- 1Department of Bioscience and Biotechnology, National Taiwan Ocean University, Keelung City, Taiwan
- 2Institute of Marine Environment and Ecology, National Taiwan Ocean University, Keelung City, Taiwan
- 3Center of Excellence for the Oceans, National Taiwan Ocean University, Keelung City, Taiwan
- 4School of Veterinary Medicine, National Taiwan University, Taipei, Taiwan
- 5Department of Biological Sciences and Technology, National University of Tainan, Tainan, Taiwan
Bacterial infectious diseases cause a huge economic loss in aquaculture. Active biological control that uses bacteriovorous organisms to remove pathogens is an ecologically friendly approach for the cultural system to counteract the bacterial infection. The ciliate is one of the main predators of bacteria in aquatic ecosystems, but whether it can be effectively adopted to protect aquaculture organisms from bacterial pathogens remains to be investigated. In this study, we optimized the culturing method for a marine ciliate Strombidium sp. NTOU1 and analyzed its bacteriovorous properties. Strombidium sp. NTOU1 could feed on a variety of bacteria including pathogenic species. By controlling the amount of frozen bacteria Erwinia spp. in the medium, the ciliate grew to the maximum density within 4 days and could reach 1.2 × 105 cells/mL after the suction filtration enrichment. Ingested bacteria were observed in the food vacuole of the ciliate, and the average bacterial clearance rate of a single NTOU1 cell was ∼300 cells/hr. In the challenge trial which grouper larvae were exposed to an extreme environment containing a high density of the pathogen Vibrio campbellii, only 33% of the grouper larvae could survive after 5 days. However, preincubating with Strombidium sp. NTOU1 for an hour resulted in their survival rate to rise to 93%. Together, our results demonstrated that Strombidium sp. NTOU1 has the potential to become a biological control species to actively remove pathogens in aquaculture. In addition, the technical improvement to culture Strombidium sp. NTOU1 provides an advantage for this ciliate in the future academic research or biotechnological application.
Introduction
As global capture fisheries resources are gradually depleted, aquaculture has become an important source of high-quality animal proteins to meet the need of the world’s continuously growing population (Goldburg and Naylor, 2005; Brander, 2007). Modern intensive aquaculture improves production, but it also increases the risk of infectious disease outbreaks, which cause an economic loss of US$6 billion per year for the aquaculture industry (Bostock et al., 2010; Oliva-Teles, 2012; FAO, 2018). Among fish diseases, bacterial infection is the biggest threat.
To date, antibiotics are still the most effective way to fight bacterial infectious diseases, but their excessive use in aquaculture has become a serious problem (Cabello, 2006; Defoirdt et al., 2011). The abuse of antibiotics induces the resistance of bacterial pathogens in the environment, indirectly causing the prevalence of super bacteria, which in turn threatens human health (Sapkota et al., 2008; Heuer et al., 2009). In addition, antibiotics also harm probiotics in the environment and the intestine of animals, leading to an imbalance in microbial ecology that contributes to the instability of the aquaculture system (Martinez, 2011). Many countries have reached a consensus to ban the use of antibiotics in aquatic animals. Alternative methods, which can effectively control the infectious disease but also maintain the ecological balance of the culture, are urgently on demand (Pérez-Sánchez et al., 2018).
Biological control is using natural enemies of a harmful organism in the ecosystem to control its population density and to prevent the occurrence of harm. Passive biological control in aquaculture is to create an adverse environment for the pathogen, which, in turn, facilitates the prevention of the disease. For example, probiotics compete with bacterial pathogens for the resources needed for their growth (Verschuere et al., 2000). Probiotics and microalgae have also been reported to secrete antibacterial and immune-enhancing substances, which indirectly reduce the damage caused by bacterial pathogens (Muller-Feuga, 2000; Nayak, 2010). In general, passive biological control can prevent the growth of bacterial pathogens, but it is not able to completely avoid the outbreak of the disease. On the other hand, active biological control is aimed at directly removing harmful organisms from the environment. For example, the bacteriophage can be used to infect and destroy pathogenic bacteria to prevent disease outbreaks (Letchumanan et al., 2016). Nevertheless, it can only selectively eliminate the specific host, and it has yet to find a bacteriophage that possesses the ability to fight against diverse bacterial pathogens in the actual aquaculture environment (Kalatzis et al., 2018).
In addition to bacteriophages, ciliates are the main predators of bacteria in the microbial ecosystem (Sherr and Sherr, 2002; Becks et al., 2005). Bacteriovorous ciliates, such as Halteria grandinella, Paramecium caudatum, Tetrahymena pyriformis, and Tetrahymena vorax, were reported to have the ability to prey on microbes not restricted to any type of bacteria through the non-selective phagocytosis (Grønlien et al., 2002; Ali and Saleh, 2014). Therefore, ciliates have been applied to lower the bacterial density in the aquatic environment. For example, Stentor roeselii was used to control the bloom caused by the cyanobacteria Microcystis aeruginosa in freshwater (Kim et al., 2007). In an outdoor algal cultivation system, the Colpoda sp. was deployed to protect the green algae Chlorella sp. from the bacterial contamination dominated by Microbacterium sp. (Cho et al., 2019). However, applying a ciliate to antagonize bacterial pathogens in aquaculture has not yet been reported. It is probably because suitable ciliate species, which can be practically cultured on a large scale and have a property ideal for field utilization in aquaculture, remain largely underexplored.
An oligotrich ciliate, termed Strombidium sp. NTOU1 hereby, was isolated from the coastal water of Taiwan (Lee, 2015). As an ecologically successful species, it may have advantageous properties potentially adaptable for human utilization, such as a broad bacteriovorous ability. In this study, we established a robust and efficient culturing method for the Strombidium sp. NTOU1 and characterized its feeding and ability of bacterial clearance. The result from pathogen challenging assay demonstrated the effectiveness to use this ciliate as an active biological control species for grouper larvae to against Vibrio campbellii.
Materials and Methods
Chemicals, Enzymes, and Antibodies
All general chemicals used in this work, including nutrient salts and antibiotics, were purchased from Sigma-Aldrich Chemical Co. (St. Louis, MO, United States). PCR related reagents were provided from Ten Giga Bio (Keelung, Taiwan). Restriction enzymes were obtained from New England Biolabs (Ipswich, MA, United States). Immunostaining related reagents were purchased from Thermo Fisher Scientific Inc. (Wilmington, DE, United States).
Source of Bacterial Feeds
There are six strains of bacteria used in this study (Supplementary Table S2). Escherichia coli BL21 strain (DE3) was purchased from New England Biolabs. Vibrio campbellii and Vibrio harveyi were provided by Dr. Han-You Lin’s laboratory at National Taiwan University (Wang et al., 2010; Lam et al., 2011). The Erwinia spp. and Kluyvera spp. were isolated from the rice medium (see below) made of 5 grains of raw rice in 500 mL of artificial seawater to enrich the natural bacterial populations. The identification of these bacterial species was in accordance with the API-20E commercial kit following the manufacturer’s instructions.
To create the pET-EGFP E. coli strain, the enhanced green fluorescence protein (EGFP) DNA fragment was amplified by PCR (Supplementary Table S1) from the pCX-EGFP plasmid (Addgene, Watertown, MA, United States) and then ligated into the pET28a vector (Addgene) between the restriction enzyme sites of EcoRI and XhoI to yield the pET28-EGFP plasmid. Subsequently, the pET28-EGFP plasmid was transformed into E. coli BL21 strain to obtain the genetically engineered E. coli pET-EGFP strain. 0.2 mM isopropyl β-D-1-thiogalactopyranoside (IPTG) was added in the log phase (OD600 = 0.4−0.6) cells for 5 h to induce the production of EGFP recombinant proteins.
The cell density of each bacterial strain is obtained from an actual number of colonies after plating. All bacteria frozen stocks were prepared by overnight cultured bacterial suspension containing 20% glycerol and then stored in a −80°C refrigerator.
Source and General Maintenance of the Ciliate
The marine oligotrich ciliate used in this study, termed Strombidium sp. NTOU1, was initially isolated in Prof. Kuo-Ping Chiang’s laboratory (Lee, 2015). Wild ciliates were collected using a 20-μm mesh plankton net from the coastal waters of northeastern Taiwan (25° 08′ 30″ N; 121° 47′ 42″ E) on September 8, 2011. A single-cell-isolated strain was obtained and identified as a Strombidium sp. based on the morphological analysis using the protargol impregnated method (Ng and Nelsen, 1977).
The primary culture and general preservation of Strombidium sp. NTOU1 was carried out with the rice medium at a starting concentration of 1 cell/mL. The rice medium is an unboiled infusion filled with naturally grown bacterial feed and was prepared by adding one grain of raw rice to 100 mL of artificial seawater (ASW) and standing overnight at 25 °C.
High-Density Culture and Handling of Strombidium sp. NTOU1
The high-density culture of ciliates took place in 5 liters of sterile ASW with a starting density of 100 cells/mL. During the culturing period, ciliate cells were harvested daily for proper serial dilution and cell counts. The food source, frozen Erwinia spp., was daily applied to the culture flask in an amount of 10,000 times the number of ciliates. For the preparation of the frozen Erwinia spp., ∼5.00 × 1010 cells were washed twice with sterile artificial seawater after centrifugation (3,000 g, 10 min, 4°C) and stored in the form of pellet at −20°C. The bacterial density of frozen Erwinia spp. was adjusted using sterile ASW. After 5-day culture, these ciliates were further subjected to the suction filtration enrichment using the water-jet aspirator pump (A-1000S, Eyela, Tokyo, Japan) at a pressure of 5 cm/Hg with a pore size of 10 μM filter membrane (Whatman, Buckinghamshire, United Kingdom) to further concentrate the ciliate culture solution to a volume of 500 mL.
To determine the cell density of Strombidium sp. NTOU1, 10 mL of the culture medium were sampled into a six-well plate and 0.5 mL of Lugol’s regent (final concentration 5%) was added for cell fixation. After the cells were serially diluted to the appropriate concentration, the total number of ciliates in the well was counted using a dissecting microscope (ZM-160A, Optima, Taichung, Taiwan) at a magnification of 40×. The number of ciliates in per milliliter of culture was calculated according to the counted numbers in the 10-mL culture and the folds of dilution.
Palatability and Clearance Rate for Strombidium sp. NTOU1 on Different Bacteria
Before the experiment, ciliates were moved into the sterile ASW for 24 h after 5 times of washes. Thereafter, 5 ciliates were randomly picked and inspected under the microscope to confirm that no obvious food vacuole was present in the cell.
For measuring the ciliate growth rate when feeding different bacteria, 100 ciliates were first moved into flasks with 100 mL sterile ASW using a mouth pipette under a dissecting microscope (Optima). Each bacteria strain was subsequently added into the flask to reach the concentration of 2.00 × 107 CFU/mL, respectively. The cell density of ciliates was calculated every 24 h during the 3-day culture. Then, the growth rates (μ; day–1) were calculated as μ = ln(Nt/N0)/dt (Montagnesa and Lessard, 1999).
In this equation, N0 and Nt are represent the initial cell number and cell density after culture, where dt is the time of culture. The above palatability test contained multiple independent setup groups, which was suitable for analyzing the differences among population mean values, such as one-way analysis of variance (ANOVA). Significance was accepted with p <0.05.
The palatability of frozen bacterial fodder for Strombidium sp. NTOU1 was carried out in culture flasks with 100 mL sterilized ASW, with 2.00 × 107 CFU/mL live Erwinia spp. or frozen-treatment bacteria, respectively. The initial number of ciliates added to the culture flask was 100 cells/mL and ciliate concentrations were counted every 24 h for the next 3 days. Student’s t-test was used to compare the mean of the two groups (corresponding to the control group) for statistical analysis.
The bacteria clearance rate of Strombidium sp. NTOU1 was measured using a bacterial strain that emits fluorescence and a pathogenic species, respectively. To visualize and to quantify the ingestion of bacteria, 50 and 150 cells of ciliates were inoculated in 3 mL of sterile artificial seawater and starved for 24 h followed by adding ∼3.75 × 105 cells of the E. coli pET-EGFP strain, which provided ∼3,000 fluorescence intensity. The fluorescence intensity (excitation/emission: 475/575, sensitivity 100) of each sample was measured using the SynergyMx microplate fluorescence reader (Biotek, Winooski, VT, United States) every hour for 12 h. In the same period, samples from the experiment with 150 ciliates feeding on fluorescent bacteria were taken at 1, 2, 4, and 6 h for observation using the BX60 fluorescence microscope (Olympus, Tokyo, Japan) at 1,000×magnification.
The clearance test of V. campbellii was carried out in a flask containing 20 mL of sterile ASW with 2.00×106 CFU/mL of vibrio cells and/or 5,000 cells/mL of Strombidium sp. 100 μl of the sample containing the ciliate or vibrio was taken every 20 min for 1 h for plate count to estimate the residual concentration of the bacteria.
Immunofluorescence Staining
Ciliate samples at the density of 100 cells/mL were washed 5 times with sterile artificial seawater. 200 ciliate cells were transferred to a coverslip by a mouth pipette under a dissecting microscope (Optima). An equal volume of 2× fixatives regent (8% paraformaldehyde, 0.1% Triton X 100 in PHEM buffer, pH 6.9) were added and allowed for air drying, following by soaking the coverslip with cells in PBS-0.1% Tween (PBST) and blocking solution (3% bovine serum albumin with PBST). The fixed cells were stained with the alpha-tubulin monoclonal antibody (DM1A, 1:1000), Alexa Fluor 568-conjugated anti-mouse secondary antibody (1:2000), and DAPI staining solution (10 ng/mL). The coverslip was counted with 10 μL of DABCOTM onto the glass slide. All specimens were stored in a dark box at 4°C and observed with a fluorescence microscope (Olympus) at a magnification of 1,000 times.
Strombidium sp. NTOU1 in the Protection of Grouper Larvae
The grouper Epinephelus coioides larva about 5–8 cm in length were used to be the protective object in the challenge experiment. The pathogen V. campbellii was cultured in TSB medium with 3% NaCl to reach an OD600 of 1.00 and washed twice with aerated seawater before use. At the beginning of the protection test, three biological combinations were first adapted to a culture tank with 5 L of aerated seawater at room temperature for an hour, including a group of without additional bacteria, 3.00×106 CFU/mL of V. campbellii alone, and the same number of vibrio co-cultured with 5,000 cells/mL of Strombidium sp. NTOU1. Next, added 5 grouper larvae to each tank for a 1-h challenge test. After treatment, each fish was rinsed with 500 mL of aerated seawater and returned to the culture tank with 8 L of aerated seawater. These grouper larvae were monitored for 5 days and fish mortality were calculated every 24 h.
Groupers are high-commercial value fish species in Taiwan aquaculture and are well-considered challenge trial test species. To avoid cannibalism in the larva stage, a suitable culture density <1 fish/L or less was used in the challenge test to mimic the real culture condition. The same density was used in our challenge test to mimic the real culture condition. The challenge was repeated three times to bring up the total number to 15 larvae for each group, and we realized that under such experimental settings, individual differences in the immune response of each batch of grouper larvae did exist and might affect the statistical results. A nonparametric statistical analysis (Kruskal-Wallis H-test) was applied in the dataset of 3 challenge experiments. Using this method to convert the measured values into a ranking order, the difference in survival rates between three groups can be analyzed.
Results
Strombidium sp. NTOU1 Feeds on a Variety of Bacterial Species in the Laboratory
Strombidium sp. NTOU1 could be cultured in the laboratory using the unboiled rice medium made of rice grains and artificial seawater (ASW) (Lee, 2015; Supplementary Figure S1). To distinguish whether the growth of the ciliate is dependent on the organic substance released from the rice grain and/or on the bacteria sustained in the rice medium, an additional preparation of the same infusion was autoclaved for the comparison. The ciliate cultured in the rice medium reached a growth rate at 0.215 day–1 but it did not grow in the sterilized rice medium (Figure 1). This result indicates that the bacterium from the rice grain and proliferating in the medium is required to provide Strombidium sp. NTOU1 with nutrients for survival.
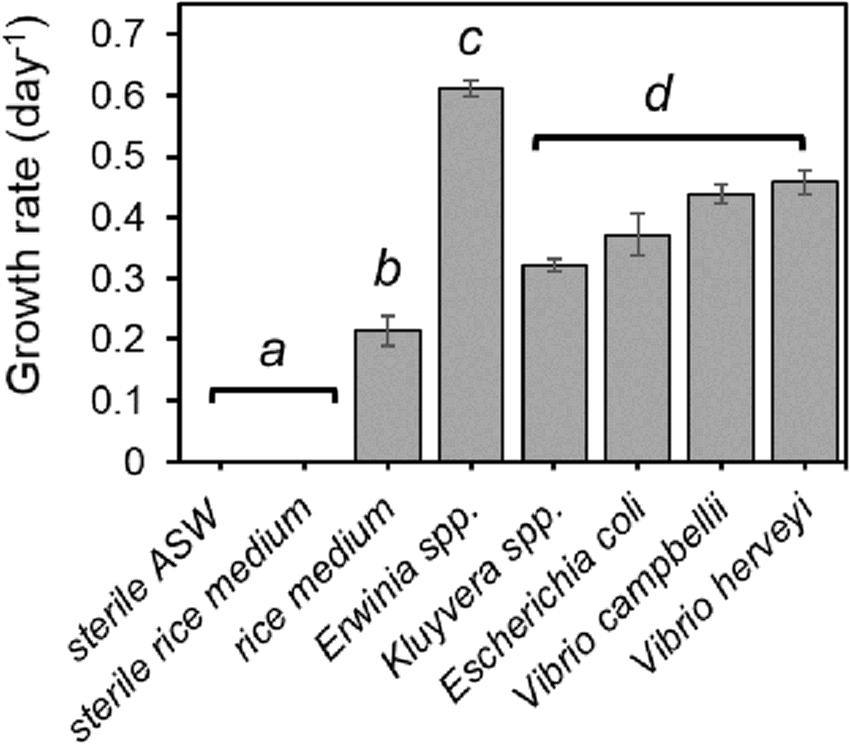
Figure 1. The specific growth rate of Strombidium sp. NTOU1 fed with different bacteria. The error bar represents the standard deviation (SD) of the average from three independent experiments. The statistical significance of the growth rate between each group was analyzed by the ANOVA. Different letters (a, b, c, and d) indicate a significant difference (p <0.05) between different treatments.
Two main bacteria, an Erwinia spp. and a Kluyvera spp., were isolated from the rice medium. In order to verify whether these bacteria could be the nutrient source for Strombidium sp. NTOU1, the ciliate growth was monitored after either bacterium was added into the sterile ASW, respectively. The result confirmed that feeding either of the two bacteria alone was sufficient to culture the ciliate (Figure 1). To further investigate the bacteriovorous preference of Strombidium sp. NTOU1, other bacteria commonly found in the aquaculture environment, including Escherichia coli, Vibrio harveyi, and Vibrio campbellii, were also tested as the sole food source. At the initial bacterial concentration of 2.00×107 CFU/mL, all the bacteria we tested were able to sustain the culture of Strombidium sp., but the growth rate of ciliates was significantly higher when they were fed with the Erwinia spp. than in other conditions (Figure 1). Together, these results demonstrated that Strombidium sp. could utilize a variety of bacterial species as the food source, and the Erwinia spp. isolated from the rice medium is preferential in our culturing condition.
Establishment of a Standardized Procedure to Culture and Enrich Strombidium sp. NTOU1 in Large Scale
To optimize the utilization of bacterial food to culture Strombidium sp. NTOU1, Erwinia spp. received a pre-freezing treatment that allowed its batch-wise preparation and storage to reduce the possible variation in the nutritional status. As shown in Figure 2, freeze-treated Erwinia spp., similar to live bacteria, could be utilized to culture Strombidium sp. NTOU1 although the growth rate of ciliates was slightly reduced in 3 days (Δμ <0.05). This result indicated that the frozen Erwinia could be adopted as an amenable alternative of ciliate food.
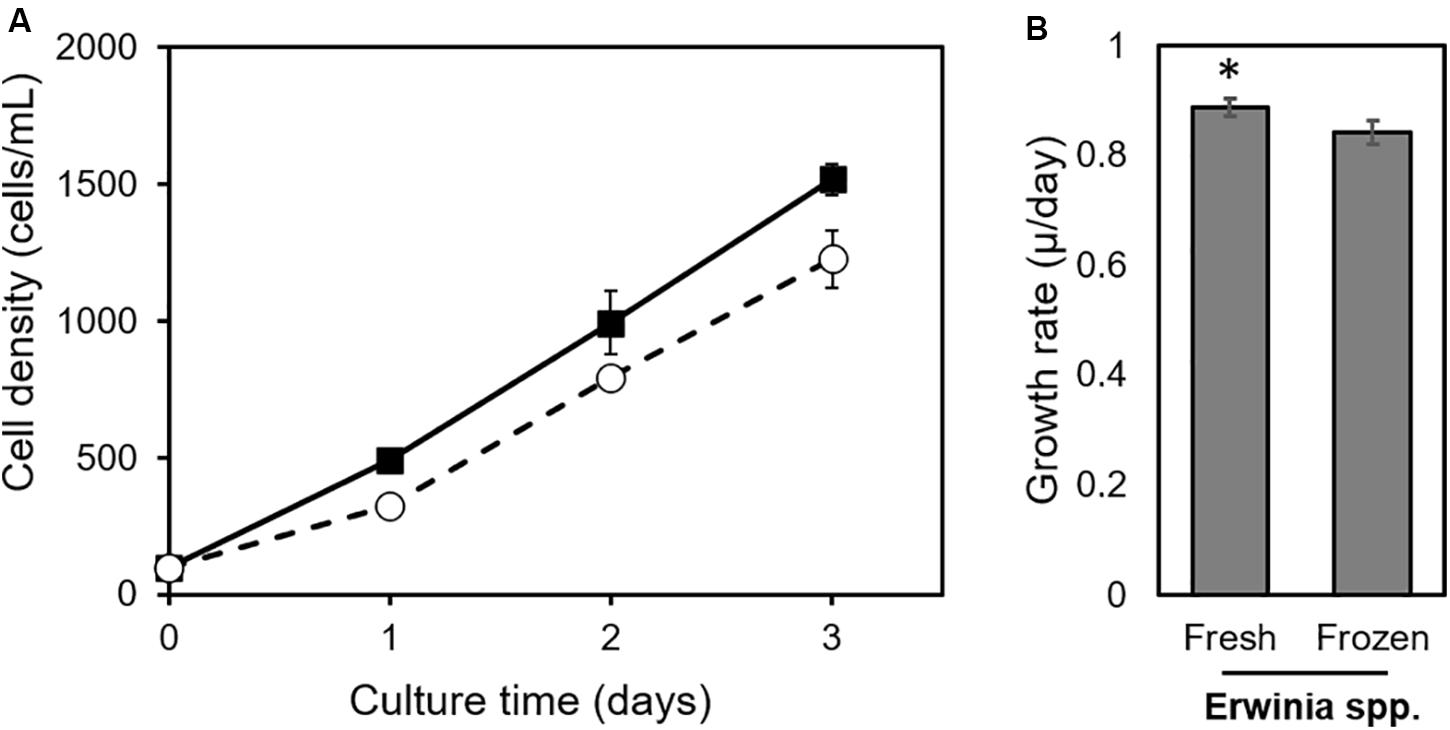
Figure 2. Growth of ciliates fed with fresh or frozen Erwinia spp. (A) Cell density of Strombidium spp. NTOU1. Black rectangles, fed with fresh Erwinia spp. fodder; white circles, fed with frozen bacterial feeds. (B) Average growth rate of Strombidium spp. NTOU1. The asterisk indicates a significant difference between two groups (Student’s t-test, p <0.05). The error bars represent the standard deviations of the average from three independent experiments.
Based on previous observation, it is noticed that excessive Erwinia spp. in the culture environment, especially when the initial ciliate density is low, might affect the growth rate of Strombidium sp. NTOU1 (Figures 1, 2B). To minimize the number of environmental bacteria while maintaining enough nutrients for ciliates, a batch feeding approach was tested using less initial amounts of bacteria but adding the supplement daily. When Strombidium sp. NTOU1 was fed the daily bacteria dose about 10,000-fold of the ciliate number (less than 1/3 of the initial bacterial amount in the prior experiment), the ciliates with a starting density of 100 cells/mL were able to be raised to 1.07×104 cells/mL, approximately a hundred folds, through this batch feeding method in 4 days (Figure 3, μ = 1.21 ± 0.033).
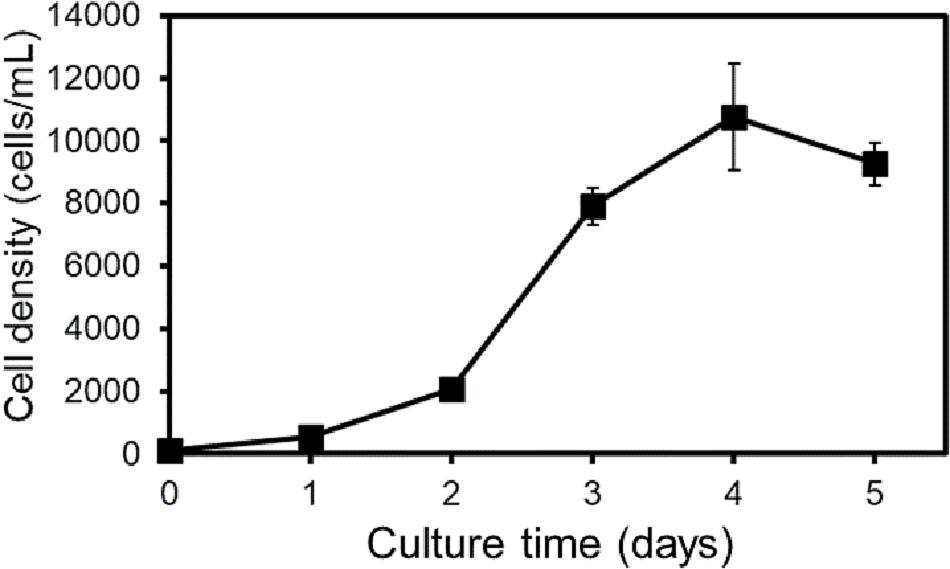
Figure 3. Continuous culture of Strombidium sp. NTOU1 with frozen Erwinia spp. A continuous culture was carried out by adding 10,000 times the ciliate number of frozen Erwinia spp. as a food source with an initial ciliate density of 100 cells/mL. The error bar represents the SD of the average from three independent experiments.
In parallel, a suction filtration procedure was tested to enrich the ciliate density. Through a mild pressure control to effectively reduce the volume of culture medium, the concentration of the ciliates could be further increased by about 10 folds to reach 1.20×105 cells/mL. Microscopy observation showed that Strombidium sp. NTOU1 remained motile, and they could continue to grow in the subsequent culturing, suggesting that the suction filtration caused limited physical damages and the ciliate could recover from the enrichment process. In the further assessment, the cells were stained against alpha-tubulin, which is one of the major cytoskeleton proteins in the ciliate. The immunofluorescence observation also confirmed that the cilia structure of Strombidium sp. was not significantly impaired after our culture and enrichment processes (Figure 4). All these results demonstrated that we have established a feasible culturing and handling procedure in the laboratory.
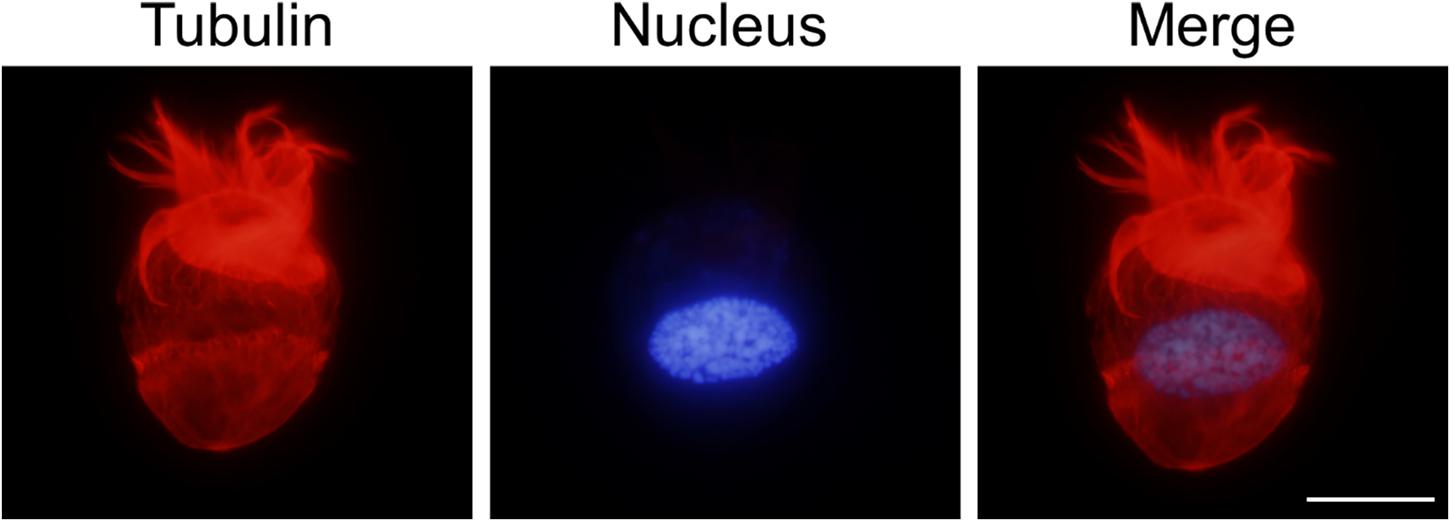
Figure 4. Immunofluorescent staining of Strombidium sp. NTOU1. The microtubule structure and nucleus of Strombidium sp. NTOU1 were labeled using the anti-alpha tubulin antibody (red) and DAPI stain (blue), respectively. Scale bar, 50 μm.
Strombidium sp. NTOU1 Could Ingest and Remove Bacteria in the Culture Efficiently
To further characterize the ability of ciliates to ingest bacteria, the bacterial clearance rate of Strombidium sp. NTOU1. was estimated using a fluorescent E. coli pET-EGFP strain, in which an exogenous plasmid carrying the EGFP gene expression cassette was introduced to overexpress the traceable and quantifiable green fluorescent protein (GFP). After Strombidium sp. were starved for 24 h in advance to ensure no residual bacteria inside the ciliate cell, the ciliates were incubated with 3.75×105 cells of the E. coli pET-EGFP strain and the GFP fluorescent intensity in the culture was measured.
Without co-incubation with the ciliate, the fluorescence intensity of E. coli pET-EGFP strain in the ASW culture did not markedly change for 12 h (Figure 5A). When 50 or 150 cells of Strombidium sp. NTOU1 were added, however, the fluorescence decreased after 1 hr and the intensity continued to drop. Within 6 hrs, 150 cells of the ciliate could eliminate the fluorescent bacteria to reach an equilibrium state and to reduce the fluorescence intensity by ∼75.5 % (Figure 5A). Incubation with 50 ciliate cells also could lower the GFP fluorescence in the culture with a slower kinetic change. During this period, the fluorescent microscopy observation revealed that fluorescence signals were accumulated into multiple 2-μm spheres in the ciliate cell, presumably the food vacuole of Strombidium sp., after 1 hr (Figure 5B). The number of food vacuoles in the ciliate, along with the vacuolar fluorescent intensity, gradually decreased within 6 hrs (Figure 5). Together, these results indicated that a significant fraction of pET-EGFP E. coli has been ingested and digested by the ciliate and removed from the culture. Calculating the number of fluorescent bacteria reduced during this period shows that the average clearance rate of each ciliate for fluorescent bacteria is 315 cells/hr.
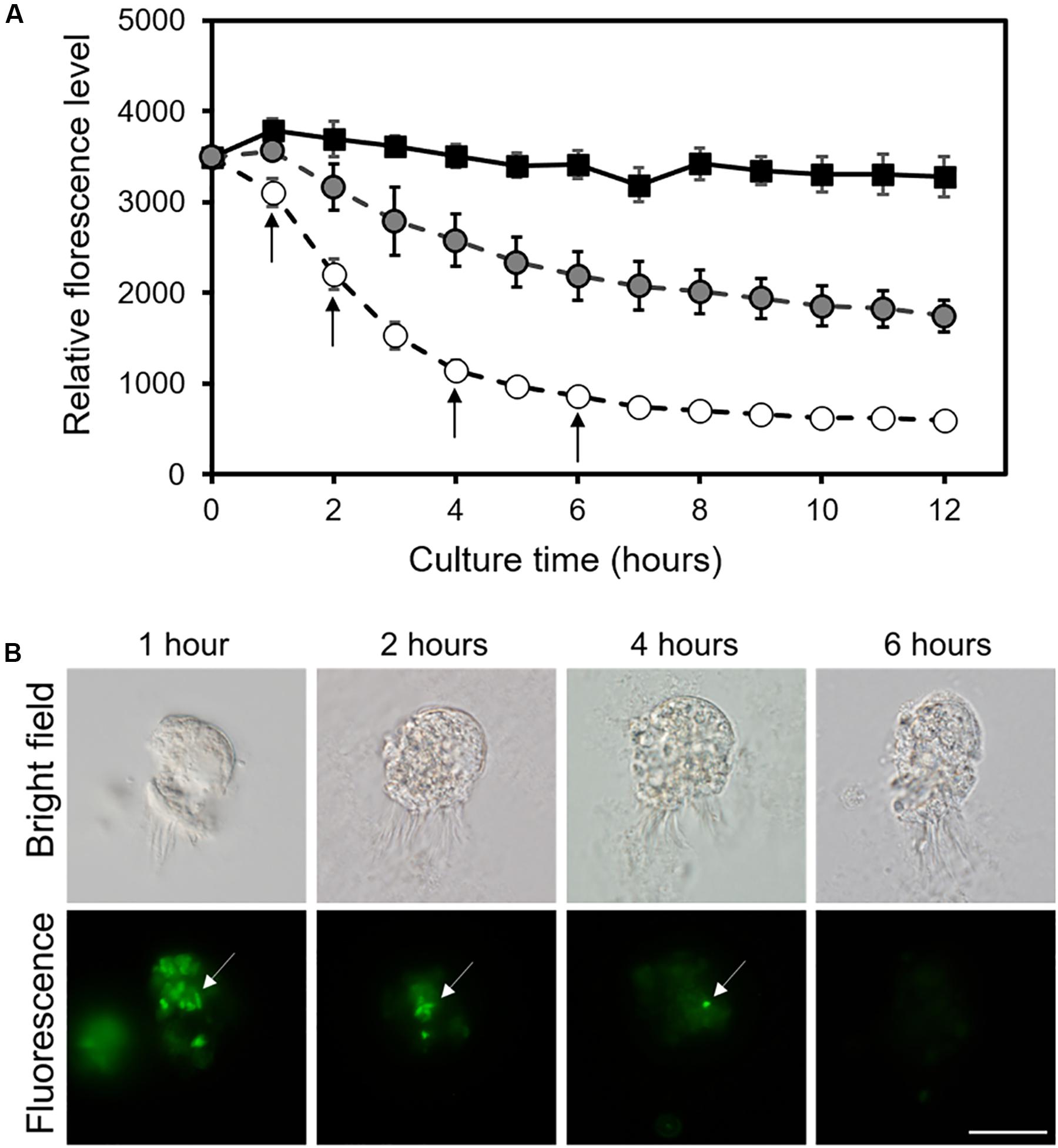
Figure 5. The intensity and localization of the fluorescence from E. coli pET-EGFP strain after its feeding to Strombidium spp. NTOU1. (A) The fluorescent intensity of the E. coli pET-EGFP strain alone (black square) and E. coli with incubation with 50-cell (gray circle) or 100-cell (white circle) of ciliates at different time points. The error bar represents the SD of the average from three independent experiments. The black arrow indicates the time point for fluorescent microscopy observation. (B) Localization of fluorescent bacteria in the ciliate cell after 1, 2, 4, and 6 h of incubation. The white arrow indicates the food vacuole of Strombidium sp. NTOU1. Scale bar, 50 μm.
Strombidium sp. NTOU1 Could Markedly Eliminate Vibrio in a Simulated Environment
That Strombidium sp. NTOU1 could lower the bacterial density in the culture prompted us to explore the potential to utilize this ciliate for biological control of marine pathogens in aquaculture. In order to verify whether Strombidium sp. NTOU1 possessed the ability to fight pathogenic bacteria in the actual environment, the bacterial clearance was measured under a milliliter-scale condition that mimicked the aquaculture environment. Aerated aquaculture seawater which contained 3,000–5,000 CFU/mL of bacteria (Figure 6), was chosen as the assaying background. In addition, based on the LC50 of the marine vibrio V. campbellii for grouper larvae reported in previous studies (Lam et al., 2011; Noor et al., 2019), a condition with a high dose of pathogens was set up to simulate the extreme situation in which vibrio infectious diseases have erupted.
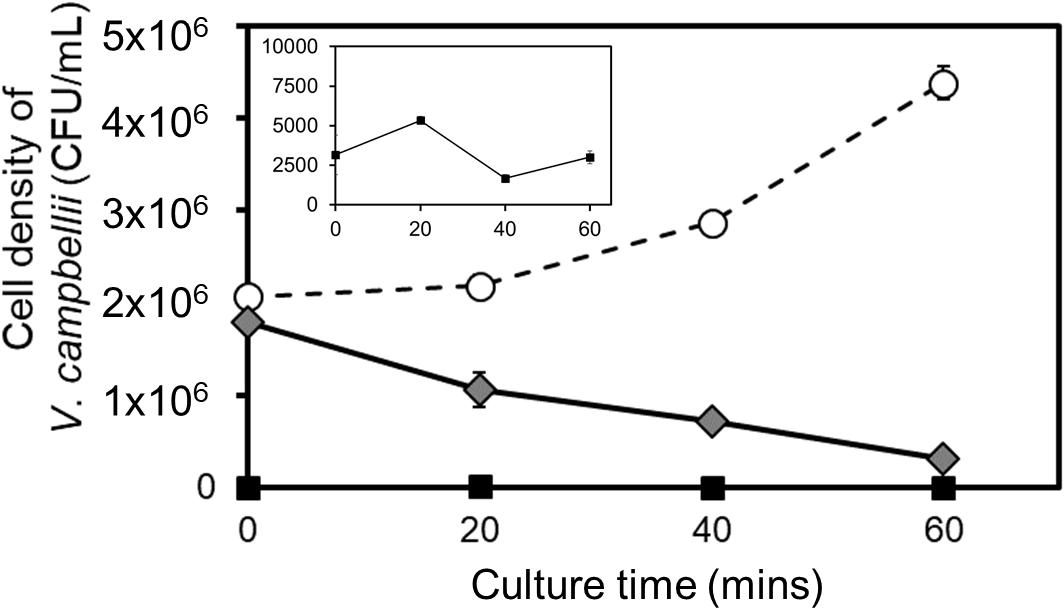
Figure 6. Bacterial clearance by Strombidium spp. NTOU1. Black squares (also shown in the inset using an adjusted scale for clarity) indicate the number of bacteria in the aerated seawater as the background controlled group. White circles indicate the cell density of V. campbellii cultured alone. Gray diamonds represent the bacterial density after 5,000 cells/mL of ciliates were added. The error bar represents the S. D. of the average from three independent experiments.
In the background environment, the bacterial concentration was slightly fluctuated but remained at a low level ∼103 CFU/mL. When V. campbellii was inoculated at a starting concentration of 2.00×106 CFU/mL, the pathogen further increased for more than one-fold to reach 4.38×106 CFU/mL in the aerated seawater (Figure 6). Adding 5,000 cells/mL of Strombidium sp. to such an environment, however, rapidly lowered the bacterial density within 20 mins and eliminated 80% of the bacteria in an hour (Figure 6). This result argues that it is possible to protect aquaculture fish from the vibrio infection using Strombidium sp. NTOU1.
Incubation With Strombidium sp. NTOU1 Lowered the Fish Lethality Caused by Vibrio Infection
To investigate whether Strombidium sp. NTOU1 could effectively alleviate the damage of fish caused by the actual vibrio infection, a pathogen challenge assay was performed using the grouper Epinephelus coioides, an economically important aquaculture fish in Taiwan, as the testing species. No vibrio, a high-dose of vibrio, or a high-dose of vibrio co-incubated with Strombidium sp. were, respectively, adapted into a tank with 5 L aerated water for an hour. 5 grouper larvae were added to those tanks and returned to normal condition after 1 h of the vibrio infection, and the fish mortality were monitored for 5 days.
Without challenging with vibrio (the control), grouper larvae were able to survive in aerated seawater without any mortality (Table 1). Once infected with V. campbellii at 3×106 CFU/mL, grouper larvae started to die after the 2nd day even after they returned to normal aerated seawater. The overall mortality rate was 66.67% after 5 days (Figure 7). In such an extreme environment, however, if additional 5,000 cells/mL of ciliates were co-cultured with the vibrio for an hour, the residual pathogen could not effectively infect the grouper larvae. In three sets of independent assays, only one grouper larva died on the 5th day after the challenge when they were protected by Strombidium sp., and the survival rate of groupers increased to 93.33% (Figure 7 and Table 1). These experiments demonstrated the potential of Strombidium sp. NTOU1 to be applied to active biological control of pathogenic bacteria for grouper larvae.
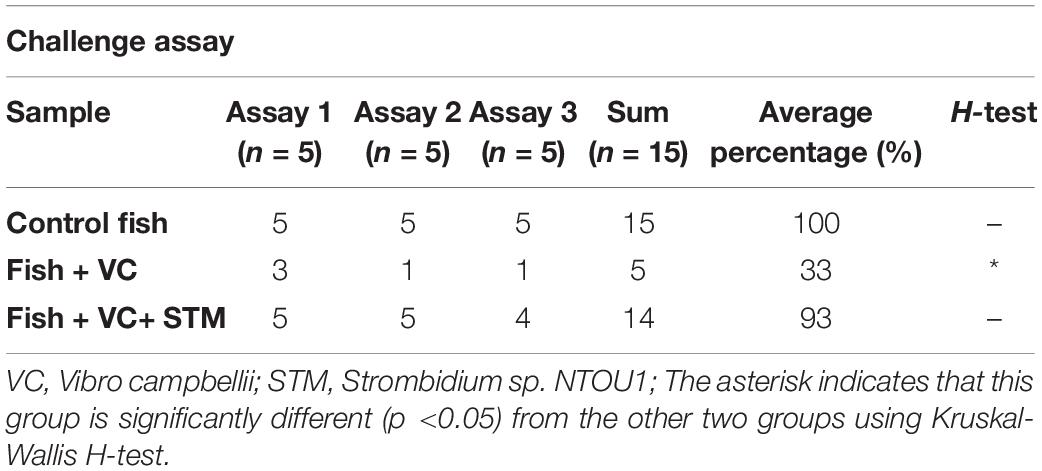
Table 1. Summary of the pathogen challenge assay from grouper larvae infected by Vibro campbellii with or without the addition of Strombidium sp. NTOU1.
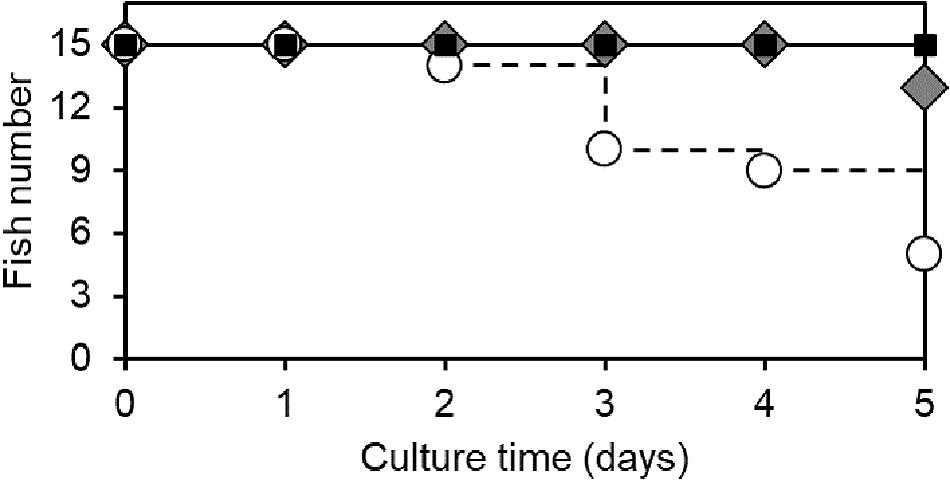
Figure 7. Survival rate of grouper larvae infected with Vibrio campbellii with or without the addition of Strombidium spp. NTOU1. Black squares indicate the control group without either vibrio infection or addition of ciliates. White circles represent the group of vibrio-infected grouper without the addition of ciliates. Gray diamonds represent the group with the simultaneous addition of both vibrio and ciliates. The data is the sum of three independent experiments. Five fish larvae were used for each set of the experiment.
Discussion
We had established a robust procedure for large-scale culturing and handling of the marine ciliate Strombidium sp. NTOU1 and characterized its ability to ingest bacteria. The excellent bacteriovorous properties prompted us to investigate its potential for active biological control in aquaculture. Our result demonstrated that it could efficiently remove Vibrio campbellii from the environment and protect grouper larvae from infection.
As shown in Figure 1, Strombidium sp. NTOU1 could not survive in the sterilized medium, but it could utilize different types of bacteria as the nutritional source. Fluorescent microscopy observation confirmed that the preyed GFP-expressing bacteria appeared within food vacuoles in the ciliate, and the subsequent diminishing of the fluorescence suggested that the bacteria were degraded (Figure 5B). These results collectively argue that Strombidium sp. may mainly obtain nutrients from the preyed bacteria, which is similar to the bacteriovorous ciliate Cyclidium glaucoma (Posch et al., 2001). The growth rate of ciliates for feeding different bacteria was not significantly different except for Erwinia spp. and feeding Strombidium sp. with Erwinia spp. gave the maximum growth efficiency, indicating that Erwinia spp. can be used as a suitable nutrition provider in the routine culture of Strombidium sp. (Figure 1). Furthermore, the common marine bacterial pathogens in aquaculture, such as V. campbellii and V. herveyi, also could sustain Strombidium sp. NTOU1 growth, indicating that this ciliate did have the potential as a biological control species to prey on pathogens (Figure 1).
To develop an active biological control method for aquaculture, it is necessary to supply enough ciliate biomass to affect the pathogen population in the culture pond. Therefore, methods for improving the culture efficiency of Strombidium sp. is an important issue. In general, most culture methods for bacteriovorous ciliates rely on rice/wheat grain medium containing naturally grown bacteria or an organic medium rich in a single strain of bacteria (Christaki et al., 1998; Chen et al., 2013; Schaafsma and Peperzak, 2013; Jiang et al., 2019). Although the preparation in such methods is simple, the growth rate of ciliates was fluctuated due to the constant change of bacterial numbers in the medium (Christaki et al., 1998; Cho et al., 2019). Not only was it difficult to accurately control the growth rate of ciliates, but it also took a relatively long time to reach the maximum density of the culture. Here, we tested an alternative approach to supply the ciliate with a given amount of frozen bacteria. As shown in Figure 2, although the growth rate is slightly lower than that of the fresh bacteria feeding, the Strombidium sp. did use the frozen Erwinia spp. as a nutrient source. Another marine ciliate Parauronema acutum could also survive on dead bacteria-based medium (Soldo and Merlin, 1977; Hamsher et al., 2018), indicating that this method could be applied to culture other ciliates. Frozen bacteria can be stored and is convenient to use, and this approach also allows us to precisely control the total amount of bacterial food in the medium, making it feasible to consistently culture ciliates for practical applications.
Previous studies have shown that excess food organisms in the environment limited the growth of ciliates (Berk et al., 1976; Taylor, 1977). Ideally, the number of bacteria should be minimized while sufficient nutrients are provided. Therefore, a batch feeding approach was conducted to replenish the frozen Erwinia spp. daily with the cell number at the 10,000:1 ratio to the ciliate numbers during the culture process. From the bacterial clearance rate of Strombidium sp. for E. coli and V. campbellii (315/340 bacterial CFU/hr/ciliate; see Figures 5, 6), the amount of bacterial feeding that we added was indeed close to the daily bacterial consumption of the ciliate. Through this optimized culture method, the growth rate of the ciliate gradually increased within 3 days and entered the stationary phase on the 4th day. It allowed ciliates to grow faster and to reach a higher cell density (1.07×104 cells/mL, Figure 3) than ciliates cultured in the rice medium (Supplementary Figure S1). Meanwhile, we tested an enrichment step that the ciliate density could be further concentrated by suction filtration to reach 1.20×105 cells/mL without apparent physical damages to the ciliate (Figure 4). Altogether, we made a critical technical improvement to culture and handle this ciliate, which lays down the foundation for utilizing Strombidium sp. NTOU1 strain in future academic research or biotechnology applications.
Feeding with the fluorescent E. coli confirmed that Strombidium sp. NTOU1 could rapidly remove bacteria from the water body by capturing bacteria and digesting them in food vacuoles (Figure 5). Several lines of evidence argue that this bacteriovorous property can be adopted for active biological control. The bacterial clearance rate of Strombidium sp. NTOU1 (∼300 bacteria/hr/ciliate) is slightly higher than that of other marine ciliates in previous reports (Berk et al., 1976; Christaki et al., 1998), indicating that this species potentially has advantages over other reported marine ciliates in biological protective effects against bacterial infections. In the simulated extreme environment at the milliliter scale, Strombidium sp. NTOU1 was able to effectively eliminate 85 % of bacterial pathogens V. campbellii within an hour (Figure 6). Increasing the testing scale to 5 L, Strombidium sp. also maintained the bacterial clearance ability. During three independent trials to protect grouper larvae from vibrio challenge, the survival rate was significantly increased to 93%, a striking contrast to 33% of the no-ciliate group (Figure 7). For the ciliate-protected group, only one grouper larva died on the last day of the 5-day observation. Furthermore, we noticed that no typical symptom of vibrio infectious was observed in the sole dead larva from the ciliate-protected group while the other grouper larvae died from V. campbellii infection showed obvious white wounds (data not shown), implying that there might be other causes unrelated to vibrio infection. These results argue that the ability to limit vibrio growth by Strombidium sp. and its biological protection was as good as using bacteriophages (Wagner and Waldor, 2002). Also, utilizing ciliates for biological control of pathogenic bacteria offers several advantages. First, a bacteriophage may promote the pathogen to develop and select for antiviral mutations, and there is a risk of transduction-mediated horizontal gene transfer between bacteria (Labrie et al., 2010; Keen et al., 2017). Using ciliates is relatively safer. Second, due to the specificity between a phage to its host pathogen, it is necessary to evaluate the bacteria strains in the culture environment before selecting a suitable phage (Pereira et al., 2011), which limits its usage and may not be feasible in practical applications. Strombidium sp. NTOU1 could use different bacteria as the food source in our experiment (Figure 1), and the average bacterial clearance efficiency for E. coli and V. campbellii was similar (Figures 5, 6). These results imply that Strombidium sp. NTOU1 has the potential to combat multiple pathogenic bacteria in the actual culture environment where the infection source is unknown and variable from case to case.
As shown in Figure 5, we found that even with the presence of enough Strombidium sp. NTOU1, bacteria at a density less than 2.5×104 cells/mL were unable to be further reduced. This suggests that, unlike the environmental hazard caused by antibiotics (Cabello, 2006; Martinez, 2011), the excess Strombidium sp. might not affect the original microbial population in the culture pond, which helps to maintain the ecological balance. In addition, while parasitic ciliates such as Uronema nigricans, Ichthyophthirius multifiliis, or Cryptocaryon irritans could pose a potential risk to infect aquatic organisms, to our knowledge, no studies have found that any ciliate in the genus Strombidium has the potential to affect the health of aquatic organisms (Montagnesa et al., 1996; Montagnesa and Lessard, 1999; Dolan, 2018; McManus et al., 2018). In fact, several studies have suggested that Strombidium ciliates are ideal biological feed for larvae of crustaceans and fish (Maeda and Liao, 1994; Thompson et al., 1999; Côrtes et al., 2013). Strombidium ciliates feeding on different food sources could accumulate specific nutrients, such as amino acids and unsaturated fatty acids, within their cells (Kramhøft et al., 1997; Wallberg et al., 1997; Fujibayashi et al., 2018). These studies and our results collectively support that there is great potential to use Strombidium for versatile applications in aquaculture.
In summary, we successfully overcome the technical limitations to utilize Strombidium sp. NTOU1 and establish a standard process for culturing and preparation. The concept of culture can be applied to increase the growth rate of other ciliates. In addition, the challenging assay result demonstrated that Strombidium sp. NTOU1 could protect aquatic organisms from the threat of bacterial disease. These studies provide a new perspective on the environmentally safe approach to reduce the biomass of harmful bacteria in aquaculture environments.
Data Availability Statement
These data generated from this study are available on request to the corresponding author.
Ethics Statement
The animal study was reviewed and approved by Institutional Animal Care and Use Committee, College of Life Sciences, National Taiwan Ocean University (IACUC Approval No. 102025).
Author Contributions
H-YL, C-CT, and H-JL: conceptualization. W-YY: experimental operation. H-YL: writing – original draft preparation. S-FT, C-CT, and H-JL: writing – review and editing. S-FT and K-PC: field sampling. S-FT: ciliate identification. JL: Vibro isolation and identification. H-JL: funding acquisition. All authors read and approved the final manuscript.
Funding
This study was funded by Ministry of Science and Technology (Taipei, Taiwan), grant numbers MOST 106-2313-B-019-004, MOST 108-2611-M-019-013, and MOST 108-2311-B-019-002.
Conflict of Interest
The authors declare that the research was conducted in the absence of any commercial or financial relationships that could be construed as a potential conflict of interest.
Acknowledgments
The authors thank Prof. Jeng Chang for giving appropriate advice on statistics. Also, the authors would like to thank the two reviewers for their valued criticism and suggestions.
Supplementary Material
The Supplementary Material for this article can be found online at: https://www.frontiersin.org/articles/10.3389/fmars.2020.00373/full#supplementary-material
References
Ali, T. H., and Saleh, D. S. (2014). A simplified experimental model for clearance of some pathogenic bacteria using common bacterivorous ciliated spp. in Tigris river. Appl. Water Sci. 4, 63–71. doi: 10.1007/s13201-013-0130-1
Becks, L., Hilker, F. M., Malchow, H., Jürgens, K., and Arndt, H. (2005). Experimental demonstration of chaos in a microbial food web. Nature 435, 1226–1229. doi: 10.1038/nature03627
Berk, S. G., Colwell, R. R., and Small, E. B. (1976). A study of feeding responses to bacterial prey in estuarine ciliates. Trans. Am. Microsc. Soc. 95, 514–520.
Bostock, J., McAndrew, B., Richards, R., Jauncey, K., Telfer, T., Lorenzen, K., et al. (2010). Aquaculture: global status and trends. Philos. Trans. R. Soc. B 365, 2897–2912. doi: 10.1098/rstb.2010.0170
Brander, K. M. (2007). Global fish production and climate change. Proc. Natl. Acad. Sci. U.S.A. 104, 19709–19714. doi: 10.1073/pnas.0702059104
Cabello, F. C. (2006). Heavy use of prophylactic antibiotics in aquaculture: a growing problem for human and animal health and for the environment. Environ. Microbiol. 8, 1137–1144. doi: 10.1111/j.1462-2920.2006.01054.x
Chen, X. M., Zhao, Y., Al-Farraj, S. A., Al-Quraishy, S., El-Serehy, H. A., Shao, C., et al. (2013). Taxonomic descriptions of two marine ciliates, Euplotes dammamensis n. sp. and Euplotes balteatus (Dujardin, 1841) Kahl, 1932 (Ciliophora, Spirotrichea, Euplotida), collected from the Arabian Gulf, Saudi Arabia. Acta Protozool. 52, 73–89. doi: 10.4467/16890027AP.13.008.1087
Cho, K., Lee, S. M., Cho, D. H., Heo, J., Lee, Y. J., and Kim, H. S. (2019). Novel biological method for controlling bacterial contaminants using the ciliate Colpoda sp. HSP-001 in open pond algal cultivation. Biomass Bioenerg. 127:105258. doi: 10.1016/j.biombioe.2019.105258
Christaki, U., Dolan, J. R., Pelegri, S., and Rassoulzadegan, F. (1998). Consumption of picoplankton–size particles by marine ciliates: effects of physiological state of the ciliate and particle quality. Limnol. Oceanogr. 43, 458–464. doi: 10.4319/lo.1998.43.3.0458
Côrtes, G. F., Tsuzuki, M. Y., and Melo, E. M. C. (2013). Monoculture of the ciliate protozoan Euplotes sp. (Ciliophora; Hypotrichia) fed with different diets. Acta Sci. 35, 15–19. doi: 10.4025/actascibiolsci.v35i1.11795
Defoirdt, T., Sorgeloos, P., and Bossier, P. (2011). Alternatives to antibiotics for the control of bacterial disease in aquaculture. Curr. Opin. Microbiol. 14, 251–258. doi: 10.1016/j.mib.2011.03.004
Dolan, J. R. (2018). The villefranche Strombidium sulcatum: a review. Eur. J. Protistol. 66, 68–76. doi: 10.1016/j.ejop.2018.08.002
FAO (2018). The State of World Fisheries and Aquaculture 2018 – Meeting the Sustainable Development Goals. Rome: FAO.
Fujibayashi, M., Tanaka, N., Hashido, S., Takasawa, A., and Nishimura, O. (2018). Nutritional quality of fish faeces is enhanced by highly unsaturated fatty acid-producing heterotrophic protozoa. Acta Oecol. 89, 21–26. doi: 10.1016/j.actao.2018.04.003
Goldburg, R., and Naylor, R. (2005). Future seascapes, fishing, and fish farming. Front. Ecol. Environ. 3:21–28. doi: 10.1890/1540-92952005003[0021:FSFAFF]2.0.CO;2
Grønlien, H. K., Berg, T., and Løvlie, A. M. (2002). In the polymorphic ciliate Tetrahymena vorax, the non-selective phagocytosis seen in microstomes changes to a highly selective process in macrostomes. J. Exp. Biol. 205, 2089–2097.
Hamsher, S. E., Sung, C. Y., and Sanders, R. W. (2018). Effects of temperature and photorepair radiation on a marine ciliate exposed to UVB radiation. J. Eukaryot. Microbiol. 65, 458–467. doi: 10.1111/jeu.12491
Heuer, O. E., Kruse, H., Grave, K., Collignon, P., Karunasagar, I., and Angulo, F. J. (2009). Human health consequences of use of antimicrobial agents in aquaculture. Clin. Infect. Dis. 15, 1248–1253. doi: 10.1086/605667
Jiang, Y. H., Zhang, T. T., Vallesi, A., Yang, X. Y., and Gao, F. (2019). Time-course analysis of nuclear events during conjugation in the marine ciliate Euplotes vannus and comparison with other ciliates (Protozoa, Ciliophora). Cell Cycle 18, 288–298. doi: 10.1080/15384101.2018.1558871
Kalatzis, P. G., Castillo, D., Katharios, P., and Middelboe, M. (2018). Bacteriophage interactions with marine pathogenic vibrios: implications for phage therapy. Antibiotics(Basel) 7:15. doi: 10.3390/antibiotics7010015
Keen, E. C., Bliskovsky, V. V., Malagon, F., Baker, J. D., Prince, J. S., Klaus, J. S., et al. (2017). Novel “superspreader” bacteriophages promote horizontal gene transfer by transformation. mBio 8:e02115-16. doi: 10.1128/mBio.02115-16
Kim, B., Hwang, S. J., Kim, Y. O., Hwang, S., Takamura, N., and Han, M. S. (2007). Effects of biological control agents on nuisance cyanobacterial and diatom blooms in freshwater systems. Microbes Environ. 22, 52–58. doi: 10.1264/jsme2.22.52
Kramhøft, B., Mollerup, J., and Lambert, I. H. (1997). Regulation of taurine accumulation in the ciliate protozoan Tetrahymena pyriformis. Amino Acids 13, 281–297. doi: 10.1007/BF01372593
Labrie, S. J., Samson, J. E., and Moineau, S. (2010). Bacteriophage resistance mechanisms. Nat. Rev. Microbiol. 8, 317–327. doi: 10.1038/nrmicro2315
Lam, F. W., Wu, S. Y., Lin, S. J., Lin, C. C., Chen, Y. M., Wang, H. C., et al. (2011). The expression of two novel orange-spotted grouper (Epinephelus coioides) TNF genes in peripheral blood leukocytes, various organs, and fish larvae. Fish Shellfish Immunol. 30, 618–629. doi: 10.1016/j.fsi.2010.12.011
Lee, M. L. (2015). Conjugation in the Marine Oligotrich Ciliates (Ciliophora, Oligotrichida)- Its Systematic Taxonomy and Occurrence. Master’s thesis, National Taiwan Ocean University, Keelung.
Letchumanan, V., Chan, K. G., Pusparajah, P., Saokaew, S., Duangjai, A., Goh, B. H., et al. (2016). Insights into bacteriophage application in controlling vibrio species. Front. Microbiol. 7:1114. doi: 10.3389/fmicb.2016.01114
Maeda, M., and Liao, I. C. (1994). Microbial processes in aquaculture environment and their importance for increasing crustacean production. Jpn. Agric. Res. Q. 28, 283–288.
Martinez, J. L. (2011). Environmental pollution by antibiotics and by antibiotic resistance determinants. Environ. Pollut. 157, 2893–2902. doi: 10.1016/j.envpol.2009.05.051
McManus, G. B., Liu, W. W., Cole, R. A., Biemesderfer, D., and Mydosh, J. L. (2018). Strombidium rassoulzadegani: a model species for chloroplast retention in oligotrich ciliates. Front. Mar. Sci. 5:205. doi: 10.3389/fmars.2018.00205
Montagnesa, D. J. S., Bergerb, J. D., and Taylora, F. J. R. (1996). Growth rate of the marine planktonic ciliate Strombidinopsis cheshiri Snyder and Ohman as a function of food concentration and interclonal variability. J. Exp. Mar. Biol. Ecol. 206, 121–132. doi: 10.1016/S0022-0981(96)02626-3
Montagnesa, D. J. S., and Lessard, E. J. (1999). Population dynamics of the marine planktonic ciliate Strombidinopsis multiauris: its potential to control phytoplankton blooms. Aquat. Microb. Ecol. 20, 167–181. doi: 10.3354/ame020167
Muller-Feuga, A. (2000). The role of microalgae in aquaculture: situation and trends. J. Appl. Phycol. 12, 527–534. doi: 10.1023/A:1008106304417
Nayak, S. K. (2010). Probiotics and immunity: a fish perspective. Fish Shellfish Immunol. 29, 2–14. doi: 10.1016/j.fsi.2010.02.017
Ng, S. F., and Nelsen, E. M. (1977). The protargol staining technique: an improved version for Tetrahymena pyriformis. Trans. Am. Microsc. Soc. 96, 369–376. doi: 10.2307/3225867
Noor, N. M., Defoirdt, T., Alipiah, N., Karim, M., Daud, H., and Natrah, I. (2019). Quorum sensing is required for full virulence of Vibrio campbellii towards tiger grouper (Epinephelus fuscoguttatus) larvae. J. Fish Dis. 42, 489–495. doi: 10.1111/jfd.12946
Oliva-Teles, A. (2012). Nutrition and health of aquaculture fish. J. Fish Dis. 35, 83–108. doi: 10.1111/j.1365-2761.2011.01333.x
Pereira, C., Salvador, S., Arrojado, C., Silva, Y., Santos, A. L., Cunha, A., et al. (2011). Evaluating seasonal dynamics of bacterial communities in marine fish aquaculture: a preliminary study before applying phage therapy. J. Environ. Monit. 13, 1053–1058. doi: 10.1039/c0em00434k
Pérez-Sánchez, T., Mora-Sánchez, B., and Balcázar, J. L. (2018). Biological approaches for disease control in aquaculture: advantages, limitations and challenges. Trends Microbiol. 26, 896–903. doi: 10.1016/j.tim.2018.05.002
Posch, T., Jezbera, J., Vrba, J., Simek, K., Pernthaler, J., Andreatta, S., et al. (2001). Size selective feeding in Cyclidium glaucoma (Ciliophora, Scuticociliatida) and its effects on bacterial community structure: a study from a continuous cultivation system. Microb. Ecol. 42, 217–227. doi: 10.1007/s002480000114
Sapkota, A., Sapkota, A. R., Kucharski, M., Burke, J., McKenzie, S., Walker, P., et al. (2008). Aquaculture practices and potential human health risks: Current knowledge and future priorities. Environ. Int. 34, 1215–1216. doi: 10.1016/j.envint.2008.04.009
Schaafsma, F. L., and Peperzak, L. (2013). Phytoplankton growth inhibited by the toxic and bacterivorous ciliate Uronema marinum (Protozoa, Ciliophora). Mar. Ecol. Prog. Ser. 475, 35–48. doi: 10.3354/meps10124
Sherr, E. B., and Sherr, B. F. (2002). Significance of predation by protists in aquatic microbial food webs. Antonie Van Leeuwenhoek 81, 293–308. doi: 10.1023/a:1020591307260
Soldo, A. T., and Merlin, E. J. (1977). The nutrition of Puruuronerna muturn. J. Protozool. 24, 556–562.
Taylor, W. D. (1977). Growth responses of ciliate protozoa to the abundance of their bacterial prey. Microb. Ecol. 4, 207–214. doi: 10.1007/BF02015077
Thompson, F. L., Abreu, P. C., and Cavalli, R. (1999). The use of microorganisms as food source for Penaeus paulensis larvae. Aquaculture 174, 139–153. doi: 10.1016/S0044-8486(98)00511-0
Verschuere, L., Rombaut, G., Sorgeloos, P., and Verstraete, W. (2000). Probiotic bacteria as biological control agents in aquaculture. Microbiol. Mol. Biol. Rev. 64, 655–671. doi: 10.1128/mmbr.64.4.655-671.2000
Wagner, P. L., and Waldor, M. K. (2002). Bacteriophage control of bacterial virulence. Infect. Immun. 70, 3985–3993. doi: 10.1128/iai.70.8.3985-3993.2002
Wallberg, P., Bergqvist, P. A., and Andersson, A. (1997). Potential importance of protozoan grazing on the accumulation of polychlorinated biphenyls (PCBs) in the pelagic food web. Hydrobiologia 357, 53–62. doi: 10.1023/A:1003122500557
Wang, H. C., Tseng, C. W., Lin, H. Y., Chen, I. T., Chen, Y. H., Chen, Y. M., et al. (2010). RNAi knock-down of the Litopenaeus vannamei toll gene (LvToll) significantly increases mortality and reduces bacterial clearance after challenge with Vibrio harveyi. Dev. Comp. Immunol. 34, 49–58. doi: 10.1016/j.dci.2009.08.003
Keywords: biological control, aquacultrure, marine ciliate, bacteriovorus ability, high-density cultivation
Citation: Lin H-Y, Yeh W-Y, Tsai S-F, Chiang K-P, Lin JH-Y, Tsao C-C and Lin H-J (2020) Biological Protective Effects Against Vibrio Infections in Grouper Larvae Using the Strombidium sp. NTOU1, a Marine Ciliate Amenable for Scaled-Up Culture and With an Excellent Bacteriovorous Ability. Front. Mar. Sci. 7:373. doi: 10.3389/fmars.2020.00373
Received: 17 January 2020; Accepted: 30 April 2020;
Published: 29 May 2020.
Edited by:
Sami Souissi, Lille University of Science and Technology, FranceReviewed by:
Nor Azman Kasan, University of Malaysia Terengganu, MalaysiaNajiah Musa, University of Malaysia Terengganu, Malaysia
Copyright © 2020 Lin, Yeh, Tsai, Chiang, Lin, Tsao and Lin. This is an open-access article distributed under the terms of the Creative Commons Attribution License (CC BY). The use, distribution or reproduction in other forums is permitted, provided the original author(s) and the copyright owner(s) are credited and that the original publication in this journal is cited, in accordance with accepted academic practice. No use, distribution or reproduction is permitted which does not comply with these terms.
*Correspondence: Che-Chia Tsao, Y2N0c2FvQG1haWwubnV0bi5lZHUudHc=; Han-Jia Lin, aGFuamlhQG1haWwubnRvdS5lZHUudHc=
†These authors have contributed equally to this work