- 1Marine Botany, Faculty Biology/Chemistry, University of Bremen, Bremen, Germany
- 2Station Biologique de Roscoff, Plateforme ABiMS, CNRS: FR2424, Sorbonne Université (UPMC), Roscoff, France
- 3Integrative Biology of Marine Models (LBI2M), Station Biologique de Roscoff, Sorbonne Université (UPMC), CNRS, Roscoff, France
- 4Institute for Plant Science and Microbiology, University of Hamburg, Hamburg, Germany
- 5Alfred Wegener Institute, Helmholtz Centre for Polar and Marine Research, Bremerhaven, Germany
- 6Institute for Biochemistry I, Medical Faculty, University of Cologne, Cologne, Germany
Saccharina latissima is an economically and ecologically relevant kelp species in Europe and North America. In kelps, the sexuality is expressed during the haploid life stage and the microscopic gametophytes exhibit significant sexual dimorphism. To understand the sex-dependent impact of temperature on the gametophyte stage, we analyzed for the first time, gene expression profiles of male and female gametophytes at three different temperatures (4, 12, and 20°C) characteristic for the species distribution range by using RNA-sequencing. We identified several differentially expressed genes (DEGs) between sexes; while female biased genes were enriched in general metabolism and energy production, male biased genes function within cell cycle and signaling. In our study, temperature modulated sex-biased gene expression, with only a small percentage of DEGs consistently male (7%) or female-biased (12%) at the three temperatures. Female gametophytes responded stronger to higher temperatures than males, suggesting that males are more heat tolerant. Differences between S. latissima and other brown algal gender-dependent gene expression might mirror the different evolutionary and ecological contexts. Genomic information on kelp gametophyte is still scarce and thus this study adds to our knowledge on sex differences in abiotic stress responses in macroalgae at the transcriptomic level.
Introduction
Kelps (order Laminariales, Phaeophyceae) feature a haplo-diplontic life cycle in which microscopic haploid gametophytes alternate with macroscopic diploid sporophytes. The two stages are very distinct: sporophytes are structured in holdfast, stipe and blade featuring different tissues (cortex and medulla) and may reach up to several meters in length, while gametophytes are composed of one to few cells (Hurd et al., 2014b). These morphological differences between the life stages entail different susceptibility to abiotic stressors (Coelho et al., 2000), namely high irradiance, ultraviolet radiation, and temperature (e.g., Bolton and Lüning, 1982; Fredersdorf et al., 2009; Gao et al., 2019). Therefore, any prediction of future impacts of global warming on kelps must not only consider the responses of the sporophytes but also of the other life-history stages (Roleda, 2016; Assis et al., 2017; Leal et al., 2018).
Reproduction in kelps is tightly connected to environmental cues. Gametophytes may enter gametogenesis under the specific combination of nutrients, light, and temperature or remain vegetative if conditions are not favorable (Martins et al., 2017; Bartsch, 2018). In the latter case, vegetative gametophytes are considered to be dormant and may act as a “seed bank” allowing for the recovery of the population after a disturbance, e.g., storms or heat-waves (Edwards, 2000; Barradas et al., 2011). Moreover, vegetative gametophytes might be more resistant than macroscopic sporophytes to heat stress during summer, especially at the rear edge of the distribution. Lee and Brinkhuis (1986) suggested that the gametophyte and/or early life stages of Saccharina latissima “oversummer” at the southern distribution limit in United States, as sporophytes are not visible during the hot summer months but are already found in early autumn. Hsiao and Druehl (1973) reported gametophyte survival and gamete production of S. latissima throughout the year, however, absence of macroscopic sporophytes during later spring and summer. Given the challenges of studying the microscopic stages in the field, the relevance of the vegetative gametophyte pool for population dynamics is not well understood (Schiel and Foster, 2006). Nevertheless, manipulation of life-cycle transitions is a common practice in aquaculture to allow for year-round production of sporophytes (Charrier et al., 2017). Control of gametogenesis starts by separating male and female gametophytes based on their sexual dimorphism. After spore release and germination, sexual dimorphism soon becomes visible: while female gametophytes are constituted of a few bigger rounder cells that tend to grow in diameter, male cells are much smaller and increase faster in cell numbers (Lüning and Neushul, 1978). Under non-fertilizing conditions, male and female gametophytes form vegetative filaments consisting of several cells that are able to continuously grow over decades (e.g., Martins et al., 2019) and may form mm-sized clumps (Bartsch, 2018). The significance of sex to differences in performance, behavior, and ecology are also currently understudied in brown algae (Luthringer et al., 2014). Sexual dimorphism is to a large extent mediated by differential gene expression between sexes in a wide variety of organisms (Ellegren and Parsch, 2007). Up to the present, only a few gametophyte gene expression datasets are available for brown algae: Ectocarpus sp. (order Ectocarpales) (Lipinska et al., 2015), Undaria pinnatifida (Shan et al., 2015), Saccharina japonica (order Laminariales) (Ye et al., 2015), S. latissima (Pearson et al., 2019). Transcriptomic differences between sexes in Phaeophyceae have been seldomly explored, except for reproductive tissues in Fucus vesiculosus (Martins et al., 2013), S. japonica gametophytes (Bi and Zhou, 2014) Ectocarpus sp. gametophytes and gametes (Lipinska et al., 2013, 2015) and in S. latissima gametophytes in response to gametogenesis induction and light quality changes (Pearson et al., 2019). Furthermore, so far, the influence of temperature has not been addressed. In the present study we provide the first data on transcriptomic responses of male and female gametophytes of the sugar kelp, S. latissima, to temperature. S. latissima is a relevant foundation species in temperate to Arctic coasts (Steneck et al., 2002; Teagle et al., 2017) and it is the selected species in several aquaculture facilities in Europe and North America (Forbord et al., 2012; Kim et al., 2015; Broch et al., 2018).
Our experiment targeted gametophytes of S. latissima originating from Helgoland (German Bight, North Sea) representing its center of distribution in respect to latitude (Araújo et al., 2016). Despite this, summer temperatures observed at this site are high (up to 20°C) (Breitbach et al., 2016) and close to the upper thermal tolerance limit of the species (Bolton and Lüning, 1982). Moreover, a decline in S. latissima relative presence and abundance has been described in Helgoland and were paralleled by increases in temperature and water clarity (Pehlke and Bartsch, 2008).
To our knowledge, this is the first study targeting brown algae gametophyte responses to temperature at the gene expression level. Hence, the present dataset will largely contribute to the understanding of the genomic basis of sex-specific differences in response to the environment of ecosystem engineering brown algae.
Materials and Methods
Algal Material and Experimental Design
Gametophytes from uniparental stock cultures of Saccharina latissima [formerly Laminaria saccharina L. (Lamour)] (Lane et al., 2006) [AWI culture number 3094 (males) and 3096 (females)] from Helgoland, Germany were cultivated in glass flasks under red light (16hL:8hD) at 12°C (± 1°C) in an environmentally controlled room. Sterile seawater enriched with an adapted Provasoli medium without the addition of iron was replaced weekly. These conditions prevented the onset of gametogenesis (Lüning and Dring, 1975; Motomura and Sakai, 1981; Lewis et al., 2013), which was checked under the microscope regularly. To eliminate the confounding factor of red irradiance in our transcriptomic analysis, red light was replaced by white light with a photon fluence rate of 10–15 μmol photons m–2 s–1 (16hL:8hD) provided by fluorescent lamps OSRAM L 18W for 1 week prior to and during the experiment. These conditions retained our gametophytes’ cultures at a vegetative stage. At the start of the experiment 3.10 ± 0.10 g of fresh weight of female gametophyte clumps and 2.20 ± 0.10g of male gametophytes clumps were each transferred separately to plastic petri dishes (diameter 9.5 cm × height 3 cm) (n = 4) and exposed to three temperatures (4, 12, and 20°C). Experimental temperatures were chosen to mirror mean minimum (4°C) and maximum temperatures (20°C) at Helgoland (Breitbach et al., 2016) and a control temperature (12°C) that falls within the optimal growth range for the species (Bolton and Lüning, 1982; Lee and Brinkhuis, 1988). Experiments were conducted in three environmentally controlled rooms and additionally temperatures were kept constant by circulating water baths (models Haake DC1, VWR 1136D). After 14 days of temperature exposure, gametophytes were sampled for RNA extraction. Samples were frozen in liquid nitrogen and stored at −80°C until further use. RNA extraction was performed within 3 weeks after sampling.
Maximum photosynthetic quantum yield of PS II (Fv/Fm) was measured at the start and end of the experiment (n = 4). Gametophytes were dark-adapted for 10 min prior to the measurements. Afterwards, Fv/Fm was measured with an Imaging PAM (Pulse Amplitude Fluorometer; Walz, Effeltrich, Germany).
Fresh weight (n = 4) was measured at the beginning and at the end of the experiment with a laboratory scale 40SM-200A (PRECISA, Switzerland). All data were tested for normality using the Shapiro–Wilk normality test and for homogeneity of variances using the Levene’s test. Differences in growth and Fv/Fm were tested using a two-way ANOVA with the fixed factors temperature and sex. Significant differences and interaction of means were compared with the post hoc Tukey test (HSD). All statistical analyses were carried out using SPSS software version 25 (IBM, Armonk, United States). The significance level for all analyses was set at α = 0.05.
RNA Extraction, Illumina Sequencing, and Data Processing
Total RNA extraction was conducted using the method described in Heinrich et al. (2012). Samples were first ground in liquid nitrogen. Then, samples were mixed with 1 ml extraction buffer (2% CTAB, 1 M NaCl, 100 mM Tris pH 8, 50 mM EDTA, pH 8) and 10 μL β-mercaptoethanol, followed by incubation at 45°C for 15 min. After, we added 1 mL chloroform. After mixing, samples were centrifuged for 20 min. 750 ml of supernatant were mixed with 1/3 volume of ethanol and 1 ml of chloroform. The tubes were then centrifuged again under the same settings as before. After centrifugation, RNA extraction was performed with a Qiagen Plant Mini Kit (Qiagen, Germany) following the manufacturer’s protocol. RNA quality was analyzed by the NanoDrop ND-1000 UV-Vis Spectrophotometer and Agilent 2100 Bioanalyzer (Agilent Technologies, Germany). Three biological replicates per treatment were sequenced. cDNA libraries were prepared with an Illumina TruSeq RNA Library Prep Kit according to the manufacturer protocol. The libraries were sequenced on an Illumina Hiseq 2500 and 75 bp paired reads were clipped using default values of the Illumina software. Raw reads were quality controlled by FastQC1 and quality filtered using Trimmomatic v. 0.36 (Bolger et al., 2014). Quality filtering was performed using the following parameters: leading 3, trailing 3, sliding window 4:15, minlen 30.
Reads from all treatments (three temperatures × two sexes) were assembled de novo with the RNAseq software Trinity v 2.4.0 with default parameters including the reads normalization step corresponding to the Trinity implementation of the diginorm method (Grabherr et al., 2011). The quality of the transcriptome assemblies was evaluated by using BUSCO v3.0.2 (Waterhouse et al., 2017) applying an eukaryote dataset (OrthoDB v9.1). Gametophytes reads were pseudo-aligned with Salmon (Patro et al., 2017) against the de novo assembled transcriptome, the previously assembled sporophyte transcriptome (Li et al., 2019, Array express:E-MTAB-7348), the available S. japonica genome (Ye et al., 2015) and previously assembled transcriptomes of S. latissima (Heinrich et al., 2012; Jackson et al., 2017). A principal component analysis (PCA) plot of the counts-per-million, followed by a log2 transformation, of all treatments was generated by a Trinity script. Differential expression was calculated using DESeq2 (Love et al., 2014) at Trinity’s gene level with an adjusted level of p ≤ 0.001 and a log2 fold change of at least two indicating significance. Tools were executed using the scripts included in the Trinity package v 2.4.0 (Grabherr et al., 2011). Functional annotation was performed using the Trinotate functional annotation pipeline (Bryant et al., 2017) with the UniRef90 database as additional reference (all databases up to date in October 2017). In addition, annotation of transcripts via DIAMOND v0.9.13 similarity search (Buchfink et al., 2015), using “more-sensitive” option against the NR database (October 2017) was performed. Effects of temperature, sex and the interaction of temperature and sex were calculated using the multivariate analysis of variance function “adonis” of the package “vegan” (Dixon, 2003) in R studio version 1.1.442.
To investigate the function of significantly up- and down-regulated genes, Gene Ontology (GO) enrichments were conducted using GOseq (over-represented p-value < 0.05, Young et al., 2010). Results were summarized with CateGOrizer by applying GO slim (Hu et al., 2008). Venn diagrams were produced applying a webtool2.
Results
Photosynthetic Efficiency and Growth
To estimate overall fitness of the gametophytes, we assessed maximum photosynthetic quantum yield by measuring Fv/Fm and fresh weight at the beginning and end of the experiment. There was no significant effect of temperature and sex on fresh weight of gametophytes, measured as percentage of initial at the end of the 14 days exposure (temperature, p = 0.159, sex, p = 0.525). In all treatments a modest increase in fresh weight up to a maximum of 20% in females at 12°C was observed. No significant treatment changes were detected for Fv/Fm (data not shown).
De novo Transcriptome
To explore patterns of gene expression, we extracted RNA obtained from male and female gametophyte samples exposed to three different temperatures (4, 12, and 20°C). The number of reads per complementary DNA (cDNA) library ranged from 20 to 42 million with an average of 33 million reads. The remapping rate of gametophytes cDNA libraries on the de novo assembled transcriptome was on average 86% which is 5–18% better than on other available transcriptomes assemblies (data not shown). The transcriptome assembly consisted of a total of 256,118 transcripts (corresponding to 211,947 Trinity genes) with an average contig size of 713 bp and N50 of 1350 bp3 (Supplementary Data Sheet 1 Table S1). This high total number of genes is a common feature of the Trinity pipeline (e.g., Bryant et al., 2017). Ninety per cent of total expression was present in 58,741 of transcripts with a N50 of 1895 bp. Results of BUSCO analysis indicate that the assembled transcriptome has near-complete gene sequence information for 93% of the genes, 34% complete and single-copy BUSCOs, 59% completed and duplicated BUSCOs, 5% fragmented BUSCOs and 1% missing BUSCOs. 36,379 transcripts were functionally annotated using the UniProt Swiss-Prot database, 33,606 using Pfam and 60,961 with Uniref90 and 45,240 using NR. A total of 14,240 GO terms and 17,562 KEGG orthology (KO) were assigned to the transcripts (see text footnote 3; Supplementary Data Sheet 1 Table S1).
Sex-Biased Gene Expression at the Control Temperature
Several analyses performed in our dataset revealed distinct transcriptomic profiles between male and female gametophytes of S. latissima. Gender explained 34% of the variability in the transcriptome as shown in the PCA (Figure 1). The second axis of the PCA accounted for 16% of the variability and divided between the three temperatures tested. Gene expression profiles were significantly different between sexes (pPERMANOVA = 0.001) and between temperatures (pPERMANOVA = 0.009). Many genes were differentially expressed between males and females at the three temperatures (23,698 – 11.2%; Figure 2). Differential gene expression between sexes at the control temperature (12°C – 13,991 DEGs) was higher than at a temperature of 20°C (10,590) and at 4°C (9,411; Figure 2). Differential expression between sexes was more similar between 4 and 12°C than between 12 and 20°C or 4 and 20°C (Figure 3). Across temperatures, a female-biased gene expression was observed, with more up-regulated DEGs in females compared to males. Among DEGs up-regulated in females, 2,072 (12%) were up-regulated at the three temperatures, 4,937 (29%) were uniquely up-regulated at 20°C, 4,798 (28%) at 12°C and 1,496 (9%) at 4°C. In turn, among DEGs up-regulated in males, only 500 (7%) were consistent across temperatures, 1,930 (29%) were specifically regulated at 4°C, 1,873 (28%) at 12°C and 1,930 (29%) at 20°C (Figure 3). Consistently, several GO terms were significantly enriched in the comparison between gender and patterns shifted with temperature (Figures 4, 5). The lower temperature, 4°C drove more metabolic reorganization in males than females and the opposite held true at 12 and 20°C for females. Taken together, these results reveal a clear sex-biased gene expression that was modulated by temperature.
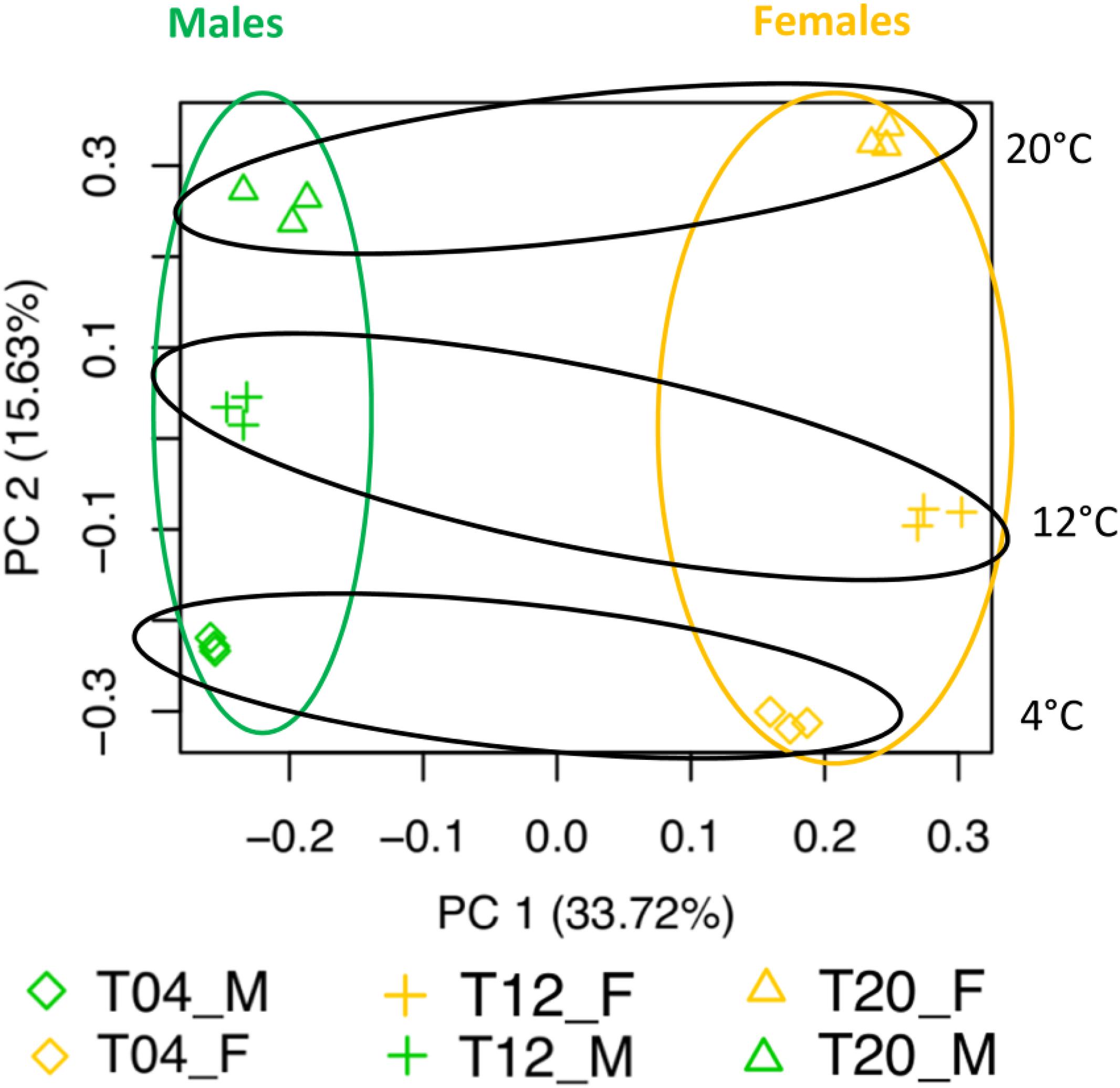
Figure 1. Principal component analysis of the counts per million in the treatments (n = 3) after 14 days in the experimental temperature treatment. Orange: female samples, green: male samples.
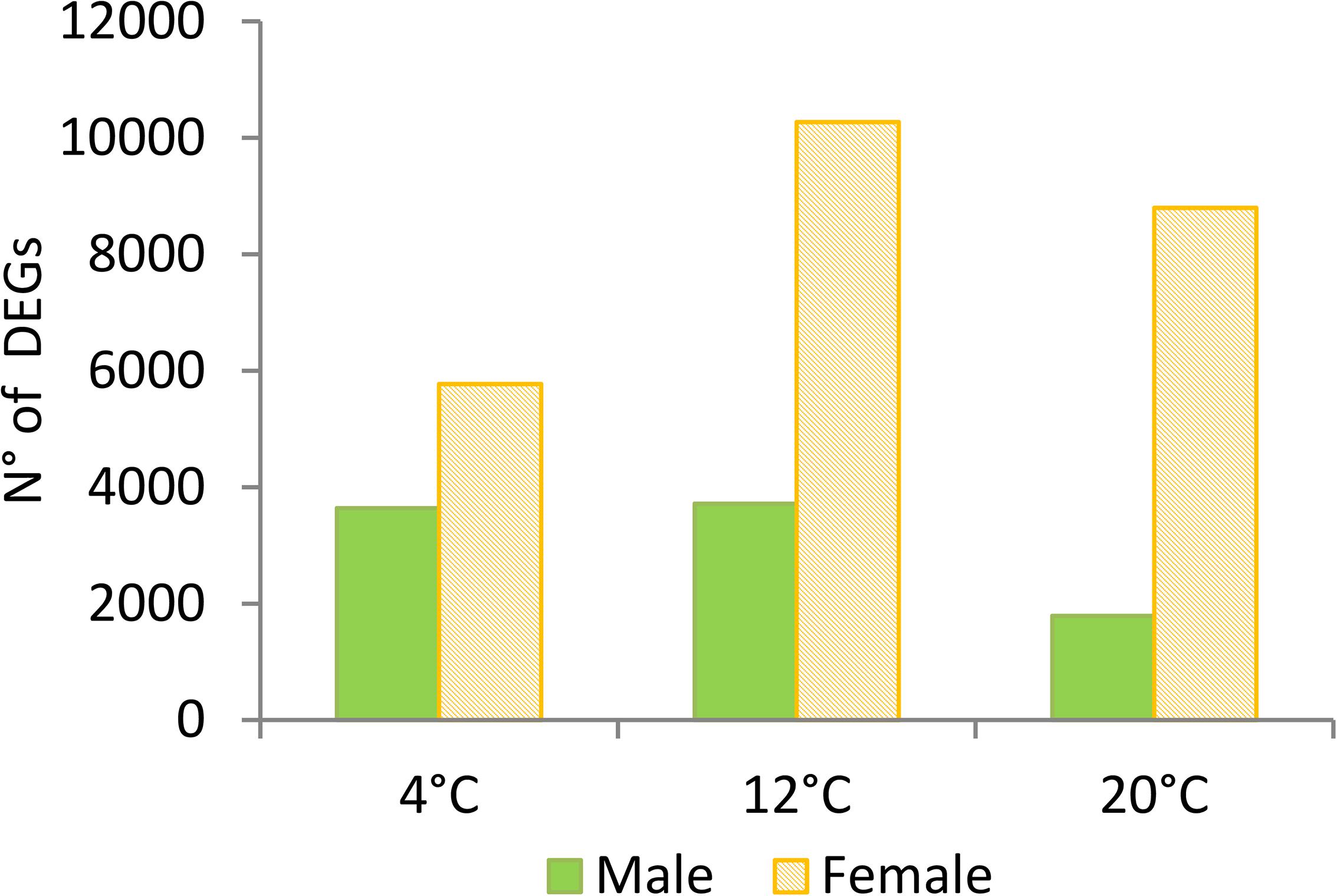
Figure 2. Total number of significant differentially expressed genes (DEGs) (Deseq2, p < 0.001; log2FC > 2, n = 3) in Saccharina latissima gametophytes. Up-regulated DEGs in male compared to female gametophytes are indicated in green, and orange in diagonal stripes bars, respectively.
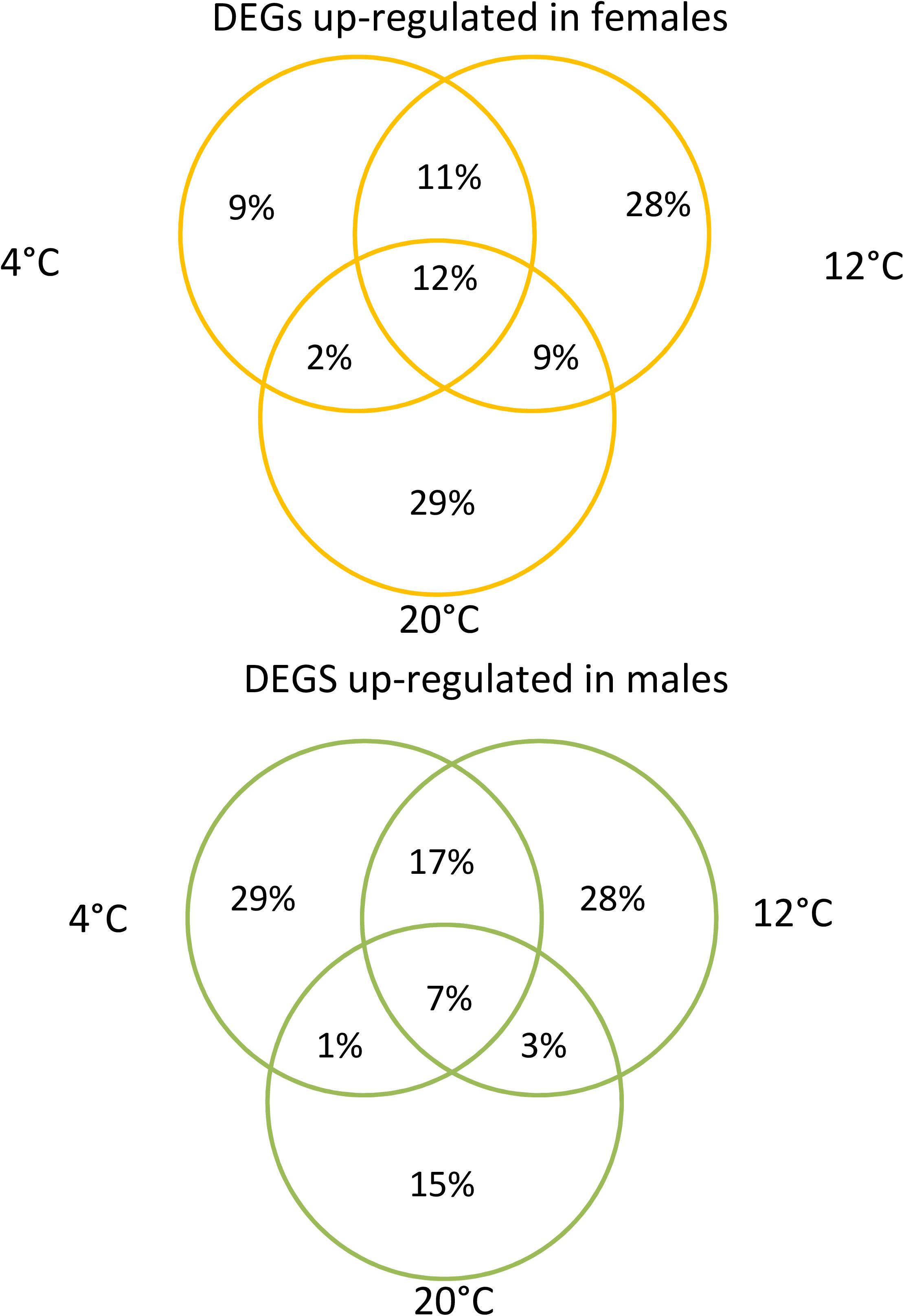
Figure 3. Venn diagrams of DEGs between sexes of Saccharina latissima by temperature (Deseq2, p < 0.001; log2FC > 2, n = 3). Up and in orange: DEGs up-regulated in females, Down and green: DEGs up-regulated in males by temperature.
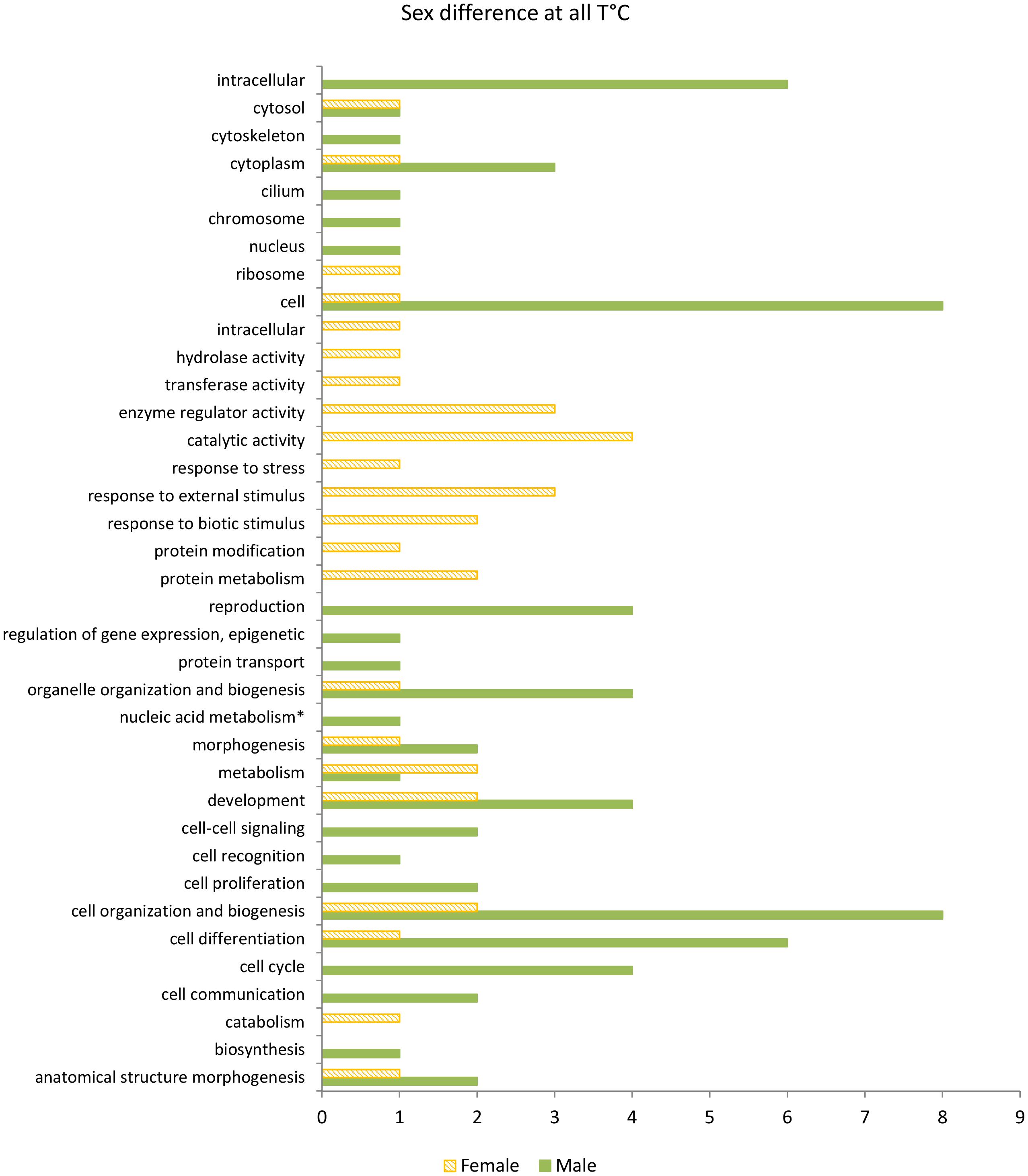
Figure 4. Over-represented GO terms of DEGs consistently up-regulated in female (orange in diagonal stripes) and male (green) gametophytes of Saccharina latissima across all temperatures, according to the categories of GO slim (GOseq, p < 0.05, n = 3). Nucleic acid metabolism∗ stands for nucleobase, nucleoside, nucleotide, and nucleic acid metabolism.
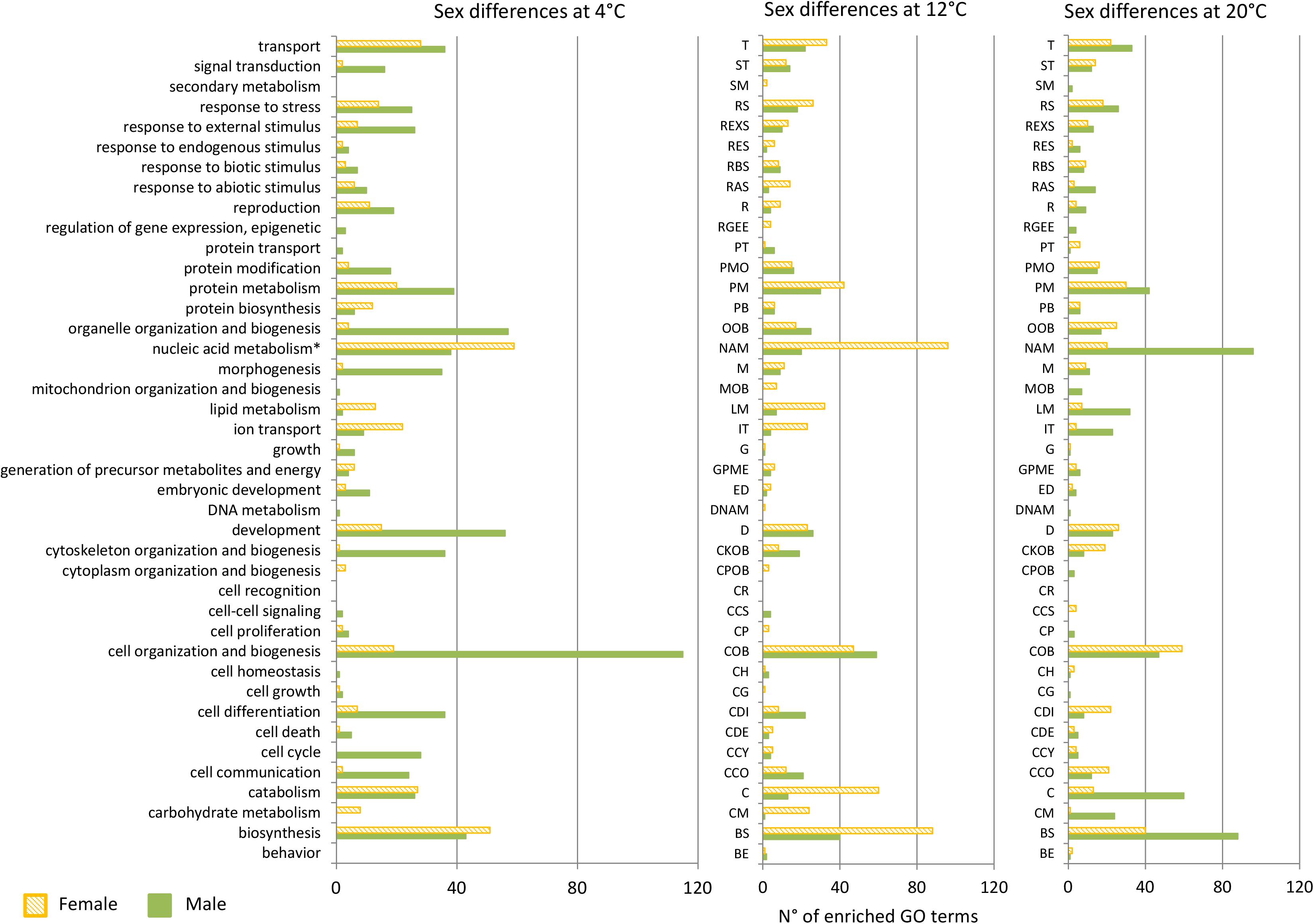
Figure 5. Over-represented GO terms belonging to the ontology Biological Process in male (green) and over-represented in female (orange in diagonal stripes) gametophytes of Saccharina latissima at 4, 12, and 20°C according to the categories of GO slim (GOseq, p < 0.05, n = 3). Nucleic acid metabolism∗ stands for nucleobase, nucleoside, nucleotide, and nucleic acid metabolism. Total for the category metabolism at 4°C – Females: 170, Males: 153, at 12°C: Females: 354, Males: 120, at 20°C: Females: 55, Males: 17.
Putative Sex Marker Genes
Analysis of the genes with the highest contribution to the Principal Component axis 1, measured by its loading values (Legendre and Legendre, 2012), revealed genes potentially related to sex determination in S. latissima (Supplementary Data Sheet 1 Table S2). Namely, one gene highly expressed in females and another one highly expressed in males both match one gene coding for homoaconitate hydratase that has been described as female-specific in Ectocarpus sp. and S. japonica (Lipinska et al., 2017). Also, a gene identified in six other brown algal species as male-specific, the high mobility group protein 1 (Lipinska et al., 2017), was also male-specific in our data. Moreover, we identified two protein kinases: dual specificity protein kinase TTK and serine/threonine-protein kinase OSR1 associated with male gene expression. Two other genes functioning in protein modification, ubiquitin-conjugating enzyme E2 D2 and galactose-3-O-sulfotransferase 3 were associated with male samples. A gene coding for serine protease/ABC transporter B family protein tagC and a gene coding for calcium channel YVC1 were associated with females.
Functional Annotation of Sex-Biased Genes
To identify the functions of sex-biased genes, we performed a GO enrichment analysis in the set of genes consistently up-regulated in females (2,072) and males (500) across temperatures (Figure 4) and on the full set of genes differentially expressed between sexes at 4, 12, and 20°C (Figure 5). Sex-biased genes identified in this study were associated with several metabolic processes. Results of the GO enrichment analysis revealed that at 12 and 4°C the top 15 overrepresented GO terms were related to general metabolism, such as “peptide biosynthetic process,” “organonitrogen compound biosynthetic process,” and “translation” for both females and males up-regulated DEGs (Supplementary Data Sheet 2 Table S3). Terms related to cytoskeleton and signaling, e.g., “cellular component assembly,” “actin filament organization,” “small GTPase mediated signal transduction” were uniquely overrepresented in males while GO terms related to aminoacid metabolism (“carboxylic acid metabolic process”) and nucleic acid metabolism (“nucleoside triphosphate metabolic process”) were uniquely overrepresented in females at both 4 and 12°C. Considering the extensive list of enriched GO terms and that it contained several human-related biological processes, we applied GO slim to provide an overview. Among GO slim functional categories exclusively over-represented in females consistently across temperatures, we identified GO terms related to metabolism and responses to cues. In the category metabolism, the GO terms “protein metabolism” and “catabolism” were over-represented, while response to cues included the GO terms “response to stress,” “response to external stimulus,” and “response to biotic stimulus” (Figure 4). Accordingly, we identified considerably more stress response related DEGs up-regulated in females at the three different temperatures, namely heat-shock and antioxidant proteins (Supplementary Data Sheet 1 Table S4). In turn, functional categories solely over-represented in males included “cell-cell signaling,” “cell cycle,” and “cell proliferation.” Moreover, some categories were over-represented in both males and females and could be connected to processes that we expect to take place in both sexes during gametophyte growth and development, namely “cell differentiation” and “cell organization and biogenesis.”
Sex-Biased Gene Expression at Low and High Temperatures
Shifts in the expressed metabolic pathways were observed in response to changes in temperature. The low temperature tested, 4°C, drove more metabolic reorganization in males than in females (Figure 5). “Morphogenesis,” “reproduction,” “transport,” and responses to endogenous, biotic and abiotic stimulus gathered more enriched GO terms in males than in females at 4°C (Figure 5), and the reverse was observed at 12°C. However, biological processes such as lipid and carbohydrate metabolism and “ion transport” were proportionally higher in females at 4°C and at 12°C than males. Concomitantly, “cell differentiation,” cell and organelle organization and biogenesis were consistently over-represented in males compared to females at both 4 and 12°C.
At 20°C, we observed a decrease in the differentiation between sexes, revealed by the lower number of enriched GO terms within the root biological processes differentially expressed between males and females. Almost all the functional categories were over-represented in females: “transport,” “signal transduction,” “response to stress,” “protein metabolism,” “cell organization and biogenesis,” and “cell communication” gathered the higher number of GO terms. The top 15 over-represented GO terms in females included “superoxide anion generation,” “positive regulation of oxidative stress-induced intrinsic apoptotic signaling pathway,” “transposition,” “DNA integration” (Supplementary Data Sheet 2 Table S3).
Discussion
Sex-Biased Gene Expression Underlies Gender-Specific Metabolic Needs
In this study, we identified a high number of genes differentially expressed between male and female gametophytes which were in the vegetative stage. This pattern is consistent with descriptions for Ectocarpus sp. by Lipinska et al. (2015) who reported that differential gene expression between males and females was higher during the immature stage than during the fertile one. Furthermore, more female- than male-biased genes were identified, which agrees with results obtained during gametogenesis in S. latissima (Pearson et al., 2019). However, this is in contrast to the situation in two other brown algae, F. vesiculosus (order Fucales) (Martins et al., 2013), and Ectocarpus sp. (order Ectocarpales) (Lipinska et al., 2015). Although there are contrasting patterns in sex-biased gene expression within brown algae, the three species still belong to different orders and even high variability in sex-biased gene expression among species of the same genus has been described before (Metta et al., 2006). Moreover, such differences may mirror the different life cycles (Coelho et al., 2019). While Laminariales and Ectocarpales have a haplo-diplontic life cycle, Fucales display a diplontic life cycle. Moreover, the degree of sexual dimorphism in the haplo-phase (gametophytes) of Laminariales is stronger than in the order Ectocarpales and while the first produce anisogamous gametes with a large non-motile female egg cell and small motile male sperms, Ectocarpales gametes are both motile and isogamous (Luthringer et al., 2014).
Functional analysis of the differentially expressed genes (DEGs) revealed that several metabolic pathways are distinctly regulated between female and male gametophytes. In females, several GO terms related to general metabolism are enriched, such as “carbohydrate metabolism,” “lipid metabolism,” “nucleobase, nucleoside, nucleotide and acid metabolism.” In addition, the over-represented GO term “generation of precursor metabolites and energy” suggest that female gametophyte cells are actively investing in cell growth and energy production. This is consistent with observational microscopy studies, as female gametophyte cells tend to grow in size while male gametophyte cells tend to grow in number (Lüning, 1980; Destombe and Oppliger, 2011; Park et al., 2017). This investment in cell growth might be advantageous for later sporophyte growth as larger female gametophyte cells will release bigger eggs that tend to generate larger sporophytes (Bell, 1997). Within lipid metabolism, we identified four genes coding for lipoxygenase that were sex-specific in S. latissima in this study (Supplementary Data Sheet 1 Table S5) and during gametogenesis (Pearson et al., 2019), as in Ectocarpus sp. (Lipinska et al., 2015) although female-specific in the first and male-specific in the latter species. Lipoxygenases are involved in lipid oxidation and play an important role in the biosynthesis of oxylipins – lipophilic mediators that have several physiological functions such as senescence, growth and development, tolerance to stress, and cell homeostasis (Hou et al., 2015; Maynard et al., 2018). In brown algae, they are involved in the defense response to pathogens and grazing (Cosse et al., 2009; Ritter et al., 2017) and their synthesis was induced under copper stress (Ritter et al., 2008). Considering the sex-biased gene expression of lipoxygenases in both Ectocarpus sp. and S. latissima we hypothesize that they are involved in regulating sexual differentiation in brown algae. Further work is required to understand the specific function of lipoxygenases in sexual differentiation and its different expression between brown algae species. In addition, two genes coding for mannuronan C5-epimerase were female-specific and one was male-specific in S. latissima, while in Ectocarpus sp. two genes were male and one was female-specific. Mannuronan C5-epimerases belong to large multigenic families that are involved in the synthesis of alginate, a major component of cell walls in brown algae (Michel et al., 2010). Previously, Fischl et al. (2016) reported four mannuronan C5-epimerases genes preferentially expressed in Ectocarpales gametophytes over sporophytes, suggesting a role in the gametophyte development. Differential expression of some of the genes encoding this enzyme might contribute to the different morphologies of the male and female gametophytes’ cells.
A closer inspection of the functional annotation of DEGs revealed that some genes involved in oxidative stress responses were up-regulated in female gametophytes of S. latissima. Similar patterns were also reported for S. latissima during gametogenesis (Pearson et al., 2019) and in the female gametophyte of Arabidopsis (Wuest et al., 2010). Reactive oxygen species play a role in embryo sac development and fertilization in Arabidopsis (Martín et al., 2013), cell division (Livanos et al., 2012a) and cytoskeleton homeostasis (Livanos et al., 2012b) in plants. Thus, we hypothesize that reactive oxygen species might function as signaling molecules regulating gametophyte development, as previously reported in gender-biased gene expression in primates and mice (Yang et al., 2006; Blekhman et al., 2010), suggesting that these are highly conserved processes.
In vegetative males, GO terms in the GO slim functional categories “cell communication” and “cell-cell signaling” were over-represented which is probably connected to the fact that gametophyte maturation is dependent on both environmental factors and biotic cues, namely the release of pheromones (Lüning and Müller, 1978). Signal transduction might be crucial to allow for temporal and spatial overlap in gametophyte maturation which is critical for successful fertilization in kelps (Schiel and Foster, 2006). During our experiments no reproductive structures were visible and males did not receive female cues for maturation as sexes were kept separated. Nevertheless, our data suggests that male gametophytes prepare in advance for maturation at the transcriptomic level probably to be able to readily synchronize gamete release with females once specific cues are received. This might confer a competitive advantage and ensure successful fertilization. Li et al. (2013) have observed that the best fertilization rates in S. japonica occur 60–120 min after male gamete release and their viability is limited to 12 h. This is further supported by the expression of a “flagellar associated” gene reported from Ectocarpus which was only expressed in males of S. latissima at 4 and 12°C. Two “flagellar associated proteins” also displayed male-biased gene expression in immature gametophytes of Ectocarpus (Lipinska et al., 2015).
Furthermore, higher enriched GO terms within the categories “cell,” “cytoskeleton,” and “organelle organization and biogenesis” are in accordance with cell division being enhanced in male gametophyte filaments and these being more highly branched than female gametophyte filaments. Among these functional categories, we found two genes encoding DNA helicases with very high expression in males. DNA helicases are evolutionary conserved among eukaryotes and involved in DNA replication, namely by positive regulation of telomere length, replication of highly transcribed RNA polymerase II genes and unwinding G–quadruplex DNA structures (Rhodes and Lipps, 2015; Sabouri, 2017). Therefore, higher expression of these genes in males might be connected to higher cell division rates.
Changes in Temperature Modulated Sex-Biased Gene Expression
Functional analysis revealed that sex-biased gene expression shifted with temperature. Differences between sexes were less pronounced at 20°C than at 4 and 12°C suggesting that the gametophytes alter their metabolism from developmental and sex differentiation processes to a heat stress protection response that involves similar pathways in both sexes. This is in agreement with 4 and 12°C being more suitable temperatures for gametophyte growth and gametogenesis than 20°C (Lüning, 1980).
It is widely accepted that temperature is a major factor controlling reproduction in seaweeds (Egan et al., 1989; Andrews et al., 2014; Hurd et al., 2014a). This is further supported in our study by the interactive effect of temperature and sex in gene expression patterns in S. latissima. Only a small proportion of DEGs were consistent across temperatures (12% for females and 7% for males) and females responded stronger to the higher temperature (20°C) while more genes were overexpressed in males at lower temperatures (4°C). The effect of temperature on growth of S. latissima gametophytes has been previously demonstrated: Lüning (1980) observed optimum vegetative growth between 10 and 19°C and gametophyte death after 1 week at 22°C. Another study revealed that gametophytes survived 22°C and even 23°C but with visible cell damage (Bolton and Lüning, 1982). Hence, the higher temperature tested in the present study (20°C) is very close to the upper survival temperature in gametophytes and to the maximum temperature reached during summer at the original collection site of the gametophytes (Helgoland, North Sea, Breitbach et al., 2016). Other studies have suggested that male kelp gametophytes are slightly more thermotolerant than females (Lee and Brinkhuis, 1988; tom Dieck, 1993). This is supported by the differential gene expression of our study. The male gametophytes seem to be more resilient to the heat stress as functional categories such as “response to stress,” response to external and abiotic stimuli and “cell death” were over-represented in females and categories such as “cell cycle” were over-represented in males. In addition, induction of transposable elements by stress and their effects on gene regulation has been observed in plants, namely in response to heat stress (Makarevitch et al., 2015; Dubin et al., 2018). The over-representation of GO terms related to transposition in females at 20°C suggests a role in heat stress response in S. latissima gametophytes. Concomitantly, other enriched GO terms connected to stress response, such as “superoxide anion generation,” “positive regulation of oxidative stress-induced intrinsic apoptotic signaling pathway” were also among the top 15 over-represented GO terms in females at 20°C. This further supports the higher sensitivity to heat stress of female gametophytes. Assessing differential sensitivity to high temperatures between sexes in gametophytes is relevant for hybridization protocols aiming to produce more tolerant cultivars in aquaculture, as it is already current practice in crop plant species (Zinn et al., 2010). Recently, Martins et al. (2019) demonstrated that for the kelps Laminaria digitata and L. pallida female parent gametophytes contributed more for the thermal response of the hybrids than males. Our work suggests that the same might be true for S. latissima and informs current ongoing efforts to maximize sugar kelp cultivation practices.
Shifts in sex ratio in response to sub-optimal temperatures have already been described in several kelp species (Izquierdo et al., 2002; Nelson, 2005; Oppliger et al., 2011). In S. latissima, Lee and Brinkhuis (1988) described a greater proportion of male compared to female gametophytes at higher temperatures (between 17 and 20°C). Female gametophyte grew at 20°C, but with a lower rate than between 4 and 17°C and their fecundity was repressed. Pronounced temperature-mediated sex ratio shifts might lead to population declines in several species, especially at sites where species are close to their upper thermal tolerance limit, and may have an impact on the range distribution of species (Janzen, 1994; Ospina-Alvarez and Piferrer, 2008; Hays Graeme et al., 2017). Differences in the sex ratio between populations along the distributional range have been observed for several plants (Barrett and Hough, 2012) and for the kelp Lessonia nigrescens (Oppliger et al., 2011), which warrants concern that skewed sex ratios due to global warming might compromise populations viability. At the gametophyte stage, global warming may lead to a mismatch between male and female gametophyte performance that might compromise sexual maturation and fertilization, as has been recently highlighted by de Bettignies et al. (2018). At the same time, the high levels of gene expression reported here indicate that vegetative gametophytes are still metabolically active rather than “dormant” as in plant seeds (Schiel and Foster, 2006) and therefore more vulnerable to abiotic stress. Therefore, the sex differences in gene expression patterns in response to temperature described here for S. latissima prompts for further investigation on the vulnerability of the gametophyte life-stage to temperature and its potential effects in reproductive success and phenology.
Author’s Note
This manuscript has been released as a Pre-Print at https://www.biorxiv.org/content/10.1101/750455v1.
Data Availability Statement
The Illumina sequence reads generated during the present study are available in the Array express repository under the accession number E-MTAB-8267 (Athar et al., 2019; Monteiro et al., 2019) (https://www.ebi.ac.uk/arrayexpress/experiments/E-MTAB-8267/). The transcriptome assembly, peptide and annotation generated in this study are available on http://application.sb-roscoff.fr/blast/slatissima/index.html.
Author Contributions
CM performed the experiment, carried out the analyses and RNA extractions, analyzed the data, and wrote the manuscript. EC assembled the de novo transcriptome and performed the bioinformatics analyses related to RNA – sequencing data with help from LH. KB, IB, SH, JC, EC, LH, and KV supervised the project and helped with the data interpretation and discussion. CM, SH, KB, IB, and KV designed the experimental set-up. IB further provided the algae cultures. GG carried out the RNA-sequencing and commented on the manuscript. All authors read and approved the manuscript.
Funding
This work was supported by the German Research Foundation for funding within the ERA-Net Cofund BiodivERsA 3 program MARFOR (ANR-16-EBI3-0005-01). Further funding was provided by the MARES Joint Doctoral Programme on Marine Ecosystem Health & Conservation funded through Erasmus Mundus (grant number MARES_14_09) and the Alfred Wegener Institute, Helmholtz Centre for Polar and Marine Research (Bremerhaven, Germany).
Conflict of Interest
The authors declare that the research was conducted in the absence of any commercial or financial relationships that could be construed as a potential conflict of interest.
Supplementary Material
The Supplementary Material for this article can be found online at: https://www.frontiersin.org/articles/10.3389/fmars.2019.00769/full#supplementary-material
TABLE S1 | Summary of transcriptome statistics. Summary of transcript annotations in the public databases.
TABLE S2 | Top 100 genes contributing to PC1 of the Principal Component analysis according to their loading values.
TABLE S3 | Top 15 over-represented GO terms by sex (male, female) and temperature (4°C, 12°C, and 20°C), both total GO terms and sex-exclusive.
TABLE S4 | List of stress response related DEGs between sexes at the three different temperatures.
TABLE S5 | List of DEGS between sexes found in common in Ectocarpus sp. and S. latissima.
Footnotes
- ^ https://www.bioinformatics.babraham.ac.uk/projects/fastqc/
- ^ http://bioinformatics.psb.ugent.be/webtools/Venn/
- ^ http://application.sb-roscoff.fr/blast/slatissima/
References
Andrews, S., Bennett, S., and Wernberg, T. (2014). Reproductive seasonality and early life temperature sensitivity reflect vulnerability of a seaweed undergoing range reduction. Mar. Ecol. Prog. Ser. 495, 119–129. doi: 10.3354/meps10567
Araújo, R., Assis, J., Aguillar, R., Airoldi, L., Bárbara, I., Bartsch, I., et al. (2016). Status, trends and drivers of kelp forests in Europe: an expert assessment. Biodivers. Conserv. 25, 1319–1348. doi: 10.1007/s10531-016-1141-7
Assis, J., Berecibar, E., Claro, B., Alberto, F., Reed, D., Raimondi, P., et al. (2017). Major shifts at the range edge of marine forests: the combined effects of climate changes and limited dispersal. Sci. Rep. 7:44348. doi: 10.1038/srep44348
Athar, A., Füllgrabe, A., George, N., Iqbal, H., Huerta, L., Ali, A., et al. (2019). ArrayExpress update – from bulk to single-cell expression data. Nucleic Acids Res. 47, D711–D715. doi: 10.1093/nar/gky964
Barradas, A., Alberto, F., Engelen, A. H., and Serrão, E. A. (2011). Fast sporophyte replacement after removal suggests banks of latent microscopic stages of Laminaria ochroleuca (Phaeophyceae) in tide pools in northern Portugal. Cahiers De Biol. Mar. 52:435.
Barrett, S. C., and Hough, J. (2012). Sexual dimorphism in flowering plants. J. Exp. Bot. 64, 67–82. doi: 10.1093/jxb/ers308
Bartsch, I. (2018). “Derivation of clonal stock cultures and hybridization of kelps,” in Protocols for Macroalgae Research, eds B. Charrier, T. Wichard, and C. Reddy (New York, NY: CRC Press), 61–78. doi: 10.1201/b21460-3
Bell, G. (1997). The evolution of the life cycle of brown seaweeds. Biol. J. Linnean Soc. 60, 21–38. doi: 10.1111/j.1095-8312.1997.tb01481.x
Bi, Y.-H., and Zhou, Z.-G. (2014). What does the difference between the female and male gametophytes of Saccharina japonica remind us of? Algol. Stud. 145, 65–79. doi: 10.1127/1864-1318/2014/0145
Blekhman, R., Marioni, J. C., Zumbo, P., Stephens, M., and Gilad, Y. (2010). Sex-specific and lineage-specific alternative splicing in primates. Genome Res. 20, 180–189. doi: 10.1101/gr.099226.109
Bolger, A. M., Lohse, M., and Usadel, B. (2014). Trimmomatic: a flexible trimmer for Illumina sequence data. Bioinformatics 30, 2114–2120. doi: 10.1093/bioinformatics/btu170
Bolton, J. J., and Lüning, K. (1982). Optimal growth and maximal survival temperatures of Atlantic Laminaria species (Phaeophyta) in culture. Mar. Biol. 66, 89–94. doi: 10.1007/bf00397259
Breitbach, G., Krasemann, H., Behr, D., Beringer, S., Lange, U., Vo, N., et al. (2016). Accessing diverse data comprehensively–CODM, the COSYNA data portal. Ocean Sci. 12, 909–923. doi: 10.5194/os-12-909-2016
Broch, O., Alver, M., Bekkby, T., Gundersen, H., Forbord, S., Handå, A., et al. (2018). Kelp cultivation potential in Norwegian coastal and offshore regions. Front. Mar. Sci. 5:529. doi: 10.3389/fmars.2018.00529
Bryant, D. M., Johnson, K., DiTommaso, T., Tickle, T., Couger, M. B., Payzin-Dogru, D., et al. (2017). A tissue-mapped axolotl de novo transcriptome enables identification of limb regeneration factors. Cell Rep. 18, 762–776. doi: 10.1016/j.celrep.2016.12.063
Buchfink, B., Xie, C., and Huson, D. H. (2015). Fast and sensitive protein alignment using DIAMOND. Nat. Methods 12:59. doi: 10.1038/nmeth.3176
Charrier, B., Abreu, M. H., Araujo, R., Bruhn, A., Coates, J. C., De Clerck, O., et al. (2017). Furthering knowledge of seaweed growth and development to facilitate sustainable aquaculture. N. Phytol. 216, 967–975. doi: 10.1111/nph.14728
Coelho, S. M., Mignerot, L., and Cock, J. M. (2019). Origin and evolution of sex-determination systems in the brown algae. N. Phytol. 222, 1751–1756. doi: 10.1111/nph.15694
Coelho, S. M., Rijstenbil, J. W., and Brown, M. T. (2000). Impacts of anthropogenic stresses on the early development stages of seaweeds. J. Aqua. Ecosyst. Stress Recov. 7, 317–333. doi: 10.1023/a:1009916129009
Cosse, A., Potin, P., and Leblanc, C. (2009). Patterns of gene expression induced by oligoguluronates reveal conserved and environment-specific molecular defense responses in the brown alga Laminaria digitata. N. Phytol. 182, 239–250. doi: 10.1111/j.1469-8137.2008.02745.x
de Bettignies, T., Wernberg, T., and Gurgel, C. F. D. (2018). Exploring the influence of temperature on the reproductive phenology aspects of temperate seaweeds. Front. Mar. Sci. 5:218. doi: 10.3389/fmars.2018.00218
Destombe, C., and Oppliger, L. V. (2011). Male gametophyte fragmentation in Laminaria digitata: a life history strategy to enhance reproductive success. Cahiers Biol. Mar. 52:385.
Dixon, P. (2003). VEGAN, a package of R functions for community ecology. J. Veg. Sci. 14, 927–930. doi: 10.1111/j.1654-1103.2003.tb02228.x
Dubin, M. J., Mittelsten Scheid, O., and Becker, C. (2018). Transposons: a blessing curse. Curr. Opin. Plant Biol. 42, 23–29. doi: 10.1016/j.pbi.2018.01.003
Edwards, M. S. (2000). The role of alternate life-history stages of a marine macroalga: a seed bank analogue? Ecology 81, 2404–2415. doi: 10.1890/0012-96582000081
Egan, B., Vlasto, A., and Yarish, C. (1989). Seasonal acclimation to temperature and light in Laminaria longicruris de la Pyl.(Phaeophyta). J. Exp. Mar. Biol. Ecol. 129, 1–16. doi: 10.1016/0022-0981(89)90059-2
Ellegren, H., and Parsch, J. (2007). The evolution of sex-biased genes and sex-biased gene expression. Nat. Rev. Gene. 8:689. doi: 10.1038/nrg2167
Fischl, R., Bertelsen, K., Gaillard, F., Coelho, S., Michel, G., Klinger, M., et al. (2016). The cell-wall active mannuronan C5-epimerases in the model brown alga Ectocarpus: from gene context to recombinant protein. Glycobiology 26, 973–983. doi: 10.1093/glycob/cww040
Forbord, S., Skjermo, J., Arff, J., Handå, A., Reitan, K. I., Bjerregaard, R., et al. (2012). Development of Saccharina latissima (Phaeophyceae) kelp hatcheries with year-round production of zoospores and juvenile sporophytes on culture ropes for kelp aquaculture. J. Appl. Phycol. 24, 393–399. doi: 10.1007/s10811-011-9784-y
Fredersdorf, J., Müller, R., Becker, S., Wiencke, C., and Bischof, K. (2009). Interactive effects of radiation, temperature and salinity on different life history stages of the Arctic kelp Alaria esculenta (Phaeophyceae). Oecologia 160, 483–492. doi: 10.1007/s00442-009-1326-9
Gao, X., Kim, J.-H., Park, S. K., Yu, O. H., Kim, Y. S., and Choi, H. G. (2019). Diverse responses of sporophytic photochemical efficiency and gametophytic growth for two edible kelps, Saccharina japonica and Undaria pinnatifida, to ocean acidification and warming. Mar. Poll. Bull. 142, 315–320. doi: 10.1016/j.marpolbul.2019.03.063
Grabherr, M. G., Haas, B. J., Yassour, M., Levin, J. Z., Thompson, D. A., Amit, I., et al. (2011). Full-length transcriptome assembly from RNA-Seq data without a reference genome. Nat. Biotechnol. 29:644. doi: 10.1038/nbt.1883
Hays Graeme, C., Mazaris Antonios, D., Schofield, G., and Laloë, J.-O. (2017). Population viability at extreme sex-ratio skews produced by temperature-dependent sex determination. Proc. R. Soc. B 284:20162576. doi: 10.1098/rspb.2016.2576
Heinrich, S., Frickenhaus, S., Glöckner, G., and Valentin, K. (2012). A comprehensive cDNA library of light- and temperature-stressed Saccharina latissima (Phaeophyceae). Eur. J. Phycol. 47, 83–94. doi: 10.1080/09670262.2012.660639
Hou, Y., Meng, K., Han, Y., Ban, Q., Wang, B., Suo, J., et al. (2015). The persimmon 9-lipoxygenase gene DkLOX3 plays positive roles in both promoting senescence and enhancing tolerance to abiotic stress. Front. Plant Sci. 6:1073. doi: 10.3389/fpls.2015.01073
Hsiao, S. I., and Druehl, L. D. (1973). Environmental control of gametogenesis in Laminaria saccharina IV. in situ development of gametophytes and young sporophytes. J. Phycol. 9, 160–165. doi: 10.1111/j.0022-3646.1973.00160.x
Hu, Z.-L., Bao, J., and Reecy, J. M. (2008). CateGOrizer: a web-based program to batch analyze gene ontology classification categories. Online J. Bioinformatics 9, 108–112.
Hurd, C. L., Harrison, P. J., Bischof, K., and Lobban, C. S. (2014a). Life Histories, Reproduction, and Morphogenesis in Seaweed Ecology and Physiology. Cambridge: Cambridge University Press.
Hurd, C. L., Harrison, P. J., Bischof, K., and Lobban, C. S. (2014b). Seaweed Thali and Cells in Seaweed Ecology and Physiology. Cambridge: Cambridge University Press.
Izquierdo, J., Pérez-Ruzafa, I. M., and Gallardo, T. (2002). Effect of temperature and photon fluence rate on gametophytes and young sporophytes of Laminaria ochroleuca Pylaie. Helgoland Mar. Res. 55, 285–292. doi: 10.1007/s10152-001-0087-6
Jackson, C., Salomaki, E. D., Lane, C. E., and Saunders, G. W. (2017). Kelp transcriptomes provide robust support for interfamilial relationships and revision of the little known Arthrothamnaceae (Laminariales). J. Phycol. 53, 1–6. doi: 10.1111/jpy.12465
Janzen, F. J. (1994). Climate change and temperature-dependent sex determination in reptiles. Proc. Natl. Acad. Sci.U.S.A. 91, 7487–7490. doi: 10.1073/pnas.91.16.7487
Kim, J. K., Kraemer, G. P., and Yarish, C. (2015). Use of sugar kelp aquaculture in long island sound and the Bronx River Estuary for nutrient extraction. Mar. Ecol. Prog. Ser. 531, 155–166. doi: 10.3354/meps11331
Lane, C. E., Mayes, C., Druehl, L. D., and Saunders, G. W. (2006). A multi-gene molecular investigation of the Kelp (Laminariales, Phaeophyceae) supports substantial taxonomic reorganization. J. Phycol. 42, 493–512. doi: 10.1111/j.1529-8817.2006.00204.x
Leal, P. P., Hurd, C. L., Sander, S. G., Armstrong, E., Fernández, P. A., Suhrhoff, T. J., et al. (2018). Copper pollution exacerbates the effects of ocean acidification and warming on kelp microscopic early life stages. Sci. Rep. 8:14763. doi: 10.1038/s41598-018-32899-w
Lee, J. A., and Brinkhuis, B. H. (1986). Reproductive phenology of Laminaria saccharina (L.) Lamour. (Phaeophyta) at the southern limit of its distribution in the northwestern Atlantic Ocean. J. Phycol. 22, 276–285. doi: 10.1111/j.1529-8817.1986.tb00024.x
Lee, J. A., and Brinkhuis, B. H. (1988). Seasonal light and temperature interaction effects on development of Laminaria saccharina (Phaeophyta) gametophytes and juvenile sporophytes. J. Phycol. 24, 181–191. doi: 10.1111/j.1529-8817.1988.tb00076.x
Lewis, R. J., Green, M. K., and Afzal, M. E. (2013). Effects of chelated iron on oogenesis and vegetative growth of kelp gametophytes (Phaeophyceae). Phycol. Res. 61, 46–51. doi: 10.1111/j.1440-1835.2012.00667.x
Li, H., Monteiro, C., Heinrich, S., Bartsch, I., Valentin, K., Harms, L., et al. (2019). Responses of the kelp Saccharina latissima (Phaeophyceae) to the warming Arctic: from physiology to transcriptomics. Physiol. Plant. doi: 10.1111/ppl.13009 [Epub ahead of print].
Li, J., Pang, S., Liu, F., Shan, T., and Gao, S. (2013). Spermatozoid life-span of two brown seaweeds, Saccharina japonica and Undaria pinnatifida, as measured by fertilization efficiency. Chin. J. Oceanol. Limnol. 31, 774–781. doi: 10.1007/s00343-013-2207-y
Lipinska, A., Cormier, A., Cock, J. M., Luthringer, R., Coelho, S. M., Peters, A. F., et al. (2015). Sexual dimorphism and the evolution of sex-biased gene expression in the brown alga Ectocarpus. Mol. Biol. Evol. 32, 1581–1597. doi: 10.1093/molbev/msv049
Lipinska, A. P., D’hondt, S., Van Damme, E. J., and De Clerck, O. (2013). Uncovering the genetic basis for early isogamete differentiation: a case study of Ectocarpus siliculosus. BMC Geno. 14:909. doi: 10.1186/1471-2164-14-909
Lipinska, A. P., Toda, N. R., Heesch, S., Peters, A. F., Cock, J. M., and Coelho, S. M. (2017). Multiple gene movements into and out of haploid sex chromosomes. Geno. Biol. 18;104. doi: 10.1186/s13059-017-1201-7
Livanos, P., Apostolakos, P., and Galatis, B. (2012a). Plant cell division. Plant Signal. Behav. 7, 771–778. doi: 10.4161/psb.20530
Livanos, P., Galatis, B., Quader, H., and Apostolakos, P. (2012b). Disturbance of reactive oxygen species homeostasis induces atypical tubulin polymer formation and affects mitosis in root-tip cells of Triticum turgidum and Arabidopsis thaliana. Cytoskeleton 69, 1–21. doi: 10.1002/cm.20538
Love, M. I., Huber, W., and Anders, S. (2014). Moderated estimation of fold change and dispersion for RNA-seq data with DESeq2. Geno. Biol. 15:550. doi: 10.1186/s13059-014-0550-8
Lüning, K. (1980). Critical levels of light and temperature regulating the gametogenesis of three Laminaria species (Phaeophyceae). J. Phycol. 16, 1–15. doi: 10.1111/j.1529-8817.1980.tb02992.x
Lüning, K., and Dring, M. J. (1975). Reproduction, growth and photosynthesis of gametophytes of Laminaria saccharina grown in blue and red light. Mar. Biol. 29, 195–200. doi: 10.1007/bf00391846
Lüning, K., and Müller, D. G. (1978). Chemical interaction in sexual reproduction of several Laminariales (Phaeophyceae): release and attraction of spermatozoids. Zeitschrift Fuer Pflanzenphysiologie 89, 333–341. doi: 10.1016/s0044-328x(78)80006-3
Lüning, K., and Neushul, M. (1978). Light and temperature demands for growth and reproduction of laminarian gametophytes in southern and central California. Mar. Biol. 45, 297–309. doi: 10.1007/bf00391816
Luthringer, R., Cormier, A., Ahmed, S., Peters, A., Cock, J., and Coelho, S. (2014). Sexual dimorphism in the brown algae. Perspect. Phycol. 1, 11–25. doi: 10.1127/2198-011x/2014/0002
Makarevitch, I., Waters, A. J., West, P. T., Stitzer, M., Hirsch, C. N., Ross-Ibarra, J., et al. (2015). Transposable elements contribute to activation of maize genes in response to abiotic stress. PLoS Gene. 11:e1004915. doi: 10.1371/journal.pgen.1004915
Martín, M. V., Distefano, A. M., Zabaleta, E. J., and Pagnussat, G. C. (2013). New insights into the functional roles of reactive oxygen species during embryo sac development and fertilization in Arabidopsis thaliana. Plant Signal. Behav. 8:e25714. doi: 10.4161/psb.25714
Martins, M. J. F., Mota, C. F., and Pearson, G. A. (2013). Sex-biased gene expression in the brown alga Fucus vesiculosus. BMC Geno. 14:294. doi: 10.1186/1471-2164-14-294
Martins, N., Pearson, G. A., Gouveia, L., Tavares, A. I., Serrão, E. A., and Bartsch, I. (2019). Hybrid vigour for thermal tolerance in hybrids between the allopatric kelps Laminaria digitata and L. pallida (Laminariales, Phaeophyceae) with contrasting thermal affinities. Eur. J. Phycol. 54, 548–561. doi: 10.1080/09670262.2019.1613571
Martins, N., Tanttu, H., Pearson, G. A., Serrão, E. A., and Bartsch, I. (2017). Interactions of daylength, temperature and nutrients affect thresholds for life stage transitions in the kelp Laminaria digitata (Phaeophyceae). Bot. Mar. 60, 109–121.
Maynard, D., Gröger, H., Dierks, T., and Dietz, K.-J. (2018). The function of the oxylipin 12-oxophytodienoic acid in cell signaling, stress acclimation, and development. J. Exp. Bot. 69, 5341–5354. doi: 10.1093/jxb/ery316
Metta, M., Gudavalli, R., Gibert, J.-M., and Schlötterer, C. (2006). No accelerated rate of protein evolution in male-biased Drosophila pseudoobscura genes. Genetics 174, 411–420. doi: 10.1534/genetics.106.057414
Michel, G., Tonon, T., Scornet, D., Cock, J. M., and Kloareg, B. (2010). The cell wall polysaccharide metabolism of the brown alga Ectocarpus siliculosus. Insights into the evolution of extracellular matrix polysaccharides in Eukaryotes. N. Phytol. 188, 82–97. doi: 10.1111/j.1469-8137.2010.03374.x
Monteiro, C., Heinrich, S., Bartsch, I., Valentin, K. U., Corre, E., Collén, J., et al. (2019). Temperature dependent sex-biased gene expression in the gametophytes of the kelp Saccharina latissima. bioRxiv. [Preprint]. Available at: https://www.terkko.helsinki.fi/article/21379670_temperature-dependent-sex-biased-gene-expression-in-the-gametophytes-of-the-kelp-saccharina-latissima (accessed August 30, 2019).
Motomura, T., and Sakai, Y. (1981). Effect of chelated iron in culture media on oogenesis in Laminaria angustata. Nippon Suisan Gakkaishi 47, 1535–1540. doi: 10.2331/suisan.47.1535
Nelson, W. (2005). Life history and growth in culture of the endemic New Zealand kelp Lessonia variegata J. Agardh in response to differing regimes of temperature, photoperiod and light. J. Appl. Phycol. 17, 23–28. doi: 10.1007/s10811-005-5521-8
Oppliger, L. V., Correa, J. A., Faugeron, S., Beltrán, J., Tellier, F., Valero, M., et al. (2011). Sex Ratio Variation in the Lessonia nigrescens Complex (Laminariales, Phaeophyceae): effect of Latitude, Temperature, and Marginality. J. Phycol. 47, 5–12. doi: 10.1111/j.1529-8817.2010.00930.x
Ospina-Alvarez, N., and Piferrer, F. (2008). Temperature-dependent sex determination in fish revisited: prevalence, a single sex ratio response pattern, and possible effects of climate change. PLoS One 3:e2837. doi: 10.1371/journal.pone.0002837
Park, J., Kim, J. K., Kong, J.-A., Depuydt, S., Brown, M. T., and Han, T. (2017). Implications of rising temperatures for gametophyte performance of two kelp species from Arctic waters. Bot. Mar. 60, 39–48.
Patro, R., Duggal, G., Love, M. I., Irizarry, R. A., and Kingsford, C. (2017). Salmon: fast and bias-aware quantification of transcript expression using dual-phase inference. Nat. Methods 14, 417–419. doi: 10.1038/nmeth.4197
Pearson, G. A., Martins, N., Madeira, P., Serrão, E. A., and Bartsch, I. (2019). Sex-dependent and -independent transcriptional changes during haploid phase gametogenesis in the sugar kelp Saccharina latissima. PLoS One 14:e0219723. doi: 10.1371/journal.pone.0219723
Pehlke, C., and Bartsch, I. (2008). Changes in depth distribution and biomass of sublittoral seaweeds at Helgoland (North Sea) between 1970 and 2005. Clim. Res. 37, 135–147. doi: 10.3354/cr00767
Rhodes, D., and Lipps, H. J. (2015). G-quadruplexes and their regulatory roles in biology. Nucleic Acids Res. 43, 8627–8637. doi: 10.1093/nar/gkv862
Ritter, A., Cabioch, L., Brillet-Guéguen, L., Corre, E., Cosse, A., Dartevelle, L., et al. (2017). Herbivore-induced chemical and molecular responses of the kelps Laminaria digitata and Lessonia spicata. PLoS One 12:e0173315. doi: 10.1371/journal.pone.0173315
Ritter, A., Goulitquer, S., Salaün, J. P., Tonon, T., Correa, J. A., and Potin, P. (2008). Copper stress induces biosynthesis of octadecanoid and eicosanoid oxygenated derivatives in the brown algal kelp Laminaria digitata. N. Phytol. 180, 809–821. doi: 10.1111/j.1469-8137.2008.02626.x
Roleda, M. Y. (2016). Stress physiology and reproductive phenology of Arctic endemic kelp Laminaria solidungula J. Agardh. Pol. Biol. 39, 1967–1977. doi: 10.1007/s00300-015-1813-x
Sabouri, N. (2017). The functions of the multi-tasking Pfh1Pif1 helicase. Curr. Gene. 63, 621–626. doi: 10.1007/s00294-016-0675-2
Schiel, D. R., and Foster, M. S. (2006). The population biology of large brown seaweeds: ecological consequences of multiphase life histories in dynamic coastal environments. Annu. Rev. Ecol. Evol. Syst. 37, 343–372. doi: 10.1146/annurev.ecolsys.37.091305.110251
Shan, T. F., Pang, S. J., Li, J., and Li, X. (2015). De novo transcriptome analysis of the gametophyte of Undaria pinnatifida (Phaeophyceae). J. Appl. Phycol. 27, 1011–1019. doi: 10.1007/s10811-014-0393-4
Steneck, R. S., Graham, M. H., Bourque, B. J., Corbett, D., Erlandson, J. M., Estes, J. A., et al. (2002). Kelp forest ecosystems: biodiversity, stability, resilience and future. Environ. Conserv. 29, 436–459. doi: 10.1017/S0376892902000322
Teagle, H., Hawkins, S. J., Moore, P. J., and Smale, D. A. (2017). The role of kelp species as biogenic habitat formers in coastal marine ecosystems. J. Exp. Mar. Biol. Ecol. 492, 81–98. doi: 10.1016/j.jembe.2017.01.017
tom Dieck, I. (1993). Temperature tolerance and survival in darkness of kelp gametophytes (Laminariales, Phaeophyta): ecological and biogeographical implications. Mar. Ecol. Prog. Ser. 100, 253–253.
Waterhouse, R. M., Seppey, M., Simão, F. A., Manni, M., Ioannidis, P., Klioutchnikov, G., et al. (2017). BUSCO applications from quality assessments to gene prediction and phylogenomics. Mol. Biol. Evol. 35, 543–548. doi: 10.1093/molbev/msx319
Wuest, S. E., Vijverberg, K., Schmidt, A., Weiss, M., Gheyselinck, J., Lohr, M., et al. (2010). Arabidopsis female gametophyte gene expression map reveals similarities between plant and animal gametes. Curr. Biol. 20, 506–512. doi: 10.1016/j.cub.2010.01.051
Yang, X., Schadt, E. E., Wang, S., Wang, H., Arnold, A. P., Ingram-Drake, L., et al. (2006). Tissue-specific expression and regulation of sexually dimorphic genes in mice. Geno. Res. 16, 995–1004. doi: 10.1101/gr.5217506
Ye, N., Zhang, X., Miao, M., Fan, X., Zheng, Y., Xu, D., et al. (2015). Saccharina genomes provide novel insight into kelp biology. Nat. Commun. 6:6986. doi: 10.1038/ncomms7986
Young, M. D., Wakefield, M. J., Smyth, G. K., and Oshlack, A. (2010). Gene ontology analysis for RNA-seq: accounting for selection bias. Geno. Biol. 11, R14–R14. doi: 10.1186/gb-2010-11-2-r14
Keywords: brown algae, gametophyte, gene expression, kelp, life cycle, sex, temperature, transcriptomics
Citation: Monteiro C, Heinrich S, Bartsch I, Valentin K, Corre E, Collén J, Harms L, Glöckner G and Bischof K (2019) Temperature Modulates Sex-Biased Gene Expression in the Gametophytes of the Kelp Saccharina latissima. Front. Mar. Sci. 6:769. doi: 10.3389/fmars.2019.00769
Received: 24 September 2019; Accepted: 28 November 2019;
Published: 12 December 2019.
Edited by:
Sandie M. Degnan, The University of Queensland, AustraliaReviewed by:
Naihao Ye, Yellow Sea Fisheries Research Institute (CAFS), ChinaVittoria Roncalli, University of Barcelona, Spain
Copyright © 2019 Monteiro, Heinrich, Bartsch, Valentin, Corre, Collén, Harms, Glöckner and Bischof. This is an open-access article distributed under the terms of the Creative Commons Attribution License (CC BY). The use, distribution or reproduction in other forums is permitted, provided the original author(s) and the copyright owner(s) are credited and that the original publication in this journal is cited, in accordance with accepted academic practice. No use, distribution or reproduction is permitted which does not comply with these terms.
*Correspondence: Cátia Monteiro, bW9udGVpcm9AdW5pLWJyZW1lbi5kZQ==