- 1Dipartimento di Scienze della Vita e dell'Ambiente, Università Politecnica delle Marche, Ancona, Italy
- 2Departamento de Estratigrafía y Paleontología, Universidad de Granada, Granada, Spain
- 3Équipe Écogéochimie et Fonctionnement des Écosystèmes Benthiques, Laboratoire Adaptation et Diversité en Milieu Marin, Station Biologique de Roscoff, Roscoff, France
- 4Grupo BioCost, Departamento de Bioloxía, Universidade da Coruña, A Coruña, Spain
- 5Institut Systématique Evolution Biodiversité (ISYEB), Muséum National d'Histoire Naturelle, CNRS, Sorbonne Université, Paris, France
In this review we assess the state of knowledge for the coralline algae of the Mediterranean Sea, a group of calcareous seaweeds imperfectly known and considered highly vulnerable to long-term climate change. Corallines have occurred in the Mediterranean area for ~140 My and are well-represented in the subsequent fossil record; for some species currently common the fossil documentation dates back to the Oligocene, with a major role in the sedimentary record of some areas. Some Mediterranean corallines are key ecosystem engineers that produce or consolidate biogenic habitats (e.g., coralligenous concretions, Lithophyllum byssoides rims, rims of articulated corallines, maerl/rhodolith beds). Although bioconstructions built by corallines exist virtually in every sea, in the Mediterranean they reach a particularly high spatial and bathymetric extent (coralligenous concretions alone are estimated to exceed 2,700 km2 in surface). Overall, composition, dynamics and responses to human disturbances of coralline-dominated communities have been well-studied; except for a few species, however, the biology of Mediterranean corallines is poorly known. In terms of diversity, 60 species of corallines are currently reported from the Mediterranean. This number, however, is based on morphological assessments and recent studies incorporating molecular data suggest that the correct estimate is probably much higher. The responses of Mediterranean corallines to climate change have been the subject of several recent studies that documented their tolerance/sensitivity to elevated temperatures and pCO2. These investigations have focused on a few species and should be extended to a wider taxonomic set. Phylogeography, genomics, transcriptomics, and associated microbiomes are fields in which the information for Mediterranean corallines is very limited. We suggest that future work on Mediterranean corallines should be based on a multidisciplinary perspective combining different approaches, and that it should consist of large-scale efforts by scientists based both in western and eastern Mediterranean areas.
Introduction
The Mediterranean is the largest (2,969,000 km2) and deepest (average 1,460 m, maximum 5,267 m) enclosed sea on Earth (Coll et al., 2010). Although it represents only 0.82% in surface area and 0.32% in volume of the world oceans (Bianchi and Morri, 2000), it is a well-known hotspot of marine biodiversity, with not <20,000 species recorded (Pascual et al., 2017). Such diversity originates from its complex paleoceanographic history and changes in its paleogeographic configurations (particularly through the Cenozoic) and from the current diversity of oceanographic conditions among different regions of the basin (Bianchi and Morri, 2000). The events that characterized the geological history of the Mediterranean in the last 15 My produced a high number of endemic species (which led Bianchi and Morri, 2000 to define the Mediterranean “a factory designed to produce endemics”).
The Mediterranean, however, is also highly impacted and threatened. Climatic models predict that the Mediterranean basin will be one of the regions most affected by the ongoing warming trend and by an increase in extreme events (Lejeusne et al., 2010; Galli et al., 2017). These predictions are supported by climatological data: the average maximum summer seawater temperature has risen by 1°C in 20 years in some areas of the western Mediterranean (Marbà and Duarte, 2010) and a 0.4°C warming per decade since 1986 was reported for the entire Mediterranean Sea by Sakalli (2017). Seasonal and depth-related warming trends were documented by Nykjaer (2009) and Coma et al. (2009), respectively. Studies that measured and modeled pH changes since preindustrial times in the Mediterranean (Hassoun et al., 2015; Goyet et al., 2016) provided evidence of acidification related to excessive increase of atmospheric CO2. The mean surface pH has decreased by ~0.002 units per year from 1994 to 2006 in the northwestern Mediterranean (Howes et al., 2015; Kapsenberg et al., 2017) and by 0.004 units at the Strait of Gibraltar (Flecha et al., 2015). These trends are projected to continue throughout the twenty-first century.
The impact of climate change on Mediterranean marine biota is expected to be strong, because it will interact with anthropogenic disturbances operating at local scales (e.g., chemical pollution, eutrophication, increase in sediment load, habitat degradation caused by trawling). Mediterranean coastal ecosystems have been exploited by humans for millennia, and have been therefore altered in many ways. Nowadays Mediterranean shores are heavily urbanized and support a high population density; impacts of human activities are proportionally stronger in the Mediterranean than in any other sea of the world (Coll et al., 2010). Habitat loss, degradation and pollution, overexploitation of marine resources and invasions of alien species are the main drivers of change, which in future decades will overlap and interact with climate-related changes.
Coralline algae have existed in the Mediterranean (or in the area corresponding to the present Mediterranean) for ~140 My (Chatalov et al., 2015) and are ubiquitous on modern Mediterranean rocky shores. They are key components in some of the most common Mediterranean benthic communities, such as coralligenous concretions (Figure 1A), Lithophyllum byssoides rims (Figure 1B), maerl/rhodolith beds (Figure 1C), barrens formed at sites subjected to heavy grazing (Figure 1D), rims of Ellisolandia elongata and other articulated corallines (Figure 1E), and the epiphytic assemblage of the seagrass Posidonia oceanica (Figure 1F). In these communities coralline species often play a key role as ecosystem engineers: the accumulation of their calcareous thalli produces bioconstructions that modify the tridimensional structure of the substratum and profoundly influence ecosystem functioning (Bressan et al., 2009; Ingrosso et al., 2018). Mediterranean bioconstructions built by corallines are known as major repositories of biodiversity (e.g., coralligenous communities host not <1,700 animal and algal species; Ballesteros, 2006) and carbonate-producing ecosystems (Cebrián et al., 2000; El Haikali et al., 2004; Canals and Ballesteros, 2007; Bracchi and Basso, 2012). Furthermore, although bioconstructions formed by corallines exist in every sea, in the Mediterranean they reach a particularly high spatial and bathymetric extent (coralligenous concretions alone are estimated to exceed 2,700 km2 in surface; Martin et al., 2014). There is evidence that some of these bioconstructions are undergoing substantial degradation and that the corallines that produce them are suffering a loss of vitality (Laborel et al., 1993; Blanfuné, 2016); in general, observations of bleaching and necroses in Mediterranean corallines have become increasingly common in recent years (Hereu and Kersting, 2016; Basso et al., 2018; Quéré et al., 2019; Figure 2).
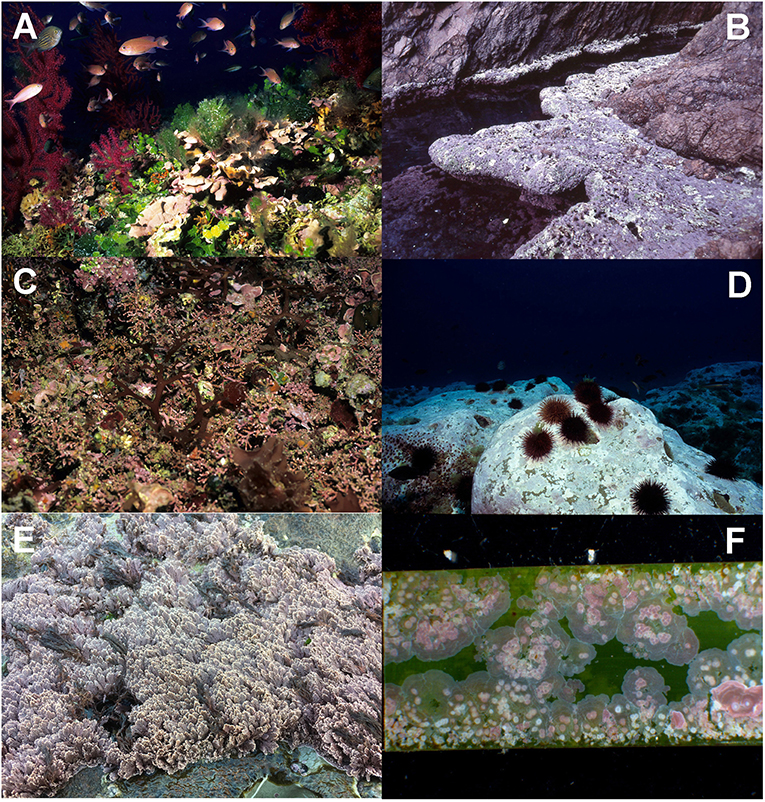
Figure 1. Examples of coralline-dominated habitats in the Mediterranean. (A) A coralligenous community (Islas Columbretes, Spain); copyright: Enric Ballesteros (a2lrZUBjZWFiLmNzaWMuZXM=). (B) A Lithophyllum byssoides rim (Cala Litizia, Scandola Nature Reserve, Corsica, France); copyright: Marc Verlaque (bWFyYy52ZXJsYXF1ZUBtaW8ub3N1cHl0aGVhcy5mcg==). (C) Detail of a maerl bed (Islas Columbretes, Spain); copyright: Enric Ballesteros (a2lrZUBjZWFiLmNzaWMuZXM=). (D) A barren ground dominated by encrusting corallines on a bottom heavily grazed by sea urchins (Alboran Island, Spain); copyright: Enric Ballesteros (a2lrZUBjZWFiLmNzaWMuZXM=). (E) An intertidal rim of articulated corallines (Passetto di Ancona, Italy). (F) Detail of a leaf of the seagrass Posidonia oceanica covered by epiphytic encrusting corallines (Ischia Island, Italy); copyright: Maria Cristina Gambi (Z2FtYmltY0BnbWFpbC5jb20=). All pictures were provided by the copyright owners and reproduced with their permission.
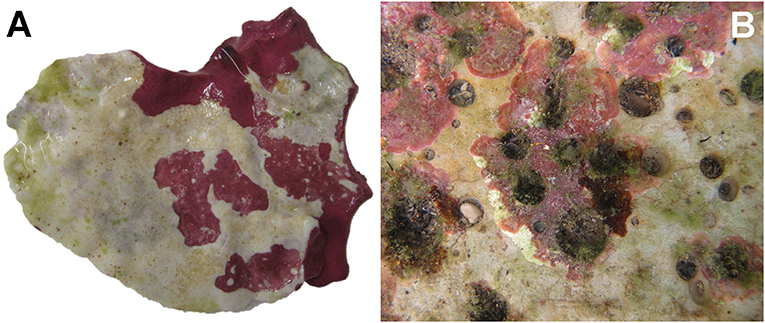
Figure 2. Examples of necroses in Mediterranean corallines. (A) Lithophyllum cabiochae, close-up view of a specimen largely bleached. (B) Encrusting corallines showing incipient bleaching at the edges (Piscinetta del Passetto, Ancona, Italy).
The need of a deep understanding of the biology of Mediterranean corallines is therefore more important than ever. Mediterranean corallines have a long history of study and in the last few decades have attracted great interest from marine biologists. Despite of this, many aspects of their biology are still poorly or insufficiently known. It is also noteworthy that detailed information is available for relatively few species (mainly those shown in Figure 3). It is thus timely to summarize the state of knowledge for these seaweeds and highlight gaps on which future research should concentrate, which we aim to do in this review. This review focuses strictly on the biology of coralline species and does not deal with Mediterranean communities/habitats built or dominated by corallines, for which detailed summaries are already available (coralligenous communities: Ballesteros, 2006; rhodolith beds: Basso et al., 2017; Bracchi et al., 2019a; Lithophyllum byssoides rims: Laborel, 1987; Bressan et al., 2009; Verlaque, 2010; Ellisolandia elongata rims: Laborel, 1987; Ballesteros, 1988; Bressan et al., 2009; epiphytic community of Posidonia oceanica: Piazzi et al., 2015).
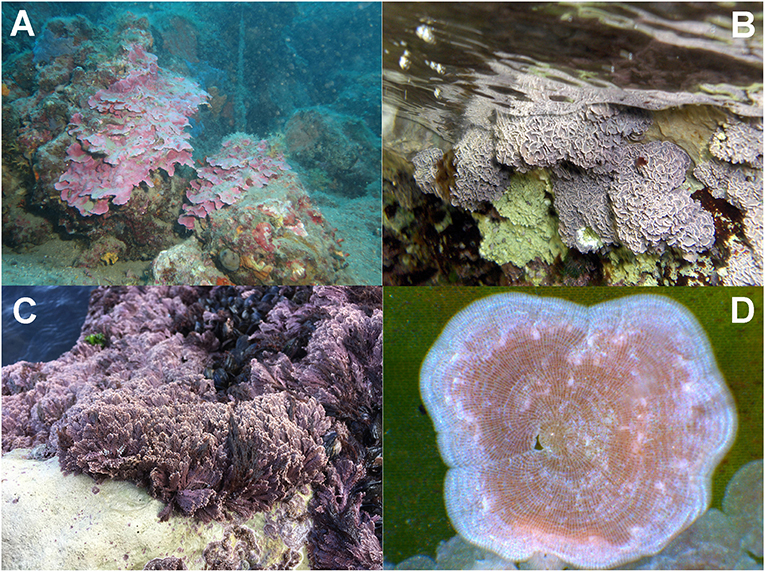
Figure 3. Examples of Mediterranean corallines that have been subject of many studies. (A) Lithophyllum stictiforme, bioconstructor species of coralligenous concretions (reported in many studies as Lithophyllum cabiochae); copyright: Carlo Cerrano (Yy5jZXJyYW5vQHVuaXZwbS5pdA==). (B) Lithophyllum byssoides forms biogenic rims (”trottoirs”) in the intertidal zone; copyright: Sara Kaleb (c2FyYS5rYWxlYkBnbWFpbC5jb20=). (C) Articulated corallines (usually identified as Ellisolandia elongata) are common in the Mediterranean low intertidal and shallow subtidal zones. (D) Pneophyllum fragile, a common epiphyte on the leaves of the seagrass Posidonia oceanica; copyright: Maria Cristina Gambi (Z2FtYmltY0BnbWFpbC5jb20=). All pictures were provided by the copyright owners and reproduced with their permission.
Historical Summary
Being unusual among algae, corallines have long intrigued scientists. If detailed observations were provided in the eighteenth century, corallines were often studied together with other calcified organisms currently classified among animals. Calcareous algae in the Mediterranean were first described more than two centuries ago, and some species of the genus Corallina with distribution encompassing the Mediterranean were among the first seaweeds described with Latin binomials in the Linnaean system (Linnaeus, 1758). Many species that are common in the Mediterranean were described in the late eighteenth and early nineteenth centuries (Ellis, 1768; Ellis and Solander, 1786; Esper, 1796; de Lamarck, 1801; Bory de Saint-Vincent, 1832). The subsequent work carried out until the 70s−80s of the twentieth century consisted mainly of paleontological studies, floristic inventories, traditional taxonomy based on morphology, and descriptive distributional and ecological studies (Bressan, 1974; Bressan and Babbini, 1995, and references therein). Most ecological studies had in fact a general perspective and concerned coralline-dominated habitats (mainly coralligenous concretions and Lithophyllum byssoides rims), rather than coralline biology itself.
A critical review of the literature concerning Mediterranean corallines (results summarized in Figure 4; the list of references used is reported in the File SM1) shows that in the last decades paleontological record, taxonomy and phylogenetic diversity, responses to climate change and physiology are the main aspects on which research has focused. So, in this review we discuss primarily the state of knowledge in these fields. Remarkably, phylogeography, genomics, transcriptomics, study of associated microbial communities and mineralization are fields in which the information for Mediterranean corallines is still very limited.
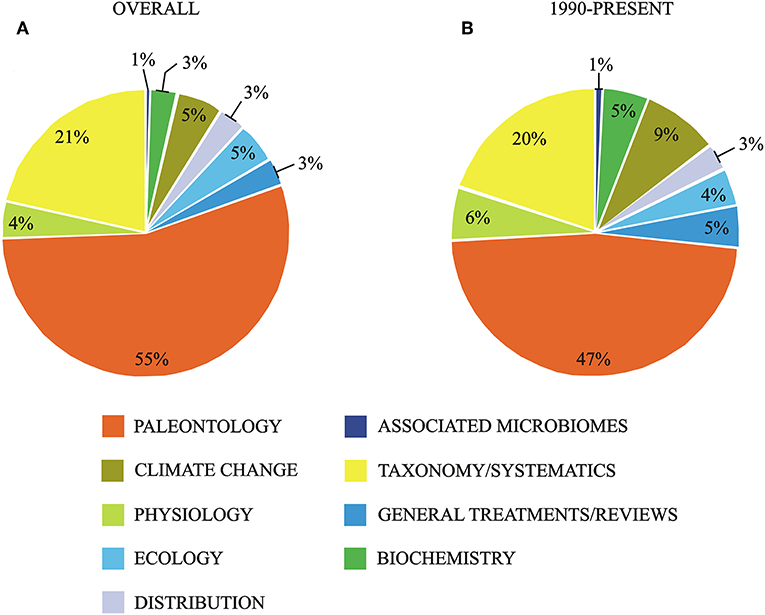
Figure 4. Summary of the literature for Mediterranean corallines based on a critical bibliographic review and subdivided in research fields. (A) Overall information. (B) Information for the period 1990-present. The list of references used for the preparation of the figure (and details about the criteria used for the compilation of the list) is presented in the File SM1.
Geological History of the Mediterranean and Paleontological Record of Mediterranean Corallines
The present-day configuration of the Mediterranean Sea is the result of a complex geological history. The evolutionary history of Mediterranean corallines has taken place in a changing environment with long-term but major changes in paleogeography, climate and oceanography since the early Lower Cretaceous, for about 140 My. The paleogeographic evolution of the Mediterranean is part of the progressive closing and partitioning of the Tethys, the ancient circumtropical ocean, due to the convergence of Southern Hemisphere crustal plates with the northern ones (Scotese, 2014a; Figure 5). The global trends of ocean temperatures during such a long period show an initial rise up to the mid-Cretaceous climate maximum (some 95 Mya) and, since then, temperature has been declining with frequent and sometimes large reversals (Zachos et al., 2001; Friedrich et al., 2012; O'Brien et al., 2017).
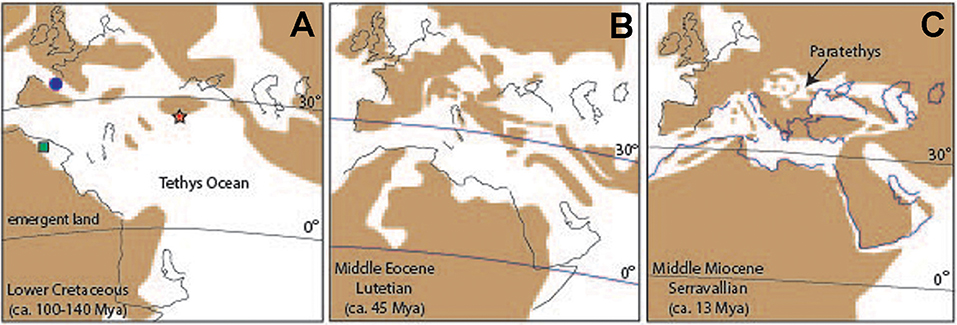
Figure 5. Paleogeographical sketches of the Mediterranean region at three time intervals in the last 140 My. Brown areas: emergent land; white areas: ocean/seas. Black lines: present-day shorelines for geographic reference. Position of Equator (0°) and parallel 30° N at each time. (A,B) The northward movement of Southern Hemisphere crustal plates converging with the Eurasian plates, progressively narrowed and divided the circumtropical Tethys Ocean (modified from Barrier et al., 2018). (C) By the Middle Miocene, the Mediterranean Sea became isolated from the Indo-Pacific and remained connected to the open ocean only at its western end (modified from Rögl, 1998). Location of the first records of Spolithales (red star), Hapalidiales (green square), and Corallinales (blue circle).
A critical review of hundreds of publications on fossil corallines shows that 359 species have been reported in Cretaceous to Pleistocene deposits in different regions of the Mediterranean (File SM2, list of references in File SM6). These can be attributed to the orders Sporolithales (80 species, File SM3), Hapalidiales (159 species, File SM4), and Corallinales (120 species, File SM5). The oldest known fossil corallines attributable to extant orders occur in the Lower Cretaceous (Valanginian, 137 Mya) in northern Greece. They are scarce specimens of Sporolithon that lived on a carbonate platform surrounding an island at the northern margin of the Tethys (Chatalov et al., 2015; Figure 5A). For millions of years, the only recorded corallines are rare encrusting Sporolithon associated to coral reefs (Conrad and Masse, 1989; Arias et al., 1995; Tomás et al., 2007; Bucur, 2008; Woelkerling et al., 2014). In the late Barremian-Aptian (126–113 Mya), the Sporolithales became more frequent and diverse with several extinct genera (Agardhiellopsis, Kymalithon, Paraphyllum) (Moussavian, 1993). In this time interval, there are also poorly contrasted reports of Hapalidiales (Lithothamnion) at the southern margin of the Tethys (Algeria, Lemoine, 1939) and Corallinales (Lithoporella and Corallinoideae) in the marine passage that corresponds to the present-day Pyrenees (Poignant, 1968; Lemoine, 1970). In the Albian (113–100 Mya), the morpho-species diversity of Sporolithales increases and locally they are common components of carbonate rocks (Lemoine, 1970; Moussavian, 1993; Bucur, 1994; Rosales, 1995; Lopez-Horgue et al., 2009). Reports of Hapalidiales (as Lithothamnion and Melobesia) are scarce and inconclusive (Lemoine, 1970; Poignant, 1981). Few scattered records might correspond to geniculate Corallinoideae (Maslov, 1956; Lemoine, 1970). For most of the Upper Cretaceous (Cenomanian-Campanian, 100–72 Mya) coralline algae continue to be generally scarce components of carbonate platforms. The relatively few records scattered all over the region indicate an increase in Sporolithales (mainly of Sporolithon) with the appearance of a new genus in this order, Hemiphyllum, now extinct. Reports of Hapalidiales, both Lithothamnion and Mesophyllum (Lemoine, 1970; Poignant, 1981), are poorly illustrated and remain doubtful. Within Corallinales, the first occurrence in the region of reliable representatives of Neogoniolithoideae sensu Rösler et al. (2016) is recorded (Spongites as Lithophyllum, Bucur and Baltres, 2002). In the Maastrichtian (72–66 Mya), the last stage of the Cretaceous, the number of Hapalidiales increases (Poignant, 1978, 1981). The oldest-known Hydrolithoideae (Karpathia, Bassi et al., 2005 and references therein) also appear in this time interval, together with a few more Corallinales (Rösler et al., 2017).
The Tethys narrowed during the Cretaceous in its western end with the progressive convergence of African and European plates, but in the Paleocene (66–56 Mya) an uninterrupted marine connection continued to exist at low latitudes around the globe. The partial emergence of the Pyrenees and large areas of western Europe reduced the passages from the Tethys to the Atlantic. Two-thirds of the coralline species disappeared during the Cretaceous mass extinction (Aguirre et al., 2000a,b), but the number of recognized morpho-species recovered and substantially increased during the Paleocene (Aguirre et al., 2000a,b, 2007). This recovery was mainly due to the increase in Hapalidiales (Lithothamnion, Mesophyllum, and Phymatolithon) and Corallinales, whilst the Sporolithales started a decrease that continued throughout the Cenozoic with minor reversals. All genera of Sporolithales other than Sporolithon recorded in Cretaceous rocks disappeared before the Paleocene. Corallines from this epoch occur in coral-bearing carbonates, either in situ in shallow-water paleoenvironments (e.g., Moussavian, 1993; Aguirre et al., 2007) or re-deposited in deeper settings (Stockar, 2000).
As a marked reversal of the general global cooling trend initiated in the mid-Cretaceous, during the Early and Middle Eocene epochs (56–38 Mya) global temperatures were higher than in older and younger Cenozoic times (Zachos et al., 2001, 2008; Anagnostou et al., 2016). During this warm interval, large coral reefs disappeared from low latitudes (Scheibner and Speijer, 2008; Kiessling, 2010; Perrin and Kiessling, 2010). The global sea level was several tens of meters higher than in the modern ocean (Miller et al., 2011), favoring the development of extensive carbonate platforms dominated by larger benthic foraminifers and algae (Nebelsick et al., 2005; Scheibner and Speijer, 2008; Norris et al., 2013). The emergence of the antecedent reliefs of the Alpine mountain belts (Alps, Carpatian mountains) as many small islands caused a complex paleogeography of the northern margin of the Tethys, which extended north of the modern Black and Caspian seas (Scotese, 2014b; Figure 5B). Coralline algae occurred in rhodolith beds and in bioclastic facies in platform deposits or in deep-water, re-worked sediments (e.g., Aroldi and Bucur, 2002; Nebelsick et al., 2005). Numbers of reported species and relative proportions of coralline orders did not change throughout this interval (Aguirre et al., 2000a).
In the Late Eocene (38–34 Mya) global temperatures and sea level progressively decreased (Zachos et al., 2001; Miller et al., 2011). In addition to rhodolith beds (and maerl) and bioclastic facies, coralline algae of this age occurred associated with corals in shallow water carbonates (Bassi, 1998; Rasser, 2000; Nebelsick et al., 2005; Barattolo et al., 2007) at both margins of the Tethys in the Mediterranean region. The number of reported morpho-species of Hapalidiales and Corallinales slightly increased, whereas Sporolithales resumed their diversity decline after a long interval of stability.
The most dramatic global cooling of the Cenozoic era took place at the Eocene–Oligocene transition (at 34 Mya). Development of Antartic ice sheets and onset of glaciation marked the beginning of the modern icehouse world (Zachos et al., 2001). A significant sea-level drop was coeval of these processes. The continued rising of Alpine belts led to the separation of the Paratethys, a large marine body extending to the north of the Alps, from the Rhône Basin over eastern Europe to the east of the modern Caspian Sea (Popov et al., 2004). Since the Oligocene (34–23 Mya) the Paratethys was an individual paleobiogeographic province with connections to the Tethys/Mediterranean Sea during most of its paleogeographic evolution (Harzhauser and Piller, 2007). The marine connections remained continuous from the present-day Indo-Pacific to the North Atlantic oceans (Rögl, 1998).
In the majority of localities, Oligocene corallines occur in coral reef deposits or in laterally associated sediments as rhodoliths, coralline debris, or as crusts directly growing on the soft sea floor (Fravega et al., 1987; Brandano, 2017). Occasionally, rhodoliths and algal debris have also contributed as important carbonate producers in late Oligocene homoclinal carbonate ramps in the western Tethys (Bover-Arnal et al., 2017). They also occur in deep-water, re-deposited carbonates (Rasser and Nebelsick, 2003). Most reports of Oligocene coralline algae derive from the northern margin of the Western Tethys, especially from the Piedmont and Ligurian basins, and the circumalpine area (north-eastern Italy, northern Slovenia, Austria and southern Bavaria) (Nebelsick et al., 2005). Hundreds of species of Oligocene corallines were described in these areas in the last century (Airoldi, 1932; Conti, 1950; Mastrorilli, 1968; Fravega et al., 1987; further references in Braga et al., 2010). Long species lists were also reported from the Balkan and Carpatian mountains (Lemoine, 1977; Bucur et al., 1989). Oligocene corallines were also described from Algeria at the southern margin of the Western Tethys (Lemoine, 1939) and from ancient islands in the Tethys, such as Maltese Islands (Brandano, 2017), Salento Peninsula, southern Italy (Bosellini and Russo, 1992), and the Malaguide Complex in southern Spain (Braga and Bassi, 2011). Titanoderma pustulatum occurred in the early Oligocene from NW Iran (Basso et al., 2019), an area connecting the Indian Ocean and the Tethys before its final closure in the middle Miocene. This new finding places the oldest record of the Titanoderma/Lithophyllum pustulatum group in this area rather than in the late Oligocene from the Central Pacific as previously recorded (Bassi et al., 2009). Reported diversity of morpho-species of Corallinales and Hapalidiales continued the rise initiated in the late Eocene. By contrast, the number of species of Sporolithon decreased (Aguirre et al., 2000a).
Continued convergence of African and European plates and emergence of Alpine mountain belts led to significant palaeoceanographic changes during the Miocene (23–5.3 Mya). The long-lasting circum-tropical ocean was partitioned by land masses, changing the global geography and major current patterns. The connections of the Mediterranean to the Indian Ocean were interrupted in the middle Miocene (at about 14 Mya, Rögl, 1998) by the emergence of the Middle East reliefs. The Paratethys was strongly isolated during the late Miocene and its European sub-basins became continental (Rögl, 1998; Popov et al., 2004; Harzhauser and Piller, 2007). At the western end of the Mediterranean, uplift of the Betic-Rifean reliefs gradually closed the seaways to the Atlantic Ocean. The connections were temporarily interrupted during the Messinian Salinity Crisis at about 5.5 Mya (Hsü et al., 1977). The opening of the Gibraltar Straits established the present-day configuration of Mediterranean Sea as a partially isolated, evaporitic water body, which needs inflow from the Atlantic Ocean to maintain its level (Mariotti et al., 2002). A global warm phase, the Mid-Miocene Climatic Optimum (about 15 Mya), took place after a brief glaciation interval in the earliest Miocene. Since that phase, the Earth's climate has been cooling with reversals (Zachos et al., 2001). The Northern Hemisphere ice-sheets started growing in the late Miocene. During the Miocene, global sea-level fluctuations of 50–60 m on the million-year scale and superimposed oscillations controlled by orbital obliquity (41,000 year cycles) did not show a marked ascending or descending trend (Miller et al., 2011).
Rhodolith beds were widespread in Miocene platform carbonate and siliciclastic deposits in the Mediterranean and Paratethys (Halfar and Mutti, 2005; Braga, 2017). They were most common in carbonate ramps together with small coral buildups, and locally they occurred in reef-rimmed shelf deposits (Hrabovský et al., 2016; Braga, 2017). Coralline biostromes (coralligène de plateau) were reported in the Maltese Islands (Bosence and Pedley, 1982). Rhodoliths and coralline debris also occur as deep re-deposited sediments (Bassi et al., 2017).
The number of fossil non-geniculate coralline species reported in the literature reached its maximum in the early Miocene and then decreased with small reversals (Aguirre et al., 2000a). Some available modern accounts based on comparable diagnostic characters seem to confirm a morphospecies richness reduction throughout the Miocene. While Checconi (2006) describes 35 species in the early Miocene (Burdigalian) of the Southern Apennines (Italy), only 21 morphospecies were found in the late Miocene (Messinian) reefs in Salento and Sorbas Basin together (Braga et al., 2009). The reduction is even more marked within some genera, such as Spongites, encompassing 13 species during the Burdigalian (Checconi, 2006) and only 5 during the Messinian in southern Italy (Braga et al., 2009). Lithophyllum (and Lithophyllum gr. pustulatum –Titanoderma, Bassi et al., 2009) is first recorded in the Mediterranean region in the early Miocene from SE France (Burdigalian; Coletti et al., 2018a). In the Miocene, for the first time in the evolutionary history of corallines, Mediterranean assemblages differ from their pantropical counterparts at the genus level (Braga et al., 2010). Spongites, Neogoniolithon, and Lithophyllum are the main components in shallow-water Miocene Mediterranean paleoenvironments, including coral reefs. Porolithon, Hydrolithon gr. boergesenii, and Aethesolithon J.H. Johnson, which occur with those genera in coral reefs in low latitudes, are absent in the Mediterranean basins. This biogeographical differentiation was probably due to the isolation of the Mediterranean from the Indian Ocean, complete by the middle Miocene (Rögl, 1998; Figure 5C). Although coral reefs persisted in the Mediterranean until the latest Miocene, after its eastern closure the coral diversity decreased (Bosellini and Perrin, 2008). The strong decline of species number of Sporolithon, a genus of tropical affinity, after the middle Miocene, might also be due to the isolation from the Indian Ocean (Braga and Bassi, 2007).
The general configuration of the modern Mediterranean was established in the Pliocene (5.3–2.6 Mya). Paleogeography within the basin, however, changed substantially due to the uplift of modern reliefs, with a progressive reduction of the areas invaded by the sea. Global temperatures continued the decrease initiated in the middle Miocene (Zachos et al., 2001) and sea level followed a falling trend modulated by oscillations with amplitude of several tens of meters (Miller et al., 2011).
Rhodolith beds are scarcer than in Miocene rocks but have been reported in southern Spain (Aguirre, 1998; Aguirre and Jiménez, 1998; Aguirre et al., 2012) and northern Italy (Vannucci et al., 1996; Checconi et al., 2007). Corallines also occur in vermetid reefs in early Pliocene deposits of northeastern Spain (Aguirre et al., 2014). Here, Spongites fruticulosus is the main coralline, differently from modern Mediterranean vermetid reefs, in which Neogoniolithon brassica-florida is the dominant species (Boudouresque, 2004; Langar et al., 2011). The Pliocene coralline morphospecies in the few published accounts are similar to those of the present-day Mediterranean. More than 90% of the 19–20 species identified in early Pliocene in southern Spain (Aguirre et al., 2012) and 88% of the 8 reported in the late Pliocene of Tuscany (Italy) (Checconi et al., 2007) are living in the Mediterranean and Lusitanian provinces.
Except in areas with intense recent uplift such as Sicily, the Quaternary (last 2.6 My) shorelines of the Mediterranean at high sea levels were close to the present-day situation. The few Pleistocene and Holocene inventories suggest that Mediterranean Quaternary corallines were not different from the modern ones. They occurred in build-ups (coralligène de plateau) (Nalin et al., 2006; Coletti et al., 2018b; Bracchi et al., 2019b), in rhodolith beds, and in rhodoliths and debris dispersed in siliciclastic deposits (Di Geronimo, 1998; Coletti et al., 2018b). The dramatic global climatic and sea level changes that took place repeatedly during the Pleistocene (e.g., Rohling et al., 2014) did not substantially affect coralline assemblages in the Mediterranean, although a few species might have migrated into the Mediterranean from the North Atlantic in the colder periods (Di Geronimo, 1998).
Floristic Diversity
Due to the long history of taxonomic studies, it is not surprising that a plethora of names (both species and intraspecific taxa) has accumulated for Mediterranean corallines. The nomenclatural history of these algae is very complicated and would be impossible to summarize in a concise form. A comprehensive summary of the names available and information about their taxonomic validity can be found in the account of Cormaci et al. (2017) and in AlgaeBase (Guiry and Guiry, 2019). Here we follow primarily the taxonomy of Cormaci et al. (2017). These authors reported for the Mediterranean 57 species; with the addition of Lithophyllum yessoense (recorded by Verlaque, 2001), Pneophyllum cetinanensis (freshwater species described by Žuljević et al., 2016) and Lithophyllum nitorum (recorded by Peña et al., 2018), the Mediterranean coralline flora as currently known consists of 60 species (Table 1). The genus Lithophyllum is the species-richest (16 species), followed by Mesophyllum (6 species), and Amphiroa, Jania, and Lithothamnion (5 species each). Twenty-seven species were originally described based on Mediterranean material (see type localities in Table 1). For details of morphology and habitat for each species, see Irvine and Chamberlain (1994), Bressan and Babbini (2003), and Cormaci et al. (2017).

Table 1. Species of coralline algae reported for the Mediterranean Sea and currently regarded as taxonomically valid.
Molecular Studies of Mediterranean Corallines
Taxonomy, Phylogeny, and Geographical Distribution
There are well-founded reasons to believe that the current estimate of 60 species is an underestimation of the real species number. The taxonomy of Mediterranean corallines so far has been based almost entirely on morphological data. Species circumscriptions have been based on gross morphology and morpho-anatomical characters observed in light and electron microscopy (Hamel and Lemoine, 1953; Huvé, 1962; Bressan, 1974; Bressan et al., 1977; Boudouresque and Verlaque, 1978; Woelkerling, 1983, 1985; Woelkerling et al., 1985; Athanasiadis, 1989, 1995, 1997, 1999a,b; Basso, 1995; Bressan and Babbini, 1995; Basso et al., 1996, 2004, 2011; Furnari et al., 1996; Chamberlain, 1997; Cabioch and Mendoza, 1998, 2003; Bressan and Cabioch, 2004; Basso and Rodondi, 2006; Athanasiadis and Neto, 2010; Kaleb et al., 2011, 2012; Cormaci et al., 2017).
In recent decades, DNA sequence data have become a widespread tool in coralline taxonomy and have plaid an increasingly important role in species circumscriptions. After the first studies published around the end of the last century (Bailey and Chapman, 1996, 1998), the last decade has seen an exponential increase of DNA-based phylogenetic and taxonomic studies. Investigations of molecular-assisted alpha taxonomy (MAAT) have now become the normality in coralline taxonomy and systematics. This approach uses molecular markers to assign collections to genetic groups followed by detailed morphological observations (Hind et al., 2014). Such new information has revolutionized our understanding of coralline diversity and evolution (Nelson et al., 2015) and has drawn a new scenario in which some points are now well-established. First, coralline algae are characterized by high levels of cryptic diversity. The genetic diversity of these organisms unraveled by DNA sequence data is much higher than indicated by morpho-anatomical data, both in geniculate and non-geniculate species. Cryptic diversity has been shown to abound in marine macroalgae (De Clerck et al., 2013; Verbruggen, 2014); although corallines have a morpho-anatomical structure that offers more characters for species discrimination compared to other red seaweeds, they are perhaps the group of rhodophytes in which this situation is most pervasive (Pezzolesi et al., 2019). Second, many morpho-anatomical features traditionally used to identify corallines are not reliable for identification purposes. Therefore, a taxonomy based entirely or mostly on these characters (as it is the case for the Mediterranean) is likely to be misleading, with substantial risk of misidentifications and underestimation of species numbers (e.g., for the genus Lithophyllum Hernandez-Kantun et al. (2016) estimated a species diversity likely two to four times greater than currently estimated in each geographic region). Third, special care must be used in the application of species names. When cryptic diversity is demonstrated and a morphospecies turns to represent a complex of cryptic species, it may be very difficult to decide to which cryptic species the Linnaean binomial is correctly assigned. This may create great confusion when different cryptic species have different geographical distributions. Considering this problem, in absence of molecular data the practice of identifying specimens from a certain geographical region with names of species described from widely separated regions should be abandoned (Pezzolesi et al., 2019).
The first study presenting molecular data for Mediterranean corallines was published by Walker et al. (2009). These authors presented cox1 and SSU rRNA sequences for 2 samples of Corallina ferreyrae (as Corallina elongata) from northern Greece in their taxonomic study of European articulated corallines. At present, there are in GenBank 1153 sequences obtained from Mediterranean corallines, which were produced in 18 different studies (among which De Jode et al., 2019 provided the largest contribution, 812 sequences; Table S1). These sequences were generated by PCR amplification and Sanger sequencing of selected markers. The plastid gene of the photosystem II protein D1 (psbA), the mitochondrial COI-5P fragment of the cytochrome c oxydase I gene, the mitochondrial cox2,3 spacer, and the nuclear genes of the small subunit (SSU) and large subunit (LSU) of the ribosomal RNA have been the main markers used (Table S1). To date, the transcriptomic data presented by De Jode et al. (2019) are the only molecular data other than Sanger sequences produced from Mediterranean corallines.
Of the 18 studies, only 8 focused totally or mainly on Mediterranean taxa: Hernandez-Kantun et al. (2015a); Peña et al. (2015a); Wolf et al. (2015, 2016); Žuljević et al. (2016); Pezzolesi et al. (2017, 2019), and De Jode et al. (2019). Five of these (Peña et al., 2015a; Žuljević et al., 2016; Pezzolesi et al., 2017, 2019; De Jode et al., 2019) provided most of the DNA sequence data available (Table S1) and have particularly contributed to our current understanding of the genetic diversity of Mediterranean corallines.
The integrative study of Peña et al. (2015a) on the genus Mesophyllum, based on molecular (COI-5P, psbA) and morphological data redefined the distribution of four species in Atlantic Europe and the Mediterranean. M. expansum, M. macroblastum, and M. sphaericum were detected in the Mediterranean. The results suggested that M. expansum is a major contributor to bioconstruction of coralligenous concretions and occurs also in the Atlantic Iberian Peninsula and Macaronesia. This species was found from the intertidal to −50 m, with a positive correlation between depth and the maximum sea surface temperature, suggesting that this species may mitigate future consequences of global warming by changes in depth profile. By contrast, M. macroblastum appears to be restricted to the Mediterranean. Two Mesophyllum species reported in the Mediterranean literature (M. lichenoides and M. alternans) were not recorded and their presence in the Mediterranean requires confirmation based on molecular data. The Atlantic Iberian M. sphaericum was first reported in the Mediterranean, under two different growth-forms (rhodolith and crustose).
The study of Pezzolesi et al. (2017) on the intertidal bioconstructor Lithophyllum byssoides was the first phylogeographic investigation of a Mediterranean coralline. Using psbA and cox2,3 sequences, these authors unraveled a high haplotypic diversity and detected 7 lineages, whose geographical distribution did not follow the main biogeographical boundaries recognized in the central Mediterranean. For several lineages the distribution was restricted to one or few sites. The results also showed a clear genetic differentiation between Mediterranean and Atlantic Lithophyllum byssoides, suggesting a likely separation at species level (which could not be confirmed due to insufficient data from the Atlantic).
The two recent studies of Pezzolesi et al. (2019) and De Jode et al. (2019) unraveled a striking case of cryptic diversity: the Lithophyllum stictiforme complex. Lithophyllum stictiforme and Lithophyllum cabiochiae have been long known as common species in the Mediterranean subtidal, where they are major contributors to the formation of coralligenous concretions (Athanasiadis, 1999a; see also Boudouresque and Verlaque, 1978; Furnari et al., 1996). Whereas some authors have separated these species, others (Cormaci et al., 2017; Guiry and Guiry, 2019) consider L. cabiochiae and L. stictiforme conspecific. Pezzolesi et al. (2019), sequencing three markers (cox2,3, psbA, rbcL) in samples collected from many sites in the western and central Mediterranean, concluded that Lithophyllum stictiforme represents a complex of at minimum 13 cryptic species. De Jode et al. (2019), combining Sanger sequencing (COI, psbA, LSU) and transcriptomics, reached very similar conclusions, showing that on the French coasts specimens with the L. stictiforme/L. cabiochiae morphology represent a complex of 8 cryptic species. De Jode et al. (2019) demonstrated also the reproductive isolation of these species and documented differences in their depth range. Both Pezzolesi et al. (2019) and De Jode et al. (2019) noted that for several cryptic species the geographical distribution appears restricted to one particular area or site.
Pezzolesi et al. (2017, 2019) interpreted the patterns observed for L. byssoides and the L. stictiforme complex as consequences of past hydrogeological and climatic events, in combination with modern oceanographic features. The fossil documentation suggests that these algae have existed in the Mediterranean, respectively, since the Messinian (7.3–5.3 Mya, Braga and Aguirre, 2001; Braga et al., 2009) and the late Langhian (14.5 Mya, Hrabovský et al., 2016). Spatial fragmentation of populations that took place during the Messinian Salinity Crisis (5.96–5.33 Mya) may have stimulated genetic differentiation, and, in the case of the Lithophyllum stictiforme complex, allopatric speciation. Subsequent climatic events such as the Quaternary glaciations (2.6 Mya to present), which also altered the Mediterranean coastline and partially separated different sectors, probably further contributed to shape the current distribution of these algae.
The relevance of past climatic events in the diversification of Mediterranean corallines is highlighted by a discovery of particular interest made in recent years, i.e., the first known freshwater coralline alga: Pneophyllum cetinaensis, endemic to the Cetina River, Croatia (Žuljević et al., 2016). This species, which is fully adapted to freshwater conditions, descends from an ancestor that was preadapted to changes in water salinity produced in landlocking events such as the last glaciation (120,000–20,000 years ago). In addition, molecular data obtained in this study exclude a close phylogenetic relationship between the freshwater P. cetinaensis and other Mediterranean and Atlantic Pneophyllum species.
Among other studies, Hernandez-Kantun et al. (2015a) reassessed the taxonomic identity of the generytype species Lithophyllum incrustans using a partial rbcL sequence obtained from the lectotype specimen (epizoic on a sea snail shell, collected in Sicily). The results re-defined the distribution of L. incrustans and highlighted that this is mainly a subtidal species. The presence in the Mediterranean of Lithophyllum hibernicum was also demonstrated. Additional data were provided by Bittner et al. (2011), who sequenced 9 unidentified samples of Mediterranean corallines in their phylogenetic assessment of the order Corallinales (psbA and, in part, COI-5P). Rösler et al. (2016) published 21 new sequences for Mediterranean collections, including one for a specimen therein designated as epitype of Spongites fruticulosus. Additional data produced new records for the Mediterranean flora: Harveylithon rupestre (as Hydrolithon rupestre, Wolf et al., 2015); Phymatolithon lusitanicum (Peña et al., 2015b); the attached encrusting form of the maerl-forming Phymatolithon calcareum (Wolf et al., 2016); and Lithophyllum nitorum (Peña et al., 2018). Finally, some sequences of Mediterranean fossil corallines were published by Hughey et al. (2008).
Molecular Data and Application of Taxonomic Names in Mediterranean Corallines
In situations where cryptic diversity is discovered, the only definitive solution for a correct application of Linnaean names is to obtain sequences from the type specimen, the only one to which a species name is unambiguously attached. In this way, the type specimen can be linked to one of the lineages recovered in molecular phylogenies, and the species name will therefore be attached to that lineage. Fortunately, in the case of coralline algae this approach has generally worked. In air-dried coralline specimens, DNA is preserved in a form that is often adequate to obtain partial sequences for one or more markers (either by high-throughput sequencing methods or by nested PCR performed with adequate equipment). Sequences of sufficient quality have been produced from many type specimens, allowing an accurate assessment of many species (e.g., Adey et al., 2015; Hernandez-Kantun et al., 2015a, 2016; Hind et al., 2016; Richards et al., 2017, 2018; Gabrielson et al., 2018; Peña et al., 2018). Conversely, for some species to obtain sequences from type specimens is impossible (either because the type material is in very limited amount, or because it is formol-preserved). In these cases, a different specimen should be sequenced and used as molecular reference for the species. Such specimen should be collected at the type locality, and the details of its morphology and habitat should be in agreement with the protologue of the original description. If the nature of the original type is ambiguous, this specimen can be designated as epitype following the article 9.9 of the ICN (Turland et al., 2018).
For corallines occurring in the Mediterranean, an assessment based on sequences generated from type specimens has been made for 11 species. Sequences were obtained from holotypes, lectotypes, or isotypes for Corallina ferreyrae (Bustamante et al., 2019; previously reported in the Mediterranean as Corallina caespitosa, Walker et al., 2009), Lithophyllum incrustans (Hernandez-Kantun et al., 2015a), Lithophyllum nitorum (Peña et al., 2018), Lithophyllum stictiforme (Pezzolesi et al., 2019), Mesophyllum sphaericum (Peña et al., 2011), Phymatolithon lusitanicum (Peña et al., 2015b), and Pneophyllum cetinanensis (Žuljević et al., 2016). For Spongites fruticulosus, Rösler et al. (2016) generated SSU, LSU, COI, and 23S sequences from the epitype specimen. For Corallina officinalis and Ellisolandia elongata, Brodie et al. (2013) designated epitype specimens, from which they obtained cox1 and rbcL sequences. Finally, Hernandez-Kantun et al. (2015b) produced SSU and psbA sequences from the neotype of Phymatolithon calcareum (designated by Woelkerling and Irvine, 1986).
Present-Day Distribution and Biogeography
Our knowledge of the distribution of Mediterranean corallines is largely based on records derived from morpho-anatomical identifications (see Bressan and Babbini-Benussi, 1996, for a synthesis of the information available until the late 90s of the last century). Six species were recorded in recent years based on molecular data: Corallina ferreyrae, reported as the heterotypic synonym C. caespitosa (Walker et al., 2009), Harveylithon rupestre (Wolf et al., 2015), Lithophyllum hibernicum (Hernandez-Kantun et al., 2015a), Lithophyllum nitorum (Peña et al., 2018), Mesophyllum sphaericum (Peña et al., 2015a), and Phymatolithon lusitanicum (Peña et al., 2015b).
Based on the present knowledge, 7 species are Mediterranean endemics (Amphiroa rubra, Lithophyllum trochanter, L. woelkerlingii, Lithothamnion minervae, L. valens, Mesophyllum macedonis, Pneophyllum cetinaensis) (Table 1). In terms of distribution, it is possible to recognize three main groups. Some species are clearly widespread throughout the Mediterranean, having been recorded in all regions of the basin. This group includes 27–28 species, which usually also occur on Atlantic European coasts and in Macaronesia; examples are Ellisolandia elongata, Hydrolithon farinosum, Jania rubens, Lithophyllum incrustans, and Pneophyllum fragile. A smaller number of species (16–17) occurs only in the western and central Mediterranean, or is much more common there than in the eastern Mediterranean. Examples are Choreonema thuretii, Mesophyllum macroblastum, Lithophyllum byssoides, L. dentatum, and Lithothamnion valens. Finally, two species (Lithophyllum trochanter and Tenarea tortuosa) are clearly associated with the warmer parts of the basin and reliable records refer mostly to the eastern Mediterranean. At present, two species are considered introduced. Lithophyllum yessoense was recorded by Verlaque (2001) from the Lagune de Thau (Hérault, France), a well-known hotspot of introduction of alien species; the species was probably introduced from the North Pacific by transfer of Asian oysters. Harveylithon rupestre was recorded by Wolf et al. (2015) from Vis Island (Croatia) (as Hydrolithon rupestre). These authors believed that the species was probably introduced in the area by shellfish aquaculture activities.
It can be expected, however, that future studies incorporating molecular data will lead to geographical reassessments for many species. The main change that we expect is an increase in the number of endemic species. This expectation is based on theoretical grounds (the hydrogeological history of the Mediterranean in the last 15 My and the high number of endemics in many animal groups) and on the results of some recent studies. For the Lithophyllum stictiforme complex, De Jode et al. (2019) and Pezzolesi et al. (2019) discovered numerous cryptic species for which the present known distribution is restricted to the Mediterranean. Although some of these species surely will be shown to occur in neighboring Atlantic regions, we feel that almost certainly several others will turn to be real Mediterranean endemics. Lithophyllum byssoides is another candidate for Mediterranean endemicity: Pezzolesi et al. (2017) showed that Mediterranean samples of this species are genetically distinct from Atlantic samples to an extent that may justify separation at species level. If future studies incorporating additional extra-Mediterranean samples confirm this separation, the Mediterranean population will have to be considered an endemic species. The same situation applies to Mesophyllum macroblastum: Peña et al. (2015a) noted that extra-European specimens identified with this name were resolved in molecular analyses as different species from Mediterranean specimens (which can be considered the real M. macroblastum: the type locality is the Gulf of Naples, Italy); thus, the occurrence of this species out of the Mediterranean has to be definitively demonstrated yet. Pardo et al. (2015), in a taxonomic investigation focusing on Corallina and Ellisolandia of the Atlantic Iberian Peninsula, discovered a species of Corallina with distribution encompassing Atlantic and Mediterranean shores (for which they did not provide a formal description and named it Corallina sp.2). Pardo et al. (2015) remarked that Atlantic and Mediterranean forms of this species showed clear morphological differences and had different COI-5P haplotypes. It cannot be discounted that future studies will lead to separation at species level between the two forms; in that case, the Mediterranean form would probably be an endemic Mediterranean. Based on these examples we suggest that, for all species originally described from the Mediterranean, the distribution should be reassessed using DNA sequence data produced from the whole geographic range.
Reproductive Biology
The life histories of Mediterranean corallines have not been investigated in detail using culture studies. Evidence based on observation of field-collected material suggests that these algae have the triphasic life history typical of later-divergent florideophytes, with one haploid generation reproducing sexually (the gametophyte) and two diploid generations reproducing asexually by spores (the carposporophyte and the tetrasporophyte) (Graham et al., 2018).
Nearly all information available on the reproductive biology of Mediterranean coralline is based on observation of reproductive specimens in the field and in the laboratory. The only information based on molecular evidence has been provided by De Jode et al. (2019). Analyzing their multilocus genotypes for clonality, these authors documented sexual reproduction in the Lithophyllum stictiforme/cabiochiae complex and demonstrated sexual isolation among the 8 cryptic species occurring on French shores. This is to date the only study providing robust support for the biological species concept in a taxon of Mediterranean corallines.
In general, observations of reproductive specimens/structures in Mediterranean corallines are not infrequent. Bressan and Babbini (2003) provided general information about the reproductive periods for many species (i.e., reported the months in which a species is reproductive); however, they did not specify the source of this information and if they referred to sexual or asexual phases. Most observations of reproduction available in the literature have been published as records of reproductive specimens in field investigations, reporting the reproductive structures observed (spermatangia, carposporangia, tetrasporangia, bisporangia; or sexual vs. asexual conceptacles) (Feldmann, 1939; Cecere et al., 1996; Cormaci et al., 1997, 2000; Catra et al., 2006; Falace et al., 2011).
Most records are based on observations made in a limited timespan. Investigations over extended periods are more useful to define temporal patterns and understand the environmental factors influencing reproduction, but unfortunately are less frequent. Vatova (1948) summarized the reports of fertility available for the seaweeds of the area of Rovinj (Croatia). Gómez-Garreta et al. (1982) studied for 2 years the phenology of the most common seaweeds, including several coralline species, in four benthic communities in the Balearic Islands. Soto and Conde (1989) made similar observations over a 5 years period at several sites in southeastern Spain. Overall, inconsistent patterns were found in these studies, i.e., different reproductive periods were reported for the same species in the different areas considered. A common aspect, however, is that reproductive specimens were more frequently recorded in articulated species (Amphiroa spp., Jania spp., Ellisolandia elongata) than in encrusting species (possibly because observation of conceptacles in encrusting forms is generally more difficult, especially in the field). Another common feature (remarked by Soto and Conde, 1989) is that asexual structures were much more frequent than sexual (i.e., sporangial conceptacles were more frequently observed than gametangial conceptacles). This has been reported in many field studies of red seaweeds, even Mediterranean (Rindi and Cinelli, 2000) and is considered related to the presumed low rate of fertilization due to absence of flagellate gametes in red algae.
Additional information on reproductive phenology has been reported separately for few species (usually ecologically important). For Lithophyllum byssoides, the reproduction of tetrasporophytes has been reported in March-July at Marseilles and in February-December in the Balearic Islands; the reproduction of gametophytes only in autumn (October) (Verlaque, 2010, based on Huvé, 1956a,b; Gómez-Garreta, 1981; Chamberlain, 1997). The main period of recruitment for this species appears to be the autumn (Verlaque, 2010, according to Huvé, 1954, 1970).
The most detailed studies of reproduction in Mediterranean corallines focused on forms of coralligenous habitats (Garrabou and Ballesteros, 2000; Rodriguez-Prieto, 2016). Garrabou and Ballesteros (2000) studied populations of Lithophyllum and Mesophyllum (identified as L. frondosum and M. alternans, respectively) in a coralligenous community in Catalonia for 2 years. By photographic sampling, they estimated the percentage of reproductive specimens throughout the study period (by observation of mature conceptacles in their images). They found no seasonal trends in Mesophyllum, for which ~25% of the specimens had mature conceptacles in each sampling date. Conversely, in Lithophyllum the percentage of thalli with mature conceptacles was significantly higher in early autumn in both years. Based on these differences and differences in growth patterns, Garrabou and Ballesteros (2000) remarked the ecological differences between the two species, concluding that Mesophyllum has a more opportunistic life strategy, whereas Lithophyllum has a more conservative strategy. Rodriguez-Prieto (2016) carried out experiments in controlled conditions (temperature, daylength and photon irradiance) on Lithophyllum stictiforme from Catalonia. She concluded that an irradiance of 20 μmol photons m−2 s−1 combined with 10–12°C and a 8:16 h light:dark regime was the most favorable condition for the species. In culture, the conceptacles matured in conditions simulating late summer-early autumn, in agreement with the behavior of field specimens. Rodriguez-Prieto (2016) noted that, in culture, maturation and release of reproductive cells are rare events, since no development of new conceptacles was observed after the release of reproductive cells.
Information on reproduction by vegetative propagules or thallus fragmentation in Mediterranean corallines is very limited. Coppejans (1978) documented multicellular vegetative propagules in Hydrolithon farinosum (as Fosliella farinosa) from Corsica, describing their development and release (previously reported only by Solms-Laubach, 1881). Additional records of these structures in the same species were provided by Cormaci and Furnari (1988). Thallus fragmentation is frequent in maerl/rhodolith-forming species, especially branched species with thin branches such as Lithothamnion corallioides and Phymatolithon calcareum (Bosence, 1976; Peña et al., 2014). It can be expected that this type of reproduction plays an important role in Mediterranean rhodolith beds, but there are no experimental studies or genetic data that allow generalizations about its relative contribution compared to reproduction by spores.
No direct information is available about the dispersal of Mediterranean corallines. Some indirect information can be inferred from the genomic data of De Jode et al. (2019) for the most common cryptic species of the Lithophyllum stictiforme/cabiochiae complex of the French shores. These authors detected genetic differences at population level that suggested limited gene flow (and, indirectly, limited dispersal) even at distances of a few km. Conversely, no significant genetic differentiation was found between populations occurring in two different depth ranges (24–31 m and 37–46 m), suggesting that depth is not a barrier to dispersal. These conclusions are consistent with theoretical prediction of a generally limited dispersal in coralline algae. As for all other rhodophytes, the principal mean of dispersal is represented by non-flagellate spores (bispores, carpospores, and tetraspores). These are small-sized cells with very limited active movement, which are able to settle only on hard substrata and remain viable for relatively short periods (Guiry, 1990; Pickett-Heaps et al., 2001). Although the magnitude of water flow greatly influences their dispersal (Norton, 1992), it is generally believed that they do not disperse over long distances. It is likely that long-distance dispersal may take place in small-sized species (Hydrolithon spp., Pneophyllum spp., Melobesia membranacea) that grow as epiphytes on drifting leaves of Posidonia oceanica or larger seaweeds. This theoretical prediction, however, needs to be corroborated by population genetic data.
Microbiomes of Mediterranean Corallines
Marine macroalgae host a wide range of microbial organisms, among which bacteria are typically the dominant group. Bacterial communities associated with seaweeds establish strict biochemical interactions with their algal hosts and differ significantly from those found in the surrounding seawater (Brodie et al., 2016). Epiphytic bacterial communities have been reported as essential for normal morphological development of the algal host, and bacteria with antifouling properties are thought to protect chemically undefended macroalgae from detrimental, secondary colonization by other epibionts (Egan et al., 2012). In the case of some tropical corallines associated with coral reefs, the microbiome also facilitates the larval settlement of multiple species of corals (Sneed et al., 2015).
Studies concerning the microbiomes of corallines highlighted a great diversity of these assemblages (Cavalcanti et al., 2014; Sneed et al., 2015; Brodie et al., 2016) and suggested that the associated bacterial communities contribute to biomineralization and host fitness (Cavalcanti et al., 2014). Microbiomes are therefore likely to play an important role in the responses of corallines to long-term climatic changes. Studies focusing on microbial communities, however, have mostly concerned encrusting tropical species associated with coral reefs (Porolithon spp., Hydrolithon spp., Neogoniolithon spp.). Limited information is available for temperate species and, to date, only the studies of Ismail-Ben Ali et al. (2012) and Quéré et al. (2019) considered the microbiomes of Mediterranean corallines. Ismail-Ben Ali et al. (2012), in a study with pharmacological focus, isolated 19 bacterial strains from the surface of Jania rubens. Their results revealed that the main bacterial groups were Alphaproteobacteria, Gammaproteobacteria, Bacteroidetes and Firmicutes, and that 36% of the isolates produced antibiotics effective against Gram + and Gram – bacteria and the yeast Candida albicans. Quéré et al. (2019), compared the microbiome of Neogoniolithon brassica-florida affected by white-band syndrome with that of healthy specimens; they could not identify a potential causative agent of the disease, but characterized several opportunistic bacteria colonizing diseased tissues.
Biochemistry and Physiology
Research on Mediterranean corallines in these fields is relatively recent. Excluding work focusing on responses to climate change and ocean acidification, biochemical and physiological research has considered very few species, mainly articulate. For easiness of sampling due to its intertidal/shallow subtidal habitat, Ellisolandia elongata has been a favored target for this type of investigations.
Early work on this species examined photosynthetic performances (Häder et al., 1996, 1997), synthesis of chlorophylls and phycobiliproteins in response to light composition (López-Figueroa et al., 1989; López-Figueroa and Niell, 1990) and effects of red and blue light on the N-metabolism (Figueroa, 1993). Estimates of productivity and calcification rates were provided by El Haikali et al. (2004) for French populations. Overall, these studies depicted Ellisolandia elongata as a versatile species, capable to regulate its pigment content and metabolism based on quantity and quality of the light irradiation available. It should be noted, however, that these studies were performed before the description of Corallina caespitosa (Walker et al., 2009), a species morphologically similar to Ellisolandia elongata (now considered a synonym of C. ferreyrae, Bustamante et al., 2019). So, the taxonomic identity of the material used in these studies should be reassessed (especially for Häder et al., 1997: these authors distinguished two morphotypes, sun- and shade-adapted, which might represent different species).
More recent studies investigated populations of Ellisolandia elongata from the Alboran Sea, highlighting several metabolic features that make this alga well-adapted to withstand the environmental stresses typical of its interidal habitat (Celis-Plá et al., 2014; Figueroa et al., 2014a; Korbee et al., 2014; Parages et al., 2014; Stengel et al., 2014). This alga is able to improve its photoprotective capacity by regulating its content in mycosporine-like aminoacids (MAAs, compounds well-known for their photoprotective role in numerous algae) in response to environmental conditions (Celis-Plá et al., 2014; Korbee et al., 2014). Stengel et al. (2014) demonstrated a reduction in the effective photosystem II quantum efficiency in the central hours of the day, and showed that the highest phycocyanin content occurred in the evening; Parages et al. (2014) performed proteomic studies on the same samples and concluded that mitogen-activated protein kinase (MAPK)-like proteins are involved in the response of this species to environmental stress. Figueroa et al. (2014b) argued that the species is resistant to UVB-radiation thanks to the high reflectance of its calcareous thallus.
Jania rubens has been another common subject for biochemical work. Biochemical data for samples identified with this name were provided in several studies performed mainly by northern African investigators. These examined the content of chemical contaminants in environmental monitoring (Al-Masri et al., 2003; Abdallah and Abdallah, 2008; Olgunoglu and Polat, 2008; Hernández et al., 2011; Laib and Legouchi, 2012), biological activities of algal extracts (Abd-Elnaby, 2010; Khairy and El-Sheikh, 2015) and biochemical composition in relation to nutritional value (Polat and Ozogul, 2009, 2013) or for biodiesel production (El Maghraby and Fakhry, 2015; Soliman et al., 2018).
Responses of Mediterranean Corallines to Climate Change and Ocean Acidification
Approaches Used in the Study of the Responses of Coralline Algae to Climate Change and Acidification
Coralline algae are sensitive to changes in temperature and CO2 conditions and identified as being among the most vulnerable organisms to ocean acidification, because of the solubility of their high-magnesium (high-Mg) calcite skeleton (McCoy and Kamenos, 2015; Martin and Hall-Spencer, 2017). The responses of Mediterranean corallines to climate change and ocean acidification have been the subject of several studies that documented their tolerance or sensitivity to elevated temperatures and CO2. The effects of elevated CO2 on Mediterranean coralline algae were studied on single species (Martin and Gattuso, 2009; Martin et al., 2013a,b), in association with a small group of taxa (Asnaghi et al., 2013) and at the community scale (Porzio et al., 2011; Kroeker et al., 2012; Cox et al., 2015, 2017a,b; Marchini et al., 2019). These investigations have focused on a few species, mainly restricted to the group of (non-geniculate) crustose coralline algae (CCA), including epiphytic (on seagrass leaves) and engineering (coralligenous bio-constructors) species. Their response to ocean acidification and/or warming was studied through a variety of different approaches, including laboratory and field experiments, in situ observations in natural volcanic CO2 vent sites, and in situ manipulations using a Free Ocean Carbon dioxide Enrichment (FOCE) system. This variety of approaches provides critical insights in the effects of climate-related stressors on corallines isolated from their surrounding environment and in more complex ecosystems or naturally variable environments.
Impacts of Current Warming on the Health and Survival of Mediterranean Coralline Algae
Although some corallines can adapt to their local environment and acclimatize to a novel thermal regime, as recently suggested for some tropical species able to cope with thermal stress (Siboni et al., 2015), Mediterranean corallines appear particularly sensitive to warming. Several observations have been reported in different localities of the northwestern Mediterranean after summer seasons characterized by positive thermal anomalies. CCA mortality was reported at down to 30 m depth in late summer 1999, when seawater temperature was higher than normal by 2–4°C (Cerrano et al., 2000). In the laboratory, long exposure to elevated temperature (25°C) during summer was the cause of increased frequency of tissue necroses and mortality in Lithophyllum stictiforme (as L. cabiochae, Martin and Gattuso, 2009). Diseases of corallines described as white band syndrome or white patch disease (Figure 2) have recently been reported associated with high seawater temperature in the northwestern Mediterranean (Hereu and Kersting, 2016). These diseases affected the encrusting Lithophyllum incrustans, Mesophyllum alternans, and Neogoniolithon sp., and the geniculate Ellisolandia elongata, Jania rubens and Amphiroa rigida at shallow depths. The emergence of these thermo-dependent diseases is one of the most serious threats to Mediterranean coralline-dominated communities in the context of climate change.
Physiological Response of Mediterranean Coralline Algae to Climate Change and Ocean Acidification
Temperature directly affects enzymatic processes and is a dominant factor in determining physiological rates in corallines (Lüning, 1990). Rising temperature, within the range of temperature experienced in natural habitats, is beneficial for coralline algae with an increase in photosynthetic and calcification rates (Martin et al., 2006, 2013a), but increased temperature above these levels is detrimental. For example, a +3°C increase in seawater temperature was beneficial to calcification in Lithophyllum stictiforme (as L. cabiochae) in winter, when temperature is lowest, but a +3°C above maximum summer temperature caused increased frequency of necroses and mortality, and subsequent net calcification drop and further dissolution (Martin and Gattuso, 2009).
Effects of thermal stress were studied by Nannini et al. (2015) in populations of Ellisolandia elongata from western Italy and by Guy-Haim et al. (2016) in populations from Israel. Nannini et al. (2015) compared growth and calcification in field specimens with specimens cultured at different temperatures. They reported that thallus extension was higher in culture than in the field; the carbonate mass in the field was higher than in cultured material after 2 and 4 months, but decreased after 6 months. Guy-Haim et al. (2016) measured primary production, respiration and calcification of Ellisolandia elongata in the temperature range 15–35°C. In the population examined, the alga consists of the typical frondose form at temperatures <23°C; above this temperature, it switches to a reduced crustose form with short erect axes, but photosynthesis and calcification occur optimally in the interval 15–31°C. Above 31°C there is a metabolic breakdown, with bleaching and tissue necrosis. The authors argued that in the eastern Mediterranean, with continued warming, the species will experience a westward range contraction with phenological shifts, performance, and reproduction declines, population decreases and possible local extinctions.
Decreasing pH in the sea surface will cause major shifts in seawater chemistry over the course of this century, with changes in the relative proportion of the three forms of dissolved inorganic carbon (DIC) species (, , and CO2). These changes are likely to affect photosynthesis and calcification, since these physiological processes use DIC as substrate. Increase in CO2 may be beneficial for photosynthesis in some primary producers but the decrease in pH and may be detrimental for the precipitation of CaCO3 in calcifiers (Koch et al., 2013). Most of the studies on coralline algae showed that calcification is negatively affected under elevated pCO2 (Martin and Hall-Spencer, 2017) and that this effect is exacerbated by warming (Anthony et al., 2008). However, some work shows a significant pCO2 effect on calcification only when this is combined with an increase in temperature (Martin and Gattuso, 2009). The physiological response of coralline algae to increased pCO2 is variable among species (Martin and Hall-Spencer, 2017) and very few studies have focused on Mediterranean species. For Lithophyllum stictiforme (as L. cabiochae) Martin and Gattuso (2009) and Martin et al. (2013a) provided evidence of the ability to maintain or even enhance rate of calcification at near future levels of pCO2 (700 ppm). Such response may be related to the ability of this species to maintain an elevated pH at the site of calcification, despite reduced external pH that would favor CaCO3 precipitation (Cornwall et al., 2017).
The combination of ocean warming and acidification may cause a much greater effect on corallines. In healthy specimens of L. stictiforme, sensitivity of photosynthesis and calcification to high temperature increased in summer when combined with elevated pCO2 (Martin et al., 2013a). In the geniculate Ellisolandia elongata, such combined changes in pH and temperature can impair algal growth (Marchini et al., 2019). The combined effects of ocean warming and acidification can also make corallines more sensitive to other environmental stressors as shown by Fine et al. (2017), who studied thermal tolerance and resilience to low pH, high light intensity and desiccation in Neogoniolithon brassica-florida, an encrusting species acting as consolidator of vermetid reefs. This species resulted sensitive to increased light intensity, particularly in conditions of elevated temperature and low pH, with substantial decrease in photosynthetic performances; calcification was significantly impaired at lower pH. The authors concluded that this species is likely to lose its role of reef consolidator in future, mutated climatic conditions.
Effects of Climate Change and Ocean Acidification on the Mineralogy of Mediterranean Coralline Algae
Different forms of biogenic CaCO3 have different solubilities in seawater (aragonite > calcite). In calcite, the replacement of some Ca2+ by Mg2+ increases solubility. High-Mg calcite (>8–12 mol% MgCO3) is the most soluble CaCO3 form (Morse et al., 2007) and coralline algae skeletons composed of 8–29 mol% MgCO3 (Kamenos et al., 2013) are considered highly susceptible to dissolution in the context of ocean acidification. However, potential resilience of coralline algae may occur through changes in skeletal mineralogy, either by producing calcite with lower Mg content (Agegian, 1985; Egilsdottir et al., 2013) or by favoring accumulation of carbonate forms with lower solubility such as dolomite (Diaz-Pulido et al., 2014). However, recent studies on Mg incorporation in the skeleton of Mediterranean coralline algae found no pCO2 effect. Acidification did not drive any significant change in the Mg content of Lithophyllum stictiforme grown experimentally at 700 ppm (Nash et al., 2016). Similarly, no pCO2 effect was found on the Mg carbonate composition of Posidonia oceanica coralline epiphytes exposed to a decrease of 0.3 pH unit by using a FOCE system (Cox et al., 2017b). Kamenos et al. (2016) also found that CCA recruited on tiles had similar Mg content in ambient and low pH (7.8) sites in CO2 vents off Ischia Island (Italy). This lack of a pCO2 effect is consistent with findings suggesting that skeletal mineralogy may be under biological control (Nash et al., 2015). The high total alkalinity of Mediterranean waters (Palmiéri et al., 2015) may also have a potential role in buffering the effect of ocean acidification.
The Mg content in coralline algae is also known to vary as function of seawater temperature (Kamenos et al., 2008; Ragazzola et al., 2019). This has been confirmed in Mediterranean Lithophyllum stictiforme, for which the mineralogy is primarily controlled by temperature as shown experimentally with an increase of Mg incorporation of 1 mol% MgCO3 for an increase of 3°C (Nash et al., 2016). The high vulnerability of Mediterranean CCA skeleton to dissolution was already shown by Martin et al. (2008) and Cox et al. (2015) near and below pHT 7.7. Under experimental conditions of elevated temperature (+3°C) and elevated pCO2 (700 ppm), the percentage of death for Mediterranean Lithophyllum stictiforme was 2- to 3-fold higher and was accompanied by a rate of dissolution of dead algal thalli 2- to 4- times higher (Martin and Gattuso, 2009), suggesting that net dissolution is likely to exceed net calcification in L. stictiforme by the end of this century.
Effects of Climate Change and Ocean Acidification on Early Life Stages of Mediterranean Coralline Algae
Early life stages of corallines are particularly vulnerable to ocean acidification. The first studies were conducted on tropical species and showed that recruitment was drastically reduced under elevated pCO2 (Agegian, 1985; Kuffner et al., 2008).
In the Mediterranean, several studies were performed in CO2 vents of Ischia (Italy), where pH decreases naturally along a gradient from ambient (pHT 8.1) to very low pH (<7), in a range greater than expected under future climate scenarios. At these sites, the decrease in coralline cover with decreasing pH may be due to changes in physiological and competitive ability of these algae, but also to lowered reproduction (Porzio et al., 2011) or lowered recruitment (Kroeker et al., 2012). Decreased reproductive capacity has been reported for some coralline species. Cumani et al. (2010) showed in an artificial culture that CCA spore production and growth are inhibited by ocean acidification with an increase in the mortality of germination disks. Reduction in reproductive structures was also observed in the geniculate Jania rubens at pH 7.8 in CO2 vents of Ischia (Porzio et al., 2011). Since these vents are open systems, the negative effects due to the lower capacity of reproduction may be masked in these sites due to the import of spores and zygotes from external or control nearby sites. In the CO2 vents of Ischia, the recruitment of CCA appears to be inhibited at low pH. Changes in the succession of algae with a replacement of corallines by fleshy seaweeds were observed on settlement tiles at low pH (Porzio et al., 2011, 2013; Kroeker et al., 2012). The lowest pH (pHT < 7.2) caused failure in coralline algal recruitment but the genera Hydrolithon and Corallina were still recruited under medium pH (pHT 7.8; Porzio et al., 2013), suggesting that some species of corallines may be able to persist at pH levels expected for the end of this century. However, the high pH variability observed in the medium pH sites means that pH rises to/close to current pH levels regularly and may lead to an underestimation of the impact of acidification, as these sites are not remaining constantly under low pH conditions (Porzio et al., 2013). In the same area, Kamenos et al. (2016) observed that the largest individuals of CCA recruited on tiles maintained growth and were of similar size in low (7.8) and ambient (8.0–8.1) pH zones. The ability of some thalli to continue growing in lower pH suggests acclimation/adaptation to low pH conditions and ability to provide recruits for populations adapted to survive in lower pH environment in the future. Through in situ pH manipulation using a FOCE system in a Posidonia oceanica meadow, Cox et al. (2017a) also found that early stages of CCA are sensitive to decreased pH, with lower coverage of CCA on recruitment tiles placed in a pH-manipulated enclosure (−0.3 pH unit offset) compared to an un-manipulated enclosure (ambient pH). Although previous studies suggested post-settlement competition between fleshy and calcareous algae (Porzio et al., 2011; Kroeker et al., 2012; Kamenos et al., 2016), Cox et al. (2017a) suggested that losses of CCA were driven by taxa sensitivity, because the other taxa were also reduced by the lower pH conditions and there was still bare space available for colonization.
Field Assessment of the Impacts of Ocean Acidification on Mediterranean Coralline Algae
The responses of coralline algae to acidification can be altered by biotic (e.g., competition for space and resource or herbivory) and abiotic (e.g., irradiance or nutrient supply) interactions. Although most investigations on corallines were conducted in the laboratory with single taxa or relatively small groups of taxa isolated from the surroundings, some studies in the Mediterranean used whole ecosystem approaches (CO2 vents or FOCE system) that allow to consider these interactions. These studies focused mostly on the epiphytic CCA in seagrass meadows. In situ observations near CO2 vents reported clear reductions or losses of corallines where the pH is naturally lower (Hall-Spencer et al., 2008; Martin et al., 2008; Porzio et al., 2011; Kroeker et al., 2012; Baggini et al., 2014; Donnarumma et al., 2014). In the CO2 vents of Ischia, Martin et al. (2008) showed a complete disappearance of epiphytic CCA on Posidonia oceanica at an average pHT of 7.7, consistent with pH expected for the end of this century, but with large temporal pH variations from <7 to >8.1. Vent systems are not perfect predictors of future ocean ecology, due to the high pH variability in space and time that makes difficult to identify threshold or tipping points (Hall-Spencer et al., 2008; Kerrison et al., 2011). Recently, some studies reached conclusions in contrast with previous findings on ocean acidification projections. Cox et al. (2017b) found no pH effect on epiphytic CCA on Posidonia oceanica exposed to a decrease of −0.3 pH unit in a pH-manipulated enclosure using a FOCE system. Additional insights into community-level effects of warming and ocean acidification are beginning to emerge from longer-term multispecies laboratory experiments (Hale et al., 2011; Legrand et al., 2017). Similarly, Asnaghi et al. (2013) demonstrated that grazing activity exacerbated the effects of pCO2 on corallines with higher weight loss in the geniculate Ellisolandia elongata under elevated pCO2 in the presence of urchins. It is clear that the impact of global changes on corallines will depend on the combined influence of direct environmental effects on individual species and indirect effects mediated by changes in interspecific interactions (Harley et al., 2012). Long-term multispecies experiments combining warming and ocean acidification appear essential to improve our future understanding of Mediterranean coralline algae.
Conclusions and Directions for Future Work
The present summary shows that there are still substantial gaps in our knowledge of Mediterranean corallines, despite of the large amount of information available for some coralline-dominated habitats (particularly coralligenous). Giving the ecological importance of these algae and their sensitivity to climate change, the body of information available should be now substantially expanded.
As general recommendation, we suggest that future work on Mediterranean corallines should be based on a multidisciplinary perspective combining different approaches. So far, work on these algae has consisted mostly of separate efforts/projects, carried out by researchers working in different fields and interested in different aspects. The integration of different approaches will be essential, in particular, to address major large-scale and long-term issues, such as the responses of individual species and populations to future, mutated climatic scenarios. We also note that most of the information currently available for Mediterranean corallines has been produced by scientists based in a relatively small number of countries (mainly France, Italy and Spain). As consequence, the body of information available for the western Mediterranean is currently much larger than for the eastern Mediterranean. This represents a major limit for the interpretation of general patterns, especially for coralline species with distribution extending to the whole Mediterranean. Within a same species, populations from the western Mediterranean (especially northwestern) and from the eastern Mediterranean are presumably characterized by different ecophysiological traits and therefore are likely to respond differently to future climatic changes. Therefore, we suggest that future research on basic aspects of the biology of Mediterranean corallines should involve many scientists with different backgrounds, based in several countries, both in the western and eastern Mediterranean.
More specifically, there are some tasks that we identify as prioritary for each of the main fields of investigation:
1) Further paleontological investigations, focusing on identification of morpho-anatomical species groups with paleoecological and/or paleobiogeographical meanings, study of the evolution of Mediterranean coralline assemblages in the context of important paleogeographic changes, and how global environmental parameters such as sea-level, ocean acidification, and global temperature affected in the past the evolution of Mediterranean species.
2) An accurate taxonomic reassessment of the Mediterranean coralline flora based on a modern combination of molecular, morphoanatomical and ecological data. This is also a critical requirement for an accurate biogeographic reassessment. Taxa surrounded by taxonomic uncertainty or for which molecular data are lacking should receive priority; among these, we suggest:
- Neogoniolithon, a genus for which the only species N. brassica-florida is currently recognized in the Mediterranean, but in which species circumscription is unclear (Kato et al., 2013).
- Members of Sporolithales, for which there are no molecular data from Mediterranean collections.
- Maerl/rhodolith-forming species for which no sequences are available (Lithothamnion minervae, L. valens), or for which conspecifity with Atlantic counterparts should be verified (Lithothamnion corallioides).
- Lithophyllum of intertidal and shallow subtidal zones; these algae in the Mediterranean have been usually identified as L. incrustans, but the data of Hernandez-Kantun et al. (2015a) highlighted that this is mainly a subtidal species, suggesting that the identity of intertidal specimens requires reassessment.
- Lithophyllum trochanter and L. woelkerlingii, two species separated by Bressan and Cabioch (2004) based on subtle characters.
3) Studies of reproductive biology combining observations in the field and experiments in the laboratory with molecular and biochemical studies aiming to elucidate the mechanisms triggering fertility and release of reproductive cells.
4) Transcriptomic studies and characterization of associated microbiomes, starting from species important as bioconstructors or habitat-formers (Lithophyllum stictiforme, L. byssoides, Ellisolandia elongata). Investigations of this type are necessary for a deep understanding of the molecular and biochemical processes determining responses to environmental disturbances, and for early detection of stress in populations of ecologically important species.
5) Extending studies on the effects of climate change and acidification to a wider set of species, and expanding them to long-term experimental investigations. We suggest that Mesophyllum species contributing to coralligenous concretions, maerl/rhodolith-forming species (Lithophyllum racemus, Lithothamnion corallioides, L. minervae, L. valens, Phymatolithon calcareum) and bioconstructor species of the intertidal/shallow subtidal zone (Lithophyllum byssoides, L. trochanter) are natural candidates for such studies. New studies focusing on the main aspects (physiology, mineralogy, vitality of early life stages) in which Mediterranean corallines may be affected by climate change and ocean acidification will represent valuable contributions.
6) Basic studies on mineralization. The complete lack of information for Mediterranean species is striking, considering the importance of this aspect for growth, carbonate formation, and bioconstruction activity of these algae.
7) Accurate phylogeographic studies based on high-resolution genetic methods, such as microsatellites, Single Nucleotide Polymorphisms (SNPs) or sequencing of Restriction Site Associated DNA markers (RAD), starting from ecologically significant species (Lithophyllum stictiforme, L. byssoides, Mesophyllum expansum, Ellisolandia elongata, Lithothamnion corallioides). Data of this type are essential to draw effective conservation measures for marine species. It is striking that in general studies of this type are in great shortage for Mediterranean seaweeds (ironically, from this point of view some introduced taxa have been so far the best-studied seaweeds in the Mediterranean). This is even more surprising considering that, in contrast, the amount of similar studies concerning Mediterranean fishes and invertebrates is ponderous (see Patarnello et al., 2007; Pascual et al., 2017).
We remark that these tasks can be best tackled by integrating the work in each specific field with other types of data/approaches. The integration of basic ecological, physiological and biochemical work with genomics, taxonomy and molecular phylogeny will be especially important to predict both changes in distribution and abundance of individual coralline species, and shifts in the structure of Mediterranean ecosystems built or dominated by corallines. This will be mandatory when the corallines studied turn to be complexes of cryptic species that differ in ecological and physiological traits, as recently stressed by De Jode et al. (2019). In general, cryptic diversity in corallines is an aspect that in the future will require much more attention from Mediterranean marine biologists. Neglecting it might lead to erroneous interpretations of the results of studies in many different fields. At ecosystem level, changes in diversity of coralline assemblages, if undetected due to the cryptic nature of the species involved, may lead to shifts in ecosystem structure and functioning (Hind et al., 2019).
Even for this reason, we recommend that all future studies on Mediterranean corallines (of any type, not just taxonomic) should base their identifications on DNA sequence data and take care to deposit voucher specimens in herbaria or other permanent collections. This will give the possibility to verify the correctness of the identifications, which will be a critically important requirement for comparison of the results of different studies.
Author Contributions
FR led the conceptual design of the paper, contributed to the writing of the sections Introduction, Historical Summary, Floristic Diversity, Molecular Studies of Mediterranean Corallines, Present-Day Distribution and Biogeography, Reproductive Biology, Microbiomes of Mediterranean Corallines, Biochemistry and Physiology, and Conclusions and Directions for Future Work, assembled the first version of the manuscript, and prepared the Table 1, the Figures 1, 2, and 3, and the File SM1. JA and JB wrote the section Geological History of the Mediterranean and Paleontological Record of Mediterranean Corallines, and prepared the Figure 5 and the Files SM2 – SM6. SM wrote the section Responses of Mediterranean Corallines to Climate Change and Ocean Acidification. VP, LG, and AC contributed to the writing of the sections Introduction, Historical Summary, Floristic Diversity, Molecular Studies of Mediterranean Corallines, Present-Day Distribution and Biogeography, and Conclusions and Directions for Future Work. AC prepared the Figure 4. VP prepared the Table S1.
Conflict of Interest
The authors declare that the research was conducted in the absence of any commercial or financial relationships that could be construed as a potential conflict of interest.
The reviewer AC declared a past co-authorship with one of the authors LG to the handling editor.
Acknowledgments
We are grateful to Enric Ballesteros, Marc Verlaque, Carlo Cerrano, Maria Cristina Gambi, and Sara Kaleb for kindly allowing use of pictures. The ideas presented in this article are partially based on results produced in a P.R.I.N. 2010–2011 project (Coastal bioconstructions: structure, function and management), for which FR acknowledges financial support from the Italian Ministry for Education, Universities and Research. VP acknowledges support from the Universidade da Coruña (Programa Campus Industrial de Ferrol).
Supplementary Material
The Supplementary Material for this article can be found online at: https://www.frontiersin.org/articles/10.3389/fmars.2019.00723/full#supplementary-material
Table S1. Detail of DNA sequence data obtained from Mediterranean corallines.
File SM1. List of references used for the compilation of the bibliographic summary of Mediterranean corallines.
File SM2. List of fossil corallines reported from the Mediterranean area.
File SM3. Records of fossil Sporolithales reported for the Mediterranean area.
File SM4. Records of fossil Hapalidiales reported for the Mediterranean area.
File SM5. Records of fossil Corallinales reported for the Mediterranean area.
File SM6. List of references for supplementary files SM2, SM3, SM4, and SM5.
References
Abdallah, M. A. M., and Abdallah, A. M. A. (2008). Biomonitoring study of heavy metals in biota and sediments in the South Eastern coast of Mediterranean Sea, Egypt. Environ. Monitor. Assess. 146, 139–145. doi: 10.1007/s10661-007-0066-8
Abd-Elnaby, H. (2010). Bacteria-algae interactions in Abu-Qir marine ecosystem and some applied aspects of algal extracts. J. Appl. Sci. Res. 6, 345–357.
Adey, W. H., Hernandez-Kantun, J. J., Johnson, G., and Gabrielson, P. W. (2015). DNA sequencing, anatomy, and calcification patterns support a monophyletic, subarctic, carbonate reef-forming Clathromorphum (Hapalidiaceae, Corallinales, Rhodophyta). J. Phycol. 51, 189–203. doi: 10.1111/jpy.12266
Agegian, C. R. (1985). The biogeochemical ecology of porolithon gardineri (Foslie) (Ph.D. thesis). University of Hawaii, Honolulu, Hawaii.
Aguirre, J. (1998). El Plioceno marino del SE de la Península Ibérica (provincia de Almería). Síntesis estratigráfica, sedimentaria, bioestratigráfica y paleogeográfica. Rev. Soc. Geol. España 11, 297–315.
Aguirre, J., Baceta, J. I., and Braga, J. C. (2007). Recovery of primary marine producers after the Cretaceous-Tertiary mass extinction: paleocene calcareous red algae from the Iberian Peninsula. Palaeogeog. Palaeoclimatol. Palaeoecol. 249, 393–411. doi: 10.1016/j.palaeo.2007.02.009
Aguirre, J., Belaústegui, Z., Domènech, R., Gibert, J. M., and de Martinell, J. (2014). Snapshot of a lower pliocene dendropoma reef from sant onofre (Baix Ebre Basin, Tarragona, NE Spain). Palaeogeog. Palaeoclimatol. Palaeoecol. 395, 9–20. doi: 10.1016/j.palaeo.2013.12.011
Aguirre, J., Braga, J. C., Martín, J. M., and Betzler, C. (2012). Palaeoenvironmental and stratigraphic significance of Pliocene rhodolith beds and coralline algal bioconstructions from the Carboneras Basin (SE Spain). Geodiversitas 34, 115–136. doi: 10.5252/g2012n1a7
Aguirre, J., and Jiménez, A. P. (1998). Analogues of present-day Cladocora caespitosa coral banks; sedimentary setting, dwelling community, and taphonomy (late Pliocene, W Mediterranean). Coral Reefs 17, 203–213. doi: 10.1007/s003380050119
Aguirre, J., Riding, R., and Braga, J. C. (2000a). Diversity of coralline red algae: origination and extinction patterns from the early cretaceous to the pleistocene. Paleobiology 26, 651–667. doi: 10.1666/0094-8373(2000)026<0651:DOCRAO>2.0.CO;2
Aguirre, J., Riding, R., and Braga, J. C. (2000b). Late Cretaceous incident light reduction: evidence from benthic algae. Lethaia 33, 205–213. doi: 10.1080/00241160025100062
Airoldi, M. (1932). Contributo allo studio delle corallinacee del terziario italiano. 1. Le Corallinacee dell'Oligocene Ligure-Piemontese. Palaeontogr. Ital., Mem. Paleont. 33, 55–83.
Al-Masri, M. S., Mamish, S., and Budier, Y. (2003). Radionuclides and trace metals in eastern Mediterranean Sea algae. J. Environ. Riadioactiv. 67, 157–168. doi: 10.1016/S0265-931X(02)00177-7
Anagnostou, E., John, E., Edgar, K., Foster, G., Ridgwell, A., Inglis, G., et al. (2016). Changing atmospheric CO2 concentration was the primary driver of early Cenozoic climate. Nature 533, 380–384. doi: 10.1038/nature17423
Anthony, K. R. N., Kline, D. I., Diaz-Pulido, G., Dove, S., and Hoegh-Guldberg, O. (2008). Ocean acidification causes bleaching and productivity loss in coral reef builders. Proc. Nat. Acad. Sci. U.S. A. 105, 17442–17446. doi: 10.1073/pnas.0804478105
Arias, C., Masse, J. P., and Vilas, L. (1995). Hauterivian shallow marine calcareous biogenic mounds: S. E. Spain. Palaeogeog. Palaeoclimatol. Palaeoecol. 119, 3–17. doi: 10.1016/0031-0182(95)00056-9
Aroldi, C., and Bucur, I. I. (2002). Palaeogene microfacies in some calcareous blocks of the Roaia Formation (Wildflysch Nappe – Maramures). Stud. Univ. Babes-Bolyai Geol. Ep. Issue 1, 13–30.
Asnaghi, V., Chiantore, M., Mangialajo, L., Gazeau, F., Francour, P., Alliouane, S., et al. (2013). Cascading effects of ocean acidification in a rocky subtidal community. PLoS ONE 8:e61978. doi: 10.1371/journal.pone.0061978
Athanasiadis, A. (1989). North Aegean marine algae. III. Structure and development of the encrusting coralline Titanoderma cystoseirae (Rhodophyta, Lithophylloideae). Nord. J. Bot. 9, 435–441. doi: 10.1111/j.1756-1051.1989.tb01023.x
Athanasiadis, A. (1995). Morphology, anatomy and reproduction of the eastern Mediterranean coralline Tenarea tortuosa and its relationship to members of the Lithophylloideae and Mastophoroideae (Rhodophyta, Corallinales). Nord. J. Bot. 15, 655–663. doi: 10.1111/j.1756-1051.1995.tb02136.x
Athanasiadis, A. (1997). On the typification and taxonomic status of Melobesia notarisii Dufour (Rhodophyta, Corallinales). Phycologia 36, 410–415. doi: 10.2216/i0031-8884-36-5-410.1
Athanasiadis, A. (1999a). The taxonomic status of Lithophyllum stictaeforme (Rhodophyta, Corallinales) and its generic position in light of phylogenetic considerations. Nord. J. Bot. 19, 735–745. doi: 10.1111/j.1756-1051.1999.tb00682.x
Athanasiadis, A. (1999b). Mesophyllum macedonis, nov. sp. (Rhodophyta, Corallinales), a putative Tethyan relic in the North Aegean Sea. Eur. J. Phycol. 34, 239–252. doi: 10.1017/S0967026299002103
Athanasiadis, A., and Neto, A. I. (2010). On the occurrence of Mesophyllum expansum (Philippi) Cabioch et Mendoza (Melobesioideae, Corallinales, Rhodophyta) in the Mediterranean Sea, the Canary Isles and the Azores. Bot. Mar. 53, 333–341. doi: 10.1515/BOT.2010.042
Baggini, C., Salomidi, M., Voutsinas, E., Bray, L., Krasakopoulou, E., and Hall-Spencer, J. M. (2014). Seasonaility affects macroalgal community response to increases in pCO2. PLoS ONE 9:e106520. doi: 10.1371/journal.pone.0106520
Bailey, J. C., and Chapman, R. L. (1996). “Evolutionary relationships among coralline red algae (Corallinaceae, Rhodophyta) determined by 18S rRNA gene sequence analysis”, in Cytology, Genetics and Molecular Biology of Algae, eds B. R. Chaudhary and S. B. Agrawal (Amsterdam: SPB Academic Publishing), 363–376.
Bailey, J. C., and Chapman, R. L. (1998). A phylogenetic study of the Corallinales (Rhodophyta) based on nuclear small-subunit rRNA gene sequences. J. Phycol. 34, 692–705. doi: 10.1046/j.1529-8817.1998.340692.x
Ballesteros, E. (1988). Composiciòn y estructura de la comunidad de Corallina elongata Ellis and Solander, 1786, de la Costa Brava (Mediterraneo occidental). Inv. Pesq. 52, 135–151.
Ballesteros, E. (2006). Mediterranean coralligenous assemblages: a synthesis of present knowledge. Oceanogr. Mar. Biol. Ann. Rev. 44, 123–195. doi: 10.1201/9781420006391.ch4
Barattolo, F., Bassi, D., and Romano, R. (2007). Upper Eocene larger foraminiferal–coralline algal facies from the Klokova Mountain (southern continental Greece). Facies 53, 361–375. doi: 10.1007/s10347-007-0108-2
Barrier, E., Vrielynck, B., Bergerat, F., Brunet, M.-F., Mosar, J., Poisson, A., et al. (2018). Palaeotectonic Maps of the Middle East: Tectono-Sedimentary-Palinspastic Maps From Late Norian to Pliocene. Commission for the Geological Map of the World (CGMW / CCGM), Paris, France.
Bassi, D. (1998). Coralline algal facies and their palaeoenvironments in the Late Eocene of Northern Italy (Calcare di Nago). Facies 39, 179–202. doi: 10.1007/BF02537016
Bassi, D., Braga, J. C., and Iryu, Y. (2009). Palaeobiogeographic patterns of a persistent monophyletic ineage: Lithophyllum pustulatum species group (Corallinaceae, Corallinales, Rhodophyta). Palaeogeogr. Palaeoclimatol. Palaeoecol. 284, 237–245. doi: 10.1016/j.palaeo.2009.10.003
Bassi, D., Braga, J. C., Zakrevskaya, E., and Radionova, E. P. (2005). Re-assessment of the type collections of corallinalean genera (Corallinales, Rhodophyta) described by Maslov (1935–1962). Palaeontology 48, 1–17. doi: 10.1111/j.1475-4983.2005.00503.x
Bassi, D., Simone, L., and Nebelsik, J. H. (2017). “Re-sedimented rhodoliths in channelized depositional systems”, in Rhodolith/Maërl Beds: A Global Perspective, eds. R. Riosmena-Rodríguez, W. Nelson, and J. Aguirre (Basel: Springer International Publishing), 139–167. doi: 10.1007/978-3-319-29315-8_6
Basso, D. (1995). Living calcareous algae by a paleontological approach: the genus Lithothamnion Heydrich nom. cons. from the soft bottoms of the Tyrrhenian Sea (Mediterranean). Riv. It. Paleont. Strat. 101, 349–366.
Basso, D., Babbini, L., Ramos-Esplá, A. R., and Salomidi, M. (2017). “Mediterranean rhodolith beds” in Rhodolith/Maërl Beds: a Global Perspective, eds R. Riosmena-Rodriguez, W. Nelson, and J. Aguirre (Basel: Springer International Publishing), 281–298. doi: 10.1007/978-3-319-29315-8_11
Basso, D., Caronni, S., Caragnano, A., Hereu, B., Angeletti, L., and Bracchi, V. (2018). “Evidence of coralline white patch disease in a rhodolith bed of the Egadi Islands,” in Abstract Book, VI International Rhodolith Workshop (Roscoff), 60.
Basso, D., Coletti, G., Bracchi, V. A., and Yazdi-Moghadam, M. (2019). Lower oligocene coralline algae of the Uromieh section (Qom Formation, NW Iran) and the oldest record of Titanoderma pustulatum (Corallinophycidae, Rhodophyta). Riv. It. Paleontol. Stratig. 125, 197–218.
Basso, D., Fravega, P., and Vannucci, G. (1996). Fossil and living corallinaceans related to the Mediterranean endemic species Lithophyllum racemus (Lamarck) Foslie. Facies 35, 257–292. doi: 10.1007/BF02536965
Basso, D., and Rodondi, G. (2006). A Mediterranean population of Spongites fruticulosus (Rhodophyta, Corallinales), the type species of Spongites, and the taxonomic status of S. stalactitica and S. racemosa. Phycologia 45, 403–416. doi: 10.2216/04-93.1
Basso, D., Rodondi, G., and Bressan, G. (2011). A re-description of Lithothamnion crispatum and the status of Lithothamnion superpositum (Rhodophyta, Corallinales). Phycologia 50, 144–155. doi: 10.2216/10-20.1
Basso, D., Rodondi, G., and Mari, M. (2004). A comparative study between Lithothamnion minervae and the type material of Myllepora fasciculata (Corallinales, Rhodophyta). Phycologia 43, 215–223. doi: 10.2216/i0031-8884-43-2-215.1
Bianchi, C. N., and Morri, C. (2000). Marine biodiversity of the Mediterranean Sea: situation, problems and prospects for future research. Mar. Poll. Bull. 40, 367–376. doi: 10.1016/S0025-326X(00)00027-8
Bittner, L., Payri, C., Maneveldt, G., Couloux, A., Cruaud, C., de Reviers, B., et al. (2011). Evolutionary history of the Corallinales (Corallinophycidae, Rhodophyta) inferred from nuclear, plastidial and mitochondrial genomes. Mol. Phylogen. Evol. 61, 697–713. doi: 10.1016/j.ympev.2011.07.019
Blanfuné, A. (2016). Global change in the NW mediterranean sea: the fate of forests of Cystoseira and Sargassum, Lithophyllum rims and blooms of ostreopsis (Ph.D. thesis). Université d'Aix-Marseille, Marseilles, France.
Bory de Saint-Vincent, J. B. G. M. (1832). “Notice sur les polypiers de la Gréce,” in Expédition Scientifique de Morée (Section des Sciences Physiques). Tome III [1.re Partie, 1.re Section]. Zoologie [pl. LIV - Atlas] (Paris; Strasbourg: F.G. Levrault, imprimeur-libraire), 204-209,
Bosellini, F. R., and Perrin, C. (2008). Estimating mediterranean oligocene-miocene sea-surface temperatures: an approach based on coral taxonomic richness. Palaeogeogr. Palaeoclimatol. Palaeoecol. 258, 71–88. doi: 10.1016/j.palaeo.2007.10.028
Bosellini, F. R., and Russo, A. (1992). Stratigraphy and facies of an oligocene fringing reef (Castro Limestone, Salento Peninsula, Southern Italy). Facies 26, 145–166. doi: 10.1007/BF02539798
Bosence, D. W. J. (1976). Ecological studies on two unattached coralline algae from western Ireland. Palaeontology 19, 365–395.
Bosence, D. W. J., and Pedley, H. M. (1982), Sedimentology palaeoecology of a Miocene coralline algal biostrome from the Maltese Islands. Palaeogeog. Palaeoclimatol. Palaeoecol. 38, 9–43. doi: 10.1016/0031-0182(82)90062-1
Boudouresque, C. F. (2004). Marine biodiversity in the Mediterranean: status of species, populations and communities. Trav. Sci. Parc Nat. Port-Cros 20, 97–146.
Boudouresque, C. F., and Verlaque, M. (1978). Végétation marine de la Corse (Méditerranée). Documents pour la flore des algues. Bot. Mar. 21, 265–275. doi: 10.1515/botm.1978.21.5.265
Bover-Arnal, T., Ferràndez-Cañadell, C., Aguirre, J., Esteban, M., Fernández-Carmona, J., Albert-Villanueva, E., et al. (2017). Late Chattian platform carbonates with benthic foraminifera and coralline algae from the SE Iberian Plate. Palaios 32,61–82. doi: 10.2110/palo.2016.007
Bracchi, V. A., Angeletti, L., Marchese, F., Taviani, M., Cardone, F., Hajdas, I., et al. (2019a). A resilient deep-water rhodolith bed off the Egadi Archipelago (Mediterranean Sea) and its actuopaleontological significance. Alp. Medit. Quat. 32, 1–20. doi: 10.26382/AMQ.2019.09
Bracchi, V. A., and Basso, D. (2012). The contribution of calcareous algae to the biogenic carbonates of the continental shelf: Pontian Islands, Tyrrhenian Sea, Italy. Geodiversitas 34, 61–76. doi: 10.5252/g2012n1a4
Bracchi, V. A., Basso, D., Savini, A., and Corselli, C. (2019b). Algal reefs (Coralligenous) from glacial stages: origin and nature of a submerged tabular relief (Hyblean Plateau, Italy). Mar. Geol. 411, 119–132. doi: 10.1016/j.margeo.2019.02.008
Braga, J. C. (2017). “Neogene rhodoliths in the Mediterranean basins”, in Rhodolith/Maërl Beds: A Global Perspective, eds R. Riosmena-Rodríguez, W. Nelson, and J. Aguirre (Basel: Springer Inter. Publ.), 169–193. doi: 10.1007/978-3-319-29315-8_7
Braga, J. C., and Aguirre, J. (2001). Coralline algal assemblages in upper Neogene reef and temperate carbonates in Southern Spain. Palaeogeogr. Palaeoclimatol. Palaeoecol. 175, 27–41. doi: 10.1016/S0031-0182(01)00384-4
Braga, J. C., and Bassi, D. (2007). Neogene history of Sporolithon Heydrich (Corallinales, Rhodophyta) in the Mediterranean region. Palaeogeogr. Palaeoclimatol. Palaeoecol. 243, 189–203. doi: 10.1016/j.palaeo.2006.07.014
Braga, J. C., and Bassi, D. (2011). Facies and coralline algae from Oligocene limestones in the Malaguide Complex (SE Spain). Ann. Naturhist. Mus. Wien Serie A 113, 291–308.
Braga, J. C., Bassi, D., and Piller, W. (2010). “Palaeoenvironmental significance of Oligocene-Miocene coralline red algae - a review,” in Carbonate Systems During the Oligocene-Miocene Climatic Transition, Vol. 42. eds M. Mutti, W. E. Piller, and C. Betzler (Oxford; Chichester; Hoboken, NJ: IAS Spec. Publ.; Wiley-Blackwell), 165–182. doi: 10.1002/9781118398364.ch10
Braga, J. C., Vescogni, A., Bosellini, F., and Aguirre, J. (2009). Coralline algae (Corallinales, Rhodophyta) in western and central Mediterranean Messinian reefs. Palaeogeogr. Palaeoclimatol. Palaeoecol. 275, 113–128. doi: 10.1016/j.palaeo.2009.02.022
Brandano, M. (2017). “Oligocene rhodolith beds in the central Mediterranean area”, in Rhodolith/Maërl Beds: A Global Perspective, eds R. Riosmena-Rodríguez, W. Nelson, and J. Aguirre (Basel: Springer Inter. Publ.), 195–219. doi: 10.1007/978-3-319-29315-8_8
Bressan, G., and Babbini, L. (1995). Inventario delle Corallinales del Mar Mediterraneo: considerazioni tassonomiche. Giorn. Bot. It. 129, 367–390. doi: 10.1080/11263509509436152
Bressan, G., and Babbini, L. (2003). Corallinales del Mar Mediterraneo: guida alla determinazione. Biol. Mar. Medit. 10, 1–237.
Bressan, G., and Babbini-Benussi, L. (1996). Phytoceanographical observations on coralline algae (Corallinales) in the Mediterranean Sea. Rend. Fis. Acc. Lincei 7, 179–207. doi: 10.1007/BF03001802
Bressan, G., and Cabioch, J. (2004). Titanoderma trochanter (Bory) Benhissoune, Boudouresque, Perret Boudouresque et Verlaque, et Titanoderma ramosissimum (Heydrich) comb. nov. (Corallinales, Rhodophytes) une redefinition. Cah. Biol. Mar. 45, 225–242.
Bressan, G., Chemello, R., Gravina, M. F., Gambi, M. C., Peirano, A., Cocito, S., et al. (2009). “Other types of bioconcretions” in Italian habitats - Marine bioconstructions, eds A. Cosentino, A. La Posta, C. Morandini, and G. Muscio (Udine: Museo Friulano di Storia Naturale), 89–150.
Bressan, G., Miniati-Radin, D., and Smundin, L. (1977). Ricerche sul genere Fosliella (Corallinaceae - Rhodophyta): Fosliella cruciata sp.nov. Giorn. Bot. It. 111, 27–44. doi: 10.1080/11263507709426571
Brodie, J., Walker, R. H., Williamson, C., and Irvine, L. M. (2013). Epitypification and redescription of Corallina officinalis L., the type of the genus, and C. elongata Ellis et Solander (Corallinales, Rhodophyta). Cryptog. Algol. 34, 49–56. doi: 10.7872/crya.v34.iss1.2013.49
Brodie, J., Williamson, C., Barker, G. L., Walker, R. H., Briscoe, A., and Yallop, M. (2016). Characterising the microbiome of Corallina officinalis, a dominant calcified intertidal red alga. FEMS Microbiol. Ecol. 92:fiw110. doi: 10.1093/femsec/fiw110
Bucur, I. I. (1994). Algues calcaires de la zone de Resita-Moldova Noua (Carpathes meridionales, Roumanie). Rev. Paléobiol. 13, 147–209.
Bucur, I. I. (2008). Barremian-Aptian calcareous algae from Romania: an overview. Boll. Soc. Geol. It. 127, 245–255.
Bucur, I. I., and Baltres, A. (2002). Cenomanian microfossils in the shallow water limestones from Babadag Basin: biostratigraphic significance. Stud. Univ. Babes-Bolyai, Geol. 1, 79–95.
Bucur, I. I., Onac, B. P., and Todoran, V. (1989). Algues Calcaires dans les Dépôts Oligocènes Inférieurs de la Région Purcâret-Mesteacân-Valea Chioarului (NW du Bassin de Transylvanie). The Oligocene from the Transylvanian Basin (Petrescu-Iustinian Editions, Univ. Cluj-Napoca, Cluj-Napoca), 141–148.
Bustamante, D. E., Calderon, M. S., and Hughey, J. R. (2019). Conspecifity of the Peruvian Corallina ferreyrae with C. caespitosa (Corallinaceae, Rhodophyta) inferred from genomic analysis of the type specimen. Mitochondrial DNA 4, 1285–1286. doi: 10.1080/23802359.2019.1591203
Cabioch, J., and Mendoza, M. L. (1998). Mesophyllum alternans (Foslie) comb. nov. (Corallinales, Rhodophyta), a mediterraneo-atlantic species, and new considerations on the Lithothamnion philippii Foslie complex. Phycologia 37, 208–221. doi: 10.2216/i0031-8884-37-3-208.1
Cabioch, J., and Mendoza, M. L. (2003). Mesophyllum expansum (Philippi) comb. nov. (Corallinales, Rhodophytes), et mise au point sur les Mesophyllum des mers d'Europe. Cah. Biol. Mar. 44, 257–273.
Canals, M., and Ballesteros, E. (2007). Production of carbonate particles by phytobenthic communities on the Mallorca-Menorca shelf, northwestern Mediterranean Sea. Deep Sea Res. 44, 611–629. doi: 10.1016/S0967-0645(96)00095-1
Catra, M., Alongi, G., Serio, D., Cormaci, M., and Furnari, G. (2006). The benthic algal flora on rocky substrata of the Egadi islands, a marine protected archipelago off the western coast of Sicily (Italy, Mediterranean Sea). Nova Hedwigia 82, 489–538. doi: 10.1127/0029-5035/2006/0082-0489
Cavalcanti, G. S., Gregoracci, G. B., dos Santos, E. O., Silveira, C. B., and Meirelles, P. M. (2014). Physiologic and metagenomic attributes of the rhodoliths forming the largest CaCO3 bed in the South Atlantic Ocean. ISME J. 8, 52–62. doi: 10.1038/ismej.2013.133
Cebrián, E., Ballesteros, E., and Canals, M. (2000). Shallow rocky bottom benthic assemblages as calcium carbonate producers in the Alboran Sea (southwestern Mediterranean). Oceanol. Acta 23, 311–322. doi: 10.1016/S0399-1784(00)00131-6
Cecere, E., Cormaci, M., Furnari, G., Petrocelli, A., Saracino, O., and Serio, D. (1996). Benthic algal flora of Cheradi Islands (Gulf of Taranto, Mediterranean Sea). Nova Hedwigia 62, 191–214.
Celis-Plá, P. S. M., Martínez, B., Quintano, E., García-Sánchez, M., Pedersen, A., et al. (2014). Short-term ecophysiological and biochemical responses of Cystoseira tamariscifolia and Ellisolandia elongata to environmental changes. Aquat. Biol. 22, 227–243. doi: 10.3354/ab00573
Cerrano, C., Bavestrello, G., Bianchi, C. N., Cattaneo-Vietti, R., Bava, S., Morganti, C., et al. (2000). A catastrophic mass-mortality episode of gorgonians and other organisms in the Ligurian Sea (NW Mediterranean), summer 1999. Ecol. Lett. 3, 284–293. doi: 10.1046/j.1461-0248.2000.00152.x
Chamberlain, Y. M. (1985). The typification of Melobesia membranacea (Esper) Lamouroux (Rhodophyta, Corallinaceae). Taxon 34, 673–677. doi: 10.2307/1222213
Chamberlain, Y. M. (1997). Observations on Lithophyllum lichenoides Philippi (Rhodophyta, Corallinaceae) and its reproductive structures. Cryptog. Algol. 18, 139–149.
Chatalov, A., Bonev, N., and Ivanova, D. (2015). Depositional characteristics and constraints on the mid-Valanginian demise of a carbonate platform in the intra-Tethyan domain, Circum-Rhodope Belt, northern Greece. Cret. Res. 55, 84–115. doi: 10.1016/j.cretres.2015.02.001
Checconi, A. (2006). Le Associazioni ad Alghe Calcaree Corallinacee (Corallinales, Rhodophyta) delle Successioni Carbonatiche d'acqua Bassa del Miocene Inferiore-Medio dell'Appennino Meridionale (Formazione di Cusano): analisi paleoecologica e paleoambientale (Ph.D. thesis). Università degli Studi di Perugia, Perugia, Italy.
Checconi, A., Bassi, D., Passeri, L., and Rettori, R. (2007). Coralline red algal assemblage from the Middle Pliocene shallow-water temperate carbonates of the Monte Cetona (Northern Apennines, Italy). Facies 53, 57–66. doi: 10.1007/s10347-006-0085-x
Coletti, G., Basso, D., and Corselli, C. (2018a). Coralline algae as depth indicators in the Sommières Basin (early Miocene, southern France). Geobios 51, 15–30. doi: 10.1016/j.geobios.2017.12.002
Coletti, G., Bracchi, V. A., Marchese, F., Basso, D., Savini, A., Vertino, A., et al. (2018b). Quaternary build-ups and rhodalgal carbonates along the Adriatic and Ionian coasts of the Italian Peninsula: a review. Riv. It. Paleontol. Stratig. 124, 387–406. doi: 10.13130/2039-4942/10269
Coll, M., Piroddi, C., Steenbek, J., Kaschner, K., Lasram, F. B. R., Aguzzi, J., et al. (2010). The biodiversity of the Mediterraneran Sea: estimates, patterns and threats. PLoS ONE 5:e11842. doi: 10.1371/journal.pone.0011842
Coma, R., Ribes, M., Serrano, E., Jiménez, E., Salat, J., and Pascual, J. (2009). Global warming-enhanced stratification and mass mortality events in the Mediterranean. Proc. Nat. Acad. Sci U.S.A. 106, 6176–6181. doi: 10.1073/pnas.0805801106
Conrad, M. A., and Masse, J. P. (1989). Les algues calcaires des formations carbonatées de l'Hauterivien-Barrémien pro partedu Jura vaudois et neuchâtelois (Suisse). Mém. Soc. Neuchâteloise Sci. Nat. 11, 277–290.
Conti, S. (1950). Alghe Corallinacee fossili. Pubbl. Ist. Geol. Univ. Genova, Quad. 4 ser. A, 1–156.
Coppejans, E. (1978). Sur les propagules de Fosliella farinosa (Lamouroux) Howe var. farinosa (Rhodophyceae - Cryptonemiales). Bull. Soc. Roy. Bot. Belg. 111, 55–61.
Cormaci, M., and Furnari, G. (1988). Sulla presenza nell'Italia meridionale di alcune alghe marine bentoniche rare per il Mediterraneo. Giorn. Bot. Ital. 122, 215–226. doi: 10.1080/11263508809429401
Cormaci, M., Furnari, G., and Alongi, G. (2017). Flora marina bentonica del Mediterraneo: Rhodophyta (Rhodymeniophycidae escluse). Boll. Acc. Gioenia Sci. Nat. 50, 1–391.
Cormaci, M., Furnari, G., Alongi, M., Catra, M., and Serio, D. (2000). The benthic algal flora on rocky substrata of the Tremiti Islands (Adriatic Sea). Plant Biosyst. 134, 133–152. doi: 10.1080/11263500012331358404
Cormaci, M., Lanfranco, E., Borg, J. A., Buttigieg, S., Furnari, G., Micallef, S. A., et al. (1997). Contribution to the knowledge of benthic marine algae on rocky substrata of the Maltese Islands (Mediterranean Sea). Bot. Mar. 40, 203–215. doi: 10.1515/botm.1997.40.1-6.203
Cornwall, C. E., Comeau, S., and McCulloch, M. T. (2017). Coralline algae elevate pH at the site of calcification under ocean acidification. Glob. Change Biol. 23, 4245–4256. doi: 10.1111/gcb.13673
Cox, T. E., Díaz-Castañeda, V., Martin, S., Alliouane, S., Mahacek, P., Le Fur, A., et al. (2017a). Effects of in situ CO2 enrichment on epibiont settlement on artificial substrata within a Posidonia oceanica meadow. J. Exp. Mar. Biol. Ecol. 497, 197–211. doi: 10.1016/j.jembe.2017.10.003
Cox, T. E., Nash, M., Gazeau, F., Déniel, M., Legrand, E., Alliouane, S., et al. (2017b). Effects of in situ CO2 enrichment on Posidonia oceanica epiphytic community composition and mineralogy. Mar. Biol. 164:103. doi: 10.1007/s00227-017-3136-7
Cox, T. E., Schenone, S., Delille, J., Díaz-Castañeda, V., Alliouane, S., Gattuso, J. P., et al. (2015). Effects of ocean acidification on Posidonia oceanica epiphytic community and shoot productivity. J. Ecol. 103, 1594–1609. doi: 10.1111/1365-2745.12477
Cumani, F., Bradassi, F., Di Pascoli, A., and Bressan, G. (2010). Marine acidification effects on reproduction and growth rates of Corallinaceae spores (Rhodophyta), Rapp. Comm. Int. Mer Medit. 39:735.
De Clerck, O., Guiry, M. D., Leliaert, F., Samyn, Y., and Verbruggen, H. (2013). Algal taxonomy: a road to nowhere? J. Phycol. 49, 215–225. doi: 10.1111/jpy.12020
De Jode, A., David, R., Haguenauer, A., Cahill, A., Erga, Z., Guillemain, D., et al. (2019). From seascape ecology to population genomics and back. Spatial and ecological differentiation among cryptic species of the red algae Lithophyllum stictiforme/L. cabiochiae, main bioconstructors of coralligenous habitats. Mol. Phylogen. Evol. 137, 104–113. doi: 10.1016/j.ympev.2019.04.005
Di Geronimo, R. (1998). Le Corallinales del quaternario dell'Italia meridionale (sistematica, paleoecologia e paleobiogeografia) (Ph.D. thesis). Università di Messina, Messina, Italy.
Diaz-Pulido, G., Nash, M. C., Anthony, K. R. N., Bender, D., Opdyke, B. N., Reyes-Nivia, C., et al. (2014). Greenhouse conditions induce mineralogical changes and dolomite accumulation in coralline algae on tropical reefs. Nat. Commun. 5:3310. doi: 10.1038/ncomms4310
Donnarumma, L., Lombardi, C., Cocito, S., and Gambi, M. C. (2014). Settlement pattern of Posidonia oceanica epibionts along a gradient of ocean acidification: an approach with mimics. Medit. Mar. Sci. 15, 498–509. doi: 10.12681/mms.677
Egan, S., Harder, T., Burke, C., Steinberg, P., Kjellenberg, S., and Thomas, T. (2012). The seaweed holobiont: understanding seaweed-bacteria interactions. FEMS Microbiol. Rev. 37, 462–476. doi: 10.1111/1574-6976.12011
Egilsdottir, H., Noisette, F., Laure, M. L. N., Olafsson, J., and Martin, S. (2013). Effects of pCO2 on physiology and skeletal mineralogy in a tidal pool coralline alga, Corallina elongata. Mar. Biol. 160, 2103–2112. doi: 10.1007/s00227-012-2090-7
El Haikali, B., Bensoussan, N., Romano, J. C., and Bousquet, V. (2004). Estimation of photosynthesis, and calcification rates of Corallina elongata Ellis et Solander, 1786, by measurements of dissolved oxygen, pH and total alkalinity. Sci. Mar. 68, 45–56. doi: 10.3989/scimar.2004.68n145
El Maghraby, D. M., and Fakhry, E. M. (2015). Lipid content and fatty acid composition of Mediterranean macro-algae as dynamic factors for biodiesel production. Oceanologia 57, 86–92. doi: 10.1016/j.oceano.2014.08.001
Ellis, J. (1768). Extract of a letter from John Ellis, Esquire, F.R.S. to Dr. Linnaeus of Upsala, F.R.S. on the animal nature of the genus of zoophytes, called Corallina. Phil. Trans. Roy. Soc. London 57, 404–425. doi: 10.1098/rstl.1767.0042
Ellis, J., and Solander, D. (1786). The Natural History of Many Curious and Uncommon Zoophytes, Collected From Various Parts of the Globe. London: Benjamin White and Son.
Esper, E. J. C. (1796). Fortsetzungen der Pflanzenthiere in Abbildungen nach der Natur mit Farben erleuchtet nebst Beschreibungen. Nuremberg: Lieferung 5.
Falace, A., Kaleb, S., Orlando-Bonaca, M., Mavrič, B., and Lipej, L. (2011). First contribution to the knowledge of coralline algae distribution in the Slovenian circalittoral zone (Northern Adriatic). Ann. Ser. Hist. Nat. 21, 27–40.
Feldmann, J. (1939). Les algues marines de la Côte des Albères. IV. Rhodophycées. Rev. Algol. 11, 247–330.
Figueroa, F. L. (1993). Photoregulation of nitrogen metabolism and protein accumulation in the red alga Corallina elongata Ellis et Soland. Z. Naturforsch. 48, 788–794. doi: 10.1515/znc-1993-9-1017
Figueroa, F. L., Barufi Bonomi, J., Malta, E. J., Conde-Álvarez, R., Nitschke, U., Arenas, F., et al. (2014a). Short-term effects of increasing CO2, nitrate and temperature on three Mediterranean macroalgae: biochemical composition. Aquat. Biol. 22, 177–193. doi: 10.3354/ab00610
Figueroa, F. L., Domínguez-González, B., and Korbee, N. (2014b). Vulnerability and acclimation to increased UVB radiation in three intertidal macroalgae of different morpho-functional groups. Mar. Environ. Res. 97, 30–38. doi: 10.1016/j.marenvres.2014.01.009
Fine, M., Tsadok, R., Meron, D., Cohen, S., and Milazzo, M. (2017). Environmental sensitivity of Neogoniolithon brassica-florida associated with vermetid reefs in the Mediterranean Sea. ICES J. Mar. Sci. 74, 1074–1082. doi: 10.1093/icesjms/fsw167
Flecha, S., Pérez, F. F., García-Lafuente, J., Sammartino, S., Ríos, A. F., and Huertas, I. E. (2015). Trends of pH decrease in the Mediterranean Sea through high frequency observational data: indication of ocean acidification in the basin. Sci. Rep. 5:16770. doi: 10.1038/srep16770
Fravega, P., Giammarino, S., Piazza, M., Russo, A., and Vannucci, G. (1987). Significato paleoecologico degli episodi coralgali a Nord di Sassello. Nuovi dati per una ricostruzione paleogeografica-evolutiva del margine meridionale del Bacino Terziario del Piemonte. Atti Soc. Tosc. Sc. Nat. Mem. Ser. 94, 19–76.
Friedrich, O., Richard, D., Norris, R. D., and Erbacheret, J. (2012). Evolution of middle to Late Cretaceous oceans - A 55 m.y. record of Earth's temperature and carbon cycle. Geology 40, 107–110. doi: 10.1130/G32701.1
Furnari, F., Cormaci, G., and Alongi, G. (1996). Lithophyllum frondosum (Dufour) comb. nov. (Corallinaceae, Rhodophyta): the species to which Mediterranean “Pseudolithophyllum expansum” should be referred. Eur. J. Phycol. 31, 117–122. doi: 10.1080/09670269600651281
Gabrielson, P. W., Hughey, J. R., and Diaz-Pulido, G. (2018). Genomics reveals abundant speciation in the coral reef building alga Porolithon onkodes (Corallinales, Rhodophyta). J. Phycol. 54, 429–434. doi: 10.1111/jpy.12761
Galli, G., Solidoro, C., and Lovato, T. (2017). Marine heat waves hazard 3D maps and the risk for low motility organisms in a warming Mediterranean Sea. Front. Mar. Sci. 4:136. doi: 10.3389/fmars.2017.00136
Garrabou, J., and Ballesteros, E. (2000). Growth of Mesophyllum alternans and Lithophyllum frondosum (Corallinales, Rhodophyta) in the northwestern Mediterranean. Eur. J. Phycol. 35, 1–10. doi: 10.1080/09670260010001735571
Gómez-Garreta, A. (1981). Estudio fenologico de la vegetation marina de la isla de Mallorca (Ph.D. thesis). Universitat de Barcelona, Barcelona, Spain.
Gómez-Garreta, A., Ribera Siguan, A., and Seoane Camba, J. A. (1982). Aportacion al estudio fenologico de las algas de la isla de Mallorca. Boll. Soc. Hist. Nat. Balears 26, 37–62.
Goyet, C., Hassoun, A. E. R., Gemayel, E., Touratier, F., Saab, M. A. A., and Guglielmi, V. (2016). Thermodynamic forecasts of the mediterranean sea acidification. Mediterr. Mar. Sci. 17, 508–518. doi: 10.12681/mms.1487
Graham, L. E., Graham, J. M., Wilcox, L. W., and Cook, M. E. (2018). Algae. 3rd Edn. Madison, WI: LJLM Press, LLC.
Guiry, M. D. (1990). “Sporangia and spores” in Biology of the Red Algae, eds K. M. Cole and R.G. Sheath (Cambridge: Cambridge University Press), 347–376.
Guiry, M. D., and Guiry, G. M. (2019). AlgaeBase. World-wide electronic publication, National University of Ireland, Galway. Available online at http://www.algaebase.org (last accessed July 30, 2019).
Guy-Haim, T., Silverman, J., Raddatz, S., Wahl, M., Israel, A., and Rilov, G. (2016). The carbon turnover response to thermal stress of a dominant coralline alga on the fast warming Levant coast. Limnol. Oceanogr. 61, 1120–1133. doi: 10.1002/lno.10279
Häder, D.-P., Herrmann, H., Schafer, J., and Santas, R. (1996). Photosynthetic fluorescence induction and oxygen production in corallinacean algae measured on site. Bot. Acta 109, 285–291. doi: 10.1111/j.1438-8677.1996.tb00575.x
Häder, D.-P., Lebert, M., Flores-Moya, A., Jiménez, C., Mercado, J., Salles, S., et al. (1997). Effects of solar radiation on the photosynthetic activity of the red alga Corallina elongata Ellis et Soland. J. Photochem. Photobiol. 37, 196–202. doi: 10.1016/S1011-1344(96)07402-7
Hale, R., Calosi, P., McNeill, L., Mieszkowska, N., and Widdicombe, S. (2011). Predicted levels of future ocean acidification and temperature rise could alter community structure and biodiversity in marine benthic communities. Oikos 120, 661–674. doi: 10.1111/j.1600-0706.2010.19469.x
Halfar, J., and Mutti, M. (2005). Global dominance of coralline red algal facies: a response to Miocene oceanographic events. Geology 33, 481–484. doi: 10.1130/G21462.1
Hall-Spencer, J. M., Rodolfo-Metalpa, R., Martin, S., Ransome, E., Fine, M., Turner, S. M., et al. (2008). Volcanic carbon dioxide vents show ecosystem effects of ocean acidification. Nature 454, 96–99. doi: 10.1038/nature07051
Hamel, G., and Lemoine, M. (1953). “Corallinacées de France e d'Afrique du Nord,” in Arch Mus. Nat. Hist. Nat. Paris 1, 15–136.
Harley, C. D. G., Anderson, K. M., Demes, K. W., Jorve, J. P., Kordas, R. L., Coyle, T. A., et al. (2012). Effects of climate change on global seaweed communities. J. Phycol. 48, 1064–1078. doi: 10.1111/j.1529-8817.2012.01224.x
Harzhauser, M., and Piller, W. E. (2007). Benchmark data of a changing sea - Palaeogeography, palaeobiogeography and events in the Central Paratethys during the Miocene. Palaeogeogr. Palaeoclimatol. Palaeoecol. 253, 8–31. doi: 10.1016/j.palaeo.2007.03.031
Hassoun, A. E. R., Gemayel, E., Krasakopoulou, E., Goyet, C., Saab, M. A. A., Gugliemi, V., et al. (2015). Acidification of the Mediterranean sea from anthropogenic carbon penetration. Deep Sea Res. 102, 1–15. doi: 10.1016/j.dsr.2015.04.005
Hereu, B., and Kersting, D. K. (2016). Diseases of coralline algae in the Mediterranean Sea. Coral Reefs 35:713. doi: 10.1007/s00338-016-1428-x
Hernández, R. Y. S., Zucchetti, M., Aumento, F., Gual, M. R., Cozzella, M. L., and Hernández, C. M. A. (2011). Measurement of plutonium pollution in sediments and algae in marine environment: cienfuegos bay and la Maddalena Islands. Fresenius Environ. Bull. 20, 802–809.
Hernandez-Kantun, J. J., Gabrielson, P. W., Hughey, J. R., Pezzolesi, L., Rindi, F., Robinson, N. M., et al. (2016). Reassessment of branched Lithophyllum spp. (Corallinales, Rhodophyta) in the Caribbean Sea with global implications. Phycologia 55, 609–635. doi: 10.2216/16-7.1
Hernandez-Kantun, J. J., Rindi, F., Adey, W. A., Heesch, S., Peña, V., Le Gall, L., et al. (2015a). Sequencing type material resolves the identity and distribution of the generitype Lithophyllum incrustans, and related European species L. hibernicum and L. bathyporum (Corallinales, Rhodophyta). J. Phycol. 51, 791–807. doi: 10.1111/jpy.12319
Hernandez-Kantun, J. J., Riosmena-Rodriguez, R., Hall-Spencer, J. M., Peña, V., Maggs, C. A., and Rindi, F. (2015b). Phylogenetic analysis of rhodolith formation in the Corallinales (Rhodophyta). Eur. J. Phycol. 50, 46–61. doi: 10.1080/09670262.2014.984347
Hind, K. R., Gabrielson, P. W., Jensen, C., and Martone, P. T. (2016). Crusticorallina gen. nov., a nongeniculate genus in the subfamily Corallinoideae (Corallinales, Rhodophyta). J. Phycol. 52, 929–941. doi: 10.1111/jpy.12449
Hind, K. R., Gabrielson, P. W., and Saunders, G. W. (2014). Molecular-assisted alpha taxonomy reveals pseudocryptic diversity among species of Bossiella (Corallinales, Rhodophyta) in the eastern Pacific Ocean. Phycologia 53, 443–456. doi: 10.2216/13-239.1
Hind, K. R., Starko, S., Burt, J. M., Lemay, M. A., Salomon, A. K., and Martone, P. T. (2019). Trophic control of cryptic coralline algal diversity. Proc. Nat. Acad. Sci. U.S. A. 116, 15080–15085. doi: 10.1073/pnas.1900506116
Howes, E. L., Stemmann, L., Assailly, C., Irisson, J.-O., Dima, M., Bijma, J., et al. (2015). Pteropod time series from the North Western Mediterranean (1967–2003): impacts of pH and climate variability. Mar. Ecol. Prog. Ser. 531, 193–206. doi: 10.3354/meps11322
Hrabovský, J., Basso, D., and Doláková, N. (2016). Diagnostic characters in fossil coralline algae (Corallinophycidae: Rhodophyta) from the Miocene of southern Moravia (Carpathian Foredeep, Czech Republic). J. Syst. Paleontol. 14, 499–525. doi: 10.1080/14772019.2015.1071501
Hsü, K. J., Montadert, L., Bernoulli, D., Cita, M. B., Erickson, A., Garrison, R. E., et al. (1977). History of the Messinian salinity crisis. Nature 267, 399–403. doi: 10.1038/267399a0
Hughey, J. R., Braga, J. C., Aguirre, J., Woelkerling, W. J., and Webster, J. M. (2008). Analysis of ancient DNA from fossil corallines (Corallinales, Rhodophyta). J. Phycol. 44, 374–383. doi: 10.1111/j.1529-8817.2008.00462.x
Huvé, H. (1962). Taxonomie, écologie et distribution d'une mélobesiée méditerraneenne: Lithophyllum papillosum (Zanardini) comb. nov., non Lithophyllum (Dermatolithon) papillosum (Zanard.) Foslie. Bot. Mar. 4, 219–240. doi: 10.1515/botm.1962.4.3-4.219
Huvé, P. (1954). Etude expérimentale de la réinstallation d'un “trottoir à Tenarea,” en Méditerranée occidentale. C.R. Acad. Sci. Fr. 239, 323–325.
Huvé, P. (1956a). Sur la réinstallation d'un “trottoir à Tenarea” en Méditerranée occidentale. C.R. Acad. Sci. Fr. 243, 2157–2158.
Huvé, P. (1956b). Résultats sommaires de l'étude expérimentale de la réinstallation d'un “trottoir à Tenarea” en Méditerranée occidentale. Rapp. P.V. Commiss. internation. Explor. Sci. Mer Médit. 14, 429–448.
Huvé, P. (1970). Recherches sur la genèse de quelques peuplements algaux marins dela roche littorale dans la région de Marseille (Ph.D. thesis). Comité National de la Recherche Scientifique, Paris, France.
Ingrosso, G., Abbiati, M., Badalamenti, F., Bavestrello, G., Belmonte, G., Cannas, R., et al. (2018). Mediterranean bioconstructions along the Italian coast. Adv. Mar. Biol. 79, 61–136. doi: 10.1016/bs.amb.2018.05.001
Irvine, L. M., and Chamberlain, Y. M. (1994). Seaweeds of the British Isles. Vol. 1, Rhodophyta, Part 2B. Corallinales, Hildenbrandiales. London: The Natural History Museum.
Ismail-Ben Ali, A., El Bour, M., Ktari, L., Bolhuis, H., Ahmed, M., Bouddabous, A., et al. (2012). Jania rubens-associated bacteria: molecular identification and antimicrobial activity. J. Appl. Phycol. 24, 525–534. doi: 10.1007/s10811-011-9758-0
Kaleb, S., Falace, A., Sartoni, G., and Woelkerling, W. J. (2011). Morphology-anatomy of Mesophyllum macroblastum (Hapalidiaceae, Corallinales, Rhodophyta) in the Northern Adriatic Sea and a key to Mediterranean species of the genus. Cryptog. Algol. 32, 223–242. doi: 10.7872/crya.v32.iss3.2011.223
Kaleb, S., Falace, A., and Woelkerling, W. J. (2012). Phymatolithon lamii (Hapalidiaceae, Corallinales, Rhodophyta): a first report for the Mediterranean Sea. Bot. Mar. 55, 377–386. doi: 10.1515/bot-2012-0141
Kamenos, N. A., Burdett, H. L., Aloisio, E., Findlay, H. S., Martin, S., Longbone, C., et al. (2013). Coralline algal structure is more sensitive to rate, rather than the magnitude, of ocean acidification. Glob. Change Biol. 19, 3621–3628. doi: 10.1111/gcb.12351
Kamenos, N. A., Cusack, M., and Moore, P. G. (2008). Coralline algae are global palaeothermometers with bi-weekly resolution. Geochim. Cosmochim. Ac. 72, 771–779. doi: 10.1016/j.gca.2007.11.019
Kamenos, N. A., Perna, G., Gambi, M. C., Micheli, F., and Kroeker, K. J. (2016). Coralline algae in a naturally acidified ecosystem persist by maintaining control of skeletal mineralogy and size. Proc. R. Soc. B. 283:20161159. doi: 10.1098/rspb.2016.1159
Kapsenberg, L., Alliouane, S., Gazeau, F., Mousseau, L., and Gattuso, J.-P. (2017). Coastal ocean acidification and increasing total alkalinity in the northwestern Mediterranean Sea. Ocean Sci. 13, 411–426. doi: 10.5194/os-13-411-2017
Kato, A., Baba, M., and Suda, S. (2013). Taxonomic circumscription of heterogeneous species Neogoniolithon brassica-florida (Corallinales, Rhodophyta) in Japan. Phycol. Res. 61, 15–26. doi: 10.1111/j.1440-1835.2012.00665.x
Kerrison, P., Hall-Spencer, J. M., Suggett, D. J., Hepburn, L. J., and Steinke, M. (2011). Assessment of pH variability at a coastal CO2 vent for ocean acidification studies. Estuar. Coast. Shelf Sci. 94, 129–137. doi: 10.1016/j.ecss.2011.05.025
Khairy, H. M., and El-Sheikh, M. A. (2015). Antioxidant activity and mineral composition of three Mediterranean common seaweeds from Abu-Qir Bay, Egypt. Saudi J. Biol. Sci. 22, 623–630. doi: 10.1016/j.sjbs.2015.01.010
Kiessling, W. (2010). Geologic and biologic controls on the evolution of reefs. Ann. Rev. Ecol. Evol. Syst. 40, 173–192. doi: 10.1146/annurev.ecolsys.110308.120251
Koch, M., Bowes, G., Ross, C., and Zhang, X. H. (2013). Climate change and ocean acidification effects on seagrasses and marine macroalgae. Glob. Change Biol. 19, 103–132. doi: 10.1111/j.1365-2486.2012.02791.x
Korbee, N., Navarro, N. P., García-Sánchez, M., Celis-Plá, P. S. M., Quintano, E., Copertino, M. S., et al. (2014). A novel in situ system to evaluate the effect of high CO2 on photosynthesis and biochemistry of seaweeds. Aquat. Biol. 22, 245–259. doi: 10.3354/ab00594
Kroeker, K. J., Micheli, F., and Gambi, M. C. (2012). Ocean acidification causes ecosystem shifts via altered competitive interactions. Nat. Clim. Change 3, 156–159. doi: 10.1038/nclimate1680
Kuffner, I. B., Andersson, A. J., Jokiel, P. L., Rodgers, K. S., and Mackenzie, F. T. (2008). Decreased abundance of crustose coralline algae due to ocean acidification. Nat. Geosci. 1, 114–117. doi: 10.1038/ngeo100
Laborel, J. (1987). Marine biogenic constructions in the Mediterranean, a review. Sci. Rep. Port-Cros Nat. Park 13, 97–126.
Laborel, J., Morhange, C., and Laborel-Denguen, F. (1993). Dégradation récente des formations construites superficielles à Lithophyllum lichenoides Philippi dans la Réserve marine de Scandola. Trav. Sci. Parc Nat. Rég. Rés. Nat. Corse. 41, 19–23.
Laib, E., and Legouchi, E. (2012). Cd, Cr, Cu, Pb, and Zn concentrations in Ulva lactuca, Codium fragile, Jania rubens, and Dictyota dichotoma from Rabta Bay, Jijel (Algeria). Environ. Monitor. Assess. 184, 1711–1718. doi: 10.1007/s10661-011-2072-0
Langar, H., Bessibes, M., Djellouli, A., Pergent-Martini, C., and Pergent, G. (2011). The Neogoniolithon brassica-florida (Harvey) Setchell and LR Mason (1943) Reef of Bahiret el Bibane Lagoon (Southeastern Tunisia). J. Coast. Res. 27, 394–398. doi: 10.2112/JCOASTRES-D-10-00082.1
Legrand, E., Riera, P., Lutier, M., Coudret, J., Grall, J., and Martin, S. (2017). Species interactions can shift the response of a maerl bed community to ocean acidification and warming. Biogeosciences 14, 5359–5376. doi: 10.5194/bg-14-5359-2017
Lejeusne, C., Chevaldonné, P., Pergent-Martini, C., Boudouresque, C.-F., and Pérez, T. (2010). Climate change effects on a miniature ocean: the highly diverse, highly impacted Mediterranean Sea. Tr. Ecol. Evol. 25, 250–260. doi: 10.1016/j.tree.2009.10.009
Lemoine, M. P. (1939). Algues calcaires fossiles de l'Algérie. Matériaux pour la Carte Géologique de L'Algérie. 1re Série Paléontol. 9, 1–128.
Lemoine, M. P. (1970). Les algues floridées calcaires du Crétacé du sud de la France. Arch. Mus. Nat. d'Hist. Nat. 7, 129–240.
Lemoine, M. P. (1977). Étude d'une collection d'algues corallinacées de la région de Skopje (Yougoslavie). Rev. Micropaléont. 20, 10–42.
Linnaeus, C. (1758). Systema Naturae per Regna Tria Naturae, Secundum Classes, Ordines, Genera, Species, Cum Characteribus, Differentiis, Synonymis, Locis. Holmiae: Impensis direct. Laurentii Salvi.
López-Figueroa, F., and Niell, F. X. (1990). Effects of light quality on chlorophyll and biliprotein accumulation in seaweeds. Mar. Biol. 104, 321–327. doi: 10.1007/BF01313274
López-Figueroa, F., Perez, R., and Niell, F. X. (1989). Effects of red and far-red light pulses on the chlorophyll and biliprotein accumulation in the red alga Corallina elongata. J. Photochem. Photobiol. 4, 185–193. doi: 10.1016/1011-1344(89)80004-1
Lopez-Horgue, M. A., Owen, H. G., Aranburu, A., Fernandez-Mendiola, P. A., and Garcia-Mondéjar, J. (2009). Early late Albian (Cretaceous) of the central region of the Basque-Cantabrian Basin, northern Spain: biostratigraphy based on ammonites and orbitolinids. Cret. Res. 30, 385–400. doi: 10.1016/j.cretres.2008.08.001
Lüning, K. (1990). Seaweeds. Their Environment, Biogeography, and Ecophysiology. London: Wiley Interscience.
Marbà, N., and Duarte, C. M. (2010). Mediterranean warming triggers seagrass (Posidonia oceanica) shoot mortality. Glob. Change Biol. 16, 2366–2375. doi: 10.1111/j.1365-2486.2009.02130.x
Marchini, A., Ragazzola, F., Vasapollo, C., Castelli, A., Cerrati, G., Gazzola, F., et al. (2019). Intertidal Mediterranean coralline algae habitat is expecting a shift toward a reduced growth and a simplified associated fauna under climate change. Front. Mar. Sci. 6:106. doi: 10.3389/fmars.2019.00106
Mariotti, A, Struglia, M. V., Zeng, N., and Lau, K. M. (2002). The hydrological cycle in the Mediterranean region and implications for the water budget of the Mediterranean Sea. J. Climate 15, 1674–1690. doi: 10.1175/1520-0442(2002)015<1674:THCITM>2.0.CO;2
Martin, C. S., Giannoulaki, M., De Leo, F., Scardi, M., Salomidi, M., Knittweis, L., et al. (2014). Coralligenous and maerl habitats: predictive modeling to identify their spatial distributions across the Mediterranean Sea. Sci. Rep. 4:5073. doi: 10.1038/srep06646
Martin, S., Castets, M. D., and Clavier, J. (2006). Primary production, respiration and calcification of the temperate free-living coralline alga Lithothamnion corallioides. Aquat. Bot. 85, 121–128. doi: 10.1016/j.aquabot.2006.02.005
Martin, S., Charnoz, A., and Gattuso, J. P. (2013b). Photosynthesis, respiration and calcification in the Mediterranean crustose coralline alga Lithophyllum cabiochae (Corallinales, Rhodophyta). Eur. J. Phycol. 48, 163–172. doi: 10.1080/09670262.2013.786790
Martin, S., Cohu, S., Vignot, C., Zimmerman, G., and Gattuso, J. P. (2013a). One-year experiment on the physiological response of the Mediterranean crustose coralline alga, Lithophyllum cabiochae, to elevated pCO2 and temperature. Ecol. Evol. 3, 676–693. doi: 10.1002/ece3.475
Martin, S., and Gattuso, J. P. (2009). Response of Mediterranean coralline algae to ocean acidification and elevated temperature. Glob. Change Biol. 15, 2089–2100. doi: 10.1111/j.1365-2486.2009.01874.x
Martin, S., and Hall-Spencer, J. M. (2017). “Effects of ocean warming and acidification on rhodolith/maërl beds” in Rhodolith/maërl beds: a global perspective, eds R. Riosmena-Rodríguez, W. Nelson, and J. Aguirre (Basel: Springer International Publishing), 55–85. doi: 10.1007/978-3-319-29315-8_3
Martin, S., Rodolfo-Metalpa, R., Ransome, E., Rowley, S., Buia, M. C., Gattuso, J. P., et al. (2008). Effects of naturally acidified seawater on seagrass calcareous epibionts. Biol. Lett. 4, 689–692. doi: 10.1098/rsbl.2008.0412
Maslov, V. P. (1956). Fossil Calcareous Algae of USSR (in Russian). Trudy Inst. Geol. Nauk Akad. Nauk SSSR 160, 1–301.
Mastrorilli, V. I. (1968). Nuovo contributo allo studio delle Corallinacee dell'Oligocene Ligure-Piemontese: i reperti della tavoletta Ponzone. Atti Ist. Geol. Univ. Genova 5, 153–406.
McCoy, S. J., and Kamenos, N. A. (2015). Coralline algae (Rhodophyta) in a changing world: integrating ecological, physiological, and geochemical responses to global change. J. Phycol. 51, 6–24. doi: 10.1111/jpy.12262
Miller, K. G., Mountain, G. S., Wright, J. D., and Browning, J. V. (2011). A 180-million-year record of sea level and ice volume variations from continental margin and deep-sea isotopic records. Oceanography 24, 40–53. doi: 10.5670/oceanog.2011.26
Morse, J. W., Arvidson, R. S., and Lüttge, A. (2007). Calcium carbonate formation and dissolution. Chem. Rev. 107, 342–381. doi: 10.1021/cr050358j
Moussavian, E. (1993). “Eocene corallinacean-dominated localities of the Helvetic zone / Upper Bavaria,” in Facial Development of Algae-Bearing Carbonate Sequences in the Eastern Alps. Field Trip Guidebook, Munich. Excursion B1, eds R. Höfling, E. Moussavian, and W.E. Piller (Munich: Alpine Algae, International Symposium on Fossil Algae), 1–11.
Nalin, R., Basso, D., and Massari, F. (2006). “Pleistocene coralline algal build-ups (coralligéne de plateau) and associated bioclastic deposits in the sedimentary cover of Cutro marine terrace (Calabria, southern Italy)”, in Cool-water carbonates: depositional systems and palaeoenvironmental controls, eds H.M. Pedley, and G. Carannante (London: Geol. Soc. Spec. Publ. 255), 11–22. doi: 10.1144/GSL.SP.2006.255.01.02
Nannini, M., De Marchi, L., Lombardi, L., and Ragazzola, F. (2015). Effects of thermal stress on the growth of an intertidal population of Ellisolandia elongata (Rhodophyta) from N-W Mediterranean Sea. Mar. Environ. Res. 112, 11–19. doi: 10.1016/j.marenvres.2015.05.005
Nash, M. C., Martin, S., and Gattuso, J. P. (2016). Mineralogical response of the Mediterranean crustose coralline alga Lithophyllum cabiochae to near-future ocean acidification and warming. Biogeosciences 13, 5937–5945. doi: 10.5194/bg-13-5937-2016
Nash, M. C., Uthicke, S., Negri, A. P., and Cantin, N. E. (2015). Ocean acidification does not affect magnesium composition or dolomite formation in living crustose coralline algae, Porolithon onkodes in an experimental system. Biogeosciences 12, 5247–5260. doi: 10.5194/bg-12-5247-2015
Nebelsick, J., Rasser, M., and Bassi, D. (2005). Facies dynamics in Eocene to Oligocene circumalpine carbonates. Facies 51, 197–216. doi: 10.1007/s10347-005-0069-2
Nelson, W. A., Sutherland, J. E., Farr, T. J., Hart, D. R., Neill, K. F., Kim, H. J., et al. (2015). Multi-gene phylogenetic analyses of New Zealand coralline algae: Corallinapetra novaezelandiae gen. et sp. nov. and recognition of the Hapalidiales ord. nov. J. Phycol. 51, 454–468. doi: 10.1111/jpy.12288
Norris, D., Turner, S. K., Hull, P. M., and Ridgwell, A. (2013). Marine ecosystem responses to Cenozoic global change. Science 341, 492–498. doi: 10.1126/science.1240543
Norton, T. A. (1992). Dispersal by macroalgae. Br. Phycol. J. 27, 293–301. doi: 10.1080/00071619200650271
Nykjaer, L. (2009). Mediterranean Sea surface warming 1985-2006. Climate Res. 39, 11–17. doi: 10.3354/cr00794
O'Brien, C. L., Robinson, S. A., Pancost, R. D., Damsté, J. S. S., Schoutten, S., Lunt, D. J., et al. (2017). Cretaceous sea-surface temperature evolution: Constraints from TEX86 and planktonic foraminiferal oxygen isotopes. Earth-Sci. Rev. 172, 224–247. doi: 10.1016/j.earscirev.2017.07.012
Olgunoglu, M. P., and Polat, S. (2008). Trace metals in marine macroalgae samples from the Iskenderun Bay, Turkey. Fres. Environ. Bull. 17, 589–595.
Palmiéri, J., Orr, J. C., Dutay, J.-C., Béranger, K., Schneider, A., and Beuvier, J. (2015). Simulated anthropogenic CO2 storage and acidification of the Mediterranean Sea. Biogeosciences 12, 781–802. doi: 10.5194/bg-12-781-2015
Parages, M. L., Figueroa, F. L., Conde-Álvarez, R. M., and Jiménez, C. (2014). Phosphorylation of MAPK-like proteins in three intertidal macroalgae under stress conditions. Aquat. Bot. 22, 213–226. doi: 10.3354/ab00592
Pardo, C., Peña, V., Barreiro, R., and Barbara Criado, I. (2015). A molecular and morphological study of Corallina sensu lato (Corallinales, Rhodophyta) in the Atlantic Iberian Peninsula. Cryptog. Algol. 36, 31–54. doi: 10.7872/crya.v36.iss1.2015.31
Pascual, M., Rives, B., Schunter, C., and Macpherson, E. (2017). Impact of life history traits on gene flow: a multispecies systematic review across oceanographic barriers in the Mediterranean Sea. PLoS ONE 12:e0176419. doi: 10.1371/journal.pone.0176419
Patarnello, T., Volckaert, F., and Castilho, R. (2007). Pillars of Hercules: is the Atlantic-Mediterranean transition a phylogeographical break? Mol. Ecol. 16, 4426–4444. doi: 10.1111/j.1365-294X.2007.03477.x
Peña, V., Adey, W. H., Riosmena-Rodriguez, R., Jung, M. Y., Afonso-Carillo, J., Choi, H. G., et al. (2011). Mesophyllum sphaericum sp. nov. (Corallinales, Rhodophyta): a new maerl-forming species from the northeast Atlantic. J. Phycol. 47, 911–927. doi: 10.1111/j.1529-8817.2011.01015.x
Peña, V., De Clerck, O., Afonso-Carrillo, J., Ballesteros, E., Bárbara, I., Barreiro, R., et al. (2015a). An integrative systematic approach to species diversity and distribution in the genus Mesophyllum (Corallinales, Rhodophyta) in Atlantic and Mediterranean Europe. Eur. J. Phycol. 50, 20–36. doi: 10.1080/09670262.2014.981294
Peña, V., Hernández-Kantun, J. J., Adey, W. H., and Le Gall, L. (2018). Assessment of coralline species diversity in the European coasts supported by sequencing of type material: the case study of Lithophyllum nitorum (Corallinales, Rhodophyta). Cryptog. Algol. 39, 123–337. doi: 10.7872/crya/v39.iss1.2018.123
Peña, V., Hernández-Kantún, J. J., Grall, J., Pardo, C., López, L., Bárbara, I., et al. (2014). Detection of gametophytes in the maerl-forming species Phymatolithon calcareum (Melobesioideae, Corallinales) assessed by DNA barcoding. Cryptog., Algol. 35, 15–25. doi: 10.7872/crya.v35.iss1.2014.15
Peña, V., Pardo, C., López, L., Hernandez-Kantun, J. J., Adey, W. H., Bárbara, I., et al. (2015b). Phymatolithon lusitanicum sp. nov. (Hapalidiales, Rhodophyta): the third most abundant maerl-forming species in the Atlantic Iberian Peninsula. Cryptog. Algol. 36, 429–459. doi: 10.7872/crya/v36.iss4.2015.429
Perrin, C., and Kiessling, W. (2010). “Latitudinal trends in Cenozoic reef patterns and their relationship to climate” in Carbonate Systems During the Oligocene-Miocene Climatic Transition, eds M. Mutti, W.E. Piller, and C. Betzler (Oxford; Chichester; Hoboken, NJ: IAS Spec. Publ.; Wiley-Blackwell) 42, 17–34. doi: 10.1002/9781118398364.ch2
Pezzolesi, L., Falace, A., Kaleb, S., Hernandez-Kantun, J. J., Cerrano, C., and Rindi, F. (2017). Genetic and morphological variation in an ecosystem engineer, Lithophyllum byssoides (Corallinales, Rhodophyta). J. Phycol. 53, 146–160. doi: 10.1111/jpy.12488
Pezzolesi, L., Peña, V., Le Gall, L., Gabrielson, P. W., Kaleb, S., Hughey, J. R., et al. (2019). Mediterranean Lithophyllum stictiforme is a genetically diverse species complex: implications for species circumscription, biogeography and conservation of coralligenous habitats. J. Phycol. 55, 473–492. doi: 10.1111/jpy.12837
Piazzi, L., Balata, D., and Ceccherelli, G. (2015). Epiphyte assemblages of the Mediterranean seagrass Posidonia oceanica: an overview. Mar. Ecol. 37, 3–41. doi: 10.1111/maec.12331
Pickett-Heaps, J. D., West, J. A., Wilson, S. M., and McBride, D. L. (2001). Time-lapse videomicroscopy of cell (spore) movement in red algae. Eur. J. Phycol. 36, 9–22. doi: 10.1080/09670260110001735148
Poignant, A. F. (1968). Les algues des calcaires Aptiens et Albiens d'Aquitaine méridionale. Rev. Micropaléontol. 10, 271–276.
Poignant, A. F. (1978). Les algues rouges crétacées. Relations mer Boréale-Téthys. Aspekte der Kreide Europas. IUGS Ser. A 6, 273–278.
Poignant, A. F. (1981). Sur des formes nouvelles d'algues rouges crétacées. Cret. Res. 2, 187–195. doi: 10.1016/0195-6671(81)90008-2
Polat, S., and Ozogul, Y. (2009). Fatty acid, mineral and proximate composition of some seaweeds from the northeastern Mediterranean coast. Ital. J. Food Sci. 21, 317–324.
Polat, S., and Ozogul, Y. (2013). Seasonal proximate and fatty acid variations of some seaweeds from the northeastern Mediterranean coast. Oceanologia 55, 375–391. doi: 10.5697/oc.55-2.375
Popov, S. V., Rögl, F., Rozanov, A. Y., Steininger, F. F., Shcherba, I. G., and Kováè, M. (2004). Lithological-paleogeographic maps of Paratethys 10 Maps Late Eocene to Pliocene. Cour Forsch Senckenberg 250, 1–46.
Porzio, L., Buia, M. C., and Hall-Spencer, J. M. (2011). Effects of ocean acidification on macroalgal communities. J. Exp. Mar. Biol. Ecol. 400, 278–287. doi: 10.1016/j.jembe.2011.02.011
Porzio, L., Garrard, S. L., and Buia, M. C. (2013). The effect of ocean acidification on early algal colonization stages at natural CO2 vents. Mar. Biol. 160, 2247–2259. doi: 10.1007/s00227-013-2251-3
Quéré, G., Intertaglia, L., Payri, C., and Garland, P. E. (2019). Disease specific bacterial communities in a coralline alga of the northwestern Mediterranean Sea: a combined culture dependent and -independent approach. Front. Microbiol. 10:1850. doi: 10.3389/fmicb.2019.01850
Ragazzola, F., Caragnano, A., Basso, D., Schmidt, D. N., and Fietzke, J. (2019). Establishing temperate crustose early Holocene coralline algae as archives for palaeoenvironmental reconstructions of the shallow water habitats of the Mediterranean Sea. Palaeontology 1–16. doi: 10.1111/pala.12447
Rasser, M., and Nebelsick, J. (2003). Provenance analysis of Oligocene autochthonous and allochthonous coralline algae: a quantitative approach towards reconstructing transported assemblages. Palaeogeogr Palaeoclimatol. Palaeoecol. 201, 89–111. doi: 10.1016/S0031-0182(03)00512-1
Rasser, M. W. (2000). Coralline red algal limestones of the late Eocene Alpine Foreland Basin in Upper Austria: component analysis, facies and palecology. Facies 42, 59–92. doi: 10.1007/BF02562567
Richards, J. L., Gabrielson, P. W., Hughey, J. R., and Freshwater, D. W. (2018). A re-evaluation of subtidal Lithophyllum species (Corallinales, Rhodophyta) from North Carolina, USA, and the proposal of L. searlesii sp. nov. Phycologia 57, 318–330. doi: 10.2216/17-110.1
Richards, J. L., Sauvage, T., Schmidt, W. E., Fredericq, S., Hughey, J. R., and Gabrielson, P. W. (2017). The coralline genera Sporolithon and Heydrichia (Sporolithales, Rhodophyta) clarified by sequencing type material of their generitypes and other species. J. Phycol. 53, 1044–1059. doi: 10.1111/jpy.12562
Rindi, F., and Cinelli, F. (2000). Phenology and small-scale distribution of some rhodomelacean red algae on a western Mediterranean rocky shore. Eur. J. Phycol. 35, 115–125. doi: 10.1080/09670260010001735701
Rodriguez-Prieto, C. (2016). Light and temperature requirements for survival, growth and reproduction of the crustose coralline Lithophyllum stictaeforme from the Mediterranean Sea. Bot. Mar. 59, 95–104. doi: 10.1515/bot-2015-0070
Rögl, F. (1998). Palaeogeographic considerations for Mediterranean and Paratethys seaways (Oligocene to Miocene). Ann. Natur. Mus. Wien 99, 279–310.
Rohling, E. J., Foster, G. L., Grant, K. M., Marino, G., Roberts, A. P., Tamisiea, M. E., et al. (2014). Sea-level and deep-sea-temperature variability over the past 5.3 million years. Nature 508, 477–482. doi: 10.1038/nature13230
Rosales, I. (1995). La plataforma carbonatada de castro urdiales (Aptiense-Albiense, Cantabria) (Ph.D. thesis). Universidad del País Vasco, Bilbao, Spain.
Rosas-Alquicira, E. F., Riosmena-Rodriguez, R., and Neto, A. I. (2010). Typification and status of Amphiroa cryptarthrodia Zanardini (Lithophylloideae, Corallinales, Rhodophyta). Phycol Res. 58, 248–257. doi: 10.1111/j.1440-1835.2010.00584.x
Rösler, A., Perfectti, F., Peña, V., Aguirre, J., and Braga, J. C. (2017). Timing of the evolutionary history of Corallinaceae (Corallinales, Rhodophyta). J. Phycol. 53, 567–576. doi: 10.1111/jpy.12520
Rösler, A., Perfectti, F., Peña, V., and Braga, J. C. (2016). Phylogenetic relationships of Corallinaceae (Corallinales, Rhodophyta): taxonomic implications for reef-building corallines. J. Phycol. 52, 412–431. doi: 10.1111/jpy.12404
Sakalli, A. (2017). Sea surface temperature in the Mediterranean Sea under climate change: a linear model simulation of the sea surface temperature up to 2100. Appl. Ecol. Environ. Res. 15, 707–716. doi: 10.15666/aeer/1501_707716
Scheibner, C., and Speijer, R. P. (2008). Late Paleocene–early Eocene Tethyan carbonate platform evolution—a response to long- and short-term paleoclimatic change. Earth Sci. Rev. 90, 71–102. doi: 10.1016/j.earscirev.2008.07.002
Scotese, C. R. (2014a). Atlas of Early Cretaceous Paleogeographic Maps, PALEOMAP Atlas for ArcGIS, Vol. 2, The Cretaceous, Maps 23-31, Mollweide Projection, PALEOMAP Project, Evanston, IL.
Scotese, C. R. (2014b). Atlas of Paleogene Paleogeographic Maps (Mollweide Projection), Maps 8-15, Vol. 1, The Cenozoic, PALEOMAP Atlas for ArcGIS, PALEOMAP Project, Evanston, IL.
Siboni, N., Abrego, D., Evenhuis, C., Logan, M., and Motti, C. A. (2015). Adaptation to local thermal regimes by crustose coralline algae does not affect rates of recruitment in coral larvae. Coral Reefs 34, 1243–1253. doi: 10.1007/s00338-015-1346-3
Sneed, J. M., Ritson-Williams, R., and Paul, V. J. (2015). Crustose coralline algal species host distinct bacterial assemblages on their surfaces. ISME J. 9, 2527–2536. doi: 10.1038/ismej.2015.67
Soliman, R. M., Younis, S. A., El-Gendy, N. S., Mostafa, S. S. M., El-Temtamy, S. A., and Hashim, A. I. (2018). Batch bioethanol production via the biological and chemical saccharification of some Egyptian marine macroalgae. J. Appl. Microbiol. 125, 422–440. doi: 10.1111/jam.13886
Solms-Laubach, H. (1881). Die Corallinenalgen des Golfes von Neapel und der angrenzenden Meeres-Abschnitte. Fauna Fl. Neapel 4, 1–64.
Soto, J., and Conde, F. (1989). Sobre la fenologia reproductora de las algas rojas en el litoral de Sudeste de España (Alboran oriental). Acta Bot. Malacitana 14, 5–12.
Stengel, D. B., Conde-Álvarez, R., Connan, S., Nitschke, U., Arenas, F., Abreu, H., et al. (2014). Short-term effects of CO2, nutrients and temperature on three marine macroalgae under solar radiation. Aquat. Biol. 22, 159–176. doi: 10.3354/ab00576
Stockar, R. (2000). Fossil coralline algae from the Paleocene Montorfano Member type-section (Tabiago Formation, northern Italy). Ecol. Geol. Helvet. 93, 409–427.
Tomás, S., Aguirre, J., Braga, J. C., and Martín-Closas, C. (2007). Late Hauterivian coralline algae (Rhodophyta, Corallinales) from the Iberian Chain (E Spain). Taxonomy and the evolution of multisporangial reproductive structures. Facies 53, 79–95. doi: 10.1007/s10347-006-0088-7
Turland, N. J., Wiersema, J. H., Barrie, F. R., Greuter, W., Hawksworth, D. L., Herendeen, P. S., et al. (2018). International Code of Nomenclature for algae, fungi, and plants (Shenzhen Code) Adopted by the Nineteenth International Botanical Congress Shenzhen, China, July 2017. Regnum Vegetabile 159. Glashütten: Koeltz Botanical Books.
Vannucci, G., Piazza, M., Fravega, P., and Abate, C. (1996). Litostratigrafia e paleoecologia di successioni a rodoliti della “Pietra da Cantoni” (Monferrato orientale, Italia nord-occidentale). Atti Soc. Tosc. Sc. Nat. Mem. Ser. A 103, 69–86.
Verbruggen, H. (2014). Morphological complexity, plasticity, and species diagnosability in the application of old species names in DNA-based taxonomy. J. Phycol. 50, 26–31. doi: 10.1111/jpy.12155
Verlaque, M. (2001). Checklist of the macroalgae of Thau Lagoon (Hérault, France), a hot spot of marine species introduction in Europe. Oceanol. Acta 24, 29–49. doi: 10.1016/S0399-1784(00)01127-0
Verlaque, M. (2010). Field-methods to analyse the condition of Mediterranean Lithophyllum byssoides (Lamarck) Foslie rims. Sci. Rep. Port-Cros Natl. Park 24,185–196.
Walker, R. H., Brodie, J., Russell, S., Irvine, L. M., and Orfanidis, S. (2009). Biodiversity of coralline algae in the Northeastern Atlantic including Corallina caespitosa sp. nov. (Corallinoideae, Rhodophyta). J. Phycol. 45, 287–297. doi: 10.1111/j.1529-8817.2008.00637.x
Williamson, C. J., Walker, R. H., Robba, L., Yesson, C., Russell, S., Irvine, L. M., et al. (2015). Towards resolution of species diversity and distribution in the calcified red algal genera Corallina and Ellisolandia (Corallinales, Rhodophyta) and related genera. Phycologia 54, 2–11. doi: 10.2216/14-024.1
Woelkerling, W. J. (1983). A taxonomic reassessment of Lithophyllum (Corallinaceae, Rhodophyta) based on studies of R.A. Philippi's original collections. Br. Phycol. J. 183, 299–328. doi: 10.1080/00071618300650301
Woelkerling, W. J. (1985). A taxonomic reassessment of Spongites (Corallinaceae, Rhodophyta) based on studies of Kützing's original collections. Br. Phycol. J. 20, 123–153. doi: 10.1080/00071618500650151
Woelkerling, W. J., Chamberlain, Y. M., and Silva, P. C. (1985). A taxonomic and nomenclatural reassessment of Tenarea, Titanoderma and Dermatolithon (Corallinaceae, Rhodophyta) based on studies of type and other critical specimens. Phycologia 24, 317–337. doi: 10.2216/i0031-8884-24-3-317.1
Woelkerling, W. J., Granier, B., and Dias-Brito, D. (2014). Heydrichia (?) poignantii, sp. nov. (Sporolithaceae, Sporolithales, Rhodophyta), a 100 million year old fossil coralline alga from north-eastern Brazil, and a new Hauterivian record of Sporolithon from Switzerland. Carnéts Geol. 14, 139–158. doi: 10.4267/2042/53736
Woelkerling, W. J., Gustavsen, G., Myklebost, H. E., Prest,ø, T., and Såstad, S. M. (2005). The coralline red algal herbarium of Mikael Foslie: revised catalogue with analyses. Gunneria 77, 1–625.
Woelkerling, W. J., and Irvine, L. M. (1986). The neotypification and status of Phymatolithon (Corallinaceae, Rhodophyta). Br. Phycol. J. 21, 55–80. doi: 10.1080/00071618600650071
Wolf, M. A., Falace, A., Kaleb, S., and Moro, I. (2016). Molecular data confirm the existence of attached crustose tetrasporangial thalli in Phymatolithon calcareum (Melobesioideae, Hapalidiaceae, Rhodophyta) from the Mediterranean Sea. Aquat. Bot. 134, 75–81. doi: 10.1016/j.aquabot.2016.07.006
Wolf, M. A., Maneveldt, G. W., Kaleb, S., Moro, I., and Falace, A. (2015). Morphological and molecular characterization of Hydrolithon rupestre (Corallinaceae, Corallinales, Rhodophyta): first report from the Mediterranean Sea. Phytotaxa 224, 59–71. doi: 10.11646/phytotaxa.224.1.4
Zachos, J., Pagani, M., Sloan, L., Thomas, E., and Billups, K. (2001). Trends, rhythms, and aberrations in global climate 65 Ma to Present. Science 292, 686–693. doi: 10.1126/science.1059412
Zachos, J. C., Dickens, G. R., and Zeebe, R. E. (2008). An early Cenozoic perspective on greenhouse warming and carbon-cycle dynamics. Nature 451, 279–283. doi: 10.1038/nature06588
Keywords: climate change, Corallinales, ecosystem engineers, Hapalidiales, marine bioconstructions, paleontological records, Sporolithales, taxonomy
Citation: Rindi F, Braga JC, Martin S, Peña V, Le Gall L, Caragnano A and Aguirre J (2019) Coralline Algae in a Changing Mediterranean Sea: How Can We Predict Their Future, if We Do Not Know Their Present? Front. Mar. Sci. 6:723. doi: 10.3389/fmars.2019.00723
Received: 30 October 2018; Accepted: 08 November 2019;
Published: 29 November 2019.
Edited by:
Susana Carvalho, King Abdullah University of Science and Technology, Saudi ArabiaReviewed by:
Steeve Comeau, UMR7093 Laboratoire d'océanographie de Villefranche (LOV), FranceAnne Chenuil, Centre National de la Recherche Scientifique (CNRS), France
Copyright © 2019 Rindi, Braga, Martin, Peña, Le Gall, Caragnano and Aguirre. This is an open-access article distributed under the terms of the Creative Commons Attribution License (CC BY). The use, distribution or reproduction in other forums is permitted, provided the original author(s) and the copyright owner(s) are credited and that the original publication in this journal is cited, in accordance with accepted academic practice. No use, distribution or reproduction is permitted which does not comply with these terms.
*Correspondence: Fabio Rindi, Zi5yaW5kaUB1bml2cG0uaXQ=