- 1California Water Science Center, U.S. Geological Survey, Sacramento, CA, United States
- 2Golden Gate National Recreation Area, National Park Service, San Francisco, CA, United States
- 3College of Environmental Science and Forestry, State University of New York, Syracuse, NY, United States
- 4Fisheries Ecology Division, Southwest Fisheries Science Center, National Oceanic and Atmospheric Administration Fisheries, Santa Cruz, CA, United States
- 5Center for Watershed Sciences, University of California, Davis, Davis, CA, United States
Bar-built coastal lagoons are dynamic ecosystems at the land-sea interface that are important habitats for a variety of species. This study examined the habitat ecology of two lagoon species, the endangered Tidewater Goby (Eucyclogobius newberryi) and the Prickly Sculpin (Cottus asper) by reconstructing individual life histories from patterns in the concentration of the element Sr (as ratioed to Ca; Sr:Ca) in otoliths. Specific objectives were to (1) elucidate any movements of individual fishes among three primary habitat components of typical bar-built lagoon systems: coastal ocean, brackish lagoon, and freshwater watershed streams, and (2) determine if either species exhibited a consistent life history as defined by a stereotypical otolith Sr:Ca chronology, which could be indicative of a consistent range of salinity or temperature occupied through ontogeny. Results suggested that Tidewater Goby was a lagoon resident and that Prickly Sculpin exhibited migrations between lagoon and watershed stream habitats. There was no strong evidence in either species of ocean occupancy or of a stereotypical Sr:Ca chronology, the latter suggesting the full range of available lagoon habitat in terms of salinity and temperature was likely utilized at all life stages. These findings add to the body of evidence that bar-built lagoons are not isolated habitats, and holistic management of these habitats with adjoining watershed and marine environments could increase habitat connectivity across the landscape, with potential benefits to fishes.
Introduction
Coastal lagoons are dynamic ecosystems at the land-sea interface that are important habitats for a variety of species (Barnes, 1980; Yáñez-Arancibia, 1985; Pérez-Ruzafa et al., 2019). They can be generally characterized as relatively small, shallow habitats typically connected to the ocean by a small inlet and exhibit a broad range of physical habitat and water quality conditions (Kjerfve, 1994). Bar-built lagoons represent a special form of the habitat in which the inlet is open to the ocean only episodically in response to a combination of factors driven primarily by wave energy from the ocean and flow dynamics from the watershed (Fong and Kennison, 2010; Behrens et al., 2013; Mcsweeney et al., 2014). Bar-built lagoons are common features along coastlines worldwide, including North and South America, Australia, New Zealand, and South Africa (e.g., Bell et al., 2001; Smakhtin, 2004; Mouillot et al., 2005; Haines et al., 2006; Hume et al., 2016).
The spatio-temporal dynamism of bar-built lagoons provides a variety of habitat functions for fishes, which can be broadly characterized as either temporary habitat for transient species or permanent habitat for resident species (Yáñez-Arancibia et al., 1994). In many cases, especially in CA, United States, where bar-built estuaries represent ∼50% of the region’s inland-coastal confluences (Heady et al., 2015; Clark and O’Connor, 2019), lagoon fish faunas are dominated by small-bodied demersal species (Monaco et al., 1990; Allen et al., 2006). Quantifying the habitat and movements of small, demersal fishes is a challenge in marine science and presents obstacles to fully understanding the ecology of coastal lagoons and their biota. An increasingly popular tool to address the challenge of tracking fish among aquatic ecosystems and elucidating coastal dispersal/migration is the application of otolith chemistry as a natural marker of fish life history (Elsdon et al., 2008; Walther and Limburg, 2012; Shao et al., 2018).
Otolith chemistry markers are an effective tool for reconstructing fish life histories, in part, because otolith elemental strontium (Sr) is positively correlated with Sr concentration in water and its salinity (Campana, 1999; Bath et al., 2000). While it is recognized that temperature and physiology can play a role in controlling otolith chemistry (Elsdon and Gillanders, 2004, 2002; Sturrock et al., 2015), it has been shown that approximately 80% of otolith Sr content is derived from the surrounding water for both freshwater and marine species (Farrell and Campana, 1996; Walther and Thorrold, 2009).
The objective of this study was to elucidate the habitat ecology of two fish species common to bar-built lagoons in CA, United States, using the chemical composition of their otoliths: the small-bodied, demersal fishes Tidewater Goby (Eucyclogobius newberryi) and Prickly Sculpin (Cottus asper). Otolith chemistry was an appropriate tool for this study because behavior and movement of Tidewater Gobies and Prickly Sculpins cannot be directly observed efficiently via traditional technologies. Tidewater gobies are generally considered lagoon residents but are thought to disperse among coastal lagoons via the Pacific Ocean (Lafferty et al., 1999a, b). Tidewater gobies are broadly distributed in lagoons but there is no knowledge of the movements of individuals across habitats or if the species exhibits a consistent life history in terms of salinity or temperature occupied through ontogeny (Swift et al., 1989; Swenson, 1999; Chamberlain, 2006). Prickly sculpins are thought to exhibit a range of life history strategies that could include lagoon residency or migrations between coastal estuaries and watershed streams. While amphidromy has been suggested in some Cottus species (Goto and Arai, 2006; Dennenmoser et al., 2014), similar movements have only been inferred in coastal California populations of Prickly Sculpin indirectly based on inferences from size distributions across space (Brown et al., 1995; Moyle, 2002).
This study uses otolith chemistry to contribute new empirical information on movement patterns and habitat use for both species. Specifically, otolith chemistry was applied to (1) elucidate movements of individual Tidewater Gobies or Prickly Sculpins among three primary habitat components of typical bar-built lagoon systems: coastal ocean, brackish lagoon, and freshwater watershed streams, and (2) determine if either species exhibited a consistent life history as defined by a stereotypical otolith Sr chronology, which could be indicative of a consistent range of salinities or temperatures occupied through ontogeny. This information would be useful for conservation and management as it would provide greater knowledge of the habitat needs and life histories of coastal lagoon fishes, many of which, including the Tidewater Goby, are imperiled.
Materials and Methods
Study System
Rodeo Lagoon has similar characteristics to many of the bar-built lagoons along the northeastern Pacific. It is located within the Marin Headlands of the Golden Gate National Recreation Area, CA, United States, and is a key component of the United Nations Educational, Scientific, and Cultural Organization’s (UNESCO) Golden Gate Biosphere Reserve1. There are four key aquatic habitats comprising the system: Pacific Ocean, Rodeo Lagoon, Rodeo Lake, and watershed streams (Figure 1). Rodeo Lagoon is a relatively small (15.2 ha), shallow (1–2 m in depth) brackish coastal lagoon that is intermittently (∼30 days per year) connected to the Pacific Ocean when a sand bar at its seaward end breaches in response to sand erosion from high water levels in the lagoon and strong wave action from the Pacific Ocean. A weir and associated vehicle bridge have isolated the landward, eastern tip of Rodeo Lagoon to form Rodeo Lake. Connectivity between Rodeo Lagoon and Rodeo Lake is primarily limited to wet seasons when there is enough freshwater inflow from the watershed to overtop the ∼1.5 m weir. Freshwater inflow originates primarily from Gerbode and Rodeo Creeks, which drain the relatively small (∼777 ha) Rodeo Valley watershed.
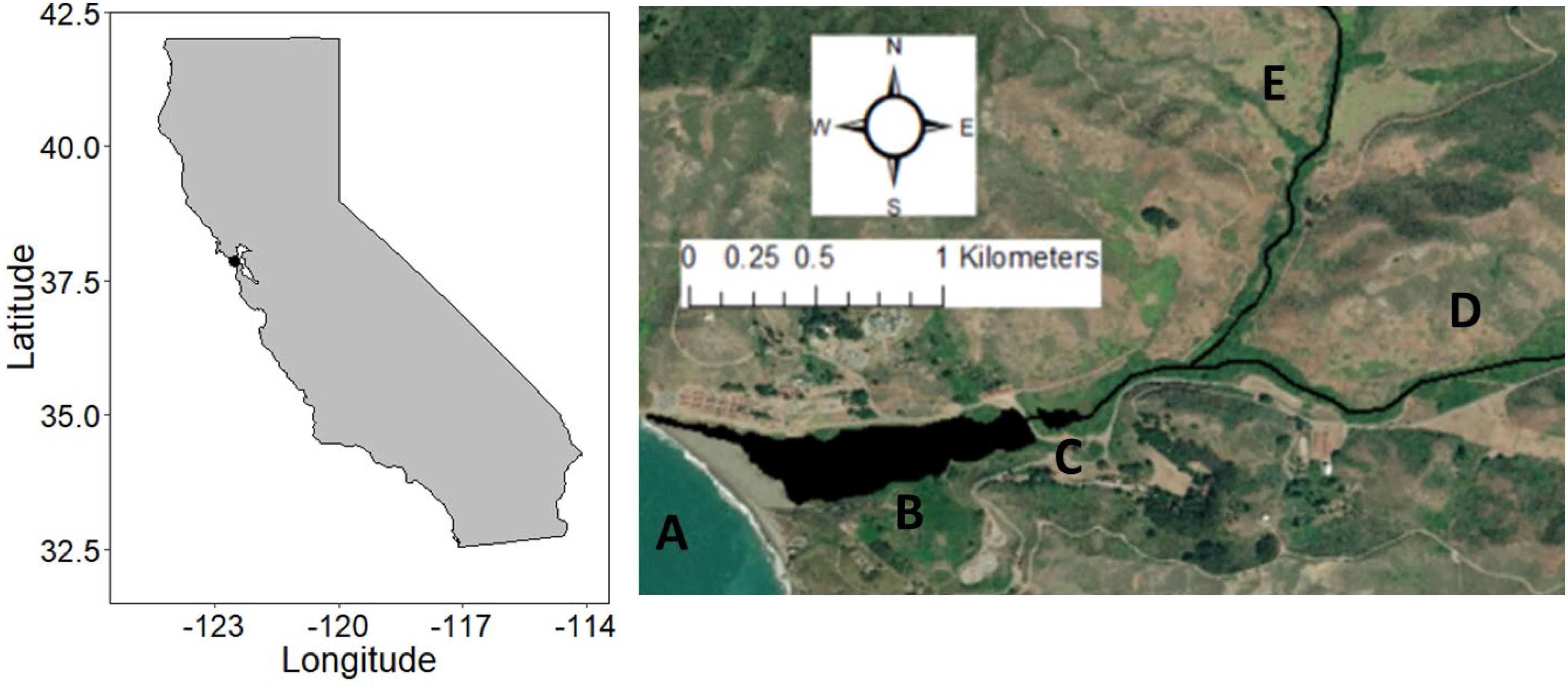
Figure 1. Map of the study area. A = Pacific Ocean. B = Rodeo Lagoon. C = Rodeo Lake. D = Rodeo Creek. E = Gerbode Creek. Imagery source: Esri: DigitalGlobe, GeoEye, EarthStar Geographics, CNES/Airbus DS, USDS, USGS, AeroGRID, IGN, and the GIS User community.
Rodeo Lagoon is considered hypereutrophic and characterized by extremely high productivity, spatio-temporal variability in stratification, large fluctuations in dissolved oxygen concentration, and limited water circulation (Cousins et al., 2010; Drake et al., 2010). Salinity varies spatially and seasonally in Rodeo Lagoon. Salinity in the seaward end of Rodeo Lagoon can temporarily match local seawater [∼28 practical salinity units (PSU)] during breaches. For the time period of approximately 1 year leading up to the collection of fishes in our study, there were at least 5 instances of wave overwash from the Pacific Ocean into the Rodeo Lagoon and 3 instances of a breach with full connectivity (totaling 32 days). When the lagoon is isolated from the ocean, salinity typically ranges from approximately 0–10 PSU spatially (horizontally and vertically) and temporally (seasonally) in response to freshwater inflow from the watershed. Rodeo Lake and the watershed streams are perennially freshwater with a salinity of 0 PSU. Water temperature ranges seasonally from approximately 8–20°C in Rodeo Lagoon and Rodeo Lake.
Water Chemistry
Baseline Sr and Ca concentrations in the system were determined from discrete water samples collected from the Pacific Ocean, Rodeo Lake, and Rodeo Lagoon. Rodeo Lake was assumed to be a surrogate for the watershed streams since it is directly fed by them and all are freshwater. A total of 17 water samples was collected in April, May, and August 2016. Samples were collected with sterile containers and passed through 0.45 μm filters into acid-washed polyethylene containers. Concentrations of Sr and Ca were measured at the U.S. Geological Survey’s National Water Quality Laboratory in Reston, VA, United States. Ambient salinity and temperature conditions associated with each water sample were measured at the time of collection with a handheld YSI EXO2 sonde (Yellow Springs Instruments, Yellow Springs, OH, United States). One-way analysis of variance with Tukey’s pairwise comparisons was used to test for differences in Sr:Ca among Pacific Ocean, Rodeo Lagoon, and Rodeo Lake.
Study Species
Tidewater Goby and Prickly Sculpin are sympatric and relatively abundant in Rodeo Lagoon. Prickly Sculpin also occupies Rodeo Lake and the watershed streams. The two species, along with Threespine Stickleback (Gasterosteus aculeatus), are the dominant fishes of Rodeo Lagoon as determined from annual fish surveys conducted by the National Park Service (Fong, unpublished data). Tidewater Gobies and Prickly Sculpins are readily collected in beach seine samples and can often be visually observed in shallow water under suitable viewing conditions. Despite its abundance in Rodeo Lagoon, Tidewater Goby is listed as endangered under the U.S. Endangered Species Act. Primary threats to the species across its range include the alteration and loss of coastal lagoons, which are its sole habitat (U.S. Fish and Wildlife Service [USFWS], 2005). In contrast, Prickly Sculpin is a common species broadly distributed in streams, lakes, and estuaries across ∼5,000 km of eastern Pacific coastline, including inland California (Krejsa, 1967; Moyle, 2002). Tidewater Gobies grow to approximately 5 cm and reach 1 year of age while Prickly Sculpins grow to approximately 10 cm and reach 3 years of age. Both species have pelagic larvae and are omnivorous as juvenile and adults feeding primarily on a variety of micro- and macro-crustaceans and insects (Swenson and McCray, 1996; Moyle, 2002; Feyrer et al., 2003; Spies et al., 2014).
Individual Tidewater Gobies and Prickly Sculpins examined in this study were collected freshly dead from around the perimeter of Rodeo Lagoon following a hypoxia-induced fish kill that occurred on 08 August 2016. Additional Prickly Sculpins were collected on 12 April 2016 from Rodeo Lagoon using a beach seine and from Rodeo Creek using a minnow trap. In total, 14 Tidewater Gobies (mean standard length = 40 mm, standard deviation = 2) and 10 Prickly Sculpins (mean standard length = 62 mm, standard deviation = 12) were examined (Table 1).
Otolith Preparation and Analysis
Sagittae otoliths were extracted from Tidewater Gobies and Prickly Sculpins. A single otolith from each individual was cleaned and embedded in West Systems 105 epoxy resin and sectioned in the transverse plane for Tidewater Goby and the frontal plane for Prickly Sculpin using a low speed diamond saw. Otoliths were polished to reveal the growth plane and a smooth surface from core to edge using 1500 grit sandpaper and 3 μm lapping film. Finished preparations were cleaned by sonicating in deionized water and wiped with ethanol prior to elemental measurements.
Chemical composition of otoliths was measured at Cornell University’s High Energy Synchrotron Source (CHESS; Cornell University, Ithaca, NY, United States) using scanning X-ray fluorescence microscopy (SXFM) on the F3 beamline per established techniques (Limburg et al., 2007). This instrument allows for two-dimensional spatial mapping of elemental concentrations across the full otolith surface using a non-destructive technique with minimal interferences among elements. Briefly, a multi-layer monochromater (0.6% bandwidth) produced an X-ray with 16 KeV energy focused on the otolith with a single glass capillary necessary to achieve 5–20 μm spot resolution over the entire otolith. The photon flux was about 0.5 × 1011 counts per second and a florescence spectrum integrated for 1 s. Fluorescence spectra were calibrated using an in-house otolith pellet previously described (Limburg et al., 2007, 2011).
Distributions of absolute concentrations (ppm) of Sr and Ca were processed with PyMCA (Sole et al., 2007). Commercial geographic information system software (ArcMap v10.5, ESRI, Redlands, CA, United States) was used to process elemental maps of the otolith surfaces and analyze spatial patterns following established practices (Limburg et al., 2007). Sr and Ca concentrations along linear transects across otolith surfaces were extracted from the elemental maps using tools available in ArcMap. Transects were made along the primary growth axis of each otolith from core to edge (Figure 2). Discrete values of Sr and Ca concentration were captured approximately every 1 μm along the transects to generate a chronological time series of data for each individual from approximately birth to death. Sr was ratioed to Ca (Sr:Ca) for data analyses and interpretation. Individual chronologies were visually inspected for patterns. Additionally, hierarchical time series cluster analysis was used to test for similarities in chronologies among individuals of each species. Analyses were implemented in the “dtwclust” package in the R statistical computing environment (Sardá-Espinosa, 2019a, b). Cluster validity indices were calculated to objectively determine the appropriate number of clusters.
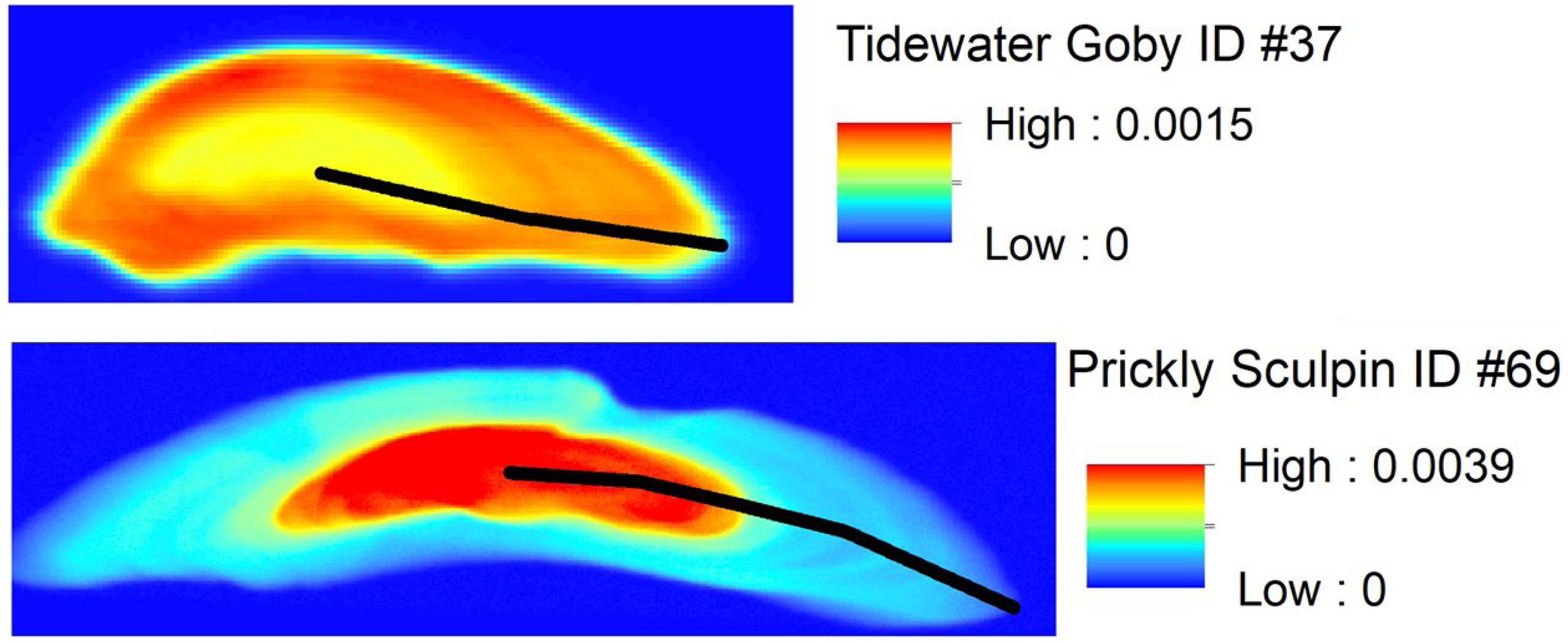
Figure 2. Spatial distribution of Sr concentration (ppm) in example otoliths from a Tidewater Goby (frontal section) and Prickly Sculpin (transverse section). Lines on each otolith represent the growth plane where Sr:Ca chronologies were obtained. Note that images are not on the same scale: line lengths are 773 μm on the Tidewater Goby otolith and 2,683 μm on the Prickly Sculpin otolith.
Results
Water Chemistry
The 17 water samples examined in this study spanned the full range of salinity that fish could have potentially encountered (0–28 PSU) and a wide temperature range (12–20°C) (Table 2). Absolute Sr concentrations ranged from 56 to 7,568 ppm (mean = 1902, standard deviation = 2350). Absolute Ca concentrations ranged from 8,346 to 381,300 ppm (mean = 101,849, standard deviation = 113,407). Sr:Ca values ranged from 0.0067 to 0.0205 (mean = 0.0157, standard deviation = 0.0044). The relation between Sr:Ca and salinity was exponentially asymptotic in that Sr:Ca values increased rapidly with salinity up to about 8 PSU and then remained relatively flat thereafter (Figure 3). Sr:Ca values were statistically unique among habitat types (P < 0.001) with values highest in the Pacific Ocean (mean = 0.0201, standard deviation = 0.0005), intermediate in Rodeo Lagoon (mean = 0.01724, standard deviation = 0.0013), and lowest in Rodeo Lake (mean = 0.0070, standard deviation = 0.0005).
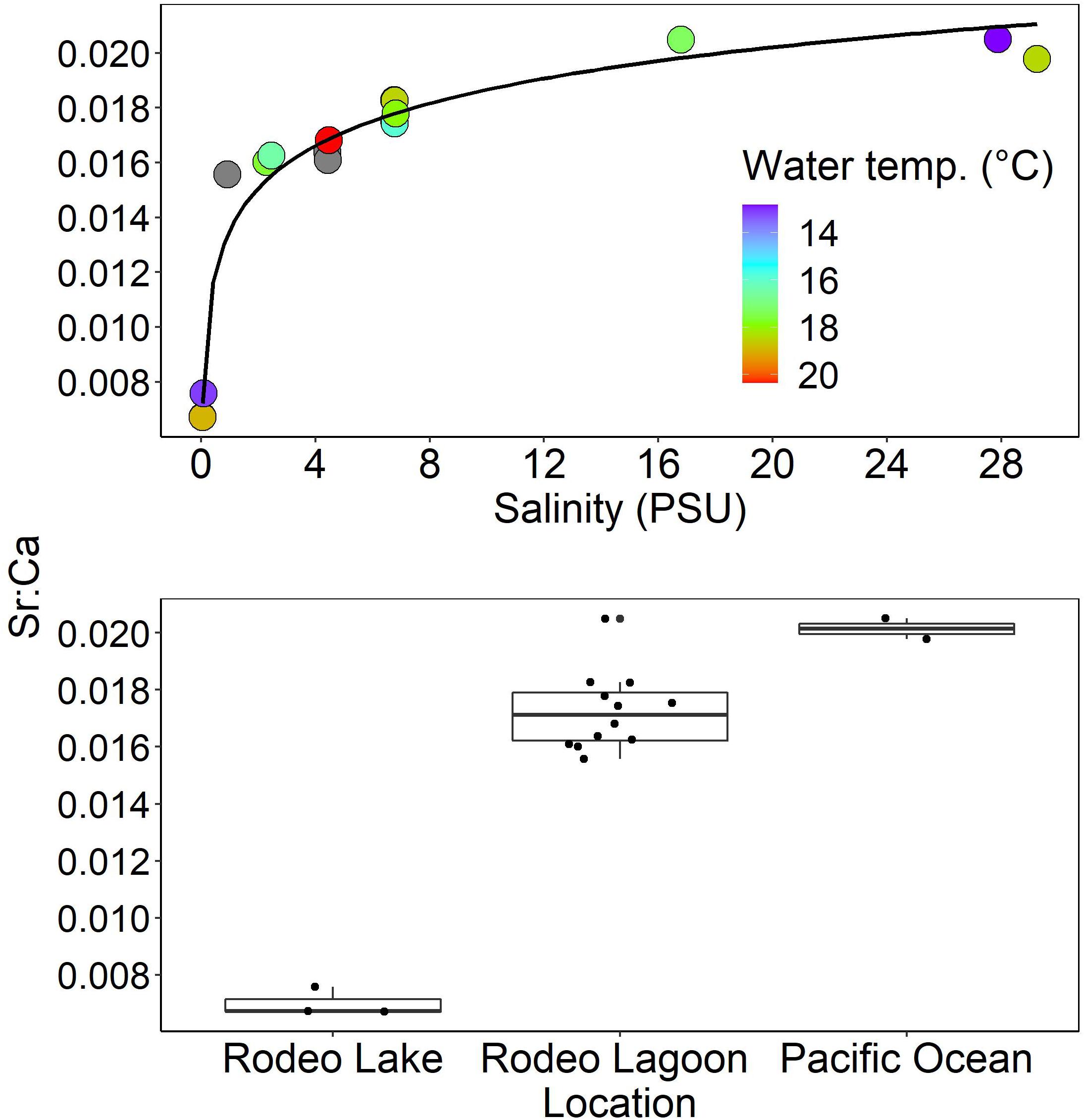
Figure 3. Upper: Relation between salinity and Sr:Ca in the Rodeo Lagoon system. The line is an exponential curve fitted to the data. Water temperature values were measured at the time of water sample collection; dark gray points do not have corresponding temperature data. Lower: Boxplot representation of the same Sr:Ca data aggregated according to samples collected in Pacific Ocean, Rodeo Lagoon, and Rodeo Lake. Jitter was imposed on the data points in the lower plot to avoid superimposition. Box plot shows the median, 25th and 75th percentile and upper and lower values of 1.5* (inter quartile range).
Otolith Chemistry
A Sr:Ca salinity and otolith relationship was developed using salinity and Sr:Ca measured in water across habitats and Sr:Ca values measured in otoliths associated with capture locations for individuals. It was posited that 0.002 was a conservative breakpoint value of otolith Sr:Ca that distinguished occupancy in Rodeo Lagoon (>0.002) versus upstream habitats such as Rodeo Lake and watershed streams (<0.002) for both species. This value was based on a weight of evidence that included corresponding salinity and Sr:Ca water measurements across the habitats and Sr:Ca otolith values at capture locations for individuals. There was further support for this freshwater-lagoon cut-off given that none of the Tidewater Gobies had Sr:Ca values < 0.002 and that they have never been documented in upstream habitats. The Sr:Ca values comprising Tidewater Goby otolith chronologies ranged from 0.0024 to 0.0066 (mean = 0.0043, standard deviation = 0.0009) while the Sr:Ca values comprising Prickly Sculpin otolith chronologies ranged from 0.0008 to 0.0113 (mean = 0.0058, standard deviation = 0.0019) (Figure 4).
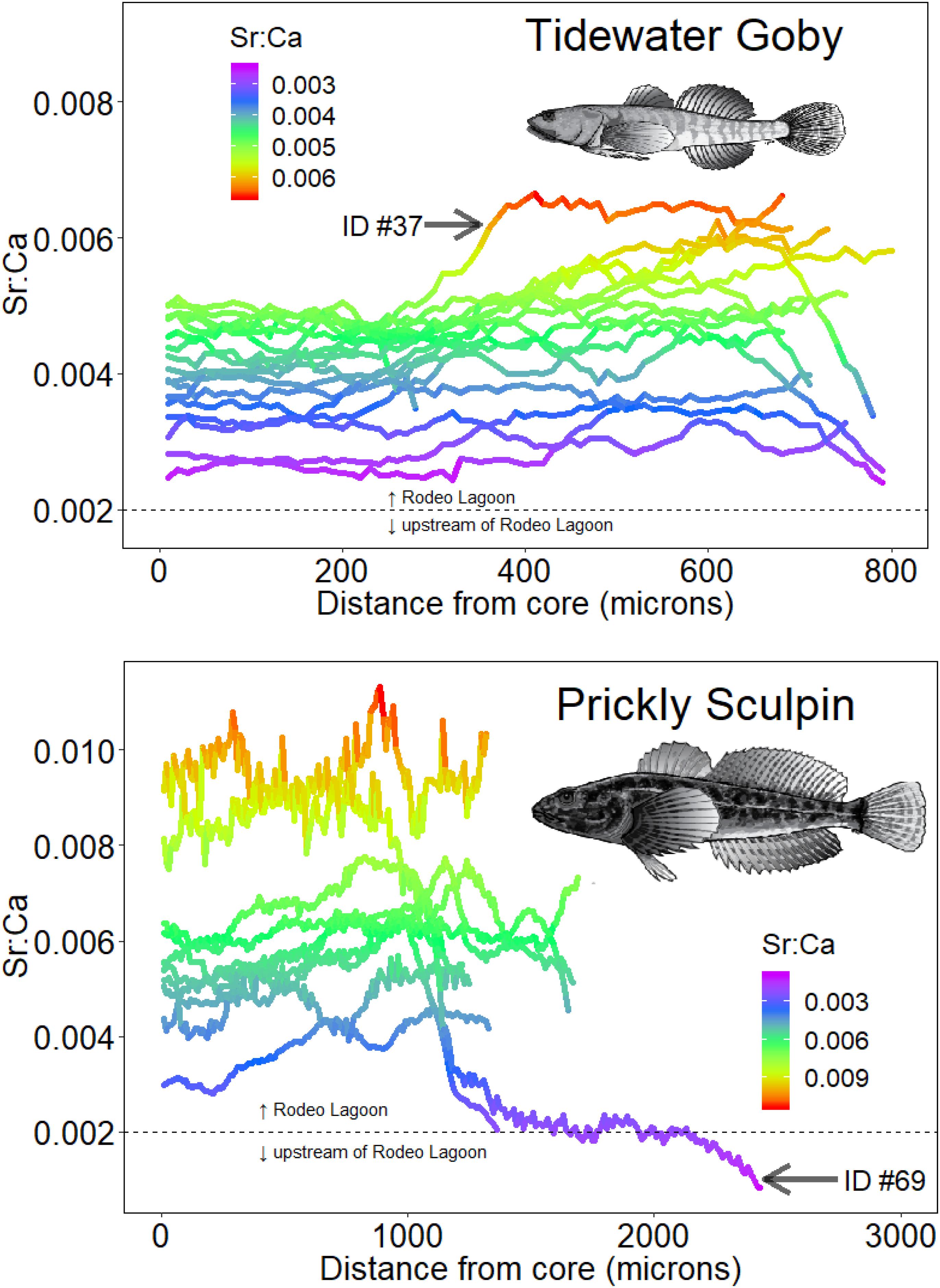
Figure 4. Otolith Sr:Ca chronologies of Tidewater Gobies (upper panel) and Prickly Sculpins (lower panel). The horizontal dashed lines show the Sr:Ca value distinguishing Rodeo Lagoon from upstream habitats. For illustrative purposes, otoliths of the noted individuals are shown in Figure 2. The two Prickly Sculpin with ending Sr:Ca values at or below 0.002 were the individuals that were collected from Rodeo Creek upstream of Rodeo Lagoon.
The range of Sr:Ca values exhibited in Tidewater Goby otoliths were consistent with all individuals having solely inhabited Rodeo Lagoon (Figure 4). In contrast, the range of Sr:Ca values exhibited in Prickly Sculpin otoliths demonstrated movement between Rodeo Lagoon and the watershed streams (Figure 4). Specifically, the chronologies of two Prickly Sculpins that were captured in Rodeo Creek suggested they had been born in Rodeo Lagoon. While we cannot discount possible marine habitat use given the limited resolution of Sr:Ca to resolve brackish from fully marine habitats, we would have expected higher Sr:Ca values in otoliths if individuals had occupied the Pacific Ocean given the water values were statistically higher in the Pacific Ocean compared to Rodeo Lagoon (Figure 3). We did not find any strong evidence that any individuals of either species occupied the Pacific Ocean.
Hierarchical time series cluster analysis and cluster validation indices suggested little similarity in Sr:Ca chronologies among individuals of each species, indicating that there was no consistent life history in either species (Supplementary Material). Specifically, eighty-six percent (12 of 14) of individual Tidewater Gobies and eighty percent (8 of 10) of individual Prickly Sculpins exhibited unique chronologies, thereby providing no evidence of a stereotypical otolith Sr:Ca chronology in either species.
Discussion
This study demonstrated the utility of otolith chemistry as a tool to generate habitat and life history information on fishes that would have otherwise been costly and challenging to obtain. In this case, reconstructing habitat use of individual fishes with otolith chemistry was possible because of sufficient variation in aqueous Sr:Ca among key habitat components of the Rodeo Lagoon system. This facilitated observations that sampled Tidewater Gobies were resident to Rodeo Lagoon and that some Prickly Sculpins exhibited migration from Rodeo Lagoon upstream into watershed streams.
The relatively small number of individuals examined was unavoidable given the endangered status of Tidewater Goby. Nonetheless, this study took advantage of a rare opportunity to examine an endangered species and generate missing and direct empirical information on the habitat and movements of coastal lagoon fishes. It is also important to note that while this study provides information on the range of habitat and behaviors possible, behaviors may vary in other systems in response to unique hydrology and associated physico-chemical properties.
The absence of stereotypical Sr:Ca chronologies in Tidewater Goby and Prickly Sculpin suggests individuals used a diversity of microhabitats and exhibited variable movement patterns and/or that individuals experienced a range of different physio-chemical habitat conditions expressed by the dynamic nature of the lagoon. The range of Sr:Ca values exhibited within and between each species suggested that the full range of salinity and temperature within Rodeo Lagoon was likely occupied across all life stages. What remains unclear is if a specific range of temperature or salinity provides fitness benefits to either species. This could potentially be resolved with studies that integrate health indicators with other otolith chemistry markers, such as oxygen isotopes (δ18O) that could potentially provide a higher level of resolution of salinities or temperatures occupied by individuals (Walther and Limburg, 2012; Willmes et al., 2019).
The information generated by this study has important implications for the conservation and management of bar-built lagoons and their biota. Foremost, these findings add to the body of evidence that bar-built lagoons are not isolated habitats, and holistic management of these habitats with adjoining watershed and marine environments could increase habitat connectivity across the landscape, with potential benefits to fishes. Ecosystem-level management of bar-built lagoon systems would benefit a diverse suite of fishes. In addition to the species examined in this study, bar-built lagoons are important habitats that provide consequential fitness benefits for anadromous salmonids, many of which are protected under the U.S. Endangered Species Act (e.g., Shapovalov and Taft, 1954; Bond et al., 2008; Hayes et al., 2008). Additionally, it has been demonstrated that artificial manipulation of connectivity between bar-built lagoons and the ocean can cause devastating fish kills (Swift et al., 2018). Specific to the study system, modification or removal of the weir that forms Rodeo Lake would potentially increase the amount of habitat area available to support the endangered Tidewater Goby and remove a passage barrier that would potentially benefit highly mobile anadromous and amphidromous species if the reclaimed habitat is suitable (Hale et al., 2016).
Data Availability Statement
Data generated for this study are available from the U.S. Geological Survey’s ScienceBase catalog (Steinke and Feyrer, 2020; https://doi.org/10.5066/P9PZMELL).
Ethics Statement
Samples examined in this study were collected with authority granted in U.S. National Park Service Scientific Research and Collecting Permit GOGA-2016-SCI-0005 and U.S. Fish and Wildlife Service Permit #TE036499-8.
Author Contributions
RJ, FF, and KL analyzed the samples. FF analyzed the data and prepared the figures. All authors contributed to writing the manuscript and design of the study.
Funding
Funding was provided by the U.S. Geological Survey-National Park Service Water Quality Partnership. The Cornell High Energy Synchrotron Source was supported by the National Science Foundation under award DMR-1332208.
Conflict of Interest
The authors declare that the research was conducted in the absence of any commercial or financial relationships that could be construed as a potential conflict of interest.
Acknowledgments
We extend our appreciation to O. Patton and D. Ayers for assistance with field work, to T. Kraus and E. Stumpner for processing water samples, to G. Whitman for preparing and processing otoliths, to R. Huang for assistance at the Cornell High Energy Synchrotron Source, to E. Gusto for creating the fish illustrations, and to V. Larwood for assistance creating the map. Any use of trade, firm, or product names is for descriptive purposes only and does not imply endorsement by the U.S. Government.
Supplementary Material
The Supplementary Material for this article can be found online at: https://www.frontiersin.org/articles/10.3389/fmars.2020.00417/full#supplementary-material
Footnotes
References
Allen, L. G., Yoklavich, M. M., Cailliet, G. M., and Horn, M. H. (2006). “Bays and estuaries,” in The Ecology of Marine Fishes: California and Adjacent Waters, eds L. G. Allen and M. H. Horn (Berkeley, CA: University of California Press), 119–148.
Bath, G. E., Thorrold, S. R., Jones, C. M., Campana, S. E., McLaren, J. W., and Lam, J. W. H. (2000). Strontium and barium uptake in aragonitic otoliths of marine fish. Geochim. Cosmochim. Acta 64, 1705–1714. doi: 10.1016/s0016-7037(99)00419-6
Behrens, D. K., Bombardellia, F. A., Largier, J. L., and Twohy, E. (2013). Episodic closure of the tidal inlet at the mouth of the Russian River — A small bar-built estuary in California. Geomorphology 189, 66–80. doi: 10.1016/j.geomorph.2013.01.017
Bell, K. N. I., Cowley, P. D., and Whitfield, A. K. (2001). Seasonality in frequency of marine access to an intermittently open estuary. Estuar. Coast. Shelf Sci. 52, 327–337. doi: 10.1006/ecss.2000.0709
Bond, M. H., Hayes, S. A., Hanson, C. V., and MacFarlane, R. B. (2008). Marine survival of steelhead (Oncorhynchus mykiss) enhanced by a seasonally closed estuary. Can. J. Fish. Aquat. Sci. 65, 2242–2252. doi: 10.1139/f08-131
Brown, L. R., Matern, S. A., and Moyle, P. B. (1995). Comparative ecology of prickly sculpin, Cottus asper, and coastrange sculpin, C. aleuticus, in the Eel River, California. Environ. Biol. Fish. 42, 329–343. doi: 10.1007/BF00001462
Campana, S. E. (1999). Chemistry and composition of fish otoliths: pathways, mechanisms and applications. Mar. Ecol. Prog. Ser. 188, 263–297. doi: 10.3354/meps188263
Chamberlain, C. D. (2006). Environmental Variables of Northern California Lagoons and Estuaries and the Distribution of Tidewater Goby (Eucyclogobius newberryi). Arcata Technical Report TR 2006–2004. Washington, DC: U.S. Fish and Wildlife Service.
Clark, R., and O’Connor, K. (2019). A systematic survey of bar-built estuaries along the California coast. Estuar. Coast. Shelf Sci. 226:106285. doi: 10.1016/j.ecss.2019.106285
Cousins, M., Stacey, M. T., and Drake, J. L. (2010). Effects of seasonal stratification on turbulent mixing in a hypereutrophic coastal lagoon. Limnol. Ocean 55, 172–186. doi: 10.4319/lo.2010.55.1.0172
Dennenmoser, S., Rogers, S. M., and Varnosi, S. M. (2014). Genetic population structure in prickly sculpin (Cottus asper) reflects isolation-by-environment between two life-history ecotypes. Biol. J. Linn. Soc. 113, 943–957. doi: 10.1111/bij.12384
Drake, J. L., Carpenter, E. J., Cousins, M., Nelson, K. L., Guido-Zarate, A., and Loftin, K. (2010). Effects of light and nutrients on seasonal phytoplankton succession in a temperate eutrophic coastal lagoon. Hydrobiologia 654, 177–192. doi: 10.1007/s10750-010-0380-y
Elsdon, T., and Gillanders, B. (2002). Interactive effects of temperature and salinity on otolith chemistry: challenges for determining environmental histories of fish. Can. J. Fish. Aquat. Sci. 59, 1796–1808. doi: 10.1139/f02-154
Elsdon, T., and Gillanders, B. (2004). Fish otolith chemistry influenced by exposure to multiple environmental variables. J. Exp. Mar. Biol. Ecol. 313, 269–284. doi: 10.1016/j.jembe.2004.08.010
Elsdon, T. S., Wells, B. K., Campana, S. E., Gillanders, B. M., Jones, C. M., Limburg, K. E., et al. (2008). Otolith chemistry to describe movements and life history parameters of fishes: hypotheses, assumptions, limitations and inferences. Ocean Mar. Biol. 46, 297–330. doi: 10.1201/9781420065756.ch7
Farrell, J., and Campana, S. E. (1996). Regulation of calcium and strontium deposition on the otoliths of juvenile tilapia. Oreochromis niloticus. Comp. Biochem. Physiol. 115, 103–109. doi: 10.1016/0300-9629(96)00015-1
Feyrer, F., Herbold, B., Matern, S. A., and Moyle, P. B. (2003). Dietary shifts in a stressed fish assemblage. Environ. Biol. Fish. 67, 277–288. doi: 10.1023/A:1025839132274
Fong, P., and Kennison, R. L. (2010). “Phase shifts, alternative stable states, and the status of southern California lagoons,” in Coastal Lagoons; Critical Habitats of Environmental Change, eds M. J. Kennish and H. W. Paerl (Boca Raton, FL: CRC Press), 227–251. doi: 10.1201/ebk1420088304-c10
Goto, A., and Arai, T. (2006). Diverse migratory histories of Japanese Trachidermus and Cottus species (Cottidae) as inferred from otolith microchemistry. J. Fish Biol. 68, 1731–1741. doi: 10.1111/j.1095-8649.2006.01040.x
Haines, P. E., Tomlinson, R. B., and Thom, B. G. (2006). Morphometric assessment of intermittently open/closed coastal lagoons in New South Wales. Australia. Estuar. Coast Shelf Sci. 67, 321–332. doi: 10.1016/j.ecss.2005.12.001
Hale, R., Morrongiello, J. R., and Swearer, S. E. (2016). Evolutionary traps and range shifts in a rapidly changing world. Biol. Lett. 12:20160003. doi: 10.1098/rsbl.2016.0003
Hayes, S. A., Bond, M. H., Hanson, C. V., Fruend, E. V., Smith, J. J., Anderson, E. C., et al. (2008). Steelhead growth in a Small central California watershed: upstream and estuarine rearing patterns. Trans. Amer. Fish. Soc. 137, 114–128. doi: 10.1577/t07-043.1
Heady, W. N., Clark, R. P., O’Connor, K., Clark, C., Endris, C., Ryan, S., et al. (2015). Assessing California’s bar-built estuaries using the California rapid assessment method. Ecol. Ind. 58, 300–310. doi: 10.1016/j.ecolind.2015.05.062
Hume, T., Gerbeaux, P., Hart, D., Kettles, H., and Neale, D. (2016). A Classification of New Zealand’s Coastal Hydrosystems. New Zealand Technical Report, Report No. HAM2016-062. Auckland: National Institute of Water & Atmospheric Research Ltd.
Kjerfve, B. (1994). “Coastal lagoons,” in Coastal Lagoon Processes, ed. B. Kjerfve (Amsterdam: Elsevier Oceanographic Series), 1–8. doi: 10.1016/s0422-9894(08)70006-0
Krejsa, R. J. (1967). The systematics of the prickly sculpin, cottus asper richardson, a polytypic species. Part I. Synonymy, nomenclatural history, and distribution. Pacific Sci. 21, 241–251. doi: 10.1093/jhered/esw045
Lafferty, K. D., Swift, C. C., and Ambrose, R. F. (1999a). Extirpation and recolonization in a metapopulation of an endangered fish, the tidewater goby. Conserv. Biol. 13, 1447–1453. doi: 10.1046/j.1523-1739.1999.98016.x
Lafferty, K. D., Swift, C. C., and Ambrose, R. F. (1999b). Postflood persistence and recolonization of endangered tidewater goby populations. North Am. J. Fish. Manag. 19, 618–622. doi: 10.1577/1548-8675(1999)019<0618:pparoe>2.0.co;2
Limburg, K. E., Huang, R., and Bilderback, D. H. (2007). Fish otolith trace element maps: new approaches with synchrotron microbeam x-ray fluorescence. X Ray Spectrometry 36, 336–342. doi: 10.1002/xrs.980
Limburg, K. E., Olson, C., Walther, Y., Dale, D., Slomp, C., and Høie, H. (2011). Tracking Baltic hypoxia and cod migration over millennia with natural tags. Proc. Natl. Acad. Sci. U.S.A. 108, E177–E182. doi: 10.1073/pnas.1100684108
Mcsweeney, S. L., Kennedy, D. M., and Rutherfurd, I. D. (2014). “Classification of intermittently closed and open coastal lakes and lagoons in Victoria, Australia,” in Proceedings of the 7th Australian Stream Management Conference, Townsville, QLD, 1–8.
Monaco, M. E., Emmett, R. L., Nelson, D. M., and Hinton, S. A. (1990). Distribution and Abundance of Fishes and Invertebrates in West Coast Estuaries Volume I: Data summaries. ELMR Rp. No. 4. Silver Spring, MD: NOAA/NOS Strategic Environmental Assessments Division, 322.
Mouillot, D., Gaillard, S., Aliaume, C., Verlaque, M., Belsher, T., Troussellier, M., et al. (2005). Ability of taxonomic diversity indices to discriminate coastal lagoon environments based on macrophyte communities. Ecol. Indicat. 5, 1–17. doi: 10.1016/j.ecolind.2004.04.004
Moyle, P. B. (2002). Inland fishes of California. Revised and expanded. Berkeley, CA: University of California Press.
Pérez-Ruzafa, A., Mompeán, M. C., and Marcos, C. (2019). Hydrographic, geomorphologic and fish assemblage relationships in coastal lagoons. Hydrobiologia 577, 107–125. doi: 10.1007/s10750-006-0421-428
Sardá-Espinosa, A. (2019a). Dtwclust: Time Series Clustering Along With Optimizations for the Dynamic Time Warping Distance. R Package Version 5.5.6. Avaliable online at: https://cran.r-project.org/web/packages/dtwclust/index.html
Sardá-Espinosa, A. (2019b). Time-series clustering in R using the dtwclust package. R J. 11, 22–43. doi: 10.32614/RJ-2019-023
Shao, Y., Farkas, J., Holmden, C., Mosley, L., Kell-Duivestein, I., Izzo, C., et al. (2018). Calcium and strontium isotope systematics in the lagoon-estuarine environments of South Australia: implications for water source mixing, carbonate fluxes and fish migration. Geochim. Cosmochim. Acta 239, 90–108. doi: 10.1016/j.gca.2018.07.036
Shapovalov, L., and Taft, A. C. (1954). The life histories of the steelhead rainbow trout (Salmo gairdneri gairdneri) and silver salmon (Oncorhynchus kisutch) with special reference to Waddell Creek, California, and recommendations regarding their management. Calif. Dep. Fish Game Fish Bull. 98:375.
Smakhtin, V. U. (2004). Simulating the hydrology and mouth conditions of small, temporarily closed/open estuaries. Wetlands 24, 123–132. doi: 10.1672/0277-5212(2004)024[0123:sthamc]2.0.co;2
Sole, V. A., Papillon, E., Cotte, M., Walter, P., and Susini, J. (2007). A multiplatform code for the analysis of 477 energy-dispersive X-ray fluorescence spectra. Spectroc. Acta Pt. B Atom. Spectr. 62, 63–68. doi: 10.1016/j.sab.2006.12.002
Spies, B. T., Tarango, B. C., and Steele, M. A. (2014). Larval Duration, Settlement, and Larval Growth Rates of the Endangered Tidewater Goby (Eucyclogobius newberryi) and the Arrow Goby (Clevelandia ios) (Pisces, Teleostei). Bull. Soc. Calif. Acad. Sci. 113, 165–175. doi: 10.3160/0038-3872-113.3.165
Steinke, D., and Feyrer, F. (2020). Otolith chemistry of fishes in Rodeo Lagoon, Marin County, California, 2016. Reston, VA: U.S. Geological Survey Data Release.
Sturrock, A. M., Hunter, E., Milton, J. A., Johnson, R. C., Waring, C. P., and Trueman, C. N. (2015). Quantifying physiological influences on otolith microchemistry. Methods Ecol. Evol. 6, 806–816. doi: 10.1111/2041-210X.12381
Swenson, R. O. (1999). The ecology, behavior, and conservation of the tidewater goby, Eucyclogobius newberryi. Environ. Biol. Fish. 55, 99–114. doi: 10.1023/A:1007478207892
Swenson, R. O., and McCray, A. T. (1996). Feeding ecology of the tidewater goby. Trans. Am. Fish. Soc. 125, 956–970. doi: 10.1577/1548-8659(1996)125<0956:feottg>2.3.co;2
Swift, C. C., Mulder, J., Dellith, C., and Kittleson, K. (2018). Mortality of native and non-native fishes during artificial breaching of coastal lagoons in southern and central California. Southern Calif. Acad. Sci. 117, 157–168. doi: 10.3160/1767.1
Swift, C. C., Nelson, J. L., Maslow, C., and Stein, T. (1989). Biology and distribution of the tidewater goby, Eucyclogobius newberryi (Pisces: Gobiidae) of California. Contrib. Sci. 404, 1–19.
U.S. Fish and Wildlife Service [USFWS] (2005). Recovery plan for the tidewater goby (Eucyclogobius newberryi). Portland, OR: Pacific Region, U.S. Fish and Wildlife Service, 199.
Walther, B. D., and Limburg, K. (2012). The use of otolith chemistry to characterize diadromous migrations. J. Fish Biol. 81, 796–825. doi: 10.1111/j.1095-8649.2012.03371.x
Walther, B. D., and Thorrold, S. R. (2009). Inter-annual variability in isotope and elemental ratios recorded in otoliths of an anadromous fish. J. Geochem. Explor. 102, 181–186. doi: 10.1016/j.gexplo.2008.10.001
Willmes, M., Lewis, L. S., Davis, B. E., Loiselle, L., James, H. F., Denney, C., et al. (2019). Calibrating temperature reconstructions from fish otolith oxygen isotope analysis for California’s critically endangered Delta Smelt. Rapid Commun. Mass Spectrom. 33, 1207–1220. doi: 10.1002/rcm.8464
Yáñez-Arancibia, A. (ed.) (1985). Fish Community Ecology in Estuaries, and Coastal Lagoons: Towards an Ecosystem Integration. Mexico: UNAM Press.
Keywords: otolith, strontium, synchrotron, Tidewater Goby (Eucyclogobius newberryi), Prickly Sculpin (Cottus asper), amphidromy, bar-built estuary, Rodeo Lagoon
Citation: Feyrer F, Young M, Fong D, Limburg K and Johnson R (2020) Cryptic Lives of Conspicuous Animals: Otolith Chemistry Chronicles Life Histories of Coastal Lagoon Fishes. Front. Mar. Sci. 7:417. doi: 10.3389/fmars.2020.00417
Received: 24 January 2020; Accepted: 12 May 2020;
Published: 26 June 2020.
Edited by:
Alejandra Vanina Volpedo, University of Buenos Aires, ArgentinaReviewed by:
Stephen E. Swearer, University of Melbourne, AustraliaJed Ian Macdonald, Pacific Community (SPC), New Caledonia
Copyright © 2020 Feyrer, Young, Fong, Limburg and Johnson. This is an open-access article distributed under the terms of the Creative Commons Attribution License (CC BY). The use, distribution or reproduction in other forums is permitted, provided the original author(s) and the copyright owner(s) are credited and that the original publication in this journal is cited, in accordance with accepted academic practice. No use, distribution or reproduction is permitted which does not comply with these terms.
*Correspondence: Frederick Feyrer, ZmZleXJlckB1c2dzLmdvdg==