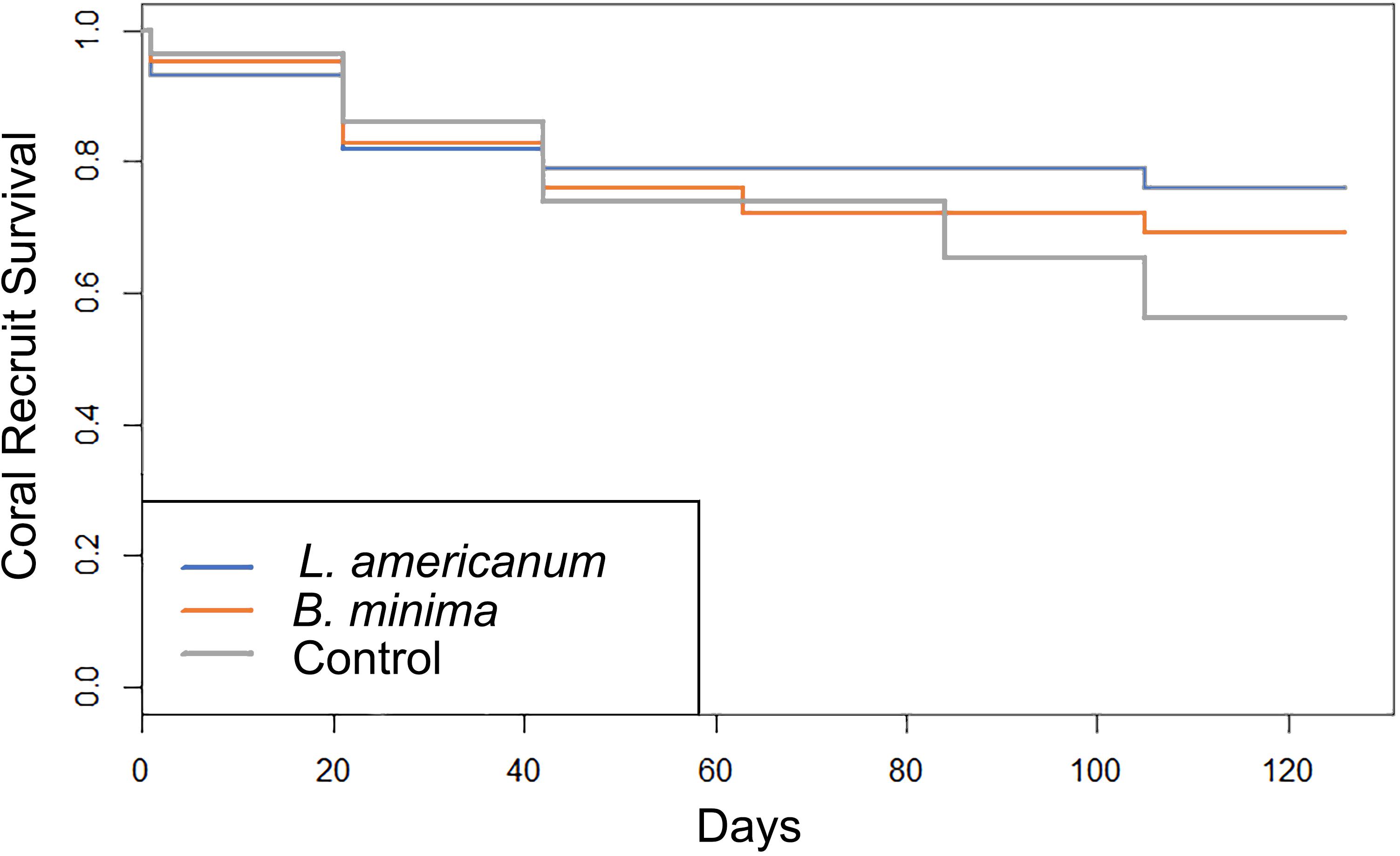
Figure 1. Acropora cervicornis recruit survival for three experimental treatments over the course of the 126-day study (Kaplan–Meier X2 = 7.3, df = 2, p = 0.03).
Coral Growth
Coral recruits were larger in both treatments containing snails than in the no-snail controls (Figures 2, 3). Significant differences in area (mm2) per coral recruit were detected among treatments (Ftreatment(2,6) = 7.4, p = 0.02) as well as a significant interaction between treatment and time (Ftreatment × day(12,36) = 10.4, p < 0.001), with post hoc analysis indicating that corals in the no-snail control were smaller than either treatment containing snails.
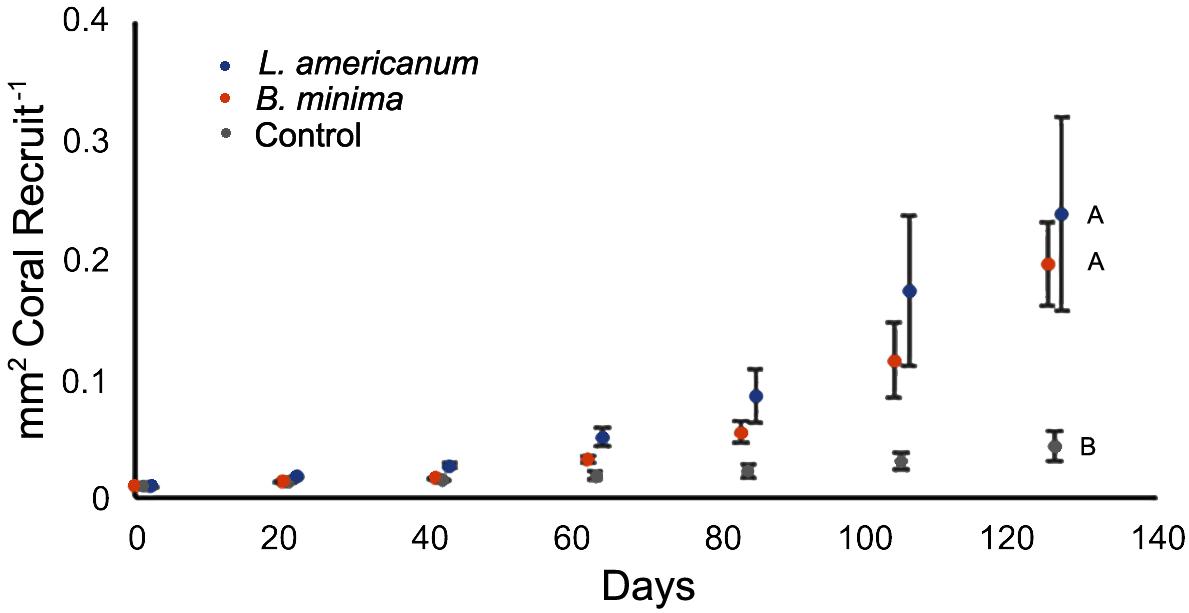
Figure 2. Mean Acropora cervicornis recruit size (mm2) over time (Ftreatment(2,6) = 7.4, p = 0.02; Ftreatment × day(12,36) = 10.4, p < 0.001). n = 3 replicate aquaria per treatment. Letters indicate differences among treatments as determined by the Tukey’s HSD post hoc test.
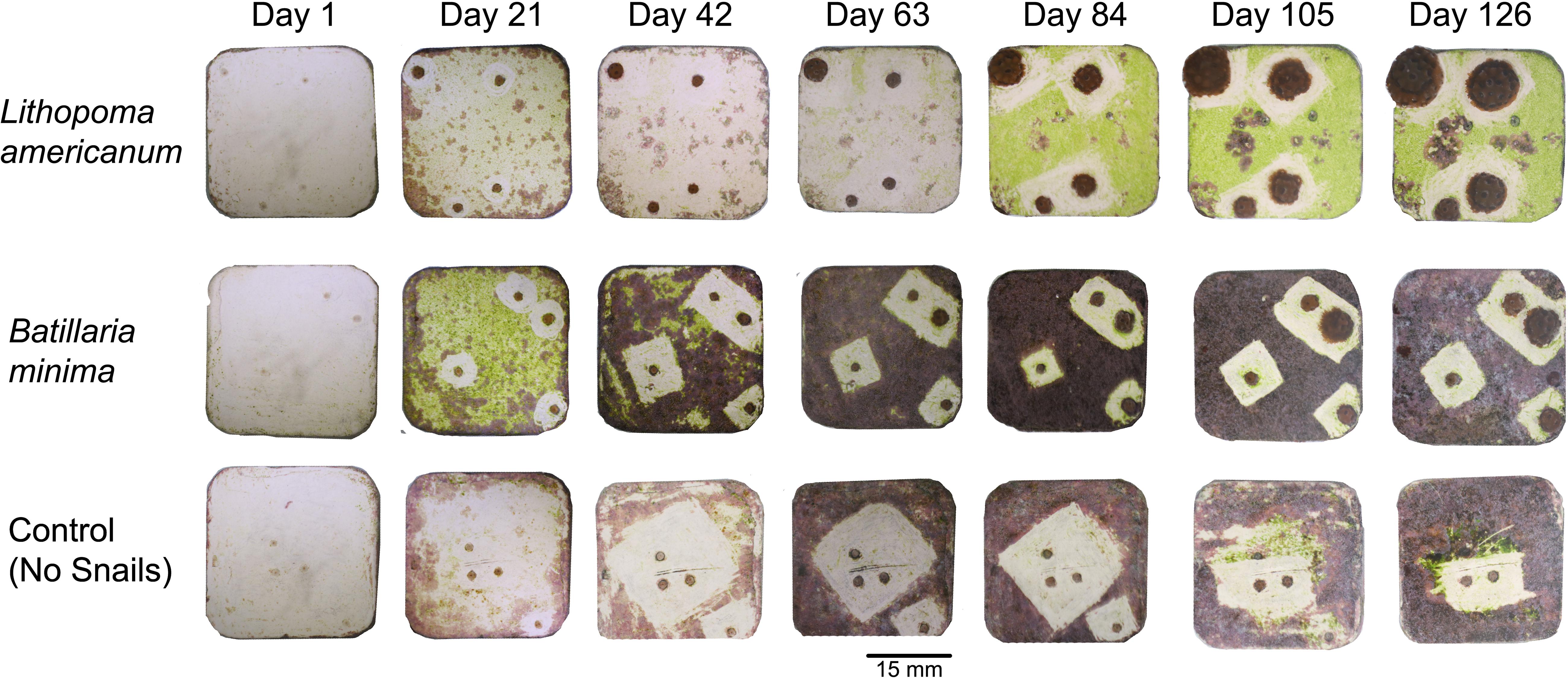
Figure 3. Repeated photographs of individual representative tiles with Acropora cervicornis sexual recruits from each experimental treatment following turf algae removal at each 21-day sampling interval.
Turf Algae and CCA Growth
No turf algae was recorded growing on tiles in treatments containing B. minima or L. americanum at any sampling date (Figure 4A). All no-snail control tiles had 100% turf algae cover at each monitoring event through day 63. Beginning at day 63, turf algae cover gradually decreased through the last day of monitoring.
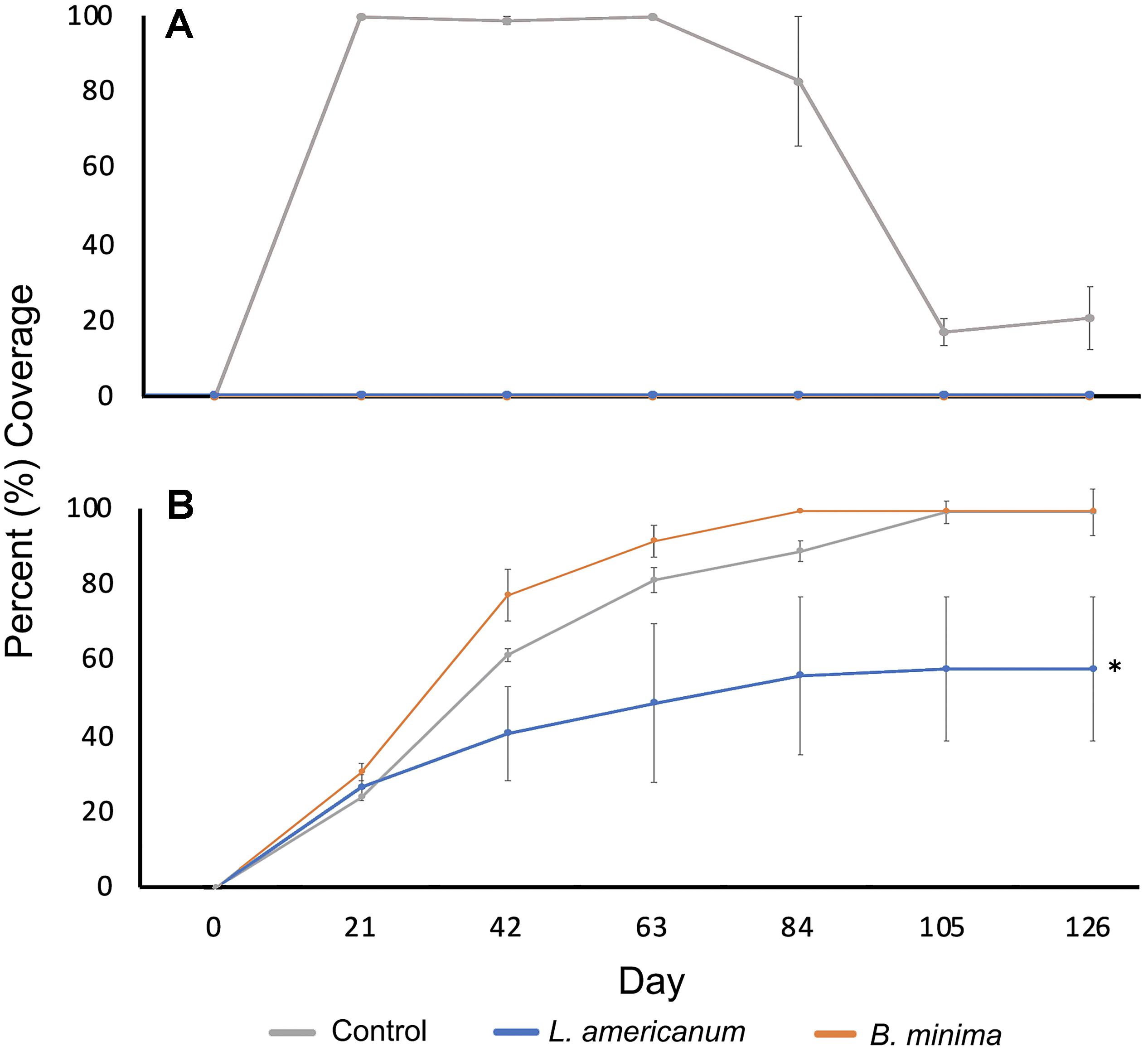
Figure 4. Mean percentage of each tile containing Acropora cervicornis sexual recruits covered with (A) turf algae (statistical comparison precluded by large number of zero values) and (B) CCA (Ftreatment(2,6) = 7.1, p = 0.03; Ftreatment × day(12,36) = 5.4, p < 0.0001) from each snail species treatment and the no snail control at each 21-day sampling interval. n = 3 replicate aquaria per treatment. The asterisk (∗) indicates differences among treatments as determined by the Tukey’s HSD post hoc test.
Crustose coralline algae cover increased throughout the study (Figure 4B). Significant differences in CCA coverage were detected among treatments (Ftreatment(2,6) = 7.1, p = 0.03) along with a significant interaction between treatment and time (Ftreatment × day(12,36) = 5.4, p < 0.0001). Post hoc analysis indicated that aquaria containing L. americanum experienced less CCA growth than those containing B. minima or the no snail control. All tiles in the no-snail control treatments were completely covered in CCA by day 104. All tiles in the treatments containing B. minima were completely covered at day 84 while tiles in L. americanum treatments never became fully covered in CCA.
Cleaning Times
Cleaning time was significantly dependent on treatment (Ftreatment(2,6) = 56.8, p = 0.0001) with a significant interaction between treatment and time (Ftreatment × day(10, 30) = 3.0, p = 0.01). Post hoc analysis indicated that both snails significantly reduced cleaning time relative to the no snail control, but no difference between snail treatments was observed. It took 19 min and 20 s ± 1 min and 16 s to fully clean the tiles in replicate aquariums containing L. americanum and 19 min and 42 s ± 1 min and 2 s to clean tiles in an aquarium containing B. minima. The average time to clean tiles in a control aquarium was 47 min and 4 s ± 5 min and 51 s.
Discussion
Our results support the hypothesis that native Caribbean herbivorous snails represent a viable means of increasing sexual propagation efficiency in A. cervicornis. Both juvenile L. americanum and B. minima maintained tiles with coral recruits completely free of turf algae throughout the duration of the experiment. Importantly, there were no apparent negative consequences on coral recruit survival from snail predation or shell drag damage at day 142. Toh et al. (2013) found similar results using adults of the urchin Salmacis sphaeroides and snail Trochus maculatus in co-culture with Pocillopora damicornis recruits. These herbivores were effective at reducing turf algae and thereby enhancing coral growth. However, while coral recruit survival was not analyzed statistically, it was numerically lower in the T. maculatus snail treatment (68.8%) than in either the S. sphaeroides (87.7%) or no-grazer control (75.0%) treatments (Toh et al., 2013). In contrast to the present study, Toh et al. (2013) used adult herbivores of equal biomass at much lower individual densities (one urchin or four snails per 30-L tank) and did not report complete removal of turf algae from the coral culture substrate. Snails in the present study were small juveniles, standardized by number of individuals per unit of tank surface area. A greater number of smaller grazers may have facilitated more complete removal of turf algae with less physical damage to coral recruits. Villanueva et al. (2013) found increased survivorship of Acropora valida recruits when reared with juvenile Trochus niloticus snails. Snail densities were much lower than in the present experiment, at a maximum of 8 per 10,000 cm2. Coral recruit survival to 5 weeks at this snail density averaged 18.3 versus 5.5% in a no snail control (Villanueva et al., 2013). More recently, juvenile sea urchins Mespilia globulus were shown to improve growth and survival of Acropora millepora sexual recruits (Craggs et al., 2019). Multiple herbivore densities were tested, and increasing densities produced higher growth and survival up to the maximum density tested (75 urchins per 10,000 cm2 tank surface area; Craggs et al., 2019).
While initial herbivore densities in the present study were relatively high, snail mortalities during the experiment revealed that it was possible to maintain zero turf algae cover with fewer individuals. By day 126, L. americium density averaged 1 snail per 84 cm2 tank surface area and no turf algae growth was observed. In practice, this represents a reduction from ∼55 to ∼30 snails per aquarium from day 42 (when initial mortalities were last replaced) to the end of the experiment on day 126. This suggests that a carrying capacity was reached and food resources were insufficient to support initial L. americanum densities. All data including live snail counts for each replicate aquarium at the beginning and end of all 21-day sampling intervals are available in the Supplementary Materials. While other studies have found increasing coral survival up to the highest herbivore density tested (Villanueva et al., 2013; Craggs et al., 2019), the present study clearly surpassed the maximum density required to control turf algae. Further research to optimize herbivore density in relation to system characteristics is warranted. It is important to reiterate that glass aquarium surfaces in the present experiment were cleaned to mimic typical shallow production trays that allow full sunlight exposure. Relying on snails rather than manual cleaning of these surfaces may have resulted in more food resources and lower rates of mortality.
Toh et al. (2013), Villanueva et al. (2013), and Craggs et al. (2019) are the only other studies we are aware of to test grazing herbivores in ex situ culture of coral sexual recruits, albeit in Pacific rather than Caribbean species. However, herbivory as a tool for ex situ culture of A. cervicornis asexual fragments has also been explored (Serafy et al., 2013). These authors used three treatment conditions to examine the efficacy of juvenile variegated sea urchins Lytechinus variegatus in removing algae and improving coral growth. These treatments included manually scraping algae with a razor blade, the presence of L. variegatus, and a control absent of both urchins and razor blade cleaning. The study found significant differences in growth between all treatments. Manual cleaning resulted in the highest growth rate (3.1 mm2 d–1), followed by L. variegatus (1.9 mm2 d–1) while control treatments lost tissue (−0.8 mm2 d–1) (Serafy et al., 2013). Although our experimental design was slightly different, both studies show the utility of grazing herbivores to remove competitive algae and improve coral growth. Furthermore, grazing herbivores reduced labor in both studies; an important factor to consider as operations scale-up.
Future research should seek to identify other high-performing herbivores to improve coral growth and reduce manual labor. During early stages of development, coral recruits also compete with CCA for space. CCA has been shown to be one of few algal taxa capable of overgrowing healthy corals by direct contact (McCook et al., 2001). Interestingly, L. americanum snails were more effective than B. minima at controlling CCA on the tiles. This was the only metric in which performance between the two snail species differed. This warrants further investigation because it has direct impact on growing coral recruits and potential ecological implications.
Decreasing cost through reducing labor hours is essential to the continued development of coral sexual propagation in land-based systems. During our study, manual cleaning of corals was greatly reduced in treatments containing snails as tiles in control aquaria took nearly 2.5-fold longer to clean. This can be extrapolated to over 40 h of labor savings every 3 weeks for a land-based facility containing 1,000 tiles with coral recruits. Such an increase in efficiency might allow budget-constrained conservation organizations to increase the scale of their coral sexual propagation efforts.
The present study also demonstrated post larval settlement survival rates that increase the viability of sexual propagation as a scalable tool for coral restoration. Chamberland et al. (2015) observed ∼10% survival in A. palmata at 6 months post settlement. In our study, survival rates as high as 76% were observed at 5 months post settlement. To our knowledge, this represents the highest survivorship reported for Acropora recruits reared in land-based systems. Increased survival may be at least partially attributable to increased labor and resource inputs. For comparison, corals cultured by Chamberland et al. (2015) were maintained in a flow-through sea water system and received weekly maintenance to remove algae and sediment. Juvenile doctorfish (Acanthurus chirurgus) and blue legged hermit crabs (Clibanarius tricolor) were used to suppress algae growth on substrates. In contrast, our system was a closed recirculating system using RO-DI with a commercially available salt mixture. Algae and sediment were removed from aquaria three times a week and settlement tiles were manually cleaned every 3 weeks. Thus, further consideration should be given to the tradeoffs involved in system design and husbandry protocols to improve coral recruit survivorship in land-based systems.
Further research addressing additional sexual propagation bottlenecks is necessary. Light intensity is considered a critical factor and shading investigations could further increase survival and growth. High variability in the L. americanum treatment was driven by a single replicate aquarium that performed substantially better than the others. Although treatments were rotated throughout the study, we speculate that this was due to tank location within the system resulting in lower light levels for this replicate during the first 21 days of development. This suggests that greater shading could increase survival and growth of A. cervicornis recruits. Little is known about how sexually produced corals reared ex situ will perform once placed back into the ocean. In order to determine the long-term utility of sexually producing and land rearing A. cervicornis it is important to systematically evaluate reintroduction to natural systems and ocean-based nurseries. Lastly, the present study portends a threat faced by larger coral colonies upon outplant to natural reefs. Although herbivorous snails would not be effective for algae control in natural systems, native herbivores including Diadema antillarum could eventually be propagated and employed in more holistic coral reef restoration efforts (Sharp et al., 2018).
Ultimately, scaling up production and improving sexual propagation techniques is imperative to building a genetically diverse population of this species along the Florida Reef Tract (Young et al., 2012). This study demonstrated the benefits of a simple husbandry modification to land-based coral culture and the positive impacts on survival and growth. Labor requirements were also significantly reduced. Continuing to refine the process of raising juvenile A. cervicornis through simple cost-efficient strategies will ultimately enhance sexual propagation and restoration of Caribbean reefs with the potential for translation to other coral species regionally and globally.
Data Availability Statement
All datasets generated for this study are included in the article/Supplementary Material.
Author Contributions
JH, KO’N, and JP conceived the study. JH and KO’N carried out the study. JH and JP conducted the statistical analysis and co-wrote the manuscript.
Funding
JH received support from the U.S. GI Bill. In-kind support was provided by The Florida Aquarium. This work was also partially supported by the USDA National Institute of Food and Agriculture Project FLA-FOR-005375 to JP. Publication of this article was funded in part by the University of Florida Open Access Publishing Fund.
Conflict of Interest
The authors declare that the research was conducted in the absence of any commercial or financial relationships that could be construed as a potential conflict of interest.
Acknowledgments
We thank The Florida Aquarium for the time and expertise of their staff to assist in the development and execution of this study. We also thank the Coral Restoration Foundation for facilitating this study by providing access to coral gametes. Finally, we thank Austyn Bushman for assistance with tile cleaning and data collection, as well as Aaron Pilnick and other members of the Patterson lab for their help with statistical analyses and system maintenance.
Supplementary Material
The Supplementary Material for this article can be found online at: https://www.frontiersin.org/articles/10.3389/fmars.2019.00713/full#supplementary-material
References
Acropora Biological Review Team (2005). Atlantic Acropora Status Review Document. Seattle, WA: Southeast Regional Office.
Aronson, R., and Precht, W. (2001). “White-band disease and the changing face of Caribbean coral reefs,” in The Ecology and Etiology of Newly Emerging Marine Diseases. ed. J. W. Porter. (London: Kluwer), 25–38. doi: 10.1007/978-94-017-3284-0_2
Burke, L., Reytar, K., Spalding, M., and Perry, A. (2011). Reefs at Risk Revisited. Washington, WA: WRI.
Chamberland, V., Petersen, D., Latijnhouwers, K., Snowden, S., Mueller, B., and Vermeij, M. (2016). Four-year-old caribbean Acropora colonies reared from field-collected gametes are sexually mature. Bull. Mar. Sci. 92, 263–264. doi: 10.5343/bms.2015.1074
Chamberland, V., Vermeij, M., Brittsan, M., Carl, M., Schick, M., Snowden, S., et al. (2015). Restoration of critically endangered elkhorn coral (Acropora palmata) populations using larvae reared from wild-caught gametes. Glob. Ecol. Conserv. 4, 526–537. doi: 10.1016/j.gecco.2015.10.005
Cinner, J. (2014). Coral reef livelihoods. Curr. Opin. Enviro. Sustain. 7, 65–71. doi: 10.1016/j.cosust.2013.11.025
Craggs, J., Guest, J., Bulling, M., and Sweet, M. (2019). Ex situ co culturing of the sea urchin, Mespilia globulus and the coral Acropora millepora enhances early post-settlement survivorship. Sci. Rep. 9:12984. doi: 10.1038/s41598-019-49447-9
Goldberg, W. (1973). The ecology of the coral-octocoral community of the southeast florida coast: geomorphology, species composition and zonation. Bull. Mar. Sci. 23, 465–488.
Goreau, T., and Hilbertz, W. (2005). Marine ecosystem restoration: costs and benefits for coral reefs. World Res. Rev. 17, 375–409.
Griffin, S., Spathias, H., Moore, T., Baums, I., and Griffin, B. (2012). “Scaling up Acropora nurseries in the caribbean and improving techniques,” in Proceedings of the 12th International Coral Reef Symposium, Townsville
Hunt, J., and Sharp, W. (2014). Developing a Comprehensive Strategy for Coral Restoration for Florida. Report No SWG T-32-R. 1169, California, CA.
Johns, G., Kelble, C., Lee, D., Leeworthy, V., and Nuttle, W. (2013). Ecosystem Services Provided by the South Florida Coastal Marine Ecosystem. MARES Whitepaper: Ecosystem Services. Available at: https://nccospublicstor.blob.core.windows.net/projects-attachments/200/MARES_White%20Paper_Ecosystem%20Services.pdf (accessed July 26, 2019).
Lirman, D. (2001). Competition between macroalgae and corals: effects of herbivore exclusion and increased algal biomass on coral survivorship and growth. Coral Reefs 19, 392–399. doi: 10.1007/s003380000125
Lirman, D., Thyberg, T., Herlan, J., Hill, C., Young-Lahiff, C., Schopmeyer, S., et al. (2010). Propagation of the threatened staghorn coral Acropora cervicornis: methods to minimize the impacts of fragment collection and maximize production. Coral Reefs 29, 729–735. doi: 10.1007/s00338-010-0621-6
Maciá, S. (2000). The effects of sea urchin grazing and drift algal blooms on a subtropical seagrass bed community. J. Exp. Mar. Biol. Ecol. 246, 53–67. doi: 10.1016/s0022-0981(99)00172-0
Massel, S., and Gourlay, M. (2000). On the modelling of wave breaking and set-up on coral reefs. Coast Eng. 39, 1–27. doi: 10.1016/s0378-3839(99)00052-6
McCook, L., Jompa, J., and Diaz-Pulido, G. (2001). Competition between corals and algae on coral reefs: a review of evidence and mechanisms. Coral Reefs 19, 400–417. doi: 10.1007/s003380000129
Mumby, P., and Steneck, R. (2008). Coral reef management and conservation in light of rapidly evolving ecological paradigms. Trends Ecol. Evol. 23, 555–563. doi: 10.1016/j.tree.2008.06.011
Nakamura, R., Ando, W., Yamamoto, H., Kitano, M., Sato, A., Nakamura, M., et al. (2011). Corals mass-cultured from eggs and transplanted as juveniles to their native, remote coral reef. Mar. Ecol. Prog. Ser. 436, 161–168. doi: 10.3354/meps09257
National Marine Fisheries Service [NMFS] (2006). Endangered and threatened species: final listing determinations for elkhorn and staghorn coral. Fed. Reg. 71, 26852–26861.
Patterson, J., Flint, M., Than, J., and Watson, C. (2016). Evaluation of substrate properties for settlement of Caribbean staghorn coral Acropora cervicornis larvae in a land-based system. N. Am. J. Aquacult. 78, 337–345.
R Core Team (2014). R: A language and environment for statistical computing. Vienna, Austria: R Foundation for Statistical Computing.
Rinkevich, B. (2008). Management of coral reefs: we have gone wrong when neglecting active reef restoration. Mar. Pollut. Bull. 56, 1821–1824. doi: 10.1016/j.marpolbul.2008.08.014
Serafy, J., Gillette, P., Miller, M., Lirman, D., and Capo, T. (2013). Incorporating herbivorous sea urchins in ramet culture of staghorn coral Acropora cervicornis. Endanger Species Res. 22, 183–189. doi: 10.3354/esr00544
Sharp, W., Delgado, G., Hart, J., and Hunt, J. (2018). Comparing the behavior and morphology of wild-collected and hatchery-propagated long-spined urchins (Diadema antillarum): implications for coral reef ecosystem restoration. Bull. Mar. Sci. 94, 103–122. doi: 10.5343/bms.2017.1068
Suchley, A., and Alvarez-Filip, L. (2017). Herbivory facilitates growth of a key reef-building Caribbean coral. Ecol. Evol. 7, 11246–11256. doi: 10.1002/ece3.3620
Toh, T., Ng, C., Guest, J., and Chou, L. (2013). Grazers improve health of coral juveniles in ex situ mariculture. Aquaculture 414, 288–293. doi: 10.1016/j.aquaculture.2013.08.025
Van Oppen, M., Gates, R., Blackall, L., Cantin, N., Chakravarti, L., Chan, W., et al. (2017). Shifting paradigms in restoration of the world’s coral reefs. Glob. Chang. Biol. 23, 3437–3448. doi: 10.1111/gcb.13647
Villanueva, R., Baria, M., and dela Cruz, D. (2013). Effects of grazing by herbivorous gastropod (Trochus niloticus) on the survivorship of cultured coral spat. Zool. Stud. 52:44.
Keywords: coral restoration, herbivory, aquaculture, coral reef, threatened species
Citation: Henry JA, O’Neil KL and Patterson JT (2019) Native Herbivores Improve Sexual Propagation of Threatened Staghorn Coral Acropora cervicornis. Front. Mar. Sci. 6:713. doi: 10.3389/fmars.2019.00713
Received: 26 August 2019; Accepted: 05 November 2019;
Published: 21 November 2019.
Edited by:
Jesús Ernesto Arias González, Center for Research and Advanced Studies of the National Polytechnic Institute (CINVESTAV), MexicoReviewed by:
Ronaldo Bastos Francini-Filho, Federal University of Paraíba, BrazilAldo Cróquer, Simón Bolívar University, Venezuela
Copyright © 2019 Henry, O’Neil and Patterson. This is an open-access article distributed under the terms of the Creative Commons Attribution License (CC BY). The use, distribution or reproduction in other forums is permitted, provided the original author(s) and the copyright owner(s) are credited and that the original publication in this journal is cited, in accordance with accepted academic practice. No use, distribution or reproduction is permitted which does not comply with these terms.
*Correspondence: Joshua T. Patterson, joshpatterson@ufl.edu