- 1College of Science, Health, Engineering and Education, Murdoch University, Perth, WA, Australia
- 2Data 61, CSIRO, Hobart, TAS, Australia
- 3Cawthron Institute, Nelson, New Zealand
- 4Anderson Cabot Center for Ocean Life, New England Aquarium, Boston, MA, United States
- 5Southwest Fisheries Science Center, National Marine Fisheries Service, NOAA, La Jolla, CA, United States
Spatial risk assessments are an effective management tool used in multiple-use marine parks to balance the needs for conservation of natural properties and to provide for varying socio-economic demands for development. The multiple-use Great Barrier Reef Marine Park (GBRMP) has recently experienced substantial increases in current and proposed port expansions and subsequent shipping. Globally, large whale populations are recovering from commercial whaling and ship strike is a significant threat to some populations and a potential welfare issue for others. Within the GBRMP, there is spatial conflict between the main breeding ground of the east Australian humpback whale population and the main inner shipping route that services several large natural resource export ports. The east coast humpback whale population is one of the largest humpback whale populations globally, exponentially increasing (11% per annum) close to the maximum potential rate and estimated to reach pre-exploitation population numbers in the next 4–5 years. We quantify the relative risk of ship strike to calving and mating humpback whales, with areas of highest relative risk coinciding with areas offshore of two major natural resource export ports. We found females with a dependent calf had a higher risk of ship strike compared to groups without a calf when standardized for group size and their inshore movement and coastal dependence later in the breeding season increases their overlap with shipping, although their lower relative abundance decreases risk. The formalization of a two-way shipping route has provided little change to risk and projected risk estimates indicate a three- to five-fold increase in risk to humpback whales from ship strike over the next 10 years. Currently, the whale Protection Area in the GBRMP does not cover the main mating and calving areas, whereas provisions within the legislation for establishment of a Special Management Area during the peak breeding season in high-risk areas could occur. A common mitigation strategy of re-routing shipping lanes to reduce risk is not a viable option for the GBRMP due to physical spatial limitations imposed by the reef, whereas speed restrictions could be the most feasible based on current ship speeds.
Introduction
Shipping is one of the world’s largest industries and extremely important to world economic trade, accounting for 80% of global trade by volume and more than 70% of its value (UNCTAD, 2019). The world shipping fleet has been continuously growing since the 1990s and has doubled in number over the last 12 years, with ships increasing in both size and designed speed capacity to accommodate this trade growth (UNCTAD, 2018). Globally, seaports and other restricted waterways (like canals) are expanding and adapting to meet changes in the industry, resulting in infrastructure expansion projects tied to evolving development plans to take advantage of regional and global opportunities. Shipping is one of the most extensive and pervasive uses of the marine environment, which is exacerbated in coastal areas due to increased interaction with other human uses (i.e. fishing) and protected marine species (Tournadre, 2014). Marine protected areas (MPA’s) are recognized as one of the best ways to conserve and protect marine habitats and species in our oceans (Kelleher, 1999). The management of multiple-use marine parks though, particularly in World Heritage Areas, requires a balance between conserving the natural properties of the area and providing for increasing or shifting socio-economic demands for development. Marine spatial planning and spatially explicit risk assessments are important management tools to balance these interests and manage multiple users.
While there are a range of potential impacts associated with shipping activity (e.g. groundings, collisions, oil and chemical spills and introduction of invasive species), ship strike and noise pollution have the greatest impact on marine mammals. Ship strike and ship noise are the main, current anthropogenic threats to whales worldwide (Cates et al., 2017; Erbe et al., 2019) due largely to the global increase in shipping. While increases in shipping traffic have resulted in the rise in ambient noise at low frequencies (10–100 Hz) in many ocean regions, ships also emit significant energy at higher frequencies (10 of kHz) and can therefore have potential impacts on low frequency specialist (e.g. baleen whales) as well as higher frequency specialists (e.g. odontocetes) (Erbe et al., 2019). However, the impacts from ship noise are less tangible than that of ship strike. Ship strikes represent a conservation concern for some whale species in their recovery from 20th century commercial whaling, and a welfare issue for other species exhibiting significant population recovery and increasing interactions with vessels. Quantifying the population-level extent of ship strike mortality on whales, however, is notoriously difficult due to inherent reporting biases and because collisions with large vessels are frequently unnoticed and consequently go unreported (Laist et al., 2001; Panigada et al., 2006; Vanderlaan and Taggart, 2006; Peel et al., 2018). The most well-documented example of ship strike having a detrimental population-level effect on whale recovery is that of the North Atlantic right whale (Eubalena glacialis), with the major cause of population decline directly linked to ship strike (Laist et al., 2001; Laist et al., 2014). In contrast, for other whale species (e.g. humpback whale, Megaptera novaeangliae) that show strong recovery toward pre-exploitation population levels, ship strike is less of an impact at the population-level and more of a potential welfare issue at the individual-level as a result of non-fatal injuries (Bejder et al., 2016). Analysis of records worldwide (Vanderlaan and Taggart, 2006) and within Australia (Peel et al., 2018), demonstrate that humpback and right whales are the most frequently reported species involved in ship strikes. In Australia, despite the lack of reported incidents involving large ships (one reported case), there are indications that collisions between large ships and humpback whales occur and that the number of reports do not reflect the number of incidents. This is demonstrated by photographs of live humpback whales showing significant wounds consistent with propeller cuts from large ships and stranding events resulting in mortality of humpback whales with wounds suggestive of large ships given the nature and severity of the wound (Peel et al., 2018).
In Australia, the Great Barrier Reef (GBR) is a UNESCO World Heritage Area (GBRWHA) covering approximately 348,000 km2, within which the Great Barrier Reef Marine Park (GBRMP) comprises 99% of this area. The Marine Park is managed by the Great Barrier Reef Marine Park Authority (GBRMPA) as a multiple-use marine park, which supports a wide range of activities such as tourism, defense, fishing, boating and shipping. The GBRMP is recognized as one of the world’s best managed marine protected areas (UNESCO, 2012), although management of it is complex due to overlapping State and Federal jurisdictions and that sometimes the two levels of government are politically ideologically opposed. The GBR is designated a Particularly Sensitive Sea Area (PSSA) by the International Maritime Organization (IMO) because of its potential risk of damage from international shipping activities. Consequently, shipping is well regulated through an established Vessel Traffic Service (REEFVTS) monitoring system and mandatory vessel reporting for vessels >50 m in length. In 2014, a two-way shipping route through the GBR was formalized by the IMO, which predominantly follows previous ship traffic patterns and now provides well-defined lanes to enhance the safety and efficiency of shipping (Figure 1). However, there are current and projected increases in shipping throughout the GBRWHA, predominantly due to the export of natural resources. Australia is one of the world’s largest exporters of natural resources, with approximately 87% of Australia’s total cargo in 2014–15 attributed to international exports predominantly of coal and liquefied natural gas (LNG) (Bureau of Infrastructure, Transport and Regional Economics [BITRE], 2017). Due to substantial coastal development and port expansions related to the mining industry, UNESCO is closely monitoring Australia’s commitment to the sustainability of the GBR as a World Heritage Area.
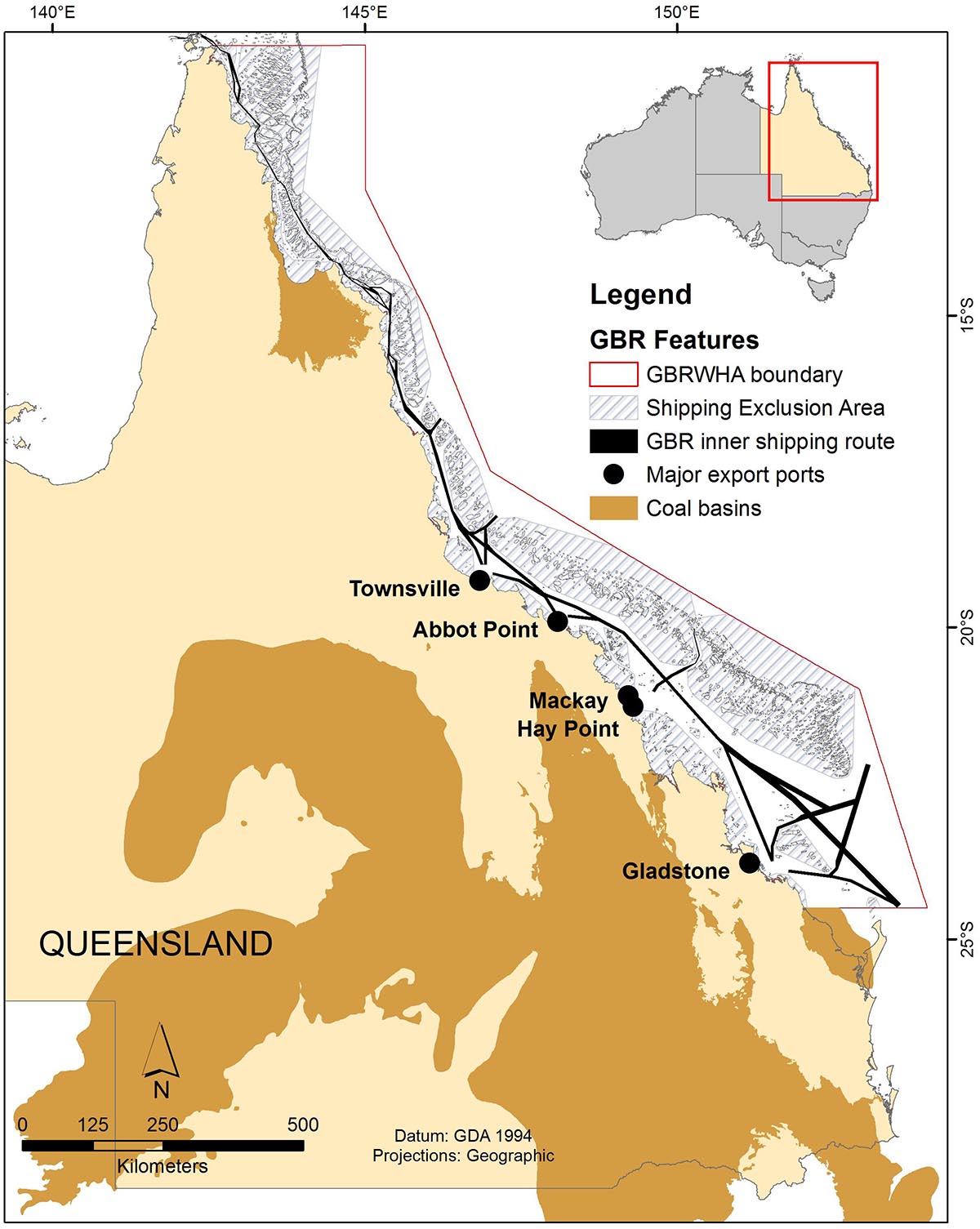
Figure 1. Spatial extents of coal basins, locations of major coal export ports in the State of Queensland, and two-way inner shipping route within the Great Barrier Reef World Heritage Area.
Concurrent to Australia’s growth in shipping, the eastern Australian population of humpback whales is one of the world’s fastest growing population of humpback whales. The population has been undergoing an exponential rate of recovery (approx. 11% increase per annum) over the last couple of decades after facing near extinction from commercial whaling (Noad et al., 2016) and their breeding ground also occurs in the GBRMP (Smith et al., 2012). In 2015, the estimated population size was 25,000 whales and projected to be ∼41,000 whales in 2020. There is little evidence of slowing, with estimates of recovery ranging between 58–98% due to uncertainty of the historical abundance (Noad et al., 2008; Bejder et al., 2016; Noad et al., 2016). Their core breeding aggregation overlaps with the inner shipping route that services all ports on the Queensland coast (Smith et al., 2012). Ship strikes involving large vessels and whales can result in death or serious injury to individuals with the level of risk depending on whale density, behavior, the time of year, vessel density and vessel speed (Cates et al., 2017). With increased shipping activity and whale population size, there is concern for an increased risk in whale fatalities from vessel strikes and increases in non-fatal injuries. While ship strike is unlikely to have a population-level effect on humpback whales in the GBR given the increasing population size, their increased interaction with ships on their breeding ground is likely to be an emerging management issue that could result in welfare issues to the whales from non-fatal injuries (Peel et al., 2018).
To understand the risk of ship strike to whales, it is necessary to understand both distribution and densities of whales and shipping. We provide a spatially explicit ship strike risk framework using humpback whales on their breeding grounds in the multiple-use GBRMP. We modeled relative ship strike risk for whales involved in two different reproductive behaviors of calving (groups containing the presence of a calf) and mating (groups without a calf present), to determine whether there were spatial differences in risk related to reproductive behavior. We compare ship strike risk before and after the formalization of the two-way inner shipping route, to evaluate the effect that defined lanes of ship traffic has on risk to the whales. Finally, we modeled future projected risk of ship strike to humpback whales based on an annual rate of whale population increase for several growth rates in ship traffic. The quantitative risk assessment of ship strike to whales allowed an evaluation of current measures of protection for humpback whales in the multiple-use marine park and potential mitigation measures available to reduce risk.
Methodology
Aerial Surveys
The GBRWHA is a large area (348,000 km2), which makes systematic surveys of the entire area prohibitively costly. Based on a predictive spatial habitat model that was developed using opportunistic presence-only whale sighting data (Smith et al., 2012), line transect aerial surveys were undertaken in 2012 and 2014. The aerial surveys sub-sampled specific regions of the GBRWHA according to their own specific objectives. The 2012 aerial survey was designed to validate the predictive spatial habitat model by surveying three main areas predicted to have low, medium and high habitat suitability, at a time representing peak whale abundance during the breeding season. The aerial survey was undertaken over 8 days (3rd to 10th August) with a total areal coverage of 63,723 km2; Mackay (34,626 km2), Townsville (17,126 km2) and Port Douglas (11,971 km2). On-effort flight time was 15.75 h, of which 97.1% of time conditions were in Beaufort sea-state ≤3. The objective of the 2014 aerial survey was to determine the coastal distribution of humpback whales around major coastal/port areas within a region in the GBRWHA of high whale density later in the breeding season, past peak whale abundance when there are more females with newborn calves. The survey was undertaken offshore of Gladstone and Mackay over 11 days (26th August – 5th September), with a total areal coverage of 72,752 km2. On-effort flight time was 18.3 h, of which 98.8% of time was undertaken in Beaufort sea-state ≤3. The aerial surveys were undertaken using a Partenavia Observer P-68B 6-seater, twin engine, high-wing aircraft and a double platform observer configuration. Rear observers were acoustically and visually (using curtains) isolated from the front observers to allow perception bias to be calculated. Whale sightings included species identification, declination (using a Suunto PM-5/360PC clinometer) and horizontal (protractor) angles to the group, total number of animals’ visible, number of calves and sighting cue.
Species Distribution Model
The distribution and densities of humpback whales in the GBR from the 2012 and 2014 aerial surveys were modeled using the method described in Hedley and Buckland (2004). This requires a detection function fitted to the sighting data to estimate the “effective strip width,” to create a detection-adjusted density surface model using generalized additive models (GAM’s). Detection probabilities, and corrections for perception bias, were estimated using Mark-Recapture Distance Sampling models as described in Laake and Borchers (2004) and Burt et al. (2014) using the MRDS package (Laake et al., 2015) in R (R Development Core Team, 2015). To improve detection function fit, perpendicular sighting distances were left truncated at 0.2 km and right truncated at 4 km, and sightings of uncertain species identification were excluded from the analyses. A final detection function was selected using Akaike Information Criterion (AIC) and examining model diagnostics. A density surface model was then developed using a GAM model by segmenting track lines into pre-defined lengths of approximately 10 km to capture adequate environmental variability using functions for spherical geometry from the R “geosphere” library (Hijmans, 2016). Values of each environmental covariate were converted into rasters in ArcMap 10.1 (ESRI) and matched to the midpoints of each along-track segment. The numbers of whale groups and total animals (including the presence and number of calves) were then summed and a total effective strip area estimated for each segment. These models use a smooth over geographical space, informative environmental covariates and an offset term provided by the effective strip area of each segment. A Tweedie distribution was used to account for over-dispersion in the counts of groups per segment. Collinearity in the various spatial/environmental covariates were assessed using multi-panel scatterplots and Pearson correlation coefficients. All sightings that were included in the distance analyses were used to fit the spatial models. Uncertainty in the estimation of the detection function was incorporated into the variance of the spatial model using a method described in Williams et al. (2011) and Miller et al. (2013). This procedure involves fitting the density surface model with an additional random effect term that characterizes the uncertainty in the estimation of the detection function, via the derivatives of the probability of detection with respect to their parameters.
Physiographic variables of water depth, seabed slope, and (geodesic) distances to the nearest coastline and reef features were estimated for the midpoints of each along-track segment. Monthly mean values of dynamic remotely sensed environmental predictor variables were interpolated to the midpoint of each along-track segment. Daily sea surface temperature (Integrated Marine Observing System [IMOS], 2015a; in °C, gridded at 0.02°), sea surface height anomaly (IMOS, 2015b; in meters, gridded at 0.58°>0.51°), and sea surface chlorophyll a (IMOS, 2015c; mg m-3, gridded at 0.01°) values for the GBR region were averaged at each grid point for the month of August in 2012 and August and September in 2014. Predictions of whale densities across the GBR were undertaken at a 1 × 1 km grid cell resolution to produce density models for three different whale groups: (1) all whales, (2) groups that contained a calf (hereafter, calf groups) and (3) groups in which a calf was not present (hereafter, non-calf groups). Sightings and modeled distributions of calf groups are used as a proxy to identify likely calving areas and non-calf groups to identify potential mating areas. A 1 × 1 km grid cell size was chosen to provide enough spatial resolution to distinguish a specific shipping lane and to avoid the issue of vessels and animals not in close proximity being classed as co-occurring and contributing to risk within the spatial risk assessment.
Shipping Data
All large vessels transiting through the GBRWHA are monitored with AIS by the REEFVTS and ships are only permitted to transit through Designated Shipping Areas. In December 2014, the IMO formalized a two-way shipping route in the GBR that extends from the Torres Strait in the north and terminates at the southern boundary of the GBRMP (Figure 1). The two-way shipping route follows pre-existing traffic patterns through the GBR and now encourages shipping to follow well-defined northbound and southbound lanes, although it is not mandatory to travel within these lanes.
AIS data were obtained from AMSA in the form of their craft tracking system (CTS) product, which provides processed ship locational data sampled to a 5 min frequency. AIS data were analyzed for each year between 2013 and 2016, which covered the time period when systematic aerial surveys for humpback whales were undertaken and the formalization of the two-way shipping route. Shipping data were restricted to Class “A” cargo, tanker and passenger vessels ≥80 m in length for 3 months of the humpback whale breeding season (July, August, and September). Only vessels ≥80 m were included for the following reasons: vessels of this size and larger predominantly inflict fatal or severe injuries (Laist et al., 2001), larger vessels traverse predictable routes, AIS data provides relatively accurate ship positional data and previous risk assessments of ship strike to whales (e.g. Redfern et al., 2013) have focused on larger vessel size classes. The AIS data did not have navigational status of the vessel available, which can be used to filter out vessels not underway (e.g. anchored). Consequently, we applied a filter of >0.4 knots to the data to remove stationary/anchored vessels that will have limited risk for ship strike.
To use the AIS data in the risk assessment framework, we created trackline data from the point data representing 5-min AIS positions of each individual vessel based on a unique ship-related identifier, the Maritime Mobile Service Identity (MMSI). This converts the data in each cell from time to distance data. Trackline data were created by joining contiguous unique point positions of ship locations less than 60 min apart, with the exception of positions separated in time between 30 and 60 min with a change in ship’s course over ground greater than 5 degrees (due to uncertainty of the ship’s path of travel). Positions greater than 60 min apart were excluded. The 1 × 1 km whale density grid over the entire GBRWHA region was used to summarize the distance traveled by ships within each grid cell from the trackline data.
Risk Modeling Framework
To quantify relative risk of ship strike we calculated the Relative Expected Fatality (REF) of a whale from the risk of a ship strike. This incorporates a measure of co-occurrence of a whale and ship in a given grid cell (Redfern et al., 2013), and uses vessel beam as an exposure factor and the equation from Conn and Silber (2013) to estimate the probability of a lethal whale strike given vessel speed. This approximates the risk of a fatal ship strike more accurately than co-occurrence alone, because the severity of a ship strike is related to the speed of a vessel. A whale risk index was calculated by multiplying the ship and whale density with the mean vessel beam and the probability of a lethal whale strike given the mean vessel speed for each grid cell for each of the years 2013 to 2016. We summarized the risk for each year and three whale group categories (all whale groups, calf and non-calf groups). The cumulative total, mean, minimum, and maximum risk observed were calculated and the estimates were then standardized to account for differences in the number of vessels between years by dividing the risk estimates by the total km’s traveled by all vessels in the GBR. Relative risk was also summarized at a decreased resolution of 50 × 50 km grid cells to identify risk patterns at the broader regional scale. To investigate whether there was a change in the risk of ship strike to humpback whales due to the IMO formalization of the inner GBR two-way shipping route, relative risk was compared before (2013/2014 ship data) and after (2015/2016 ship data) the formalization.
Projected Future Risk of Ship Strike
Predicting future relative risk based on projected growth rates can be difficult because it is uncertain how increases in shipping and whale population size will change temporally or spatially. To predict future relative risk we assumed that there are no changes to the spatial distribution of ships or whales, which is likely to be more uncertain for whales due to an increasing population size (e.g. through range expansion) than for shipping that follow formalized shipping lanes. We calculated future risk for each grid cell by multiplying an annual proportional increase of whale abundance (11%) and five ship traffic growth rates around a 3.5% expected mean growth rate, from 1.5 to 5.5%. The expected growth rate of the whale population can be considered robust due to surveys since the 1990s producing consistent estimates of approximately 11% per year (Noad et al., 2016). The Australian Bureau of Resources and Energy Economics (BREE) predicted the average annual growth in coal ship traffic between 2011 and 2025 in the GBR, according to a range of likely scenarios; optimistic was 6.31, 5.12% was moderate, 3.71% low and 3.06% the most conservative case (Braemar Seascope, 2013).
Results
Humpback Whale Distribution Model
There were a total of 637 sightings of humpback whale groups from the combined aerial surveys, 365 group sightings (589 individuals) in 2012 and 272 (461 individuals) in 2014 (Figure 2). The breakdown of calf groups between years and mean whale encounter rates are in Table 1. There was a lower relative abundance of whales in 2014 compared to 2012 due to undertaking the aerial survey later in the breeding season, past the expected peak of whale abundance. The detection function was fit using sighting data pooled across both survey years and a total 561 sightings remained after truncation of the data were used for density surface modeling.
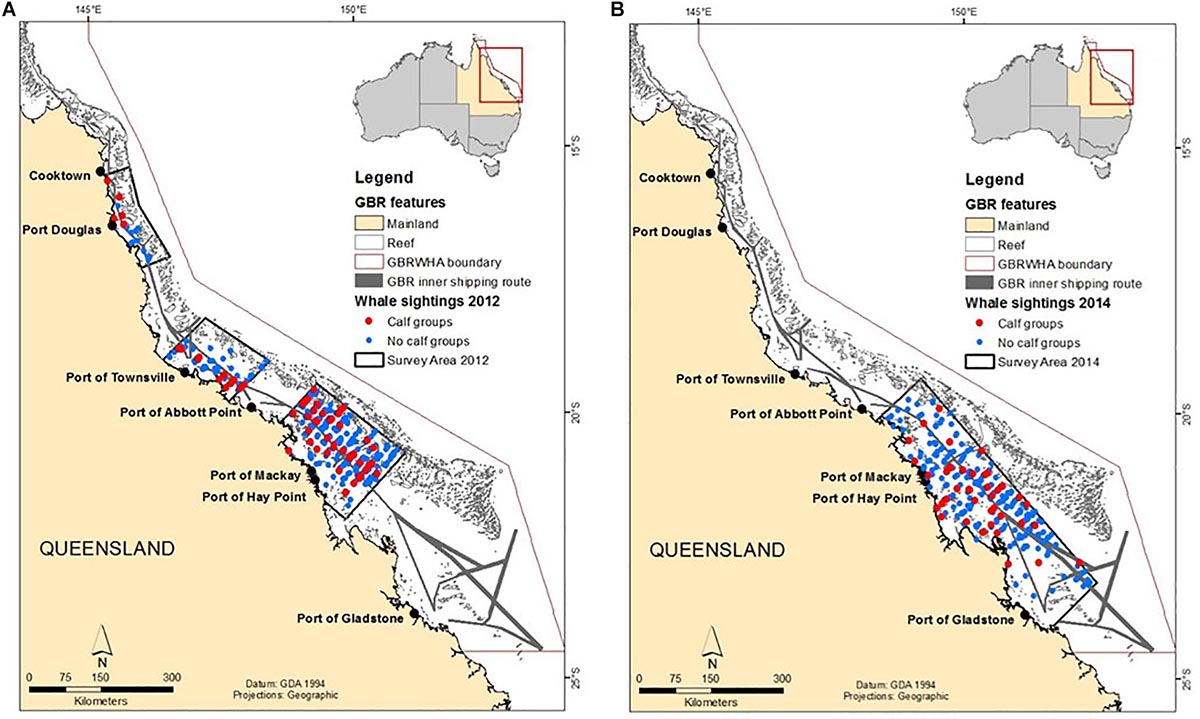
Figure 2. Distribution of humpback whale sightings of calf and non-calf groups during the (A) 2012 and (B) 2014 aerial surveys.

Table 1. The number of sightings, relative abundance and mean encounter rates for groups of all whales and groups containing a calf during the 2012 and 2014 aerial surveys.
The prediction from the best density surface model of humpback whale density for each of the three reproductive categories are in Supplementary Figure S2. Due to only a small amount of survey effort in bathymetric values of 90 m and deeper (only 122 km of a total of 6650 km across both survey years), no density predictions were made for waters deeper than 90 m. The most significant parameters in describing humpback whale distribution and density were depth and SST. The models predicted higher densities of humpbacks in shallow water (e.g. 20–60 m deep) and within a sea surface temperature range between 21 and 23°C.
The predicted distribution of whale densities for all whale groups combined in the GBR followed a similar pattern for both 2012 and 2014 (Supplementary Figure S1). Two main areas of higher whale density during peak whale abundance are located approximately 120 km to the north and southeast of Mackay (Figure 3). The modeled distribution of calving areas (sightings of groups with a calf present) in 2012, and to a lesser extent in 2014, occurred throughout the length of the GBR whereas mating areas (groups without a calf) were predominantly restricted to the southern GBR (Figure 3). However, given calf groups were sighted among non-calf groups in inshore and offshore waters, there does not appear to be any distinct separation of calving versus mating areas. The highest number of whale sightings was in the southern GBR region, although the northern GBR region offshore of Cairns had a proportionally higher calf-to-adult ratio (1:4) compared to the southern GBR offshore of Mackay (1:7.9) (Figure 2). In 2014, there was a significant change in the distribution of calf groups closer to the coast compared to non-calf groups (Figures 2, 3). If we compare the distribution of calf groups in 2012 to those in 2014 (Figures 3B,D) and assume little inter-annual variation in whale distribution, the predicted distribution suggests that groups with a calf move closer to the coast later in the breeding season.
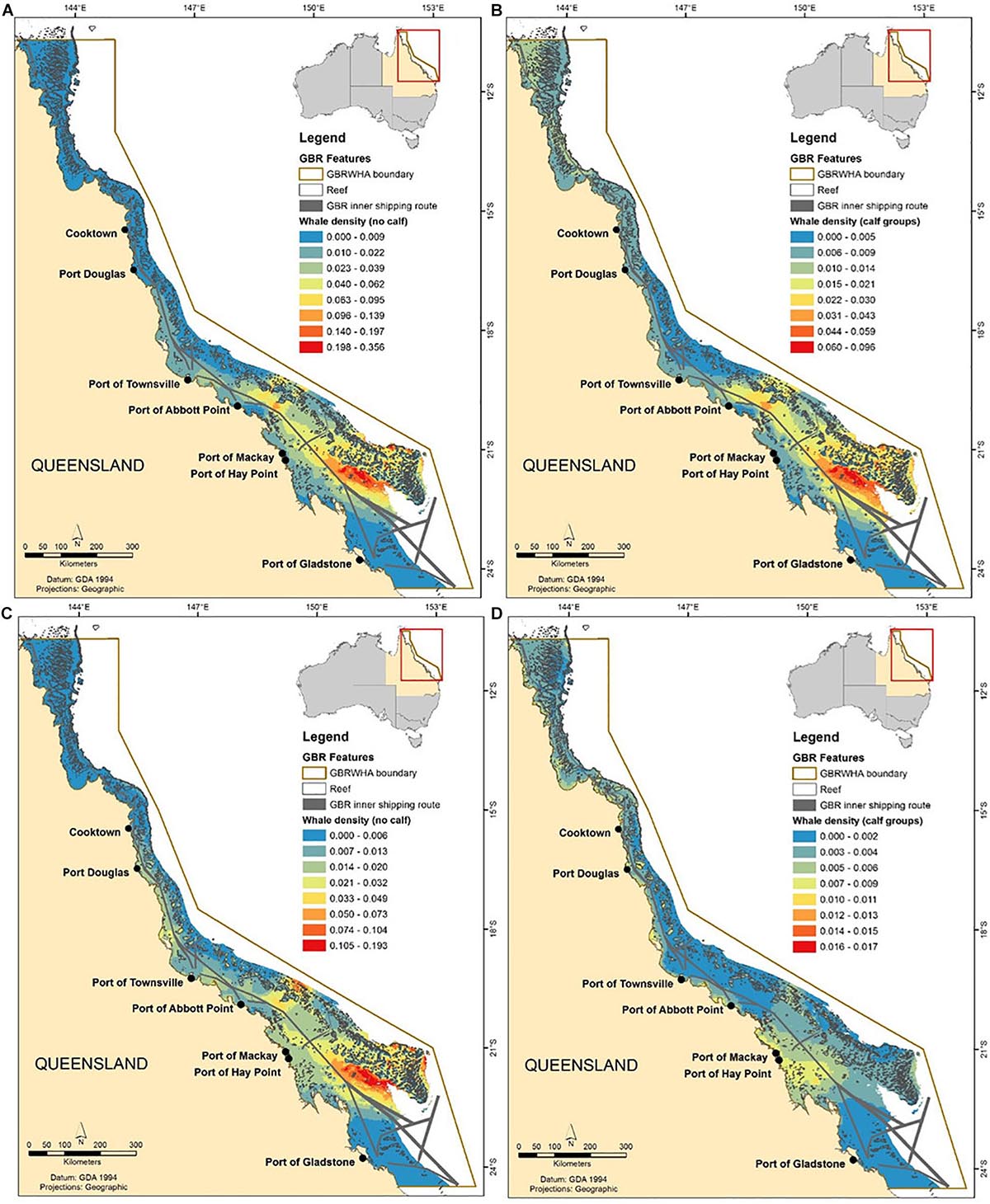
Figure 3. Modeled distribution and densities of humpback whale (A) non-calf groups and (B) calf groups in August 2012 and (C) non-calf groups and (D) calf groups in September 2014 in the Great Barrier Reef Marine Park with the overlayed inner shipping route.
Shipping Data
There was a slight increase in the number of ships per year between 2013 (N = 1466) to 2016 (N = 1687) and no detectable within year variation, such that the 3 months within the year were comparable. The majority of class A vessels (≥80 m in length) used in the analysis over the four years were cargo vessels (87%), followed by Tankers (12%) and a small number of passenger vessels (1%). There was a consistent pattern in the length of the vessels across all years that ranged from 80 (the minimum cut-off) to 300 m, with a higher frequency of vessel length closer to larger sized ships (mean = 205 m and median = 222 m). There was also a consistent pattern in vessel beam with a mean and median of 32 m (range = 10–50 m). The average vessel speed was 12.6 knots (median = 12.4 knots, max = 25 knots) with 85% of vessel transits faster than 10 knots and 11% faster than 15 knots (74% >10 knots ≤15 knots). Vessel speeds greater than 15 knots occurred in specific areas of the GBRMP, specifically the Whitsunday Islands offshore Abbott Point port and Gladstone port.
Relative Ship Strike Risk Maps
Formalization of IMO Two-Way Shipping Route
Given there was little change in shipping numbers between years and a higher relative abundance of whales in 2012 compared to 2014, there was a higher average relative risk of ship strike (two-fold increase) at the peak abundance of the season in 2012 compared to later in the breeding season in 2014 (Supplementary Figure S3). A comparison of ship strike risk to whales pre- and post-formalization of the inner shipping route shows minimal difference in the risk to whales (Supplementary Figure S3). Fundamentally, the two-way route formalized existing traffic patterns into well-defined shipping lanes, such that there was little variation in shipping traffic distributions pre and post the IMO formalization.
Spatial Risk of Ship Strike to Humpback Whales
At the time of peak whale abundance on the breeding grounds (2012 whale model), the areas of higher relative risk of ship strike to humpback whales occur in areas where shipping traverses two areas of higher predicted whale density (Figures 3A,B, 4) in the southern GBR. All patterns of risk were consistent across all years of shipping data, due to negligible differences in ship numbers between years. At the finer spatial resolution (1 × 1 km) several areas of high ship strike risk (>80%) were identified, including offshore of the Port of Abbott Point and Port of Mackay/Hay Point (Figure 4). At the coarser spatial resolution (50 × 50 km), the areas of high risk (>80%) were restricted to the one location offshore of the Port of Mackay/Hay Point thus corresponding to a greater area of high risk (Figure 4).
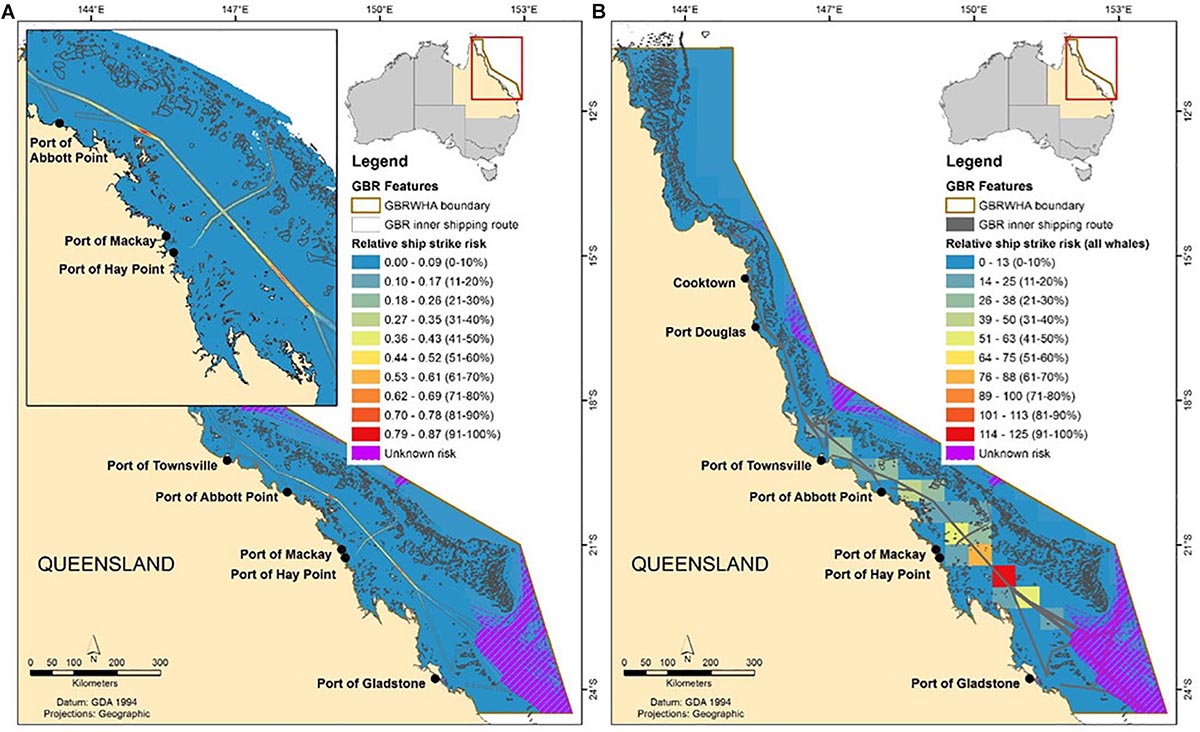
Figure 4. Relative ship strike risk for all humpback whale groups in the GBRWHA based on the 2012 whale model and 2016 shipping data at (A) 1 × 1 km and (B) 50 × 50 km grid cell resolution.
Overall, cumulative risk of ship strike for humpback whales at the group level is higher for non-calf groups compared to groups with a calf, due to there being significantly more sightings of non-calf groups (75%) compared to calf-groups (25%). However, when standardized for the total number of whales in each group, the risk was consistently higher for groups containing females with a dependent calf in both 2012 and 2014 (Supplementary Figure S4). During peak abundance within the breeding season (using the 2012 whale model), there was consistency in the areas of high risk of ship strike for both calf and non-calf groups in areas located in the southern GBR lagoon, offshore of the Port of Hay Point and Mackay (Figures 5A,B). However, as the breeding season progresses there was a change in the spatial distribution of groups with a calf from offshore to inshore waters (Figures 3B,D). This resulted in a spatial change in risk to a greater area of overlap with the shipping lane and coastal waters. While the area of ship strike risk for groups with calves increased later in the breeding season (Figures 5B,D), there was a reduction in the level of risk due to a decrease in relative whale abundance.
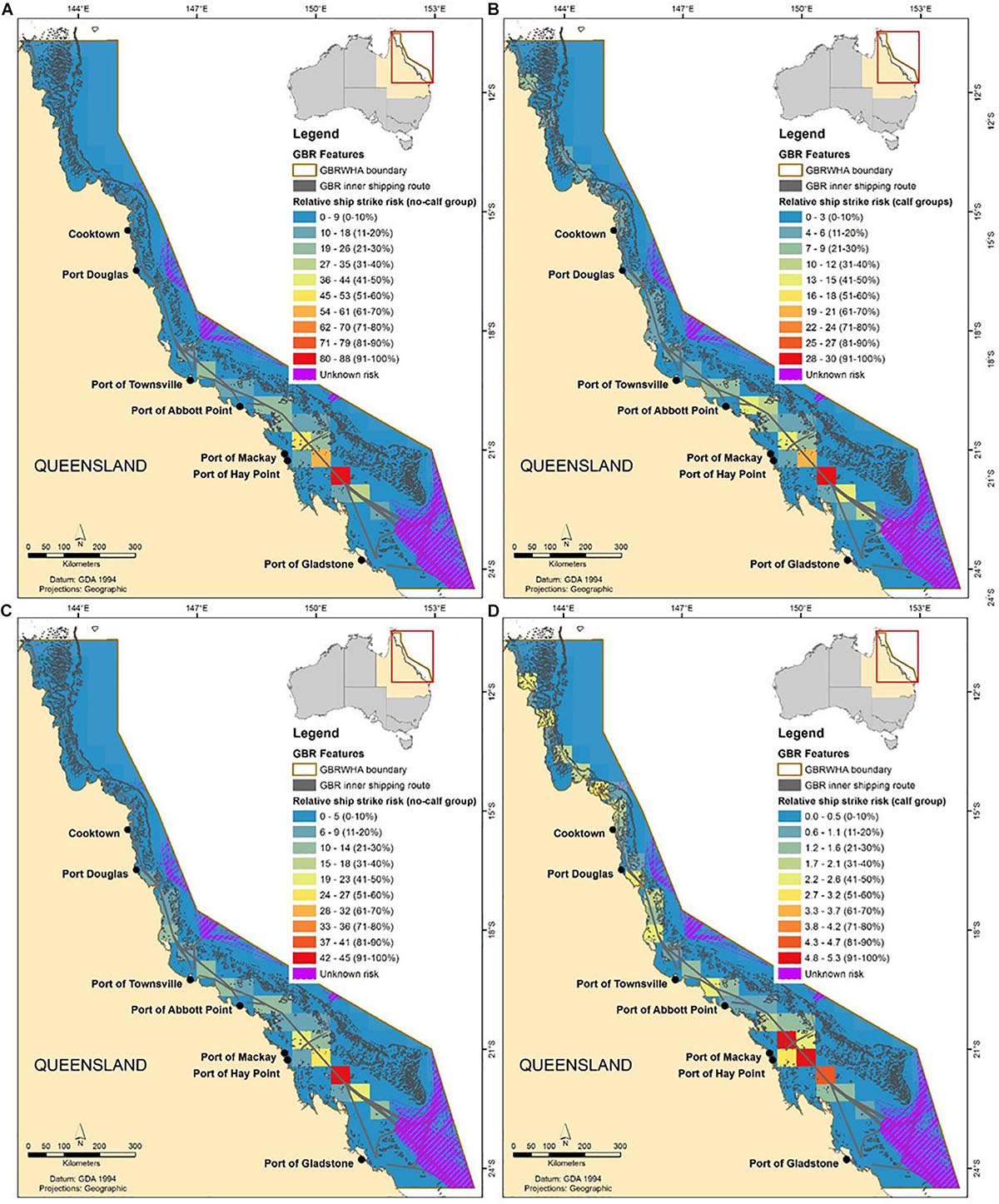
Figure 5. Relative ship strike risk for humpback whale (A) non-calf groups and (B) calf groups in August 2012 and (C) non-calf groups and (D) calf groups in September 2014 based on 2016 ship data at a 50 × 50 km grid cell resolution.
Projected Ship Strike Risk
The population of whales is increasing at an exponential rate and concurrently there are current and projected increases in shipping in the GBR. We calculated a projected risk of ship strike to humpback whales over a 10-year period based on an 11% annual increase in whale population size (Noad et al., 2016). We used four different shipping traffic growth rates based on a conservative (1.5%) to optimistic (5.5%) estimate and projected forward to 2028. Based on the different shipping traffic growth rates, there is predicted to be between a three (conservative ship growth) and five-fold increase (optimistic ship growth) in the risk of ship strike to humpback whales in the GBR within the next 10 years (Figure 6).
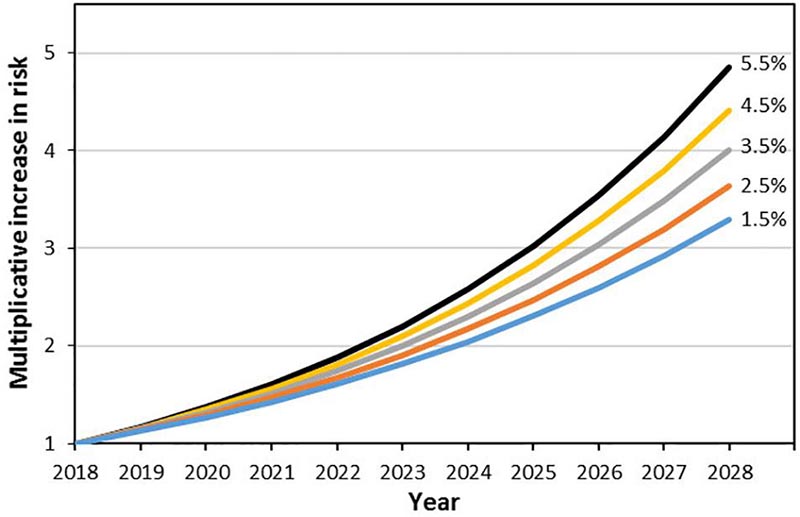
Figure 6. Projected increase in relative expected fatality based on an annual 11% increase in whale population size and various projected annual shipping traffic increases (colored lines).
Discussion
Management of multiple-use marine parks and World Heritage Areas requires a balance between conservation and socio-economic demands for development. Spatially explicit risk assessments provide the ability to manage multiple users of the marine environment, reduce environmental impacts and reduce conflict among users (Hope, 2006). Within the GBRWHA, there is considerable overlap between shipping lanes and the breeding aggregation of humpback whales for which shipping traffic and whale population size are both increasing. Furthermore, the expansive physical structure of the GBR limits the ability to segregate these two uses of the Marine Park and implement a common mitigation measure of re-routing shipping channels away from Biologically Important Areas. It is unlikely that ship strikes will have a population-level effect on the whales given the population is increasing close to its maximum potential rate (∼11% per annum). However, there is concern for a potential increase in whale fatalities from ship strikes, and welfare concerns arising from non-fatal injuries, due to greater interaction between breeding whales and ships. The spatially explicit risk assessment has identified specific areas within the GBRMP of higher relative risk of ship strike to whales from large commercial ships. This should provide the basis to evaluate the level of threat to whales from ship strike and focus future research areas to aid informed management decisions on the types of mitigation measures necessary.
The Importance of Spatial Resolution on Risk Estimates
To understand the risk of ship strike to whales, it is necessary to understand both the distribution and densities of whales and shipping. Generally, there will be a degree of uncertainty when quantifying both of these. Accurately identifying whale distribution and density within and between years for a mobile marine species’ is difficult without considerable sampling effort, and shipping traffic can vary based on specific port activities and global economic factors. This is an important consideration when undertaking spatially explicit risk assessments and identifying an appropriate spatial resolution for the data. Often shipping lanes are only several km’s in width and necessitate high-resolution data (e.g. 1 × 1 km). However, uncertainty in whale distribution data may not support such high resolution. In our study we sub-sampled whale distribution within the GBRMP then modeled, and extrapolated on 2 years of survey data, which incorporates a certain degree of uncertainty in whale distribution. The spatial resolution also depends on the spatial scale over which management decisions are being conducted (e.g. tens, hundreds or thousands of km). In the case of the GBRMP, we advocate the large area of the Marine Park (344,000 km2), covering 14 degrees of latitude, requires undertaking the spatial risk assessment at a resolution coarser (e.g. 50 × 50 km) than what AIS shipping data necessitates (e.g. 1 km). Finer spatial resolution in the data might be required for localized, small-scale applications such as port developments, whereas regional management planning and zoning would necessitate coarser resolution.
We quantified risk at a fine scale (1 × 1 km) and coarse scale (50 × 50 km) and Figure 4 demonstrates the effect that spatial resolution can have on identifying areas of risk. At the finer spatial resolution, several areas of high ship strike risk (>80%) were identified, including the area offshore of Abbott Point. While this is an area of high risk, the extent of it covered approximately 20 km of the shipping lane and consequently at the coarser spatial resolution it did not comprise an adequate proportion of the area as high risk. Consequently, the areas of high risk were restricted to the one location offshore of the Port of Mackay/Hay Point.
Higher Ship Strike Risk to Females With Calves
The relative risk of ship strike differed for whales of different reproductive class, with groups without calves having a higher overall cumulative risk of ship strike. This was due to there being significantly more non-calf groups (75% in total) than groups with calves (25%). However, when risk is standardized for the total number of whales in each group type, the risk is higher throughout the GBRMP for groups with a female and dependent calf compared to non-calf groups. While there was no distinct separation of calving versus mating areas, given calf groups were sighted among non-calf groups in both inshore and offshore waters, calving areas (based on sightings of groups with calves) occurred throughout the length of the GBR and mating areas (groups without a calf) were predominantly restricted to the southern GBR (Figure 2). This northern GBR had a higher calf-to-adult ratio (1:4) compared to the southern GBR (1:8) offshore of Mackay.
During peak whale abundance within the breeding season, areas of relative high ship strike risk to calf and non-calf whale groups were consistently identified in the southern GBR lagoon offshore of the Port of Mackay and Hay Point (Figures 4A,B). Later in the breeding season when more females have given birth and the southward migration away from the breeding ground has started, the relative abundance of whales decreases and there is a shift in the distribution by groups with females and a dependent calf from offshore to coastal, inshore waters (Figures 2, 3D). This assumes minimal inter-annual variation in whale distribution, which seems plausible given non-calf groups in 2014 occurred in a similar area to non-calf and calf groups in 2012. Females with calves from several other populations of humpback whales (e.g. Ecuador, Hawaii, Brazil) also display a preference for coastal, shallow water habitat on their breeding grounds (Félix and Botero-Acosta, 2011; Craig et al., 2014; Guidino et al., 2014; Gonçalves et al., 2018; Pack et al., 2018). The shift in distribution of calf groups to inshore waters resulted in greater overlap with the shipping lane, and an increase in the area of higher (>80%) ship strike risk. However, the level of risk was considerably lower in September compared to peak whale abundance in July/August (Figure 5) due to a lower relative abundance. Currently, the ship strike risk framework does not incorporate whale behavioral data that could differ among age and social classes (e.g. vessel avoidance). Estimates of risk will be affected if certain classes of whales exhibit behavioral attributes that make them more or less susceptible to ship strike. For example, calf groups could be more at risk of ship strike compared to non-calf groups if they have a higher level of exposure to a ships’ strike zone as a consequence of dive behavior e.g. frequent shallow dives. The risk framework does not incorporate time spent at the surface due to insufficient behavioral data.
Formalization of Two-Way Shipping Route and Projected Ship Strike Risk
From December 2014, the IMO formalized the inner two-way shipping route through the GBR. While the route in the northern GBR was existing, a new section was added to the southern GBR that corresponded to existing shipping traffic patterns. Therefore, patterns in shipping traffic through the GBR predominantly remained unchanged. Consequently, there has been little difference in ship strike risk to whales resulting from the formalization of the two-way route. Implementation of the formalized shipping lane had the greatest change to the distribution of shipping traffic offshore of Gladstone, in the area of unknown risk. This area could not be modeled due to insufficient whale data, although is likely a high risk area for ship strike given the large export volume of LNG from Gladstone port and the multiple shipping routes crossing a high density of whales undergoing a constrained migration movement in this region (Smith et al., 2012).
Future estimates of shipping volume in the GBR suggests a potential increase of 4–5% annual growth rate, based on Qld port industry forecasts over the period 2012–2032 for all vessels and ports (PGM Environment, 2012). Projected ship strike risk based on conservative (1.5%) to optimistic (5.5%) ship traffic growth rates show a three to five-fold increase in risk to whales over the next 10-year period (Figure 6). Over this time period, there is a potential doubling of the humpback whale population size from the current estimate of 25,000 whales (Noad et al., 2008; Bejder et al., 2016; Noad et al., 2016). The current population size is estimated to have reached over 50% of pre-exploitation levels, with estimates of recovery ranging between 58–98% due to uncertainty of the historical abundance (Noad et al., 2008; Bejder et al., 2016; Noad et al., 2016). Of particular interest over the next 10 years for the East Australian population of humpback whales is the population recovery trajectory, with the possibility of a population leveling to an uncertain carrying capacity between 26,000 to 42,000 whales (Ross-Gillespie et al., 2015). This highlights the necessity for understanding natural versus anthropogenic impacts on the recovery of whale populations.
The relative ship strike risk maps identified the southern half of the GBRMP from Townsville to north of Gladstone (approx. 19°S–22°S), including the east-west Hydrographers Passage route offshore of Mackay, to have the largest relative risk within all of the Marine Park (Figure 4). The whale density models show these areas correspond to where shipping traverses two higher predicted whale density areas (Figure 3). This encompasses four major trading ports and likely to also include Gladstone. These five ports make up the majority of export trade, particularly of natural resources such as coal, along the GBR coast representing 78% ($51.75 billion) the total throughput of all Qld. ports (Queensland Government Statistician’s Office, 2017). The Qld. commodity market is currently, and into the future, dominated by the trade of coal and liquefied natural gas (LNG), with Australia currently the second largest global exporter of LNG. Consequently, the risk to whales is only likely to increase unless there is significant downturn in coal and LNG exports. This highlights the importance of an informed understanding of the threats to the population (e.g. ship strike).
Conservation Implications and Current Protective Measures
Ship strike of whales is a global issue that has resulted in various management measures aimed at reducing the risk to whales. A spatial management approach commonly implemented involves the establishment of time and area specific modifications, for example Seasonal Management Areas and Traffic Separation Schemes (TSS) (Silber et al., 2012). Vessel routing and speed restrictions have both been shown to reduce the probability and severity of ship strikes (Vanderlaan and Taggart, 2006; Vanderlaan and Taggart, 2009; Wiley et al., 2011; Conn and Silber, 2013; Laist et al., 2014). Within the GBRMP, mitigation options are more limited because the extensive reef structure of the GBR constrains ship traffic movement between the reef and the coastline. This significantly limits the viability of re-routing measures due to the limited space within the Designated Shipping Area (Figure 1). Furthermore, there is a dynamic temporal component to the distribution of whales throughout the breeding season, with movement of calf groups from offshore to inshore waters.
A feasible management option within the GBRMP is the designation of a Special Management Area (SMA) for which there is provision under the Great Barrier Reef Marine Park Regulations 2019 for purposes outlined in the Great Barrier Reef Zoning Plan 2003, which include the conservation of a particular species or resource e.g. aggregation sites. Species Conservation areas are a type of SMA that have been implemented for dugongs in the GBRMP to restrict human activities and minimize disturbance. Currently, a Whale Protection Area (WPA) (which is not an SMA) exists for whales in the Whitsunday area to restrict the distance that vessels can approach breeding whales and minimize disturbance. However, this area was primarily established to manage tourism vessels involved in whale watching and other tourism activities. The WPA clearly does not cover the areas of highest density of whales and greatest risk of ship strike to breeding whales in the GBR (Figure 7). The relative risk maps have identified areas that represent sufficient risk to breeding humpback whales and warrants consideration of suitable mitigation to reduce the risk. Current legislation provides the opportunity to establish a Species Conservation area as part of a SMA that could help focus management effort. Specific mitigation options could be the focus of further research into understanding the magnitude of the threat of ship strike to humpback whales and could range from voluntary reporting of whale sightings by onboard observers to mandatory speed restrictions. AMSA in partnership with the IMO could impose seasonal speed restrictions in targeted areas to reduce ship strike risk. Speed restrictions could be a viable and cost-effective management option given the evidence that vessel speed reductions of large vessels to ≤10 knots significantly reduces the risk of ship strike (Vanderlaan and Taggart, 2006) and many vessels already travel close to that speed in the GBRMP (74% of vessel transits are between 10 and 15 knots and only 11% >15 knots).
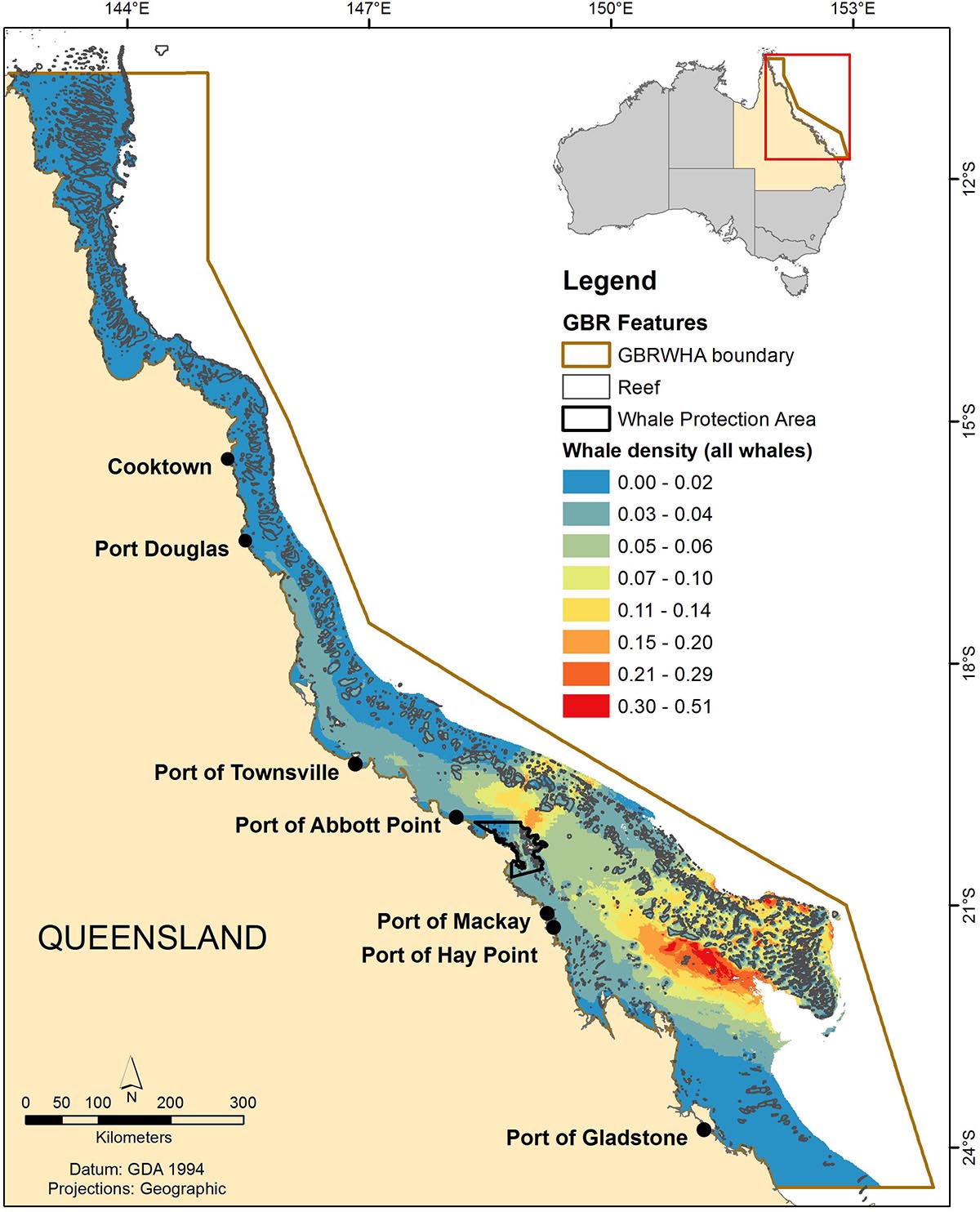
Figure 7. Map of the whale protection area and modelled distribution and density of ‘all whale’ humpback whale groups during August 2012, with red areas indicating high whale density.
Currently, the ship strike framework provides a relative metric across the study area useful for comparing relative risk, although cannot be inferred as an estimation of actual mortality. Calculating absolute risk is currently problematic due to insufficient knowledge on many parameters associated with ship strike (e.g. response/avoidance behavior of whales to vessels), large uncertainty/variance related to species spatial distributions (e.g. intra- and inter-annual variability) and unknown parameters that have not been modeled (e.g. survivorship from blunt force trauma). The framework does enable managers to assess different scenarios of speed restrictions and its effect on risk, due to incorporating vessel speed as a factor in the risk calculation. To improve the ship strike framework, incorporation of different vessel characteristics (e.g. vessel draught and potential depth of strike zones including hydrodynamic effects) and whale behavior (e.g. time at surface and avoidance) are required, if and when, data are available. While the true relationship between relative and absolute risk remains unknown, these data provide the best source of information to aid in the identification of potential hotspots of high interactions between whales and shipping in the GBR.
Data Availability Statement
The datasets generated for this study are available on request to the corresponding author.
Ethics Statement
The animal study was reviewed and approved by the Murdoch University Animal Ethics Committee permits (O2487/12 and O2680/14).
Author Contributions
JS conceived the fieldwork design, collected the data, and led the writing of the manuscript. JS, NK, SC, DP, JR, and TM analyzed the data. All authors contributed critically to the drafts and gave final approval for publication.
Funding
We acknowledge the Australian Marine Mammal Centre Grant Program as our primary funder and the International Fund for Animal Welfare Oceania Office for supporting funds. The funding bodies had no involvement in the study design, in the collection, analysis and interpretation of data, in the writing of the report and in the decision to submit the article for publication.
Conflict of Interest
The authors declare that the research was conducted in the absence of any commercial or financial relationships that could be construed as a potential conflict of interest.
Acknowledgments
We are extremely grateful to many people and organizations who have aided in the development of this project. Specifically, we would like to acknowledge the Australian Marine Mammal Centre and the International Fund for Animal Welfare Oceania Office, in particular Isabel McCrea, Matt Collis, and Sharon Livermore from IFAW for their support of the project. To Mark Read and Andrew Simmons at GBRMPA for their support of the project, providing advice and access to data. The Australian Maritime Safety Authority have been supportive of the project and helpful with access to AIS data, especially Ross Henderson. The professional and experienced flying of Brad Welch from aerial charter company Observair was invaluable for successfully undertaking aerial surveys and thanks to the aerial survey team in 2012 (Susan Sobtzick, Natalie Schmitt, Verity McCorkill, Louise Bennett, and Amanda Hodgson) and 2014 (Louise Bennett, Maria Jedjenso, Shannon McKay, Kylie Mackenzie, and Amy James) for their dedicated work. This work was undertaken under GBRMPA permits (G12/35027.1 and 14/37113.1) and Murdoch University Ethics permits (O2487/12 and O2680/14).
Supplementary Material
The Supplementary Material for this article can be found online at: https://www.frontiersin.org/articles/10.3389/fmars.2020.00067/full#supplementary-material
References
Bejder, M., Johnston, D. W., Smith, J. N., Friedlaenderd, A., and Bejder, L. (2016). Embracing conservation success of recovering humpback whale populations: evaluating the case for downlisting their conservation status in Australia. Mar. Policy 66, 137–141. doi: 10.1016/j.marpol.2015.05.007
Braemar Seascope (2013). North Queensland ship traffic growth study. Supplementary Report. 71. Available at: https://docplayer.net/17070612-North-queensland-ship-traffic-growth-study-supplementary-report-for-public -release.html (accessed March 22, 2013).
Bureau of Infrastructure, Transport and Regional Economics [BITRE] (2017). Australian Sea Freight 2014–15. Canberra, ACT: BITRE.
Burt, M. L., Borchers, D. L., Jenkins, K. J., and Marques, T. A. (2014). Using mark-recapture distance sampling methods on line transect surveys. Methods Ecol. Evol. 5, 1180–1191. doi: 10.1111/2041-210X.12294
Cates, K., DeMaster, D. P., Brownell, R. L. Jr., Silber, G., Gende, S., Leaper, R., et al. (2017). Strategic Plan To Mitigate The Impacts Of Ship Strikes On Cetacean Populations: 2017-2020. Cambridge: International Whaling Commission.
Conn, P. B., and Silber, G. K. (2013). Vessel speed restrictions reduce risk of collision-related mortality for North Atlantic right whales. Ecosphere 4:43. doi: 10.1890/ES13-00004.1
Craig, A. S., Herman, L. M., Pack, A. A., and Waterman, J. O. (2014). Habitat segregation by female humpback whales in Hawaiian waters: avoidance of males? Behaviour 151, 613–631. doi: 10.1163/1568539X-00003151
Erbe, C., Marley, S. A., Schoeman, R. P., Smith, J. N., Trigg, L. E., and Embling, C. B. (2019). The effects of ship noise on marine mammals–a review. Front. Mar. Sci. 6:606. doi: 10.3389/fmars.2019.00606
Félix, F., and Botero-Acosta, N. (2011). Distribution and behaviour of humpback whale mother–calf pairs during the breeding season off Ecuador. Mar. Ecol. Prog. Ser. 426, 277–287. doi: 10.3354/meps08984
Gonçalves, M. I. C., Sousa-Lima, R. S., Teixeira, N. N., Morete, M. E., Carvalho, G. H., Ferreira, H. M., et al. (2018). Low latitude habitat use patterns of a recovering population of humpback whales. J. Mar. Biol. Assoc. U. K. 98:1087. doi: 10.1017/S0025315418000255
Guidino, C., Llapapasca, M. A., Silva, S., Alcorta, B., and Pacheco, A. S. (2014). Patterns of spatial and temporal distribution of humpback whales at the southern limit of the southeast pacific breeding area. PLoS One 9:e112627. doi: 10.1371/journal.pone.0112627
Hedley, S. L., and Buckland, S. T. (2004). Spatial models for line transect sampling. J. Agric. Biol. Environ. Statist. 9, 181–199. doi: 10.1198/1085711043578
Hope, B. K. (2006). An examination of ecological risk assessment and management practices. Environ. Intern. 32, 983–995. doi: 10.1016/j.envint.2006.06.005
Integrated Marine Observing System [IMOS] (2015a). SRS Satellite – SST L3S – 1 Day Composite – Day and Night Time. Available at: https://imos.aodn.org.au/imos123/home (accessed April 1, 2015).
Integrated Marine Observing System [IMOS] (2015b). Gridded Sea Level Anomaly-Delayed Mode. Available at: https://imos.aodn.org.au/imos123/home (accessed April 1, 2015).
Integrated Marine Observing System [IMOS] (2015c). SRS Satellite-OC MODIS – 1 day –Chlorophyll a Concentration Algorithm. Available at: https://imos.aodn.org.au/imos123/home (accessed April 1, 2015).
Laake, J. L., Borchers, D., Thomas, L., Miller, D., and Bishop, J. (2015). MRDS: Mark-Recapture Distance Sampling. pp. (MRDS): R Package.
Laake, J. L., and Borchers, D. L. (2004). Methods for Incomplete Detection at Distance Zero. Oxford: Oxford University Press.
Laist, D. W., Knowlton, A. R., Mead, J. G., Collet, A. S., and Podesta, M. (2001). Collisions between ships and whales. Mar. Mamm. Sci. 17, 35–75. doi: 10.1111/j.1748-7692.2001.tb00980.x
Laist, D. W., Knowlton, A. R., and Pendleton, D. (2014). Effectiveness of mandatory vessel speed limits for protecting North Atlantic right whales. Endang. Spec. Res. 23, 133–147. doi: 10.3354/esr00586
Miller, D. L., Burt, M. L., Rexstad, E. A., and Thomas, L. (2013). Spatial models for distance sampling data: recent developments and future directions. Methods Ecol. Evol. 4, 1001–1010. doi: 10.1111/2041-210X.12105
Noad, M. J., Dunlop, R. A., Bennett, L., and Kniest, H. (2016). Abundance estimates of the east Australian humpback whale population (BSE1): 2015 survey and update. Paper Presented at SC/66b/SH21 Submitted for Consideration by the IWC Scientific Committee, Impington.
Noad, M. J., Dunlop, R. A., Paton, D. A., and Cato, D. H. (2008). “An update of the east Australian humpback whale population (E1) rate of increase. Paper Presented at SC/60/SH31 Submitted for Consideration by the IWC Scientific Committee, Impington.
Pack, A. A., Herman, L. M., Craig, A. S., Spitz, S. S., Waterman, J. O., Herman, E. Y. K., et al. (2018). Comparing depth and seabed terrain preferences of individually identified female humpback whales (Megaptera novaeangliae), with and without calf, off Maui, Hawaii. Mar. Mamm. Sci. 34, 1097–1110. doi: 10.1111/mms.12495
Panigada, S. G., Pesante, G., Zanardelli, M., Capoulade, F., Gannier, A., and Weinrich, M. T. (2006). Mediterranean whales at risk from fatal ship strikes. Mar. Pollut. Bull. 52, 1287–1298. doi: 10.1016/j.marpolbul.2006.03.014
Peel, D., Smith, J. N., and Childerhouse, S. (2018). Vessel strike of whales in Australia: the challenges of analysis of historical incident data. Front. Mar. Sci. 5:69. doi: 10.3389/fmars.2018.00069
PGM Environment (2012). Great Barrier Reef Shipping: Review of Environmental Implications. Safety Bay, WA: PGM Environment.
Queensland Government Statistician’s Office (2017). Trade data – Overseas Exports by Port of Loading, Commodity (3-digit SITC revision 3) and Country of Destination, Queensland and Australia, 2006–07 to 2016–17. Brisbane City QLD: Queensland Government Statistician’s Office.
R Development Core Team (2015). R: A Language and Environment for Statistical Computing. Vienna: R Foundation for Statistical Computing.
Redfern, J. V., Mckenna, M. F., Moore, T. J., Calambokidis, J., Deangelis, M. L., Becker, E. A., et al. (2013). Assessing the risk of ships striking large whales in marine spatial planning. Conserv. Biol. 27, 292–302. doi: 10.1111/cobi.12029
Ross-Gillespie, A., Butterworth, D. S., and Johnston, S. J. (2015). Assessment Results for Humpback Breeding Stocks D, E1 and Oceania Following Recommendations from SC 65a. Korea: IWC Scientific Committee.
Silber, G. K., Vanderlaan, A. S. M., Arceredillo, A. T., Johnson, L., Taggart, C. T., Brown, M. W., et al. (2012). The role of the international maritime organization in reducing vessel threat to whales: process, options, action and effectiveness. Mar. Policy 36, 1221–1233. doi: 10.1016/j.marpol.2012.03.008
Smith, J. N., Grantham, H. S., Gales, N., Double, M. C., Noad, M. J., and Paton, D. (2012). Identification of humpback whale breeding habitat in the great barrier reef. Mar. Ecol. Prog. Ser. 447, 259–272. doi: 10.3354/meps09462
Tournadre, J. (2014). Anthropogenic pressure on the open ocean: the growth of ship traffic revealed by altimeter data analysis. Geophys. Res. Lett. 7924–7932. doi: 10.1002/2014GL061786
UNCTAD (2018). 50 Years of Review of Maritime Transport, 1968–2018 - Reflecting on the Past, Exploring The Future. Geneva: UNCTAD.
UNCTAD (2019). Review of Maritime Transport 2018. Review of Maritime Transport (RMT). Geneva: UNCTAD.
UNESCO (2012). Mission Report Reactive Monitoring Mission to Great Barrier Reef (Australia). WHC-12/36.COM. Available at: http://whc.unesco.org/en/list/154/documents/
Vanderlaan, A. S. M., and Taggart, C. T. (2006). Vessel collisions with whales: the probability of lethal injury based on vessel speed. Mar. Mamm. Sci. 23, 144–156. doi: 10.1111/j.1748-7692.2006.00098.x
Vanderlaan, A. S. M., and Taggart, C. T. (2009). Efficacy of a voluntary area to be avoided to reduce risk of lethal vessel strikes to endangered whales. Conserv. Biol. 23, 1467–1474. doi: 10.1111/j.1523-1739.2009.01329.x
Wiley, D., Thompson, M., Pace, R., and Levenson, J. (2011). Modeling speed restrictions to mitigate lethal collisions between ships and whales in the Stellwagen bank national marine sanctuary USA. Biol. Conserv. 144, 2377–2381. doi: 10.1016/j.biocon.2011.05.007
Williams, R., Hedley, S. L., Branch, T. A., Bravington, M. V., Zerbini, A. N., and Findlay, K. P. (2011). Chilean blue whales as a case study to illustrate methods to estimate abundance and evaluate conservation status of rare species. Conserv. Biol. 25, 526–535. doi: 10.1111/j.1523-1739.2011.01656.x
Keywords: spatial risk assessment, ship strike, great barrier reef, humpback whale, shipping, AIS, breeding ground
Citation: Smith JN, Kelly N, Childerhouse S, Redfern JV, Moore TJ and Peel D (2020) Quantifying Ship Strike Risk to Breeding Whales in a Multiple-Use Marine Park: The Great Barrier Reef. Front. Mar. Sci. 7:67. doi: 10.3389/fmars.2020.00067
Received: 15 June 2019; Accepted: 29 January 2020;
Published: 14 February 2020.
Edited by:
Ellen Hines, San Francisco State University, United StatesReviewed by:
Danielle Kreb, Conservation Foundation for Rare Aquatic Species of Indonesia, IndonesiaKylie L. Scales, University of the Sunshine Coast, Australia
Copyright © 2020 Smith, Kelly, Childerhouse, Redfern, Moore and Peel. This is an open-access article distributed under the terms of the Creative Commons Attribution License (CC BY). The use, distribution or reproduction in other forums is permitted, provided the original author(s) and the copyright owner(s) are credited and that the original publication in this journal is cited, in accordance with accepted academic practice. No use, distribution or reproduction is permitted which does not comply with these terms.
*Correspondence: Joshua N. Smith, am9zaHVhLnNtaXRoQHVxY29ubmVjdC5lZHUuYXU=; am9zaHVhLnNtaXRoQG11cmRvY2guZWR1LmF1
†Present address: Natalie Kelly, Australian Antarctic Division, Hobart, TAS, Australia Thomas J. Moore, CSS, Inc., Office for Coastal Management, National Ocean Service, NOAA, Seattle, WA, United States