- 1Postgraduate Program in Dynamics of Oceans and Earth, Department of Geology, Institute of Geosciences, Federal Fluminense University, Niteroi, Brazil
- 2Center for Study of Water, Biomass and Oil, Federal Fluminense University, Niteroi, Brazil
- 3Laboratory of Environmental Biochemistry, Department of Oceanography and Limnology, Federal University of Rio Grande do Norte, Natal, Brazil
This brief research compares the bioconcentration of heavy metals (Cd, Cr, Cu, Pb, Ni, and Zn) in the soft tissues of native oysters Crassostrea rhizophorae (Guilding, 1828) from two areas in the Potengi estuary, northeastern coast of Brazil. The purpose was to test the hypotheses of relevant differences in bioaccumulation while the environmental conditions change upstream. The downstream station showed higher concentrations for cadmium and nickel, and the upstream one revealed increased values for body weight, condition index, chromium, lead, and zinc (p < 0.05). The copper variation between sites was negligible, but as for zinc and chromium, the metal content observed was above guidance levels. Results revealed two aspects that were mainly influencing the bioavailability and bioaccumulation of metals in oysters. First, the predominance of marine or riverine characteristics of each site, and second, the relative proximity to the point of introduction of the pollutant even though stations were not quite far apart. C. rhizophorae accumulated heavy metals in significant concentrations, endorsing its efficiency as a biomonitor.
Introduction
Heavy metals have been considered potentially toxic elements by a considerable part of the literature (Mendoza-Carranza et al., 2016; Griboff et al., 2018; Kulkarni et al., 2018). They are natural constituents of the earth’s crust, occurring in several compartments, such as the atmosphere, water bodies, sediments, and the biosphere (Garrett, 2000). Throughout modern history, industrialization and urbanization have generated a considerable risk of contamination of estuaries and coastal ecosystems (Farrington et al., 2016) as a result of the disposal of industrial production.
Mangrove ecosystems are transitional areas between marine and continental environments located in the intertidal zone (Odum, 2004) and, therefore, characterized by significant fluctuations of salinity, temperature, and conditions of oxidation (Lewis et al., 2011). The vegetation, with its euryhaline characteristic, plays great importance in the maintenance of other species offering shelter, refuge, and food sources for both marine organisms and riverine communities (Usman et al., 2013).
Organic matter levels are naturally higher in these environments compared to other marine ecosystems due to their high carbon productivity (Lacerda et al., 1988), generating a conducive environment for the accumulation of industrial pollutants, such as heavy metals and polycyclic aromatic hydrocarbons (PAH). Usually, the low hydrodynamic level allows a high concentration of fine grain–size sediments, which favors the retention of organic matter and co-precipitated elements (Hedges and Keil, 1999), among them the heavy metals (e.g., Sabadini-Santos et al., 2014). These pollutants are one of the leading causes of negative impacts on the environment, causing significant damage to the quality of ecosystems (Defew et al., 2005; Tolhurst et al., 2006).
During the last decades, different strategies of biomonitoring have been developed to monitor the presence of pollutants and evaluate negative impacts on marine and estuarine environments (Goldberg, 1975; Choi et al., 2010; Farrington et al., 2016). The bivalve mollusks are an essential tool, displaying a cosmopolitan distribution and ability to tolerate high concentrations of contaminants without significant metabolic damage. They feed basically from particulate organic matter (POM) and plankton through branchial filtration, purifying the dirt found in waters (Pereira et al., 2002; Rainbow, 2006). Within the Bivalvia class, the genus Crassostrea has been used regularly in environmental studies (Kanhai et al., 2014; Rainbow et al., 2015; Gain et al., 2017; Aguirre-Rubí et al., 2018; Shi et al., 2019; Senez-Mello et al., 2020b). The specie Crassostrea rhizophorae, found on littoral rocks and the prop roots of mangrove trees along the Potengi estuary, is popularly known as mangrove oysters or cupped oysters (Aguirre-Rubí et al., 2018) and is widely used by the local population as an important food source (Silva et al., 2001).
This study analyzes the bioavailability of heavy metals in the Potengi estuary, using the species C. rhizophorae as a biomonitoring tool and taking as a basis of comparison guidelines for human consumption established by Brazilian legislation (Decreto No° 55. 871, 1965; Portaria No° 685, 1998). It investigates the influence of environmental factors on the metabolism of C. rhizophorae concerning the accumulation of these metals, using for this purpose two different points of collection.
Study Site
The Potengi river is located on the northeast coast of Brazil (Rio Grande do Norte). It extends for 109.36 miles along a densely populated and industrialized area (IBGE, 2007), reaching the Atlantic Ocean at the city of Natal, which has about 774,205 inhabitants (IBGE, 2007), a very active shipyard, and approximately 1,500 industries (Nicodemo et al., 2010).
The prevailing climate is hot and humid with an average annual temperature of 26.8°C and two well-defined seasons with a dry summer from October to December and a rainy winter from April to June (Souza and Ramos Silva, 2011). The tidal regime is semidiurnal, and the maximum amplitude can reach 2.83 m during the spring tide (Frazão, 2003; de Souza et al., 2010).
The water column does not present vertical stratification of temperature. Still, this parameter can be influenced by atmospheric temperature and the magnitude of the tides, which can reach 20 km west of Natal, and stronger currents are observed during the rainy season (Frazão and Vital, 2007).
Two sampling sites (Figure 1), Naval Base (NB: 5°47′19.6″S; 35°13′20.6″W) and Quartel (Qt: 5°45′32.3″S; 35°12′05.5″W), were chosen based on previous studies (Silva et al., 2001, 2006). These studies monitored the Potengi estuary along 30 km upstream, testing the response of different biomonitors, showing that NB and Qt have distinct patterns in the bioaccumulation of metals.
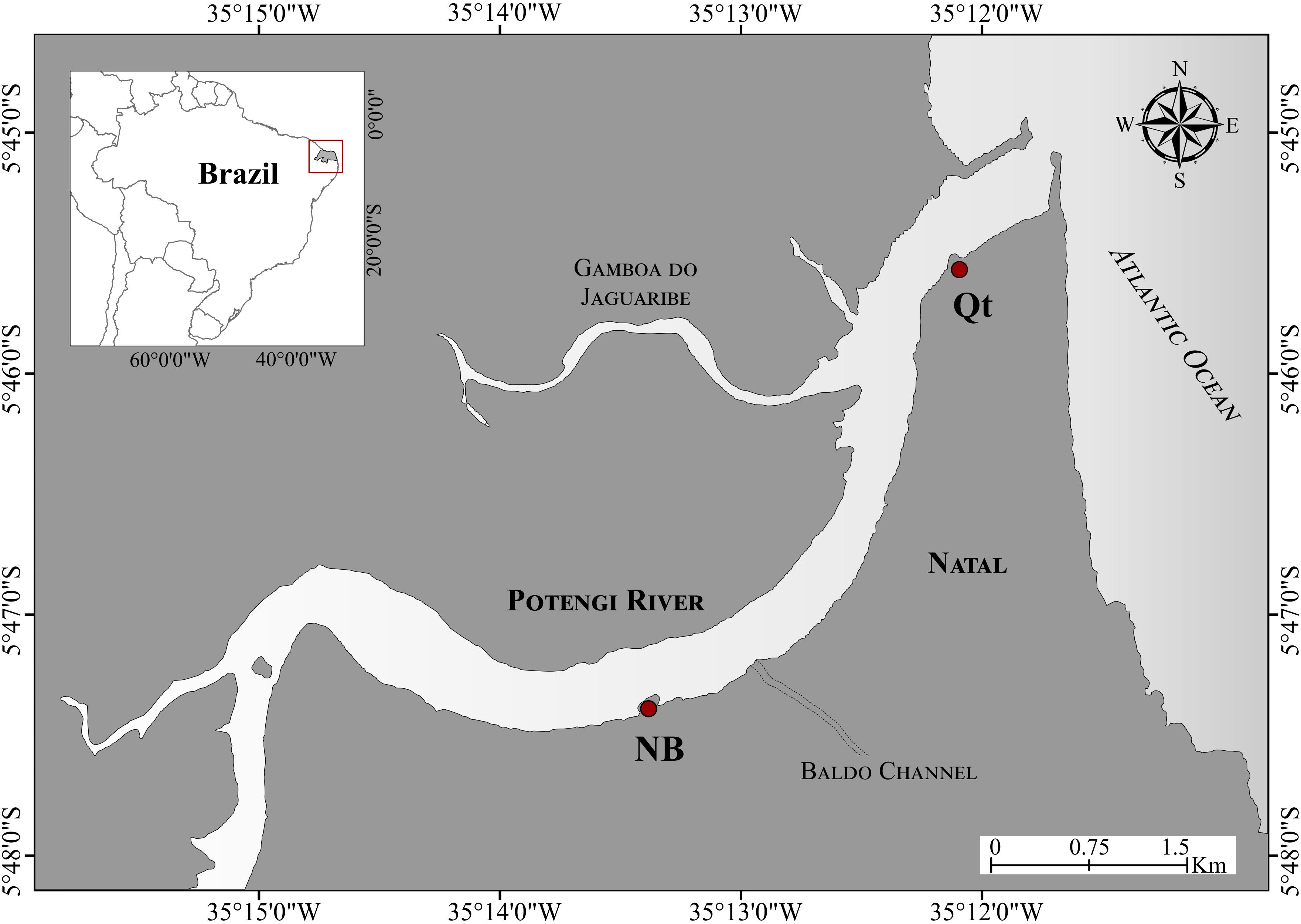
Figure 1. Oyster sampling site locations at Potengi. NB, Naval Base (Brazilian Navy pier); Qt, Quartel (Y-Beach, 17th Army Artillery Group). Map created with Google Earth Pro 7.3.2 software (Image catalog ID: 1010010004319001) and CorelDraw X8.
Upstream, oysters from NB were collected from the docking pier. According to some authors (Frazão, 2003; Dantas, 2009), this site is impacted by pollutants associated with port activities, tanneries, and untreated sewage, both domestic and industrial, that are mainly introduced through the Baldo Channel (Figure 1). Among pollutants, these authors highlight the high load of organic matter, Cr, Ni, Pb, and Zn.
Downstream, oysters were sampled at Qt. On this site, most pollutants arrive through Gamboa do Jaguaribe (Santos, 2018), a channel levee with hydrodynamics dependent on the tide (Figure 1). There, the pollutants of primary concern are Cu, Zn, and Cr compounds derived mainly from the shrimp feed and Cu compounds contained in fungicides and algicides used in the treatment of cultivation tanks (Silva et al., 2001; Cunha, 2010).
Materials and Methods
The sampling campaign was held on July 19, 2007, whereas the methodology for collecting oysters was based on the size criterion, that is, individuals with a body size similar to the average size of that population were selected (Roberts et al., 1986). In each of the sampling sites, 15 native oysters belonging to the species C. rhizophorae were collected to quantify the concentrations of Cd, Cr, Cu, Ni, Pb, and Zn in their soft tissues. The material was properly identified and preserved in an ice-cooled container to be transported to the laboratory.
Aliquots for analysis were prepared according to Silva et al. (2001), which uses the total soft tissue (meat) of the oyster after having passed through a dehydration process in a stove at approximately 80°C. The dehydration period is determined by the time required until the sample reaches a constant weight, named here as “dry weight” (Dw).
Biometric data, as the weight of the soft tissue (before and after dehydration), and shell’s measurement (length, width, and height) were recorded for later calculation of the specimens condition index (CI) accordingly to Mercado-Silva (2005) and Rebelo et al. (2005). Results are expressed in (g/cm3).
The metal analyses were performed by using inductively coupled plasma optical emission spectrometry (ICP-OES, Thermo iCAP, Series 6300); for further details, see Silva et al. (2001). The analytical quality was tested periodically through the use of standard reference material (NIST 2976 – Mollusk tissue; National Institute of Standards and Technology, 2017) as well as procedural blanks (N = 4) performed to identify possible contamination. The percentage of analyte recovery ranged from 75 to 115% for all metals (Supplementary Table S1). All analyses were carried out at the Núcleo de Estudos em Petróleo e Gás Natural – NEPGN, Universidade Federal do Rio Grande do Norte.
Statistical analyses were performed using the Statistica v.13 program (TIBCO, 2017). The assumptions for using parametric analysis were satisfied after outliers’ exclusion (±3 × standard deviation) and logarithmic transformation of values (log10). Normality was verified by the Kolmogorov–Smirnov/Lilliefors and homoscedasticity using the Brown and Forsythe tests. Comparisons between the content of metals in the soft tissue of C. rhizophorae from NB and Qt were performed by Student’s t-test. The dependence of the metal concentrations on the weight of the oysters’ soft tissue (size effect) was tested to minimize the influence of oyster sizes on the statistical results (Whitton et al., 1994; Rainbow, 2006). The test was done through a simple linear regression (Mo and Neilson, 1993; Dragun et al., 2006; Mubiana et al., 2006; Rainbow, 2006):
here, y = metal concentration; a = intercept; b = slope of the linear function, and x = dry weight of the sample.
When regressions indicated a significant dependence, Student’s t-test was performed using weighted averages. Statistical results were reported at a 95% confidence level and considered significant when p < 0.05.
Granulometric and oceanographic information were obtained from Frazão (2003), Frazão and Vital (2007) and the content of metals in the sediments from Correa (2008). The water’s physicochemical parameters were obtained from Costa (2008). All data presented were obtained in the winter period of 2007 as follows: sediment June 19, oysters July 19, and water August 21. As the water column has dynamic characteristics, these data were not used in the statistical analysis. However, they serve as a basis for environmental characterization. On the other hand, the metal content in sediments is a more persistent feature, and so the variability in the 1-month window does not interfere in the results. For more details, see Supplementary Tables S2, S3.
The principal component analysis (PCA) was employed to aid the interpretation of the results. It was done through a variance–covariance matrix between heavy metals in oysters, CI, and metals in the sediments. PCA was carried out using PAST v3.0 statistical software (Hammer, 2013).
Results
Analysis of the water column demonstrated that, at Qt, the salinity, turbidity, pH, and dissolved oxygen (DO) were higher than those measured at NB although the water temperature, biochemical oxygen demand (BOD), and total organic carbon (TOC) were higher on the NB (Supplementary Table S2). These results are in line with the local estuarine dynamics in which tidal intrusion acts more intensely near the mouth of the estuary and gradually decreases upstream (Frazão, 2003). With the exception of the BOD, all other parameters cited above were within the standards for water quality proposed by the Brazilian legislation for saltwater (salinity > 30%) where recreational and fishing activities take place (CONAMA, 2012). In both sites, the ammoniacal nitrogen (NH3) was above the limit proposed by the same resolution, whereas nitrates had a high concentration in NB. No nitrites were detected (Supplementary Table S2).
The descriptive statistics of the biometric data showed higher weight values (0.25 ± 0.07 g) and CI (6.04 ± 1.5) for the oysters from NB when compared to those from Qt (0.11 ± 0.03 g and 3.80 ± 1.16, respectively), Table 1. Regarding the length of the shells, NB oysters showed higher means. However, there were no statistical differences (p > 0.05), indicating the efficiency of the sampling methodology (equivalence of the size of individuals for those populations). Further information can be consulted in the supplementary material (Supplementary Table S4).
Among metals, cadmium was the only metal showing a relevant size effect with a negative regression slope (b = −0.43; a = −0.99, r = 5.76, and p = 0.0055). In this case, the oysters’ dry weight was adjusted to an average value of 0.182 g before applying the Student’s t-test.
The student’s t-test confirmed a significant difference among samples of the two stations for the concentrations of Cd, Cr, Ni, Pb, and Zn. In the upstream station (NB), the metals Cr, Pb, and Zn showed higher concentrations with respective averages of 0.78, 0.35, and 1721.0 μg g–1, and in the downstream station (Qt), these values were 0.64, 0.31, and 1289 μg g–1 (Table 1). Ni and Cd showed higher concentrations in Qt samples (1.85 and 0.73 μg g–1), and in NB, the values were 1.12 and 0.19 μg g–1, respectively.
Discussion
The size effect observed in this study for Cd may be attributed to different factors (Whitton et al., 1994): (1) smaller organisms have a greater absorption area per gram of body weight relative to the bigger ones; (2) younger individuals have more active metabolism and, thus, higher filtration rates; and (3) there is a dilution effect in which concentrations seem to be lower as the oysters grow.
The condition index (CI) can be affected by a balance between multiple abiotic and physiological factors (e.g., seasonal variations and spawning) interfering in the organism’s development (Borchardt et al., 1988; Mubiana et al., 2006; Benali et al., 2017). According to Rebelo et al. (2005), specimens showing higher CI have better quality and productivity.
Cd, Ni, and Pb were within the values allowed for human consumption by Brazilian legislation (LMP – Table 2). Also, when compared with oysters (Crassostrea sp.) from other studies, these metals showed lower concentrations.
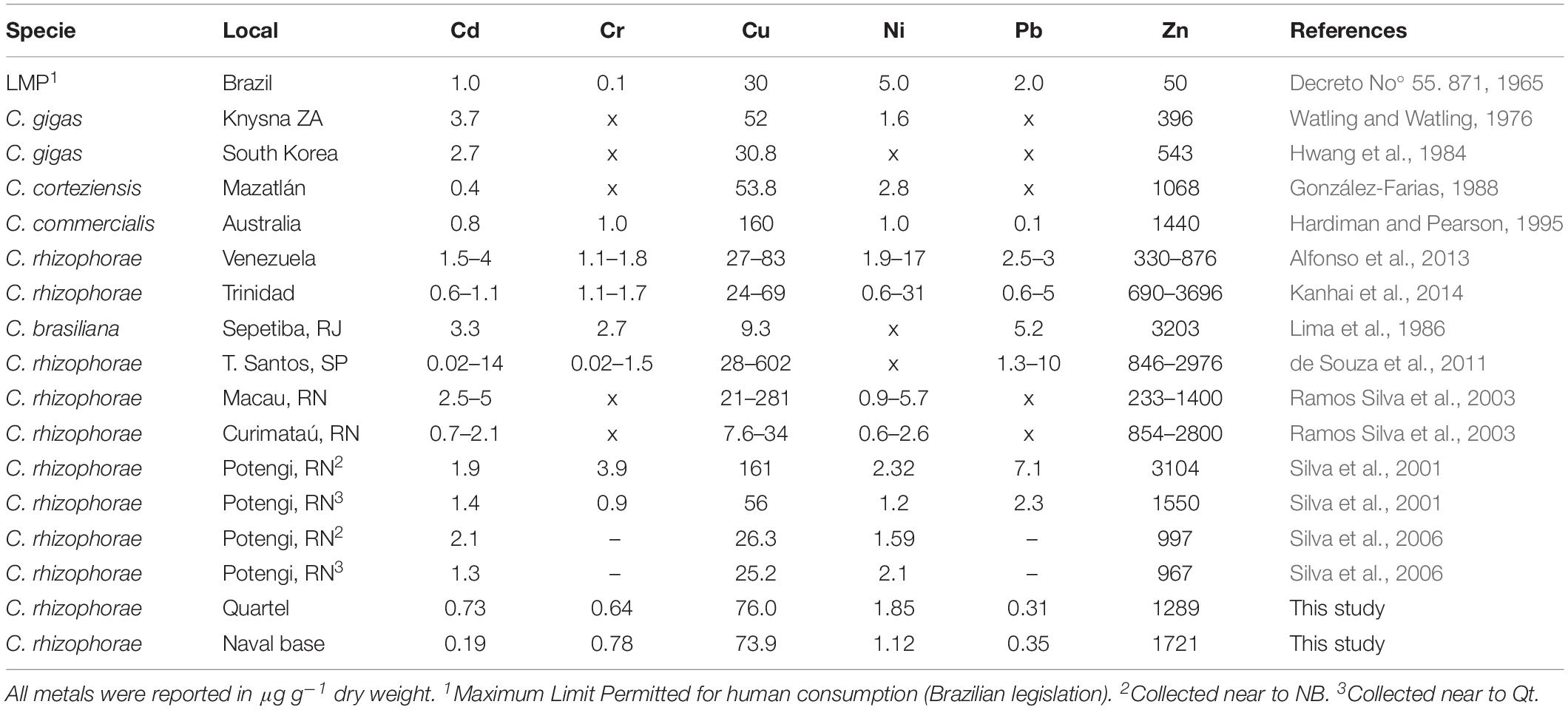
Table 2. Metals concentrations in Crassostrea sp.: Means and ranges obtained in the literature and this study.
Although Cr was not high concerning the literature, its concentration exceeded six times the maximum limit permitted for human consumption (LMP, Table 2). According to Nordberg et al. (2007), Cr may be responsible for the formation of reactive oxygen species that can cause damage (oxidative stress) to tissues and DNA (deoxyribonucleic acid), making this element potentially carcinogenic to humans.
Cu and Zn showed increased values when compared to oysters from the Potengi (Silva et al., 2001), and estuaries from elsewhere in the world (Alfonso et al., 2013; Kanhai et al., 2014). Cu exceeded the maximum limit permitted for human consumption by more than two times, and Zn exceeded it by about 30 times. Although oysters are known to contain large amounts of these metals, the values presented in this study were compatible with those of estuaries reported as highly polluted (Hardiman and Pearson, 1995; Ramos Silva et al., 2003; de Souza et al., 2011; Alfonso et al., 2013; Kanhai et al., 2014). Elevated concentrations of Zn and Cu in aquatic environments are generally associated with the disposal of untreated domestic sewage (Baptista Neto et al., 2000).
Heavy metals act directly at the cellular level; however, environmental factors may favor the bioaccumulation. As a general trend, metal is rapidly divided between the sediment and the aqueous phase, depending on the pH of the water, the salts dissolved in it, and the presence of organic complexing agents (Breysse, 2019). When there is a reduction in pH, the natural process of leaching and the availability of most metals can be intensified (Nordberg et al., 2009).
The principal component analyses (Figures 2A,B) displayed the relationship between the concentrations of metals in the oysters and the concentration in the sediments from the two sites. The sum of the first three components explained 93.86% of the total variability presented by the data (Supplementary Table S5). The first component (PC1) was responsible for 74.6% of the variability explanation. The PC1 represented the bioavailability of the metals contained in the sediment for incorporation into the biota. The cases were divided into two distinct groups corresponding to the sites (ellipses, Figures 2A,B). Positively loaded on the first axis, the bioconcentration of Cd and Ni were the vectors of the most significant weight influencing the distribution of Qt samples. With a negative load, the CI and the metals in the sediments (Cu, Ni, Pb, and Cr, in order of importance) were the variables of most significant influence in the distribution of NB samples, showing that, despite the high sediment contamination, the oysters there are healthier than in Qt. This result was compatible with the grain size of sediments at the two sites (Supplementary Table S3). Frazão (2003), Frazão and Vital (2007) described a higher content of fine grain–size sediments at NB as a result of lower hydrodynamics. The fine grain–size sediments coupled with the higher organic carbon content and more alkaline pH of domestic sewage from the Baldo Canal (Medeiros, 2009; Santos, 2018) allowed ideal conditions for the co-precipitation of metals adsorbed from organic matter in flakes.
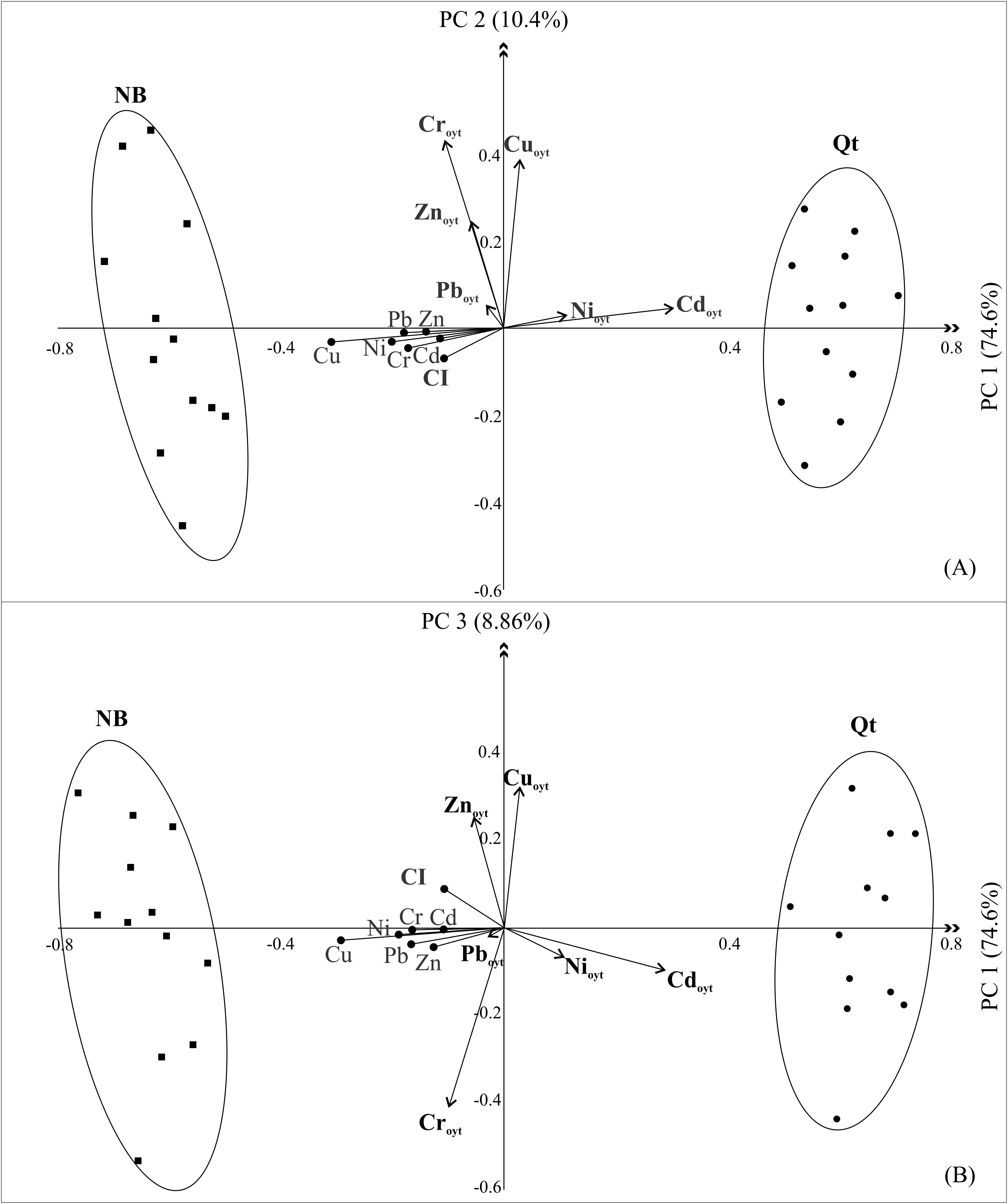
Figure 2. Principal component analysis. (A) PC 1 × 2 and (B) PC 1 × 3; Metaloyt, metal concentration in oysters’ tissue; Metal, concentration in the sediment; dots and squares: samples from Quartel (QT) and Naval Base (NB), respectively.
The second component (Figure 2A) was responsible for 10.4% of the variability explanation. PC2 demonstrated a covariance between Cr, Cu, and Zn in oysters despite the sampling sites. This covariance can be explained by the similarity in the chemical properties of these metals, which have a high capacity to form organic compounds being incorporated more easily by the oysters (Nordberg et al., 2007). In particular, these three metals above showed to be contaminants of major concern in the Potengi, way above the limits allowed for human consumption (Table 2).
Finally, the third component (PC3, Figure 2B) underlined the nature of Cu and Zn as essential nutrients, which are tolerated in higher amounts by the oysters. Meanwhile, Cr and Cd probably contributed to a toxic effect even if presented in lower quantities. The concentration of metals in biological solutions play a crucial role in determining their toxicity because they compete for the same binding sites in organic macromolecules (e.g., metallothionein and glutathione), preventing essential nutrients from being metabolized (Roesijadi, 1992, 1996; Nordberg et al., 2007).
Conclusion
The study showed that the analysis of heavy metals in biomonitoring, especially using the oyster C. rhizophorae, is more effective when carrying out an integrated analysis of environmental parameters—in particular, because the metals in the sediments or water column are not always bioavailable. In the case of a more complex and dynamic system as the estuary, monitoring only one compartment may not portray the toxicity and bioavailability of the pollutants.
The bioconcentration of cadmium was the primary variable for dividing the samples into two well-defined groups. The probable explanation is that when cadmium plasmatic concentrations rise, it competes against essential metals, such as Zn and Cu for binding sites, resulting in smaller CI values as those found at Qt.
The content of Zn and Cu in oysters was more than twice above the background values reported for mollusks in estuaries. These data are worrisome because bivalves constitute the basis of the food chain, usually occupying the second or third trophic niche. In this case, the oysters are susceptible to initiate an effect of bioaccumulation and biomagnification on the ecosystem.
Data Availability Statement
The data sets generated/analyzed for this study can be found in the Figshare repository, https://doi.org/10.6084/m9.figshare.12093456.v1 (Senez-Mello et al., 2020a).
Author Contributions
TS-M: data curation, statistical analysis, software, design, and development of the manuscript (original draft, review, and editing). TS-M and MC: investigated and wrote the original draft. MC: edited and validated the final draft. CR: did the conceptualization, methodology design, field survey, and data acquisition. ES: funding acquisition, field survey, sample preparation, and data acquisition. EF: project administration, supervision, resources, and validation. All authors contributed to the manuscript and approved the submitted version.
Funding
The authors would like to acknowledge the financial support of the Coordenação de Aperfeiçoamento de Pessoal de Nível Superior (CAPES 1525927/2015-19), Secretaria Nacional de Portos/Fundação Euclides da Cunha (SEP/FEC 3931), and Conselho Nacional de Desenvolvimento Científico e Tecnológico (CNPQ 154571/2006-07).
Conflict of Interest
The authors declare that the research was conducted in the absence of any commercial or financial relationships that could be construed as a potential conflict of interest.
Acknowledgments
The authors would like to thank MSc. Aecia Dantas for ensuring the quality of heavy metal analytical procedures.
Supplementary Material
The Supplementary Material for this article can be found online at: https://www.frontiersin.org/articles/10.3389/fmars.2020.00412/full#supplementary-material
References
Aguirre-Rubí, J., Luna-Acosta, A., Ortiz-Zarragoitia, M., Zaldibar, B., Izagirre, U., Ahrens, M. J., et al. (2018). Assessment of ecosystem health disturbance in mangrove-lined Caribbean coastal systems using the oyster Crassostrea rhizophorae as sentinel species. Sci. Total Environ. 618, 718–735. doi: 10.1016/j.scitotenv.2017.08.098
Alfonso, J. A., Handt, H., Mora, A., Vásquez, Y., Azocar, J., and Marcano, E. (2013). Temporal distribution of heavy metal concentrations in oysters Crassostrea rhizophorae from the central Venezuelan coast. Mar. Pollut. Bull. 73, 394–398. doi: 10.1016/j.marpolbul.2013.05.010
Baptista Neto, J. A., Smith, B. J., and McAllister, J. J. (2000). Heavy metal concentrations in surface sediments in a nearshore environment. Jurujuba Sound, Southeast Brazil. Environ. Pollut. 109, 1–9. doi: 10.1016/S0269-7491(99)00233-X
Benali, I., Boutiba, Z., Grandjean, D., de Alencastro, L. F., Rouane-Hacene, O., and Chèvre, N. (2017). Spatial distribution and biological effects of trace metals (Cu, Zn, Pb, Cd) and organic micropollutants (PCBs, PAHs) in mussels Mytilus galloprovincialis along the Algerian west coast. Mar. Pollut. Bull. 115, 539–550. doi: 10.1016/j.marpolbul.2016.12.028
Borchardt, T., Burchert, S., Hablizel, H., Karbe, L., and Zeitner, R. (1988). Trace metal concentrations in mussels: comparison between estuarine, coastal and offshore regions in the southeastern North Sea from 1983 to 1986. Mar. Ecol. Prog. Ser. 42, 17–31. doi: 10.3354/meps042017
Choi, H. G., Moon, H. B., Choi, M., Yu, J., and Kim, S. S. (2010). Mussel watch program for organic contaminants along the Korean coast, 2001–2007. Environ. Monit. Assess. 169, 473–485. doi: 10.1007/s10661-009-1190-4
CONAMA (2012). Resoluções do Conama: Resoluções vigentes Publicadas Entre Setembro de 1984 e Janeiro de 2012. Brazil: CONAMA.
Correa, T. D. E. L. (2008). Impactos Geoquímicos e Sócio-Ambientais no Estuário do Rio Potengi – Região Metropolitana da Grande Natal / RN. Programa Reg. Pós-Graduação em Desenvolv. e Meio Ambient. da Univ. Fed. do Rio Gd. do Norte. 104. Available online at: https://repositorio.ufrn.br/jspui/handle/123456789/18187. (accessed February 29, 2008).
Costa, E. C. T., and de, A. (2008). Diagnóstico ambiental das águas do estuário Jundiaí-Potengi pela determinação dos índices de qualidade da água e toxidez. Programa pós Grad. em Química. Available online at: https://repositorio.ufrn.br/jspui/handle/123456789/17607 (accessed March 21, 2018).
Cunha, P. E. V. (2010). Aplicação da Metodologia para Estimativa do Fator de Emissão – Nutrientes e Metais Pesados – para avaliar a Contribuição dos Efluentes de carcinicultura no estuário do rio Potengi. Natal, RN: EESC/USP.
Dantas, A. S. (2009). Análise de sedimento de fundo: uma amostragem representativa do estuário Potengi/RN. Cent. Ciências Exatas e da Terra. Programa Pós-Graduação em Química. Available online at: https://repositorio.ufrn.br/jspui/handle/123456789/17618%0A (accessed December 9, 2018).
de Souza, M. M., Windmöller, C. C., and Hatje, V. (2011). Shellfish from Todos os Santos Bay. Bahia, Brazil: treat or threat? Mar. Pollut. Bull. 62, 2254–2263. doi: 10.1016/j.marpolbul.2011.07.010
de Souza, V. M., Laut, L. L. M., da Silva, F. S., de Figueiredo, A. G., Vital, H., and Frazão, E. (2010). Benthic foraminifera and bacterial activity as a proxy for environmental characterization in Potengi estuary. Rio Grande do Norte, Brazil. Anu. do Inst. Geociencias 33, 20–34.
Decreto No° 55. 871 (1965). Dispõe Sobre Normas Regulamentadoras do Emprego de Aditivos Para Alimentos. Brasil: ANVISA.
Defew, L. H., Mair, J. M., and Guzman, H. M. (2005). An assessment of metal contamination in mangrove sediments and leaves from Punta Mala Bay. Pacific Panama. Mar. Pollut. Bull. 50, 547–552. doi: 10.1016/j.marpolbul.2004.11.047
Dragun, Z., Raspor, B., Erk, M., Ivanković, D., and Pavičić, J. (2006). The influence of the biometric parameters on metallothionein and metal level in the heat-treated cytosol of the whole soft tissue of transplanted mussels. Environ. Monit. Assess. 114, 49–64. doi: 10.1007/s10661-006-1077-6
Farrington, J. W., Tripp, B. W., Tanabe, S., Subramanian, A., Sericano, J. L., Wade, T. L., et al. (2016). Edward D. Goldberg’s proposal of “the Mussel Watch”: reflections after 40 years. Mar. Pollut. Bull. 110, 501–510. doi: 10.1016/j.marpolbul.2016.05.074
Frazão, E., and Vital, H. (2007). Estruturas rasas de gás em sedimentos no estuário potengi (nordeste do Brasil). Rev. Bras. Geofis. 25, 17–26. doi: 10.1590/s0102-261x2007000500003
Frazão, E. P. (2003). Caracterização Hidrodinâmica e Morfo-Sedimentar do Estuário Potengi e Áreas Adjacentes: Subsídios Para Controle e Recuperação Ambiental no Caso de Derrames de Hidrocarboneto. Rio Grande do Norte: Universidade Federal do Rio Grande do Norte, 195.
Gain, I. E., Brewton, R. A., Reese Robillard, M. M., Johnson, K. D., Smee, D. L., and Stunz, G. W. (2017). Macrofauna using intertidal oyster reef varies in relation to position within the estuarine habitat mosaic. Mar. Biol. 164:8. doi: 10.1007/s00227-016-3033-5
Garrett, R. G. (2000). Natural sources of metals to the environment. Hum. Ecol. Risk Assess. An Int. J. 6, 945–963. doi: 10.1080/10807030091124383
Goldberg, E. D. (1975). The mussel watch - A first step in global marine monitoring. Mar. Pollut. Bull. 6:111. doi: 10.1016/0025-326X(75)90271-4
González-Farias, F. A. (1988). Metales pesados en cuatro especies de organismos filtradores de la región costera de Mazatlán: técnicas de extracción y niveles de concentración. Rev. Int. Contam. Ambient. 4, 33–41.
Griboff, J., Horacek, M., Wunderlin, D. A., and Monferran, M. V. (2018). Bioaccumulation and trophic transfer of metals, As and Se through a freshwater food web affected by antrophic pollution in Córdoba, Argentina. Ecotoxicol. Environ. Saf. 148, 275–284. doi: 10.1016/j.ecoenv.2017.10.028
Guilding (1828). In Crassostrea rhizophorae, ed. MolluscaBase 107–114. Available online at: http://marinespecies.org/aphia.php?p=taxdetails&id=420777 (accessed June 18, 2019).
Hardiman, S., and Pearson, B. (1995). Heavy metals, TBT and DDT in the Sydney rock oyster (Saccostrea commercialis) sampled from the Hawkesbury River estuary, NSW, Australia. Mar. Pollut. Bull. 30, 563–567. doi: 10.1016/0025-326X(95)00078-2
Hedges, J. I., and Keil, R. G. (1999). Organic geochemical perspectives on estuarine processes: sorption reactions and consequences. Mar. Chem. 65, 55–65. doi: 10.1016/S0304-4203(99)00010-9
Hwang, G.-C., Kim, S.-J., and Lee, E.-H. (1984). Heavy metal content of oyster crassostrea-gigas blue mussel mytilus-edulis and growing water in hansan-geoje Bay Korea. Bull. Natl. Fish. Univ. Pusan 24, 121–128.
IBGE (2007). Contagem de População: Resultados do Diário Oficial da União (05/10/2007). Brazil: Diário Of. da União.
Kanhai, L. D. K., Gobin, J. F., Beckles, D. M., Lauckner, B., and Mohammed, A. (2014). Metals in sediments and mangrove oysters (Crassostrea rhizophorae) from the Caroni Swamp. Trinidad. Environ. Monit. Assess. 186, 1961–1976. doi: 10.1007/s10661-013-3510-y
Kulkarni, R., Deobagkar, D., and Zinjarde, S. (2018). Metals in mangrove ecosystems and associated biota: a global perspective. Ecotoxicol. Environ. Saf. 153, 215–228. doi: 10.1016/j.ecoenv.2018.02.021
Lacerda, L. D., Martinelli, L. A., Rezende, C. E., Mozeto, A. A., Ovalle, A. R. C., Victoria, R. L., et al. (1988). The fate of trace metals in suspended matter in a mangrove creek during a tidal cycle. Sci. Total Environ. 75, 169–180. doi: 10.1016/0048-9697(88)90030-7
Lewis, M., Pryor, R., and Wilking, L. (2011). Fate and effects of anthropogenic chemicals in mangrove ecosystems: a review. Environ. Pollut. 159, 2328–2346. doi: 10.1016/j.envpol.2011.04.027
Lima, N., De Lacerda, L. D., Pfeiffer, W. C., and Fiszman, M. (1986). Temporal and spatial variability in zn, cr, cd and fe concentrations in oyster tissues (Crassostrea brasiliana lamarck, 1819) from sepetiba bay, brazil. Environ. Technol. Lett. 7, 453–460. doi: 10.1080/09593338609384432
Medeiros, M. L. (2009). Estudo Multitemporal da Dinâmica Espacial do estuário Potengi / RN utilizando como base os dados de Sensoriamento Remoto. Rio Grande do Norte: Universidade Federal do Rio Grande do Norte.
Mendoza-Carranza, M., Sepúlveda-Lozada, A., Dias-Ferreira, C., and Geissen, V. (2016). Distribution and bioconcentration of heavy metals in a tropical aquatic food web: a case study of a tropical estuarine lagoon in SE Mexico. Environ. Pollut. 210, 155–165. doi: 10.1016/j.envpol.2015.12.014
Mercado-Silva, N. (2005). Condition index of the eastern oyster, Crassostrea virginica (Gmelin, 1971) in Sapelo Island Georgia - Effects of site, position on bed and pea crab parasitism. J. Shellfish Res. 24, 121–126. doi: 10.2983/0730-8000(2005)24[121:cioteo]2.0.co;2
Mo, C., and Neilson, B. (1993). Weight and salinity effects on zinc uptake and accumulation for the American oyster (Crassostrea virginica. Gmelin). Environ. Pollut. 82, 191–196. doi: 10.1016/0269-7491(93)90116-6
Mubiana, V. K., Vercauteren, K., and Blust, R. (2006). The influence of body size, condition index and tidal exposure on the variability in metal bioaccumulation in Mytilus edulis. Environ. Pollut. 144, 272–279. doi: 10.1016/j.envpol.2005.12.017
National Institute of Standards and Technology (2017). Standard Reference Material® 2976 Trace Elements and Methylmercury in Mussel Tissue (Freeze-Dried). 1–8. Available online at: http://www.nist.gov/srm (accessed January 25, 2018).
Nicodemo, S. C. T. E. S., Andrade, I. C., Silva, K. S. T., Fonseca, J. R., and Medeiros, G. F. (2010). Avaliação ecotoxicológica preliminar de amostras de coluna d água e sedimento do Complexo Estuarino do Jundiaí-Potengi, Natal / RN,. 2oCongresso Int. Tecnol. para o Meio Ambient. 8. Available online at: https://siambiental.ucs.br/congresso/getArtigo.php?id=90&ano=_segundo (accessed September 8, 2019).
Nordberg, G. F., Fowler, B. A., and Nordberg, M. (2009). Handbook of The Toxicology of Metal. 3oEd. Available online at: http://www.amazon.com/Handbook-Toxicology-Metals-Third-Edition/dp/0123694132 (accessed August 18, 2017).
Nordberg, G. F., Fowler, B. A., Nordberg, M., and Friberg, L. T. (2007). Handbook on the Toxicology of Metals. Amsterdam: Elsevier.
Pereira, O. M., Henriques, M. B., Zenebon, O., Sakuma, A., and Kira, C. S. (2002). Determination of Pb, Cd, Hg, Cu and Zn levels in molluscs (Crassostrea brasiliana, Perna perna and Mytella falcata). Rev. Inst. Adolvo Lutz 61, 19–25.
Portaria No° 685 (1998). Fixa Limites Máximos de Tolerância de Contaminantes Químicos em Alimentos. Brazil: Diário Of. da União.
Rainbow, P. S. (2006). Biomonitoring of trace metals in estuarine and marine environments. Australas. J. Ecotoxicol. 12, 107–122.
Rainbow, P. S., Liu, F., and Wang, W. X. (2015). Metal accumulation and toxicity: the critical accumulated concentration of metabolically available zinc in an oyster model. Aquat. Toxicol. 162, 102–108. doi: 10.1016/j.aquatox.2015.03.007
Ramos Silva, C. A., Rainbow, P. S., and Smith, B. D. (2003). Biomonitoring of trace metal contamination in mangrove-lined Brazilian coastal systems using the oyster Crassostrea rhizophorae: comparative study of regions affected by oil, salt pond and shrimp farming activities. Hydrobiologia 501, 199–206. doi: 10.1023/A:1026242417427
Rebelo, M. F., Amaral, M. C. R., and Pfeiffer, W. C. (2005). Oyster Condition Index in Crassostrea rhizophorae (Guilding, 1828) from a heavy-metal polluted coastal Lagoon. Brazilian J. Biol. 65, 345–351. doi: 10.1590/S1519-69842005000200019
Roberts, D. F., Elliott, M., and Read, P. A. (1986). Cadmium contamination, accumulation and some effects of this metal in mussels from a polluted marine environment. Mar. Environ. Res. 18, 165–183. doi: 10.1016/0141-1136(86)90031-0
Roesijadi, G. (1992). Metallothioneins in metal regulation and toxicity in aquatic animals. Aquat. Toxicol. 22, 81–114. doi: 10.1016/0166-445X(92)90026-J
Roesijadi, G. (1996). “Metallothionein and its role in toxic metal regulation,” in Comparative Biochemistry and Physiology - C Pharmacology Toxicology and Endocrinology, (Amsterdam: Elsevier Inc), 117–123. doi: 10.1016/0742-8413(95)02077-2
Sabadini-Santos, E., Senez, T. M., Silva, T. S., Moreira, M. R., Mendonça-Filho, J. G., Santelli, R. E., et al. (2014). Organic matter and pyritization relationship in recent sediments from a tropical and eutrophic bay. Mar. Pollut. Bull. 89, 220–228. doi: 10.1016/j.marpolbul.2014.09.055
Santos, A. B. (2018). Resposta Morfo-Sedimentar do Estuário do Rio Potengi aos Impactos de Dragagem. Rio Grande do Norte: Universidade Federal do Rio Grande do Norte.
Senez-Mello, T. M., Crapez, M. A. C., Ramos Silva, C. A., Silva, E. T., and Fonseca, E. M. (2020a). Dataset for: spatial variability of heavy metals in native oysters from an anthropogenically impacted estuary in Northeastern Brazil. doi: 10.6084/m9.figshare.12093456.v1
Senez-Mello, T. M., Crapez, M. A. C., Ramos Silva, C. A., Silva, E. T., and Fonseca, E. M. (2020b). Heavy metals bioconcentration in Crassostrea rhizophorae: a site-to-site transplant experiment at the Potengi estuary. Rio Grande do Norte, Brazil. Sci. Rep. 10:246. doi: 10.1038/s41598-019-57152-w
Shi, B., Wang, T., Zeng, Z., Zhou, L., You, W., and Ke, C. (2019). The role of copper and zinc accumulation in defense against bacterial pathogen in the fujian oyster (Crassostrea angulata). Fish Shellfish Immunol. 92, 72–82. doi: 10.1016/j.fsi.2019.05.049
Silva, C. A., Rainbow, P. S., Smith, B. D., and Santos, Z. L. (2001). Biomonitoring of TRACE metal contamination in the Potengi estuary, Natal (Brazil), using the oyster Crassostrea Rhizophorae, a local food source. Water Res. 35, 4072–4078. doi: 10.1016/S0043-1354(01)00144-0
Silva, C. A. R., Smith, B. D., and Rainbow, P. S. (2006). Comparative biomonitors of coastal trace metal contamination in tropical South America (N. Brazil). Mar. Environ. Res. 61, 439–455. doi: 10.1016/j.marenvres.2006.02.001
Souza, F. E. S., and Ramos Silva, C. A. (2011). Ecological and economic valuation of the Potengi estuary mangrove wetlands (NE. Brazil) using ancillary spatial data. J. Coast. Conserv. 15, 195–206. doi: 10.1007/s11852-010-0133-0
TIBCO (2017). StatisticaTM Software Inc. Available online at: https://www.tibco.com/products/data-science (accessed March 25, 2018).
Tolhurst, T. J., Defew, E. C., De Brouwer, J. F. C., Wolfstein, K., Stal, L. J., and Paterson, D. M. (2006). Small-scale temporal and spatial variability in the erosion threshold and properties of cohesive intertidal sediments. Cont. Shelf Res. 26, 351–362. doi: 10.1016/j.csr.2005.11.007
Usman, A. R. A., Alkredaa, R. S., and Al-Wabel, M. I. (2013). Heavy metal contamination in sediments and mangroves from the coast of Red Sea: avicennia marina as potential metal bioaccumulator. Ecotoxicol. Environ. Saf. 97, 263–270. doi: 10.1016/j.ecoenv.2013.08.009
Watling, H. R., and Watling, R. J. (1976). Trace metals in oysters from Knysna estuary. Mar. Pollut. Bull. 7, 45–48. doi: 10.1016/0025-326X(76)90090-4
Keywords: estuarine ecosystem, metal pollution, bioavailability, bioaccumulation, benthic organisms
Citation: Senez-Mello TM, Crapez MAC, Ramos e Silva CA, da Silva ET and da Fonseca EM (2020) Spatial Variability of Heavy Metals in Native Oysters From an Anthropogenically Impacted Estuary in Northeastern Brazil. Front. Mar. Sci. 7:412. doi: 10.3389/fmars.2020.00412
Received: 27 December 2019; Accepted: 12 May 2020;
Published: 16 June 2020.
Edited by:
Jérôme Cachot, Université de Bordeaux, FranceReviewed by:
Emilie Pauline Dassié, UMR 5805 Environnements et Paléoenvironnements Océaniques et Continentaux (EPOC), FranceJosé Lino Vieira De Oliveira Costa, University of Lisbon, Portugal
Copyright © 2020 Senez-Mello, Crapez, Ramos e Silva, da Silva and da Fonseca. This is an open-access article distributed under the terms of the Creative Commons Attribution License (CC BY). The use, distribution or reproduction in other forums is permitted, provided the original author(s) and the copyright owner(s) are credited and that the original publication in this journal is cited, in accordance with accepted academic practice. No use, distribution or reproduction is permitted which does not comply with these terms.
*Correspondence: Thaise Machado Senez-Mello, thaise_senez@id.uff.br