- 1Department of Nutrition and Feed Technology, Nofima – Norwegian Institute of Food, Fisheries and Aquaculture Research, Fyllingsdalen, Norway
- 2Department of Fish Health, Nofima – Norwegian Institute of Food, Fisheries and Aquaculture Research, Ås, Norway
- 3Department of Consumer and Sensory Sciences, Nofima – Norwegian Institute of Food, Fisheries and Aquaculture Research, Ås, Norway
- 4Alltech Inc., Springcroft, Mosshill, Brora, United Kingdom
Aquaculture contributes to global food security but sustainable aquaculture development in terms of biodiversity impacts requires the establishment of viable solutions in replacement of the fisheries-based components in aquafeeds. In the current work, pit-tagged Atlantic salmon individuals were grown, from fresh water (18 g body weight) to salt water in tanks (up to 800 g body weight), on diets with low fish meal (FM) (10%) and 1–1.25% total n-3 LC-PUFA levels balanced across the experimental diets. Dietary n-3 LC-PUFAs were supplemented by (1) fish oil (FO), (2) Schizochytrium limacinum biomass (ScB), or (3) a mix of the two (FO/ScB). Further, the fish from all treatments were mixed and redistributed in sea cages reared to slaughter (ca. 3 kg body weight) on either FO or ScB. As FO was rich in both EPA and DHA and ScB was rich in DHA and nearly devoid of EPA, the experimental diets differed significantly in DHA/EPA ratio (0.8 vs. 10.5 in average for FO and ScB, respectively). ScB treatment fish grew to significantly higher body weight in the end of the experiment (2.8 kg vs. 3.3 kg, for FO and ScB, respectively) but similar FCR, survival rate, and biometric indexes compared to the FO groups. ScB fish contained higher levels of EPA + DHA in the filet but lower in the liver, and better filet pigmentation already from the tank phase of the experiment as determined chemically, by salmonfan and a trained sensory panel, and lower prevalence of melanin spots at slaughter. The trained sensory panel found no differences in flavor or odor in the filets from the different dietary groups; however, filets in the FO group were perceived as softer and juicier compared to ScB. The pre-diets up to 800 g body weight had minor effects on fish performance. Global transcriptomics in liver and intestinal tissues revealed significant dietary effects on the expression of immune modulating, as well as ion, lipid, protein, and xenobiotic metabolism genes.
Introduction
Novel sustainable sources of high-quality proteins and long chain n-3 polyunsaturated fatty acid (n-3 LC-PUFA) rich oils are needed, as feed grade fisheries resources exploited for fish oil (FO) and FM production reach sustainability limits (Pauly et al., 2002; Hilborn et al., 2003; Pike and Barlow, 2003; Heal and Schlenker, 2009). In salmon feeds, FM is largely substituted by different plant protein sources (Ytrestøyl et al., 2015; Aas et al., 2019) whereas fewer alternatives are commercially relevant when it comes to dietary n-3 LC-PUFA sources. Genetically modified plants can be used for the production DHA and EPA rich oil, such as in the recently tested omega-3 canola oil successfully replacing FO in juvenile salmon diets (Ruyter et al., 2019). In recent years, large-scale fermentation technology is used for commercial production of non-genetically modified heterotrophic microalgae-like unicellular organisms such as Schizochytrium limacinum and other Thraustochytrids (Barclay, 1994b; Bowles et al., 1999), for both biofuel and other food and feed applications including fish feeds (Barclay, 1994a; Barclay and Zeller, 1996; Barclay et al., 2005). S. limacinum biomass contains high levels of fat (55–75% in dry matter) and docosahexaenoic acid (DHA) (up to 49% of total lipids) (Nakahara et al., 1996; Ren et al., 2010), and is reported as a good DHA-rich lipid source for farmed seabream Sparus aurata (Ganuza et al., 2008) and Atlantic salmon, even at 100% replacement of the supplemental dietary FO (Carter et al., 2003; Miller et al., 2007; Kousoulaki et al., 2015).
Based on records of fish growth, feed efficiency, intestine histology, survival and liver cell transcriptomics, S. limacinum biomass appears to attribute benefits on fish filet quality and n-3 LC-PUFA retention efficiency without indications of negative physiological impacts for the fish in short-term studies (Kousoulaki et al., 2015, 2016).
In the current study we present results of a lifelong study on Atlantic salmon fed practical diets with low levels of FM and either FO or whole S. limacinum biomass as supplementary dietary n-3 LC-PUFA source, at 1–1.25% total dietary EPA + DHA and similar dietary n-3/n-6 ratio and total saturated fatty acids (SFAs) levels among the experimental diets. Production performance, tissue composition, filet quality, intestinal histology, and liver and intestine microarray results are discussed.
Materials and Methods
Formulation and Chemical Analyses in Experimental Feeds
Three commercially relevant Atlantic salmon diets were formulated (Table 1), based on the trends on average raw material levels and nutrient values used in salmon feeds by the three major salmon feed producers in Norway in 2012 and 2013 (Ytrestøyl et al., 2015). The experimental diets were formulated to contain 1–1.25% EPA + DHA (in the diet) and were further balanced for crude protein, crude lipid, digestible energy, total SFAs, and n-3/n-6 fatty acid ratio, using different oil blends and plant protein mixes. FO were the control diets containing low FM level (FM in diet FO: 10%), and FO as supplementary n-3 LC-PUFA source. FO/ScB, used only in the tank phase trials, also contained 10% FM and a mix of FO and S. limacinum biomass, the latter providing 50% of total EPA + DHA in the diets, whereas ScB diets contained only S. limacinum biomass as supplementary n-3 LC-PUFA source, besides the respective dietary contribution of FM. The S. limacinum biomass used was whole and spray dried (Alltech Inc., United States). The chemical composition of the biomass is as described in Kousoulaki et al. (2016). The experimental diets were produced at the Feed Technology Center of Nofima in Bergen, Norway, in the same production series, using a Wenger TX-52 co-rotating twin-screw extruder with 150 kg h–1 capacity. The settings of the extruder were “normal,” i.e., the production can be up scaled to a feed factory [extruder settings considered: screw configuration (D), die opening (4.5 mm), knife speed (1494–1981 rpm), Specific Mechanical Energy (7.7–9.5 kW), feed rate (150 kg/h), and amount of steam (0 kg/h) and water (0.15–0.41 kg/min) added to the process]. The levels of S. limacinum biomass used in the experimental diets FO/ScB and ScB were within the range that allows the production of good hot extruded pellets under similar conditions (Samuelsen et al., 2018) with high nutrient digestibility (Kousoulaki et al., 2015, 2016).
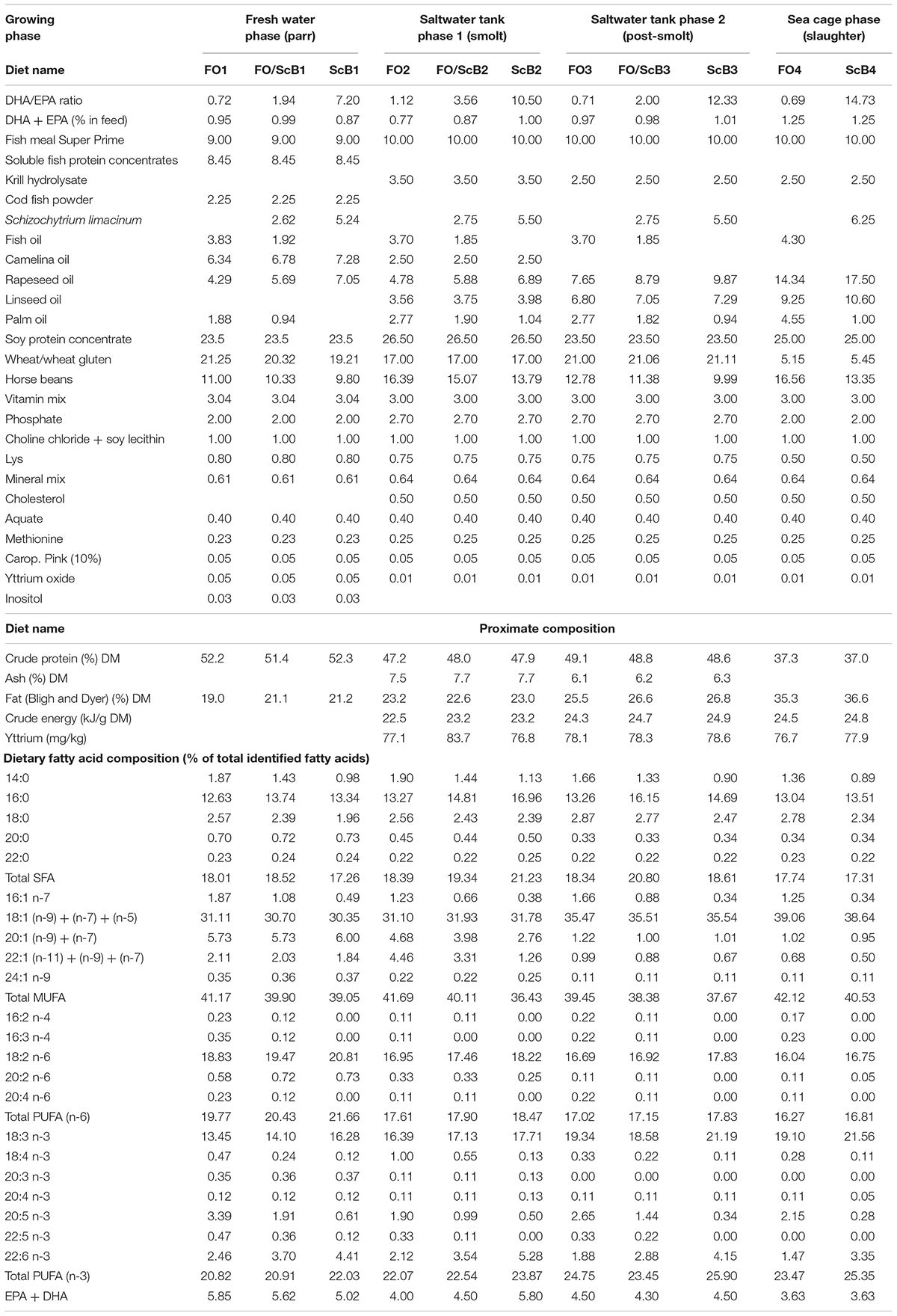
Table 1. Experimental diets’ formulations (raw material inclusion levels in %) and chemical composition.
Feeds were analyzed for proximate composition according to standard methods: Crude protein: (Kjeldahl method N × 6.25; ISO 5983,1997), moisture (ISO 6496,1999), ash (ISO 5984,2002), and lipid (Bligh and Dyer, 1959). Preparation of fatty acid methyl esters (FAMEs) for the determination of fatty acid profile in raw materials and feeds was realized according to the AOCS Official Method Ce 1b-89 using a trace GC gas chromatograph (Thermo Fisher Scientific) with flame ionization detector (GC–FID), equipped with a 60 m × 0.25 mm BPX-70 cyan propyl column with 0.25 μm film thickness (SGE, Ringwood, VIC, Australia). Helium 4.6 was used as a mobile phase under the pressure of 2.60 bar. The injector temperature was 250°C and the detector temperature was 260°C. The oven was programmed as follows: 60°C for 4 min, 30°C min–1 to 164°C, and then 1.0°C min–1 to 213°C, and 100°C min–1 to 250°C where the temperature was held for 10 min. The sample solution (3.0 μl) was injected split-less and the split was opened after 2 min. The FAMEs were identified by comparing the elution pattern and relative retention time with the reference FAME mixture (GLC-793, Nu-Chek Prep Inc., Elysian, MN, United States). Chromatographic peak areas were corrected by empirical response factors calculated from the areas of the GLC-793 mixture. FA composition was calculated by using 23:0 FAME as internal standard and reported on a sample basis as g/100 g FAMEs. Dietary gross energy was determined in a Parr adiabatic bomb calorimeter. All aforementioned analyses were performed at Nofima laboratories in duplicate. If differences between parallels exceeded standardized values, new duplicate analyses were carried out. Yttrium in feeds and feces was analyzed at an external laboratory (Eurofins, Molde, Norway).
Fish Feeding Trials
The trial period commenced in tanks in February 2015, and lasted ca. 11 months, i.e., until December 2015. During the tank phase, individually pit-tagged fish were fed one of three experimental diets (FO, FO/ScB, or ScB) in triplicate tanks and were weighed and samples were taken for tissue analyses from 10 fish per tank four times: at start (ca. 18 g fish mean body weigh BW), soon after smoltification (at ca. 60 g BW), at ca. 200 g BW and at ca. 800 g BW. At the end of the tank phase of the trial all fish were mixed and transferred to the cage facilities of Marine Harvest at Averøy, Norway, where they were distributed by equal representation of fish from each tank into each on of six trial cages and reared on either FO or ScB in triplicate, for over one more year (Supplementary Figure S1). This was realized in order to detect early life and dilution effects of variable dietary levels of DHA, EPA, and feeding salmon relatively high levels microalgae biomass as a replacement for FO. In January 2017, the trial was ended, and fish had cage mean BW of ca. 2800–3300 g. During samplings, fish that would go further in the trial were sedated using low doses of tricaine methane-sulfonate (MS-222 at 0.01–0.02 g/l) prior to further handling (weighing and pit-tag reading). Sample fish were euthanized with an overdose of the same anesthetic (MS-222 0.05–0.08 g/l) before tissue sampling.
Tank Phase (Fresh and Saltwater)
Each one of the experimental feeds was given to triplicate populations of Atlantic salmon parr kept in tanks at the land-based facilities of Nofima at Sunndalsøra, Norway. The start BW of the experimental fish was 18 g and the final BW was ca. 800 g. During the freshwater phase the fish were kept in 150-L circular fiberglass tanks, with 2.3 kg initial and 4.8 kg final biomass per tank (15.3 and 32.0 kg/m3 density, respectively). During the saltwater phase the fish were kept in 2.3 m2 fiberglass tanks with 60 cm water depth, at 4.8 kg initial biomass and 56.2 kg per tank final biomass (3.1 and 40.7 kg/m3 density, respectively). Feeding was continuous using automatic feeders. The daily feed amounts given were calculated to provide 120% of the fish’s satiation levels. Uneaten feed was collected and weighed daily for the estimation of total daily feed intake in each tank only during the salt water stage of the tank trial period, i.e., after smoltification from ca. 60 to 800 g body weight, but not during the fresh water phase as feed pellets were too small to be collected in a representative way. The mean water temperature during the trial was ca. 9°C during fresh water and saltwater phase 1, and 11.5°C during tank saltwater phase 2. In the saltwater phases, sea water with a salinity ranging between 32 and 33 ppt was used. The water system was flow-through using UV treated and filtrated fresh or sea water from 40 m depth. At the end of the tank trial phase, fish from each tank were pit-tag scanned, weighed, measured for biometric indexes, sampled for tissues, and stripped for feces, the latter were separated from urine and collected in a pre-weighed box per tank and frozen immediately at −20°C until further analyses. At the end of the tank trial phases, whole fish were used for lipid and fatty acid profile analysis and fish filets were used for sensory, lipid, and fatty acid profile analyses.
Sea Cage Phase
Atlantic salmon from the end of the tank trial [section “Tank phase (fresh and saltwater)”], at ca. 800 g average body weight, were transferred from the in-door tanks in Nofima Sunndalsøra, Norway, to six seawater net-cages at Marine Harvest’s facilities at Averøy, Norway. The fish were transported by a truck with six separate tanks containing 81 fish each dedicated for each one of the six-destination net-pens. Fish from all three pre-treatments were equally distributed to each one of the six net-cages, 27 fish from each pre-treatment (FO, FO/ScB, and ScB) in each net-cage, approximately 71 kg initial biomass per cage. Diets FO and ScB were randomly assigned to three cage each and fed until slaughter resulting in six pre-end-feed dietary treatments in total (FO:FO, FO/ScB:FO, and ScB:FO in the FO fed cages and FO:ScB, FO/ScB:ScB, and ScB:ScB in the ScB cages). The net-cages (125 m3) were equipped with automatic feeders (Betten, Vågland, Norway), feeding three times × 30 min each day, and equipment for collection of feed waste. The fish were raised to a weight of ca. 3 kg, before final harvest and sampling. Sea transfer took place in December 2015, when water temperature was 7.4°C, but declining. Minimum temperatures were recorded during the winter and were 3.8°C, and the maximum water temperature recorded was 17.9°C during summer 2016.
Lice removal was required seven times during the months from February to October 2016 and was realized by hand. Slice was also given during 1 week in September 2016. Cleaner fish Ballan wrasse (Labrus bergylta) were introduced in the cages in September 2016, at ratios up to 24% of the salmon individual numbers. Nets were changed six times during the cage trial. In October 2016, sexually mature fish and obvious “losers” were removed, as such fish tend to attract even more lice, and their predominance was similar in the two treatments. At the end of the trial all fish were pit-tag scanned, weighed, and measured for standard length. Fish were sampled for analyses in three batches, where each batch consisted of three randomly selected fish per pre-treatment group (nine fish in total per sea cage). Batches 1 and 2: Fish were anesthetized to death before sampling of (i) fish for whole body analyses and (ii) liver, skeletal muscle (the cutlet between the posterior end of the dorsal fin and the intestine, termed Norwegian Quality Cut, NQC), and intestine tissue, respectively. Batch 3: The fish were cut by the gills after anesthetization, bled for 10 min in iced seawater before filets were sampled for filet technical quality, color scoring, and analyses by a trained sensory panel. All sampled fish were visually scored for intestinal fat and liver color. Feces were collected from the rest of the fish in each net pen. Whole filets (Batch 3) were stored on ice for 1 week before they were subjected to quality analyses of raw filets and cutting into 2.5 cm × 2.5 cm pieces, vacuum packed, and frozen before sensory assessment of raw and cooked samples.
Fish Filet and Whole-Body Composition and Filet Technical Quality
Fish filet and whole-body nutritional quality were evaluated in terms of fatty acid, technical, and sensory quality. Melanin discoloration (presence of melanin spots) and gaping were evaluated on whole filet sides, while mechanical properties (firmness) and filet color were analyzed in duplicate on the dorsal (anterior) and NQC filets part (posterior). Astaxanthin and idoxanthin levels were analyzed in the NQC cutlet. NQC cutlets from nine fish per tank and three fish per dietary treatment per cage at slaughter were pooled, homogenized, and analyzed for fatty acid composition. Lipid extraction was realized according to Bligh and Dyer (1959) and the fatty acid composition according to AOCS Ce 1b-89 as described in section “Formulation and Chemical Analyses in Experimental Feeds.” Filet color was evaluated visually using DSM SalmonFanTM (score 21–34). Instrumental analyses of fish filet firmness were performed perpendicular to the muscle fibers using a Texture analyzer, TA-XT2 (Stable Micro System Ltd., Surrey, United Kingdom) equipped with a flat-ended cylindrical probe (12.5 mm diameter, type p/0.5) at a 1 mm/s traveling speed and a 30 kg load cell. Firmness predicted using this method correlates well with sensory assessment of firmness of raw and smoked salmon filets (Mørkøre and Einen, 2003). Melanin discoloration was evaluated visually as the number of dark, hyperpigmented myotomes (Mørkøre et al., 2015).
Homogenized filets from individual fish were thawed, and carotenoids were extracted using a 1:1:3 mixture of distilled water, methanol (containing 500 mg l–1 BHT), and chloroform according to Bjerkeng et al. (1997). The solvent was removed from an aliquot under reduced pressure and re-dissolved in mobile phase (acetone/n-hexane/methanol 20:80:0.1), filtered through a 0.45-μm filter (Minisart SRP 15, Göttingen, Germany), and analyzed isocratically by HPLC. A Spherisorb S5-CN nitrile column (PhaseSep, Queensferry, Clywd, United Kingdom; length 250 mm; internal diameter 4.6 mm; particle size 5 mm), using 20% acetone in n-hexane as the mobile phase was used to determine astaxanthin and 3′,4′-cis and 3′,4′-trans glycolic isomers of idoxanthin in muscle samples. The retention times (RT) of astaxanthin and the 3′,4′-cis and 3′,4′-trans glycolic isomers of idoxanthin on this system were about 4, 6.5, and 8.5 min, respectively. The Shimadzu LC-10AS Liquid Chromatograph was connected to a Shimadzu SPD-M6A Photodiode array UV–VIS detector and the detection wavelength was set to 470 nm. Standards of known concentration were prepared from crystalline all-E-astaxanthin (Hoffmann-La Roche Ltd., Basel, Switzerland), and the concentration of the standard solution was measured spectrophotometrically (UV-260, Shimadzu, Japan) using molar absorptivity E1%,1 cm = 2100 at absorbance maximum (λmax = 470 nm) in n-hexane containing 4.5% chloroform. The percentages of the different isomers were calculated from chromatogram areas and corrected for differences in extinction coefficients (E1%,1 cm). Astaxanthin concentrations were calculated using E1%,1cm-values of 2100 for all-E-astaxanthin (Britton, 1995), and 1350 and 1750 for 13Z- and 9Z-astaxanthin, respectively.
Sensory Analysis
A trained panel of 10 assessors (10 women, aged 37–64 years) at Nofima (Ås, Norway), performed a sensory descriptive analysis according to “Generic Descriptive Analysis” as described by Lawless and Heymann (2010) and (ISO 13229,2016). Sensory analysis – Methodology – General guidance for establishing a sensory profile (2016). The assessors had been tested, selected, and trained according to ISO standards (ISO 8586,2012), and the sensory laboratory follows the practice of ISO standards (ISO 8589,2007). Raw salmon was evaluated for odor (sour, marine, cucumber, algae, feed, fish, odor) and color [yellowish color (hue), intensity, whiteness]. Cooked salmon was evaluated for flavor (sour, bitter, marine, cucumber, feed, fish, rancid) and texture (hardness, juiciness, and cohesiveness). Salmon filets were thawed overnight at +0–1°C before sample preparation the following day. The muscle was baked in a combi-oven (Electrolux Air-o-steam, Model AOS061EANQ; +75°C and 50% steam/50% heat) until a core temperature of +58°C was reached. Samples were then cooled immediately to +0–1°C. Both raw and heated samples, coded at a three-digit number, were served at the same time in white plastic container with a lid at +18°C. All attributes were evaluated on unstructured line scales with labeled endpoints ranging from “no intensity” (1) to “high intensity” (9). Each assessor evaluated the samples at individual speed on a computer system for direct recording of data (EyeQuestion, Software Logic8 BV, Utrecht, Netherlands). The coded samples were evaluated in duplicate per dietary per-treatment and served in a balanced block experimental design over 2 days and 10 sessions. Tap water and unsalted crackers were available for palate cleansing.
Skin Coloration
Non-invasive skin color analyses were performed at the end of the cage trial, using a Minolta Chroma Meter (CR-400 Minolta, Konica Minolta Sensing, Inc., Japan). The tristimulus CIE L∗a∗b∗ 1976 color space was used, where the L∗ variable represents lightness (L∗ = 0 for black, L∗ = 100 for white) and the a∗ and b∗ indicate color directions: a∗ is the red direction, −a∗ is the green direction, +b∗ is the yellow direction, and −b∗ is the blue direction. Analyses were performed anterior and posterior to the dorsal fin, below and above the lateral line.
Microarray Analyses
Sample tissue (mid intestine and liver) RNA was extracted using PureLink RNA Mini kits according to the manufacturer’s protocol (Invitrogen). Concentration of total RNA (NanoDrop 1000 Spectrometer, Thermo Scientific, Waltham, MA, United States) and RNA integrity was measured (Agilent 2100 Bioanalyzer with RNA Nano kits, Agilent Technologies, Santa Clara, CA, United States). Samples with RNA integrity number (RIN) > 8 were accepted for analysis. Multiple gene expression profiling was performed with the Nofima’s Atlantic salmon 15k SIQ6 array produced by Agilent Technologies, Santa Clara, CA, United States. One-color hybridizations were performed. Analyses included 12 replicates from each study group, totally 48 microarrays were used [two tissues × 2 dietary groups (FO and ScB from start to slaughter) × 12 fish]. RNA amplification, labeling, and fragmentation were performed using the One-Color Low Input Quick Amp Labeling kit and Gene Expression Hybridization kit following the manufacturer’s instructions (Agilent Technologies, Santa Clara, CA, United States). The input of total RNA in each reaction was 100 ng. The samples were labeled with Cy3. Overnight hybridization (17 h, 65°C, and rotation speed of 10 rpm) was executed in an oven (Agilent Technologies, Santa Clara, CA, United States). The slides were washed with Gene Expression Wash Buffers 1 and 2 and scanned with Agilent scanner. Data were processed with Nofima’s pipeline STARS (Krasnov et al., 2011) as described in Pellizzari et al. (2013). Global normalization was performed by equalizing the mean intensities of all microarrays. Next, the individual values for each feature were divided to the mean value of all samples thus producing expression ratios (ER). The log2-ER were calculated and normalized with the locally weighted non-linear regression (Lowess). Differentially expressed genes (DEG) were selected by the following criteria: ER > 1.5-fold and p < 0.05 (t-test). Results were submitted to NCBI GEO (GSE140558).
Histological Observations in the Intestine
The mid and hind intestine from 12 fish from each one of two dietary groups (ScB:ScB and FO:FO) in the present trial was evaluated histologically. Samples of mid and hind intestine were fixed in 4% buffered formalin and stored at 4°C until processing. Paraplast-embedded samples were cut with a Leitz 1208 microtome (Ernst Leitz Wetzlar GmbH) (5 μm) and stained with standard hematoxylin–eosin (Merck KGaA). Stained slides were examined using a standard light microscope (Nikon Optiphot, Nikon). Images were captured by a Micropublisher 3.3 RTV camera and QCapture 2.9.13 software (QImaging). A total of 48 samples (from 24 fish) were processed blind, 12 samples per end diet. Further on, observations were realized in coded samples in order to characterize systematic differences.
Calculations and Statistics
Apparent digestibility coefficient (ADC) of dietary nutrients in the test diets was calculated from the following formula: ADC = 100 – 100 × Yd × Nf/Nd/Yf, where d is the diet, f is the feces, Y yttrium content, and N nutrient content. Feed conversion ratio (FCR) is feed consumed/biomass increase; protein efficiency ratio (PER) is the fish weight gain/protein consumption; thermal growth coefficient (TGC) is (w21/3− w11/3) × 1000/Σ(t × feeding days), where Σ(t × feeding days) is the sum of water temperatures (°C) for every feeding day in the experiment (Cho, 1992); condition factor (CF) is bw (g) × fish fork length-3 (cm) × 1000; dress out percentage (D%) is gutted fish weight/bw × 100; hepatosomatic index (HSI) is liver weight/bw × 100. Data were tested for normality using a Kolomogorov–Smirnov test and homogeneity of variance using Levene’s test. Biological and analytical data were subjected to analysis of variance (ANOVA) tests (univariate and repeated measures GLM) using IBM SPSS statistics 24. When differences among groups were identified, pairwise comparisons among means were made using Duncan’s post hoc test. Significant treatment effects were considered at a significance level of P < 0.05 and tendencies at P < 0.1. For sensory performance, ANOVA using a two-way mixed model with the assessor and interaction effects considered random and samples as a fixed effect was performed on the descriptive sensory data to identify the sensory attributes that discriminated between samples. Least significance differences were calculated by Tukey’s test (P < 0.05). A principal component analysis (PCA) analysis on the average of the sensory descriptive data was performed with mean centered data and no standardization. The statistical software used for the sensory analysis was EyeOpenR (Logic8 BV). For the multivariate data analysis, Unscrambler X Version 10.4.1 was used for the PCA.
Results
Experimental Diets
The experimental diet’s fatty acid and proximate composition is presented in Table 1. The diets per trial segment were similar in all respects where intended and as expected FO diets had the lowest, FO/ScB intermediate, and ScB diet the highest DHA/EPA ratio.
Fish Performance and Biometrics
Fish Group Performance
During the tank salt water phase and using mean values of measurements for all fish in each tank as unit, fish growth rate was high and similar for all treatments (TGC of 3–3.8), and FCR was low (0.67–0.79 on feed dry matter basis) with no significant difference between the three dietary treatments (Table 2). Likewise, CF, D%, and HSI showed no significant differences between the dietary treatments during the tank trial phase. In the cage trial phase, body growth was significantly higher for the ScB compared to FO treatment. FCR was elevated compared to the tank phase and was similar in all treatments during the cage trial phase, ranging from 1.27–1.43 for the FO and 1.32–1.41 for the ScB treatments, respectively. At 2.9–3.2 kg mean fish body weight, at the end of the cage trial, all ADC values measured were reduced in both end treatments, compared to earlier growth stages in this trial (in particular for lipids, then total energy and less for protein) or previously reported studies (Kousoulaki et al., 2015, 2016). In the smolt phase ADC of protein tended to be higher in the ScB compared to the FO diet whereas there was a gradual decrease in lipid ADC with increasing inclusion level of S. limacinum biomass in the diets. No dietary effects were noticed in terms of ADC of energy in the smolt phase. At slaughter, protein ADC was similar for the FO and ScB treatments and the results on ADC of lipids were inversed compared to the smolt phase and previous studies (Kousoulaki et al., 2015, 2016), with the ScB rich diet having significantly higher lipid ADC compared to FO. ADC of energy also tended to be higher for the ScB feed.
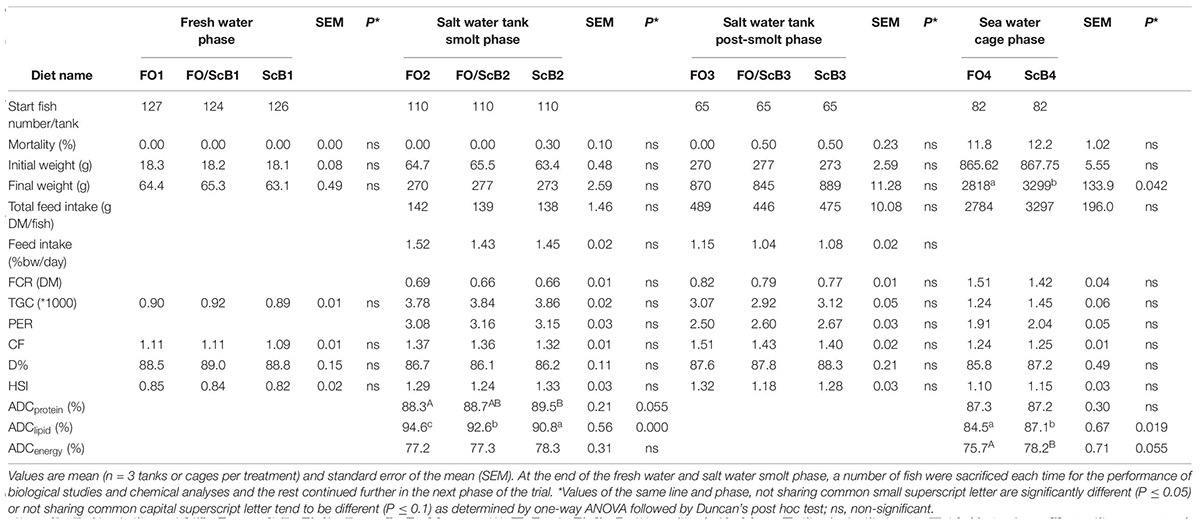
Table 2. Growth, feeding performance, and biometrics of all Atlantic salmon individuals participating each one of the successive trials, fed diets with different levels of fish oil (FO), and Schizochytrium limacinum biomass (ScB).
The final mean body weights of the fish in the FO end treatment varied compared to that of the ScB (mean fish BW in FO cage 1: 2609 g, FO cage 2: 2691 g and FO cage 3: 3154 g; ScB cage 1: 3187 g, ScB cage 2: 3355 g, and ScB cage 3: 3353 g) thus most of the observed effects, though large, were not statistically significant when analyzed with one-way ANOVA and cage as unit.
Individual Fish Performance
The observed effects were more pronounced when using the data from individually pit tagged fish analyzed for pre-diet and end diet treatment effects (Table 3). Repeated measured ANOVA was conducted to evaluate the effect of time and (pre)-feeding on fish body weight and TGC in the tank phase, and the effect of time, pre-feeding, and end-feeding on fish body weight and CF during the cage phase of the trial, using individual fish measurements and the results are summarized in Table 4. Equality of error of variances was tested with Levene’s test and was found not significant in all cases (P > 0.05). The results of the ANOVA indicated a significant effect of time (P < 0.001) and tank (P < 0.001) on both BW and TGC and also of (pre)feed on TGC during the tank phase. Pairwise comparisons with Duncan’s post hoc test show that BW increased with time whereas TGC was lowest close to smoltification, highest just after, and decreased before transfer to the sea. TGC was significantly higher in ScB compared to the FO/ScB groups whereas that in FO was not significantly different compared to the two other treatments during the tank trial phase. In the sea cage phase of the trial, ANOVA showed significant effect of cage (P < 0.001) and an indication of effect of pre-feeding (P = 0.054) and end-feeding (P = 0.062) on fish BW. The groups fed the FO diet in the cages had at slaughter significantly lower body weight compared to the ScB groups (P < 0.001), however, within these groups the fish that were fed previously with FO were the largest, followed by FO/ScB and ScB, but the effect of pre-feeding was not statistically significant (P > 0.1). Body weight was lowest in two of three FO cages compared to the 3rd one and all three ScB cages (P < 0.001). Fish CF decreased significantly from the end of the tank phase to slaughter (P = 0.000) and was affected significantly by pre-diet (P = 0.000) but not the cage or end diet (P > 0.1). Pairwise comparisons showed that CF in fish previously fed the FO feed was higher compared to those fed FO/ScB (P = 0.096) or ScB (P = 0.069), and the two latter were not different from each other (P > 0.1).
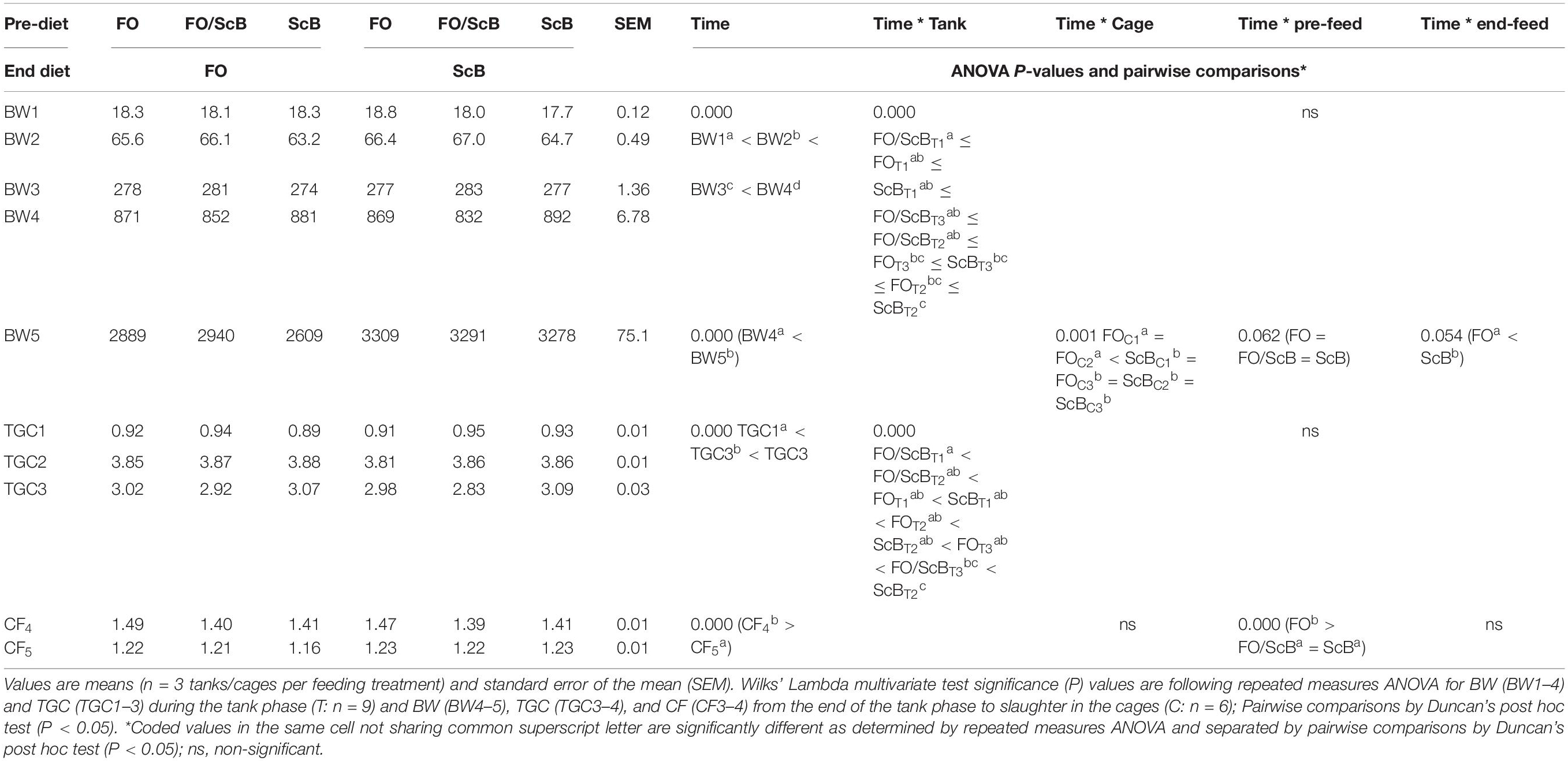
Table 3. Individually pit-tagged fish body weight (BW), TGC, and condition factor (CF) evolution from trial start to slaughter.
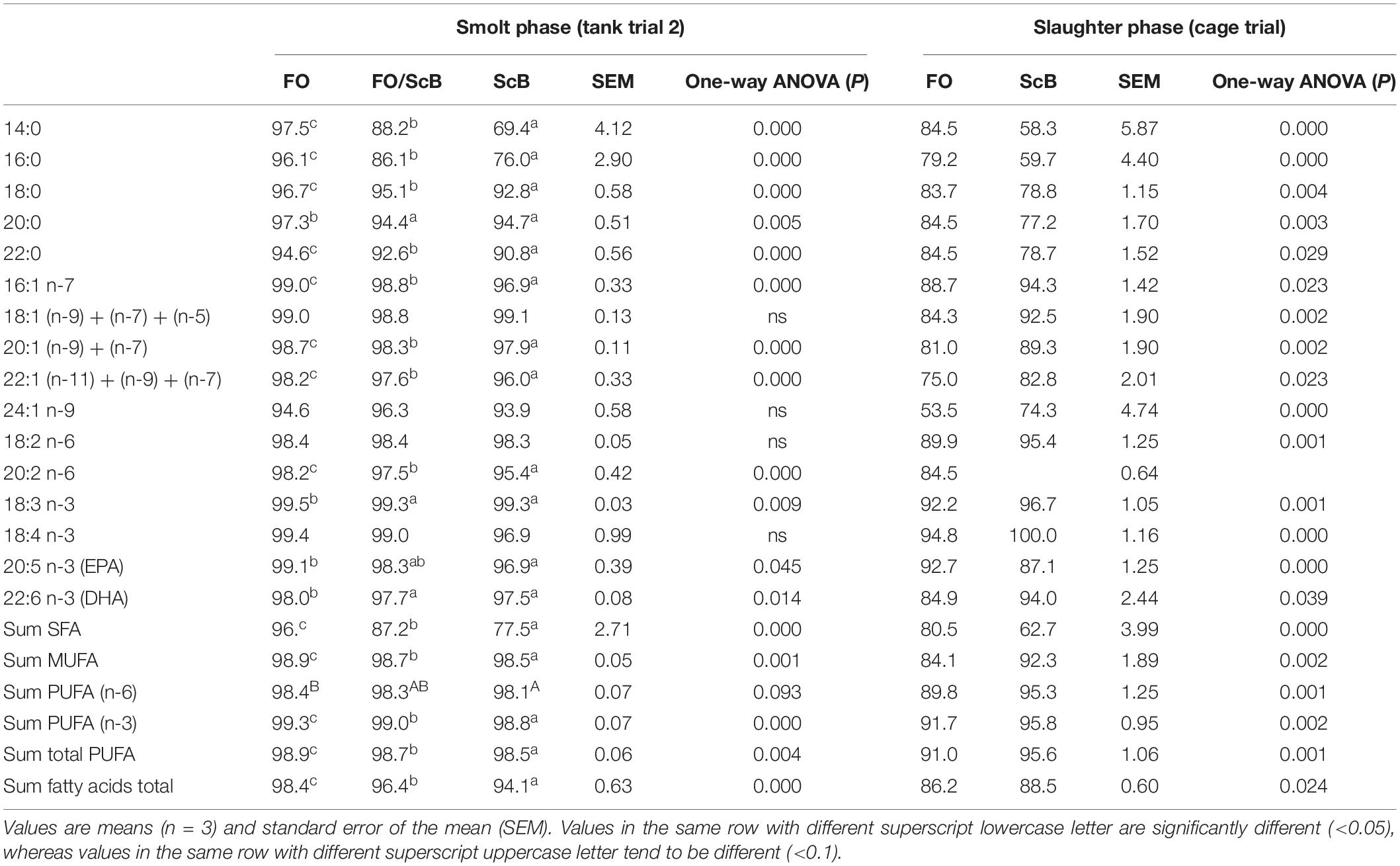
Table 4. Dietary fatty acid apparent digestibility coefficient (ADC) (%) in Atlantic salmon fed diets with fish oil (FO) or ScB as supplementary marine omega 3 fatty acid sources at slaughter (fish mean body weight 2.8–3.2 kg).
ADC of Dietary Fatty Acids
In terms of ADC of individual fatty acids (Table 4), the dietary treatments differed significantly nearly in all both phases (smolt and slaughter). In general, at smolt phase the ADC of nearly all analyzed fatty acids was highest in the FO, intermediate in the FO/ScB, and lowest in the ScB treatments. At slaughter the FO dietary group had higher ADC of SFA and lower ADC of MUFA, n-3 and n-6 LC-PUFA compared to ScB.
NQC Filet Lipid Level, Fatty Acid Composition, and Organ Scores
The NQC filet lipids generally mirrored the dietary composition and were also influenced by the apparent digestibility of the different dietary fatty acids (Table 5). Thus, the FO (end feed) fish had higher levels of total SFA and specifically 14:0, 16:0, and 18:0, total MUFA and specifically 16:1, 18:1, and 22:1, as well as 22:5 n-3 and 20:5n-3 (EPA) in their filet and lower levels of total n-6 PUFA fatty acids and specifically 18:2 n-6 and 20:2 n-6, total n-3 PUFA fatty acids, and specifically 18:3 n-3 (ALA, a-linolenic acid) and 22:6 n-3 (DHA), and total EPA + DHA compared to the ScB treatment. The respective levels in fish previously fed a mix of FO/ScB or FO and then ScB and vice versa, were in between. Liver score regarding coloration was significantly affected by the end diet. The used method for liver color scoring is developed to grade livers in terms of coloration most commonly related to lipid levels providing numbers corresponding to the different gradients of liver “paleness” where more “healthy” looking livers have a more dark red hue whereas fatty livers have a more yellowish – orange hue. Livers in ScB fish at slaughter had a dark green tone not occurring in FO fish. The authors have no previous experience regarding this observation. No differences were found in the intestinal fat score of the sample fish at the end of the sea cage trial.
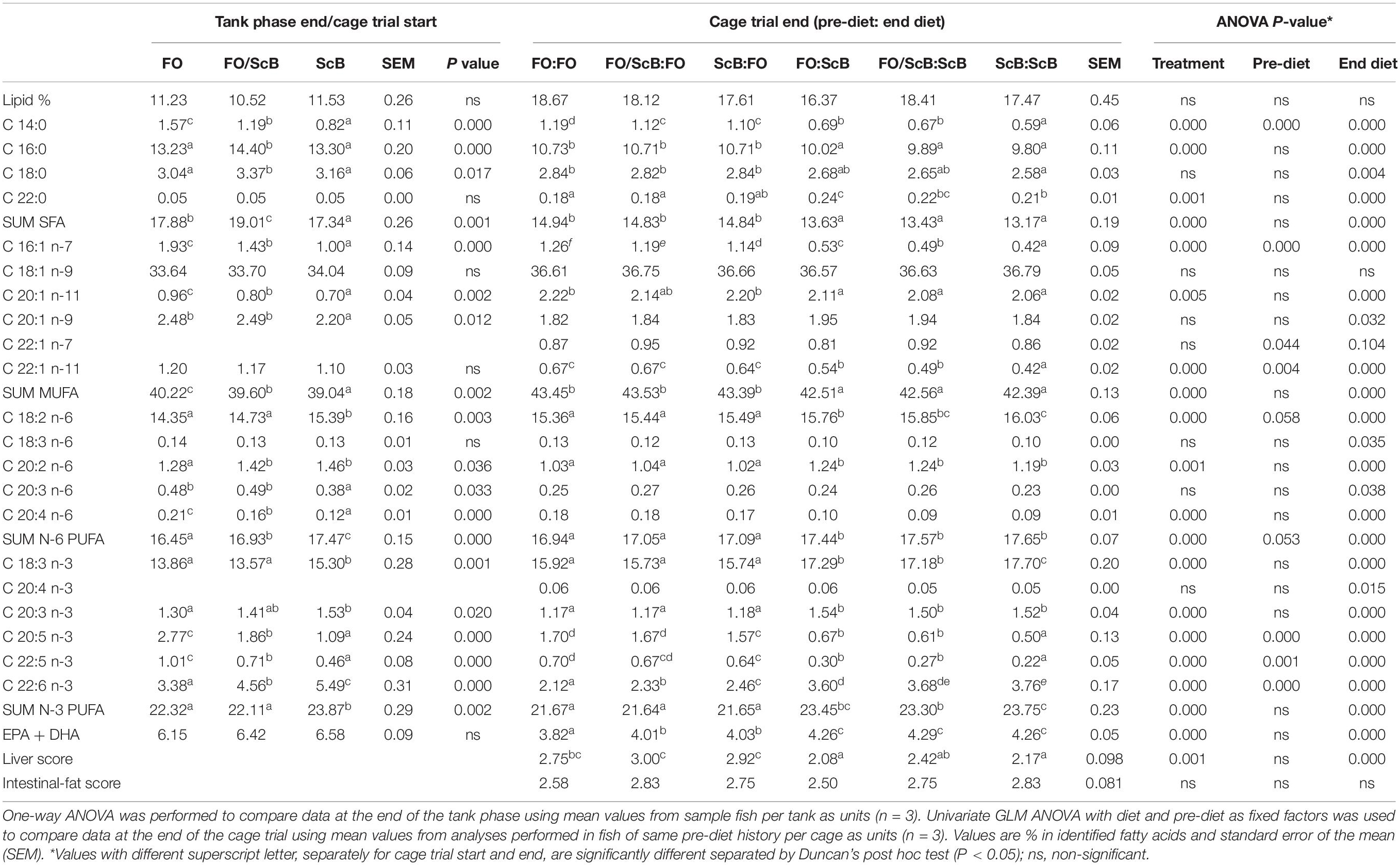
Table 5. Fatty acid composition in filets of A. salmon fed life-long diets supplemented with FO, ScB, or a mix of the two until 0.8 kg body weight and either FO or ScB to slaughter.
Liver Lipid Level and Fatty Acid Profile
Total liver lipid levels were higher in the ScB treatment at the end of the sea cage phase (Table 6). Nevertheless, within the ScB fish, one group was distinct, namely that previously fed the FO diet, having higher levels of liver lipids, the lowest levels of SFA, sum n-3 and EPA + DHA, and the highest levels of MUFA and N-6 compared to all other pre-treatments, both from the FO and ScB end-feed groups (not in all cases significantly compared to the two remaining pre-treatment groups within ScB). Pre-diet alone had no significant effect on the total lipids or liver fatty acid profile. End diet, as in the case of the filets, affected significantly nearly all analyzed liver fatty acids. Livers had higher relative levels of total SFA and N-3 PUFA and lower levels of MUFA and N-6 PUFA compared to the filet. Again, as in the filet, FO fish had significantly higher levels of SFA and N-6 PUFA in the liver compared to the ScB end diet treatment. However, unlike in the filet the levels of MUFA, N-3 PUFA, and EPA + DHA were significantly lower in the liver of the ScB compared to the FO end diet fish.
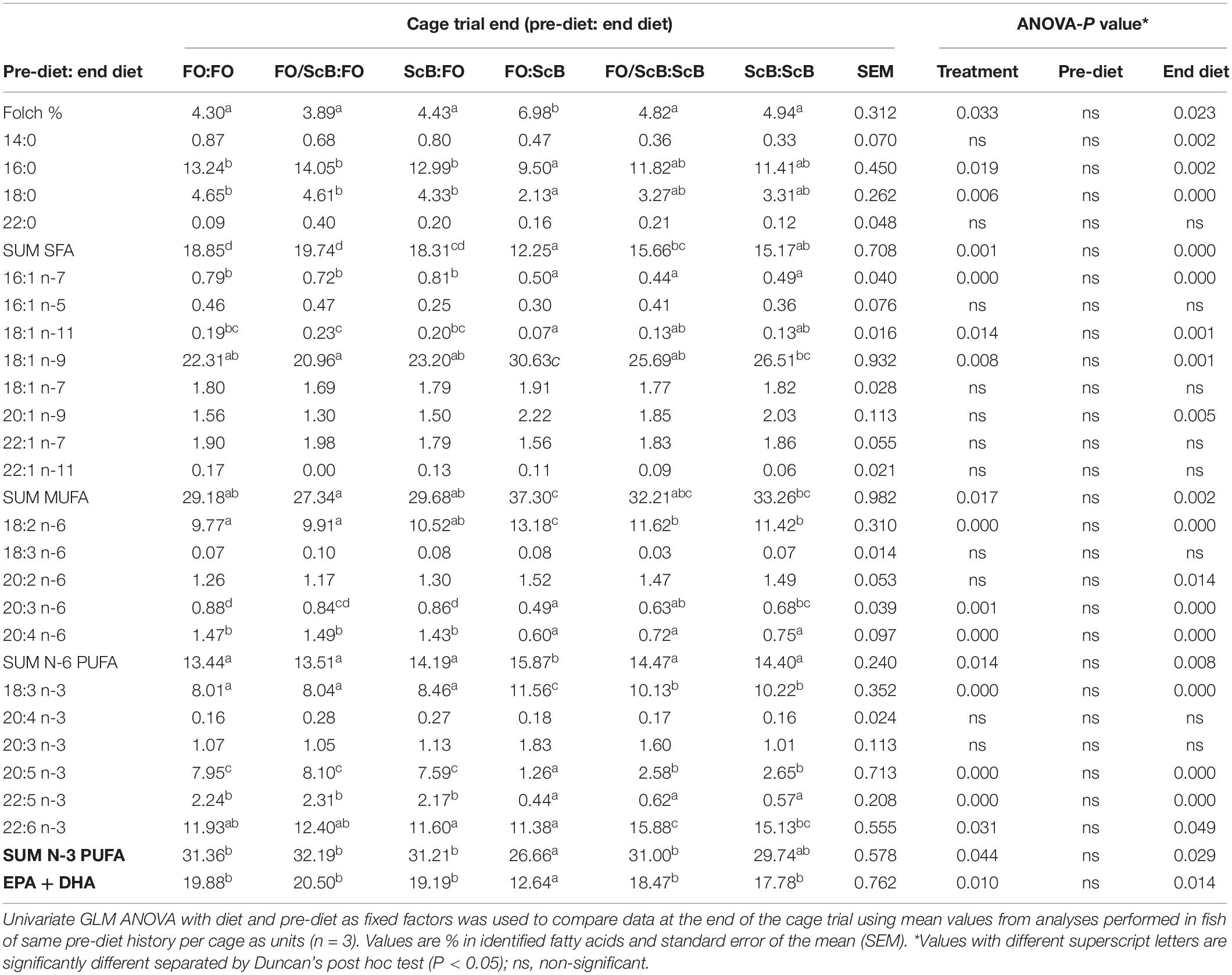
Table 6. Fatty acid composition in liver of A. salmon fed life-long diets supplemented with FO, ScB, or a mix of the two until 0.8 kg body weight and either FO or ScB to slaughter.
Whole Body Lipid Level and Fatty Acid Profile
Whole body lipids and fatty acid composition did not differ among the two dietary treatments during the sea cage period, and all effects of pre-dietary treatments which were developed during the tank-phase of the experiment were not present at the end of the cage trial phase (Table 7).
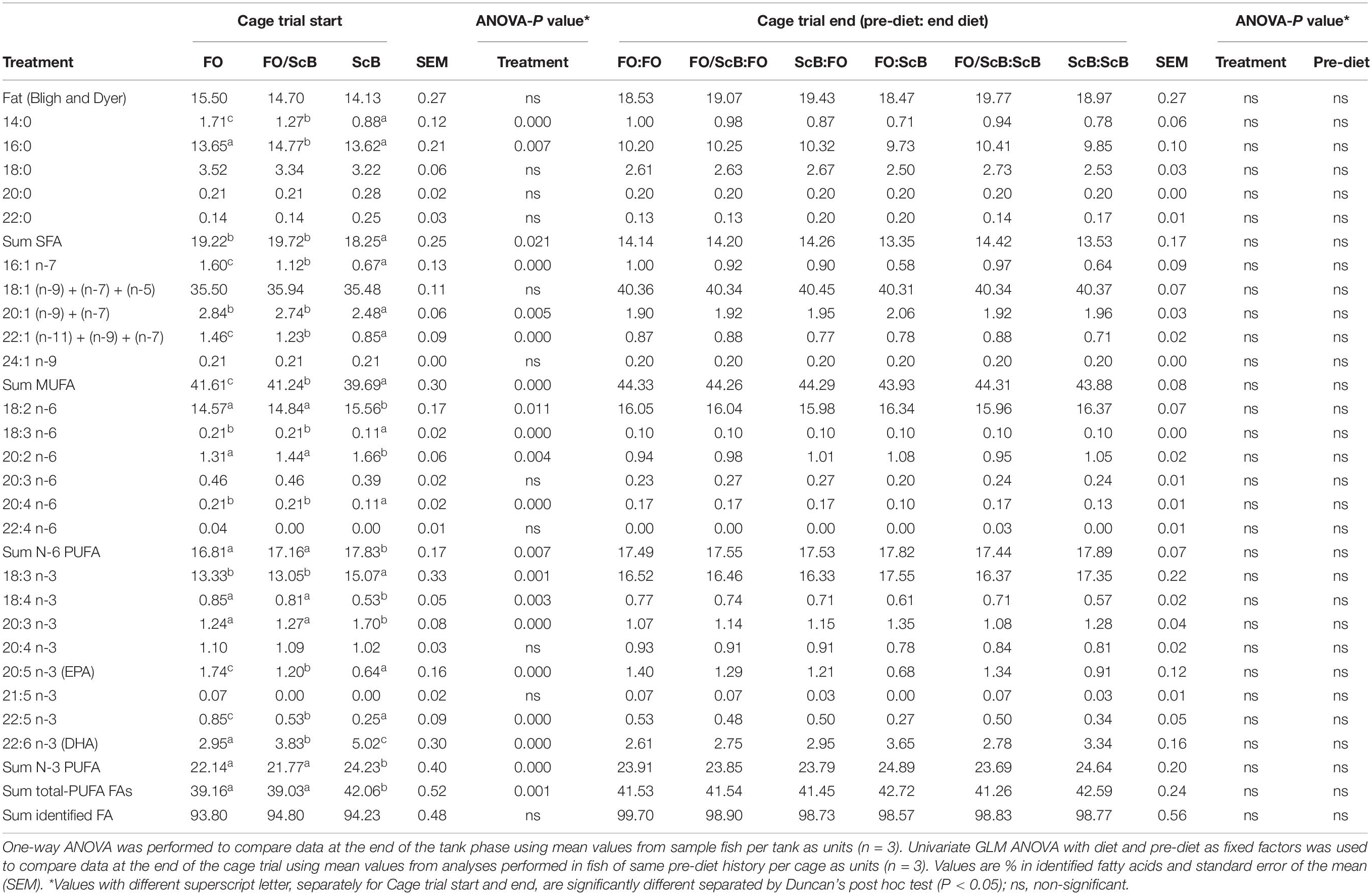
Table 7. Fish whole body fat (%), identified fatty acids (% of fat), and fatty acid composition (% of identified fatty acids) in salmon at start and end of cage trial period fed either ScB or FO diets.
Filet Pigmentation, Color, and Technical Quality
The fish in the ScB treatment had in the end of the cage trial significantly higher levels of astaxanthin but not idoxanthin compared to FO (Table 8). Pre-feeding had no effect on the final pigmentation results. ScB had also higher visual color scores in the filets compared to the FO groups. The filet color evaluated by SalmoFan in the three filet segments from head to tail were P1 = 27.85 ± 0.46, P2 = 27.00 ± 0.48, and P3 = 28.63 ± 0.55 for FO and P1 = 28.56 ± 0.11, P2 = 27.30 ± 0.45, and P3 = 29.07 ± 0.45 for ScB. Paired sample t-test showed significantly higher filet color both in the anterior part of the filet and the posterior for the fish fed the ScB compared to the FO supplemented feeds (P = 0.001; n = 3) in the end of the cage trial, but the differences were nevertheless small. Colorimetric analyses of the skin showed more greenish color (lower a∗ values) of the ScB group and a tendency to more bluish color (lower b∗ values) color compared with FO group. Moreover, pre-dietary treatment affected skin darkness. Filets of salmon fed ScB before harvesting had significantly less segments (myomera) with melanin discoloration compared to FO, but no effect was observed on filet gaping or firmness. However, filet firmness was affected by pre-diet as well as interaction of pre- and end diet.
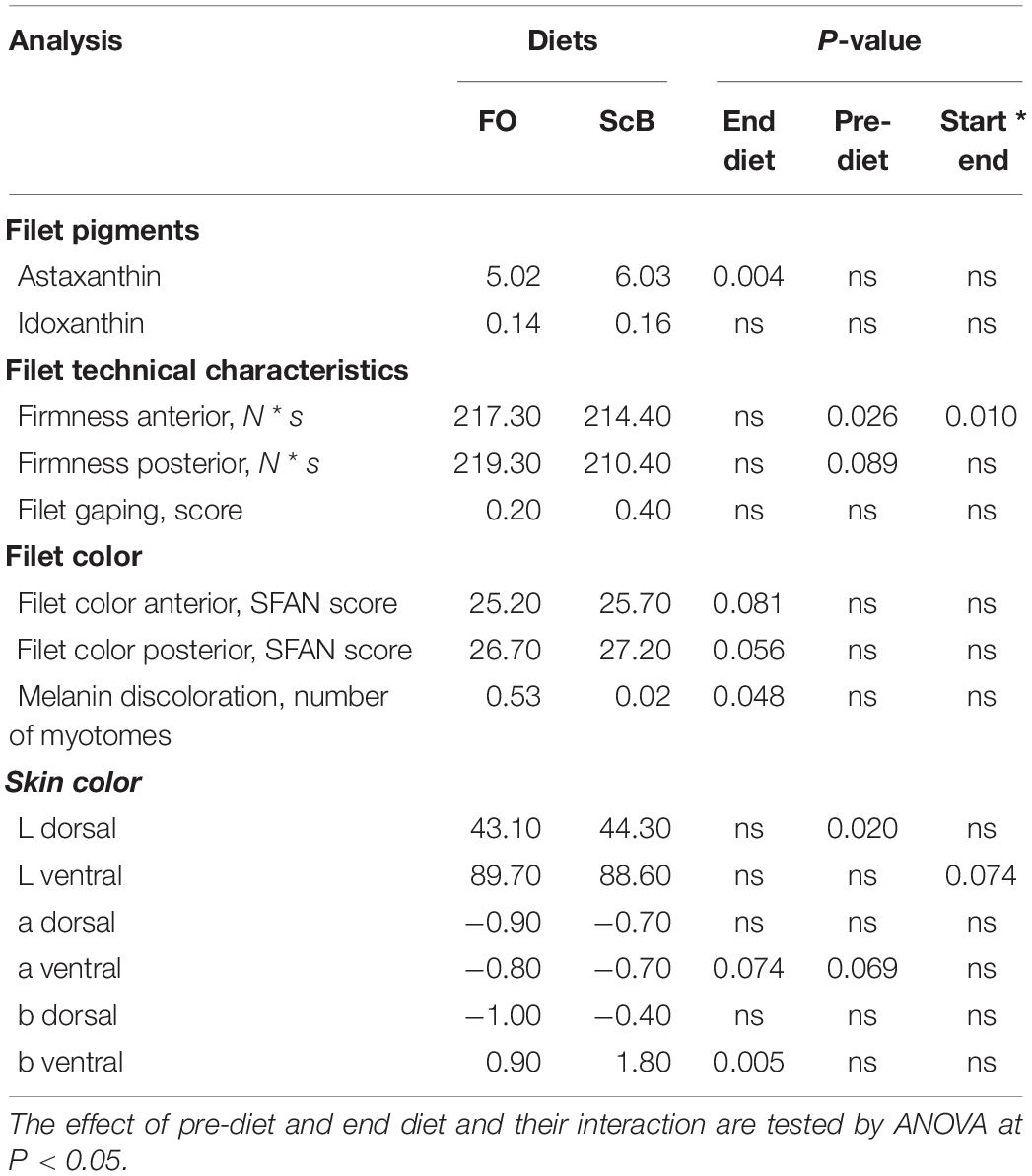
Table 8. Filet astaxanthin and idoxanthin levels (mg/kg), firmness (N * s), gaping- and color score, and colorimetric evaluation of skin coloration of A. salmon fed life-long different pre-diets and end-diets supplemented with either fish oil (FO) or Schizochytrium limacinum biomass (ScB) as n-3 LC-PUFA source.
Sensory Analysis
Parr-Post Smolt – 0.8 kg Mean Body Weight Atlantic Salmon
The results from the sensory evaluation of A. salmon post smolt filets of 0.8 kg approximate body weight at the end of tank phase and beginning of cage trial phase showed that there were some significant differences in coloration (hue) between the different feeding treatments. Fish that were fed diets supplemented with ScB had more red filets (P < 0.05) stronger coloration (P < 0.01) and had lower whiteness (P < 0.05) compared to the fish fed diets with either only FO or a mix of FO and ScB as n-3 LC-PUFA source in the diet (Figure 1).
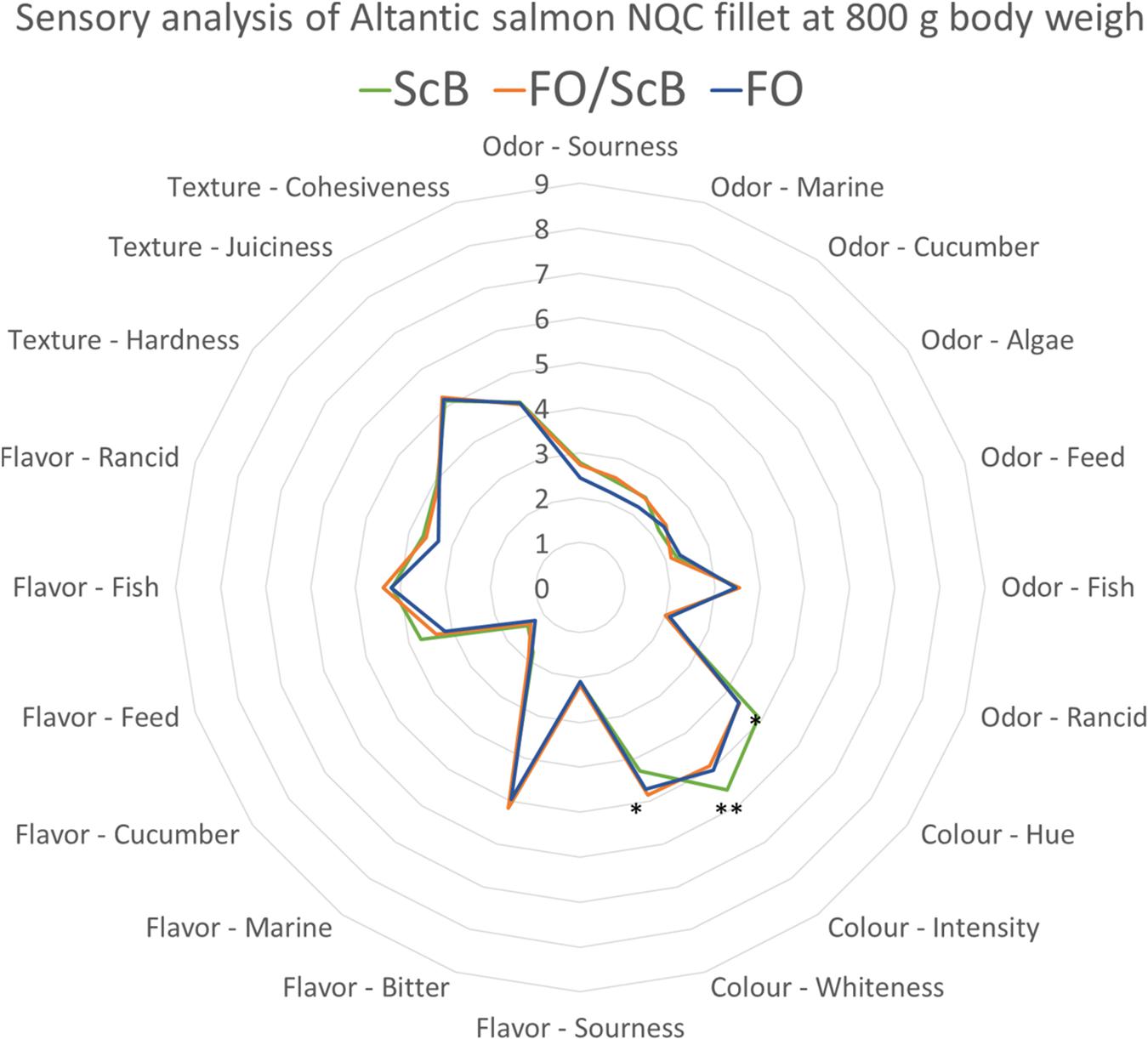
Figure 1. Sensory analysis. Parr-post smolt – 0.8 kg mean body weight Atlantic salmon. Comparisons of mean values (Tukey’s test) of evaluation on Atlantic salmon raw filet samples for odor and color and heated (cooked) filets for taste, flavor, and texture at 800 g mean body weight (∗P < 0.05; ∗∗P < 0.01).
Slaughter – 4.15 kg Mean Body Weight Atlantic Salmon
Similar dietary effects as early in the trial were also observed at final sampling where the ScB feeding regime gave filets with redder coloration compared to FO. Moreover, FO gave samples which were softer and juicier compared to ScB (Figure 2).
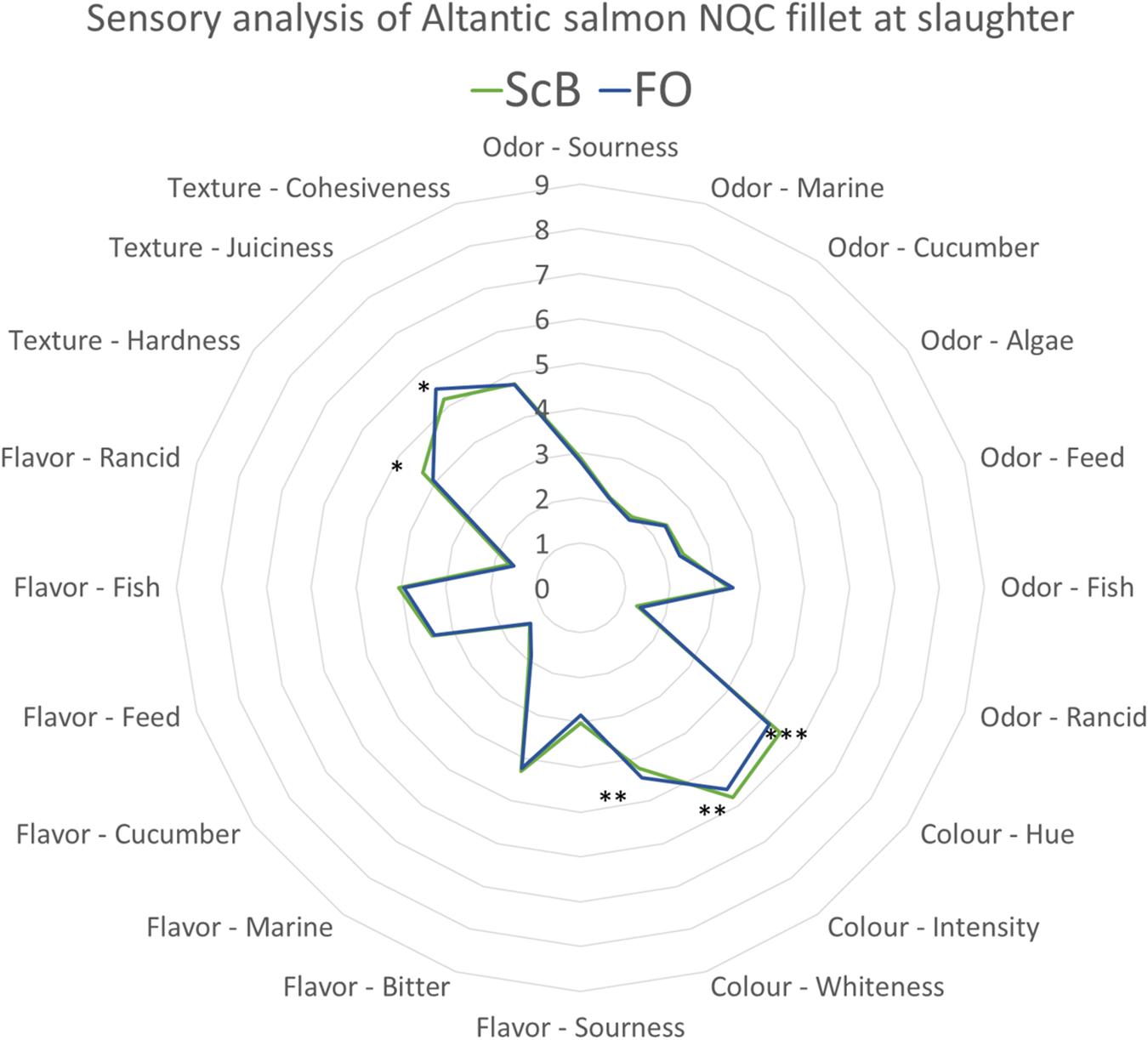
Figure 2. Sensory analysis. Effect of end diets and pre-feeding at slaughter – 4.15 kg mean body weight Atlantic salmon. Comparisons of mean values (Tukey’s test) of evaluation on Atlantic salmon raw filet samples for odor and color and heated (cooked) filets for taste, flavor, and texture at slaughter (∗P < 0.05; ∗∗P < 0.01; ∗∗∗P < 0.001).
There were no significant effects identified on the sensory quality of the filets from pre-feeding within fish fed the FO and ScB during the cage phase.
Effect of Pre-feeding on Cage Level – 4.15 kg Mean Body Weight Atlantic Salmon
Principal component analysis was used to identify similarities and differences between the sensory attributes and the 18 different treatments (six cages × three treatments per cage) (Figure 3). The first and second principal component (PCs) explained 42 and 17% of the observed variation, respectively. The biplot (scores and loadings) showed what we have seen from other results, namely that pre-feeding has had minor effects on the end sensory quality of the filets. Pre-diet samples are spread all over in the figure, whereas samples from ScB end diet (marked as bold) are positioned above or just under the middle line, correlating on PC2 with color intensity, hue, and hardness, and samples from FO end diet were positioned from the middle to the lower side of PC2 correlating with juiciness and whiteness.
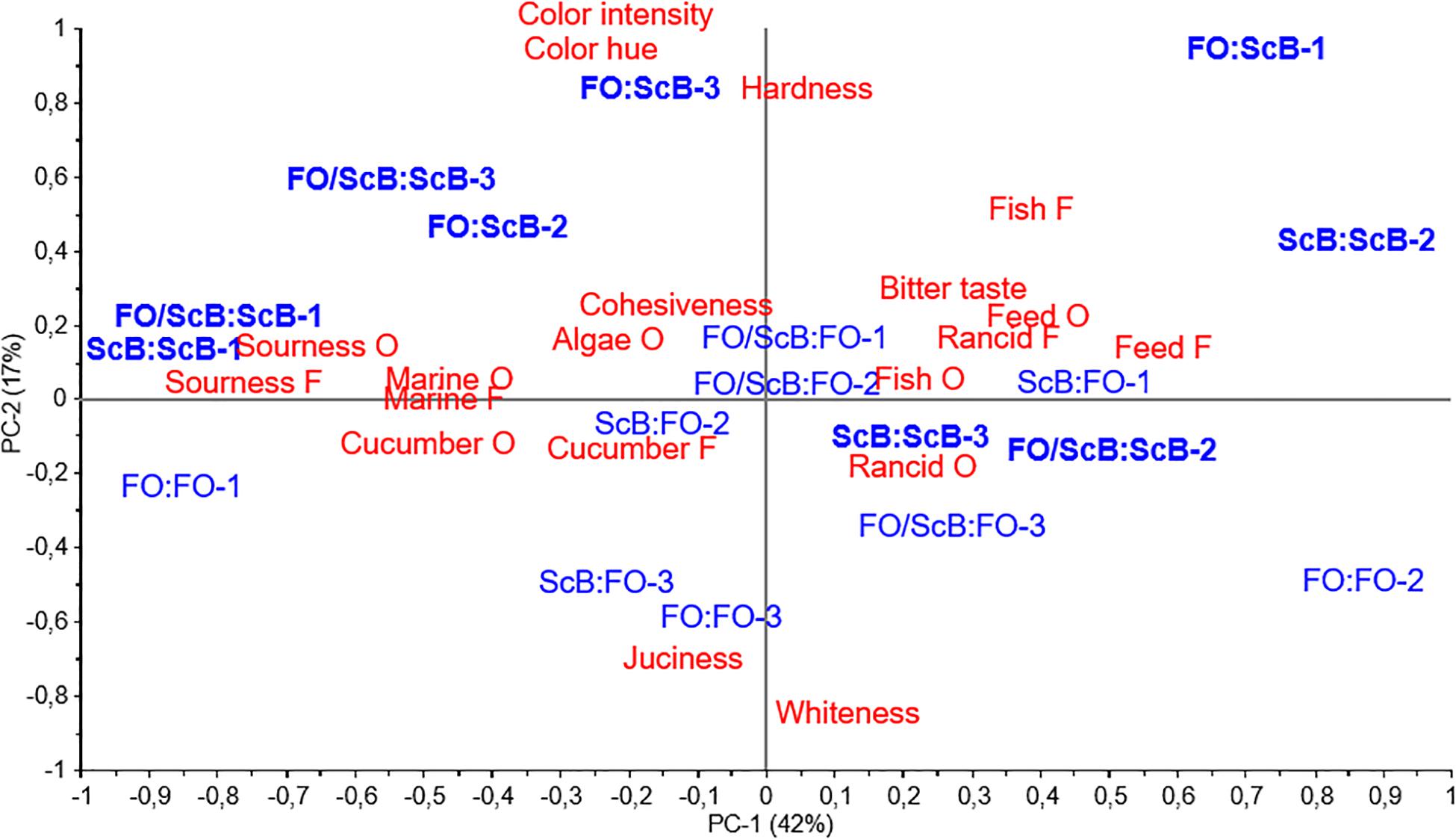
Figure 3. Sensory analysis. Effect of pre-feeding on cage level – 4.15 kg mean body weight Atlantic salmon. Principal component analysis biplot (scores and loadings) and the effect of pre-diet (FO, FO/ScB, or AcB) and end diet (FO or ScB) on Atlantic salmon filet flavors (F) and odors (O).
Microarray Analyses of Liver and Mid Intestine
Microarray (transcriptome) analysis was realized in liver and mid intestine tissues from fish in the FO:FO and ScB:ScB dietary treatments. Only a few genes were differentially expressed in the liver of the two treatments, but some of those are worth mentioning. For instance, significant upregulation was found of the hsp (heat shock protein), gpx (glutathione peroxidase), and gadd45 (growth arrest and DNA-damage-inducible protein) genes in the ScB compared to the FO group. More immune system-related genes were downregulated in the liver and mid intestine in the ScB compared to the FO groups (Table 9). There were just a few differences in gene expression between the two dietary groups in mid intestine (Table 10). It is worth mentioning that in the mid intestine of fish from the ScB group there was upregulation of several genes involved in xenobiotic metabolism, whereas there was also seen upregulation of a zinc transporter. Downregulation of some few more genes related to inflammatory response in the intestine, such as chemokine/cytokines, was also observed in the ScB group.
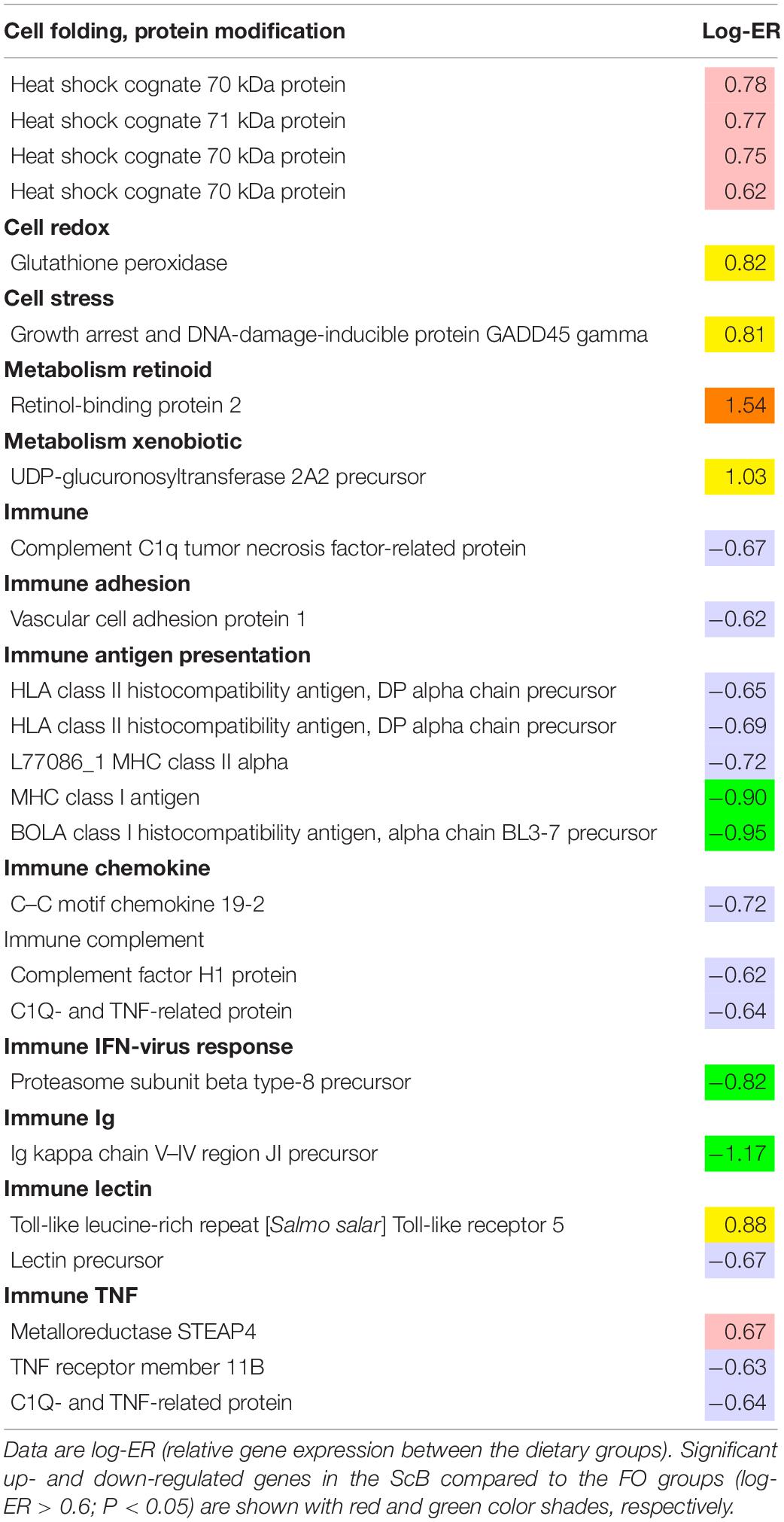
Table 9. Microarray analysis results showing genes with expression change in the liver of salmon fed ScB compared to the FO diet (n = 12).
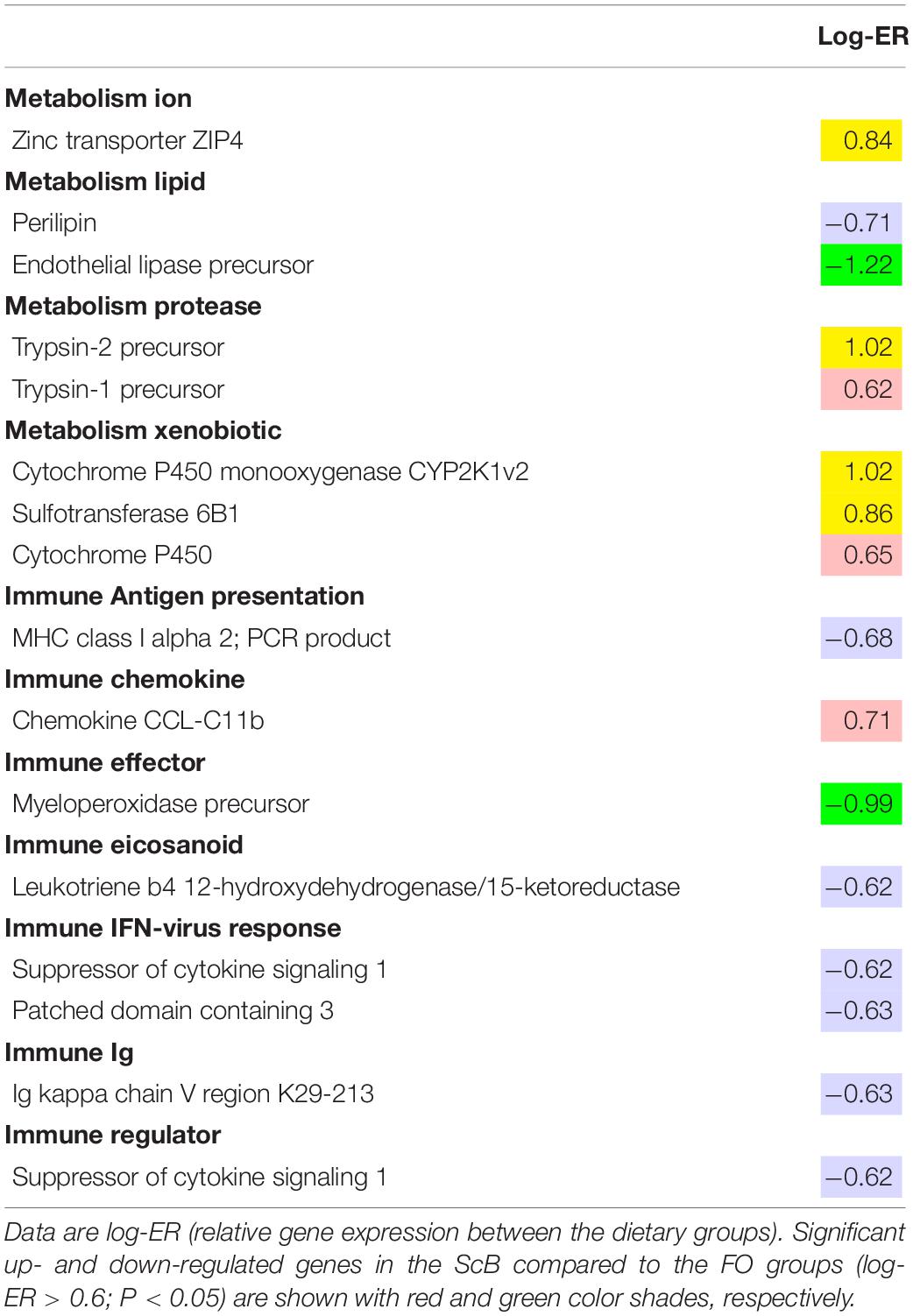
Table 10. Microarray analysis results showing genes with expression change in the mid intestine of salmon fed ScB compared to the FO diet (n = 12).
Histological Evaluation of Intestinal Tissues
Hind Intestine
No diet-related differences were found in the histology of hind intestine between fish from FO:FO and ScB:ScB groups at the end of the trial. However, the hind guts of all 24 fish studied were judged to be abnormal (Figure 4) compared to normal hind gut histology in A. salmon (e.g., as in Knudsen et al., 2007). The intestinal folds were hyperplastic, abnormally tall, and large with extensively developed branches, which locally coalesced into networks. Side-branches were observed also in the simple folds, which should normally have none. The enterocytes lacked the vacuolization which is normal for mucosa in this region. In the center of the folds, the normally delicate strands of connective tissue were replaced by thick layers of connective tissue, and there was a prominent infiltration of inflammatory cells in connective tissue, both within and below the intestinal folds. These deviations were consistent throughout the material, although some variations were present, in particular in the degree of elongation and branching of folds as well as in cell infiltration.
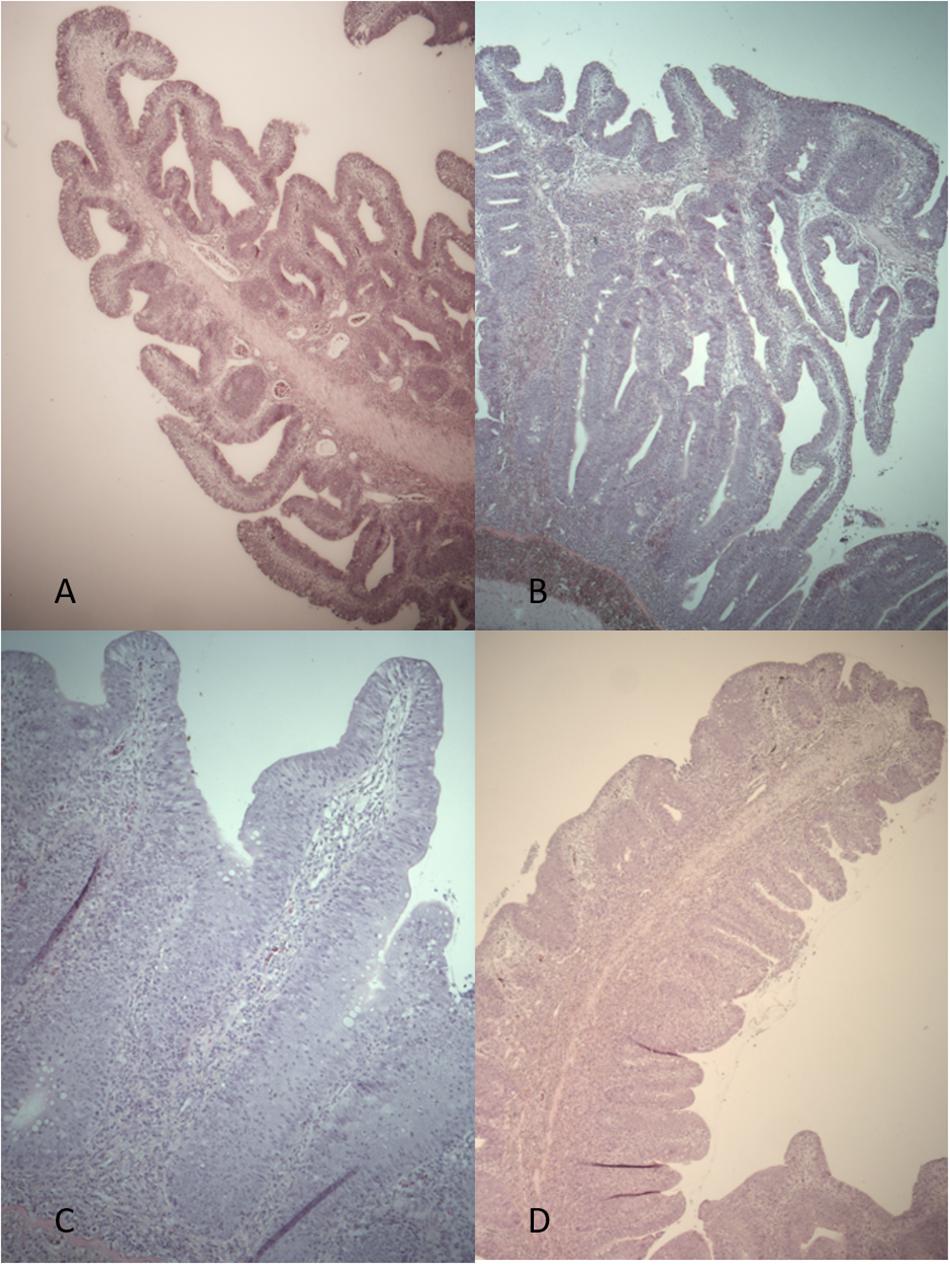
Figure 4. Histological evaluation of intestinal tissues. Hind intestine. Histological sections of hind intestine in FO (A) and ScB (B) fish and in the ScB dietary group with high cell infiltration at two magnifications: Single (C) and complex folds (D).
Mid Intestine
The mid intestine was found normal in all 24 fish observed (Figure 5). Mucosal folds were of regular heights, and the enterocytes appeared normal and well developed. Some variation was observed, e.g., in the number of goblet cells, but all were considered to be within normal range. In particular, there was no sign of any difference in tissue morphology between the two dietary groups.
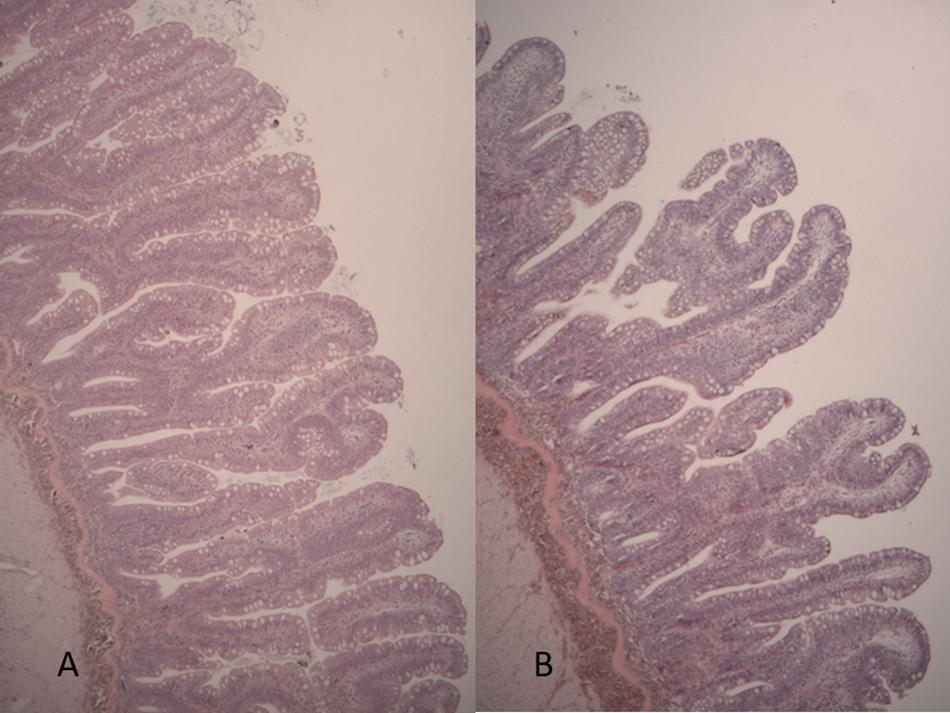
Figure 5. Histological evaluation of intestinal tissues. Mid intestine. Histological sections of mid intestine in FO (A) and ScB (B) fish.
Discussion
The main findings of this study are related to the: (a) significantly positive effect on fish growth and ADC by the ScB diet during the sea phase of the trial compared to the FO treatment, (b) differential dietary physiological effects on body lipid fatty acid composition, liver composition, and microarray and intestine microarray, and (c) positive ScB effect on Atlantic salmon filet coloration.
Atlantic Salmon Performance Replacing Dietary Fish Oil by Schizochytrium limacinum Biomass
The experimental design included replacement of supplemental dietary FO with S. limacinum biomass to achieve equal levels of total EPA + DHA in the diet. Due to the high DHA and very low level of EPA in S. limacinum biomass, the experimental diets had very different DHA/EPA ratio (0.69 in FO and 14.73 in the ScB diet – cage phase). Further formulation adjustments included balancing: (a) total dietary SFA levels, n-3/n-6 ratio, and digestible energy, by adjustments in the inclusion level of different plant oils, and (b) macronutrients by modifications in the inclusion levels of dietary binders. We chose a total dietary level of EPA + DHA as low as 1.25% in the diet during the sea cage phase, which was recently shown to be too low for farming Atlantic salmon robust enough to deal with very stressful conditions (Bou et al., 2017a). The experimental groups revealed growth performance differences only during the last phase of the study, namely during the sea cage phase, when ScB fish reached significantly higher final body weight compared to the FO fish. DHA, which ScB diet was richer of, compared to FO, is reported to be more essential than EPA in some fish species, in particular at high growth larval stages (Watanabe et al., 1989; Takeuchi et al., 1990; Copeman et al., 2002). Moreover, improved growth and non-specific immunity immune-related gene expression and disease resistance following natural infestation of parasites was reported in juvenile large yellow croaker (Larmichthys crocea) fed diets with moderately higher DHA/EPA levels (Zuo et al., 2012). In our study the best performing diet (ScB) had much higher DHA/EPA ratio and we did not see any significant differences in total mortalities or fish robustness (histology and microarray data). Improved growth was observed also in farmed Tilapia fed diets where FO was replaced by S. limacinum biomass, however, unlike in our present study, dietary EPA + DHA levels were not balanced and increased with increasing levels of S. limacinum supplementation (Sarker et al., 2016). Other than salmon lice infestation and treatment-related mortalities, none of the experimental populations suffered from any other disease during the experimental period, and fish survival was identical in both FO and ScB groups. Thus, improved growth in the ScB treatment appears to be related to metabolic and behavioral effects leading to higher feed intake rates. In ScB diets we used whole cell S. limacinum biomass, which in contrast with extracted oils and plant concentrates, is expected to contain a great number of functional components. Although diets were balanced to cover the known fish’s basic needs, additional contributions of nucleotides, minerals, phospholipids, vitamins, and cell wall components from S. limacinum biomass may have contributed to improved intestine function during the most stressful growing period for the fish, which included several handling incidences for the removal of lice. Handling of fish may result in periods of low dissolved oxygen (DO) levels which may reduce the intestinal barrier function of Atlantic salmon, as for instance measured by reduced electrical resistance and increased paracellular permeability in the distal region of the fish’s intestine in the study by Sund et al. (2010). The hypothesis of improved intestine function in the ScB groups is also supported by the observed increased ADC of dietary nutrients in this treatment, as also found in mammals fed other unicellular organism based raw materials such as yeast culture in the diet for pigs (Shen et al., 2009). Such unicellular biomass components are commonly used as dietary supplements for farmed animals at rather low inclusion levels (pro- and pre-biotics) and expected to improve intestine health (Anderson et al., 1999) both in terms of morphology (van der Peet-Schwering et al., 2007) and microflora composition (van Heugten et al., 2003). Accordingly, Lyons et al. (2016) found body growth improvement and greater level of microbial diversity in the microbial communities of rainbow trout (Oncorhynchus mykiss) fed low levels of S. limacinum in the diet compared to a control diet without, with significantly elevated bacterial taxa belonging to the genera Streptococcus, Leuconostoc, Lactobacillus, Lactococcus, and Weissella. This is also in accordance with our previous study where we saw growth improvement in Atlantic salmon post smolt by lower than in the present study dietary supplementation of S. limacinum biomass combined with yeast extract (1% in the diet) (Kousoulaki et al., 2015). However, little experience and evidences exist on intestine microbiome effects of higher life-long dietary inclusion levels of S. limacinum biomass, comparable to those used in this study. High inclusion levels of S. limacinum biomass combined with yeast extract induced a strong promotion of goblet cell, iNOS, and F-actin production in the intestinal epithelium of Atlantic salmon, compared to the FO control diet, suggesting immune system reaction without any histological evidence of enteritis or any other negative physiological effects (Kousoulaki et al., 2015).
Assimilation of Fatty Acids in Whole Fish and Filet
Atlantic salmon filet lipid fatty acid composition mostly reflected the composition of the experimental diets. Nevertheless, though the diets were balanced for total SFA and EPA + DHA, at slaughter FO fish had higher levels of SFA and lower levels EPA + DHA compared to the ScB fish. As recently identified, S. limacinum biomass contains high levels tripalmitin (16:0/16:0/16:0) with high melting point (Bogevik et al., 2018) and low digestibility, explaining the differences in the whole-body SFA levels and retention efficiency of the dietary SFA. Early in the trial both dietary DHA and EPA were slightly more digestible in the FO diet, and at slaughter ADC of EPA but not that of DHA had higher ADC compared to that of the ScB diet. In terms of total retention efficiency FO-diet fed fish had much lower retention efficiency of EPA and higher DHA compared to ScB-fed fish. The FO diet had a DHA/EPA ratio of 0.69 against 14.73 in the ScB diet which would stimulate elongation and desaturation of EPA to DHA to ensure adequate amounts of DHA in the membranes of vital tissues of the fish (Glencross et al., 2014; Kuah et al., 2015).
During all the trial, ScB fish had higher levels of EPA + DHA in their filets compared to FO fish. This may be explained by breakdown of the more available EPA in the FO diet and preservation of the more plentiful DHA in the ScB fish (Tocher, 2003). This effect may have been more evident during the stressful period in sea cages where EPA with anti-inflammatory action is consumed whereas DHA with more structural function in cell membranes is retained, even more in ScB fish which exhibited higher ADC of DHA, and higher body growth compared to FO fish. Xu et al. (2014) also reported in the Japanese sea bass (Lateolabrax japonicus) increased tissue levels of n-3 LC-PUFA and increased gene expression of Δ6 fatty acyl desaturases (FADS2) when fish were fed diets rich in palmitic acid in the form of tripalmitin, compared to diets rich in either n-3 LC-PUFA, stearic, oleic, or ALA. It is generally common to see inhibition of ALA conversion to EPA and reduced retention of EPA in fish whole body by increasing dietary DHA levels (e.g., Bou et al., 2017b). Some retro-conversion of DHA to EPA may also occur in Atlantic salmon, which is nevertheless found to be rather limited, though increasing with essential fatty acid deficiency (Ruyter and Thomassen, 1999). Our data may thus indicate that the chosen dietary levels of EPA + DHA in this study were low. There are also indications that retroconversion of supplemented DHA to EPA occurs in humans, rats, and cell cultures but as reviewed by Metherel and Bazinet (2019) it is more likely that the reason behind this response is a feedback inhibition of EPA elongation to DPA n-3 (e.g., Metherel et al., 2017).
Dietary Schizochytrium limacinum Biomass Effects on Atlantic Salmon Filet Color
Dietary S. limacinum biomass provided during the whole study a dose-related positive effect on Atlantic salmon filet pigmentation. Those effects were demonstrated in terms of increased redness, reduced paleness, and reduced melanin spots in the filets of the fish at slaughter. Intestinal uptake facilitation and antioxidant effect provided by the whole unicellular organism’s components, such as vitamins, organic Se, and DHA, may have contributed to these effects (Kanazawa, 1991). Bjerkeng et al. (1999) found a small positive effect on Atlantic salmon filet pigmentation (up to 14% increased deposition efficiency of astaxanthin) by dietary supplementation of ɑ-tocopheryl acetate (vitamin E acetate). Likewise, in a study with turbot larvae, normal pigmentation was positively correlated with DHA/EPA ratio in live feed lipids and the authors concluded that DHA requirement for this purpose could not be replaced by EPA (Reitan et al., 1994). Assimilation of astaxanthin in salmon is rather poor increasing the need for dietary supplementation, which in turn increases significantly the formulation costs (Torrissen, 1995). Improved deposition of the supplemented astaxanthin by use of S. limacinum biomass in the diet, in combination with the observed positive effect on growth, may render the use of this natural alternative source of marine omega 3 fatty acids economically viable. When it comes to melanin discoloration, Mørkøre et al. (2015) reported that every fifth commercially produced salmon has black spots which occur early in the seawater phase increasing in prevalence and size toward slaughter. In the aforementioned report, melanin spots were associated with among other chronic inflammation and increased levels of minerals and microorganisms, which may have been alleviated by the use of high levels of S. limacinum biomass acting as prebiotic in the diet of the ScB fish groups (Komiyama et al., 2011).
Liver and Mid Intestine Transcriptome
Transcriptome analyses are a useful tool to widely identify potential health effects. The observed upregulation of the hsp, gpx, and gadd45 genes in the liver of ScB compared to the FO fish, in combination with the high incidence of pale livers, higher total lipid level, increased relative level of 18:1 n-9, and low EPA (EPA is known to have anti-inflammatory effects) in the liver of the ScB group may be an indication of mild stress in the liver of ScB fish. Even though we cannot from this study conclude with certainty, potential mild stress in the liver may have partly been due to specific lack of EPA in the ScB treatment. The Schizochytrium biomass rich diet was characterized by high DHA/EPA ratio. When the present trial was initiated it was considered that 1–1.3% EPA + DHA was adequate to cover salmon’s requirement (Ruyter et al., 2000). Nevertheless, a later study by Bou et al. (2017a) shows that 1% EPA of DHA in the diet long-term results in increased mortality under challenging conditions, as well as increased incidence of hypervacuolization in the intestine and fatty liver which indicates that the requirement for these fatty acids is higher than what it was assumed to be earlier. The experimental fish were fed diets with ca. 1% EPA + DHA early on in the fresh water, and 1.3% EPA + DHA in the sea water phase. Higher growth in the ScB compared to the FO groups can also be the reason why signs of potential EPA deficiency first appeared in the ScB groups. The authors have no adequate explanation for the differential expression of other immune system-related genes which were also downregulated in the liver and mid intestine in the ScB compared to the FO group. More studies have shown that feeding zebrafish microalgae rich diets can lead to gene expression changes in the liver. Microalgae supplements containing a species rich in arachidonic acid (ARA) lead to upregulation of immune system-related genes and antioxidant defense (Nayak et al., 2018) in contrast to our study where we saw mostly downregulation. EPA and DHA are known to have anti-inflammatory effects, whereas ARA is known to be pro-inflammatory, which can be part of the explanation for the differential responses. The fact that only a few differences were identified in gene expression between the two dietary groups in mid intestine agrees in general with the fact that mid intestine in both groups was found to be normal through histological evaluation.
Regarding the observed upregulation of a zinc transporter gene in the fish from the ScB group, recent unpublished results (FHF project no. 901265) also show that increased levels of EPA and DHA in the feed lead to increased levels of Zn in the intestinal tissue which indicates better Zn uptake. It appears thus that in our study higher level of DHA from the Schizochytrium biomass in the diet may have caused upregulation of the ZIP4 Zn transporter. More animal model studies as well as studies in human cell cultures has shown a potential relation between omega-3-fatty acids, zinc transporter levels, and free zinc availability in cell level (review from De Mel and Suphioglu, 2014).
The observed downregulation of other genes related to inflammatory response in the intestine, such as chemokine/cytokines may also indicate potential anti-inflammatory effects in the mid-intestine of the ScB compared to the FO dietary groups. Mammalian studies refer to observations of anti-inflammatory effects of beta-glucans in the intestine (Raa, 2015) which are compounds present in the cell walls of unicellular organisms such as yeast and microalgae.
Intestinal Histology
No diet-related differences in intestinal histology were observed between the ScB and FO dietary groups, indicating no specific adverse effect on intestinal development in relation to long-term feeding of ScB compared to FO. Mid-intestinal mucosa displayed no signs of abnormal vacuolization, as was previously observed in A. salmon fed diets which were low in EPA + DHA (Bou et al., 2017a). In distal intestine, the observations painted a more complicated picture, although it is important to note that there were no observations indicating differences between the dietary groups. The fact remains that all samples displayed a deviant morphology, with hyperplastic intestinal folds and non-vacuolized mucosa. Despite these deviations, the tissues were seemingly viable and functional, as judged by fish performance. This unknown condition had no apparent likeness to the intestinal inflammation associated with soybean meal-induced enteritis (Knudsen et al., 2007). Although having some common features, the hyperplastic growth of the mucosal folds in the present study contrasts the markedly reduced development of these structures in the soybean meal-associated condition. The cause of the deviant state of the distal intestinal mucosa in the present study remains unknown.
Conclusion
Schizochytrium limacinum biomass appears to be a good alternative source of marine omega 3 fatty acids for the fish in our study, in particular during stressful conditions, resulting in improved growth, indications of anti-inflammatory effects in the intestine based on gene expression data, and improved filet pigmentation tested both chemically and by sensory panel. However, it is important to cover the fish’s requirement for total LCn-3HUFA, and in the present study there were indications that the dietary levels of EPA + DHA used (ca. 1.3%) were inadequate for both groups, but in particular for the faster growing S. limacinum dietary group showing increased liver fat content. It appears that S. limacinum biomass can be used in the feed for salmon throughout the whole production cycle from parr to slaughter without any negative effects on growth or product quality if the dietary requirement for EPA is covered. Lastly, the life-long use of S. limacinum biomass affects texture but not the taste or smell of the salmon filet.
Data Availability Statement
The microarray data are available at GEO accession GSE140558.
Ethics Statement
The four feeding experiments followed the Norwegian animal welfare act guidelines, in accordance with the Animal Welfare Act of 20 December 1974, amended 19 June 2009. The facility at Sunndalsøra, Nofima (division 60) was granted permission by the Norwegian Food Safety Authority (FOTS ID 8060) to run the experiments. The decision was made on the basis of Regulations 18 June 2015 on the use of animals in experiments.
Author Contributions
KK, MT, KA, CM, SJ, and RB: planning and preparing experiment. KK, GB, MT, YT, CM, and RB: participated in carrying out experiment. KK, GB, MT, KA, BG, YT, and CM: analysis. KK, GB, MT, KA, BG, YT, CM, SJ, and RB: writing and editing.
Funding
The fresh water phase of the current study was funded by Alltech Inc. (Strategic Research Alliance Alltech-Nofima, Project III), the salt water trials in tanks were funded by the Norwegian Research Council (Project Number: 234057, A to omega-3), and the sea cage trial was funded by the FHF and Salmon Group (Project Number: 901037, New omega-3 sources in salmon feed).
Conflict of Interest
The authors declare that the research was conducted in the absence of any commercial or financial relationships that could be construed as a potential conflict of interest.
Acknowledgments
The authors acknowledge the significant contributions of Nofima’s employees at the Feed Technology Center for the production of the experimental feeds; at the Research station at Sunndalsøra and Marine Harvest employees at Averøy for well-run fish feeding trials; and at Nofima laboratories for the realization of chemical analyses in fish, feeds, and raw materials.
Supplementary Material
The Supplementary Material for this article can be found online at: https://www.frontiersin.org/articles/10.3389/fmars.2020.00057/full#supplementary-material
FIGURE S1 | Atlantic salmon were kept in tanks and fed in triplicate groups from parr through smoltification and further on to body weight of ca. 800 g three diets, containing fish oil (FO), S. limacinum biomass (ScB) or a mix of the two as EPA + DHA source. Fish were then mixed and moved to sea cages where there were fed in two triplicate groups either FO or ScB to slaughter.
References
Aas, T. S., Ytrestøyl, T., and Åsgård, T. (2019). Utilization of feed resources in the production of Atlantic salmon (Salmo salar) in Norway: an update for. 2016. Aquac. Rep. 2019:100216. doi: 10.1016/j.aqrep.2019.100216
Anderson, D. B., McCracken, V. J., Aminov, R. I., Simpson, J. M., Mackie, R. I., Verstegen, M. W. A., et al. (1999). Intestine microbiology and growth-promoting antibiotics in swine. Pig News Inform. 20, 115–122.
Barclay, W., and Zeller, S. (1996). Nutritional enhancement of n-3 and n-6 fatty acids in rotifers and Artemia nauplii by feeding spray-dried Schizochytrium sp. J. World Aquac. Soc. 27, 314–322. doi: 10.1111/j.1749-7345.1996.tb00614.x
Barclay, W. R. (1994a). Food product having high concentrations of omega-3 highly unsaturated fatty acids. US Pat 5:594.
Barclay, W. R. (1994b). Process for growing Thraustochytrium and Schizochytrium using non-chloride salts to produce a micro-floral biomass having omega-3 highly unsaturated fatty acids. US Pat. 5:742.
Barclay, W. R., Weaver, C., and Metz, J. (2005). “Development of a docosahexaenoic acid production technology using Schizochytrium: a historical perspective,” in Single cell oil, eds Z. Cohen and C. Ratledge, (Champaign, IL: AOCS).
Bjerkeng, B., Følling, M., Lagocki, S., Storebakken, T., Olli, J. J., and Alsted, N. (1997). Bioavailability of all-E-astaxanthin and Z-isomers of astaxanthin in rainbow trout (Oncorhynchus mykiss). Aquaculture 157, 63–82. doi: 10.1016/S0044-8486(97)00146-4
Bjerkeng, B., Hamre, K., Hatlen, B., and Wathne, E. (1999). Astaxanthin deposition in fillets of Atlantic salmon Salmo salar L. fed two dietary levels of astaxanthin in combination with three levels of α-tochopheryl acetate. Aquac. Res. 30, 637–646. doi: 10.1046/j.1365-2109.1999.00355.x
Bligh, E. G., and Dyer, W. J. (1959). A rapid method of total lipid extraction and purification. Can. J. Biochem. Phys. 37, 911–917.
Bogevik, A. S., Nygren, H., Balle, T., Haugsgjerd, B. O., and Kousoulaki, K. (2018). Enzymatic interesterification of heterotrophic microalgal oil with rapeseed oil to decrease the levels of tripalmitin. Eur. J. Lipid Sci. Technol. 2018:10.
Bou, M., Berge, G. M., Baeverfjord, G., Sigholt, T., Østbye, T.-K., Romarheim, O. H., et al. (2017b). Requirements of n-3 very long-chain PUFA in Atlantic salmon (Salmo salar L): effects of different dietary levels of EPA and DHA on fish performance and tissue composition and integrity. Br. J. Nutri. 117, 30–47. doi: 10.1017/S0007114516004396
Bou, M., Berge, G. M., Baeverfjord, G., Sigholt, T., Østbye, T.-K., and Ruyter, B. (2017a). Low levels of very-long-chain n-3 pufa in Atlantic salmon (Salmo salar) diet reduce fish robustness under challenging conditions in sea cages. J. Nutrit. Sci. 6:e32. doi: 10.1017/jns.2017.28
Bowles, R. D., Hunt, A. E., Bremer, G. B., Duchars, M. G., and Eaton, R. A. (1999). Long-chain n-3 polyunsaturated fatty acid production by members of the marine protistan group the thraustochytrids: screening of isolates and optimization of docosahexaenoic acid production. Progr. Ind. Microbiol. 35, 193–202. doi: 10.1016/s0168-1656(99)00072-3
Britton, G. (1995). Structure and properties of carotenoids in relation to function. Fed. Am. Soci. Exp. Biol. J. 9, 1551–1558. doi: 10.1096/fasebj.9.15.8529834
Carter, C. G., Bransden, M. P., Lewis, T. E., and Nichols, P. D. (2003). Potential of Thraustochytrids to partially replace fish oil in Atlantic salmon feeds. Mar. Biotechnol. 5, 480–492. doi: 10.1007/s10126-002-0096-8
Cho, C. Y. (1992). Feeding systems for rainbow trout and other salmonids with reference to current estimates of energy and protein requirements. Aquaculture 100, 107–123. doi: 10.1016/0044-8486(92)90353-m
Copeman, L. A., Parrish, C. C., and Harel, M. (2002). Effects of docosahexaenoic, eicosapentaenoic, and arachidonic acids on the early growth, survival, lipid composition and pigmentation of yellowtail flounder (Limanda ferruginea): a live food enrichment experiment. Aquaculture 210, 285–304. doi: 10.1016/s0044-8486(01)00849-3
De Mel, D., and Suphioglu, C. (2014). Fishy business: effect of omega-3 fatty acids on zinc transporters and free zinc availability in human neuronal cells. Nutrients 6, 3245–3258. doi: 10.3390/nu6083245
Ganuza, E., Benitez-Santana, T., Atalah, E., Vega-Orellana, O., Ganga, R., and Izquierdo, M. S. (2008). Crypthecodinium cohnii and Schizochytrium sp. as potential substitutes to fisheries-derived oils from seabream (Sparus aurata) microdiets. Aquaculture 277, 109–116. doi: 10.1016/j.aquaculture.2008.02.005
Glencross, B. D., Tocher, D. R., Matthew, C., and Bell, J. G. (2014). Interactions between dietary docosahexaenoic acid and other long-chain polyunsaturated fatty acids on performance and fatty acid retention in post-smolt Atlantic salmon (Salmo salar). Fish Physiol. Biochem. 40, 1213–1227. doi: 10.1007/s10695-014-9917-8
Hilborn, R., Branco, T., Ernst, B., Magnusson, A., Minte-Vera, C. V., Scheuerell, M. D., et al. (2003). State of the world’s fisheries. Annu. Rev. f Environ. Resour. 28, 359–399.
ISO 5983 (1997). Animal Feeding Stuffs — Determination of Nitrogen Content and Calculation of Crude Protein Content – Kjeldahl Method. Geneva: ISO.
ISO 6496 (1999). Animal Feeding Stuffs — Determination of Moisture and Other Volatile Matter Content. Geneva: ISO.
ISO 13229 (2016). Sensory Analysis - General Guidance for Establishing a Sensory Profile. Geneva: International Organization for Standardization.
ISO 8586 (2012). Sensory Analysis - General Guidance for Selection, Training and Monitoring of Selected Sensory Assessors and Expert Assessors. Geneva: International Organization for Standardization.
ISO 8589 (2007). Sensory Analysis - General Guidance for the Design of Test Rooms. Geneva: International Organization for Standardization.
Kanazawa, A. (1991). “Nutritional mechanisms causing abnormal pigmentation in cultured marbled sole larvae, Limanda yokohamae (Heterosomata),” in Larvi’91 — Fish & Crustacean Larviculture Symposium, eds P. Lavens, P. Sorgeloos, E. Jaspers, and F. Ollevier, (Gent: Special Publication 15, European Aquaculture Society), 20–22.
Knudsen, D., Urán, P., Arnous, A., Koppe, W., and Frøkiær, H. (2007). Saponin-containing subfractions of soybean molasses induce enteritis in the distal intestine of atlantic salmon. J. Agric. Food Chem. 55, 2261–2267. doi: 10.1021/jf0626967
Komiyama, Y., Andoh, A., Fujiwara, D., Ohmae, H., Araki, Y., Fujiyama, Y., et al. (2011). New prebiotics from rice bran ameliorate inflammation in murine colitis models through the modulation of intestinal homeostasis and the mucosal immune system. Scand. J. Gastroenterol. 46, 40–52. doi: 10.3109/00365521.2010.513062
Kousoulaki, K., Mørkøre, T., Nengas, L., Berge, R. K., and Sweetman, J. (2016). Microalgae and organic minerals affect lipid retention efficiency and fillet quality in Atlantic salmon (Salmo salar L.). Aquaculture 451, 47–57. doi: 10.1016/j.aquaculture.2015.08.027
Kousoulaki, K., Østbye, T.-K. K., Krasnov, A., Torgersen, J. S., Mørkøre, T., and Sweetman, J. (2015). Metabolism, health and fillet nutritional quality in Atlantic salmon (Salmo salar) fed diets containing n-3-rich microalgae. J. Nutr. Scie.4:e 24. doi: 10.1017/jns.2015.14
Krasnov, A., Timmerhaus, G., Afanasyev, S., and Jørgensen, S. M. (2011). Development and assessment of oligonucleotide microarrays for Atlantic salmon (Salmo salar L.). Comp. Biochem. Physiol. Part D Genomics Proteomics 6, 31–38. doi: 10.1016/j.cbd.2010.04.006
Kuah, M.-K., Jaya-Ram, A., and Shu-Chien, A. C. (2015). The capacity for long-chain polyunsaturated fatty acid synthesis in a carnivorous vertebrate: Functional characterisation and nutritional regulation of a Fads2 fatty acyl desaturase with Δ4 activity and Elov5 elongase in striped snakehead (Channa striata). Biochim. Biophys. Acta -Mol. Cell Biol. Lipids 1851, 248–260. doi: 10.1016/j.bbalip.2014.12.012
Lawless, H. T., and Heymann, H. (2010). Sensory Evaluation of Food - Principles and Practices. Berlin: Springer.
Lyons, P. P., Turnbull, J. F., Dawson, K. A., and Crumlish, M. (2016). Effects of low-level dietary microalgae supplementation on the distal intestinal microbiome of farmed rainbow trout Oncorhynchus mykiss (Walbaum). Aquac. Res. 48, 2438–2452. doi: 10.1111/are.13080
Metherel, A. H., and Bazinet, R. P. (2019). Updates to the n-3 polyunsaturated fatty acid biosynthesis pathway: DHA synthesis rates, tetracosahexaenoic adid and (minimal) retroconversion. Progr. Lipid Res. 76:101008. doi: 10.1016/j.plipres.2019.101008
Metherel, A. H., Chouinard-Watkins, R., Trépanier, M.-O., Scott Lacombe, R. J., and Bazinet, R. P. (2017). Retroconversion is a minor contributor to increases in eicosapentaenoic acid following docosahexaenoic acid feeding as determined by compound specific isotope analysis in rat liver. Nutr. Metab 14:75. doi: 10.1186/s12986-017-0230-2
Miller, M., Nichols, P., and Carter, C. (2007). Replacement of fish oil with thraustochytrid Schizochytrium sp L oil in Atlantic salmon parr (Salmo salar L) diets. Comp. Biochem. Physiol. 148, 382–392. doi: 10.1016/j.cbpa.2007.05.018
Mørkøre, T., and Einen, O. (2003). Relating sensory and instrumental texture analyses of Atlantic salmon. J. Food Sci. 68, 1492–1497. doi: 10.1111/j.1365-2621.2003.tb09672.x
Mørkøre, T., Larsson, T., Kvellestad, A. S., Koppang, E. O., Åsli, M., Krasnov, A., et al. (2015). Mørke Flekker i laksefilet Kunnskapsstatus og Tiltak for å Begrense Omfanget.. Report 34/2015. Diamanten: Norwegian, 87.
Nakahara, T., Yokochi, T., Higashihara, T., Tanaka, S., Yaguchi, T., and Honda, D. (1996). Production of docosahexaenoic and docosapentaenoic acids by Schizochytrium sp isolated from Yap islands. J. Am. Oil Chem. Soc. 73, 1421–1426. doi: 10.1007/bf02523506
Nayak, S., Khozin-Goldberg, I., Cohen, G., and Zilberg, D. (2018). Dietary supplementation with ω6 lc-pufa-rich algae modulates zebrafish immune function and improves resistance to streptococcal infection. Front. Immunol. 9:1960. doi: 10.3389/fimmu.2018.01960
Pauly, D., Christensen, V., Guénette, S., Pitcher, T. J., Sumaila, U. R., Walters, C. J., et al. (2002). Towards sustainability in world fisheries. Nature 418, 689–695. doi: 10.1038/nature01017
Pellizzari, C., Krasnov, A., Afanasyev, S., Vitulo, N., Franch, R., Pegolo, S., et al. (2013). High mortality of juvenile gilthead sea bream (Sparus aurata) from photobacteriosis is associated with alternative macrophage activation and anti-inflammatory response: results of gene expression profiling of early responses in the head kidney. Fish Shellfish Immunol. 34, 1269–1278. doi: 10.1016/j.fsi.2013.02.007
Pike, I. H., and Barlow, S. M. (2003). Impact of fish farming on fish stocks. Fish Farmer 26, 14–16.
Raa, J. (2015). Immune modulation by non-digestible and non-absorbable beta-1, 3/1, 6-glucan. Microb Ecol. Health Dise. 26:27824.
Reitan, K. I., Rainuzzo, J., and Olsen, Y. (1994). Influence of lipid composition of live feed on growth, survival and pigmentation of turbot larvae. Aquac. Int. 2, 33–48. doi: 10.1007/bf00118531
Ren, L. J., Ji, X. J., Huang, H., Qu, L. A., Feng, Y., Tong, Q. Q., et al. (2010). Development of a stepwise aeration control strategy for efficient docosahexaenoic acid production by Schizochytrium sp. J. Appl. Microbiol. Biotechnol. 87, 1649–1656. doi: 10.1007/s00253-010-2639-7
Ruyter, B., Røsjø, C., Einen, O., and Thomassen, M. (2000). Essential fatty acids in Atlantic salmon: effects of increasing dietary doses of n-6 and n-3 fatty acids on growth, survival and fatty acid composition of liver, blood and carcass. Aquac. Nutr. 6, 119–127. doi: 10.1046/j.1365-2095.2000.00137.x
Ruyter, B., Sissener, N. H., Østbye, T.-K., Simon, C. J., Krasnov, A., Bou, M., et al. (2019). Omega-3 canola oil effectively replaces fish oil as a new safe dietary source of docosahexaenoic acid (DHA) in feed for juvenile Atlantic salmon. Br. J. Nutr. 11, 1–43.
Ruyter, B., and Thomassen, M. S. (1999). Metabolism of n-3 fatty acids in Atlantic salmon liver: stimulation by essential fatty acid deficiency. Lipids 34, 1167–1176. doi: 10.1007/s11745-999-0468-3
Samuelsen, T. A., Oterhals, Å, and Kousoulaki, K. (2018). High lipid microalgae (Schizochytrium sp.) inclusion as a sustainable source of n-3 long-chain PUFA in fish feed—Effects on the extrusion process and physical pellet quality. Anim. Feed Sci. Technol. 236, 14–28. doi: 10.1016/j.anifeedsci.2017.11.020
Sarker, P. K., Kapuscinski, A. J., Lanois, A. J., Livesey, E. D., Bernhard, K. P., and Coley, M. L. (2016). Towards sustainable aquafeeds: complete substitution of fish oil with marine microalga Schizochytrium sp. improves growth and fatty acid deposition in juvenile Nile tilapia (Oreochromis niloticus). PloS One 11:e0156684. doi: 10.1371/journal.pone.0156684
Shen, Y. B., Piao, X. S., Kim, S. W., Wang, L., Liu, P., Yoon, I., et al. (2009). Effects of yeast culture supplementation on growth performance, intestinal health, and immune response of nursery pigs. J. Anim. Sci. 87, 2614–2624. doi: 10.2527/jas.2008-1512
Sund, H., Kvamme, B. O., Fridell, F., Olsen, R. E., Ellis, T., Taranger, G. L., et al. (2010). Intestinal barrier function of Atlantic salmon (Salmo salar L.) post smolts is reduced by common sea cage environments and suggested as a possible physiological welfare indicator. BMC Physiol. 2010:22. doi: 10.1186/1472-6793-10-22
Takeuchi, T., Toyota, M., Satoh, S., and Watanabe, T. (1990). Requirement of juvenile red seabream Pagrus major for eicosapentaenoic and docosahexaenoic acids. Nippon Suisan Gakkaishi 56, 1263–1269. doi: 10.2331/suisan.56.1263
Tocher, D. R. (2003). Metabolism and functions of lipids and fatty acids in teleost fish. Rev. Fish. Sci. 11, 107–184. doi: 10.1080/713610925
Torrissen, O. J. (1995). Strategies for salmonid pigmentation. J. Appl. Ichthyol. 11, 276–281. doi: 10.1186/s12711-018-0394-x
van der Peet-Schwering, C. M. C., Jansman, A. J. M., Smidt, H., and Yoon, I. (2007). Effects of yeast culture on performance, intestine integrity, and blood cell composition of weaning pigs. J. Anim. Sci. 85, 3099–3109. doi: 10.2527/jas.2007-0110
van Heugten, E., Funderburke, D. W., and Dorton, K. L. (2003). Growth performance, nutrient digestibility, and fecal microflora in weaning pigs fed live yeast. J. Anim. Sci. 81, 1004–1012. doi: 10.2527/2003.8141004x
Watanabe, T., Izquierdo, M. S., Takeuchi, T., Satoh, S., and Kitajima, C. (1989). Comparison between eicosapentaenoic and docosahexaenoic acids in term of essential fatty acid efficacy in larval red seabream. Nippon Suisan Gakkaishi 55, 1635–1640. doi: 10.2331/suisan.55.1635
Xu, H., Dong, X., Ai, Q., Mai, K., Xu, W., Zhang, Y., et al.. (2014). Regulation of tissue LC-PUFA contents, D6 fatty acyl desaturase (FADS2) gene expression and the methylation of the putative FADS2 gene promoter by different dietary fatty acid profiles in Japanese seabass (Lateolabrax japonicus). PLoS One 9:e87726. doi: 10.1371/journal.pone.0087726
Ytrestøyl, T., Aas, T. S., and Åsgård, T. (2015). Utilisation of feed resources in production of Atlantic salmon (Salmo salar) in Norway. Aquaculture 448, 265–374.
Zuo, R., Ai, Q., Mai, K., Xu, W., Wang, J., Xu, H., et al. (2012). Effects of dietary docosahexaenoic to eicosapentaenoic acid ratio (DHA/EPA) on growth, nonspecific immunity, expression of some immune related genes and disease resistance of large yellow croaker (Larmichthys crocea) following natural infestation of parasites (Cryptocaryon irritans). Aquaculture 33, 101–109. doi: 10.1016/j.aquaculture.2011.12.045
Keywords: DHA, Atlantic salmon, n-3 LC-PUFA, filet quality, Schizochytrium limacinum
Citation: Katerina K, Berge GM, Turid M, Aleksei K, Grete B, Trine Y, Mats C, John S and Bente R (2020) Microalgal Schizochytrium limacinum Biomass Improves Growth and Filet Quality When Used Long-Term as a Replacement for Fish Oil, in Modern Salmon Diets. Front. Mar. Sci. 7:57. doi: 10.3389/fmars.2020.00057
Received: 05 September 2019; Accepted: 27 January 2020;
Published: 14 February 2020.
Edited by:
Seyed Hossein Hoseinifar, Gorgan University of Agricultural Sciences and Natural Resources, IranReviewed by:
Leonardo Julián Magnoni, University of Porto, PortugalYun-Zhang Sun, Jimei University, China
Copyright © 2020 Katerina, Berge, Turid, Aleksei, Grete, Trine, Mats, John and Bente. This is an open-access article distributed under the terms of the Creative Commons Attribution License (CC BY). The use, distribution or reproduction in other forums is permitted, provided the original author(s) and the copyright owner(s) are credited and that the original publication in this journal is cited, in accordance with accepted academic practice. No use, distribution or reproduction is permitted which does not comply with these terms.
*Correspondence: Kousoulaki Katerina, a2F0ZXJpbmEua291c291bGFraUBub2ZpbWEubm8=