- 1Department of Biology, University of Richmond, Richmond, VA, United States
- 2Centro de Estudios Avanzados de Blanes (CEAB-CSIC), Blanes, Spain
- 3Department of Evolutionary Biology, Ecology and Environmental Sciences, Faculty of Biology, Institute of Biodiversity Research (IRBio), University of Barcelona, Barcelona, Spain
The microbiota of four Antarctic sponges, Dendrilla antarctica, Sphaerotylus antarcticus, Mycale acerata, and Hemigellius pilosus, collected at two South Shetland Islands and at two locations in the Antarctic Peninsula separated by ca. 670 km, were analyzed together with surrounding seawater. We used high throughput sequencing of the V4 region of the 16S rRNA gene common to Bacteria and Archaea to investigate the prokaryotic diversity and community composition. Our study reveals that sponge-associated prokaryote communities are consistently detected within a particular sponge species regardless of the collection site. Their community structure and composition are typical of low microbial abundance (LMA) sponges. We conclude that prokaryote communities from Antarctic sponges are less diverse and differ in their composition compared to those in the water column. Microbiome analysis indicates that Antarctic sponges harbor a strict core consisting of seven OTUs, and a small variable community comprising several tens of OTUs. Two abundant prokaryotes from the variable microbiota that are affiliated to the archaeal and bacterial phyla Thaumarchaeota and Nitrospirae may be involved in the sponge nitrification process and might be relevant components of the nitrogen cycling in Antarctica. The likely generalist nature of dominant microbes and the host-specific structure of symbiont communities suggest that these Antarctic sponges represent different ecological niches for particular prokaryotic enrichments.
Introduction
Sponges (phylum Porifera) are sessile organisms widely distributed from the tropics to the poles (Hooper and Van Soest, 2004), and ecologically important constituents of benthic environments from shallow to deep waters (Bell, 2008). Sponges form symbioses with diverse and metabolically active microorganisms that make valuable contributions to many aspects of the sponge’s physiology and ecology (Taylor et al., 2007). For that reason, the concept “sponge holobiont” was introduced to refer to the sponge host and the consortium of bacteria, archaea, algae, fungi, and viruses that reside within it (Webster and Taylor, 2012). Marine sponge-associated prokaryotic communities have been widely studied, being reported to be highly complex (Thomas et al., 2016). Sponges host (even at low relative abundances) over 60 bacterial and four archaeal phyla (Reveillaud et al., 2014; Thomas et al., 2016; Moitinho-Silva et al., 2017a). Despite the continuous flux of seawater through their canal system, sponges are able to maintain a specific microbial composition remarkably different from the ambient seawater (Thomas et al., 2016; Hill and Sacristán-Soriano, 2017). Furthermore, these associations appear to be host-specific and stable under different environmental conditions (Hentschel et al., 2002; Lee et al., 2011; Erwin et al., 2012b; Schmitt et al., 2012; Pita et al., 2013; Reveillaud et al., 2014). Although sponge-microbial interactions seem to be consistent over geographic regions, there are some apparent geographical gaps in the study of host-associated prokaryotic assemblages.
Antarctic marine habitats are characterized by their uniqueness and almost intact virginity that experience extreme environmental conditions and a marked seasonality. The geographical isolation and the cyclical sea-ice formation make these ecosystems largely unexplored. Sponges are also important components of marine benthic communities in Antarctica and play key roles in community structure and nutrition cycling, also providing microhabitats for other invertebrates (McClintock et al., 2005; Angulo-Preckler et al., 2018). Few studies have examined the microbial diversity present in Antarctic marine sponges (Lo Giudice et al., 2019). There have been several approximations to unravel the composition of Antarctic microbial communities associated to sponges, but primarily focused on eukaryotic microorganisms (Bavestrello et al., 2000; Cerrano et al., 2000, 2004; Henríquez et al., 2014). However, the most comprehensive studies describing prokaryotic communities associated to Antarctic sponges using classic and high throughput sequencing described a total prokaryotic composition of 26 bacterial and three archaeal phyla and the presence of eight eukaryotic groups (Webster et al., 2004; Rodríguez-Marconi et al., 2015; Cárdenas et al., 2018, 2019; Steinert et al., 2019). Recently, functional metagenomics has been used to characterize the community composition and metabolic potential of microbiomes of two Antarctic sponges (Moreno-Pino et al., 2020). In the Bacteria domain those assemblages primarily clustered within the Gamma and Alphaproteobacteria followed by the Bacteroidetes phylum. In the Archaea domain, Crenarchaeota, and Thaumarchaeota representatives were mostly associated to Antarctic sponges. Within the Eukarya domain, fungi were predominately in association with sponges in Antarctica followed by diatoms and dinoflagellates (Lo Giudice et al., 2019).
Since the rapid development in massive sequencing methodologies, allowing for a more complete characterization of the sponge microbiomes, the specificity of the associated microorganisms is under debate. Several bacterial taxa have been reported as sponge-specific bacteria (i.e., bacterial lineages found only in sponges and not in ambient seawater or sediments) (Taylor et al., 2007). However, other studies have showed that several bacterial taxa thought to be specific to sponges also occur in other habitats, such as seawater, sediment, and other hosts (Simister et al., 2012). Therefore, it would be preferable to refer to the associated microbial partners with the term “sponge-enriched” or “host-enriched” (Moitinho-Silva et al., 2014).
In the present study, we assess and compare prokaryote communities from four sponge species and the surrounding seawater collected in two South Shetland Islands and at two locations in the Antarctic Peninsula separated by ca. 670 km. We sought to answer the following questions: (1) How is the diversity and prokaryotic community composition associated to Antarctic marine sponges, compared to the surrounding seawater? (2) Is there a core-microbiome associated to them? (3) Are these communities host-specific and consistent over a geographic scale? This is one of the first reports that used high throughput sequencing to unravel the composition and diversity of symbiotic prokaryotic communities associated to Antarctic marine sponges.
Materials and Methods
Sample Collection
During the austral summer 2016, four sponge species [Dendrilla antarctica (Topsent, 1905), Sphaerotylus antarcticus (Kirkpatrick, 1907), Mycale (Oxymycale) acerata (Kirkpatrick, 1907), and Hemigellius pilosus (Kirkpatrick, 1907)] were collected at different locations from two South Shetland Islands and the Antarctic Peninsula (Table 1). Replicate seawater samples (n = 3, 2 l samples) were aseptically collected adjacent to the sampled sponges from all locations. Sponges were processed after collection. A sample from each specimen was taken with a sterile scalpel and rinsed several times in 0.22 μm-filtered seawater to discard loose attached microorganisms. Seawater samples were sequentially passed through polycarbonate 5 and 0.22 μm filters (MilliporeSigma, Burlington, MA, United States) to avoid clogging the ones with the smaller pore diameter. The contents on the 0.22 μm filters were used to examine the ambient bacterioplankton communities. All samples were preserved in RNAlater until further use.
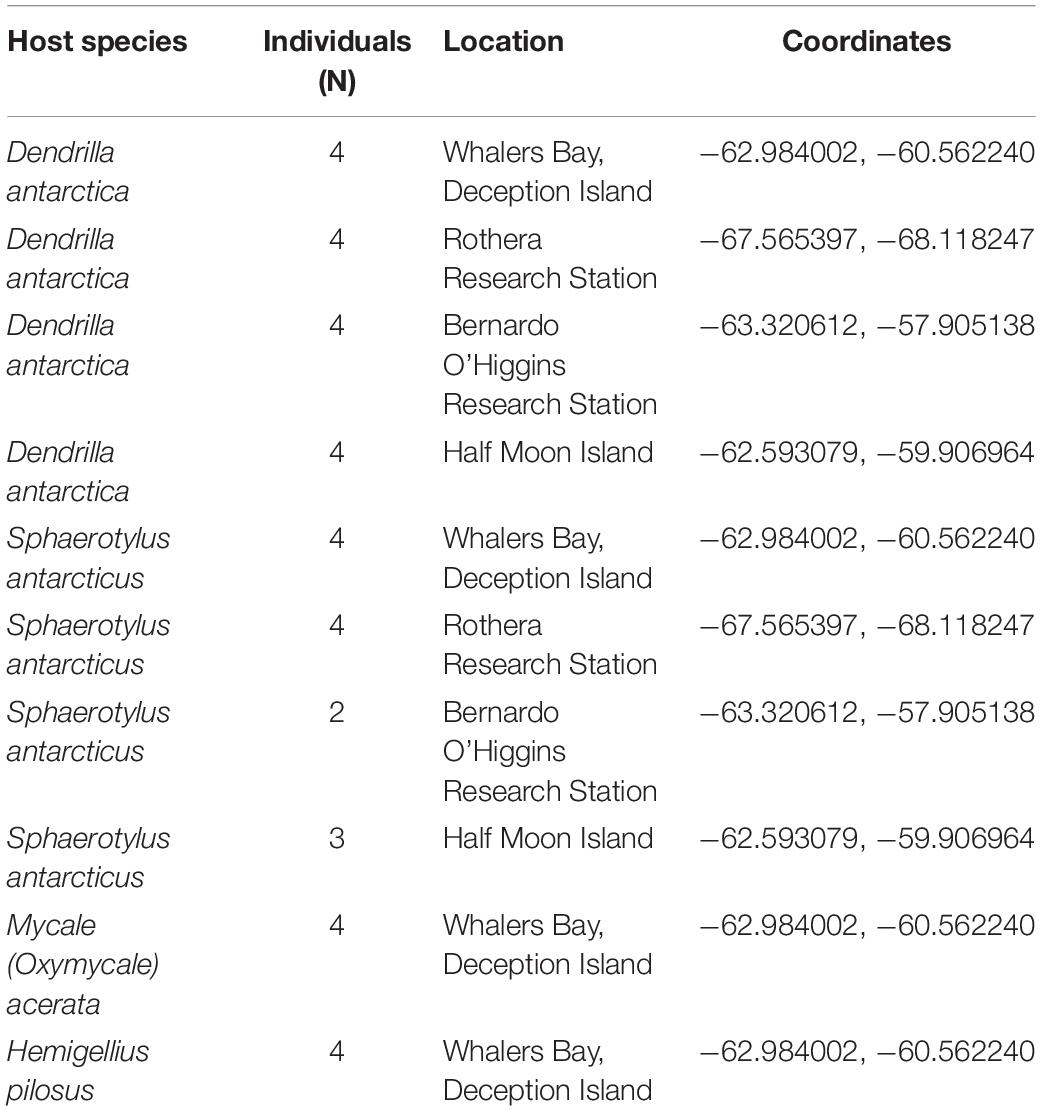
Table 1. Samples of healthy specimens of Dendrilla antarctica (Topsent, 1905), Sphaerotylus antarcticus (Kirkpatrick, 1907), Mycale (Oxymycale) acerata (Kirkpatrick, 1907), and Hemigellius pilosus (Kirkpatrick, 1907) collected at 15 to 20 m depth from two South Shetland Islands (Deception and Half Moon Islands) and the Antarctic Peninsula (Rothera and O’Higgins Research Stations).
Microbiome Analysis
DNA extracts were submitted to Molecular Research LP1 (Shallowater, TX, United States) for amplification, library construction and multiplexed sequencing of partial (V4) 16S rRNA gene sequences on an Illumina MiSeq platform. The HotStarTaq Plus Master Mix kit (Qiagen) was used for PCR amplifications using DNA extracts as templates with universal prokaryotic 515F (Parada et al., 2016) and 806R (Apprill et al., 2015). To barcode samples, a multiplex identifier barcode was attached to the forward primer. The thermocycler profile consisted of an initial denaturation step at 94°C for 3 min; 28 cycles of 94°C for 30 s, 53°C for 40 s, and 72°C for 1 min with a final elongation step at 72°C for 5 min. Equimolar concentrations of samples (Table 1) were pooled and purified using Agencourt AMPure XP beads (Beckman Coulter) to prepare DNA library by following Illumina TruSeq DNA library preparation protocol. Sequencing was then performed according to manufacturer’s guidelines on an Illumina MiSeq.
As described previously (Thomas et al., 2016), Illumina sequence reads were processed in mothur v1.39.5 (Schloss et al., 2009). Briefly, raw reads were demultiplexed, forward and reverse reads were then joined, and sequences <200 bp and/or with ambiguous base calls were removed. Sequences were aligned to the SILVA database (release 128, non-redundant, mothur-formatted), trimmed to the V4 region, and screened for chimeras and errors. A naïve Bayesian classifier and Greengenes taxonomy (August 2013 release, mothur-formatted) was used to aid in the removal of non-target sequences (e.g., chloroplasts, mitochondria). We used the SILVA database (release 132, non-redundant, mothur-formatted) for final taxonomic assignment. The resulting high-quality sequences were clustered into operational taxonomic units (OTUs) defined by clustering at 3% divergence and singletons were removed. We used rarefaction curves (mothur v1.39.5) to plot the OTUs observed as a function of sequencing depth. To avoid artifacts of varied sampling depth on subsequent diversity calculations, each sequence dataset was subsampled to the lowest read count (mothur v1.39.5). To place the determined OTUs into a greater context, we performed BLAST searches (NCBI-BLAST-2.7.1+) of these sequences against the database of the sponge EMP project (Moitinho-Silva et al., 2017a).
Community-Level Analysis
To compare prokaryotic community profiles, we constructed a Venn diagram2 from presence/absence OTU data. Non-metric multidimensional scaling (nMDS) plots of Bray-Curtis similarity matrices were also constructed with mothur (v1.39.5) and R (version 3.4.3; ggplot2 package) from square-root transformed OTU relative abundance data. We also constructed bubble charts in R (version 3.4.3; ggplot2 package) from OTU relative abundances to plot community dissimilarities among locations. Significant differences among sponge species and ambient seawater were assessed using a one-way permutational multivariate analysis of variance (PERMANOVA), with the factor source (all sponge species vs. seawater). Significant differences among sponge species were assessed with a one-way PERMANOVA, with the factor source (D. antarctica, S. antarcticus, M. acerata, and H. pilosus). Differences between sponge species and locations were assessed using a two-way PERMANOVA, with the factors source (D. antarctica and S. antarcticus), location (Deception Island, Rothera, Half Moon Island, O’Higgins), and an interaction term. Pairwise comparisons were subsequently conducted for all significant PERMANOVA results. Permutational multivariate analysis of dispersion (PERMDISP) was used to detect differences in homogeneity (dispersion) among groups for all significant PERMANOVA outcomes. All multivariate statistics were performed in R (version 3.4.3; with adonis2 and betadisper functions from vegan v2.5-6 package).
We calculated three indices of alpha diversity in mothur v1.39.5 (Schloss et al., 2009) to evaluate community richness, diversity, and evenness: observed OTU richness, the inverse of Simpson index of diversity, and the Simpson index of evenness. One-way analyses of variance (ANOVA) was used to detect differences in diversity metrics among the species from Deception Island (D. antarctica, S. antarcticus, M. acerata, and H. pilosus). Two-way analysis of variance (ANOVA) was used to detect differences in diversity metrics by the factors source (D. antarctica and S. antarcticus), location (sampling sites) and an interaction term, followed by pairwise comparisons for any significant factor. All data that did not meet the statistical assumptions was transformed accordingly (log-transformation for inverse of Simpson index). The univariate statistics were performed in R (version 3.4.3; ANOVA function from car package).
OTU-Level Analysis
We were interested in OTUs that were abundant (i.e., >0.1% relative abundance) and widespread among sponge host individuals (i.e., 90% prevalence), so we performed a core microbiome (i.e., with relative abundances >0.1% and present in all species) analysis in R (version 3.5.3, package Microbiome). We also analyzed the dataset for patterns in relative abundances of OTUs within categories (e.g., sponge vs. seawater, among locations). For this purpose, we removed from the dataset rare OTUs (<0.1% relative abundance) and OTUs with a low incidence across samples (detected in <2 samples). We used the Mann-Whitney-U test (or Wilcoxon rank sum test) with FDR p-value correction to identify significantly different patterns in OTU relative abundance among hosts and locations using QIIME 1 (Caporaso et al., 2010).
Results
Microbiome Composition Associated to Antarctic Sponges
The V4 region of the 16S rRNA gene was sequenced on an Illumina MiSeq platform and a total of 4,398,237 reads were obtained after denoising and quality filtering with a library depth ranging from 39,883 to 154,480 reads. As we had four replicates per species and location in most of the cases, we discarded those samples (n = 5) with the lowest number of reads (≤51,407) to have at least three replicates per sponge and site. The only exception was S. antarcticus from O’Higgins Station, from which we had two replicates. This may represent low replication, however, those samples clustered together with most samples of the same species and we reached the same results without the O’Higgins location. Therefore, we decided to include them in further analyses. To avoid artifacts of varied sampling depth, we rarefied our libraries to the lowest read count after removing the previous samples from the dataset (n = 52,637; Supplementary Figure S1). Twenty-eight bacterial and three archaeal phyla were detected in the 11,187 OTUs recovered from seawater and sponge samples, which were predominantly affiliated to the phyla Proteobacteria and Bacteroidetes (Supplementary Figure S2). Of these, 4,619 OTUs were recovered from D. antarctica, 3,438 OTUs from S. antarcticus, 1,381 OTUs from M. acerata, and 1,490 OTUs from H. pilosus. Seawater exhibited greater richness with 6,071 OTUs, 2,511 of which were shared with either D. antarctica or S. antarcticus or with both species, and 1,240 were shared with M. acerata and/or H. pilosus (Supplementary Figure S3). Seawater from Rothera, and especially from Deception Island contained the lowest amounts of Gammaproteobacteria (Figure 1).
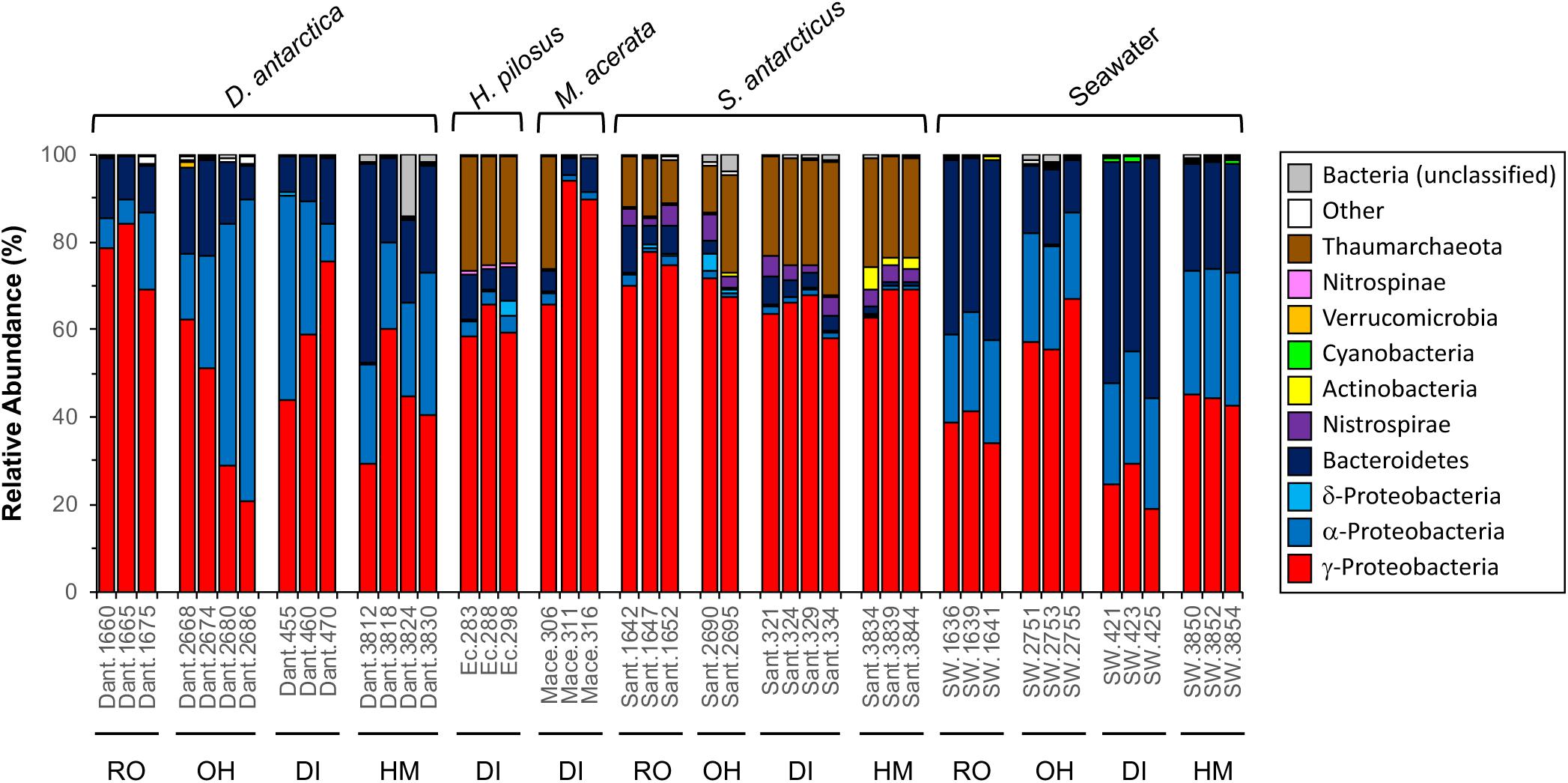
Figure 1. Taxonomic composition of prokaryotic communities in Dendrilla antarctica, Sphaerotylus antarcticus, Mycale acerata, Hemigellius pilosus and surrounding seawater from O’Higgins (OH), Half Moon Island (HM), Deception Island (DI), and Rothera (RO).
The taxonomic composition of prokaryotic communities recovered from ambient seawater, and from S. antarcticus, M. acerata and H. pilosus sponge hosts, were significantly different, while D. antarctica presented a microbial content quite similar to what we found in seawater (Figure 1). Two more phyla were detected in sponges compared to the surrounding seawater (Supplementary Figures S4, S5). However, if we discard phyla with low sequence abundances (i.e., <0.1% abundance), sponges and seawater harbored five bacterial and one archaeal phyla. The prokaryotic communities harbored by S. antarcticus, M. acerata, and H. pilosus were enriched for Gammaproteobacteria (>60, >80, >65% of the prokaryotic community on average, respectively) and Thaumarchaeota (>20% in S. antarcticus and H. pilosus, >8% in M. acerata) compared to seawater (<42 and <0.5%, respectively). Comparatively, D. antarctica hosted less Gammaproteobacteria (<54%) and its associated Thaumarchaeota were almost absent (<0.5%). Contrastingly, prokaryotic communities in the first three sponges were depleted in members of Alphaproteobacteria (<5% in all three species) and Bacteroidetes (<8% in all three species) compared to seawater (>24 and >30%, respectively) and D. antarctica (>26 and >17%, respectively). The inter-individual variability of the taxonomic composition depends on the host. While H. pilosus and S. antarcticus harbored a quite stable microbial signature, D. antarctica exhibited greater inter-individual variability and one of the specimens of M. acerata showed an enrichment for Thaumarchaeota (Figure 1).
Differences Within and Between Sponge-Associated and Seawater Prokaryotic Communities
Statistically significant differences in community structure (PERMANOVA) were detected among S. antarcticus, M. acerata, H. pilosus and D. antarctica and seawater microbes (F4,39 = 10.563; P = 0.001). The four species significantly differed from each other (Pairwise comparisons; P < 0.004) except for M. acerata and H. pilosus (P = 0.100). Symbiont communities from seawater sources exhibited no overlap with sponge species in the multidimensional space, and all sponge species occupied distinct regions of the nMDS plot (Figure 2). In addition, a significant interaction between host species (S. antarcticus and D. antarctica) and location occurred (PERMANOVA, F3,18 = 2.422; P = 0.010), though we could not detect significant pairwise differences in community structure after p-value correction. Dispersion analysis revealed equal variability within S. antarcticus and D. antarctica prokaryotic communities regardless of location (P > 0.05 in all comparisons), but microbiomes of the latter species from O’Higgins were more variable (P = 0.046; Figure 2).
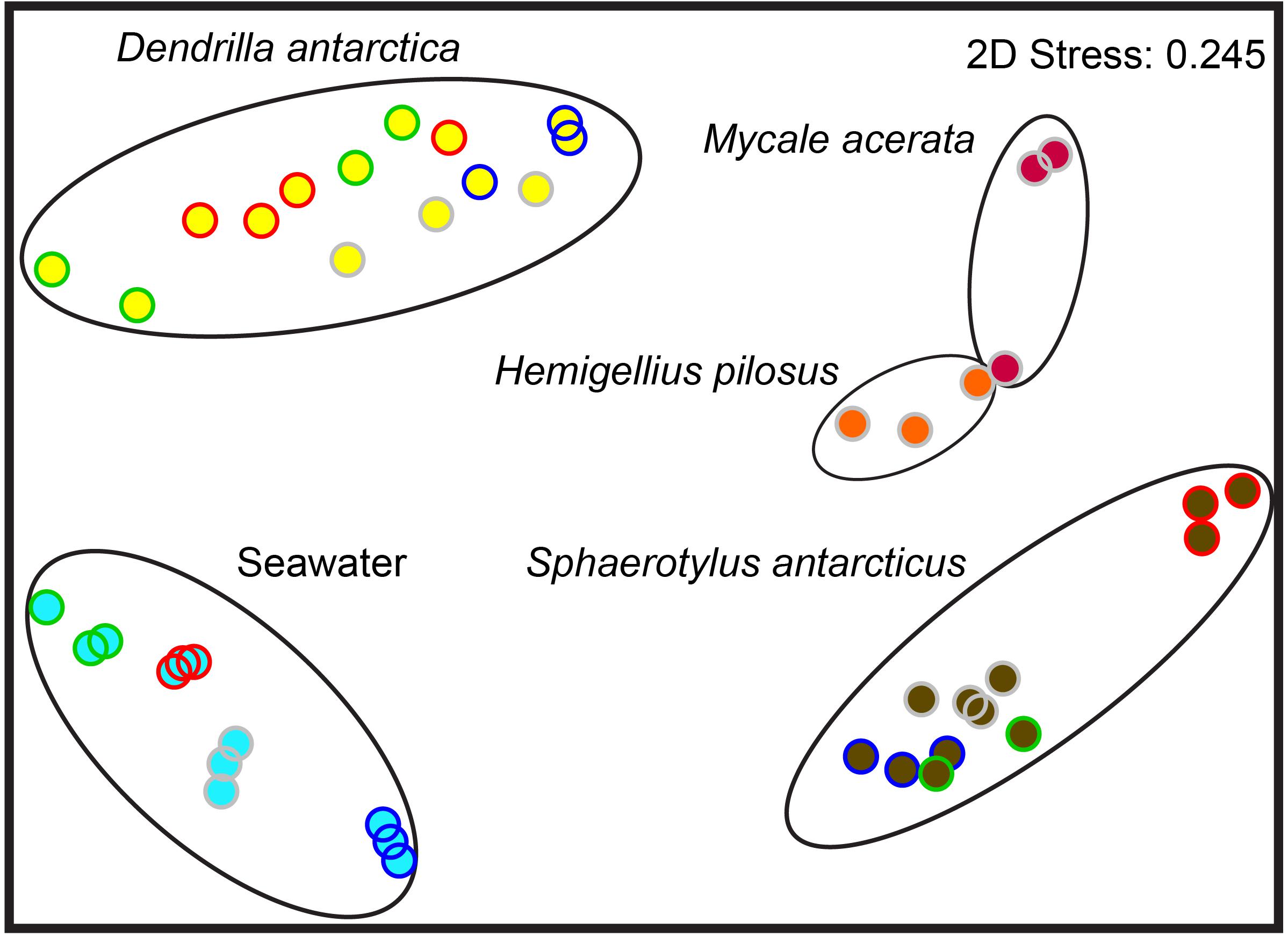
Figure 2. Non-metric multi-dimensional scaling plot of microbial community structure from replicate individuals of Dendrilla antarctica (yellow), Sphaerotylus antarcticus (brown), Mycale acerata (red), Hemigellius pilosus (orange) and surrounding seawater (light blue) from O’Higgins (green circles), Half Moon Island (red circles), Deception Island (gray circles) and Rothera (blue circles). Stress value for two-dimensional ordination is shown.
Larger mean values of richness, diversity (i.e., inverse Simpson diversity index), and evenness in symbiont communities from seawater compared to host species were observed (P < 0.001 in all pairwise comparisons; Table 2). When we analyzed the sponges from Deception Island, the microbiome of all the species seemed to be equally richer and diverse with similar evenness except for the comparison between H. pilosus and M. acerata, where the former species presented more OTU richness (P = 0.048). Comparing D. antarctica and S. antarcticus from the four locations studied, a two-way ANOVA detected significant differences between hosts and locations for species richness, diversity and evenness (P < 0.03 in all cases). D. antarctica harbored a richer microbiome but less diverse than S. antarcticus. Additionally, the sponge prokaryotic communities from Half-moon Island had greater species richness and diversity compared to those from Rothera (pairwise comparisons P < 0.05). Although there was an effect of location on the microbiome evenness, differences among pairs were not detected.
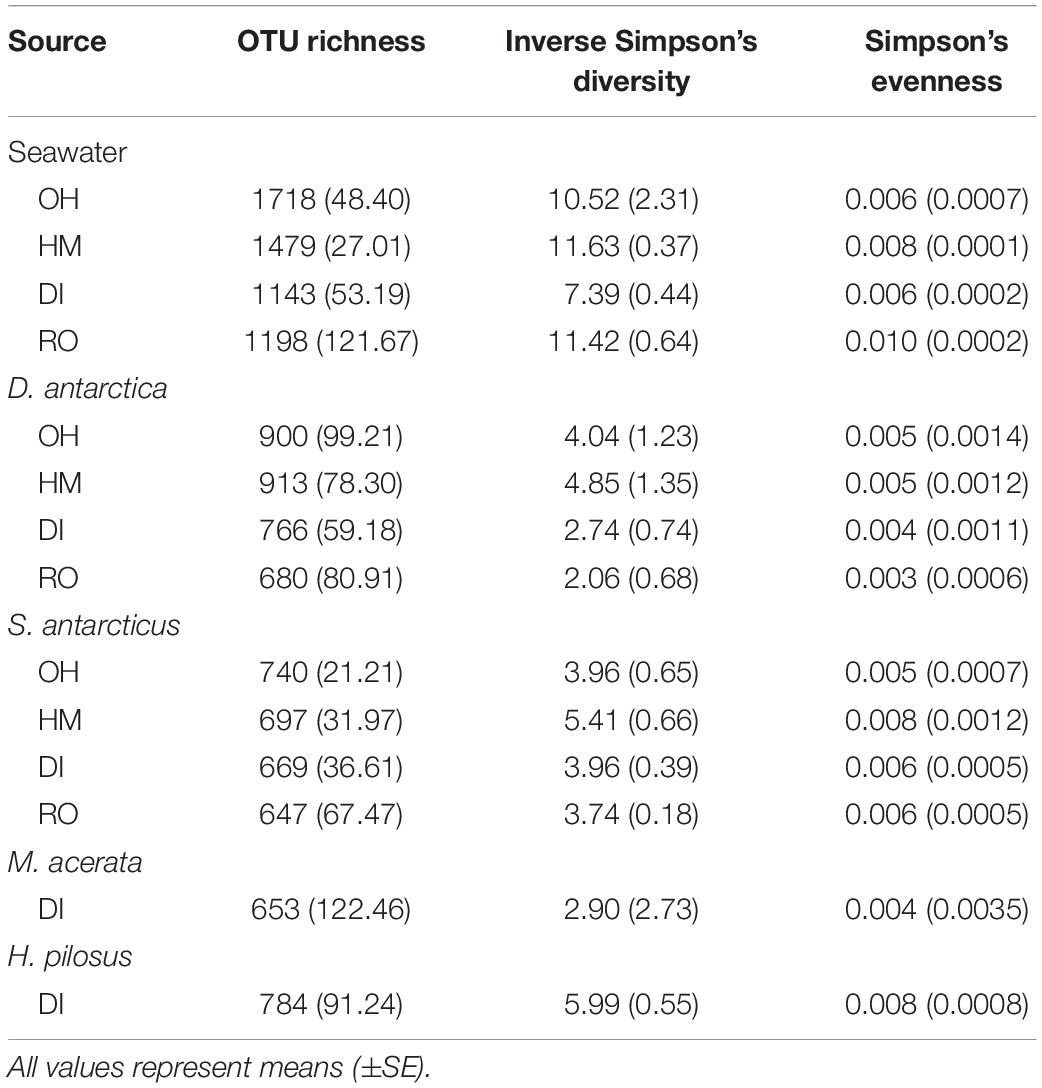
Table 2. Diversity estimators for microbial communities associated with seawater, Dendrilla antarctica, Sphaerotylus antarcticus, Mycale acerata and Hemigellius pilosus from O’Higgins (OH), Half Moon Island (HM), Deception Island (DI) and Rothera (RO).
The abundance of shared OTUs (n = 2,893) between sponge-associated and seawater prokaryotic communities was calculated and just 2.2% presented relative abundances over 0.1%. Those few OTUs (n = 65) accounted for 93 and 89% of the total relative abundance of sponge-associated and seawater prokaryotic communities, respectively, which meant that sponge-specific OTUs (64%) fell within the “rare biosphere” (<0.1% relative abundance).
Core and Variable Microbiome in Antarctic Sponges
In addition to community-level metrics of diversity and structure, we performed a core microbiome analysis to investigate patterns in abundant and prevalent individual OTUs among sponge hosts. The strict core microbiome of the sponge hosts was formed by seven OTUs accounting for 50% of total relative abundance on average (0.1% of total OTUs present in sponge hosts, 4.2% of OTUs with relative abundance >0.1% in at least one sample; Supplementary Table S1). Significant sponge enrichments in four core OTUs, affiliated to Gammaproteobacteria, were detected with a mean fold-change in abundance of 44.9 ± 8.0 (±SE) with respect to seawater (mean relative abundance 0.28%). Three additional sponge core OTUs, which were affiliated to the groups Alphaproteobacteria, Bacteroidetes, and Gammaproteobacteria, were more abundant in seawater communities (fold-change 14.3 ± 4.5; Supplementary Table S2). We also determined the variable community (i.e., with relative abundances >0.1% and present in at least two species) formed by 56 OTUs that represented on average 39% of the total abundance (0.7% of total OTUs present in sponges, 33.3% of OTUs with a relative abundance >0.1% in at least one sample; Supplementary Table S1). Forty-three sponge variable OTUs that had a mean fold-change in abundance of 49.5 ± 8.2 were extremely rare in seawater (mean relative abundance <0.03%). Thirteen additional sponge variable OTUs were enriched in seawater with mean relative abundance >2.7% (fold-change 13.7 ± 2.6). We comparatively determined the core microbiome of D. antarctica and S. antarcticus. Both species harbored ten major OTUs (six out of seven sponge core OTUs and four variable OTUs) representing over 70% of the microbiome relative abundance (Supplementary Table S1). Seawater presented instead a core microbiome of 18 OTUs (including six sponge core OTUs) accounting for over 60% of the microbiome in relative abundance (Supplementary Table S1). Comparing the sponge species analyzed, four core and 17 variable OTUs seemed to be sponge-enriched for either D. antarctica (OTUs 1, 4, 9, 11, 13, 27, 33, and 45; cumulative 84% relative abundance; Figure 3), S. antarcticus (OTUs 2, 7, 23, 31, 43, 51, and 56; 54% relative abundance; Figure 3), M. acerata (OTU 6; 65% relative abundance) or H. pilosus (OTUs 3, 14, 19, 34, and 53; 71% relative abundance), although they were also detected in the other hosts and in seawater in lower frequencies (Supplementary Table S2). If we compared locations, neither D. antarctica nor S. antarcticus presented differences in their microbiome abundances among sampling sites (Figure 3 and Supplementary Table S2). However, OTU 2 decreased its relative abundance to 0.3% in S. antarcticus at Half Moon Island, whereas the proportion was over 40% on average for the rest of locations (Figure 3).
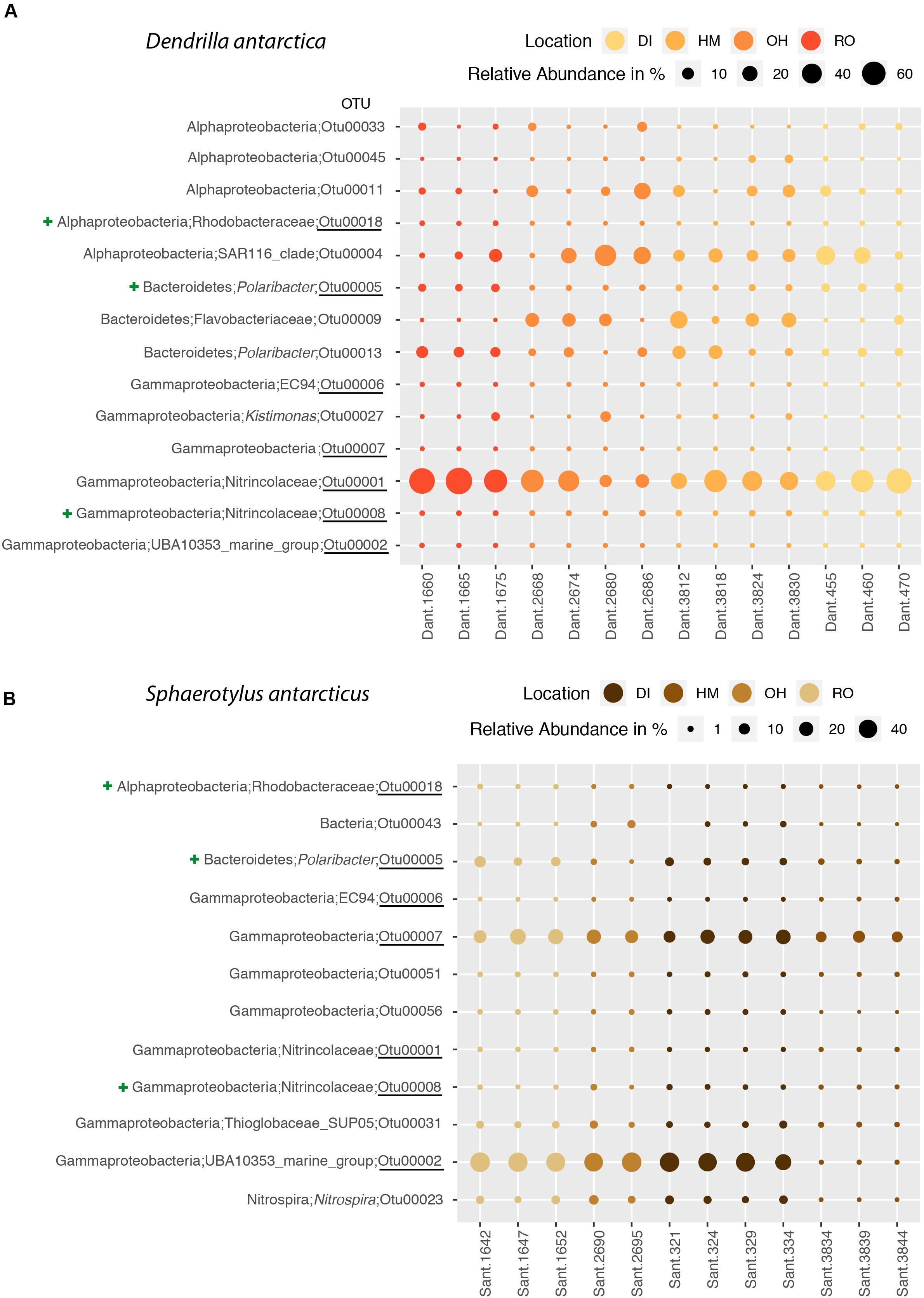
Figure 3. Bubble chart of core (underlined) and enriched variable OTUs of Dendrilla antarctica (A) and Sphaerotylus antarcticus (B) among locations defined at >0.1% relative abundance. OTU relative abundances are represented by the size of the bubbles (key on the top of each chart; notice the different scale between A and B). The smallest taxonomical level for each OTU is also shown. Location key: O’Higgins (OH), Half Moon Island (HM), Deception Island (DI), and Rothera (RO). We also show with a green cross those OTUs enriched in seawater samples.
From the 8,009 OTUs present in the sponges analyzed, 56.1% could be described as host-specific (i.e., present only in one sponge species), ranging from 254 OTUs in M. acerata to 2,230 OTUs in D. antarctica. However, all of these OTUs belonged to the rare biosphere associated to the sponge host with mean relative abundance below 0.1%. Although OTUs from core and variable prokaryotic communities were detected in all the sponge species analyzed, and even in ambient seawater, the specificity for one of the hosts of some variable OTUs (38%) could be considered due to the low number of reads recovered from the other habitats (<0.02% relative abundance).
Comparing Antarctic Sponge Associated Prokaryotic Communities With the Sponge EMP Database
Local BLAST searches against the sponge EMP database showed that 87% of the OTUs (n = 9,782) were found among the sponge microbiome collection at high sequence similarities (Supplementary File S1). Both core and variable communities associated to Antarctic sponges had a closest relative in the sponge microbiome database with sequence identities over 97%. Only three variable OTUs had similarities between 93 and 96%. The core microbiome associated to the Antarctic sponges is also associated to other sponge hosts and habitats (Supplementary File S1).
Discussion
This work describes the bacterial and archaeal diversity and the community composition of four Antarctic sponge species, revealing that the sponges had a prokaryotic signature different from the richer and diverse seawater community. In Antarctica, due to the difficult access to this region, only few studies have made comprehensive descriptions of microbial symbionts of marine sponges (Webster et al., 2004; Rodríguez-Marconi et al., 2015; Cárdenas et al., 2018, 2019; Steinert et al., 2019; Moreno-Pino et al., 2020). However, this is the first study that assesses the prokaryotic communities associated to Antarctic sponges using high throughput sequencing, considering biological replications and covering a spatial scale over 650 km.
Diversity and Taxonomic Composition of Prokaryotic Communities Associated to Antarctic Sponges
Although there is a lot of diversity to be uncovered, we have captured all abundant microbes present in Antarctic sponges and seawater (Supplementary Figure S1). The sponge associated prokaryotic communities were less diverse than surrounding seawater as previously reported for other Antarctic sponge hosts, reinforcing the view that these sponges were composed of low microbial abundance (LMA) microbiomes (Moitinho-Silva et al., 2017b). Both biotopes presented Thaumarchaeota as the predominant archaeal phylum, which accumulated 10.8% of the reads in sponges while just 0.1% in seawater. With regard to bacteria, differences lay in the fact that sponges hosted bacteria of Nitrospirae and Nitrospinae phyla, while seawater had instead representatives of Cyanobacteria and Verrucomicrobia. However, all these phyla accumulated microbial abundances ranging from 0.1 to 1.4%.
Classes Gamma- and Alpha-proteobacteria dominate bacterial assemblages in association with marine sponges from different biotopes (Thomas et al., 2016; Moitinho-Silva et al., 2017a; Pita et al., 2018; Cleary et al., 2019). These classes also dominate Antarctic sponges as previously reported on Antarctic marine shallow (Webster et al., 2004; Rodríguez-Marconi et al., 2015; Cárdenas et al., 2018, 2019) and deep waters (Steinert et al., 2019) with Bacteroidetes also as important members in terms of number of OTUs recovered (n = 1,174) and in relative abundance (10%). The presence of the phylum Nitrospirae (>3% in relative abundance) in one of the sponges analyzed (S. antarcticus) is particularly noticeable. Members of this phylum are potentially involved in nitrification processes, specifically in nitrite oxidation (Radax et al., 2012). A previous study provided a preliminary description of the bacterial communities present in Deception Island waters, which were very different than the usual Antarctic microbial diversity (Angulo-Preckler et al., 2015). We found that Bacteroidetes dominated the seawater prokaryotic community, probably influenced by the special conditions of this island, an active volcano with high concentrations of geothermal elements. This suggested a correlation between the environmental microbial diversity and the geochemical composition of the island. However, the sponges studied from the same area contained a different prokaryotic signature than the environment.
The phylum Chloroflexi, and other bacterial phyla such as Actinobacteria or Acidobacteria, have been frequently found in high microbial abundance (HMA) sponges (Schmitt et al., 2011; Moitinho-Silva et al., 2017b), occasionally reaching high percentages in relative abundances (5–10%). Contrastingly, these phyla represent a much lower percentage in Antarctic sponges (0.0005–0.4%). Our results are in agreement with previous studies carried out in Antarctica (Rodríguez-Marconi et al., 2015; Cárdenas et al., 2018; Steinert et al., 2019; Moreno-Pino et al., 2020). Other widely described but less abundant phyla associated with sponges from other geographical areas are Poribacteria and PAUC34f (Moitinho-Silva et al., 2017b). These bacterial groups were not detected neither in the Antarctic sponges from the present study nor in other species previously studied in Antarctica (Rodríguez-Marconi et al., 2015; Cárdenas et al., 2018, 2019; Steinert et al., 2019; Moreno-Pino et al., 2020). These phyla have been found overrepresented in HMA over LMA sponges and can be used as “HMA/LMA indicators” (Moitinho-Silva et al., 2017b; Glasl et al., 2018). This suggests that the microbial community structure of the sponges from the present study resemble that from LMA sponges.
Besides Bacteria, the presence of Archaea associated to marine sponges from tropical to cold waters has been previously documented (Radax et al., 2012; Cardoso et al., 2013; Jackson et al., 2013; Kennedy et al., 2014; Polónia et al., 2014; Turon and Uriz, 2020). These archaeal communities were dominated by the phylum Thaumarchaeota, which can reach high relative abundances in the sponge prokaryotic community. Three of the species analyzed in this study (S. antarcticus, M. acerata, H. pilosus) harbored abundant populations of an OTU (∼10–25%) affiliated to Candidatus Nitrosopumilus. In Antarctica, the presence of Thaumarchaeota-related sequences has been reported in multiple species (Steinert et al., 2019; Moreno-Pino et al., 2020), including M. acerata (Webster et al., 2004; Rodríguez-Marconi et al., 2015). These taxa act as ammonia oxidizers not only in tropical reefs but also in cold environments (Radax et al., 2012; Cardoso et al., 2013; Polónia et al., 2015). Indeed, the genomic potential for ammonia oxidation was recently evidenced for Leucetta antarctica microbiome from Antarctica (Moreno-Pino et al., 2020). Together with the potential nitrite oxidizers detected (members of Nitrospirae), they may be considered as relevant factors in nitrogen cycling in Antarctica. However, further studies are needed to understand the functional roles these microorganisms play in Antarctic symbiosis and their contribution to the ecosystem.
Core, Variable and Species-Specific Microbiome in Antarctic Sponges Compared to Seawater Communities
A minimal core prokaryotic community (0.1%) was found in Antarctic sponges consisting of very few OTUs (n = 7) present in all four species but also in ambient seawater. The core prokaryotic community of sponges is rather small, pattern that has been documented from different biogeographic regions, including Antarctica (Schmitt et al., 2012; Easson and Thacker, 2014; Rodríguez-Marconi et al., 2015). We also defined a small variable community (0.7%) that consisted of 56 OTUs hosted in at least two of the sponge species analyzed with relative abundances above 0.1%. Nevertheless, 43% of these variable taxa had such low abundances (<0.001% on average) in three of the species that they could be considered as species-specific OTUs. The concept of “sponge or host specificity” needs to be revised as molecular techniques now allow deep sequencing of associated prokaryotic assemblages. A taxon we thought unique of a particular biotope (e.g., sponges, a particular host), we are able now to detect it in other habitats but at much lower numbers. Thus, the term “sponge- or host-enriched” has been introduced (Moitinho-Silva et al., 2014). In this regard, D. antarctica and M. acerata presented an enrichment of one of the core OTUs and S. antarcticus was enriched with other two core taxa representing a 45-fold change compared to seawater. The additional three sponge core OTUs were enriched in planktonic communities (Supplementary Table S2). The same enrichment pattern was found in 30% of the variable OTUs (Supplementary Table S2), which were categorized as rare (0.06% on average) in the surrounding seawater representing a 50-fold increase.
The fact that predominant OTUs were detected in all sponges from different geographic locations and also in the surrounding seawater suggests a global distribution of these microbes through the Antarctic environment. These OTUs might be adapted to their particular niches representing different ecotypes of the same microorganisms, so it is possible that they are horizontally transmitted through strong selective mechanisms (Schmitt et al., 2012; Turon et al., 2018). The majority of the sponge prokaryotic community (99%) belonged to the “rare biosphere” with mean relative abundances below 0.1%, and over 50% could be described as host-specific taxa. The specificity of host-microbe associations seems to extend beyond some dominant taxa from the variable community into the rare biosphere (Reveillaud et al., 2014).
Beyond the existence of certain plasticity of microbiomes within a particular host, the dominance of a gammaproteobacterial OTU (Betaproteobacteriales) in M. acerata was also recently reported from another location of the Antarctic Peninsula (Cárdenas et al., 2019). This spatial stability was also documented in the present study for dominant OTUs in D. antarctica and S. antarcticus across a geographic scale <700 km. These results are in agreement with a recent study in the Caribbean that found little variations in the sponge microbiome of Cliona delitrix over small geographic scales (<300 km), while a considerable geographic distance impact over a large regional scale (>1,000 km) was reported (Easson et al., 2020). To date, published data support the combination of host identity, geography, and environment as the main forces determining the structure of sponge microbiomes (Webster et al., 2010; Schmitt et al., 2012; Easson et al., 2020; Sacristán-Soriano et al., 2020b). In Antarctica in particular, the prokaryotic signature of sponges might be also related to a biogeographic partitioning of Southern Ocean microorganisms caused by the Polar Front, such as the deficit of Cyanobacteria in Antarctica (Wilkins et al., 2013), as evidenced in the present and previous studies (Rodríguez-Marconi et al., 2015; Cárdenas et al., 2018; Moreno-Pino et al., 2020). Since most collection efforts of sponges have so far explored tropical and temperate environments, this study contributes to expand our knowledge on sponge microbiome structure in polar waters.
Comparison of Antarctic Sponge Microbiomes With Sponge EMP Database
The fact that nearly 90% of the Antarctic sponge microbiomes had a blast hit with a sequence similarity over 97% against the sponge EMP collection would represent the ubiquity of the sponge-associated microbes through species and habitats.
Conclusion
Antarctic sponge-associated prokaryotic communities displayed less diversity than their surrounding seawater counterparts conferring them the status of LMA sponges. Their prokaryotic composition and structure with one or two dominant OTUs also resembled that from LMA sponges. Some abundant microbes have been related to the nitrification process and may play a central role in the nitrogen cycling in Antarctica. A global distribution of sponge-associated microbes has been documented, however, symbiont communities exhibit little uniformity in species composition or structure (Thomas et al., 2016). Antarctic sponges seem to follow the same pattern of symbiotic community organization. The core microbiomes are characterized by generalists microbes with little representation of specialists, a pattern previously described as “specific mix of generalists” (Erwin et al., 2012a). Different sponge species likely represent different ecological niches for prokaryotes, each with a specific microbial community that is vertically and horizontally acquired and selectively maintained (Webster et al., 2010; Sacristán-Soriano et al., 2019). Host identity seems to be the strongest driving force in determining the composition of sponge symbiont assemblages. However, associated prokaryotic communities could be slightly influenced by biogeography and environmental factors defining a prokaryotic signature for a particular habitat (Kennedy et al., 2014; Rodríguez-Marconi et al., 2015; Easson et al., 2020). In future studies, the use of metagenomics (Moreno-Pino et al., 2020) and metatranscriptomics will allow the recovery of functional genes and improve our understanding of the physiological roles of Antarctic sponge-associated microbiota.
Data Availability Statement
Raw Illumina sequence data were deposited in NCBI SRA under the accession number PRJNA548273.
Author Contributions
OS-S conceived the study and performed the analyses. OS-S and CA collected the samples. NP did the lab work. OS-S and CA wrote the manuscript and NP revised it. All authors contributed to the article and approved the submitted version.
Funding
This work was funded by grants from the Spanish Government: DISTANTCOM (CTM2013- 42667/ANT), BlueBio (CTM2016-78901/ANT), and PopCOmics project (CTM2017-88080; MCIU, AEI/FEDER, UE), as well as the European Union’s Horizon 2020 Research and Innovation Program under the Marie Skłodowska-Curie grant agreement 705464 (“SCOOBA”) to OS-S.
Conflict of Interest
The authors declare that the research was conducted in the absence of any commercial or financial relationships that could be construed as a potential conflict of interest.
Acknowledgments
We would like to thank BIO-Hesperides and BAE Gabriel de Castilla crews for their logistic support during the XXI Spanish Antarctic Survey. Thanks are also due to C. Leiva, P. Álvarez-Campos, G. Giribet, J. Junoy, B. Figuerola, J. Moles, C. Angulo-Preckler, and L. Cardona, for their help with sample collection. Special thanks to F. J. Cristobo for his valuable help in sample collection and taxonomic identifications (visual inspections and spicule preparations when necessary). This is an ANTECO (SCAR) contribution. This manuscript has been released as a pre-print at bioRxiv (Sacristán-Soriano et al., 2020a).
Supplementary Material
The Supplementary Material for this article can be found online at: https://www.frontiersin.org/articles/10.3389/fmars.2020.00474/full#supplementary-material
Footnotes
References
Angulo-Preckler, C., Cid, C., Oliva, F., and Avila, C. (2015). Antifouling activity in some benthic Antarctic invertebrates by in situ experiments at Deception Island. Antarctica. Mar. Environ. Res. 105, 30–38. doi: 10.1016/j.marenvres.2015.02.001
Angulo-Preckler, C., Figuerola, B., Núñez-Pons, L., Moles, J., Martín-Martín, R., Rull-Lluch, J., et al. (2018). Macrobenthic patterns at the shallow marine waters in the caldera of the active volcano of Deception Island. Antarctica. Cont. Shelf Res. 157, 20–31. doi: 10.1016/j.csr.2018.02.005
Apprill, A., McNally, S., Parsons, R., and Weber, L. (2015). Minor revision to V4 region SSU rRNA 806R gene primer greatly increases detection of SAR11 bacterioplankton. Aquat. Microb. Ecol. 75, 129–137. doi: 10.3354/ame01753
Bavestrello, G., Arillo, A., Calcinai, B., Cattaneo-Vietti, R., Cerrano, C., Gaino, E., et al. (2000). Parasitic diatoms inside antarctic sponges. Biol. Bull. 198, 29–33. doi: 10.2307/1542801
Bell, J. J. (2008). The functional roles of marine sponges. Estuar. Coast. Shelf Sci. 79, 341–353. doi: 10.1016/j.ecss.2008.05.002
Caporaso, J. G., Kuczynski, J., Stombaugh, J., Bittinger, K., Bushman, F. D., Costello, E. K., et al. (2010). QIIME allows analysis of high-throughput community sequencing data. Nat. Methods 7, 335–336. doi: 10.1038/nmeth.f.303
Cárdenas, C. A., Font, A., Steinert, G., Rondon, R., and González-Aravena, M. (2019). Temporal stability of bacterial communities in antarctic sponges. Front. Microbiol. 10:2699. doi: 10.3389/fmicb.2019.02699
Cárdenas, C. A., González-Aravena, M., Font, A., Hestetun, J. T., Hajdu, E., Trefault, N., et al. (2018). High similarity in the microbiota of cold-water sponges of the Genus Mycale from two different geographical areas. PeerJ 6:e4935. doi: 10.7717/peerj.4935
Cardoso, J., van Bleijswijk, J., Witte, H., and van Duyl, F. (2013). Diversity and abundance of ammonia-oxidizing Archaea and Bacteria in tropical and cold-water coral reef sponges. Aquat. Microb. Ecol. 68, 215–230. doi: 10.3354/ame01610
Cerrano, C., Arillo, A., Bavestrello, G., Calcinai, B., Cattaneo-Vietti, R., Penna, A., et al. (2000). Diatom invasion in the antarctic hexactinellid sponge Scolymastra joubini. Polar Biol. 23, 441–444. doi: 10.1007/s003000050466
Cerrano, C., Calcinai, B., Cucchiari, E., Camillo, C. Di, Nigro, M., Regoli, F., et al. (2004). Are diatoms a food source for Antarctic sponges? Chem. Ecol. 20, 57–64. doi: 10.1080/02757540310001629198
Cleary, D. F. R., Swierts, T., Coelho, F. J. R. C., Polónia, A. R. M., Huang, Y. M., Ferreira, M. R. S., et al. (2019). The sponge microbiome within the greater coral reef microbial metacommunity. Nat. Commun. 10:1644. doi: 10.1038/s41467-019-09537-8
Easson, C. G., Chaves-Fonnegra, A., Thacker, R. W., and Lopez, J. V. (2020). Host population genetics and biogeography structure the microbiome of the sponge Cliona delitrix. Ecol. Evol. 10:ece3.6033. doi: 10.1002/ece3.6033
Easson, C. G., and Thacker, R. W. (2014). Phylogenetic signal in the community structure of host-specific microbiomes of tropical marine sponges. Front. Microbiol. 5:532. doi: 10.3389/fmicb.2014.00532
Erwin, P. M., López-Legentil, S., González-Pech, R., and Turon, X. (2012a). A specific mix of generalists: bacterial symbionts in Mediterranean Ircinia spp. FEMS Microbiol. Ecol. 79, 619–637. doi: 10.1111/j.1574-6941.2011.01243.x
Erwin, P. M., Pita, L., López-Legentil, S., and Turon, X. (2012b). Stability of sponge-associated bacteria over large seasonal shifts in temperature and irradiance. Appl. Environ. Microbiol. 78, 7358–7368. doi: 10.1128/AEM.02035-12
Glasl, B., Smith, C. E., Bourne, D. G., and Webster, N. S. (2018). Exploring the diversity-stability paradigm using sponge microbial communities. Sci. Rep. 8:8425. doi: 10.1038/s41598-018-26641-9
Henríquez, M., Vergara, K., Norambuena, J., Beiza, A., Maza, F., Ubilla, P., et al. (2014). Diversity of cultivable fungi associated with Antarctic marine sponges and screening for their antimicrobial, antitumoral and antioxidant potential. World J. Microbiol. Biotechnol. 30, 65–76. doi: 10.1007/s11274-013-1418-x
Hentschel, U., Hopke, J., Horn, M., Anja, B., Wagner, M., Hacker, J., et al. (2002). Molecular evidence for a uniform microbial community in sponges from different oceans. Appl. Environ. Microbiol. 68, 4431–4440. doi: 10.1128/AEM.68.9.4431
Hill, M. S., and Sacristán-Soriano, O. (2017). “Molecular and functional ecology of sponges and their microbial symbionts,” in Climate Change, Ocean Acidification and Sponges, eds J. L. Carballo and J. J. Bell (Cham: Springer International Publishing), 105–142. doi: 10.1007/978-3-319-59008-0_5
Hooper, J. N. A., and Van Soest, R. W. M. (2004). Book review: systema porifera. guide to the classification of sponges. Invertebr. Syst. 18, 233–234. doi: 10.1007/978-1-4615-0747-5_1
Jackson, S. A., Flemer, B., McCann, A., Kennedy, J., Morrissey, J. P., O’Gara, F., et al. (2013). Archaea appear to dominate the microbiome of Inflatella pellicula deep sea sponges. PLoS One 8:e0084438. doi: 10.1371/journal.pone.0084438
Kennedy, J., Flemer, B., Jackson, S. A., Morrissey, J. P., O’Gara, F., and Dobson, A. D. W. (2014). Evidence of a putative deep sea specific microbiome in marine sponges. PLoS One 9:e0091092. doi: 10.1371/journal.pone.0091092
Lee, O. O., Wang, Y., Yang, J., Lafi, F. F., Al-Suwailem, A., and Qian, P.-Y. (2011). Pyrosequencing reveals highly diverse and species-specific microbial communities in sponges from the Red Sea. ISME J. 5, 650–664. doi: 10.1038/ismej.2010.165
Lo Giudice, A., Azzaro, M., and Schiaparelli, S. (2019). “Microbial symbionts of antarctic marine benthic invertebrates,” in The Ecological Role of Micro-organisms in the Antarctic Environment, Ed. S. Castro-Sowinski (Berlin: Springer), 277–296. doi: 10.1007/978-3-030-02786-5_13
McClintock, J. B., Amsler, C. D., Baker, B. J., and van Soest, R. W. M. (2005). Ecology of Antarctic marine sponges: an overview. Integr. Comp. Biol. 45, 359–368. doi: 10.1093/icb/45.2.359
Moitinho-Silva, L., Bayer, K., Cannistraci, C. V., Giles, E. C., Ryu, T., Seridi, L., et al. (2014). Specificity and transcriptional activity of microbiota associated with low and high microbial abundance sponges from the Red Sea. Mol. Ecol. 23, 1348–1363. doi: 10.1111/mec.12365
Moitinho-Silva, L., Nielsen, S., Amir, A., Gonzalez, A., Ackermann, G. L., Cerrano, C., et al. (2017a). The sponge microbiome project. Gigascience 6, 1–7. doi: 10.1093/gigascience/gix077
Moitinho-Silva, L., Steinert, G., Nielsen, S., Hardoim, C. C. P., Wu, Y. C., McCormack, G. P., et al. (2017b). Predicting the HMA-LMA status in marine sponges by machine learning. Front. Microbiol. 8:752. doi: 10.3389/fmicb.2017.00752
Moreno-Pino, M., Cristi, A., Gillooly, J. F., and Trefault, N. (2020). Characterizing the microbiomes of Antarctic sponges: a functional metagenomic approach. Sci. Rep. 10:645. doi: 10.1038/s41598-020-57464-2
Parada, A. E., Needham, D. M., and Fuhrman, J. A. (2016). Every base matters: assessing small subunit rRNA primers for marine microbiomes with mock communities, time series and global field samples. Environ. Microbiol. 18, 1403–1414. doi: 10.1111/1462-2920.13023
Pita, L., Erwin, P. M., Turon, X., and López-Legentil, S. (2013). Till death do us part: stable sponge-bacteria associations under thermal and food shortage stresses. PLoS One 8:e0080307. doi: 10.1371/journal.pone.0080307
Pita, L., Rix, L., Slaby, B. M., Franke, A., and Hentschel, U. (2018). The sponge holobiont in a changing ocean: from microbes to ecosystems. Microbiome 6:46. doi: 10.1186/s40168-018-0428-1
Polónia, A. R. M., Cleary, D. F. R., Duarte, L. N., de Voogd, N. J., and Gomes, N. C. M. (2014). Composition of archaea in seawater, sediment, and sponges in the Kepulauan seribu reef system, indonesia. Microb. Ecol. 67, 553–567. doi: 10.1007/s00248-013-0365-2
Polónia, A. R. M., Cleary, D. F. R., Freitas, R., de Voogd, N. J., and Gomes, N. C. M. (2015). The putative functional ecology and distribution of archaeal communities in sponges, sediment and seawater in a coral reef environment. Mol. Ecol. 24, 409–423. doi: 10.1111/mec.13024
Radax, R., Hoffmann, F., Rapp, H. T., Leininger, S., and Schleper, C. (2012). Ammonia-oxidizing archaea as main drivers of nitrification in cold-water sponges. Environ. Microbiol. 14, 909–923. doi: 10.1111/j.1462-2920.2011.02661.x
Reveillaud, J., Maignien, L., Eren, M. A., Huber, J. A., Apprill, A., Sogin, M. L., et al. (2014). Host-specificity among abundant and rare taxa in the sponge microbiome. ISME J. 8, 1198–1209. doi: 10.1038/ismej.2013.227
Rodríguez-Marconi, S., De la Iglesia, R., Díez, B., Fonseca, C. A., Hajdu, E., and Trefault, N. (2015). Characterization of Bacterial, Archaeal and Eukaryote symbionts from antarctic sponges reveals a high diversity at a three-domain level and a particular signature for this ecosystem. PLoS One 10:e0138837. doi: 10.1371/journal.pone.0138837
Sacristán-Soriano, O., Pérez Criado, N., and Avila, C. (2020a). Host species determine symbiotic community composition in Antarctic sponges (Porifera: Demospongiae). bioRxiv [Preprint] doi: 10.1101/2020.03.09.983221
Sacristán-Soriano, O., Turon, X., and Hill, M. (2020b). Microbiome structure of ecologically important bioeroding sponges (family Clionaidae): the role of host phylogeny and environmental plasticity. Coral Reefs doi: 10.1007/s00338-020-01962-2
Sacristán-Soriano, O., Winkler, M., Erwin, P., Weisz, J., Harriott, O., Heussler, G., et al. (2019). Ontogeny of symbiont community structure in two carotenoid-rich, viviparous marine sponges: comparison of microbiomes and analysis of culturable pigmented heterotrophic bacteria. Environ. Microbiol. Rep. 11, 249–261. doi: 10.1111/1758-2229.12739
Schloss, P. D., Westcott, S. L., Ryabin, T., Hall, J. R., Hartmann, M., Hollister, E. B., et al. (2009). Introducing mothur: open-source, platform-independent, community-supported software for describing and comparing microbial communities. Appl. Environ. Microbiol. 75, 7537–7541. doi: 10.1128/AEM.01541-09
Schmitt, S., Deines, P., Behnam, F., Wagner, M., and Taylor, M. W. (2011). Chloroflexi bacteria are more diverse, abundant, and similar in high than in low microbial abundance sponges. FEMS Microbiol. Ecol. 78, 497–510. doi: 10.1111/j.1574-6941.2011.01179.x
Schmitt, S., Tsai, P., Bell, J., Fromont, J., Ilan, M., Lindquist, N., et al. (2012). Assessing the complex sponge microbiota: core, variable and species-specific bacterial communities in marine sponges. ISME J. 6, 564–576. doi: 10.1038/ismej.2011.116
Simister, R. L., Deines, P., Botté, E. S., Webster, N. S., and Taylor, M. W. (2012). Sponge-specific clusters revisited: a comprehensive phylogeny of sponge-associated microorganisms. Environ. Microbiol. 14, 517–524. doi: 10.1111/j.1462-2920.2011.02664.x
Steinert, G., Wemheuer, B., Janussen, D., Erpenbeck, D., Daniel, R., Simon, M., et al. (2019). Prokaryotic diversity and community patterns in antarctic continental shelf sponges. Front. Mar. Sci. 6:297. doi: 10.3389/fmars.2019.00297
Taylor, M. W., Radax, R., Steger, D., and Wagner, M. (2007). Sponge-associated microorganisms: evolution, ecology, and biotechnological potential. Microbiol. Mol. Biol. Rev. 71, 295–347. doi: 10.1128/MMBR.00040-06
Thomas, T., Moitinho-Silva, L., Lurgi, M., Björk, J. R., Easson, C., Astudillo-García, C., et al. (2016). Diversity, structure and convergent evolution of the global sponge microbiome. Nat. Commun. 7:11870. doi: 10.1038/ncomms11870
Turon, M., Cáliz, J., Garate, L., Casamayor, E. O., and Uriz, M. J. (2018). Showcasing the role of seawater in bacteria recruitment and microbiome stability in sponges. Sci. Rep. 8:15201. doi: 10.1038/s41598-018-33545-1
Turon, M., and Uriz, M. J. (2020). New Insights Into the Archaeal Consortium of Tropical Sponges. Front. Mar. Sci. 6:789. doi: 10.3389/fmars.2019.00789
Webster, N. S., Negri, A. P., Munro, M. M. H. G., and Battershill, C. N. (2004). Diverse microbial communities inhabit Antarctic sponges. Environ. Microbiol. 6, 288–300. doi: 10.1111/j.1462-2920.2004.00570.x
Webster, N. S., and Taylor, M. W. (2012). Marine sponges and their microbial symbionts: love and other relationships. Environ. Microbiol. 14, 335–346. doi: 10.1111/j.1462-2920.2011.02460.x
Webster, N. S., Taylor, M. W., Behnam, F., Lücker, S., Rattei, T., Whalan, S., et al. (2010). Deep sequencing reveals exceptional diversity and modes of transmission for bacterial sponge symbionts. Environ. Microbiol. 12, 2070–2082. doi: 10.1111/j.1462-2920.2009.02065.x
Keywords: Antarctic Peninsula, South Shetland Islands, sponge holobiont, archaea, benthic ecology
Citation: Sacristán-Soriano O, Pérez Criado N and Avila C (2020) Host Species Determines Symbiotic Community Composition in Antarctic Sponges (Porifera: Demospongiae). Front. Mar. Sci. 7:474. doi: 10.3389/fmars.2020.00474
Received: 17 March 2020; Accepted: 27 May 2020;
Published: 19 June 2020.
Edited by:
Zhiyong Li, Shanghai Jiao Tong University, ChinaReviewed by:
Cristiane Cassiolato Pires Hardoim, São Paulo State University, BrazilGeorg Steinert, Retired, Oldenburg, Germany
Copyright © 2020 Sacristán-Soriano, Pérez Criado and Avila. This is an open-access article distributed under the terms of the Creative Commons Attribution License (CC BY). The use, distribution or reproduction in other forums is permitted, provided the original author(s) and the copyright owner(s) are credited and that the original publication in this journal is cited, in accordance with accepted academic practice. No use, distribution or reproduction is permitted which does not comply with these terms.
*Correspondence: Oriol Sacristán-Soriano, b3NhY3Jpc283QGdtYWlsLmNvbQ==